Multi-Variate Analyses of Coral Mortality From the 2014–2015 Stony Coral Tissue Loss Disease Outbreak Off Miami-Dade County, Florida
- 1Marine Science Center, Northeastern University, Nahant, MA, United States
- 2Marine and Coastal Programs, Dial Cordy and Associates, Inc., Miami, FL, United States
Environmental compliance monitoring associated with the Port Miami dredging project (2013–2015), designed to assess the impact of project-generated sediments on the local coral community, fortuitously captured a thermal bleaching event and the first reports of an emergent, highly contagious, white-plague-like coral disease outbreak in the fall of 2014. The disease, now termed stony coral tissue loss disease (SCTLD), has decimated reefs throughout Florida and is now spreading across the Caribbean. The high prevalence of disease, the number of affected species, and the high mortality of corals affected suggests SCTLD may be the most lethal coral disease ever recorded. Previous analyses of the dredge monitoring data have reached mixed conclusions about the relative impact of dredging on coral mortality and has often parsed out disease susceptible individuals to isolate the impacts of dredging only. We use multi-variate analyses, including time-based survival analyses, to examine the timing and impacts of dredging, coral bleaching, and disease on local coral mortality. By examining the status of corals monthly from the October 2013 to July 2015 observational period, we found that coral mortality was not significantly affected by a coral’s proximity to the dredge site or sediment burial. Instead, coral mortality was most strongly impacted by disease and the emergence of SCTLD during the monitoring period. During the 2-year monitoring period, 26.3% of the monitored corals died, but the only conditions significantly affected by the dredge were partial burial and partial mortality. The strongest link to mortality was due to disease, which impacted coral species differently depending on their susceptibility to SCTLD. The focus on disturbances associated with dredging created a circumstance where the greater impacts of this emergent disease were downplayed, leading to a false narrative of the resulting mortality on the local coral communities. The results of this study reveal that while local events such as a dredging project do have quantifiable effects and can be harmful to corals, regional and global threats that result in mass coral mortality such as thermal stress and disease represent an existential threat to coral reefs and must be urgently addressed.
Introduction
In November of 2013, the Miami Harbor Phase III Deepening Project began with the goal of making Port Miami the first of four eastern seaboard locations in the United States to allow access to larger post-Panamax class vessels. The Port Miami project constituted the largest dredging project conducted in the region for more than a decade (US Army Corps of Engineers, 2021). Maintenance dredging activities began on November 20, 2013, with cutter-head dredge rock removal operations commencing on December 17, 2013. Dredging of the offshore entrance channel was completed on March 26, 2015 (Dial Cordy and Associates Inc, 2015a,b). The existing entrance channel to Port Miami was deepened from 12.8 to 15.2 m and removed approximately five million cubic yards of limestone rock and sand (US Army Corps of Engineers, 2021). The Florida Department Environmental Protection (2012) mandated compliance monitoring of surrounding coral communities and was designed to quantify the impact of local dredging stress associated with the Port Miami expansion project between October 2013 and July 2015. In addition to estimating the impacts of local dredging sediments on corals during the 21-month monitoring period (Dial Cordy and Associates Inc, 2015a,b), the monitoring program happened to captured two key events: (1) a regional thermal anomaly in the summer of 2014 that resulted in the worst coral bleaching episode recorded in Florida since 1997–1998 (Kennedy, 2014; National Oceanic Atmospheric Administration, 2014, 2015a; Gilliam et al., 2015, 2016; Manzello, 2015; Lewis et al., 2017; Walton et al., 2018; Neely et al., 2021a), and (2) the appearance of a white plague-like disease outbreak at the southernmost Port Miami far-field control monitoring sites in September and October of 2014 (see Dial Cordy and Associates Inc, 2015a,b; Precht et al., 2016, 2019; Precht, 2021). This was the first reported case of a new emergent coral disease epizootic now called stony coral tissue loss disease (SCTLD; Florida Coral Disease Response Research, and Epidemiology Team, 2018). This highly contagious, water-borne epizootic would eventually spread across the entire Florida reef tract (Precht et al., 2016; Aeby et al., 2017; Hayes et al., 2017; Lunz et al., 2017; Neely, 2018; Ruzicka, 2018; Walton et al., 2018; Dobbelaere et al., 2020; Muller et al., 2020; Sharp et al., 2020; Williams et al., 2021) and into the Bahamas and greater Caribbean (Alvarez-Filip et al., 2019; Department of Parks and Natural Resources, USVI, 2019; Martin, 2019; Talbot, 2019; Estrada-Saldívar et al., 2020; Dahlgren et al., 2021; Heres et al., 2021; summarized in AGRRA, 2021), ultimately resulting in this special Research Topic in Frontiers in Marine Science dedicated to the etiology, epidemiology, spread, impacts, and potential solutions (interventions) of the SCTLD crisis1.
To date, at least three separate research groups (Miller et al., 2016; Cunning et al., 2019; Gintert et al., 2019; Precht et al., 2019; Precht, 2021) have analyzed the publicly available monitoring data from the Port Miami dredging project, with the goal of disentangling the impacts of dredging on the surrounding coral communities from other factors like coral thermal bleaching and the outbreak of SCTLD. In all, some 643 corals were initially tagged at 26 sites for repeated measures monitoring (see section “Materials and Methods”). Of these, 162 (25.1%) of the corals died, and 28 (4.4%) others disappeared during the 21-month Port of Miami monitoring program. Removing the “lost” corals from the data set resulted in a total mortality of 26.3% of the remaining tagged colonies.
While the consensus view is that the monitored corals were negatively impacted by a combination of local, regional, and global stressors during the observational period (Dial Cordy and Associates Inc, 2015a,b, 2017; Miller et al., 2016; Precht et al., 2016, 2019; Cunning et al., 2019; Gintert et al., 2019; Precht, 2021), there continues to be considerable debate surrounding the relative impacts of local sediments caused by the Port Miami Dredge project (Miller et al., 2016; National Marine Fisheries Service, 2016; Cunning et al., 2019) vs. mortality associated with a regional thermal bleaching event in 2014 and the emergence of the SCTLD outbreak in late 2014 and early 2015 across the surveyed sites (Gintert et al., 2019; Precht et al., 2019). For example, Cunning et al. (2019) focused mainly on the effects of the dredge and only analyzed coral species that were not observed with disease during monitoring. Cunning et al. (2019) documented a significant effect of dredging on the partial burial and partial mortality of corals in the dredge monitoring program that were not infected by SCTLD. Miller et al. (2016) analyzed a small subset of the sites (only four of the 26 sites) and detected a far greater impact of mortality at two channel-side sites adjacent to the dredge operations as compared to their paired, far-field controls. Miller et al. (2016) attributed a twofold increase in mortality to the exacerbation of disease-related mortality resulting directly from sediment stress associated with dredge operations. Gintert et al. (2019) focused mainly on the impacts of SCTLD and concluded that 93.3% of mortality was attributed to disease, whereas dredge related stress only attributed to 6.7% of coral mortality.
In this study, rather than trying to exclude or isolate the impacts of the onset of the SCTLD outbreak from dredging impacts, we treat the SCTLD outbreak as a seminal event and use multi-variate analyses of the full 615 tagged coral dataset, including time-based survival analyses, to examine the combined and relative impacts of dredging, thermally induced coral bleaching, and SCTLD on coral mortality over the course of the 21-month Port Miami coral monitoring project. In addition to examining the effects of dredging on the burial, partial burial, mortality, and partial mortality of individual coral colonies, we focus on the direct impacts of bleaching and disease, examining for the first time the species-specific effects of the emerging SCTLD outbreak on coral mortality at its onset during the Port Miami monitoring project. This is especially important because the susceptibility (and survivorship) of corals to SCTLD varies significantly by species with some species being highly susceptible, while other species showed only moderate impacts, while yet others showed little or no impacts during the 21-month monitoring period (Gintert et al., 2019; Precht et al., 2019; see also Precht et al., 2016). Lastly, we explore the correlations between burial, bleaching, disease, and mortality across the nine most abundant coral species in the surveyed data set and show that the SCTLD disease prevalence was strongly and significantly correlated (R = 0.9, p = 0.0011) with coral mortality during the monitoring study at both the channel-side and far-field control sites.
Materials and Methods
Study Area
This Port Miami monitoring study was undertaken in southeastern Florida (Figure 1), off Miami-Dade County, which lies at the northern extent of reef growth along the North American continent (Precht and Miller, 2007). Cool winter sea-surface temperatures (SST’s) have most often been cited as the limiting factor for coral growth in this area (Vaughan, 1914, 1918; Ginsburg, 1956; Burns, 1985; Precht and Miller, 2007; Toth et al., 2021). Historically, Fowey Rocks off southern Key Biscayne (Cape Florida) was considered the northern extent of true modern reef growth in southeast Florida and is the northernmost reef of the Florida Reef Tract (Vaughan, 1914; Smith, 1943; Marszalek et al., 1977; Jaap, 1984; Precht and Miller, 2007; Toth et al., 2021). North of Fowey Rocks are a series of submerged, shore-parallel, relict reef terraces. These terraces are the remnants of a nearly continuous, 150-km-long, barrier-reef system that extended northward from Miami to Palm Beach County in the early to middle Holocene (Lighty et al., 1978; Precht and Aronson, 2004; Stathakopoulos and Riegl, 2015; Toth et al., 2021). In Miami-Dade County, the two main Holocene-age terraces are referred to as the inner and outer reefs while closer to shore a nearshore hardbottom is present and is locally known as the nearshore ridge complex (NRC). The NRC is comprised of Pleistocene age, fossilized coquina beach deposits of the Miami Limestone and Anatasia Formations (see Walker, 2012).
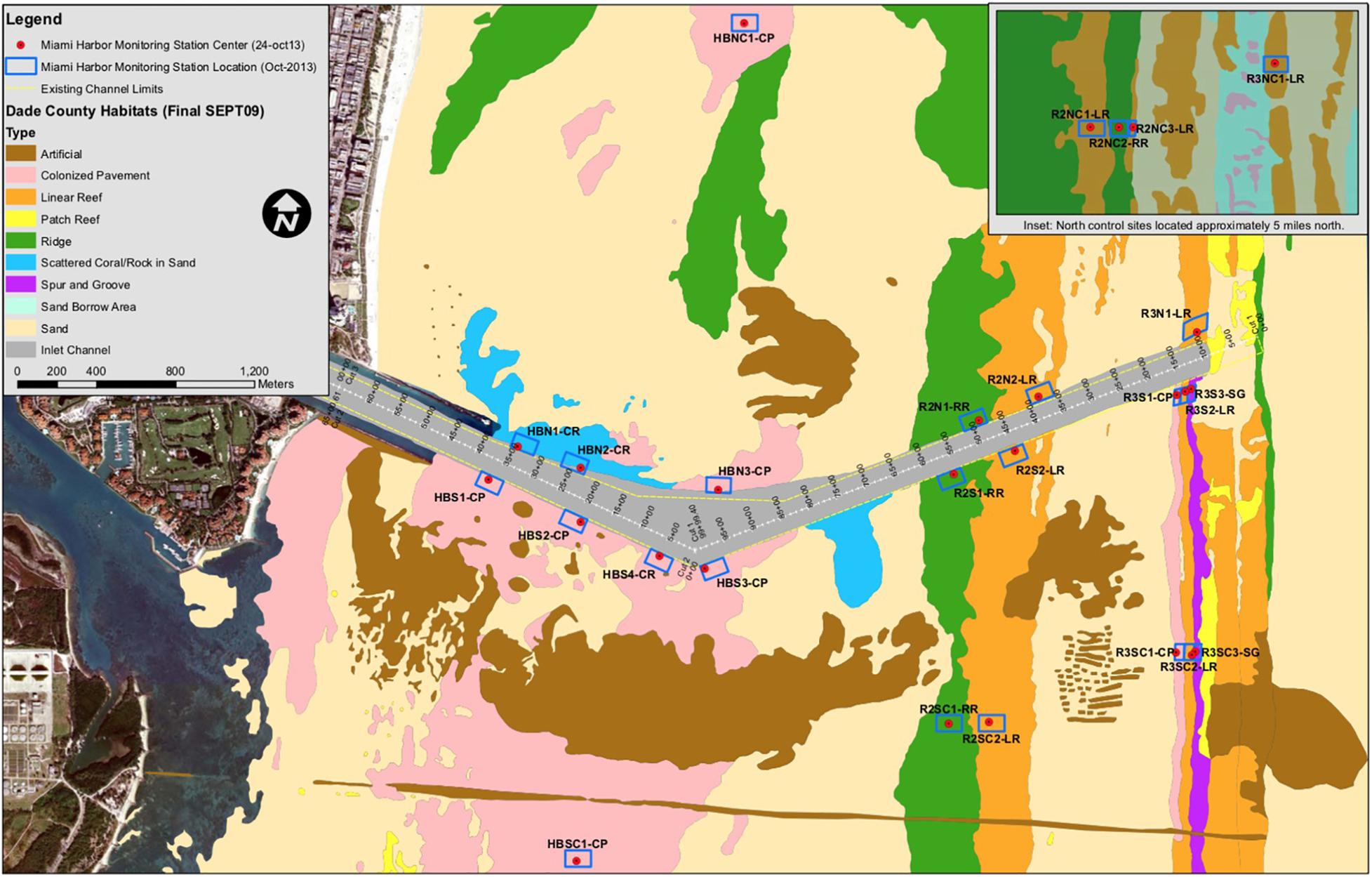
Figure 1. Location map of 26 monitoring sites (blue boxes) associated with the Port Miami Deepening Project. Site abbreviations are noted next to each box. Brown horizontal line across the lower portion of the figure is the location of the outfall pipe trench for the Central Miami-Dade Wastewater Treatment Plant.
