Development of a flow evolution network model for predicting the viscoplastic behavior of poly(L-lactide)
-
1
FDA/CDRH, Division of Applied Mechanics/ Office of Science and Engineering Laboratories, United States
-
2
Veryst Engineering, United States
Introduction: Absorbable polymers are increasingly being used for medical implants that perform a structural role[1]. Computational modeling is critical to device development in order to predict the stress distribution under typical loading conditions. This is especially true for absorbable cardiovascular devices, such as stents, which experience significant plastic deformation when crimped onto a delivery catheter and are cyclically loaded in vivo. However, computational modeling of absorbable devices is significantly hampered by a lack of appropriate constitutive models that capture their viscoelasticity and post-yield behavior. Here, we developed a constitutive model that incorporated viscoplasticity for a common medical absorbable polymer.
Materials and Methods: Candidate constitutive models were selected based on the experimental response at 37°C observed during multiple tensile test protocols performed on microtensile bars (thickness=0.5mm, width = 1.42mm, length = 3.8mm) fabricated from poly(L-lactide), i.e., PLLA. The dimensions were chosen in order to be relevant for transcatheter cardiovascular devices. Before testing, all specimens were airbrushed to create a speckled pattern and enable local strain measurements through digital image correlation[2]. The protocols encompassed monotonic ramp (strain rate=0.5%/min, 5%/min, 10%/min), stress relaxation, and “yield point” tests consisting of load/ un-load cycles below and above the yield. Yield point tests provide information on the unloading behavior of the polymer prior to and after yield. The experimental stress-strain