Age-related psychophysiological vulnerability to phenylalanine in phenylketonuria
- 1Department of Paediatrics, Child Neurology and Psychiatry, Sapienza Università di Roma, Rome, Italy
- 2Department of Neurology and Psychiatry, Sapienza Università di Roma, Rome, Italy
- 3Department of Experimental Medicine, Sapienza Università di Roma, Rome, Italy
- 4Department of Molecular Medicine, Sapienza Università di Roma, Rome, Italy
- 5Centre for Epidemiology, Surveillance and Health Promotion (CNESPS), Istituto Superiore di Sanità, Rome, Italy
Background: Phenylketonuria (PKU) is caused by the inherited defect of the phenylalanine hydroxylase enzyme, which converts phenylalanine (Phe) into tyrosine (Tyr). Neonatal screening programs and early treatment have radically changed the natural history of PKU. Nevertheless, an increased risk of neurocognitive and psychiatric problems in adulthood remains a challenging aspect of the disease. In order to assess the vulnerability of complex skills to Phe, we explored: (a) the effect of a rapid increase in blood Phe levels on event-related potentials (ERP) in PKU subjects during their second decade of life; (b) the association (if existing) between psychophysiological and neurocognitive features.
Methods: Seventeen early-treated PKU subjects, aged 10–20, underwent ERP [mismatch negativity, auditory P300, contingent negative variation (CNV), and Intensity Dependence of Auditory Evoked Potentials] recording before and 2 h after an oral loading of Phe. Neurocognitive functioning, historical and concurrent biochemical values of blood Phe, Tyr, and Phe/Tyr ratio, were all included in the statistical analysis.
Results: Event-related potential components were normally detected in all the subjects. In subjects younger than 13 CNV amplitude, W2-CNV area, P3b latency, and reaction times in motor responses were negatively influenced by Phe-loading. Independently from the psychophysiological vulnerability, some neurocognitive skills were more impaired in younger patients. No correlation was found between biochemical alterations and neurocognitive and psychophysiological findings.
Conclusion: The vulnerability of the emerging neurocognitive functions to Phe suggests a strict metabolic control in adolescents affected by PKU and a neurodevelopmental approach in the study of neurocognitive outcome in PKU.
Introduction
Phenylketonuria (PKU; OMIM#261600) is an inherited metabolic cause of mental disability due to the defect of the phenylalanine hydroxylase (PAH) enzyme that converts phenylalanine (Phe) into tyrosine (Tyr). Neonatal screening programs and early treatment have radically changed the natural history of PKU (1). Despite the favorable clinical outcome of early-treated PKU subjects, when compared with late- or untreated patients, an intelligence quotient (IQ) lower than expected (2) and minor neuropsychological (3) and psychiatric (4) problems remain challenging aspects of the disease. The pathogenesis of these alterations is unknown. A disorder of central dopaminergic and serotoninergic connectivity has been suggested (5). In general terms, the risk of failure in attaining the expected level of mental development in early-treated PKU patients is related the quality of the dietary control during the first years of life (6, 7). A specific vulnerability of nervous structures to Phenylalanine (Phe) in their critical period of development has been suggested by neuropathological (8) and neurophysiological studies (9). If such concept may be extended to the latest stages of high cortical function development, during adolescence and early adulthood, it is just a matter of conjectures. This is an important issue, considered the decline of dietary compliance after the first decade of life with several patients failing to comply with or abandoning the diet (10). Presently, there are no clinical or instrumental tools capable to establishing the potential age- and/or individual-related risk of neurocognitive impairment in case of interruption of the diet therapy during adolescence and young adulthood.
Within this frame, the aims of the present study were: (a) exploring the effect of rapid variations in blood Phe on psychophysiological components involved in attention and information processing in early-treated young PKU subjects during their second decade of life; (b) assessing the association (if existing) between psychophysiological vulnerability, biochemical control and neurocognitive functions.
Materials and Methods
The experimental design scheduled two subsequent steps. The neurocognitive assessment was performed at Examination 1. Examination 2, 2 or 3 weeks later, was dedicated to the psychophysiological study (see below), which was performed before (T1) and 2 h after (T2) an oral loading of Phe (100 mg/kg). The time span between T1 and T2 recordings was based on H-MRS studies, which showed a pick of brain Phe concentration 1–4 h after an oral loading of Phe (11, 12). As the loading procedure was concerned, all patients were instructed to continue their usual dietary regime until the evening before the day of the test, which was performed in the morning after an overnight fast (T1 recording). Afterward, an orange juice drink with purified Phe was administered, and 2 h later the T2 recording was started. No other meal was allowed until the accomplishment of this second phase of the test.
Phe, Tyr levels, and Phe/Tyr ratio were assessed on dry blood spot on the day of neuropsychological assessment, and before T1 and T2 examinations.
The study was approved by the medical ethical committee and a written informed consent was obtained from patients or patients’ parents (for minors).
Subjects
Seventeen subjects were sampled among the PKU patients in charge of the Department of Paediatrics and Child Neurology and Psychiatry in Rome, according to the following criteria: (a) genetically confirmed defect of PAH; (b) early diagnosis, by neonatal screening program, and treatment; (c) aged 10–20; (d) IQ ≥80; (e) availability of biochemical data covering the patient’s history from the diagnosis up to the study; (f) absence of medical condition, other than PKU, affecting neuropsychological and/or psychophysiological functions; (g) no history or clinical evidence of consumption of drugs or medicaments interfering with neurocognitive and/or psychophysiological functions; and (h) no pregnancy.
Demographic and clinical characteristics of the sample are shown in Table 1.
