Managing Recovery Resilience in Coral Reefs Against Climate-Induced Bleaching and Hurricanes: A 15 Year Case Study From Bonaire, Dutch Caribbean
- 1School of Marine Sciences, University of Maine, Orono, ME, United States
- 2Island Institute, Rockland, ME, United States
- 3Environmental Defense Fund, Boston, MA, United States
- 4Reef Support BV, Bonaire, Netherlands
- 5Marine Spatial Ecology Lab, University of Queensland, Brisbane, QLD, Australia
- 6Bigelow Laboratory for Ocean Sciences, Boothbay Harbor, ME, United States
- 7Bren School of Environmental Science & Management, University of California, Santa Barbara, Santa Barbara, CA, United States
Coral reefs are among the world’s most endangered ecosystems. Coral mortality can result from ocean warming or other climate-related events such as coral bleaching and intense hurricanes. While resilient coral reefs can recover from these impacts as has been documented in coral reefs throughout the tropical Indo-Pacific, no similar reef-wide recovery has ever been reported for the Caribbean. Climate change-related coral mortality is unavoidable, but local management actions can improve conditions for regrowth and for the establishment of juvenile corals thereby enhancing the recovery resilience of these ecosystems. Previous research has determined that coral reefs with sufficient herbivory limit macroalgae and improve conditions for coral recruitment and regrowth. Management that reduces algal abundance increases the recovery potential for both juvenile and adult corals on reefs. Every other year on the island of Bonaire, Dutch Caribbean, we quantified patterns of distribution and abundance of reef fish, coral, algae, and juvenile corals along replicate fixed transects at 10 m depth at multiple sites from 2003 to 2017. Beginning with our first exploratory study in 2002 until 2007 coral was abundant (45% cover) and macroalgae were rare (6% cover). Consecutive disturbances, beginning with Hurricane Omar in October 2008 and a coral bleaching event in October 2010, resulted in a 22% decline in coral cover and a sharp threefold increase in macroalgal cover to 18%. Juvenile coral densities declined to about half of their previous abundance. Herbivorous parrotfishes had been declining in abundance but stabilized around 2010, the year fish traps were phased out and fishing for parrotfish was banned. The average parrotfish biomass from 2010 to 2017 was more than twice that reported for coral reefs of the Eastern Caribbean. During this same period, macroalgae declined and both juvenile coral density and total adult coral cover returned to pre-hurricane and bleaching levels. To our knowledge, this is the first example of a resilient Caribbean coral reef ecosystem that fully recovered from severe climate-related mortality events.
Introduction
Large-scale and relatively recent coral mortality resulting in the collapse of coral reef ecosystems has been both conspicuous and well documented (Eakin et al., 2010; Hughes et al., 2018). Declines often result from climate-induced stresses such as acute El Niño warming or intense hurricanes. It is easy to conclude that the long-term prognosis for coral reefs is poor, but it is inaccurate to say there is nothing humans can do to halt or slow the decline of these beleaguered ecosystems. In fact, evidence in support of “managed resilience” (sensu Bruno et al., 2019) is central to this case study.
No management actions can “climate-proof” coral reefs. However, some management actions may improve the recovery of coral reef ecosystems following a climate-induced disturbance, and thus improve “recovery resilience”. Resilience is an ecosystem property (Holling, 1973) that affects a system’s ability to resist an extrinsic perturbation that fundamentally changes its structure or to recover from such perturbations (Gunderson, 2000).
We focus on the recovery of coral reefs because it has gone undocumented in some vast tropical regions (but see Ortiz et al., 2018). In a highly cited scientific study entitled: “Disturbance and recovery of coral assemblages” (Connell, 1997), all existing studies on patterns of recovery in coral reefs world-wide were reviewed. While over 50% of Indo-Pacific coral reefs including those in the Great Barrier Reef, Indonesia, Indian Ocean, Guam, and Hawaii were documented to have recovered, there were no examples of Caribbean coral reefs recovering from disturbance. Roff and Mumby (2012) updated this analysis with 38 studies since Connell’s publication, yet only one small part of one Caribbean reef met the original criteria for recovery (Idjadi et al., 2006). To date, no Caribbean coral reef system comprised of many smaller reefs, has been shown to have recovered from a climate-induced perturbation.
Most published studies have documented the decline of coral reef ecosystems in the Caribbean (Gardner et al., 2003; Jackson et al., 2014) and in the tropical Pacific (Bruno and Selig, 2007). The global decline of coral reefs was the impetus for several high impact scientific papers with titles such as “Confronting the coral reef crisis” (Bellwood et al., 2004) and “Rising to the challenge of sustaining coral reef resilience” (Hughes et al., 2010), or specifically asking the shocking question: “Are U.S. coral reefs on the slippery slope to slime?” (Pandolfi et al., 2005). These alarming titles and the associated press coverage caught the attention of managers and policy makers, but, to date, there has been little progress operationalizing the management of coral reef ecosystems (i.e., managed resilience) to enhance recovery resilience. Nevertheless, some studies, such as, “Capturing the cornerstones of coral reef resilience, linking theory to practice” (Nyström et al., 2008), gave clear advice to managers:
“Moving toward operationalizing resilience theory is imperative to the successful management of coral reefs in an increasingly disturbed and human-dominated environment.” (Nyström et al., 2008).
Although coral reefs are complex ecosystems, relatively few “drivers” have been shown to be most important to their structure and function. “Drivers” are processes that control critically important aspects of coral reefs. Several processes can interact with one another that ultimately improve the recovery of coral reefs (Mumby and Steneck, 2008). For example, macroalgae (seaweed) are known to poison corals (Rasher and Hay, 2010) and reduce or halt the settlement and survival of juvenile corals (Arnold et al., 2010; Steneck et al., 2014). Since herbivorous fishes, such as parrotfishes and surgeonfishes, can reduce or eliminate macroalgae from coral reefs (Lewis, 1986; Williams and Polunin, 2001), they enhance coral recruitment and facilitate the growth of reef corals (Burkepile and Hay, 2008). Some branching corals create complex coral habitats into which juvenile reef fish recruit (Caselle and Warner, 1996). The resulting feedbacks maintain healthy coral reefs (Mumby and Steneck, 2008) and are thus the “cornerstones” for managing resilience as advocated by Nyström et al. (2008).
Coral reef “health” and resilience is complicated because all components interact. Therefore, it is difficult or impossible to define a specific ecosystem attribute (i.e., state variable) as being particularly healthy or unhealthy for any given coral reef. Instead, one must measure and track important components as they change through time (Edmunds et al., 2019). Indeed, there is a consensus on trends that constitute healthy trajectories in reef condition. Trends of increasing live coral cover or decreasing macroalgal abundance are both moving toward improved conditions. Herbivores reduce algal abundance but the amount of herbivory to be effective will vary from local to ocean basin scales. For example, coral reefs at identical water depths, light, and wave exposure have significantly greater rates of algal colonization and growth in the Caribbean compared to the tropical Indo-Pacific Ocean (Roff and Mumby, 2012). Accordingly, higher rates of herbivory are necessary to limit algal growth in the Caribbean. Managers perennially would ask how much herbivory is enough (Bozec et al., 2016)? Studying the trajectory of algal growth over time allows managers to determine the success or failure of strategies to manage herbivory or other factors that contribute to algal growth and/or success of reef corals.
Here, we report on a study initiated in 2002 on the island of Bonaire, Dutch Caribbean, in the southern Caribbean (Figure 1). For over 15 years, we regularly monitored the abundance of live coral, algae, reef fishes and juvenile corals at fixed locations at multiple sites, with the aim of documenting trends in key ecosystem drivers within the ecosystem. After 5 years of monitoring, Bonaire’s coral reefs suffered two large-scale disturbances from a hurricane and a coral bleaching event. These disturbances were effectively a “stress-test” that allowed us to gauge the recovery resilience of the monitored coral reefs. All research was conducted in coordination and with the support of ”Stichting Nationale Parken (‘STINAPA’) Bonaire,” the environmental organization charged with managing Bonaire’s coral reefs during the period of study.
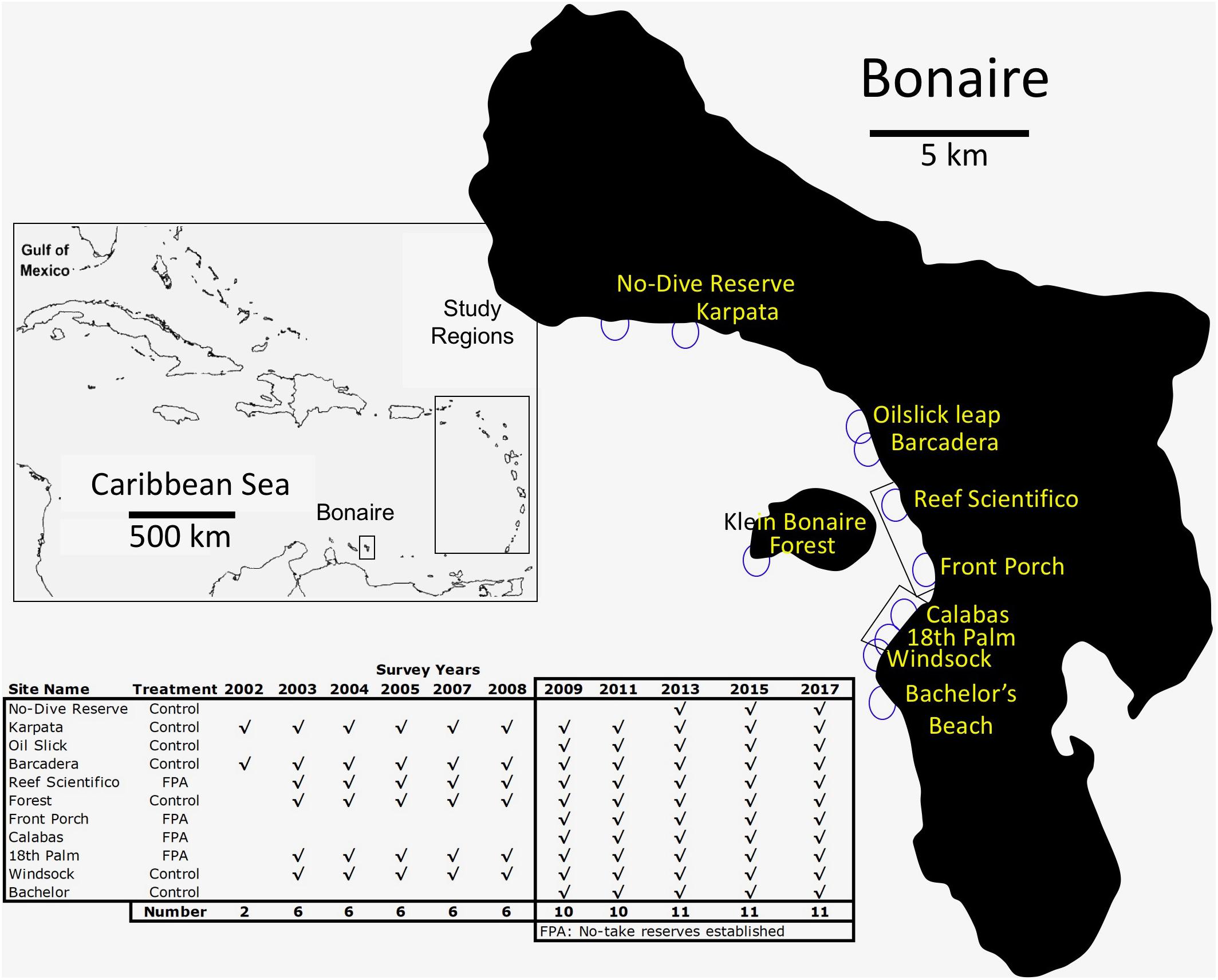
Figure 1. Bonaire, Dutch Caribbean. Inset rectangle shows the island location and the Eastern Caribbean sites from Steneck et al. (2018) used for comparison. Circles on the island map show the location of 10 m depth monitoring stations. Inset table shows duration and frequency of monitoring at those stations. Coastally located rectangles show the location of no-take reserves (called “Fish Protection Areas,” or FPAs) that were established in 2008.