Modern-day assemblages of stony corals, octocorals, and sponges have colonized the surface of these relict reefs and nearshore hardbottom habitats and those stony corals are the focus of this study. These coral assemblages are highly ephemeral and characterized by their small overall size, low species richness and diversity, and low stony coral cover (Goldberg, 1973; Marszalek, 1982; Jaap, 1984; Blair and Flynn, 1989; Precht et al., 2016; Gilliam et al., 2018). Small-scale latitudinal differences in species cover and composition (diversity and richness) have also been noted in Miami-Dade County, with an overall reduction in coral diversity and cover from south to north (Jaap, 1984; Blair and Flynn, 1989; Dial Cordy and Associates Inc, 2010, 2012).
Coral Condition Monitoring
Environmental compliance monitoring associated with the Florida Department of Environmental Protection (FDEP) mandated construction permit for the Port Miami Phase III Deepening Project (FDEP Permit No. 0305721-001-BI) required up to twice-weekly monitoring of 26 monitoring stations established within Miami-Dade County when dredging was occurring (Figure 1). This dataset represents one of the largest and most complete benthic monitoring datasets related to the environmental compliance of a dredging project (see also Stoddart et al., 2019 for a similar monitoring program performed in Western Australia). The primary goal of the Port Miami monitoring program established by the Florida Department Environmental Protection, 2012 was to “detect natural variation in the resources and to assist in determining the effects of the actual dredge operations on the resources surrounding the project area.” A critical component of the program was repeated measures monitoring of the 26 sites to examine the causes of coral stress and mortality experienced during construction and post-construction periods and, in the case of the SCTLD epizootic, it fortuitously allowed us to evaluate if the disease was initiated or exacerbated by the on-going dredging activities.
Observational data used in this study was collected both north and south of the entrance for the main shipping channel of the Port Miami over a 21-month period between October 2013 through July 2015. Of the 26 permanent monitoring sites, 15 were located at dredge-adjacent channel-side locations and 11 at far-field controls. Of the 15 dredge-adjacent sites, seven were in nearshore hardbottom habitats (code – HB), four in inner reef habitats (code – R2), and four in outer reef habitats (code – R3). Each monitoring station was established as part of a before-and-after-impact experimental design in which dredge-adjacent sites were paired with far-field control sites within the same habitat and located on the same side (north or south) of the Port Miami entrance channel (Florida Department Environmental Protection, 2012; Figure 1). Each site was named to reflect the reef type, direction, and whether it was a channel-side or control site; for example, R2NC1 represents an inner reef habitat (R2) control site located north of the dredge channel (NC) with the end number representing the site number within that habitat (Figure 1).
In all, 643 individually tagged corals were initially monitored, in situ, for coral condition. These corals were scored for burial (full or partial), bleaching, disease, and other metrics of coral health (predation, competition, physical abrasion, mucus production, polyp expansion, or contraction, etc.). Data collection and methods are described in detail in Gintert et al. (2019; see also Dial Cordy and Associates Inc, 2015a,b) and summarized here. Monitoring began on October 23, 2013, approximately 1 month before the initiation of dredging activity on November 20th and concluded in July 2015, 1-month following the cessation of all construction activities. In addition, these same sites were re-surveyed in the late summer and fall of 2016, approximately 1-year after the project had been completed to see if there were any residual or continuing impacts related to the project (Dial Cordy and Associates Inc, 2017). These 2016 data were not included in this analysis but are briefly reviewed in the Discussion. We intentionally avoided the 2016 one-off survey so our data would not be confounded by other natural events that transpired in the year after the cessation of dredging activities (such as a second major coral bleaching event observed in the summer of 2015; National Oceanic Atmospheric Administration, 2015b; Jones et al., 2021; Neely et al., 2021a), instead focusing on causes of coral stress and mortality that occurred immediately before, during, and after the Port Miami project when monitoring was occurring on a regular basis.
At each of the 26 sites, three 20-m long permanent transects were established (n = 78 transects in all) and oriented from north to south. The transects were laid parallel to each other and were spaced at least 5 m apart. Up to ten (10) stony corals were tagged within one meter of each transect at each site (n = 643 tagged corals). In situ coral condition and stress indicators were recorded for each of the tagged corals and corresponding still photographs were taken in planar view during each survey by trained scientific divers (see Gintert et al., 2019). In some cases, additional oblique angle and close-up photographs were taken of a specific stress indicator or an unknown condition for follow-up desk-top analysis, and interpretation.
Coral surveys were triggered whenever the active dredging operations occurred within 750 m of any of the channel-side sites in any given week. Underwater surveys were not performed when dive safety or weather conditions prevented access to the sites. Each of the tagged corals were visited and had their conditions recorded approximately 40 times each between October 2013 to July 2015, with some exceptions; site HBN1 (hardbottom habitat, north of dredge channel and closest to shore) was buried seasonally during winter and spring of both years and recorded as “buried” during these seasonal events (Precht et al., 2021). In addition, 28 tagged corals during the 2013–2015 observation period became dislodged and lost. A combination of natural bio-erosion, anchor damage, abandoned fishing gear, lobster trap lines, and storm effects were the likely causes of the displacement and loss of these tagged corals (Dial Cordy and Associates Inc, 2017). Similar observations of “missing” corals have been documented annually in the Southeast Florida Coral Reef Monitoring Program (Gilliam, 2012). The 28 missing corals were removed from our analysis leaving a total of 615 tagged coral colonies in the final 2015 dataset. During the 21-month monitoring program more than 25,000 in situ coral observations were recorded and compared with ∼75,000 corresponding still photographs for cross-verification and validation. Prior to entering the validated field data into a project-specific database, the field forms received a final desk-top review for completeness and accuracy. This analysis was performed weekly as part of the quality control and assurance program established for the project (Gintert et al., 2019). During active dredging, weekly reports were submitted to the agencies describing survey results of coral condition at each site monitored and included statistical comparisons between stations and their paired controls (Dial Cordy and Associates Inc, 2015a,b, 2017).
Statistical Analysis
Based upon the required protocol, coral monitoring essentially followed the path of the dredge; the condition of the corals at each of the 26 sites were recorded at least once a month resulting in a pooled condition score for each of the 615 tagged corals over the 21-month monitoring period; we simplified these conditions into six different codes (dead, partially dead, bleached, diseased, buried, or partially buried). Complete mortality represents “death of entire colony; no live tissue remaining on the skeleton” (Dial Cordy and Associates Inc, 2015a,b). Partial mortality at the time of monitoring was described as “colony appears white with no live polyps visible. Generally, occurs around the margin of the colony” (Dial Cordy and Associates Inc, 2015a,b). For this study, we were interested primarily in the code recorded for white-plague disease (WPD), which was the term used for all “white” diseases observed on non-acroporid corals in our data set as the case description for SCTLD was not developed until October 2018 (see Florida Coral Disease Response Research, and Epidemiology Team, 2018). Occurrences of WPD-like symptoms in the initial data were recorded as “diseased” in our data. During monitoring WPD/SCTLD was described as “[W]hite lines or bands of recently dead coral tissue affecting non-acroporid corals” (Dial Cordy and Associates Inc, 2015a,b; see also Gintert et al., 2019). Three different levels of bleaching stress were recorded during monitoring (i.e., paling, partial-bleaching, and bleaching). Because 89.0% of all tagged corals showed some level of bleaching stress during the monitoring program, for this study we only focused on corals that were recorded as “bleaching”; these individuals were described as having “[L]ive tissue with complete loss of color across the entire colony” (Dial Cordy and Associates Inc, 2015a,b). Four levels of sediment indicators were recorded in the original monitoring data set (i.e., sediment, sediment accumulation, partial burial, and burial). As with the bleaching codes, because most corals showed some level of sediment exposure during the monitoring program (both natural and dredge induced), in this study only partial burial and full burial were used. Partial burial was described as “portion(s) of the colony buried by sediment” (Dial Cordy and Associates Inc, 2015a,b). Full burial was recorded if the “entire colony buried by sediment” (Dial Cordy and Associates Inc, 2015a,b).
Negative binomial generalized linear mixed effect models (GLMs) were first used to examine the fixed effects of channel (dredging channel vs. channel control), habitat (nearshore, inner or outer reef) and direction (north vs. south) and random effects of transect and species [glmer model: dependent variable ∼ Dredge × Habitat × Direction + (1| Transect + (1| Species)] and run independently for each of the six dependent variables (conditions; i.e., mortality, partial mortality, burial, partial burial, disease, and bleaching; after Cunning et al., 2019) in R using the “lme4” package (Bates et al., 2007) and “bobyqa” optimizer (when necessary) to improve efficiency of the models (Chung et al., 2013). In cases where the full glmer model failed to converge, we report the simplified model in our results. Type-2 ANOVA summaries using the “Car” package (Fox and Weisberg, 2018) were used to calculate chi-squared and p-values for the main effects and interactions. Estimated marginal means (emmeans) post hoc comparisons were used to further compare any significant main (i.e., fixed) effects in the model using the “emmeans” package (Searle et al., 1980) and then directly compared using the “contrast” function using the “pairwise” method.
Survival analyses were also performed to take advantage of the monthly census data on mortality patterns over the 21-month monitoring period using the R package “Survival” (Therneau and Lumley, 2021). We first used Cox regression model [coxph(Surv(months, census) ∼ Channel + Habitat + Dir, data = df_all)] to determine if the main effects of Channel, Habitat, and Direction had a significant impact on coral mortality over the census period across all 615 tagged corals. Significance was determined using ANOVA type 2 tests using the R package “Car” (Fox and Weisberg, 2018) and the results of the Cox proportional hazard ratio (HR) models were plotted using ggforest, a function of the R package “survminer” (Kassambara et al., 2021). Kaplan–Meier survival plots were then used to visualize the significant main effects on coral survival over time.
Because some coral species are better represented in the dataset than others, we then ran survival analyses focused on the top nine species with the highest total abundances in the survey; this subset included the species Pseudodiploria clivosa (PCLI), Dichocoenia stokesi (DSTO), Pseudodiploria strigosa (PSTR), Montastrea cavernosa (MCAV), Meandrina meandrites (MMEA), Porites astreoides (PAST), Solenastrea bournoni (SBOU), Stephanocoenia intercepta (SINT), and Siderastrea siderea (SSID). This allowed us to incorporate species as a main effect into our Cox regression models, Cox proportional HRs, and Kaplan–Meier survivorship plots to examine the effect of species, channel, habitat, and direction on coral mortality. One of the top nine most abundant species – the coral species SINT – did not experience any mortality during the monitoring period and thus was removed from the survival analyses because of convergence issues. The four-letter abbreviations for coral species in our data set follow the acronym naming protocol in AGRRA (2013) as those were the codes that were used during the collection of field data during the Port Miami project. Subsequently, however, AGRRA (2016) changed some codes that match the latest taxonomic nomenclature for Caribbean corals (see Budd et al., 2012).
Lastly, correlation analyses were conducted to test for significant correlations between coral mortality and the other main coral conditions (burial, bleaching, and disease) using proportional data from the nine most abundant coral species as the unit of replication. A correlation matrix was generated using rcorr function from the R package “Hmisc” (Harrell, 2021) using the Pearson rank correlation coefficients for all possible column pairs in the matrix (n = 9), and then plotting the pairwise correlations using the R package “corrplot” (Wei and Simko, 2021).
Results
In all, 162 out of the 615 (26.3%) tagged corals monitored in this study died during the October 2013 to July 2015 observational period, and 240 (39.0%) of corals experienced partial mortality. 47 out of 615 (7.6%) of the tagged corals were fully buried at least once, and 369 out of 615 (60.0%) of the tagged corals were partially buried at some point during the monitoring period. The monitoring period also captured a thermal stress event during the late summer of 2014 (Figure 2). Onset of thermally induced coral bleaching occurred in July 2014 and peaked in September a few weeks after SSTs reached their seasonal thermal maximum (see Precht et al., 2019). Corals began to regain their zooxanthellae in October 2014 as temperatures cooled. Most colonies showed little or no outward signs of the mass bleaching event by December 2014. All reef habitats and all coral taxa were affected to some degree, with 89.0% of all tagged colonies bleaching at some point during this thermal anomaly. However, full or complete bleaching was recorded on only 70 out of 615 (11.4%) of the monitored corals. Only four of these tagged corals (<1%) died as a direct result of bleaching-related stress.