Neurocognitive Assessment
The Neurocognitive assessment was focused on the following functions: (a) IQ [Wechsler intelligence scale for children-III (WISC-III) for patients aged 6–16 and Wechsler adult intelligence scale-revised (WAIS-R) for patients ≥17 years]; (b) cognitive flexibility and rule shifting [Wisconsin card sorting test (WCST)]. The following domains of WCST were measured: perseverative responses (PR), perseverative errors (PE), non-perseverative errors (NE), and total number of errors (E); (c) visuospatial and visual memory functions (Rey–Osterrieth Complex Figure Test). To the aim of the present study the accuracy scores for the copying and immediate recall were analyzed; (d) sustained and shared attention, memory functions (Leiter-R test attention and memory battery). These Leiter subtests contribute to five composite scores: memory screener, recognition memory, memory span, attention composite, and memory process.
Biochemical Studies
Phe, Tyr, and Phe/Tyr ratio were analyzed on dry blood spot by tandem mass spectrometry at each time scheduled by the protocol.
Event-Related Potentials
Psychophysiological study included the following event-related potentials (ERPs): mismatch negativity (MMN); auditory P300 (oddball and with a discriminant motor response); contingent negative variation (CNV); and intensity dependence of auditory evoked potentials (IDAP). The brain functions potentially probed by the ERPs and the related behavioral tasks are reported in Table 2 (13–25).
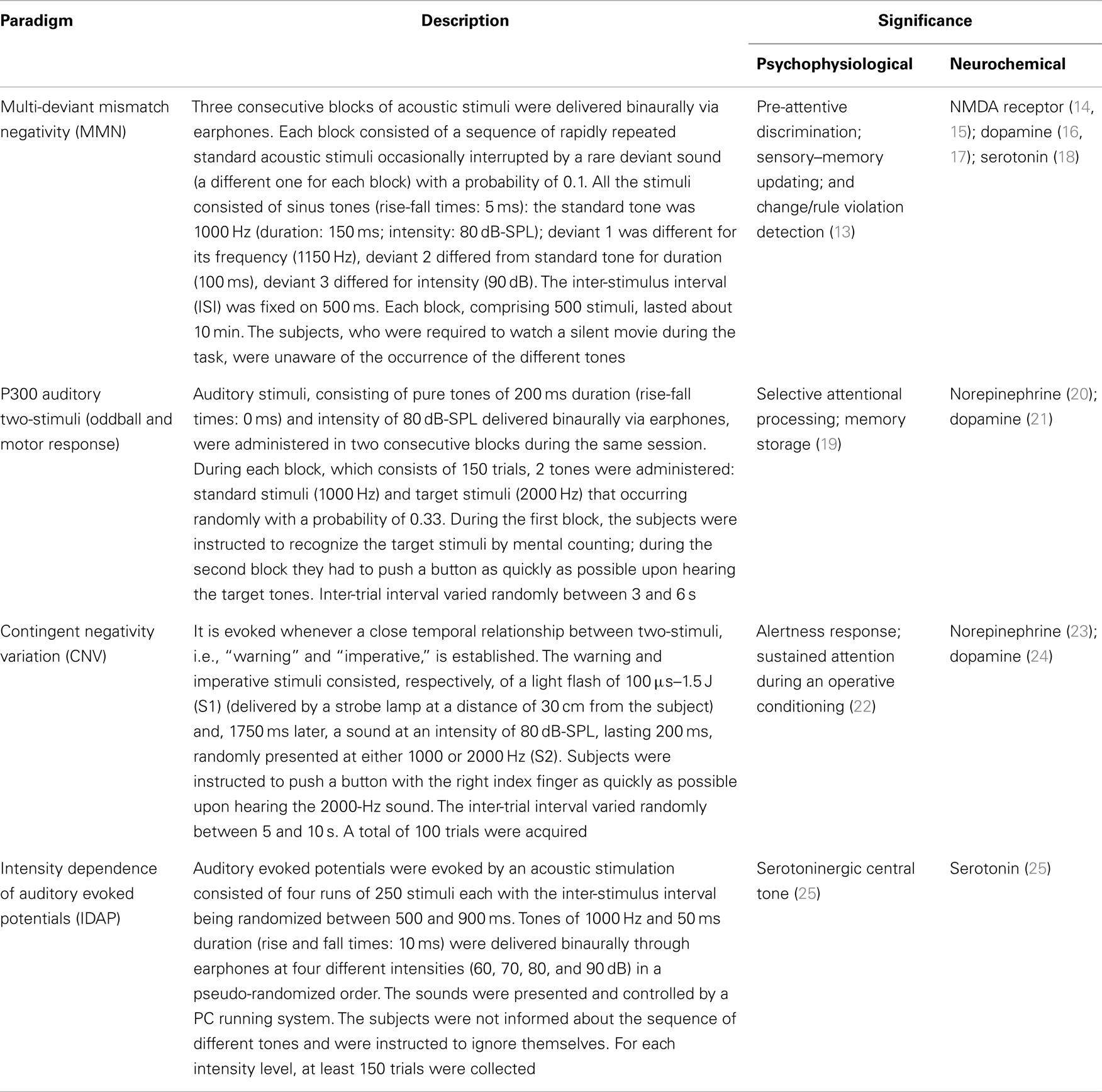
Table 2. Event-related potentials paradigm: main psychophysiological and neurochemical significance.
EEG Recording
Participants were seated on an anatomic chair in a faradized and light-attenuated room. The electrophysiological signals were recorded by Ag/AgCl electrodes fixed on the scalp at the F3, Fz, F4, C3, Cz, C4, P3, Pz, and P4 sites, according to the International 10–20 System, referred to linked mastoids and grounded at Fpz. The bipolar electrooculogram (EOG) was recorded from above and below the left eye. All inter-electrode impedances were kept below 3 KΩ. EEG signals and EOG were filtered using a 0.01–30 Hz bandpass (a narrow bandpass at 2–10 Hz was applied for MMN analysis). A notch filter was also applied. The data were entered via an analog/digital converter at a sampling rate of 1024 Hz and stored in a hard disk. A Mizar Sirius EEG-EP multifunctional system was used.
ERP Analysis
All auditory signals were visually inspected and revised by one investigator (F.F.) blinded to the patient’s age and clinical data.