As is necessary with any single-location study we address the question, “compared to what”? Accordingly, we compared our long-term study of Bonaire’s coral reefs with a recently published short-term but geographically broad study of the coral reefs of the Eastern Caribbean conducted in 2013–2014, that used identical methods at 12 islands over a 700 km area to contrast heavily fished sites with no-take reserves (Steneck et al., 2018). Like Bonaire, the reefs surveyed in the Eastern Caribbean were mostly in leeward environments and therefore comparable in terms of physical environment.
Materials and Methods
Monitoring Sites and Protocols
In 2002 we began monitoring Bonaire’s coral reefs at two sites selected by STINAPA as important and representative reef sites. Then in 2003, we began biannual monitoring expanding to six and then 11 STINAPA-selected sites (Figure 1). Monitoring was conducted during the first weeks of March during each monitoring year, so as to minimize seasonal variance.
At each site, we monitored multiple transects set at 10 m depth. At the six long-term monitored sites established in 2003, we placed 10 cm square tiles to create four permanent 10 m long transects per site for quantifying benthic organisms (methods detailed in Arnold et al., 2010). We added five new sites between 2009 and 2013, all set at 10 m depth. Since the reef slope is steep, the transect lines set during each monitoring period at all sites were at, or very near, the specific benthic location of previous monitoring.
Coral and algal abundances were determined from four to eight replicate 10 m transects. Every organism or organism-group was identified under every cm of the transect. However, to only compare abundances on hard substrates, measurements taken from unconsolidated rubble or sand were removed. Each coral species and algal functional group (sensu Steneck and Dethier, 1994) were measured to the nearest cm. Our protocol was commensurable with the Atlantic and Gulf Reef Rapid Assessment (AGRRA) protocol (Lang, 2003).
Algal abundance was quantified by both measuring percent cover (via component lengths) and canopy heights (via a ruler, to the nearest mm) along the transects. This was done separately for filamentous algal turfs, macroalgae, and articulated calcified algae such as Halimeda spp. The percent cover of calcareous crustose coralline algae and less calcified peyssonnelid algae were quantified independently. We quantified the macroalga Lobophora spp. separately from other algae because it is known to be lethal to corals. We also calculated an algal biomass proxy using the volume of algae (called an “algal index” sensu Steneck et al., 2014) that is determined by multiplying algal percent cover by canopy height (mm).
Juvenile coral densities were quantified by placing a 25 × 25 cm quadrat at five locations where adult coral cover was ≤ 25% along each 10m transect (positioned 0, 2.5, 5, 7.5, and 10 m locations). Each coral species ≤ 40 mm maximum diameter was identified and measured to the nearest mm and counted for juvenile coral densities.
Fishes were quantified by swimming 7–10 replicate 30 m × 4 m belt transects at each site each year. This is a standard protocol used by reef scientists (methods in Steneck et al., 2018) and is commensurable with AGRRA. Fish surveys were conducted before benthic surveys to minimize human presence during fish surveys. For fish surveys, the observer attached a 30 m line to dead coral substrate and swam slowly to record all fish species and their sizes (to nearest cm) within the belt. During their return visit over the 30 m belt, the observer recorded the slower moving or less vagile fish taxa.
All of these methods and others along with results and data appendices were produced as biannual reports delivered to STINAPA Bonaire starting in 2003 (see Supplementary Tables S1, S2 for benthic and fish data, respectively). The eight reports totaled 1001 pages and all are available from STINAPA Bonaire1. All data critical for this study are presented in this paper.
Benthic Community Structure Over Time
Data on the percent cover of individual coral species as well as the cover and canopy height of key algal functional groups were square root transformed to down weight the influence of the largest space occupiers. Temporal trends were then visualized using non-metric Multidimensional Scaling Ordination based on the Bray-Curtis dissimilarity coefficient (Clarke, 1993). First, an analysis of change was carried out to check that community structure varied over time. Here, site was included as a random effect and year as a fixed effect within a non-parametric analysis of variance, PERMANOVA (Anderson, 2001). The analysis was then repeated with specific contrasts of interest. These primarily included the key events in the time series; the impact of Hurricane Omar compared to prior years and the impact of the coral bleaching event compared to reefs post-Omar. Two additional comparisons were added for interest sake. The first compared the community structure in 2017 (7 years post-bleaching) to that of the first several years post-bleaching (2011–2015). This comparison was motivated by the rapid recovery observed by 2017, and because it was not specified a priori, we do not place importance on the statistical significance of that particular comparison; rather, we are interested to see which species drove that change. The final comparison asked whether the final community structure (2017) differed from that in the pre-disturbance years (i.e., prior to Hurricane Omar and the bleaching).
To understand which species drove the major changes in benthic community structure we used Similarity Percentage (SIMPER) analysis (Clarke, 1993). We confined our results to the five species or functional groups that mostly strongly accounted for the differences in community structure.
Results
Trends in Coral, Algae, and Herbivores
Coral cover measured at 10 m depth at multiple sites (Figure 1) averaged around 45% until the first decline from Hurricane Omar (October 2008) was detected during the 2009 monitoring period (Figure 2). This was followed by the second coral mortality event resulting from the October 2010 bleaching event (Alemu and Clement, 2014). Following these two disturbances, live coral cover declined 22% from over 45% in 2007 to about 35% in 2011. Coral cover remained reduced until 2015 when it began to increase. By 2017, live coral cover had recovered to its pre-mortality abundance of over 47% (Figure 2). Note that even during the period of lowest coral cover in Bonaire, it exceeded the average found throughout the Eastern Caribbean (i.e., 17.8% ± 1.9 SE, see Steneck et al., 2018). The recovery in coral cover we documented for Bonaire in 2017 was sustained during the 2019 monitoring session. Coral cover recorded at the 11 sites was 46.3% (±2.8 SE) during the 2019 monitoring study (completed after the initial submission; see STINAPA website1).
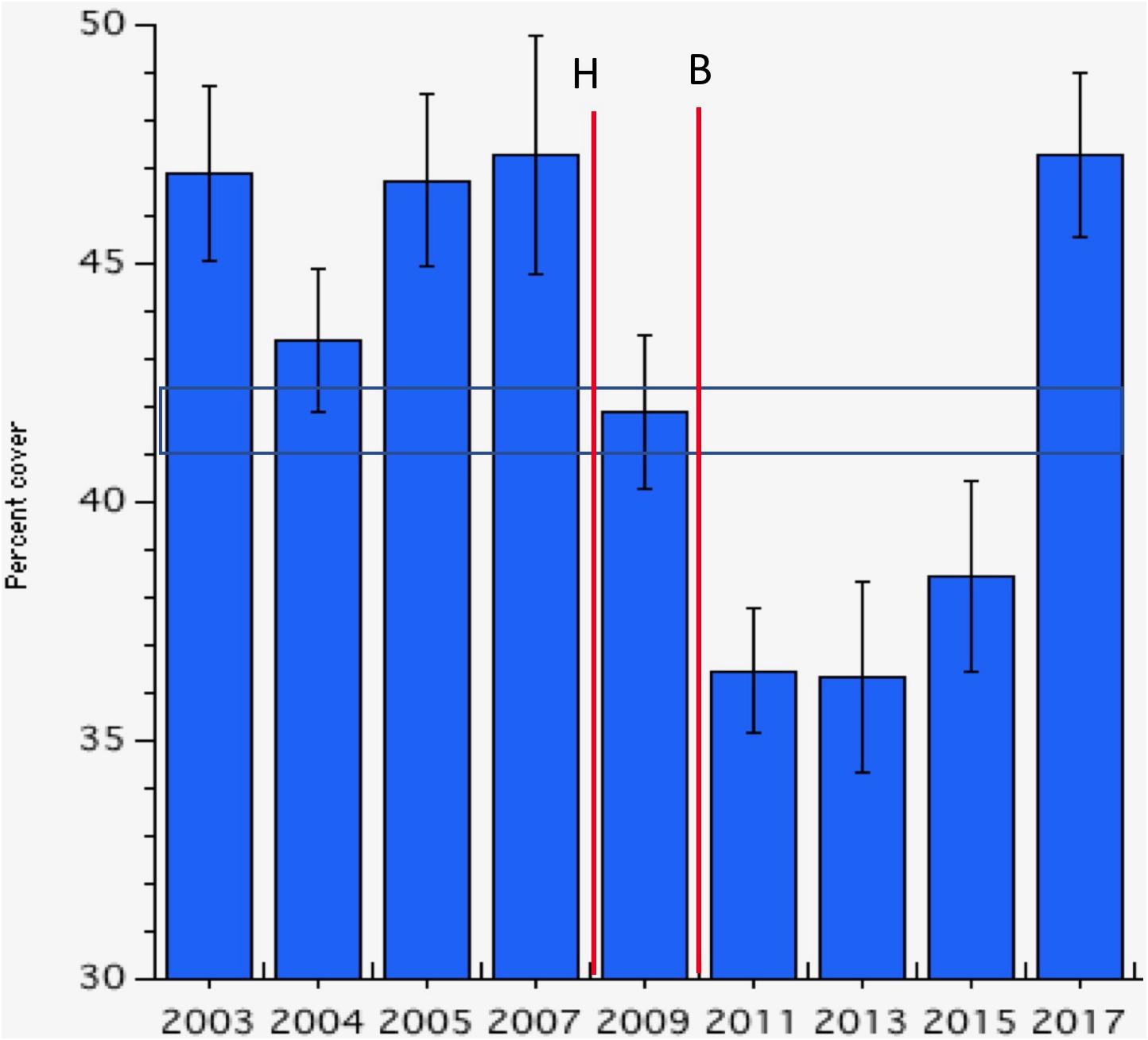
Figure 2. Mean percent cover of live coral at monitored sites (variance here and throughout is expressed as standard error). The horizontal lines illustrate one standard error of the mean over the period. Vertical lines labeled “H” and “B” denote Hurricane Omar (October 2008) and the severe coral bleaching event of October 2010, respectively. For comparison, the average coral cover in the Eastern Caribbean was (17.6% ± 1.9 SE; Steneck et al., 2018 and see below).