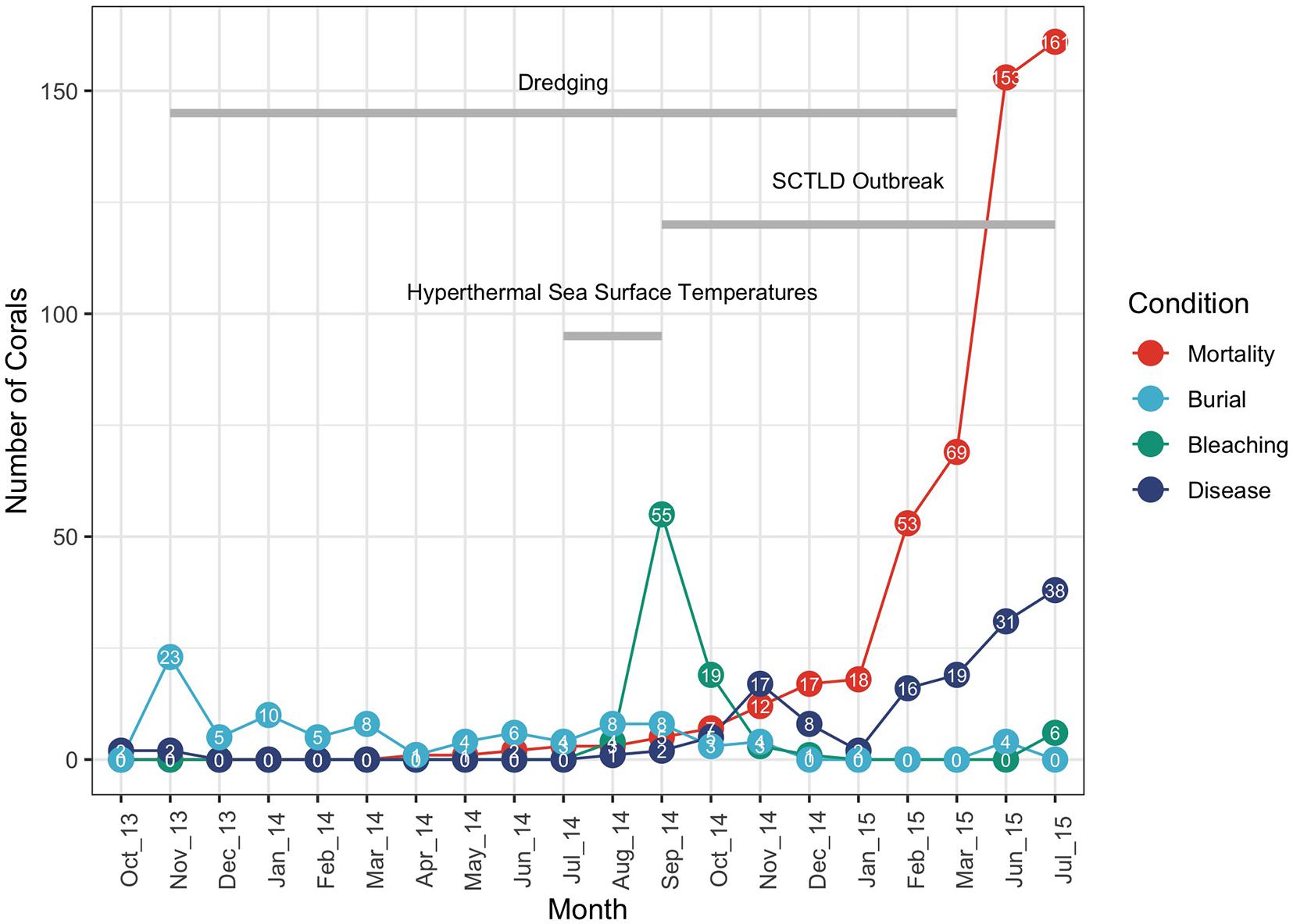
Figure 2. Coral conditions (buried, bleaching, disease, and mortality) plotted by month over the 21-month monitoring period. The timing of dredging, the 2014 summer hyperthermal event and the onset of the Stony Coral Tissue Loss Disease (SCLTD) outbreak starting in September 2014 are also shown. Number of corals with each of the conditions is labeled for each month. Cumulative mortality is plotted in red.
The first recorded appearance of a disease affecting more than (four out of 25) 5% of the corals at a particular site (R2SC2) occurred in late September of 2014 (Gintert et al., 2019). On that day three colonies of MMEA and one colony of DSTO were observed with initial signs of the emerging disease, By the end of the monitoring in July 2015, 112 out of 615 (18.2%) of the tagged corals had confirmed SCTLD disease signs (Dial Cordy and Associates Inc, 2015a,b). Figure 2 plots the number of tagged corals scored as buried, bleached or diseased over the course of the 21-month observational period. Cumulative mortality across the 21-month period is also plotted. The total number of buried corals peaked at 23 tagged corals in November 2013 after the passage of a series of early winter storms that may have been further compounded by the initiation of mantinenece dredge activities after November 20th, however, the total number of buried corals remained below 10 corals throughout the remainder of the monitoring program. The number of corals with signs of coral bleaching peaked in September 2014 at 55 tagged corals and was associated with the warm-water event recorded in the summer of 2014 (Gintert et al., 2019). Corals with signs of WPD first appear in the Port Miami dataset during the first week of baseline monitoring in October 2013 (Supplementary Figure 1) and correspond to observations of minor, background levels of coral disease being distributed across South Florida at this time (Peters and Fogarty, 2016). Many reefs throughout the region show low (<3%) WPD prevalence year-round (Borger, 2005; Gilliam et al., 2015; Aeby et al., 2017), suggesting that background levels of WPD continually exist within coral populations. Richardson and Aronson, 2002 noted that in Florida colonies of DSTO often exhibited a continuous background WPD prevalence of about 3%, however, the initial disease signs observed in these corals did not progress or result in full colony mortality. Corals that showed signs of WPD early in the Port Miami monitoring study went into remission when water conditions cooled during the winter of 2013–2014 resulting in only partial mortality of the colonies (Supplementary Figure 1). However, may have been further corals with WPD-like signs that were first noted in late-September of 2014 (initially labeled as “unknown condition” on the field data sheets on September 26, 2014; Supplementary Figure 2) appeared to be highly contagious, with the disease spreading rapidly within and between sites in the following weeks and months (Precht et al., 2016; Gintert et al., 2019). This disease outbreak was identified as distinct from WPD with many colonies dying rapidly (within weeks to months) and resulted in the case definition of SCTLD (Florida Coral Disease Response Research, and Epidemiology Team, 2018; see also Neely et al., 2021a). The number of tagged colonies with SCTLD disease signs rose in the fall of 2014 as the disease spread locally from the southern control sites north to the channel-side sites, fell slightly during the winter of 2015, and then rose sharply again when the disease reached the northernmost far-field control sites. The numbers of diseased colonies remained high until the end of the survey period in July 2015. Full coral mortality remained low over the first year of dredging and then rose sharply following the thermal bleaching event and initial outbreak of SCTLD in the fall of 2014 (see Figure 2 and Supplementary Figure 3).
Summary statistics for the six independent negative binomial GLMs examining the main (fixed) effects of dredge (channel), habitat, and direction on coral mortality, partial mortality, burial, partial burial, disease, and bleaching are summarized in Table 1. No significant effects of dredge (χ2 = 0.940, df = 1, and p = 0.332), habitat (χ2 = 3.616, df = 2, and p = 0.164), or direction (χ2 = 2.083, df = 1, and p = 0.149) were detected for full coral mortality. In our analysis, corals near the dredge channel had a 16.7% chance of experiencing full mortality while corals at control sites had a 14.4%. For full coral mortality, negative binomial GLM to converge the random effect of site was dropped. Dredging did have a significant impact on coral partial mortality (χ2 = 29.696, df = 1, and p < 0.001) and a two-way habitat:direction interaction (χ2 = 10.752, df = 2, and p < 0.005) and a three-way dredge:habitat:direction interaction (χ2 = 6.826, df = 2, and p < 0.05). Post hoc analyses show that corals near the dredge channel had a 59.2% probability of experiencing partial mortality vs. a 18% chance at the control sites (Figure 3). This increased risk did not result in a significant difference in full mortality.
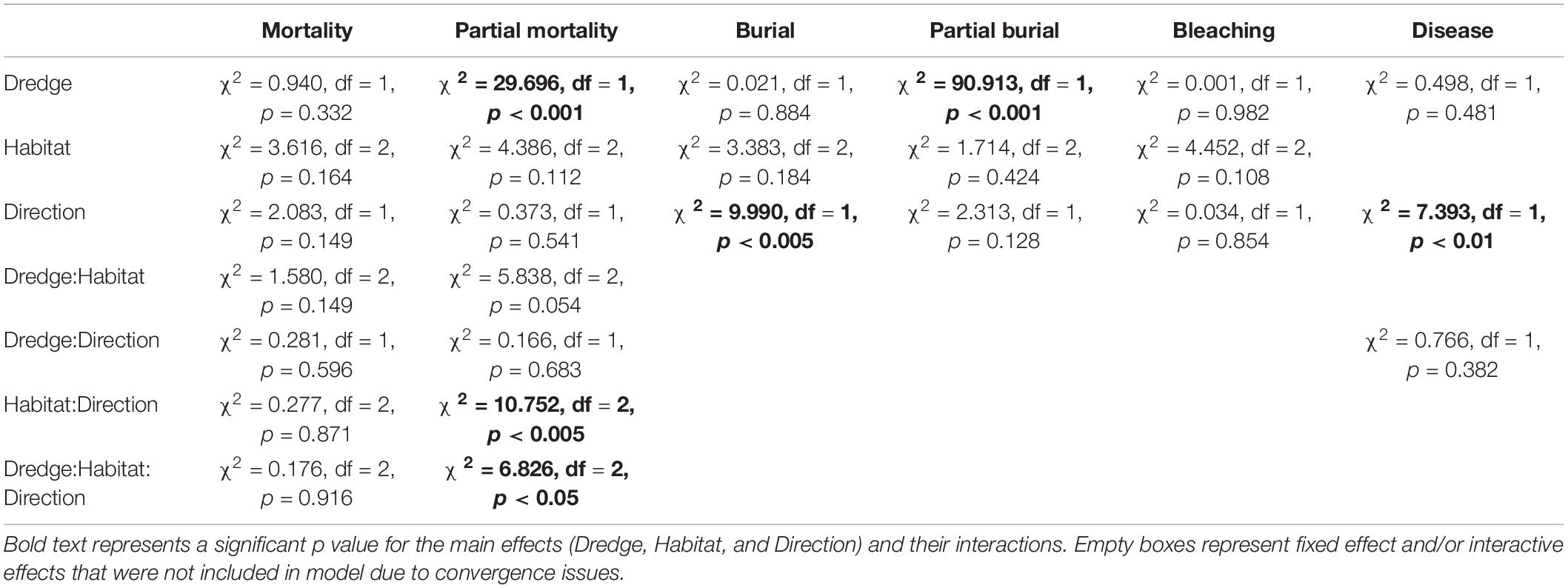
Table 1. Summary of the Type 2 ANOVA results [chi-square(χ2), degree of freedom (df), and p-value (p)] from the negative binomial generalized linear mixed effect models for each of the six coral conditions (mortality, partial mortality, burial, partial burial, bleaching, and disease).
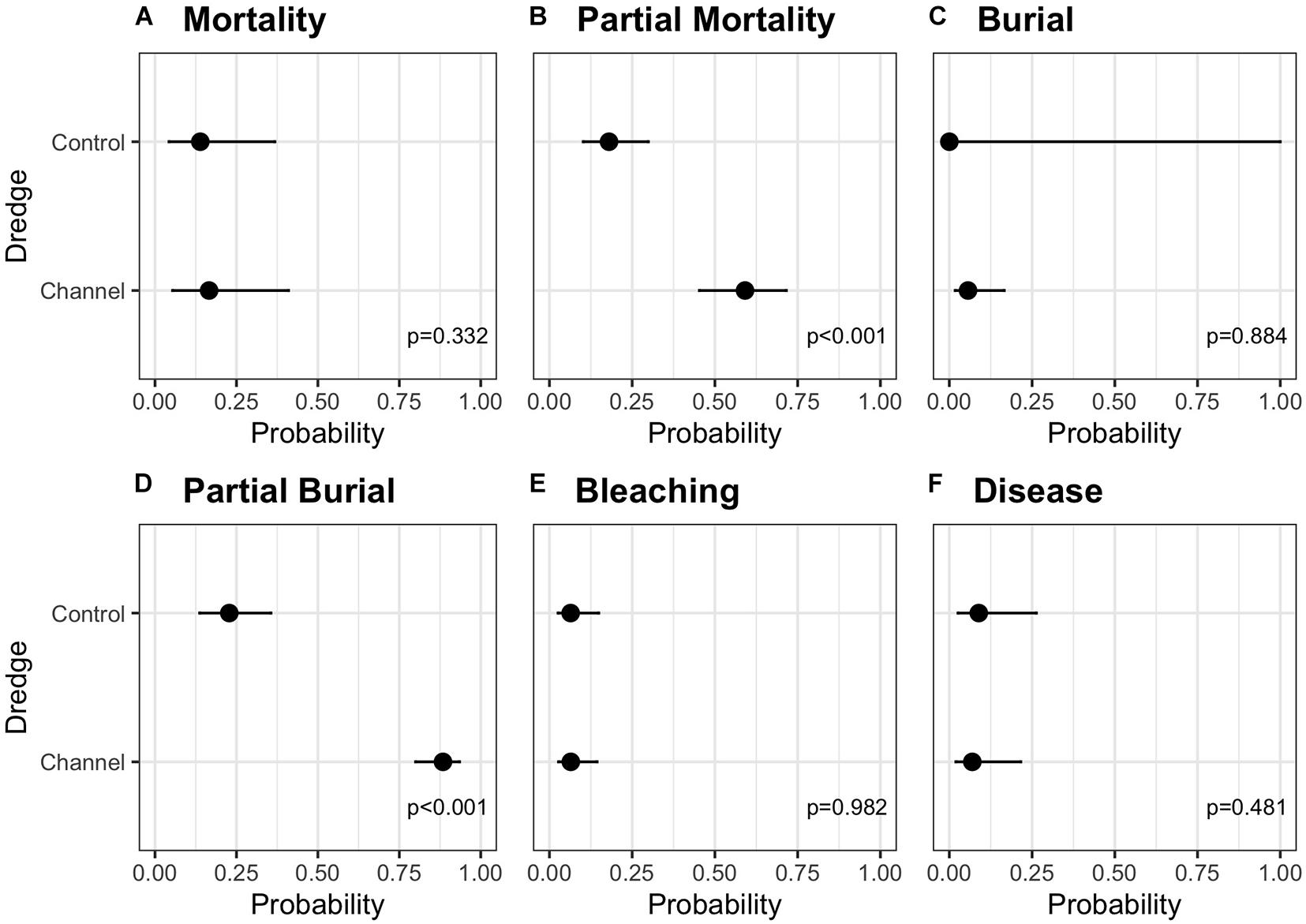
Figure 3. Estimated marginal means (emmeans) post-hoc analysis comparing the probability of (A) mortality, (B) partial mortality, (C) burial, (D) partial burial, (E) bleaching, or (F) disease based on a coral’s location to the dredge (control vs. channel site) with the upper and lower 95% confidence intervals. Analyses are for the full 615 tagged coral data set.