Trials containing eye movements were automatically rejected. A further selection was performed in the offline analysis in order to reject other kinds of artifacts, according to the clinical guidelines (26).
With regard to MMN, ERPs were obtained by averaging EEG epochs separately for all deviant and standard tones. Difference waveforms were calculated by subtracting the ERPs of standard stimuli from those elicited by each deviant stimulus. The MMN latency and amplitude for each deviant stimulus were measured from the most negative peak detected between 100 and 250 ms post the onset of the stimulus difference, at Fz, on the difference-wave.
With regard to the P3 paradigm, in the target response, the N1 component was identified as the most negative peak between 75 and 140 ms, while the P3b components were defined as the largest positive deflections between 250 and 500 ms on the stimulus onset. The amplitude of these components was identified by means of baseline-to-peak measurements. For the sessions, counting responses and mean reaction times (RTs) of correct responses were calculated (range for correct responses 100–1000 ms).
The CNV amplitude was measured as the total area (negative shift between S1 and S2) and as two temporal windows of interest: the early orienting window – W1 (between 500 and 700 ms after S1), and the late window – W2 (200 ms preceding S2) (27). The RT was evaluated as the correct motor response at S2.
With regard to IDAP, the epoch analysis for each AEP was of 600 ms with a 100 ms pre-stimulus baseline. Amplitudes of the N1 (between 50 and 150 ms post-stimulus) and P2 (between 90 and 230 ms post-stimulus) peaks were measured. The IDAP was calculated as the linear amplitude/stimulus intensity function (ASF) slope for block averages (mV/10 dB).
All ERP recorded at T1 and T2 were compared with those obtained in healthy age matched internal controls in our lab. Moreover, as the T2 recording was concerned, each subject acted as control of himself.
Statistical Analysis
The statistical analyses have been performed using t-test for continuous variables (unpaired and paired samples) and an exact Fisher test for categorical variables. A correlation analysis between demographic, clinical, biochemical, and neuropsychological and neurophysiological variables was also performed by means of Pearson’s index.
In order to explore the possible influence of the age on neurocognitive and psychophysiological performances, the sample was sub-grouped in tertiles according to the patients’ age and each subgroup was compared with each other with respect to neuropsychological and neurocognitive performances. All data were analyzed with SPSS (version 21.0). A p value ≤0.05 was considered to indicate statistical significance.
Results
Neurocognitive Assessment
Neurocognitive assessment was accomplished in all subjects (Table 3). The mean concurrent value of Phe value in blood was 697.13 μM (±279.36). In the whole sample IQ ranged 80–124 (mean 95.06; SD 15). Among the tests exploring EFs, the scores at WSCT in six subjects were under reference value of one SD in one or more domains. With regard to attention and memory, 11 out of 17 patients obtained a score lower than normal at Leiter-R battery for attention and memory. On Rey–Osterrieth Complex Figure (Copy) Test (RCFC) and Rey–Osterrieth Complex Figure (Memory) RCFM Test the mean score of PKU patients was 35.58 (SD 21.11) and 23.82 (SD 19.96) percentile of the reference population and 10 and 11 subjects out of 17, respectively, performed ≤25th percentile.
Intelligence quotient was correlated with: WCST PR (r = 0.595; p = 0.012), WCST E (r = 0.687; p = 0.002), WCST PE (r = 0.658; p = 0.004), and WCST NE (r = 0.480; p = 0.051). IQ and EFs were not significantly different in subjects on and out diet when the study was performed and neither of them was correlated with subjects’ age, and historical and/or concurrent biochemical alterations.