Macroalgal abundance at all of Bonaire’s monitored sites remained relatively low over the study period; nevertheless, there were important changes. Percent cover of macroalgae (the most common metric of algal abundance) varied between 1 and 6% cover at all sites from 2002 through 2005 (Supplementary Figure S1). Then, in 2007, average algal cover increased to 8% (±2.3 SE) and peaked in 2011 at 17.7% (±2.6 SE) cover. Algal biomass proxy (“algal index”) increased sharply in 2011 following the coral bleaching event but then declined during each subsequent monitoring period (Figure 3). By 2017, percent cover of macroalgae retreated back to the pre-disturbance level of 6% (±1 SE) although canopy heights remained relatively high (Supplementary Figure S2). Nevertheless, the average percent cover of macroalgae at all study areas of the Eastern Caribbean was 25%, four times higher than that recorded for Bonaire (Steneck et al., 2018).
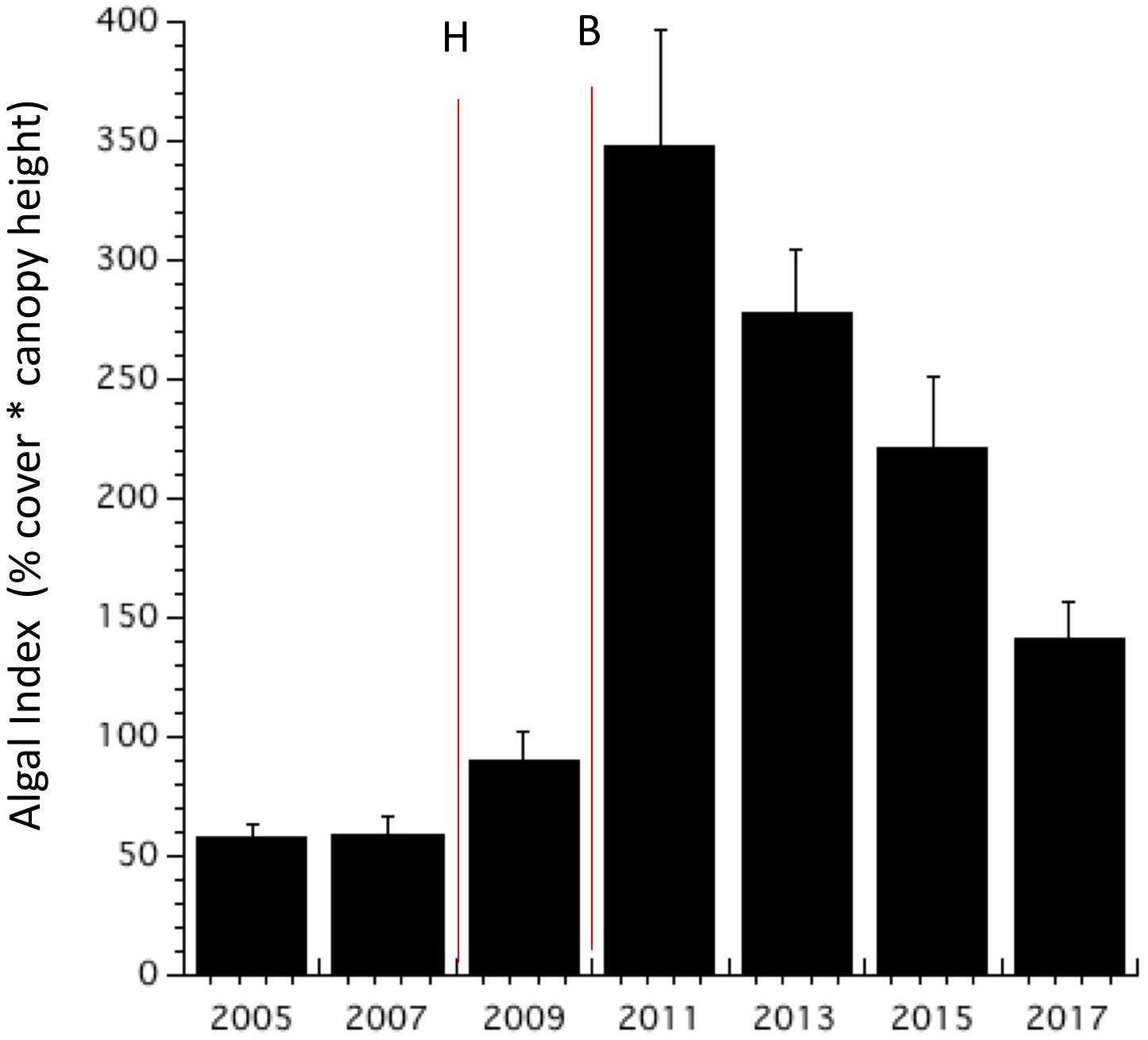
Figure 3. Algal biomass proxy, called the ”algal index” (percent cover times canopy heights for all algae) at monitored sites from 2005 to 2017 (mean + SE). For comparison, the mean algal index across 12 islands of the Eastern Caribbean (i.e., 882 ± 58 SE; Steneck et al., 2018) was more than twice the maximum value recorded for Bonaire in 2011. Vertical lines denote the hurricane (H) and bleaching (B) events.
Juvenile coral densities were relatively high throughout the study period (mean: 21.7 per m2 ± 1.12 SE, from 440 quadrats along 88 transects since 2003; Figure 4). This is high when compared to the juvenile coral densities found in the Eastern Caribbean, which ranged from 1 to 10 among fished and no-take reserve sites (Figure 4; Steneck et al., 2018). Over the 2003–2017 study period, juvenile coral densities below 10 juvenile corals/m2 were only observed in 2009 and 2013 (Figure 4).
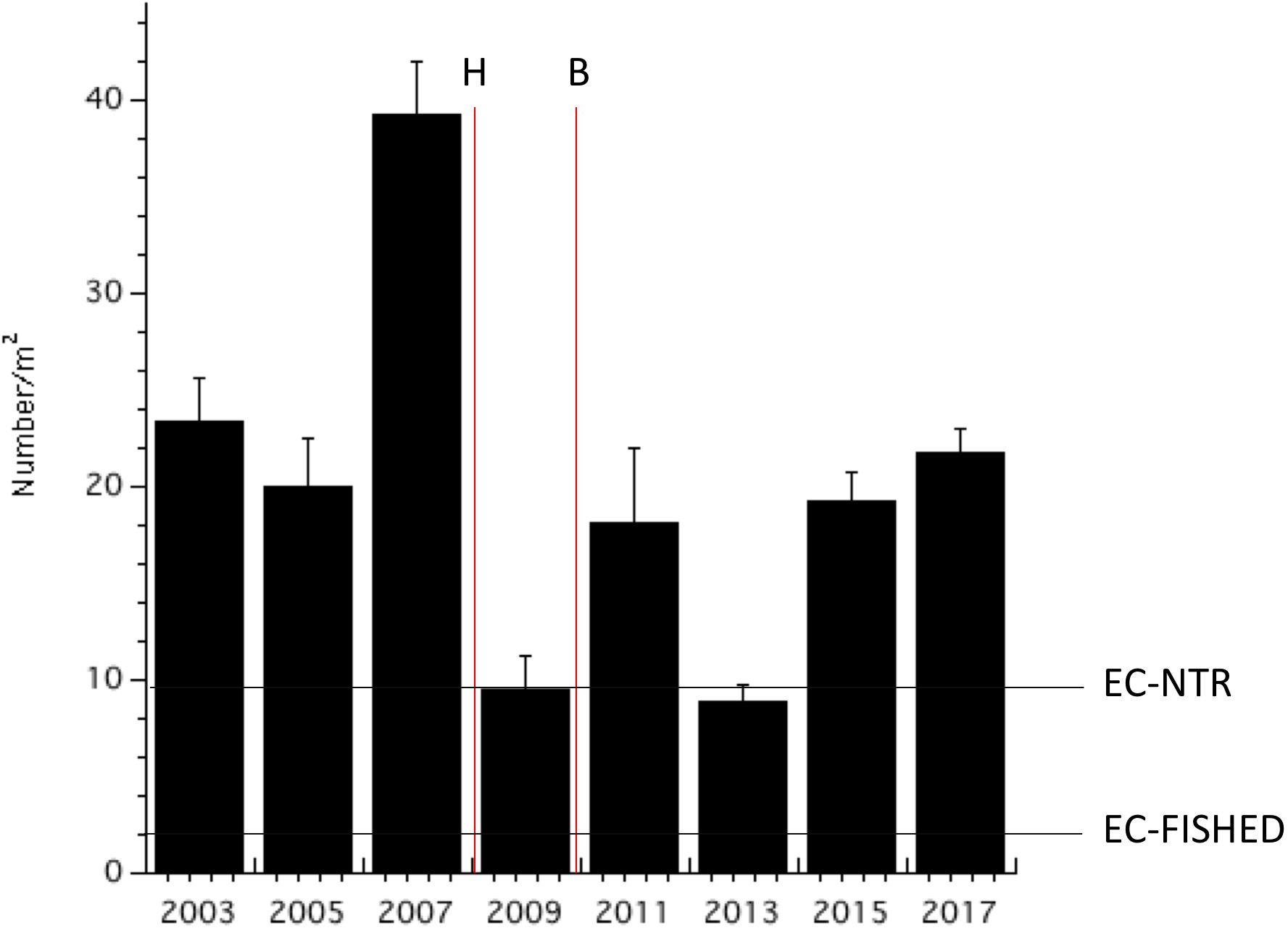
Figure 4. Mean juvenile coral densities from 2003 to 2017 (results are from 316 transects and 1580 quadrats). Vertical lines showing hurricane and bleaching impacts are labeled as in Figure 2. Note the increase in 2011 was due to recruitment of Agaricia spp. that then grew out of the juvenile size class and contributed to the overall increase in coral cover.
An analysis of spatiotemporal trends in juvenile coral density unsurprisingly found that ‘year’ had the greatest impact, explaining 26% of the variance (p = 0.001). The planned temporal contrasts found significant effects of disturbance (i.e., 2005/2007 pre-disturbance vs. 2009/2011) and differences between the pre-disturbance density (2005/2007) and that of the final year. These impacts accounted for a further 9 and 3% of the variance respectively (p = 0.001). Macroalgal cover was negatively associated with juvenile coral density and explained 3% of the variance (p = 0.005) on its own. However, significant interactions occurred between time and macroalgal cover. The overall interaction explained 5% of variance (p = 0.011) and the specific interactions, macroalgal cover vs. disturbance effect and macroalgal cover vs. pre/post disturbance, accounted for 4% (p = 0.006) and 1% (p = 0.02) respectively. There was no effect of macroalgal cover pre-disturbance but a significant negative effect post-disturbance. Site was entered as a random effect but was not significant (p = 0.57).