Full or partial burial is a possible consequence of dredging, and we did detect a significant effect of dredging on partial burial (χ2 = 90.913, df = 1, and p < 0.001) where channel-side corals had an 88.4% chance of partial burial compared to corals at control sites which had a 22.8% chance (Figure 3). For the partial burial negative binomial GLM to converge, site was dropped as a random effect and the fixed effects were additive instead of interactive. However, dredge effects were not significant for full burial (χ2 = 0.021, df = 1, and p = 0.884); instead, only direction had a significant impact on full burial (χ2 = 9.990, df = 1, and p < 0.005), with burial north of the channel being more likely. For the burial negative binomial GLM to converge, transect was dropped as a random effect and the fixed effects were additive instead of interactive. No significant effects of dredge, direction, or habitat were detected for complete thermal coral bleaching (Table 1). For the bleaching negative binomial GLM to converge, the fixed effects were additive instead of interactive. For SCTLD, only direction was significant (χ2 = 7.393, df = 1, and p < 0.01) with corals from the sites south of the channel having a 11.2% chance of contracting vs. a 5.5% chance in corals located north of the channel. For the disease negative binomial GLM to converge, site was dropped as a random effect and habitat was dropped as a fixed effect. This was the result of several factors and the causes responsible for this difference are elucidated in the discussion.
Survivorship analyses were then performed to examine the relationship between coral survival over time across the main effects of dredge, habitat, and direction for the full dataset as well as species-specific effects for the eight most abundant coral species in the dataset (as previously noted SINT did not experience any mortality during the monitoring period and was removed from this analysis lowering the total coral species from nine to eight). For the full 615 coral dataset, Cox regression models identified a significant effect of habitat (χ2 = 25.803, df = 2, and p < 0.0001) and direction (χ2 = 37.287, df = 1, and p < 0.0001), but not for location near the dredging channel on coral survival over time. Cox proportional hazard model shows that corals from the inner reef had significantly higher median HRs (HR = 1.8) compared to their counterparts from hardbottom habitats (Figure 4A) while corals from south of the dredge channel had significantly higher median HR of 1.8 compared to corals from north of the channel. Survival plots comparing the effects of habitat and direction (Figure 4B) show the sharp decline in coral survival over time on the inner reef and between the south vs. north channel sites.
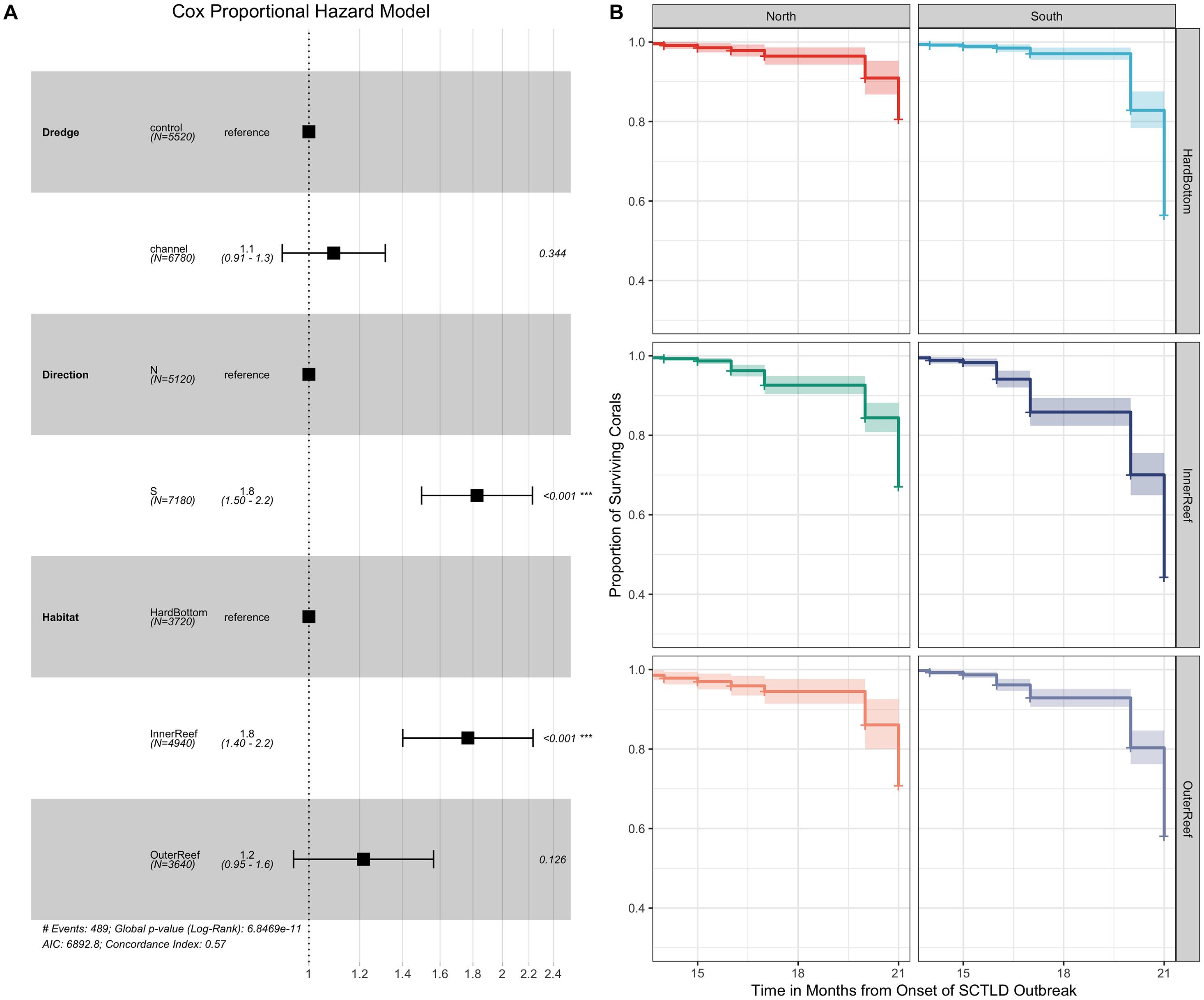
Figure 4. Cox-proportional hazard model (A) comparing the effects of dredge (control vs. channel), habitat (hardbottom vs. inner reef and outer reef), and direction (north vs. south) on coral survival across the 21-month observation period for the full 615 tagged coral dataset along with survival curves (B) plotting the significant main effects of direction and habitat on coral survival. In the Cox-proportional hazard model (A) the median hazard ratios (HR) +/– the standard errors are shown along with the p-values (far right) showing the pairwise comparisons between the reference level and the comparison (e.g., control as the reference for dredging compared to channel).
To examine the impact of coral species on survival, we then preformed survivorship analyses on the eight most abundant coral species in the dataset PCLI, DSTO, PSTR, MCAV, MMEA, PAST, SBOU, and SSID (note that SINT is abundant, but was excluded because no mortality was observed in this species). Survival analyses on the eight species dataset identified significant effects of habitat (χ2 = 29.64, df = 2, and p < 0.0001) and species (χ2 = 475.44, df = 7, and p < 0.0001), but not direction or dredging. Cox proportional hazard model (Figure 5) shows that corals from the inner and outer reef habitats had significantly higher median HRs (HR = 2.0 and 2.0, each) compared to their counterparts from hardbottom habitats (Figure 5). Median HRs comparing species against SSID (which had 95% survival) showed that MMEA (HR = 13.8), PSTO (HR = 10.2), PSTR (HR = 10.1), PCLI (HR = 6.6), followed by SBOU (HR = 1.9) all had significantly higher HRs than SSID, whereas the HRs of MCAV and PAST did not differ significantly from the HR of SSID. These dramatic differences in survival across species and habitat are apparent in the survival plots after the onset of SCTLD in the monitoring sites (Supplementary Figure 4) and in the estimated survival probabilities across species (Figure 6).
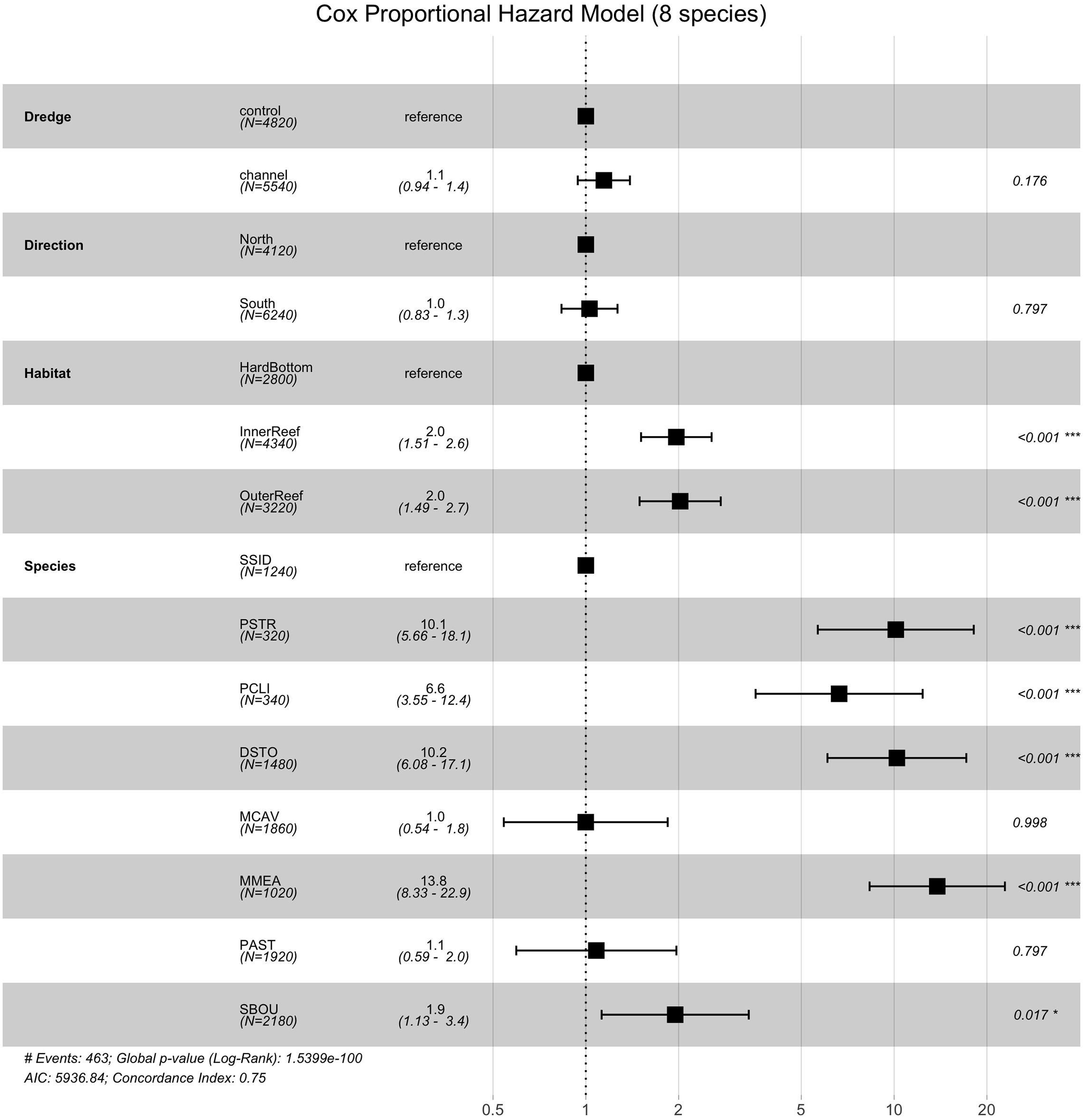
Figure 5. Cox-proportional hazard model comparing the effects of dredge (control vs. channel), habitat (hardbottom vs. inner reef and outer reef), direction (north vs. south) and species across the top eight coral species in the dataset that experienced at least some mortality. In the Cox-proportional hazard model the median hazard ratios (HR) +/– the standard errors are shown along with the p-values (far right) showing the pairwise comparisons between the reference level and the comparison (e.g., control as the reference for dredging compared to channel).