data were curve-fit to two candidate constitutive relationships, namely, the Three Network Model (TNM) and a Flow Evolution Network Model (FEN)[3], followed by refinement through finite element simulations of tensile experiments. Both the TNM and the FEN models are capable of capturing large strain viscoplastic behavior, but the FEN model has the advantage of capturing strong softening post-yield.
Results and Discussion: Monotonic testing demonstrated that PLLA exhibits rate dependent stress-strain behavior with a significant post-yield region (Fig 1). The peak stress increased with increasing strain rate (47MPa vs 62MPa) while the strain at failure decreased (0.75 vs 0.3). PLLA exhibited significant relaxation prior to and post yield with stress decreases of ~75% and 80% over 30 min, respectively (Fig 2). Yield point tests demonstrate that little hysteresis is generated in linear region. Together, these results suggest that PLLA exhibits complex viscoplasticity that influences the stress level and should be accounted for in computational models of PLLA devices. The FEN model was able to capture these relevant behaviors more accurately than the TNM (NMAD fitness_TNM=9.4, NMAD fitness_FEN=3.5).
Conclusion: The FEN constitutive model was able to accurately capture the strain rate dependent loading and unloading behavior which suggests its validity for modeling mechanics of PLLA.
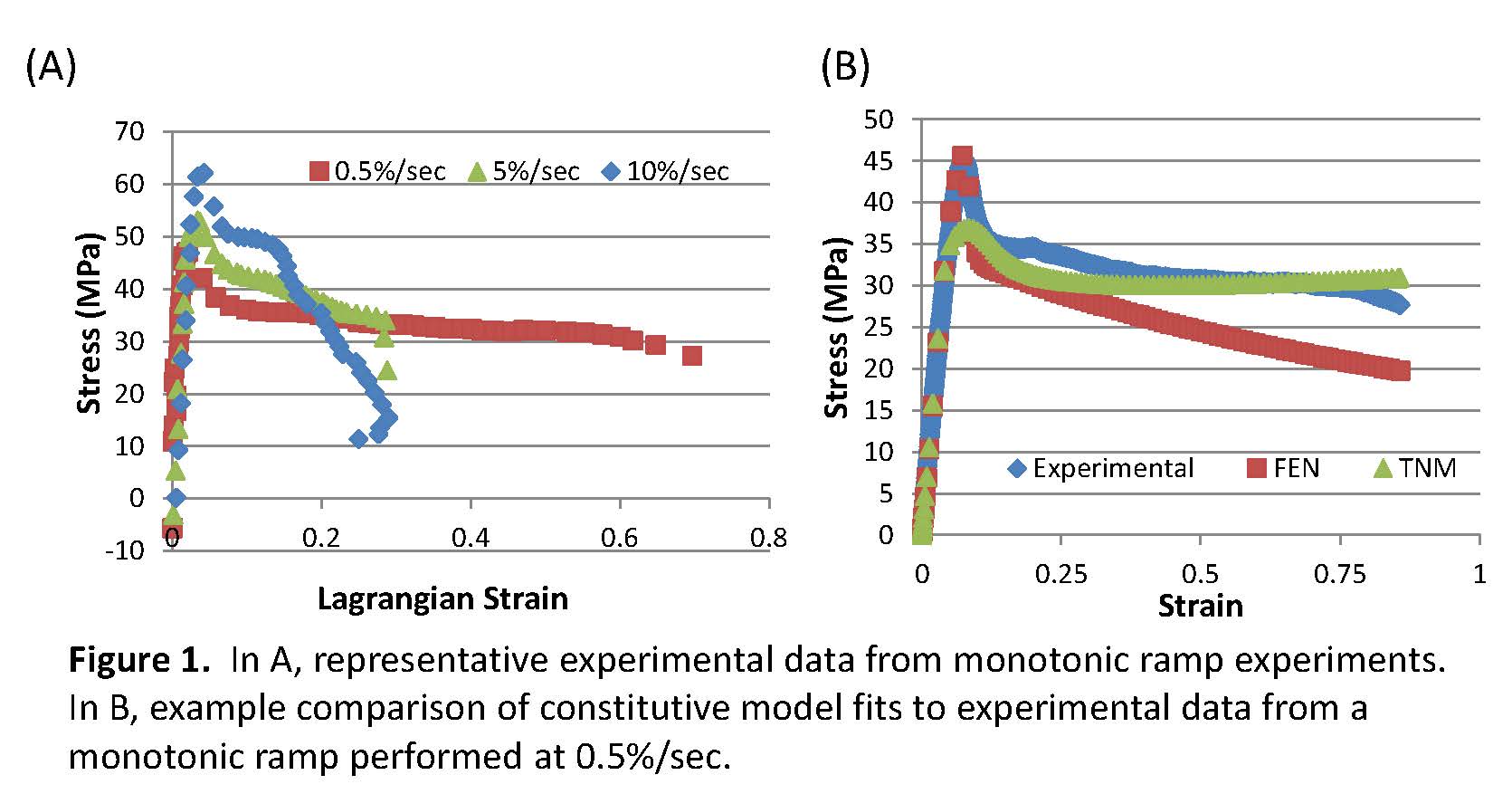
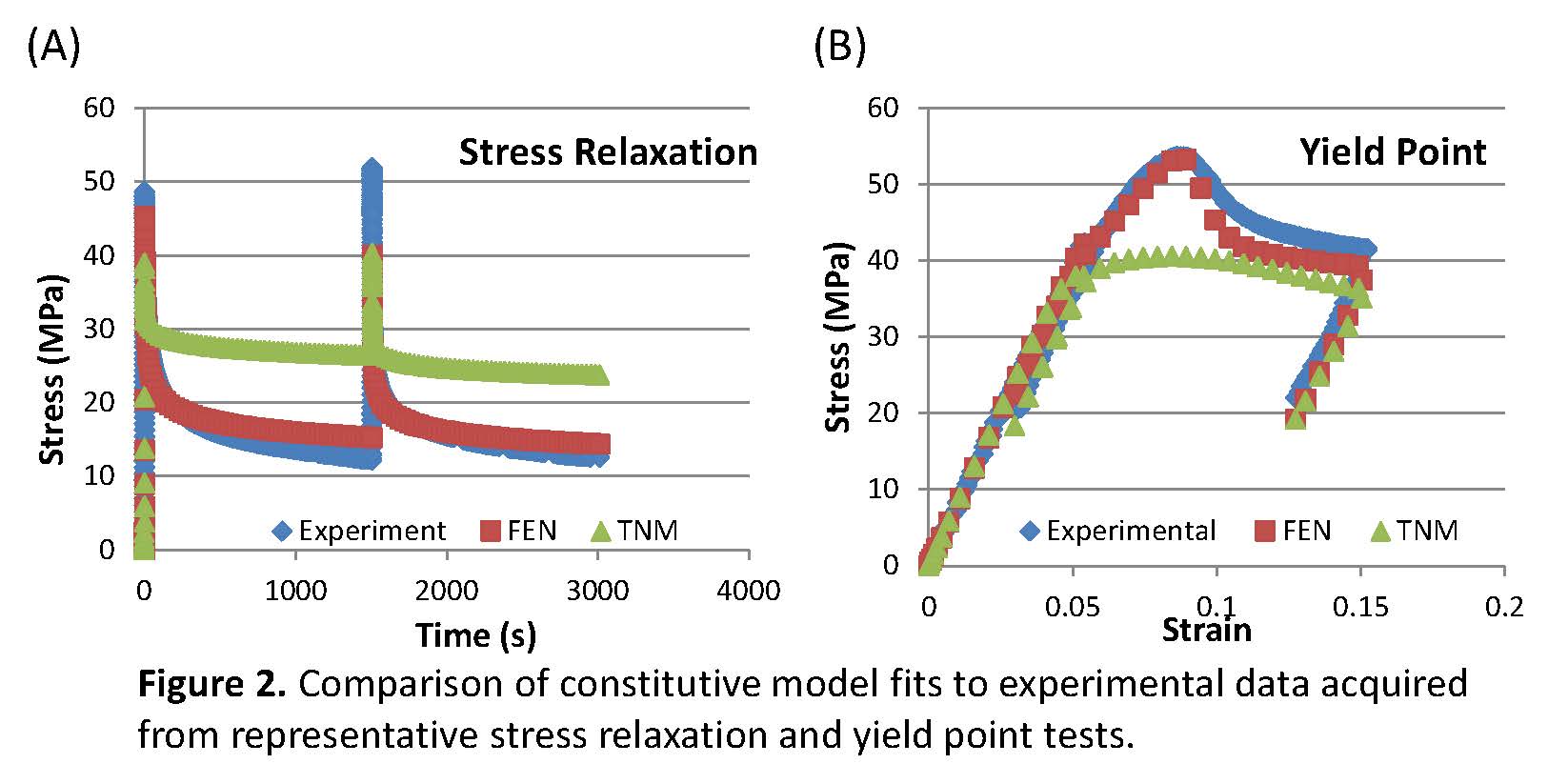
This study was funded by the CDRH Critical Path program; We gratefully acknowledge the insights and support David Quinn, PhD
References:
[1] Serruys et al., 2012, European Heart Journal, 33: 16-25.
[2] Gilchrist et al., 2004, IEEE Trans Med Imaging, 23:546-553
[3] PolyUMed Software Library, Veryst Engineering, Needham, MA
Keywords:
modeling,
biomaterial,
polymer,