Bonaire’s reef corals at 10 m depth were strongly dominated by species of the major framework-building coral Orbicella species (Figure 5). The two dominant coral species of that genus combine to about 20% cover. The decline in coral cover following the two disturbance events was sharpest in Agaricia agaricites, Madracis, and Colpophyllia (Figure 6). These declines were added to the gradual declines in the two dominant species O. annularis and O. faviolata. Montastraea cavernosa increased in abundance over the early post-recovery study period. All of the six dominant species increased in the most recent monitoring periods (Figure 6).
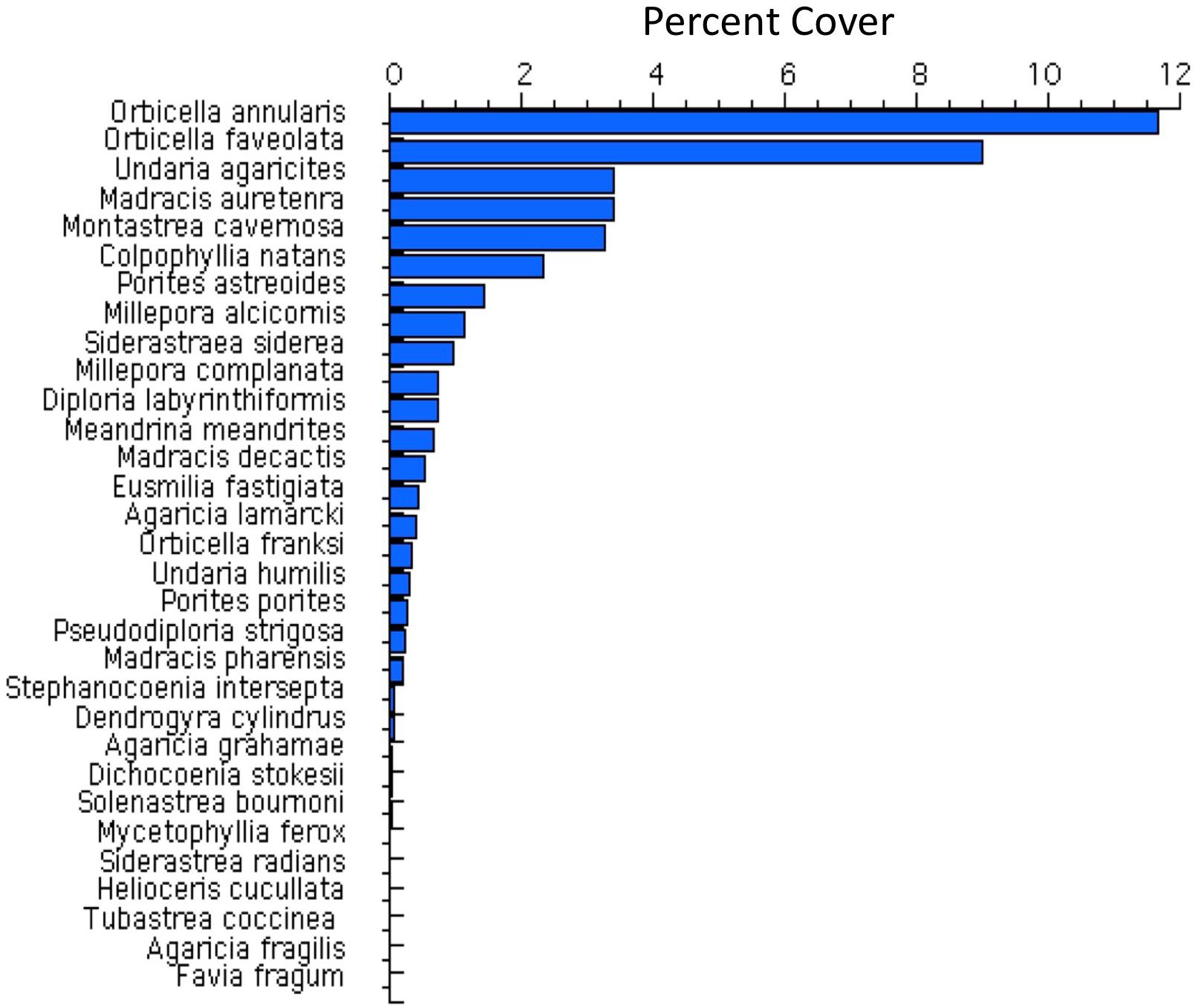
Figure 5. Average percent cover of coral species from all 307 transects quantified from 2004 (when we began documenting individual species) to 2017.
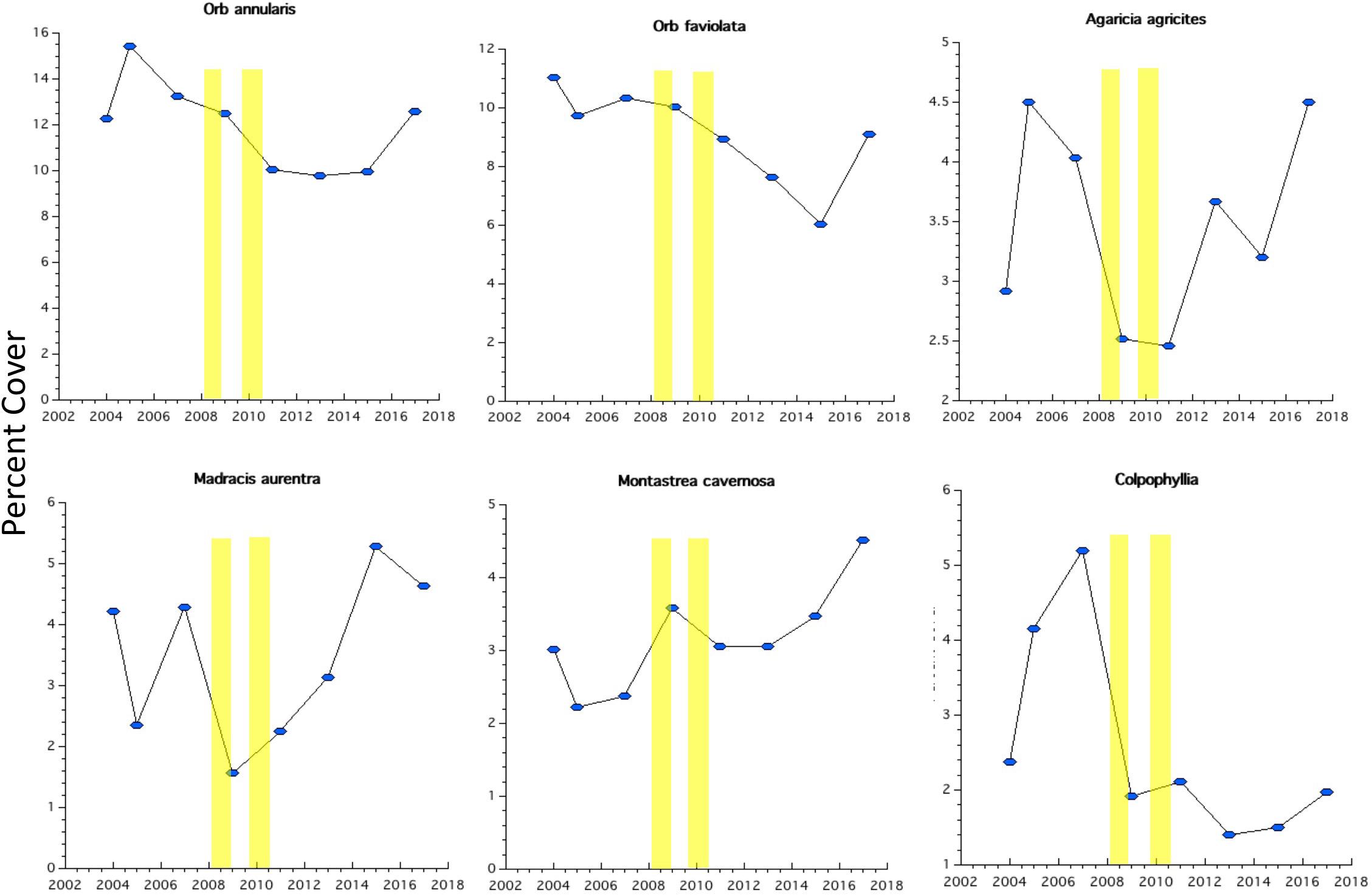
Figure 6. Abundance trajectories of the six most abundant coral species since 2004. Vertical yellow bars denote the timing of Hurricane Omar and the 2010 coral bleaching event.
Although all major species of reef fishes were censused regularly (see STINAPA website2), here we report only on scarine (parrotfish) populations. Parrotfish have been shown to be the most abundant and most important herbivores on Caribbean coral reefs and can facilitate coral recruitment by reducing algal biomass (Mumby et al., 2006; Arnold et al., 2010; Mumby and Harborne, 2010; Steneck et al., 2014).
Parrotfish biomass and population densities declined from 2003 to 2009 (Figures 7, 8). Both biomass and abundance then stabilized until 2017 when parrotfish densities increased sharply. Parrotfish biomass recorded in 2017 was twice that recorded throughout the Eastern Caribbean, including the no-take reserves (i.e., ”EC-NTR” in Figure 7). Parrotfish biomass did not change following the hurricane and bleaching events (Figure 7). In contrast, parrotfish population density declined in 2011 and remained low by Bonaire’s standards until 2017 (Figure 8). Monitoring should be continued to be sure parrotfish abundance remains sufficiently high to control algal abundance.