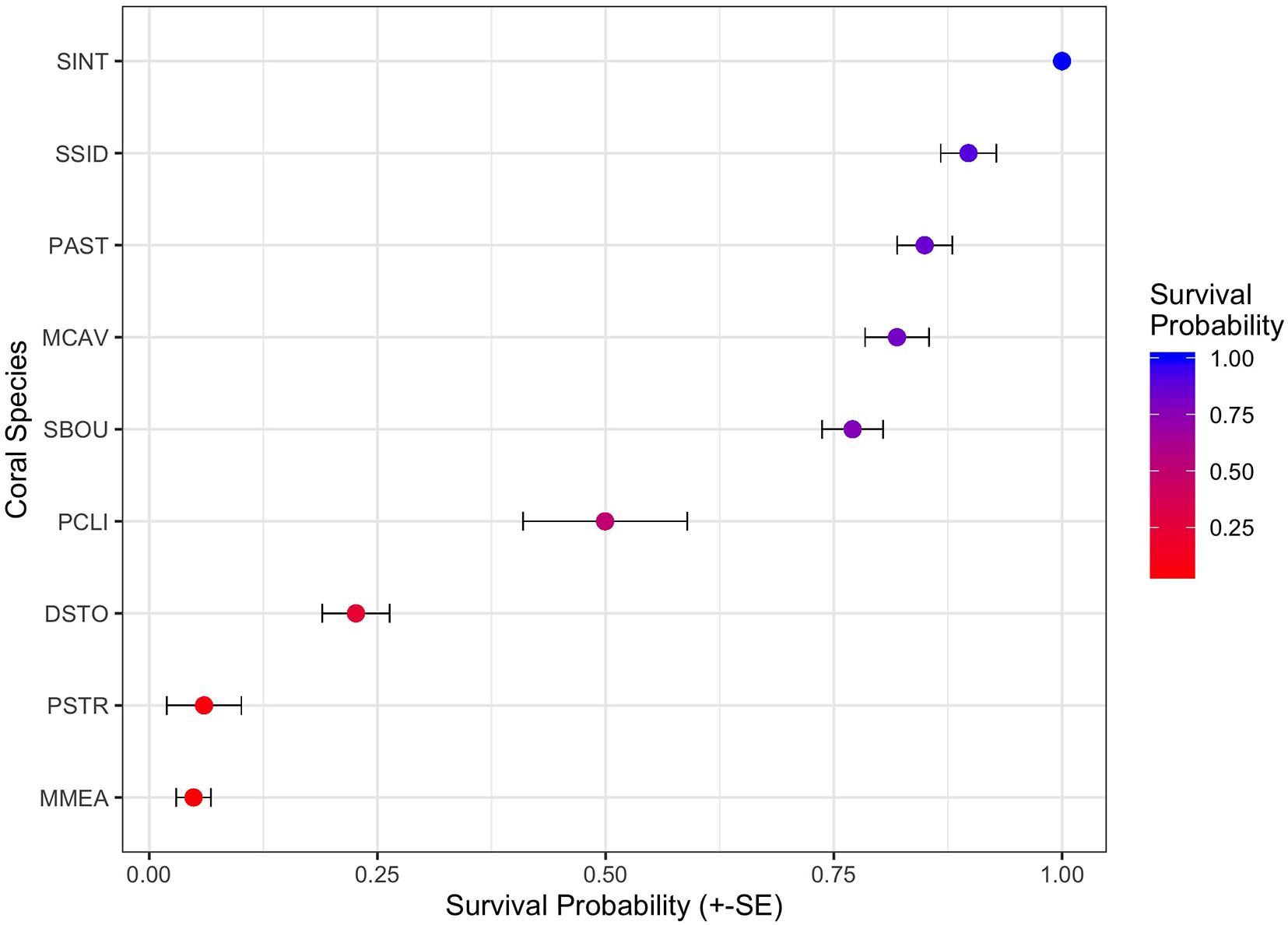
Figure 6. Twenty One-month survivorship probabilities (+/– standard errors) across the nine most common coral species in the monitoring data. Nine species include Stephanocoenia intercepta (SINT), Siderastrea siderea (SSID), Porites astreoides (PAST), Montastrea cavernosa (MCAV), Solenastrea bournoni (SBOU), Pseudodiploria clivosa (PCLI), Dichocoenia stokesi (DSTO), Psuedodiploria strigosa (PSTR), Meandrina meandrites (MMEA), and are ranked by the highest to lowest mean survival.
When examining the conditions (mortality, burial, bleaching, and disease) that affected this subset of corals by species, there was an uneven distribution of mortality across coral species (Figure 7). To determine if any of the major coral conditions (full burial, bleaching, or disease) were correlated with full coral mortality, we performed pairwise correlation analyses on the proportions of each state using the nine common corals species as replicates; the resulting correlation matrix shows that disease is strongly and significantly correlated with mortality (Pearson R = 0.9, p = 0.0011) and negatively correlated with burial (R = –0.89, p = 0.0014). The only other significant correlation was a negative correlation (R = –0.68, p = 0.0459) between corals with no observed condition and mortality (Figure 8).
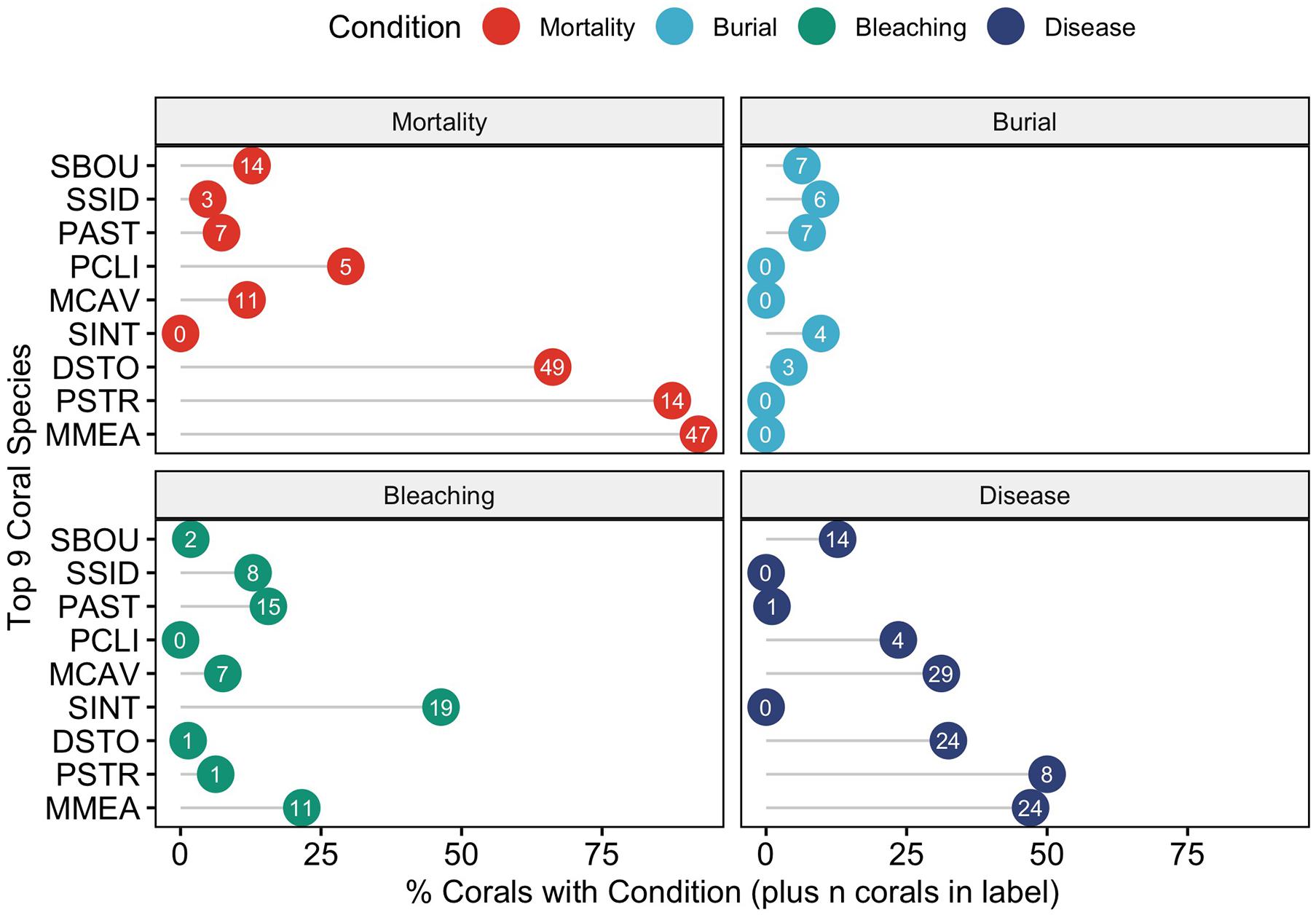
Figure 7. Coral conditions plotted for each of the nine most abundant coral species – Solenastrea bournoni (SBOU), Siderastrea siderea (SSID), Porites astreoides (PAST), Pseudodiploria clivosa (PCLI), Montastrea cavernosa (MCAV), Stephanocoenia intercepta (SINT), Dichocoenia stokesi (DSTO), Psuedodiploria strigosa (PSTR), and Meandrina meandrites (MMEA). The x-axis plots the percent of individual corals with the condition and the number shows the actual number of individuals with that condition.
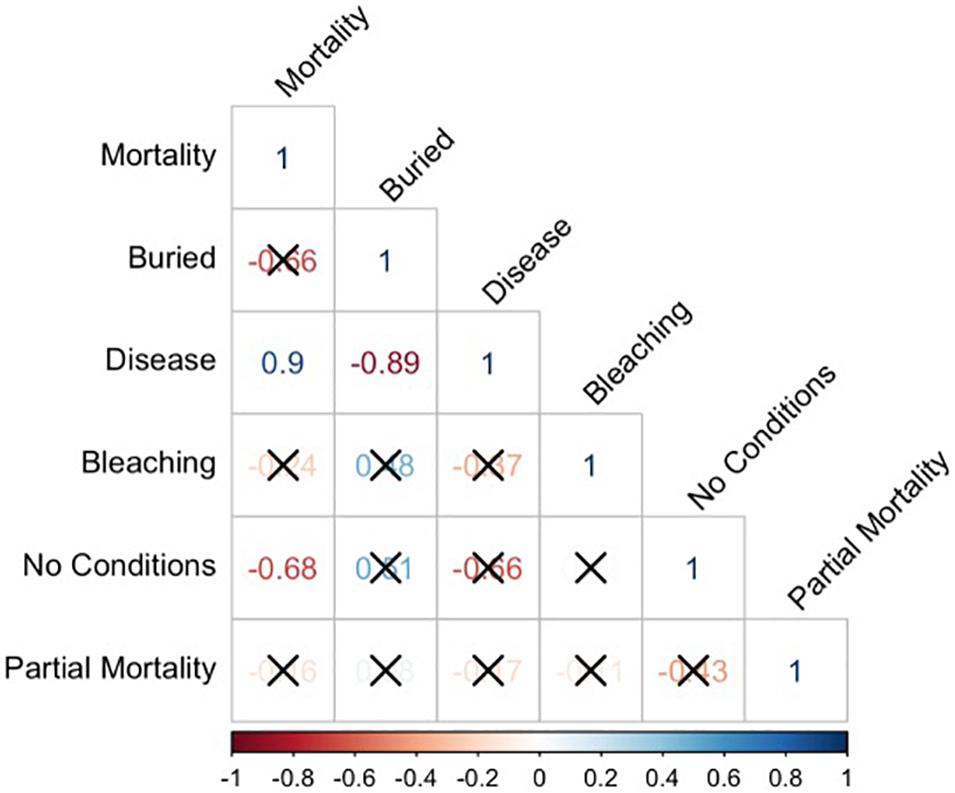
Figure 8. Pairwise correlation plots comparing mortality, burial, disease, bleaching, no conditions and partial mortality for the nine most abundant coral species – Pseudodiploria clivosa (PCLI), Dichocoenia stokesi (DSTO), Psuedodiploria strigosa (PSTR), Montastrea cavernosa (MCAV), Meandrina meandrites (MMEA), Porites astreoides (PAST), Solenastrea bournoni (SBOU), Stephanocoenia intercepta (SINT), and Siderastrea siderea (SSID). Pearson correlation (r-value) is shown with significant values (p < 0.05) shown in bold and colored by strength (blue for highest positive correlation and red for lowest negative correlation). Non-significant correlations are crossed out in the matrix.
The strong positive correlation between disease and mortality across the nine species (Figure 9) shows that the highest mortality was observed in the species that had the highest proportion of diseased corals like MMEA, PSTR, and DSTO, which are highly susceptible to SCTLD, whereas the highest survival was observed in corals like SSID, PAST, and SINT, which did not contract SCTLD at our sites during the 21-month study window.
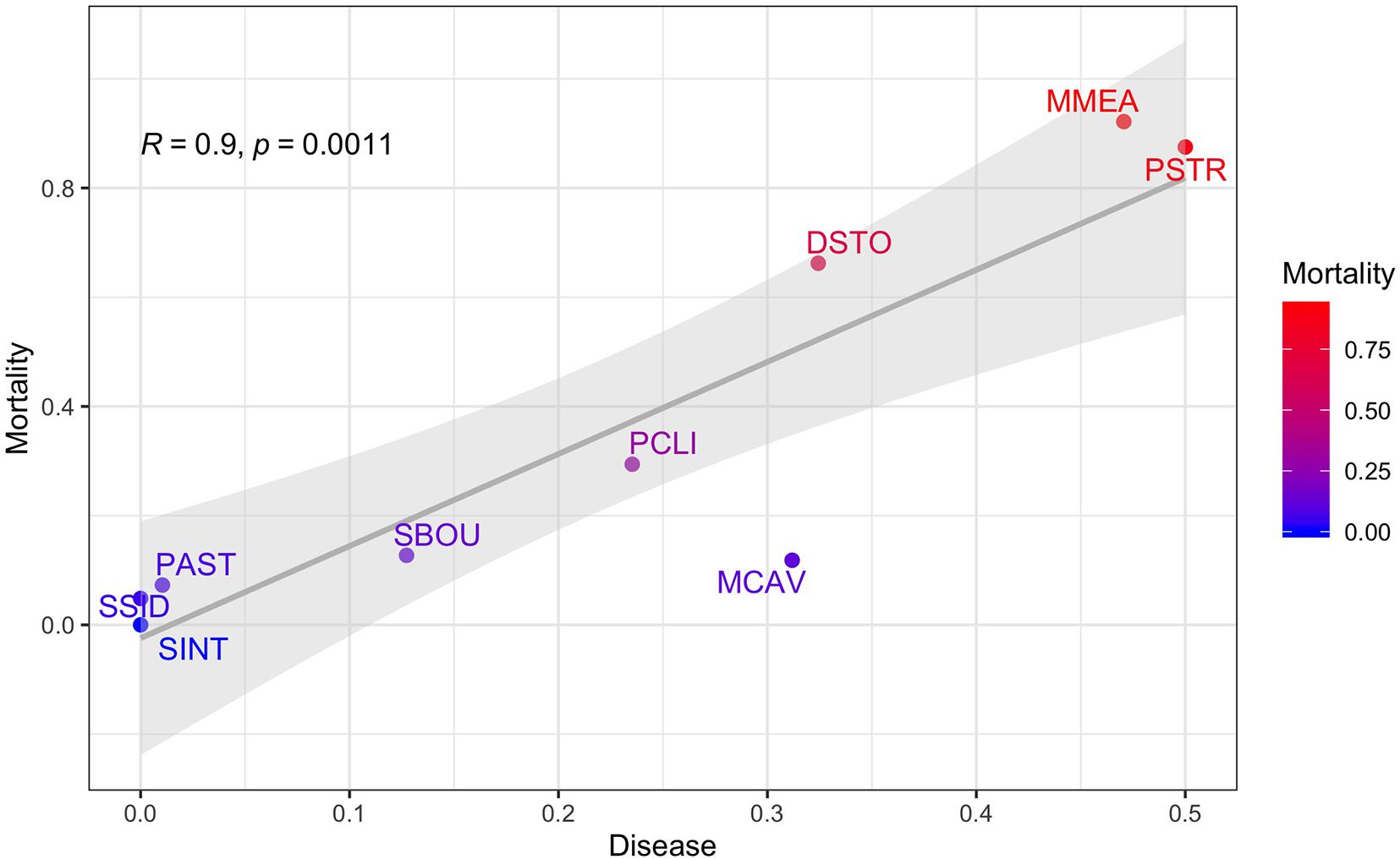
Figure 9. Proportion of diseased corals plotted against coral mortality using the proportions from the top most abundant corals as the unit of replication. R and p-values from the correlation analyses are shown along with a fitted linear regression and the 95% confidence intervals. Coral mortality is colored from red (highest) to blue (lowest). Nine species include: Pseudodiploria clivosa (PCLI), Dichocoenia stokesi (DSTO), Psuedodiploria strigosa (PSTR), Montastrea cavernosa (MCAV), Meandrina meandrites (MMEA), Porites astreoides (PAST), Solenastrea bournoni (SBOU), Stephanocoenia intercepta (SINT), and Siderastrea siderea (SSID).