Psychophysiological Assessment
Mean blood Phe concentration at T1 and T2 was 571.64 μM (±192.92) and 1129 μM (±177.31), respectively (p < 0.001).
Normal IDAP values and MMN waves morphology were obtained from all the participants, either in the basal condition or post Phe-loading (Figure 1; Table 4). No variations of amplitude and/or latency of MMN could be detected after the Phe-loading. No correlations were found between IDAP or MMN parameters and clinical, biochemical, and neuropsychological data.
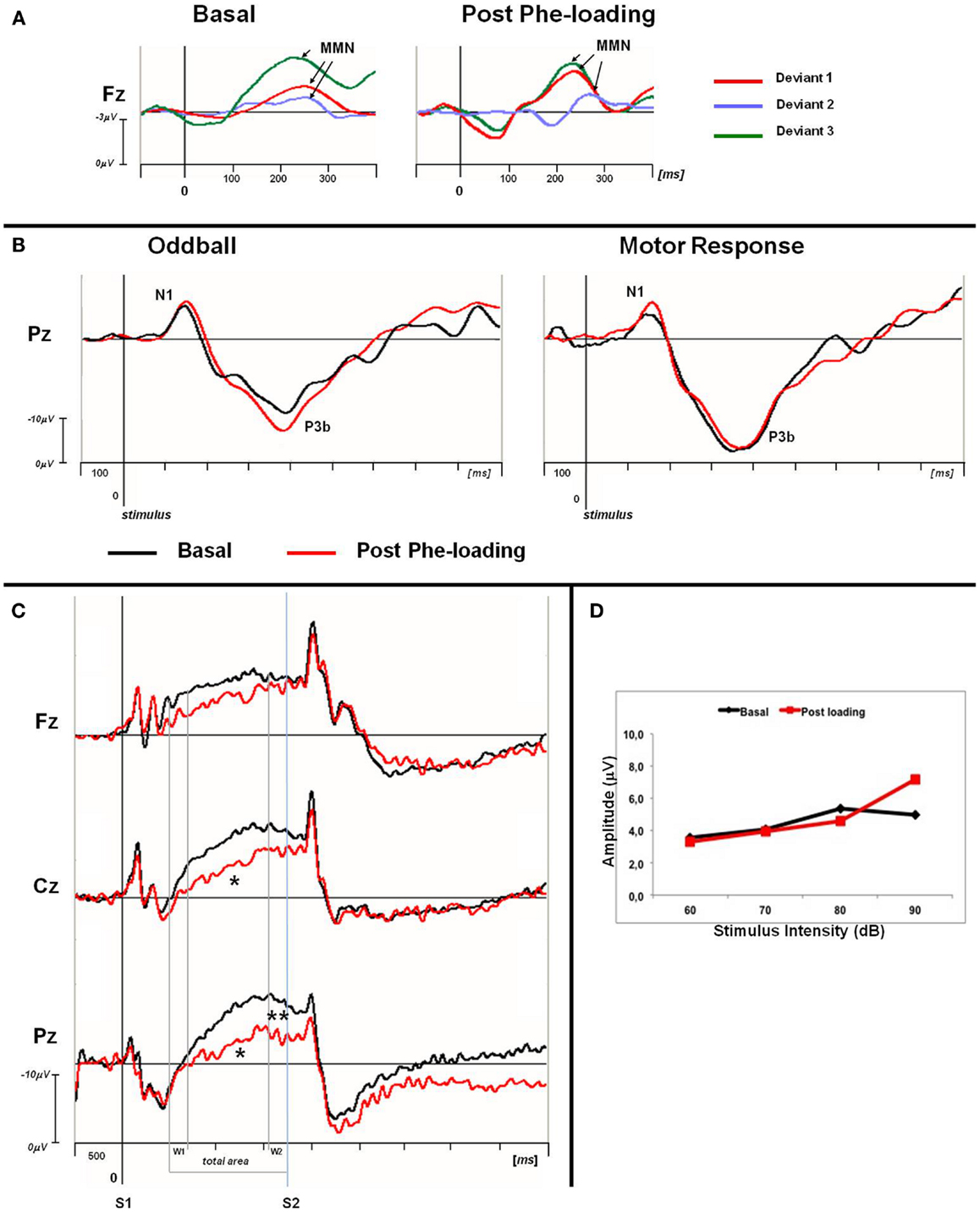
Figure 1. (A) Grand averaged MMN traces at Fz for basal and post Phe-loading condition. Difference waves are showed for each deviant stimulus (deviant 1: red line; deviant 2: blue violet line; deviant 3: green line). The analysis epoch for the MMN is 500 ms with a 100 ms pre-stimulus baseline. The arrows indicate the MMN peak for each difference waves. (B) Grand averaged P3 traces for oddball and motor response task at Pz site. For each task the basal (black) and post-loading trace (red) are superimposed. The epoch analysis of the P3 components is 1 s with a 100 ms pre-stimulus baseline. (C) Grand averaged CNV waveforms, with W1, W2, and total areas highlighted, superimposed at midline scalp sites for basal (black lines) and post-loading (red lines) evaluation. The epoch analysis is 5 s, with a 500 ms pre-stimulus baseline before S1. S1: warning stimulus (flash). S2: imperative stimulus (tone). The asterisks indicate statistical significant differences in CNV parameters (total area *p < 0.05; W2-CNV **p < 0.05). (D) ASF slopes of PKU subjects in basal (black) and post Phe-loading (red) condition.
The P3 components were well represented in all the subjects. No differences were found in the N1 and P3b (counting and motor response) amplitudes and in latencies between T1 and T2 recordings. Moreover, the number of correct responses and RTs were comparable in both the electrophysiological recordings. No correlations emerged between the P3 parameters and other clinical, biochemical, and neuropsychological data, except for a negative correlation between age and P3b latency either in counting or in motor response in T2 recording session (counting P3 latency: Fz: r = −0.512, p = 0.043; Cz: r = −0.486, p = 0.057; Pz: r = −0.433, p = 0.09; motor response P3 latency: Fz: r = −0.541, p = 0.046; Cz: r = −0.568, p = 0.027; Pz: r = −0.379, p = 0.137). A further marginal negative correlation emerged between IQ and P3 latencies at T2 (counting: Pz: r = −0.484, p = 0.057; motor response: Pz: r = −0.382, p = 0.131).
Contingent negative variation waves were elicited with a well detectable shape (28) in all the subjects; the total CNV area (Fz: p = 0.06; Cz: p = 0.02; Pz: p = 0.02), as well as the W2-CNV area at the Pz site (p = 0.05) were smaller at T2 than T1. RT values were comparable between the two ERP recording sessions.
Subgroup Analysis
Once the sample was sub-grouped in tertiles according to the age of the patients, only those younger than 13 showed a significant delay in RTs in P3 motor responses after the Phe-loading respect to basal evaluation (p = 0.05). Moreover in the same patients, the total CNV (Fz: p = 0.03; Cz: p = 0.02; Pz: p = 0.03) and the W2-CNV areas (Cz: p = 0.05; Pz: p = 0.006) were smaller after Phe-loading than in basal condition (Figure 2). None of such alterations was detected in subjects older than 13 years under the effect of Phe-loading. The blood Phe concentration (micromolar) at the T2 test was not significantly different in patients younger and older than 13 (1120.8 ± 253.7 vs. 1133.5 ± 134.4).