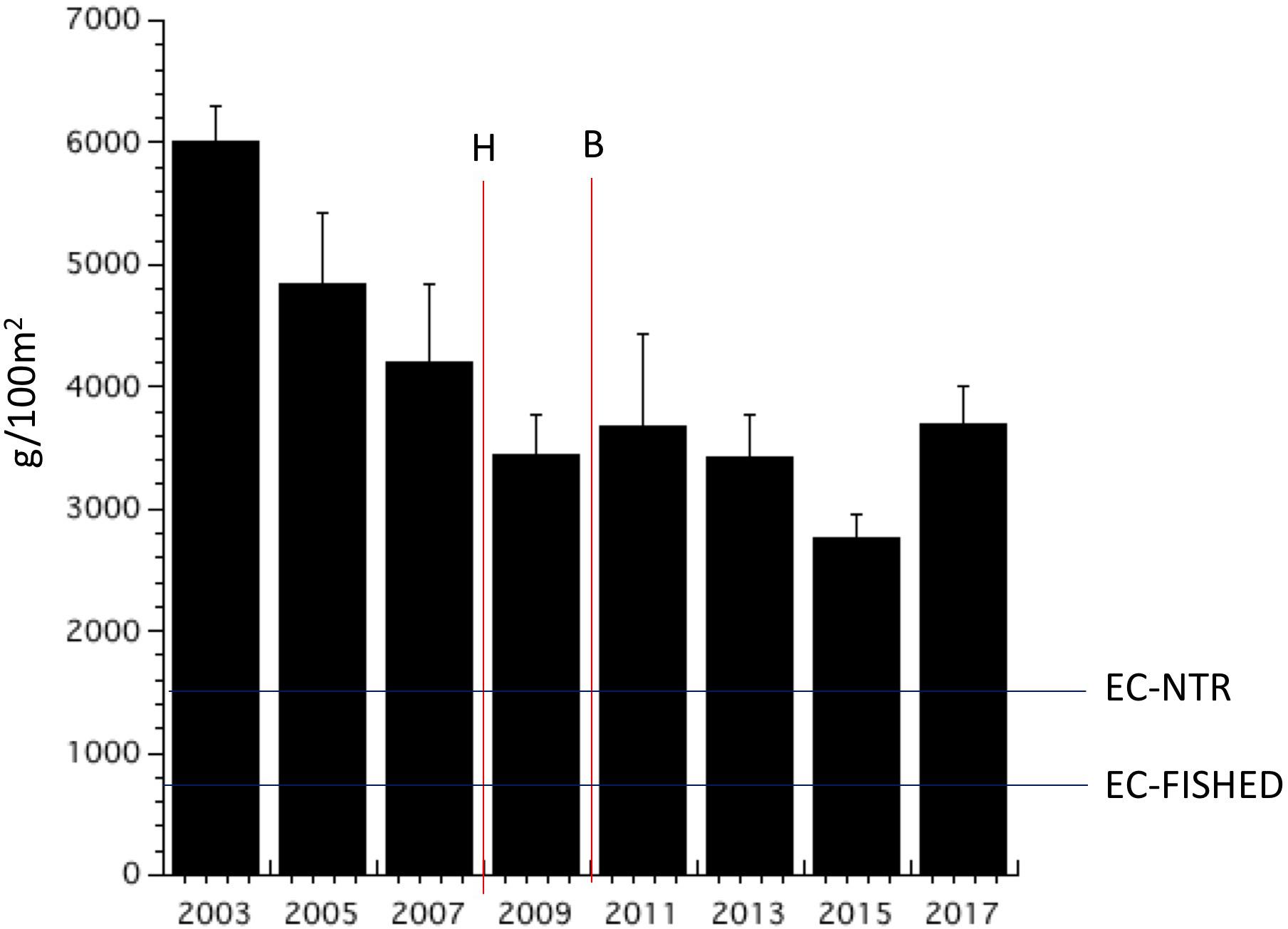
Figure 7. Mean parrotfish biomass from repeated fish transects (+SE). The horizontal lines represent the mean values for the Eastern Caribbean no-take reserves (“EC-NTR”) and fished areas (”EC-Fished”) studied in Steneck et al. (2018).
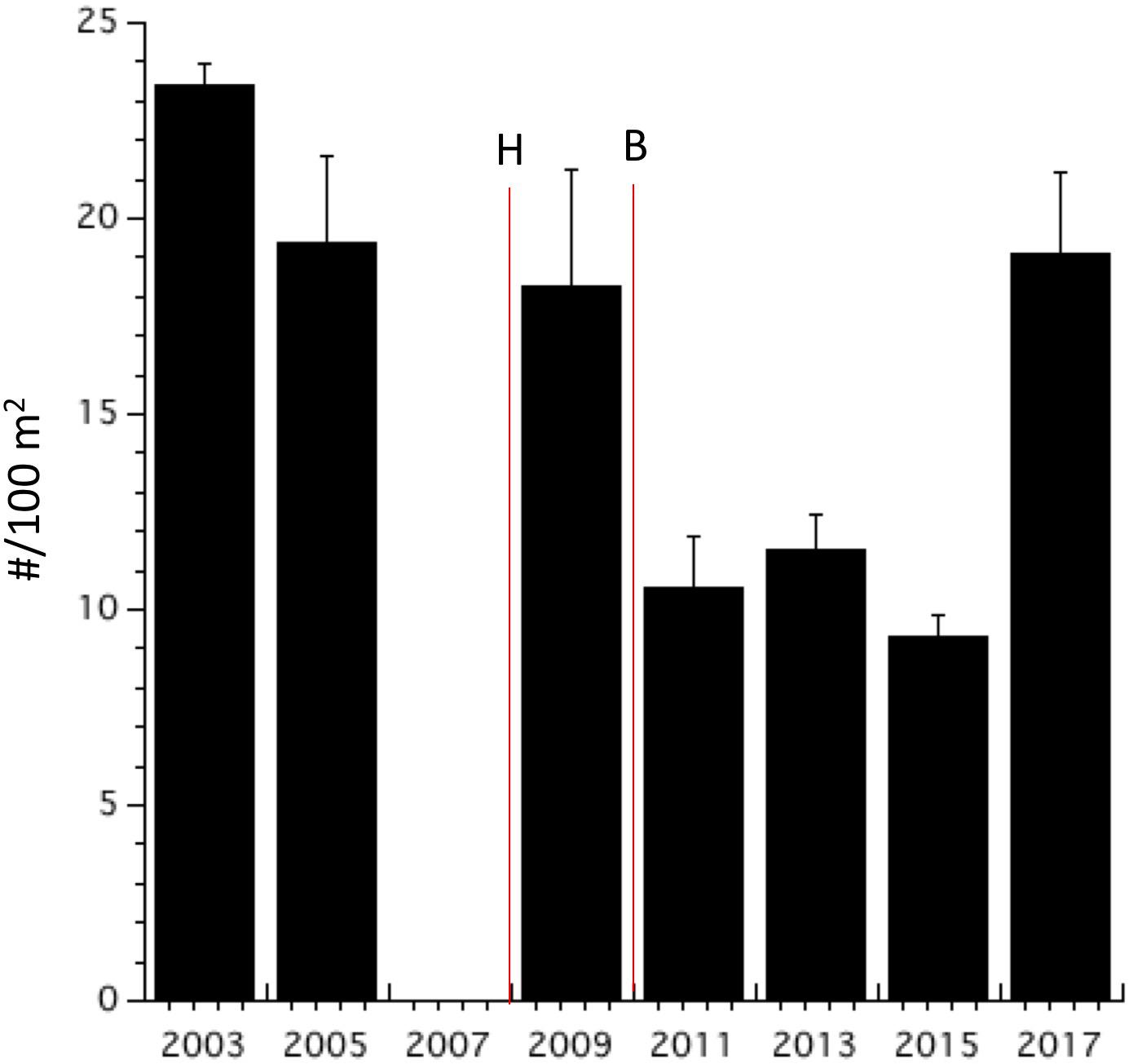
Figure 8. Parrotfish population density (mean ± SE). Notations as in Figure 2.
Benthic Community Structure Over Time
The benthic community structure varied significantly over time and space (p = 0.001; Figure 9A). Specifically, each of the major periods of reef state differed from one another (p = 0.001). The hurricane led to important declines in Orbicella faveolata and O. annularis, though Montastraea cavernosa was able to increase during this period (Figure 9B). Not surprisingly, macroalgal cover and canopy heights also increased following the hurricane. Overall, the impact of the hurricane explained 9% of the total spatio-temporal variance of benthic community structure (Figure 9C). The advent of bleaching post-hurricane led to further changes in the community, characterized by further losses in the two Orbicella species listed as well as a reduction in M. cavernosa and continued increases in macroalgal cover and canopy height. Yet the impact of the bleaching event only explained 4% of the total variance. By 2017 coral cover had recovered to pre-disturbance levels (Figure 2), but the community structure had not yet returned to earlier compositions (p = 0.001, Figure 9A). Indeed, the difference in community structure between pre-disturbance and 2017 accounted for 12% of total spatio-temporal variance (Figure 9C). Looking specifically at the final periods of reef recovery that occurred between the post-bleaching years 2011–2015 and 2017, we found that the change in benthos was characterized by increases in the major framework builders Orbicella and Montastraea with a concomitant decline of macroalgae (Figure 9C). This final period of recovery accounted for 6% of total variance. Note that 46% of all the variance in benthic community structure could not be accounted for in terms of the major disturbance and recovery events.
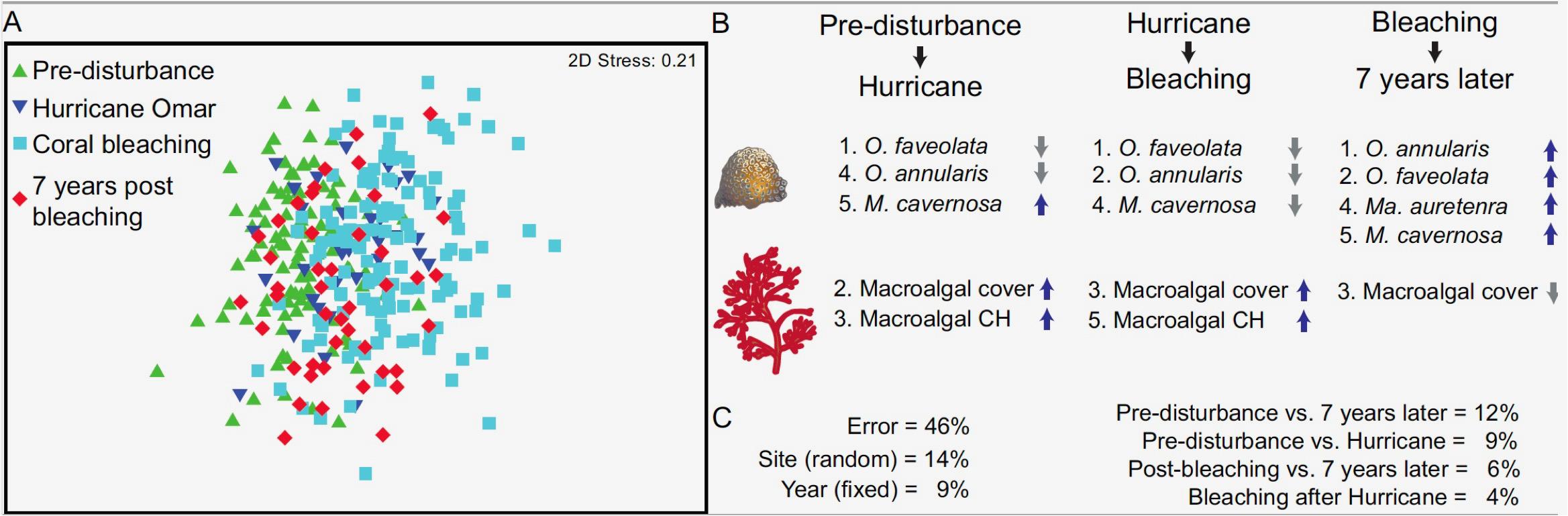
Figure 9. Temporal changes in benthic community structure for the major periods of disturbance and recovery. Visualization is provided using a non-metric MDS (A). The top five most important taxa characterizing benthic changes (B) are ordered in declining sequence (i.e., 1 being most important), and a breakdown of variance components is provided (C) for the total spatio-temporal variability in community structure, including the contrasts.