Discussion
Coral reefs are dynamic and open systems that experience a wide range of anthropogenic stressors at the local, regional, and global level. With coral reefs declining worldwide, knowing how these stressors impact coral survival is key to their protection and stewardship (Aronson and Precht, 2009, 2016). In this study, we examined how dredging, bleaching, and the first reported outbreak of SCTLD influence the survivorship of the corals monitored during the 21-month Miami Dredge project where 162 out of 615 (26.3%) corals died. Our results clearly show that dredging and the impacts of local sedimentation had an impact on the partial mortality of individual coral colonies, but importantly, did not have a significant impact on the total coral mortality over the 21-month monitoring period. An almost identical pattern was reported for a dredging project performed off Miami-Dade County in the late 1970s (see Marszalek, 1982). Our results documented a strong and significant link between the outbreak of SCTLD that initiated in late September 2014, to the widespread mortality of corals documented at the study sites. The most highly susceptible species like MMEA, PSTR, and DSTO showed the strongest effects and proportionally suffered the greatest losses (see also Precht et al., 2016; Gintert et al., 2019).
Our analyses support the findings of Cunning et al. (2019) who documented a significant effect of dredging on partial (but not full) coral mortality in the Port Miami monitoring dataset. In our analyses of the full 615 tagged coral dataset, we demonstrate that corals near the dredging sites experience a 59.2% chance of partial mortality whereas corals in the control sites have a 18.0% chance (Figure 3). Importantly, our results demonstrate that sediment burial did not result in a significant increase in full coral mortality. Instead, the data indicate that the high coral mortality observed in the study was strongly associated with the SCTLD outbreak that was first observed during this monitoring study in late September 2014 and has since spread throughout Florida and into the greater Caribbean (AGRRA, 2021). Thus, while we detected the same significant effects of dredging on the partial mortality of corals, our findings stand in stark contrast to the conclusions of Cunning et al. (2019) who attributed most of the coral mortality to the impacts of the local dredging effects rather than other regional and global factors such as bleaching and disease. Cunning et al. (2019) estimated “that over half a million corals were killed during the dredging period” directly due to burial by dredge-related sediments. Unfortunately, this conclusion by Cunning et al. (2019) is not supported by the data and likely obscured the much larger impact of the SCTLD outbreak that was documented during the monitoring period. During the dredge monitoring period, only eight (17.0%) of the 47 tagged corals that became fully buried were characterized as having experienced complete mortality between October 2013 and July 2015. However, follow-up surveys performed in the summer of 2016 found that two of these colonies were still alive (Supplementary Figure 5). Using the photographic database to evaluate the full sequence of events prior to their death, it is likely that these corals died directly from exposure to long-term, sediment burial. Because these corals were all found at Channel-side locations we assume that there was a likely association with dredge activities. However, because other sources of sediment are locally present, including the regular discharge of sediment-laden plumes from the Miami River through the Port Miami dredge channel (Government Cut), it is impossible to disentangle the proportion of sediment attributed only to the dredge project (see Supplementary Figure 6).
Instead, our findings build on previous work by Gintert et al. (2019) who demonstrated that “species-specific susceptibility to disease is the determining factor in 93.3% of coral mortality evaluated throughout Miami-Dade County, whereas local dredging stress only accurately predicted coral mortality levels 6.7% of the time.” Once we began to look at coral mortality across different species (Figure 7), the data shows that the strong species-specific effect relates to disease susceptibility (Figure 9), where SCTLD susceptible species like MMEA, PSTR, and DSTO had higher species mortality compared to SSID, PAST, and SINT. Importantly, the pattern of survivorship of corals by species (Figure 6) for the Port Miami monitoring project, where SCTLD was first documented, essentially matches the species-specific susceptibility patterns that have since been recorded across Florida and the broader Caribbean (Precht et al., 2016; Florida Coral Disease Response Research, and Epidemiology Team, 2018; Neely, 2018; Alvarez-Filip et al., 2019; Sharp et al., 2020; Dahlgren et al., 2021; Heres et al., 2021; Warrender et al., 2021). The coral species with the lowest chance of survival (MMEA, PSTR, and DSTO) are all considered highly susceptible species (see Precht et al., 2016; Florida Coral Disease Response Research, and Epidemiology Team, 2018; Gintert et al., 2019). The next three corals with slightly higher survivability (PCLI, SBOU, and MCAV) are corals that were considered moderately susceptible to SCTLD in Miami-Dade County (Gintert et al., 2019). The corals with the highest survivorship (SSID, PAST, and SINT) are all species that were initially found to have low susceptibility to SCTLD and appeared to be unaffected by the initial SCTLD outbreak in Miami-Dade County (Precht et al., 2016). However, SSID was found to show signs of an emerging disease termed Siderastrea white-botch syndrome in Miami-Dade County during the summer of 2015 (see Precht et al., 2018). There is presently some question as to whether this disease is unique or related to SCTLD (see Florida Coral Disease Response Research, and Epidemiology Team, 2018; Heres et al., 2021). The significant positive relationship between SCTLD and mortality was confirmed by a correlation plot of the nine most common coral species in this study (Figure 8).
As previously mentioned, there was a significant negative correlation (R = –0.68, p = 0.0459) between corals with no observed condition(s) and mortality, this was because 32 corals died with no recorded conditions between surveys. Even though these corals were assumed to be killed by disease (see Gintert et al., 2019), we were extremely conservative in our analysis, and did not try to attribute a cause of mortality to corals that died with no previous recorded condition. Elsewhere, the losses of these corals were termed “sudden death.” Specifically, Miller et al. (2016) stated “[T]he complete mortality of a colony between sequential photos in the time series, presumably attributable to disease, (though no active disease signs were observable) were recorded in sequence. We included ‘sudden death’ in a category of disease impact given consistency with described patterns of mortality (i.e., complete colony mortality over a period of weeks) associated with a regional outbreak of ‘White Plague’ [SCTLD] disease affecting most species of mounding corals during this time frame (Precht et al., 2016), a lack of other known disturbances such as storms, and the presumption that the longer interval of images during which most of the ‘sudden death’ occurred (winter 2014–2015) was during a period when dredging activities were distant according to permit requirements (hence transient sediment burial unlikely).” Placing these corals with “no observed condition” prior to dying in the disease category (Supplementary Figure 7), as had been done in Precht et al. (2016), Precht et al. (2019), and Gintert et al. (2019) would have increased the impact of SCTLD in our analysis from 18.2 to 23.4%. Thus, while our analysis shows a significant effect of disease on coral mortality, it likely is an under-estimate of the actual effects of the SCTLD on coral communities in Miami-Dade County and the Southeast Florida reef tract during the 21-month study. For instance, Walton et al. (2018) stated “the 2014 to 2016 disease outbreak was arguably the most devastating disturbance event yet documented in the Southeast Florida coral reef tract (SEFCRT) and has altered ecosystem function to a point where recovery is greatly challenged. Our data estimates that regionally as much as 30% of coral colony density and 60% of live tissue area was lost.”
The results of the Cox proportional hazard model (Figure 5) shows that corals from the inner and outer reef habitats had significantly higher median HRs (HR = 2.0 and 2.0, each) compared to their counterparts from nearshore hardbottom habitats. These nearshore hardbottom habitats were also some of the last to become infected with SCTLD. This result mimics the offshore to onshore patterns of SCTLD impacts recorded in the Florida Keys (Rippe et al., 2019; Kolodziej et al., 2021; Williams et al., 2021). It may be that these inshore sites may be more adapted to various stressors such as fluctuating water quality and marginal environmental conditions (Lirman and Fong, 2007), possibly making them more resistant to disease outbreaks (Rubin et al., 2021).
We believe that the repeated in situ observations made during dredge monitoring also allowed us to isolate the initial signs of the SCTLD disease outbreak and then follow the spread of the disease through time and space. North/south differences in our survival analysis support the pattern of the spread of the infection described in Precht et al. (2016) where the disease moved from the southern to northern monitoring sites with the direction of the prevailing currents (Kourafalou and Kang, 2012; Muller et al., 2020). While the disease did also move south through time, albeit more slowly (see Precht et al., 2016), we were unable to detect that spread in the Port Miami monitoring data because the disease signs were first observed at the southernmost far-field control monitoring site. In our analysis, we also found that corals south of the active dredge channel were more likely to become infected by SCTLD (Supplementary Figure 8) and therefore have a lower chance of survival than corals NC (Figure 4B). This is likely due to a combination of two main factors: (1) the species composition of the tagged corals south of the dredge channel contained more disease susceptible species than those north of the channel (Gintert et al., 2019); and (2) because the disease had only arrived at the northernmost sites toward the end of the monitoring program, the disease had not yet run its course through the coral community at these sites. Interestingly, in a subsequent re-survey of these same tagged corals performed approximately 1-year later, during the summer of 2016 (Precht et al., 2019), it was noted that the SCTLD outbreak was still active and 17 (4.3%) of the remaining 391 live tagged colonies still had progressive SCTLD lesions. This was mostly observed on colonies of MCAV and SBOU, at sites NC (Figure 4B). These subsequent observations confirm that the northernmost monitoring sites were the last to become infected with the disease. These findings support the idea that this study likely captured the onset of the SCTLD outbreak in southeast Florida (see also Gintert et al., 2019). In addition, corals north of the channel were either buried or partially buried by sediment more frequently than those to the south (again due to the prevailing currents) resulting in greater levels of partial mortality. Accordingly, overall coral survivorship patterns should have shown lower levels of mortality in corals south of the channel if dredging, not disease, were responsible for their demise during the monitoring period. As well, if sediment stress increased disease susceptibility as proffered by Miller et al. (2016), then coral disease should have been higher at channel-side sites irrespective of species composition. However, the highest mortality was observed in the species that had the highest proportion of disease susceptible coral species like MMEA, PSTR, and DSTO regardless of location, habitat, or proximity to dredge operations.
Starting in the summer of 2015, other scientific teams who were collecting data at their long-term monitoring sites throughout Miami-Dade County, including at sites far from the dredging project, also reported similar levels of devastation caused by this disease (Gilliam et al., 2015, 2016, 2018; Coastal Systems International Inc, 2016; Miami-Dade County Department Environmental Resource Management, 2016; Hayes et al., 2017; Walton et al., 2018; Sinigalliano et al., 2019). Thus, the impact of SCTLD on the mortality in the Port Miami monitoring data that we report match, and in some cases underperform, the high levels of disease mortality documented in these other studies (see Figure 3 in Precht et al., 2019). Thus, it is clear from our survivorship analysis that the impact of SCTLD should not be underestimated as the overriding cause of coral mortality at our monitoring sites as well as throughout southeastern Florida.