mechanical property
Conference:
10th World Biomaterials Congress, Montréal, Canada, 17 May - 22 May, 2016.
Presentation Type:
General Session Oral
Topic:
Mechanical properties of biomaterials
Citation:
Dreher
M,
Nagaraja
S,
Bergstrom
J and
Hayman
D
(2016). Development of a flow evolution network model for predicting the viscoplastic behavior of poly(L-lactide).
Front. Bioeng. Biotechnol.
Conference Abstract:
10th World Biomaterials Congress.
doi: 10.3389/conf.FBIOE.2016.01.01979
Copyright:
The abstracts in this collection have not been subject to any Frontiers peer review or checks, and are not endorsed by Frontiers.
They are made available through the Frontiers publishing platform as a service to conference organizers and presenters.
The copyright in the individual abstracts is owned by the author of each abstract or his/her employer unless otherwise stated.
Each abstract, as well as the collection of abstracts, are published under a Creative Commons CC-BY 4.0 (attribution) licence (https://creativecommons.org/licenses/by/4.0/) and may thus be reproduced, translated, adapted and be the subject of derivative works provided the authors and Frontiers are attributed.
For Frontiers’ terms and conditions please see https://www.frontiersin.org/legal/terms-and-conditions.
Received:
27 Mar 2016;
Published Online:
30 Mar 2016.
*
Correspondence:
Dr. Maureen Dreher, FDA/CDRH, Division of Applied Mechanics/ Office of Science and Engineering Laboratories, Silver Spring, MD, United States, Email1
Dr. Srinidhi Nagaraja, FDA/CDRH, Division of Applied Mechanics/ Office of Science and Engineering Laboratories, Silver Spring, MD, United States, srinidhi.nagaraja@fda.hhs.gov
Dr. Jorgen Bergstrom, Veryst Engineering, Needham, MA, United States, jbergstrom@veryst.com
Dr. Danika Hayman, Veryst Engineering, Needham, MA, United States, dhayman@veryst.com