Discussion
Bonaire’s Resilient Coral Reefs
Bonaire’s coral reefs are characterized by having much higher parrotfish biomass, lower abundance of macroalgae and higher coral cover than most Caribbean coral reefs (Figures 2–10). In 2017, Bonaire’s coral and algal cover were at or better than the Caribbean average from the 1970’s (Figure 10; Jackson et al., 2014). Also, Bonaire’s coral species composition is a throwback to the structure of coral reefs past (with the exception that acroporids remain lacking). For example, while most coral reefs throughout the Caribbean in recent decades have been dominated by “weedy” coral species such as Porites astreoides, P. porities, Agaricia spp., and Siderastrea spp. (Pandolfi and Jackson, 2006; Steneck et al., 2018; Bruno et al., 2019), the dominant corals on Bonaire’s reefs were the massive reef building corals of the past such as Orbicella annularis, O. faveolata, and Montastraea cavernosa (Figure 5). The relatively delayed recovery among the massive reef building Orbicella corals (Figure 6) compared to the rapid recovery in the “weedy” Agaricia sp. (Figure 6) contributed to the low proportion of variance explained post-disturbance (Figure 9). However, all of the massive reef building corals were increasing in coral cover by the most recent 2017 study (Figure 6). In sum, Bonaire’s coral reefs appear to have uniquely resisted the changes that have swept through the Caribbean and have shown the capacity to recover (Figures 2–8). Therefore, Bonaire’s coral reefs are, by definition, resilient - but why?
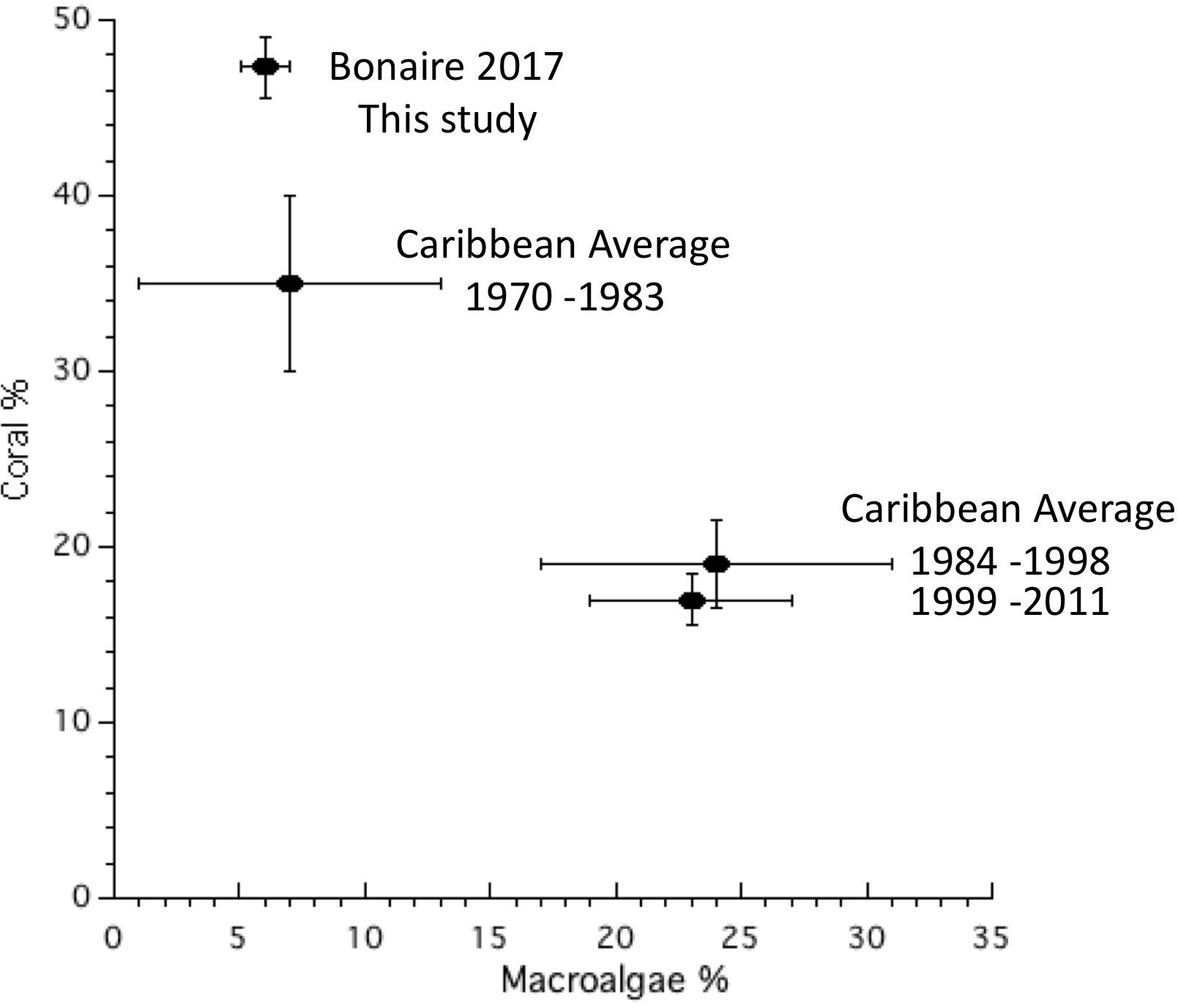
Figure 10. Comparison of mean coral and macroalgal abundance for Bonaire and the rest of the Caribbean between 1970 and 2011 (from Jackson et al., 2014).
What is unique about Bonaire’s reefs and their management? Clearly, factors such as low hurricane frequency in the Southern Caribbean and limited terrestrial runoff due to the island’s low rainfall and low relief have likely contributed to Bonaire’s reef condition. Moreover, the fact that the reefs experience low levels of wave exposure – they occur on the leeward side of the island – also suggests that macroalgal growth rates should be lower than those found in windward systems such as Belize, Florida, and Jamaica (Renken et al., 2010). However, those factors alone have not been enough to confer recovery resilience elsewhere in the Caribbean.
Effective and Sustainable Managed Resilience
The recent history and management of Bonaire’s coral reefs is unique and worthy of specific consideration because social, ecological and economic synergies may have evolved that resulted in uniquely effective but also sustainable coral reef management. Starting in the 1960s, scuba diving on Bonaire’s coral reefs became the island’s biggest tourist draw and economic engine. By 1994, nearly half of the 57,000 tourists who visited the island were scuba divers (De Meyer, 1998). In 1971 the island banned spearfishing (Table 1). We are not aware of any other island in the Caribbean (or elsewhere) having adopted that regulation at that time. The spearfishing ban preceded the 1979 establishment of the Bonaire National Marine Park (BNMP) managed by STINAPA Bonaire. STINAPA Bonaire is a non-government organization founded with the vision that: “nature is recognized and treasured as the main resource of Bonaire’s existence and sustainable development.”3
Over recent decades, Bonaire’s coral reefs became a favored destination within the scuba diving community because the reefs were known to be in relatively good condition and almost all dive sites were accessible from shore. Diving on coral reefs with abundant coral and fishes continues to be the island’s primary tourist draw (Uyarra et al., 2005). This also created a unique funding opportunity because STINAPA Bonaire is a Non-Government Organization (NGO) that began charging a fee for scuba diving in 1992. The user fee funds Bonaire’s National Marine Park management (i.e., >90% of STINAPA Bonaire’s budget is covered by the diving user fee; Solofa, 2017). Analysis of the diving user fee determined it was a good business model for sustainable financing for Bonaire’s marine conservation (Thur, 2010).
Management efforts often fail unless they conform with socioeconomic needs. Several factors contributed to Bonaire’s management success. The early and successful development of the dive and hotel industries became the island’s economic engine. In recent years, most economically important fishing targeted pelagic fish such as mahi mahi, tuna, and wahoo rather than coral reef dwelling fishes (Nenadovic, 2007). The relatively vibrant tourism-driven economy meant that relatively few people in Bonaire depended on fishing coral reef fishes for food.
Managing for Resilience, Monitoring for Trends
Overall, fishing pressure on Bonaire’s coral reefs is relatively low and management actions have actively reduced emerging threats. Traditional shore-based hook and line fishing and regulations that restricted destructive practices such as anchoring and spearfishing had been banned years ago (Nenadovic, 2007). Fish traps, that naturally capture and kill parrotfish (Hawkins et al., 2007), were rarely used in Bonaire, but once they began being used (Nenadovic, 2007) legislation was passed in 2010 to phase them out along with a complete ban on the harvest of parrotfishes (Table 1). The cumulative results of these unique traditions and long-term management practices resulted in Bonaire’s unusually abundant parrotfish, low macroalgal cover, and abundant coral (Figures 2–4, 7–9). However, rather than “grazing intensity [being] sufficiently high that macroalgal blooms are prevented” (Mumby and Steneck, 2008) we found levels of herbivory were sufficient to reverse a small macroalgal bloom over time.
Bonaire’s long duration of reduced fishing pressure on parrotfish likely resulted in the island’s unusually abundant parrotfish populations. This created grazing pressure that could have prevented an earlier phase shift to macroalgae when the herbivorous sea urchin Diadema antillarum suffered a mass mortality throughout the Caribbean (1983-1984; Lessios et al., 1984). During that time, many Caribbean reefs were under intense fishing pressure (for numerous fish species, including parrotfish). Without fish predators or herbivore competitors, Diadema populations expanded to become the primary herbivore on most heavily fished reefs (Hay, 1984). Following the mass mortality of the sea urchin, the heavily fished coral reefs rapidly became macroalgal-dominated (Hughes, 1994; Steneck, 1994). However, there is no record of Bonaire having abundant Diadema or becoming algal dominated after the sea urchin mass mortality event (Van Duyl, 1985). Even if parrotfish had been relatively rare prior to the 1971 ban on spearfishing, more than a decade had passed prior to the sea urchin decline. Since a study in Bermuda determined parrotfish abundances can recover in less than a decade (O’Farrell et al., 2015), it is likely that Bonaire’s parrotfish were sufficiently abundant to have prevented the macroalgal phase shift that swept through most of the rest of the Caribbean in the 1980s.