The results of this study show that while partial mortality of corals was affected by the Miami Deep Dredge Project (see also Dial Cordy and Associates Inc, 2015a,b), the stressor that was most influential in coral survivorship was the emergence and prevalence of SCTLD. Unfortunately, coral disease outbreaks and bleaching events are predicted to become more frequent with rising sea temperatures (Harvell et al., 2007; Hoegh-Guldberg and Bruno, 2010; Maynard et al., 2015; Zvuloni et al., 2015), which are expected to increase by 1–2°C by 2050 and up to 4°C by 2100 (Intergovernmental Panel on Climate Change, 2013). Elevated SST is one of the proximal drivers of coral disease epizootics (Bruno et al., 2007; Harvell et al., 2007; Heron et al., 2010; Randall et al., 2014). Warmer temperatures increase the virulence and abundance of pathogens, especially bacterial pathogens (Ward et al., 2007; Sokolow, 2009; Mao-Jones et al., 2010; Palmer et al., 2011; Maynard et al., 2015). Increased temperatures also increase the susceptibility of the host to disease by compromising the coral’s immune system (Harvell et al., 2007; Ainsworth and Hoegh-Guldberg, 2009; Reed et al., 2010; Burge et al., 2014; Pinzón et al., 2015; Merselis et al., 2018; Muller et al., 2018). Specifically, Pinzón et al. (2015) noted that the effects of thermal stress (bleaching) can extend for years following an event including a significant increase in disease prevalence. They stated “[T]he relationship between coral bleaching and disease outbreaks suggests that the host’s innate immune system is affected by bleaching and the changes persist long after the stressful conditions are over” (see Mydlarz et al., 2009). Eakin et al. (2010) commented that the pattern of high thermal stress followed by subsequent mortality across much of the Caribbean was consistent with outbreaks of coral diseases that emerged in the years that succeeded thermal stress and bleaching events. Lesser et al. (2007) also noted that episodes of thermal stress and coral bleaching likely facilitate disease epizootics. For instance, Muller et al. (2008) specifically showed that disease-associated mortality in the United States Virgin Islands was only apparent on Acropora palmata when the coral host had bleached prior to the disease outbreak. In a similar study, Brandt and McManus (2009) documented that colonies that contracted WPD in the Florida Keys were colonies that previously experienced extensive bleaching during a significant thermal anomaly in 2005. Similar results were documented in the eastern Caribbean following the 2005 regional thermal anomaly (Cowan, 2006; Harding et al., 2008; Cróquer and Weil, 2009; Miller et al., 2009; Rogers et al., 2009). Together, these results reflect a consistent relationship between thermal stress, bleaching, and disease prevalence.
Contrary to some of these earlier studies, the results of the 2014–2015 SCTLD outbreak reported herein shows that there was not a significant correlation between bleaching and disease (R = –0.37, p = 0.3269) or between bleaching and mortality (R = –0.24, p = 0.5409), and that bleaching was not a prerequisite of disease infection or mortality (see also Precht et al., 2016). However, because essentially every coral showed some level of bleaching stress during the monitoring program (89.0% of all tagged corals showed some outward signs of beaching between July 2014 and July 2015), we only analyzed whether “full” bleaching had an impact on coral mortality. In our analysis, only four corals were observed through the photographic database to have been killed directly from bleaching-induced stress. However, Brodnicke et al. (2019) stated that even mild bleaching caused a threefold increase in accumulated tissue loss (69.6 ± 10.5%) associated with an outbreak of white syndrome, on the Great Barrier Reef in 2017, suggesting that disease exacerbated mortality in bleached corals, and not the bleaching itself, contributed significantly to the substantial loss of corals. It should also be noted that bleaching-induced mortality should not be confused with bleaching-related stress and that the onset of SCTLD in Miami-Dade County in 2014 followed the worst thermal stress event recorded regionwide in more than two decades (Manzello, 2015; Precht et al., 2016).
The initiation of the SCTLD outbreak in Miami-Dade County appears to have been temporally linked with warm winter and spring temperatures followed by an anomalously warm summer in 2014 (Manzello, 2015). This one-two punch, a mild winter and spring followed by warm summer, corresponded to the onset widespread coral bleaching and the lethal coral disease outbreak that followed (Figure 2). Heron et al. (2010) found that mild winters frequently preceded the thermal anomalies associated with white syndrome disease outbreaks along the Great Barrier Reef, Australia. Importantly, during the summer of 2014 in southeast Florida, SST’s exceeded the regional bleaching threshold of 30.4°C (Manzello et al., 2007) for 44 days at Bear Cut and 29 days at Fowey Rocks (National Oceanic Atmospheric Administration, 2021a,b), resulting in significant temperature stress and widespread coral bleaching (Manzello, 2015; Gintert et al., 2019). However, the first signs of the onset of the SCTLD epizootic were not noted until after peak bleaching and as SST’s began to cool (see Gintert et al., 2019). An almost identical scenario of a white-syndrome coral disease outbreak followed on the heels of a significant bleaching event in Kaneohe Bay, Hawaii just as corals there were showing signs of recovery from a significant bleaching event in the fall of 2014 and as SST’s began to cool (Hawaii Department of Land and Natural Resources, 2015). Similarly, in the Red Sea, Zvuloni et al. (2015) noted that WPD prevalence lagged about 3 months behind the warmest SST’s. In the United States Virgin Islands, Miller et al. (2009) reported that the greatest amount of WPD-induced mortality occurred within several months after the highest prevalence of a major coral bleaching event in the summer of 2005. Miller et al. (2009) showed that maximum disease-induced mortality did not occur at the same time for each of their sites and that >65% of the disease-induced mortality occurred within 6 months after bleaching was first observed. Thus, in these cases the pattern of disease onset following significant thermal anomalies and bleaching events appear to show a direct causal relationship.
Although it is clear that extremely warm water temperatures preceded the onset of SCTLD throughout southeast Florida (Manzello, 2015; Precht et al., 2016; Walton et al., 2018; Jones et al., 2021), once the disease outbreak was spreading regionwide there was no clear link between the time of year, temperature, and initiation of SCTLD (Muller et al., 2020). Thus, water temperatures at the time of disease onset may be irrelevant to its initiation. This is likely a delayed response of the coral host to earlier cumulative stressors including temperature (Lesser et al., 2007) and the infectious, water-borne nature of this contagion as it progressed across the region (Dobbelaere et al., 2020). Porter et al. (2001) emphasized the repeated theme of a link between coral disease and temperature, although seasonality was not always apparent. Importantly, the outbreak of SCTLD that began in the fall of 2014, has spread regionwide during the warmest decade on record, with the warmest 6 years all having occurred since 2015 (World Meterological Organization., 2020). Warming during this period was greater in the Caribbean and the sub-tropical western Atlantic than the global average. Thus, coral colonies throughout Florida and the Caribbean have regularly and repeatedly been subjected to significant thermal stress throughout the period of the SCTLD outbreak with unknown long-term consequences.
There were localized areas in the Middle Florida Keys that saw a decrease in incidence and death rates in corals already infected with SCTLD during the warmest months in the late summer of 2018 (Sharp et al., 2020; see also Williams et al., 2021) questioning a direct link to temperature stress and the impact of SCTLD. While Sharp et al. (2020) showed that the rate of tissue loss among SCTLD-affected colonies slowed or stopped across their sites during the summer of 2018 and coincided with the seasonal peak in coral bleaching, they did not observe a similar decrease in disease rates during the summer of 2019. In the United States Virgin Islands, Meiling et al. (2020) also showed a decrease in the rate of tissue loss associated with a SCTLD outbreak as thermal stress accumulated in the late summer. Thus, the relationship between thermal stress and the pathogenesis of SCTLD is not straightforward and remains a question for the future. It has also been shown that disease outbreaks can often linger for years in a coral population following its introduction (Aronson and Precht, 2001; Miller et al., 2009). This is certainly true for SCTLD which has been ravaging coral populations throughout Florida for more than 6 years and is now actively spreading throughout the Caribbean (AGRRA, 2021).
While numerous hypotheses have been developed to explain the potential origin of where and why this disease started off Miami-Dade County when it did (Gintert et al., 2019; see also Precht, 2021), to date the origin and etiology of this disease remains poorly understood. Edge et al. (2013) noted that corals near the Port Miami inlet experienced significant changes in expression of stress responsive and symbiont (zooxanthella)-specific genes after periods of heavy precipitation. Coincidentally, Sinigalliano et al. (2019) found a salinity minimum observed in September 2014 following significant late-summer rainfall resulting in high canal flow, and increased discharge of the Miami River into the waters off Miami-Dade County. This freshwater event also resulted in elevated concentrations of nutrients and was concomitant with peak coral bleaching that was observed regionwide. In addition, Staley et al. (2017) found that microbial communities that originated primarily from wastewater treatment plant outfalls were the predominant sources of pollution that directly influenced the structure of the microbial communities in reef water and in coral tissues off Miami-Dade County. Therefore, it is important to note that the 2014 SCTLD outbreak was first observed at the southern far-field control inner-reef site (R2SC2) that was located adjacent to the oceanic outfall discharge pipe of the Miami Central District Municipal Wastewater Treatment Plant located off Virginia Key (Figure 1). This discharge pipe discharges approximately 150 million gallons of partially treated wastewater directly into the Atlantic Ocean every day and was known to be leaking (Staletovich, 2017). All these stressors were percussors to the onset of SCTLD.
Thus, while increased temperature and diminished water quality were the likely stressors that triggered this disease outbreak in Miami-Dade County in the fall of 2014 (see Staley et al., 2017; Walton et al., 2018; Gintert et al., 2019; Sinigalliano et al., 2019; Whitall et al., 2019; Jones et al., 2021), it is still important to know the origin of the purported pathogen(s). This is especially important for developing disease amelioration strategies for reefs impacted by SCTLD (Precht, 2021). Unfortunately, the specific etiology of this disease outbreak remains elusive (Aeby et al., 2019; Meyer et al., 2019; Iwanowicz et al., 2020; Landsberg et al., 2020; Rosales et al., 2020; Ushijima et al., 2020; Thome et al., 2021). Until we can unravel this mystery, we can only speculate as to the exact cause of SCTLD. Similarly, identifying the pathogen(s) responsible for white-band disease (WBD) in the Caribbean Acropora spp. continues to remain an enigma well more than four decades after it was first described (see Gignoux-Wolfsohn et al., 2020). Kline and Vollmer (2011) noted that for many coral diseases using the traditional Henle–Koch’s postulate testing method is fraught with problems because the potential pathogens either cannot be cultured or because the disease is caused by a consortium of organisms. However, because the infectious agents in WBD can be treated with antibiotics (Kline and Vollmer, 2011; Sweet et al., 2014) it has been suggested that several bacterial taxa are likely the potential pathogens. For SCTLD it has recently been shown that topical amoxicillin treatments successfully arrested disease lesion progression on multiple species of corals affected by the disease (Neely et al., 2020) giving credence to the hypothesis that the pathogen(s) responsible are also likely to be bacterial in origin (see also Miller et al., 2020; Neely et al., 2021b; Shilling et al., 2021; Walker et al., 2021). However, examination of tissues from SCTLD affected corals by transmission electron microscopy suggests that SCTLD may be a viral infection of zooxanthellae that leads to coral host cell death (Work, 2021: see also Correa et al., 2016). Viral origins of WPD have been previously ascribed for Orbicella spp. in the Caribbean (see Soffer et al., 2014).
Whatever the root cause, the reality is that the ongoing SCTLD epizootic that started off in Miami-Dade County in 2014 has changed the community structure and potentially the ecosystem dynamics of the entire Florida reef tract (Aeby et al., 2017; Hayes et al., 2017; Lunz et al., 2017; Neely, 2018; Ruzicka, 2018; Walker, 2018; Sharp et al., 2020; Jones et al., 2021; Williams et al., 2021) and is now affecting reefs at sites throughout the Caribbean (AGRRA, 2021). Given the high SCTLD-based mortality rates observed in this study and across Florida as well as the current diminished capacity for coral recovery regionwide (Moulding, 2005; Smith et al., 2013; van Woesik et al., 2014), the future of reef-building stony coral communities in Florida and the Caribbean is unsettling to say the least.
The initial outbreak of SCTLD in Southeast Florida is arguably one of the most lethal coral disease events ever recorded on a contemporary coral reef system. In a few short years, the ecological extirpation of many key species has fundamentally changed the way these reefs look and function (Precht et al., 2016; Walton et al., 2018; Gintert et al., 2019; Jones et al., 2021; Neely et al., 2021a). The resulting community shift caused by SCTLD is just the last in a series of regional coral mortality events responsible for a multi-decadal trend of reefs being dominated by a few small, highly ephemeral, stress-tolerant species (Green et al., 2008; Burman et al., 2012; Smith et al., 2013; Toth et al., 2014, 2019; Estrada-Saldívar et al., 2019). While ballast water from ships has been hypothesized to spread the disease (see Dahlgren et al., 2021), the results of SCTLD outbreaks from sites across the Caribbean currently show no direct link between local anthropogenic factors on the initiation of the disease or on the ultimate impact (prevalence and mortality) of the disease on the local coral communities. As noted above, some nearshore coral communities in Florida appear to show greater resilience to SCTLD than their offshore counterparts (Rippe et al., 2019). This is potentially the result of increased nutrient concentrations, turbidity, chlorophyll, and temperature variability seen in inshore reef and hardbottom environments (Lirman and Fong, 2007) that may convey increased coral fitness to stressors including those responsible for SCTLD (Kolodziej et al., 2021; Rubin et al., 2021). However, the composition of the coral community present in terms of susceptible vs. non-susceptible species appears to have the greatest control on the ultimate outcome and impact on the resultant coral population at any given site. While local threats such as sedimentation from dredging (Erftemeijer et al., 2012) can add stress to a coral community already overwhelmed by a combination of stressors both natural and anthropogenic in origin, mitigating local stressors while critically important (Lamb et al., 2016), will be not be sufficient to save what remains of our unique coral resources if we do not focus on the more immediate threat to the survivorship of corals by working tirelessly to address the existential threat posed by regional and global stressors including coral bleaching and disease.
Nomenclature
Resource Identification Initiative
(R Project for Statistical Computing, RRID:SCR_001905) version #1.2.5042
(tidyverse, RRID:SCR_019186) version #1.3.0
(dplyr, RRID:SCR_016708) version #0.8.3
(ggplot2, RRID:SCR_014601) version #3.2.1
(CRAN, RRID:SCR_003005) version #3.6.2
(R package: lmerTest, RRID:SCR_015656) version #3.1-2
(R package: lme4, RRID:SCR_015654) version #1.1-23.