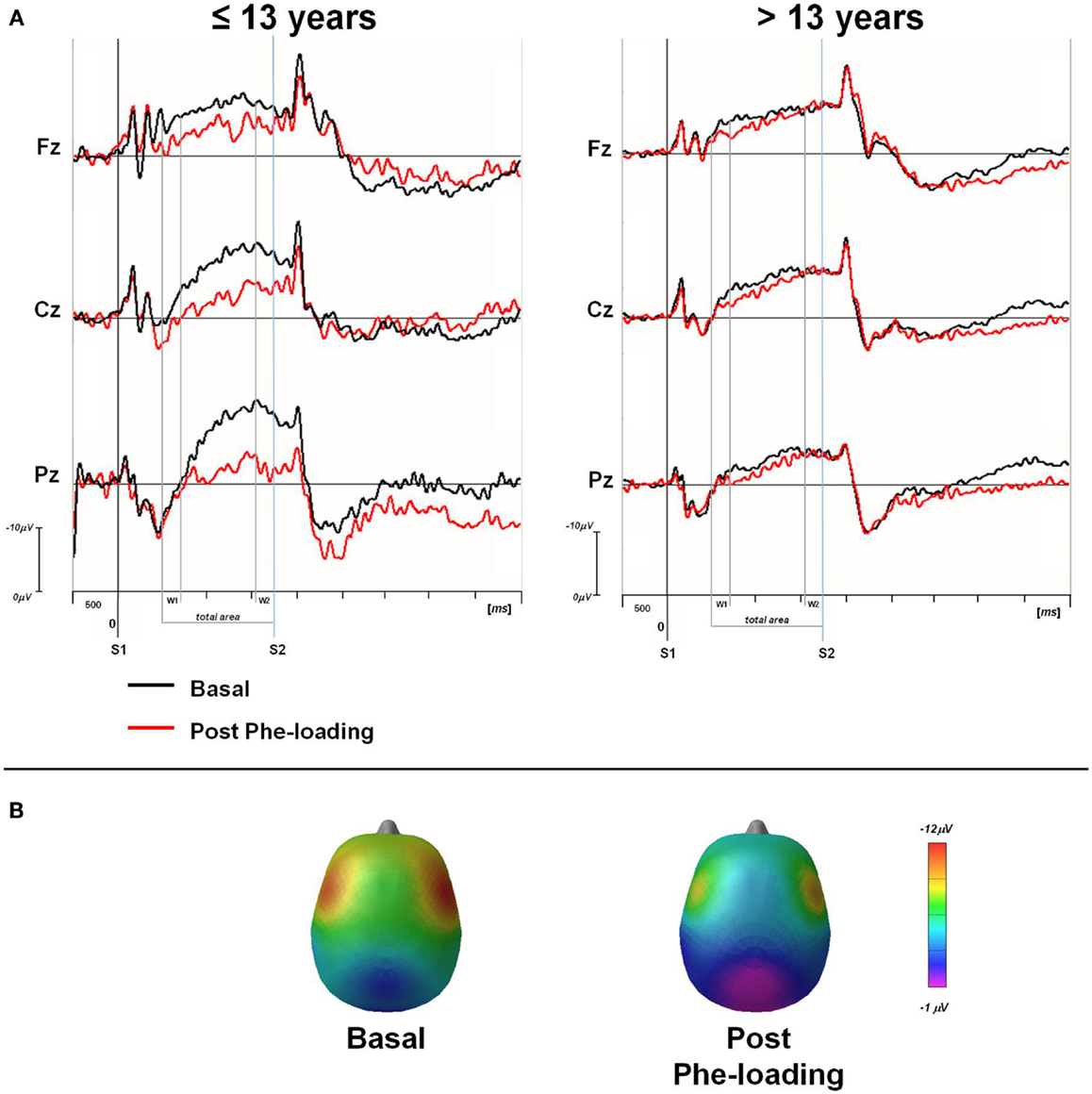
Figure 2. (A) Grand averaged CNV waveforms, with W1, W2, and total areas highlighted, superimposed at midline scalp sites for the two groups of PKU subjects (≤13 and >13 years). CNV was obtained in basal (black line) and post Phe-loading (red line) condition. S1: warning stimulus (flash). S2: imperative stimulus (tone). (B) Scalp potential maps at 1650 ms (mean value of W2-CNV) for ≤13 years PKU subjects in basal and post Phe-loading condition.
Re-examining neurocognitive results in the light of the psychophysiological data, patients younger than 13 were more impaired in the following tests: WCST total number of errors (p = 0.036); RCFC trial (p = 0.04) and immediate recall trial (p = 0.03); Leiter-R attention sustained subtest (p = 0.027); and spatial memory subtest (p = 0.039). They had also a relatively lower IQ [89.33 (SD 7.84) vs. 98.19 (SD 17.61); p = 0.132]. Moreover, a positive correlation between IQ and RCFC (r = 0.759; p < 0.01) was observed only in this group. The differences among subgroups (younger and older than 13) could not be explained on the base of any of demographic and treatment variables showed in Table 1.
Discussion
While we found a normal pattern of ERPs in early-treated PKU patients during their second decade of life, a selective psychophysiological vulnerability to a rapid increase of blood Phe could be demonstrated only in PKU subjects younger than 13. A mild neurocognitive impairment was also found in these patients with respect to the older subjects.
Mismatch negativity latency and amplitudes were preserved in early-treated PKU patients whatever the concomitant and historical quality of biochemical control. We cannot confirm the correlation between lifetime and concurrent Phe level and MMN amplitudes (29). However, we cannot rule out an age-related vulnerability of MMN (30), since our patients were older than those studied by de Sonneville et al. (29). In addition, our result shows that an acute increase in Phe does not affect the pre-attentive auditory discrimination processes, as demonstrated by the short term stability of MMN parameters after Phe-loading. We can therefore hypothesize that the connectivity between the auditory and the frontal cortices, that is thought to be is crucial for the genesis of the MMN phenomenon (13), is preserved in early-treated PKU subjects during their second decade of life.
In our subjects, IDAP value was not affected by Phe-loading. This is an interesting result since IDAP is thought to be inversely related to central serotonin neurotransmission in humans and in animal models (31), and serotonin metabolism and transmission are potentially affected by the increase of Phe levels in blood and brain (32, 33).
P300 is produced when one attends to a stimulus, and it is often interpreted as the first major component reflecting selective attentional processing of the stimulus (34).