Knowing the history of an ecosystem can help interpret its current state. Measurements of state variables such as the percent cover of live coral, macroalgae, and the abundance of reef fishes are often taken as indicators of the condition of coral reefs. In fact, based on those variables, Bonaire was identified as one of three coral reefs in the tropical western Atlantic determined to be in “better” condition following a 1-year, Caribbean-wide assessment of coral reefs (Kramer, 2003). Nevertheless state variables, when taken as a “snap shot,” cannot depict the trajectory or resilience potential of a coral reef ecosystem. What is needed is to determine the processes that drive those state variables.
The “two faces of resilience” (Holling, 1996) integrate both the capacity of an ecosystem to resist change or its capacity to recover to its previous state following a disturbance (Gunderson, 2000; Mumby and Steneck, 2011). Management actions generally cannot prevent coral mortality from coral bleaching, hurricanes or disease which means that studies that fail to detect an effect of reserves on coral resistance (Bruno et al., 2019) should not be a surprise. Rather, managed resilience primarily focuses on processes contributing to the recovery of coral reefs. Assuming a reef is not ’starved’ of coral larvae (e.g., Hughes et al., 2019), the fundamental drivers facilitating coral recruitment and regrowth include (but are not limited to) ecological processes that limit algal production.
Numerous studies determined abundant algae impedes or halts coral recruitment (Birkeland, 1977; Arnold et al., 2010; Hughes et al., 2010; Steneck et al., 2014), therefore factors limiting algal biomass are fundamental to the recovery resilience of coral reefs. Herbivory resulting in relatively algal-free Caribbean coral reefs in the 1970s (Figure 10) was maintained by both herbivorous sea urchins and parrotfishes (Hay, 1984). However, following Diadema’s mass mortality in the 1980s, parrotfish became the primary herbivore limiting algae throughout the Caribbean (Mumby, 2006). While today parrotfish is perhaps the most important herbivore group in the Caribbean, that is certainly not the case globally. Thus, there is no applicable circumtropical “parrotfish paradigm” (as asserted by Bruno et al., 2019) because a diversity of other herbivores (including acanthurids; Mumby et al., 2016) and other functional groups of fishes (Bellwood et al., 2006) can limit algae and thus indirectly drive coral reef recovery in tropical Indopacific coral reefs. Further, because the abundance of algae reflects both the rates of production and consumption of algae (Steneck and Dethier, 1994), lower rates of algal-clearing herbivory are necessary on reefs having a lower productivity potential such as tropical Indopacific coral reefs (Roff and Mumby, 2012).
Prior to our study, no systematic monitoring had been conducted on Bonaire’s coral reefs. The mass mortality of the relatively shallow (<10 m) acroporid corals in the 1980s and Hurricane Lenny in 1999 damaged coral reefs both shallower and at locations other than our monitored reefs (Figure 1). The greatest perturbations to Bonaire’s monitored coral reefs occurred between 2008 and 2010, with coral mortality from Hurricane Omar and the coral bleaching event, respectively. These disturbances resulted in a 22% decline in coral cover which is the greatest documented acute decline in Bonaire’s history (Figure 2). When coral dies, it is quickly colonized by benthic algae. Because algae respond most rapidly to the increased surface area created by the recently dead coral, herbivore per area bite rates declined. Over time, as coral cover increased (Figure 2), herbivory may once again become concentrated to pre-disturbance levels (Figure 3). By 2017 we observed a modest increase in parrotfish density and adult and juvenile coral abundances and declining macroalgal abundance (Figures 2–7). Collectively, these trends represent the first example of complete resilience of a coral reef ecosystem in the Caribbean.
Social-Ecological Feedbacks and an Uncertain Future
The stability of alternative states depends on the ecological and social feedbacks that either facilitate or inhibit key drivers of ecosystem structure and function. Experiments that reduced grazing pressure from large parrotfishes resulted in increases in algal abundance and drove coral recruitment to zero (Steneck et al., 2014). Our study suggests that managing to maintain sufficiently high levels of herbivory to control algal abundance can create conditions that facilitate survival and growth in both juvenile and adult corals. We also suggest that socio-economic feedbacks that minimize the need to fish on coral reefs for food contributed to the recovery resilience of this coral reef ecosystem. It is also important that tourists who flock to Bonaire to view healthy reefs pay for the management necessary to keep them in that condition.
While we found complete recovery occurred 8–10 years following climate-driven disturbances, we hasten to point out that both hurricane and coral bleaching events have been increasing in frequency in recent decades. The Southern Caribbean has naturally low hurricane frequency. Prior to Hurricane Lenny in 1999, the last major hurricane to hit Bonaire was in 1877 (De Meyer, 1998), however Hurricane Omar struck 9 years after Lenny. Climate change is certainly increasing the frequency, strength, and size of hurricanes (Knutson et al., 2010), and thus, the frequency of collisions with Bonaire will likely increase as well. Coral bleaching throughout the tropics has been increasing in recent decades (Hughes et al., 2018). Moreover, even in relatively well-managed systems like the Great Barrier Reef, coral recovery rates have been declining in recent decades because of a combination of water quality (MacNeil et al., 2019) and the legacies of major disturbances which have a disproportionate impact on subsequent recovery rates (Ortiz et al., 2018). Therefore, concerns continue that even the best managed coral reefs may be unable to recover in a world growing increasingly hostile to these ecosystems (Hughes et al., 2018). Nevertheless, there is much to learn from the management successes of Bonaire’s coral reefs. They demonstrate that local management today can contribute to the recovery resilience of this endangered ecosystem.
Author Contributions
RS designed the monitoring protocol and drafted the manuscript. PM contributed to concepts and methods to study reef fishes. PM and RS analyzed the data. All coauthors conducted field work, monitoring data, analyses, and final report writing.
Funding
Our research in Bonaire was initially funded by the Pew Fellows for Marine Conservation and later supported by STINAPA Bonaire.
Conflict of Interest Statement
The authors declare that the research was conducted in the absence of any commercial or financial relationships that could be construed as a potential conflict of interest.
Acknowledgments
Specifically, we thank Elsmarie Beukenboom, Kalli De Meyer, Susan Porter, and Fernando Simal for their help in sitting and assisting the monitoring program. Tim McClanahan and Michelle Paddock helped develop the monitoring protocol and fish surveys, respectively. We received considerable assistance from students of the University of Maine’s School of Marine Sciences course “Coral Reefs” (SMS 531). All of their contributions are identified in their reports on the STINAPA Bonaire website (https://stinapabonaire.org/nature/research-reports/coral-reefs-adjacent-waters/). To all we are grateful.
Supplementary Material
The Supplementary Material for this article can be found online at: https://www.frontiersin.org/articles/10.3389/fmars.2019.00265/full#supplementary-material
Footnotes
- ^https://stinapabonaire.org/nature/research-reports/coral-reefs-adjacent-waters/
- ^https://stinapabonaire.org/nature/research-reports/coral-reefs-adjacent-waters/
- ^https://stinapabonaire.org/stinapa/
References
Alemu, J. B., and Clement, Y. (2014). Mass coral bleaching in 2010 in the southern Caribbean. PLoS One 9:e83829. doi: 10.1371/journal.pone.0083829
Anderson, M. J. (2001). A new method for non-parametric multivariate analysis of variance. Austral Ecol. 26, 32–46. doi: 10.1111/j.1442-9993.2001.01070.pp.x
Arnold, S. N., Steneck, R. S., and Mumby, P. J. (2010). Running the gauntlet: inhibitory effects of algal turfs on the processes of coral recruitment. Mar. Ecol. Prog. Ser. 414, 91–105. doi: 10.3354/meps08724
Bellwood, D. R., Hughes, T. P., Folke, C., and Nyström, M. (2004). Confronting the coral reef crisis. Nature 429, 827-833.
Bellwood, D. R., Hughes, T. P., and Hoey, A. S. (2006). Sleeping functional group drives coral-reef recovery. Curr. Biol. 16, 2434–2439. doi: 10.1016/j.cub.2006.10.030
Birkeland, C. (1977). “The importance of rate of biomass accumulation in early successional stages of benthic communities to the survival of coral recruits,” in Proceedings of the Third International Coral Reef Symposium, (Coral Gables, FL: University of miami).
Bozec, Y. M., O’Farrell, S., Bruggemann, J. H., Luckhurst, B. E., and Mumby, P. J. (2016). Tradeoffs between fisheries harvest and the resilience of coral reefs. Proc. Natl. Acad. Sci. 113, 4536–4541. doi: 10.1073/pnas.1601529113
Bruno, J. F., Côté, I. M., and Toth, L. T. (2019). Climate change, coral loss, and the curious case of the parrotfish paradigm: why don’t marine protected areas improve reef resilience? Ann. Rev. Mar. Sci. 11, 307–334. doi: 10.1146/annurev-marine-010318-095300
Bruno, J. F., and Selig, E. R. (2007). Regional decline of coral cover in the Indo-Pacific: timing, extent, and subregional comparisons. PLoS One 2:e711. doi: 10.1371/journal.pone.0000711
Burkepile, D. E., and Hay, M. E. (2008). Herbivore species richness and feeding complementarity affect community structure and function on a coral reef. Proc. Natl. Acad. Sci. 105, 16201–16206. doi: 10.1073/pnas.0801946105
Caselle, J. E., and Warner, R. R. (1996). Variability in recruitment of coral reef fishes: the importance of habitat at two spatial scales. Ecology 77, 2488–2504. doi: 10.2307/2265748
Clarke, K. R. (1993). Non-parametric multivariate analyses of changes in community structure. Austral. J. Ecol. 18, 117–143. doi: 10.1111/j.1442-9993.1993.tb00438.x
De Meyer, K. (1998). CARICOMP—Caribbean coral reef, seagrass and mangrove sites. Coastal Region and Small Island Papers 3. Paris: UNESCO,
Eakin, C. M., Morgan, J. A., Heron, S. F., Smith, T. B., Liu, G., Alvarez-Filip, L., et al. (2010). Caribbean corals in crisis: record thermal stress, bleaching, and mortality in 2005. PLoS One 5:e13969. doi: 10.1371/journal.pone.0013969
Edmunds, P. J., Adam, T. C., Baker, A. C., Doo, S. S., Glynn, P. W., Manzello, D. P., et al. (2019). Why more comparative approaches are required in time-series analyses of coral reef ecosystems. Mar. Ecol. Prog. Ser. 608, 297–306. doi: 10.3354/meps12805
Gardner, T. A., Côté, I. M., Gill, J. A., Grant, A., and Watkinson, A. R. (2003). Long-term region-wide declines in Caribbean corals. Science 301, 958–960. doi: 10.1126/science.1086050
Gunderson, L. H. (2000). Ecological resilience—in theory and application. Ann. Rev. Ecol. System. 31, 425–439. doi: 10.1111/cobi.13321
Hawkins, J. P., Roberts, C. M., Gell, F. R., and Dytham, C. (2007). Effects of trap fishing on reef fish communities. Aquat. Conserv. 17, 111–132. doi: 10.1002/aqc.784
Hay, M. E. (1984). Patterns of fish and urchin grazing on Caribbean coral reefs: are previous results typical? Ecology 65, 446–454. doi: 10.2307/1941407
Holling, C. S. (1973). Resilience and stability of ecological systems. Ann. Rev. Ecol. System. 4, 1–23. doi: 10.1146/annurev.es.04.110173.000245
Holling, C. S. (1996). “Engineering resilience versus ecological resilience. 31 - 44,” in Engineering within ecological constraints. (No. 628 E57e), ed P. Schulze (Washington, DC: National Academic Press).
Hughes, T. P. (1994). Catastrophes, phase shifts, and large-scale degradation of a Caribbean coral reef. Science 265, 1547–1551. doi: 10.1126/science.265.5178.1547
Hughes, T. P., Anderson, K. D., Connolly, S. R., Heron, S. F., Kerry, J. T., Lough, J. M., et al. (2018). Spatial and temporal patterns of mass bleaching of corals in the anthropocene. Science 359, 80–83. doi: 10.1126/science.aan8048
Hughes, T. P., Graham, N. A., Jackson, J. B., Mumby, P. J., and Steneck, R. S. (2010). Rising to the challenge of sustaining coral reef resilience. Trends Ecol. Evol. 25, 633–642. doi: 10.1016/j.tree.2010.07.011
Hughes, T. P., Kerry, J. T., Baird, A. H., Connolly, S. R., Chase, T. J., Dietzel, A., et al. (2019). Global warming impairs stock–recruitment dynamics of corals. Nature 568, 387–390. doi: 10.1038/s41586-019-1081-y
Idjadi, J. A., Lee, S. C., Bruno, J. F., Precht, W. F., Allen-Requa, L., and Edmunds, P. J. (2006). Rapid phase-shift reversal on a Jamaican coral reef. Coral reefs 25, 209–211. doi: 10.1007/s00338-006-0088-7
Jackson, J. B. C., Donovan, M. K., Cramer, K. L., and Lam, V. V. (eds) (2014). Status and trends of Caribbean coral reefs: 1970-2012. Washington, D. C: Global Coral Reef Monitoring Network. (accessed May 1, 2019).
Knutson, T. R., McBride, J. L., Chan, J., Emanuel, K., Holland, G., Landsea, C., et al. (2010). Tropical cyclones and climate change. Nat. Geosci. 3, 157–163.
Kramer, P. A. (2003). Synthesis of coral reef health indicators for the western atlantic: results of the AGRRA program (1997-2000). Atoll Res. Bull. 496, 1–57. doi: 10.5479/si.00775630.496-3.1
Lang, J. C. (ed.). (2003). Status of Coral Reefs in the Western Atlantic: results of Initial Surveys, Atlantic and Gulf Rapid Reef Assessment (AGRRA) Program. Washington, DC: Smithsonian Institution.
Lessios, H. A., Robertson, D. R., and Cubit, J. D. (1984). Spread of Diadema mass mortality through the Caribbean. Science 226, 335–337. doi: 10.1126/science.226.4672.335
Lewis, S. M. (1986). The role of herbivorous fishes in the organization of a Caribbean reef community. Ecol. Monogr. 56, 183–200. doi: 10.2307/2937073
MacNeil, M. A., Mellin, C., Matthews, S., Wolff, N. H., McClanahan, T. R., Devlin, M., et al. (2019). Water quality mediates resilience on the Great Barrier Reef. Nat. Ecol. Evol. 3, 620–627. doi: 10.1038/s41559-019-0832-3
Mumby, P. J. (2006). The impact of exploiting grazers (Scaridae) on the dynamics of Caribbean coral reefs. Ecol. Appl. 16, 747–769. doi: 10.1890/1051-0761 (2006)016[0747:TIOEGS]2.0.CO;2
Mumby, P. J., Dahlgren, C. P., Harborne, A. R., Kappel, C. V., Micheli, F., Brumbaugh, D. R., et al. (2006). Fishing, trophic cascades, and the process of grazing on coral reefs. Science 311, 98–101. doi: 10.1126/science.1121129
Mumby, P. J., and Harborne, A. R. (2010). Marine reserves enhance the recovery of corals on Caribbean reefs. PLoS One 5:e8657. doi: 10.1371/journal.pone.0008657
Mumby, P. J., and Steneck, R. S. (2008). Coral reef management and conservation in light of rapidly evolving ecological paradigms. Trends Ecol. Evol. 23, 555–563. doi: 10.1016/j.tree.2008.06.011
Mumby, P. J., and Steneck, R. S. (2011). “The resilience of coral reefs and its implications for reef management,” in Coral Reefs: An Ecosystem in Transition, eds Z. Dubinsky and N. Stambler (Dordrecht: Springer Press).
Mumby, P. J., Steneck, R. S., Adjeroud, M., and Arnold, S. N. (2016). High resilience masks underlying sensitivity to algal phase shifts of Pacific coral reefs. Oikos 125, 644–655. doi: 10.1111/oik.02673
Nenadovic, M. (2007). “The impact of traditional fishing practices on the abundance of major herbivorous fish species in Bonaire, Netherlands Antilles,” in A report on the status of the coral reefs of Bonaire in 2007 with results from monitoring 2003 - 2007, eds R. S. Steneck, P. Mumby, andS. Arnold. Available at: https://stinapabonaire.org/wp-content/uploads/2018/08/2007_Steneck-et-al_-A-Report-on-the-Status-of-the-Coral-Reefs-of-Bonaire-in-2007-With-Results-from-Monitoring-2003-2007.pdf (accessed May 29, 2019).
Nyström, M., Graham, N. A. J., Lokrantz, J., and Norström, A. V. (2008). Capturing the cornerstones of coral reef resilience: linking theory to practice. Coral Reefs 27, 795–809. doi: 10.1007/s00338-008-0426-z
O’Farrell, S., Harborne, A. R., Bozec, Y.-M., Luckhurst, B. E., and Mumby, P. J. (2015). Protection of functionally important parrotfishes increases their biomass but fails to deliver enhanced recruitment. Mar. Ecol. Prog. Ser. 522, 245–254. doi: 10.3354/meps11134
Ortiz, J. C., Wolff, N. H., Anthony, K. R., Devlin, M., Lewis, S., and Mumby, P. J. (2018). Impaired recovery of the Great Barrier Reef under cumulative stress. Sci. Adv. 4:eaar6127. doi: 10.1126/sciadv.aar6127
Pandolfi, J. M., and Jackson, J. B. (2006). Ecological persistence interrupted in Caribbean coral reefs. Ecol. Lett. 9, 818–826. doi: 10.1111/j.1461-0248.2006.00933.x
Pandolfi, J. M., Jackson, J. B., Baron, N., Bradbury, R. H., Guzman, H. M., Hughes, T. P., et al. (2005). Are US coral reefs on the slippery slope to slime? Science 307, 1725–1726. doi: 10.1126/science.1104258
Rasher, D. B., and Hay, M. E. (2010). Chemically rich seaweeds poison corals when not controlled by herbivores. Proc. Natl. Acad. Sci. 107, 9683–9688. doi: 10.1073/pnas.0912095107
Renken, H., Mumby, P. J., Matsikis, I., and Edwards, H. J. (2010). Effects of physical environmental conditions on the patch dynamics of Dictyota pulchella and Lobophora variegata on Caribbean coral reefs. Mar. Ecol. Prog. Ser. 403, 63–74. doi: 10.3354/meps08441
Roff, G., and Mumby, P. J. (2012). Global disparity in the resilience of coral reefs. Trends Ecol. Evol. 27, 404–413. doi: 10.1016/j.tree.2012.04.007
Solofa, A. (2017). “History and status of management and governance of Bonaire national marine park,” in Status and Trends of Bonaire’s Reefs in 2017, eds R. S. Steneck and M. Wilson (Orono, ME: University of Maine). (accessed May 1, 2019).
Steneck, R. S. (1994). “Is herbivore loss more damaging to reefs than hurricanes? Case studies from two Caribbean reef systems (1978–1988),” in Proceedings of the Colloquium on Global Aspects of Coral Reefs: Health, Hazards, and History, (Silver Spring, MD: NOAA).
Steneck, R. S., Arnold, S. N., and Mumby, P. J. (2014). Experiment mimics fishing on parrotfish: insights on coral reef recovery and alternative attractors. Mar. Ecol. Prog. Ser. 506, 115–127. doi: 10.3354/meps10764
Steneck, R. S., and Dethier, M. N. (1994). A functional group approach to the structure of algal-dominated communities. Oikos 69, 476–498.
Steneck, R. S., Mumby, P. J., MacDonald, C., Rasher, D. B., and Stoyle, G. (2018). Attenuating effects of ecosystem management on coral reefs. Sci. Adv. 4:eaao5493. doi: 10.1126/sciadv.aao5493
Thur, S. M. (2010). User fees as sustainable financing mechanisms for marine protected areas: an application to the bonaire national marine park. Mar. Policy 34, 63–69. doi: 10.1016/j.marpol.2009.04.008
Uyarra, M. C., Cote, I. M., Gill, J. A., Tinch, R. R., Viner, D., and Watkinson, A. R. (2005). Island-specific preferences of tourists for environmental features: implications of climate change for tourism-dependent states. Environ. Conserv. 32, 11–19. doi: 10.1017/s0376892904001808
Van Duyl, F. C. (1985). Atlas of the Living Reefs of Curacao and Bonaire (Netherlands Antilles). Amsterdam: Natuurwetenschappelijke studiekring voor suriname en de Nederlandse antillen.
Keywords: Bonaire (Dutch Antilles), coral reefs, coral bleaching, hurricanes, managed resilience, Caribbean, herbivory, resilience
Citation: Steneck RS, Arnold SN, Boenish R, de León R, Mumby PJ, Rasher DB and Wilson MW (2019) Managing Recovery Resilience in Coral Reefs Against Climate-Induced Bleaching and Hurricanes: A 15 Year Case Study From Bonaire, Dutch Caribbean. Front. Mar. Sci. 6:265. doi: 10.3389/fmars.2019.00265
Received: 29 January 2019; Accepted: 03 May 2019;
Published: 07 June 2019.
Edited by:
Nancy Knowlton, Smithsonian Institution, United StatesReviewed by:
Kevin Alexander Hovel, San Diego State University, United StatesAndrew M. Fischer, University of Tasmania, Australia
Copyright © 2019 Steneck, Arnold, Boenish, de León, Mumby, Rasher and Wilson. This is an open-access article distributed under the terms of the Creative Commons Attribution License (CC BY). The use, distribution or reproduction in other forums is permitted, provided the original author(s) and the copyright owner(s) are credited and that the original publication in this journal is cited, in accordance with accepted academic practice. No use, distribution or reproduction is permitted which does not comply with these terms.
*Correspondence: Robert S. Steneck, steneck@maine.edu