Observing that P300 latency is influenced by levodopa treatment in Parkinson disease (21) has suggested it as possible component of dopaminergic network. Two relevant results emerged from our study as far as P300 is concerned: (a) an inverse correlation between age and P3b latency after Phe-loading; (b) a significant delay in RTs in motor responses in younger patients after Phe-loading. Both data suggest a specific vulnerability of the network generating this potential to Phe according to patients’ age. A severe derangement of P300 wave under oddball auditory paradigm was found in late-treated PKU patients, while early-treated patients showed an increase of P300 latency and amplitude unrelated to historical and/or concomitant levels of Phe (35). These findings were not confirmed in younger early and continuously treated PKU patients, which were studied with a visual discriminative paradigm (36). In another study, de Sonneville et al. (37) confirmed the absence of differences for selection positivity over anterior sites (P180) associated with target detection in children with PKU. Moreover, negative (N90, N180) and positive (P180) selection potentials over fronto-central sites seemed to be depending on concurrent and historical Phe levels, whereas sensory potential (N170) depended more strongly on historical Phe levels. They also found a higher number of false positive responses on orientation relevant and irrelevant trial in PKU as compared with controls, and less accurateness on PKU subjects with higher value of lifetime Phe levels in blood. Both studies confirm a correlation between age and RT. In our study, we are not able to confirm such correlation.
Contingent negative variation is associated with activation in the prefrontal cortex (PFC) (38, 39) potentially one of the most vulnerable brain structure in PKU patients because of the peculiar fragility of dopaminergic connectivity in this region (40, 41). The cognitive generator is simple attentional anticipation: a first warning stimulus is a cue that a second stimulus is about to appear, to which the subject responds (usually by pressing a key). When the initial response to the warning stimulus is completed, a negativity develops up until the second stimulus appears. It has been shown that such negativity is correlated to the performance on psychometric measures associated with executive functions, and by extension to PFC functioning (42–44). The increase of CNV amplitudes with the age over the vertex has suggested a maturational trend of this potential (30, 42, 45).
In all subjects, we recorded a normal pattern of the CNV waves in basal condition. However, a couple of hour after Phe-loading, a derangement of the total CNV amplitude emerged suggesting a suppression of the cerebral on-going activity thought to prepare the attentional system for a rapid motor response after a warning signal (24). Such alerting response seems to be compromised particularly in its late phase (W2-CNV), thus indicating a peculiar dysfunction of the premotor cerebral activation for a motor response. Conversely, the orienting phase of this attentional reaction is preserved, as suggested by the stability of the early orienting CNV window (W1-CNV). Interestingly, such dysfunction in CNV task occurred only in patients younger than 13.
Independently from psychophysiological alterations, patients younger than 13 showed some impairment in tests exploring visual-spatial and visual memory, sustained and shared attention. The occurrence of neurocognitive disorders in early-treated PKU has been the object of several studies over the last 10 years (46). A meta-analysis focusing on the speed of information processing in early-treated PKU, concluded that RT and choice RT were increased as function of concurrent levels of blood Phe with a threshold effect that was <320 and <570 μM in children and adolescents, respectively, thus suggesting an age-related vulnerability of immature brain to the abnormal concentrations of this amino acid (47). Attention, inhibition, and motor control were additional functions potentially impaired in early-treated PKU subjects in comparison with controls (48). No longitudinal designed study focusing on the developmental trajectory of neurocognitive skills in PKU subjects is so far available. So the consequences (if any) of such alterations on the neurocognitive outcome and adaptive competences in adult life remain to be explored.
While the low numerousness of the sample requires cautions in the interpretation of our results, the pattern of vulnerability we detected was congruous with other previous clinical observations.
In conclusion, our data showed that two independent measures of brain functioning, one exploring complex neurocognitive skills, the other the sensibility to a rapid increase of Phe of specific information processing networks, are impaired in younger but not in older PKU patients.
Such age-related vulnerability recommends a continuous dietary control in PKU patients during adolescence, and supports a pathogenetic model based on the relative vulnerability of the emerging functions to Phe suggesting a neurodevelopmental approach to the study of the neurocognitive outcome in PKU.
Conflict of Interest Statement
The authors declare that the research was conducted in the absence of any commercial or financial relationships that could be construed as a potential conflict of interest.
References
1. Scriver CR, Kaufman S. Hyperphenylalaninemia: phenylalanine hydroxylase deficiency. 8th ed. In: Scriver CR, Beaudet AL, Sly WS, Valle D, editors. The Metabolic and Molecular Basis of Inherited Disease. New York, NY: McGraw Hill (2001). p. 1667–724.
2. Enns GM, Koch R, Brumm V, Blakely E, Suter R, Jurecki E. Suboptimal outcomes in patients with PKU treated early with diet alone: revisiting the evidence. Mol Genet Metab (2010) 101:99–109. doi: 10.1016/j.ymgme.2010.05.017
3. Moyle JJ, Fox AM, Bynevelt M, Arthur M, Burnett JR. A neuropsychological profile of off-diet adults with phenylketonuria. J Clin Exp Neuropsychol (2007) 29:436–41. doi:10.1080/13803390600745829
4. Bilder DA, Burton BK, Coon H, Leviton L, Ashworth J, Lundy BD, et al. Psychiatric symptoms in adult with phenylketonuria. Mol Genet Metab (2013) 108:155–60. doi:10.1016/j.ymgme.2012.12.006
5. van Spronsen FJ, Hoeksma M, Reijngoud DJ. Brain dysfunction in phenylketonuria: is phenylalanine toxicity the only possible cause? J Inherit Metab Dis (2009) 32:46–51. doi:10.1007/s10545-008-0946-2
6. Smith I, Beasley MG, Ades AE. Intelligence and quality of dietary treatment in phenylketonuria. Arch Dis Child (1990) 65:472–8. doi:10.1136/adc.65.5.472
7. Waisbren SE, Noel K, Fahrbach K, Cella C, Frame D, Dorenbaum A, et al. Phenylalanine blood levels and clinical outcomes in phenylketonuria: a systematic literature review and meta-analysis. Mol Genet Metab (2007) 92:63–70. doi:10.1016/j.ymgme.2007.05.006
8. Bauman ML, Kemper TL. Morphologic and histoanatomic observations of the brain in untreated human phenylketonuria. Acta Neuropathol (1982) 58:55–63. doi:10.1007/BF00692698
9. Cardona F, Leuzzi V, Antonozzi I, Benedetti P, Loizzo A. The development of auditory and visual evoked potentials in early treated phenylketonuric children. Electroencephalogr Clin Neurophysiol (1991) 80:8–15. doi:10.1016/0168-5597(91)90036-W
10. Walter JH, White FJ. Blood phenylalanine control in adolescents with phenylketonuria. Int J Adolesc Med Health (2004) 16:41–5. doi:10.1515/IJAMH.2004.16.1.41
11. Möller HE, Weglage J, Wiedermann D, Ullrich K. Blood-brain barrier phenylalanine transport and individual vulnerability in phenylketonuria. J Cereb Blood Flow Metab (1998) 18:1184–91. doi:10.1097/00004647-199811000-00004
12. Weglage J, Wiedermann D, Denecke J, Feldmann R, Koch HG, Ullrich K, et al. Individual blood-brain barrier phenylalanine transport in siblings with classical phenylketonuria. J Inherit Metab Dis (2002) 25:431–6. doi:10.1023/A:1021234730512
13. Näätänen R, Paavilainen P, Rinne T, Alho K. The mismatch negativity (MMN) in basic research of central auditory processing: a review. Clin Neurophysiol (2007) 118:2544–90. doi:10.1016/j.clinph.2007.04.026
14. Javitt DC, Steinscheneider M, Schroeder CE, Arezzo JC. Role of cortical N-methyl-D-aspartate receptors in auditory sensory memory and mismatch negativity generation: implications for schizophrenia. Proc Natl Acad Sci U S A (1996) 93:11962–7. doi:10.1073/pnas.93.21.11962
15. Ehrlichman RS, Maxwell CR, Majumdar S, Siegel SJ. Deviance-elicited changes in event-related potentials are attenuated by ketamine in mice. J Cogn Neurosci (2008) 20:1403–14. doi:10.1162/jocn.2008.20097
16. Pekkonen E, Jousmäki V, Reinikainen K, Partanen J. Automatic auditory discrimination is impaired in Parkinson’s disease. Electroencephalogr Clin Neurophysiol (1995) 95:47–52. doi:10.1016/0013-4694(94)00304-4
17. Kähkönen S, Ahveninen J, Jääskeläinen IP, Kaakkola S, Näätänen R, Huttunen J, et al. Effects of haloperidol on selective attention: a combined whole-head MEG and high-resolution EEG study. Neuropsychopharmacology (2001) 25:498–504. doi:10.1016/S0893-133X(01)00255-X
18. Kähkönen S, Mäkinen V, Jääskeläinen IP, Pennanen S, Liesivuori J, Ahveninen J. Serotonergic modulation of mismatch negativity. Psychiatry Res (2005) 138:61–74. doi:10.1016/j.pscychresns.2004.09.006
19. Polich J, Criado JR. Neuropsychology and neuropharmacology of P3a and P3b. Int J Psychophysiol (2006) 60:172–85. doi:10.1016/j.ijpsycho.2005.12.012
20. Nieuwenhuis S, Aston-Jones G, Cohen JD. Decision making, the P3, and the locus coeruleus-norepinephrine system. Psychol Bull (2005) 131:510–32. doi:10.1037/0033-2909.131.4.510
21. Stanzione P, Fattapposta F, Giunti P. P300 variations in Parkinsonian patients before and during dopaminergic monotherapy: a suggested dopamine component in P300. Electroencephalogr Clin Neurophysiol (1991) 80:446–53. doi:10.1016/0168-5597(91)90093-D
22. Walter WG, Cooper R, Aldridge VJ, McCallum XC, Winter AL. Contingent negative variation: an electric sign of sensori-motor association and expectancy in the human brain. Nature (1964) 203:380–4. doi:10.1038/203380a0
24. Linssen AM, Vuurman EF, Sambeth A, Nave S, Spooren W, Vargas G, et al. Contingent negative variation as a dopaminergic biomarker: evidence from dose-related effects of methylphenidate. Psychopharmacology (Berl) (2011) 218:533–42. doi:10.1007/s00213-011-2345-x
25. Hegerl U, Juckel G. Intensity dependence of auditory evoked potentials as an indicator of central serotoninergic neurotransmission: a new hypothesis. Biol Psychiatry (1993) 33:173–87. doi:10.1016/0006-3223(93)90137-3
26. Duncan CC, Barry RJ, Connolly JF, Fischer C, Michie PT, Näätänen R, et al. Event-related potentials in clinical research: guidelines for eliciting, recording, and quantifying mismatch negativity, P300, and N400. Clin Neurophysiol (2009) 120:1883–908. doi:10.1016/j.clinph.2009.07.045
27. Travis F, Tecce JJ. Effects of distracting stimuli on CNV amplitude and reaction time. Int J Psychophysiol (1998) 31:45–50. doi:10.1016/S0167-8760(98)00037-3
28. Segalowitz SJ, Davies PL. Charting the maturation of the frontal lobe: an electrophysiological strategy. Brain Cogn (2004) 55:116–33. doi:10.1016/S0278-2626(03)00283-5
29. de Sonneville LM, Huijbregts SC, Licht R, Sergeant JA, van Spronsen FJ. Pre-attentive processing in children with early and continuously-treated PKU. Effects of concurrent Phe level and lifetime dietary control. J Inherit Metab Dis (2011) 34:953–62. doi:10.1007/s10545-011-9321-9
30. Bishop DV, Hardiman MJ, Barry JG. Is auditory discrimination mature by middle childhood? A study using time-frequency analysis of mismatch responses from 7 years to adulthood. Dev Sci (2011) 14:402–16. doi:10.1111/j.1467-7687.2010.00990.x
31. Ambrosini A, de Noordhout AM, Sándor PS, Schoenen J. Electrophysiological studies in migraine: a comprehensive review of their interest and limitations. Cephalalgia (2003) 23:13–31. doi:10.1046/j.1468-2982.2003.00571.x
32. Nielsen JB, Lou HC, Glütter F. Effects of diet discontinuation and dietary tryptophan supplementation on neurotransmitter metabolism in phenylketonuria. Brain Dysfunct (1988) 1:51–6.
33. Pascucci T, Ventura R, Puglisi-Allegra S, Cabib S. Deficits in brain serotonin synthesis in a genetic mouse model of phenylketonuria. Neuroreport (2002) 13:2561–4. doi:10.1097/01.wnr.0000047690.08940.39
34. Polich J. Updating P300: an integrative theory of P3a and P3b. Clin Neurophysiol (2007) 118:2128–48. doi:10.1016/j.clinph.2007.04.019
35. Leuzzi V, Seri S, Cerquiglini A, Carducci C, Carducci C, Antonozzi I. Derangement of the dopaminergic system in phenylketonuria: study of the event-related potential (P300). J Inherit Metab Dis (2000) 23:317–20. doi:10.1023/A:1005646206348
36. Henderson RM, McCulloch DL, Herbert AM, Robinson PH, Taylor MJ. Visual event-related potentials in children with phenylketonuria. Acta Paediatr (2000) 89:52–7. doi:10.1111/j.1651-2227.2000.tb01187.x
37. de Sonneville LM, Huijbregts SC, van Spronsen FJ, Verkerk PH, Sergeant JA, Licht R. Event-related potential correlates of selective processing in early- and continuously-treated children with phenylketonuria: effects of concurrent phenylalanine level and dietary control. Mol Genet Metab (2010) 99:S10–7. doi:10.1016/j.ymgme.2009.10.177
38. Rosahl SK, Knight RT. Role of prefrontal cortex in generation of the contingent negative variation. Cereb Cortex (1995) 5:123–34. doi:10.1093/cercor/5.2.123
39. Zappoli R. Permanent or transitory effects on neurocognitive components of the CNV complex induced by brain dysfunctions, lesions and ablations in humans. Int J Psychophysiol (2003) 48:189–220. doi:10.1016/S0167-8760(03)00054-0
40. Diamond A, Herzberg C. Impaired sensitivity to visual contrast in children treated early and continuously for phenylketonuria. Brain (1996) 119:523–38. doi:10.1093/brain/119.2.523
41. Leuzzi V, Rinalduzzi S, Chiarotti F, Garzia P, Trasimeni G, Accornero N. Subclinical visual impairment in phenylketonuria. A neurophysiological study (VEP-P) with clinical, biochemical, and neuroradiological (MRI) correlations. J Inherit Metab Dis (1998) 21:351–64. doi:10.1023/A:1005346422918
42. Segalowitz SJ, Unsal A, Dywan J. Cleverness and wisdom in 12-year-olds: electrophysiological evidence for late maturation of the frontal lobe. Dev Neuropsychol (1992) 8:279–98. doi:10.1080/87565649209540528
43. Segalowitz SJ, Unsal A, Dywan J. CNV evidence for the distinctiveness of frontal and posterior neural processes in a traumatic brain injured (TBI) population. J Clin Exp Neuropsychol (1992) 14:108–28. doi:10.1080/01688639208402844
44. Dywan J, Segalowitz SJ, Williamson L. Source monitoring during name recognition in older adults: psychometric and electrophysiological correlates. Psychol Aging (1994) 9:568–77. doi:10.1037/0882-7974.9.4.568
45. Timsit-Berthier M, Hausman J. Communications. Etude de la VCN et du phenomene de preparation motrice chez desengants de 5 a 15 ans. Neurophysiol Clin (1972) 2:141–6.
46. van Spronsen FJ, Huijbregts SCJ, Bosch AM, Leuzzi V. Cognitive, neurophysiological, neurological and psychosocial outcomes in early-treated PKU-patients: a start toward standardized outcome measurement across development. Mol Genet Metab (2011) 104:S45–51. doi:10.1016/j.ymgme.2011.09.036
47. Albrecht J, Garbade SF, Burgard P. Neuropsychological speed tests and blood phenylalanine levels in patients with phenylketonuria: a meta-analysis. Neurosci Biobehav Rev (2009) 33:414–21. doi:10.1016/j.neubiorev.2008.11.001
Keywords: PKU, event-related potentials, event-related potentials in PKU, neurocognitive outcome in PKU
Citation: Leuzzi V, Mannarelli D, Manti F, Pauletti C, Locuratolo N, Carducci C, Carducci C, Vanacore N and Fattapposta F (2014) Age-related psychophysiological vulnerability to phenylalanine in phenylketonuria. Front. Pediatr. 2:57. doi: 10.3389/fped.2014.00057
Received: 23 December 2013; Accepted: 23 May 2014;
Published online: 23 June 2014.
Edited by:
John Vijay Sagar Kommu, National Institute of Mental Health and Neurosciences, IndiaReviewed by:
Preeti Kandasamy, National Institute of Mental Health and Neurosciences, IndiaSuhash Chakraborty, Hindustan Aeronautics Limited Hospital, India
Copyright: © 2014 Leuzzi, Mannarelli, Manti, Pauletti, Locuratolo, Carducci, Carducci, Vanacore and Fattapposta. This is an open-access article distributed under the terms of the Creative Commons Attribution License (CC BY). The use, distribution or reproduction in other forums is permitted, provided the original author(s) or licensor are credited and that the original publication in this journal is cited, in accordance with accepted academic practice. No use, distribution or reproduction is permitted which does not comply with these terms.
*Correspondence: Vincenzo Leuzzi, Department of Paediatrics, Child Neurology and Psychiatry, Sapienza Università di Roma, via dei Sabelli 108, Rome 00185, Italy e-mail: vincenzo.leuzzi@uniroma1.it