Data Availability Statement
All monitoring field data sheets, underwater videos and photographs collected as well as the analysis and agency reports prepared by DCA as part of the environmental permit compliance for the Port Miami Phase III Deepening Project are in the public domain and can be obtained through the USACE (https://www.saj.usace.army.mil/Missions/Civil-Works/Navigation/Navigation-Projects/Miami-Harbor-Deepening/) and the FDEP (https://floridadep.gov/south/sd-outreach/documents/using-oculus-locate-public-records). The datasets generated and analyzed for this study can be found in the svvollmer/Frontiers_MiamiSurvival repository on GitHub at https://github.com/svvollmer/Frontiers_MiamiSurvival Further inquiries can be directed to the corresponding author.
Author Contributions
SV and WP conceptualized the initial study. RS and SV designed the study using a publicly available data set, performed and validated the statistical analysis. RF and WP assisted in the collection of the original data, validated causes of coral mortality with weekly in situ coral condition assessments and their corresponding underwater photographs. RF provided summary tables of the data for QA/QC. RS, SV, and WP wrote the manuscript. All authors reviewed and approved the final version of the manuscript.
Funding
Initial funding for the Port Miami data collection was supported to the environmental consulting firm Dial Cordy and Associates, Inc. (DCA), in-part, under contracts to Great Lakes Dredge and Dock Company, LLC sponsored by the United States Army Corps of Engineers (USACE), Jacksonville District (2013–2015) and Port Miami, Miami-Dade County (2014–2017) for environmental compliance monitoring and analysis under Florida Department of Environmental Protection (FDEP) Permit No.0305721-001-BI. The funders had no role in data collection and analysis, decision to publish, or preparation of this manuscript. The authors received no compensation for publishing this study. SV received funding from the Horvitz Foundation to study the impacts of SCTLD. NSF funding (NSF-OCE-1924145) to SV also helped facilitate this research.
Conflict of Interest
RF and WP were employed by the company Dial Cordy and Associates, Inc.
The remaining authors declare that the research was conducted in the absence of any commercial or financial relationships that could be construed as a potential conflict of interest.
Publisher’s Note
All claims expressed in this article are solely those of the authors and do not necessarily represent those of their affiliated organizations, or those of the publisher, the editors and the reviewers. Any product that may be evaluated in this article, or claim that may be made by its manufacturer, is not guaranteed or endorsed by the publisher.
Acknowledgments
Thank you to Molly Albecker and Chuck Roesel for their help in navigating the statistical analysis used in this article. RS would like to thank the Northeastern University Three Seas program and all the staff and professors who made this article possible. This manuscript is the outgrowth of a research project performed by RS and supervised by SV in partial fulfillment of the requirements of the Master of Science degree in Marine Biology at Northeastern University. We would like to thank the Horvitz foundation for their generous support to SV for SCTLD related disease research. RF and WP would like to thank to Alison Conner, Jeff Coward, Jason and Laura Croop, Brooke Gintert, Herve Jobert, Christina Marmet, Lindsey and Madison Precht, Martha Robbart, Kristian Rogers, Alex Schroeder, Tyler Shelley, and Christina Vilmar for assistance with scientific diving, data collection, and analysis. Mike Rice prepared Figure 1. The views, statements, findings, and conclusions expressed herein are those of the authors and do not necessarily reflect the views of GLDD, the USACE, Port Miami, Miami-Dade County, or Northeastern University. This article is dedicated to the memory of Walt Jaap. Walt was committed to understanding and protecting the reefs of Florida. He was the first scientist to document the effects of coral bleaching in Florida and throughout his storied career he watched the very reefs he lived to protect vanish before his eyes. This manuscript, like Walt’s classic review of Florida’s reefs (Jaap, 1984) was written “to all who seek the truth.” We can think of no better tribute to Walt’s legacy.
Supplementary Material
The Supplementary Material for this article can be found online at: https://www.frontiersin.org/articles/10.3389/fmars.2021.723998/full#supplementary-material
Footnotes
- ^ https://www.frontiersin.org/research-topics/16501/stony-coral-tissue-loss-disease-in-the-caribbean
References
Aeby, G. S., Ushijima, B., Campbell, J. E., Jones, S., Williams, G. J., Meyer, J. L., et al. (2019). Pathogenesis of a tissue loss disease affecting multiple species of corals along the Florida Reef Tract. Front. Mar. Sci. 6:678. doi: 10.3389/fmars.2019.00678
Aeby, G., Ruzicka, R., and Bohnsack, K. (2017). Status and Trends from A Large-Scale Coral Disease Outbreak. 38th Meeting of the US Coral Reef Task Force. Available online at: https://coralreef.Gov/meeting38/docs/status_lessons_learned_coral_disease_outbreak.pdf (accessed May 30, 2021).
AGRRA (2013). AGRRA Training Aids. Available online at: http://www.agrra.org/method/trainingid.html (accessed October 04, 2013).
AGRRA (2016). Datasheets For Coral Reef Assessment Using AGRRA Protocol. AGRRA. Available online at: https://www.agrra.org/training-tools/agrra-datasheets/ (accessed August 18, 2021)
AGRRA (2021). Coral Disease Outbreak. AGRRA. Available online at: http://www.agrra.org/coral-disease-outbreak/ (accessed August 18, 2021)
Ainsworth, T., and Hoegh-Guldberg, O. (2009). Bacterial communities closely associated with coral tissues vary under experimental and natural reef conditions and thermal stress. Aquat. Biol. 4, 289–296. doi: 10.3354/ab00102
Alvarez-Filip, L., Estrada-Saldívar, N., Pérez-Cervantes, E., Molina-Hernández, A., and González-Barrios, F. J. (2019). A rapid spread of the stony coral tissue loss disease outbreak in the Mexican Caribbean. PeerJ 7:e8069. doi: 10.7717/peerj.8069
Aronson, R. B., and Precht, W. F. (2001). White-band disease and the changing face of Caribbean coral reefs. Hydrobiol. 460, 25–38. doi: 10.1007/978-94-017-3284-0_2
Aronson, R. B., and Precht, W. F. (2009). Sustaining ecosystem services in the global coral reef crisis. AIP Conferen. Proc. 1157:48. doi: 10.1063/1.3208031
Aronson, R. B., and Precht, W. F. (2016). “Physical and biological drivers of coral-reef dynamics,” in Coral Reefs at the Crossroads. Coral Reefs of the World, eds D. Hubbard, C. Rogers, J. Lipps, and G. Stanley Jr. (Dordrecht: Springer), 261–275. doi: 10.1007/978-94-017-7567-0_11
Bates, D., Maechler, M., Bolker, B., and Walker, S. (2007). CRAN - Package Lme4. CRAN. Available online at: https://cran.r-project.org/web/packages/lme4/index.html (accessed May 30, 2021).
Blair, S. M., and Flynn, B. S. (1989). “Biological monitoring of hard bottom reef communities off Dade County Florida: community description,” in Proceedings American Academy Underwater Sciences 9th Annual Scientific Diving Symposium, 9–24.
Borger, J. (2005). Scleractinian coral diseases in south Florida: incidence, species susceptibility, and mortality. Dis. Aquat. Org. 67, 249–258. doi: 10.3354/dao067249
Brandt, M. E., and McManus, J. W. (2009). Disease incidence is related to bleaching extent in reef-building corals. Ecol. 90, 2859–2867. doi: 10.1890/08-0445.1
Brodnicke, O. B., Bourne, D. G., Heron, S. F., Pears, R. J., Stella, J. S., Smith, H. A., et al. (2019). Unravelling the links between heat stress, bleaching and disease: fate of tabular corals following a combined disease and bleaching event. Coral Reefs 38, 591–603. doi: 10.1007/s00338-019-01813-9
Bruno, J. F., Selig, E. R., Casey, K. S., Page, C. A., Willis, B. L., Harvell, C. D., et al. (2007). Thermal stress and coral cover as drivers of coral disease outbreaks. PLoS Biol. 5:e124. doi: 10.1371/journal.pbio.0050124
Budd, A. F., Fukami, H., Smith, N. D., and Knowlton, N. (2012). Taxonomic classification of the reef coral family Mussidae (Cnidaria: Anthozoa: Scleractinia). Zool. Jour. Linn. Soc. 166, 465–529. doi: 10.1111/j.1096-3642.2012.00855.x
Burge, C. A., Mark Eakin, C., Friedman, C. S., Froelich, B., Hershberger, P. K., Hofmann, E. E., et al. (2014). Climate change influences on marine infectious diseases: implications for management and society. Annu. Rev. Mar. Sci. 6, 249–277. doi: 10.1146/annurev-marine-010213-135029
Burman, S., Aronson, R., and van Woesik, R. (2012). Biotic homogenization of coral assemblages along the Florida reef tract. Mar. Ecol. Prog. Ser. 467, 89–96. doi: 10.3354/meps09950
Burns, T. P. (1985). Hard-coral distribution and cold-water disturbances in South Florida: variation with depth and location. Coral Reefs 4, 117–124. doi: 10.1007/bf00300870
Chung, Y., Rabe-Hesketh, S., Dorie, V., Gelman, A., and Liu, J. (2013). A nondegenerate penalized likelihood estimator for variance parameters in multilevel models. Psychometrika 78, 685–709. doi: 10.1007/s11336-013-9328-2
Coastal Systems International Inc (2016). Transplanted corals to artificial reefs—24 month post-transplant monitoring report, Prepared for Miami Harbor Phase III Federal Channel Expansion. Contract No. E12-SEA-03/Project No. 1999-027. Miami, FL: PortMiami.
Correa, A. M. S., Ainsworth, T. D., Rosales, S. M., Thurber, A. R., Butler, C. R., and Vega Thurber, R. L. (2016). Viral outbreak in corals associated with an in situ bleaching event: atypical herpes-like viruses and a new megavirus infecting Symbiodinium. Front. Microbiol 7:127. doi: 10.3389/fmicb.2016.00127
Cowan, C. (2006). Coral Bleaching and Disease: Recovery and Mortality on Martinique Reefs Following the 2005 Caribbean Bleaching Events. M.sc. thesis. Newcastle upon Tyne: University of Newcastle upon Tyne.
Cróquer, A., and Weil, E. (2009). Changes in Caribbean coral disease prevalence after the 2005 bleaching event. Dis. Aquat. Org. 87, 33–43. doi: 10.3354/dao02164
Cunning, R., Silverstein, R. N., Barnes, B. B., and Baker, A. C. (2019). Extensive coral mortality and critical habitat loss following dredging and their association with remotely-sensed sediment plumes. Mar. Poll. Bull. 145, 185–199. doi: 10.1016/j.marpolbul.2019.05.027
Dahlgren, C., Pizarro, V., Sherman, D., Greene, W., and Oliver, J. (2021). Spatial and temporal patterns of Stony Coral Tissue Loss Disease (SCTLD) outbreaks in The Bahamas. Front. Mar. Sci. 8:682114. doi: 10.3389/fmars.2021.682114
Department of Parks and Natural Resources, USVI (2019). Stony Coral Tissue Loss Disease: Coral Disease with High Mortality Found on Coral Reefs on St. Thomas, USVI. Available online at: https://dpnr.vi.gov/czm/sctld/ (accessed May 31, 2021).
Dial Cordy and Associates Inc (2010). Miami Harbor Hardbottom Assessment Pilot Study and Quantitative Study Plan. Jacksonville: Technical Memorandum Prepared for U.S. Army Corps of Engineers.
Dial Cordy and Associates Inc (2012). Miami Harbor Baseline Hardbottom Study. Jacksonville: U.S. Army Corps of Engineers.
Dial Cordy and Associates Inc (2015a). Quantitative Post-Construction Analysis for Hardbottom Benthic Communities, PortMiami Phase III Federal Channel Expansion Project. Florida Department of Environmental Protection, Tallahassee, FL. Florida Department of Environmental Protection. Available online at: http://www.saj.usace.army.mil/Portals/44/docs/Navigation/Ports/Miami%20Harbor/Near-ShoreHard-BottomReportNov2015.pdf. (accessed August 18, 2021)
Dial Cordy and Associates Inc (2015b). Quantitative Post-Construction Analysis for Middle and Outer Reef Benthic Communities, FDEP Final Order #0305721-001-BI. 2012, PortMiami Phase III Federal Channel Expansion Project. Florida Department of Environmental Protection, Tallahassee, FL. Florida Department of Environmental Protection. Available online at: http://www.saj.usace.army.mil/Portals/44/docs/Navigation/Ports/Miami%20Harbor/MiddleandOuterReefsReportNov2015.pdf. (accessed August 18, 2021)
Dial Cordy and Associates Inc (2017). One-Year Post-Construction Impact Assessment for Hardbottom Middle and Outer Reef Benthic Communities at Permanent Sites FDEP Final Order #0305721-001-BI. PortMiami Phase III Federal Channel Expansion Project. Tallahassee, FL: Florida Department of Environmental Protection (FDEP). Available online at: http://www.saj.usace.army.mil/Portals/44/docs/Planning/EnvironmentalBranch/EnvironmentalDocs/Dade/PortMiami_Permanent_Site_Report_04_24_17.pdf?ver=2017-05-16-080604-047. (accessed August 18, 2021)
Dobbelaere, T., Muller, E. M., Gramer, L. J., Holstein, D. M., and Hanert, E. (2020). Coupled epidemio-hydrodynamic modeling to understand the spread of a deadly coral disease in Florida. Front. Mar. Sci. 7:591881. doi: 10.3389/fmars.2020.591881
Eakin, C. M., Morgan, J. A., Heron, S. F., Smith, S., Liu, G., Álvarez-Filip, L., et al. (2010). Caribbean corals in crisis: record thermal stress, bleaching, and mortality in 2005. PLoS One 5:e13969. doi: 10.1371/journal.pone.0013969
Edge, S. E., Shearer, T. L., Morgan, M. B., and Snell, T. W. (2013). Sub-lethal coral stress: detecting molecular responses of coral populations to environmental conditions over space and time. Aqu. Toxicol. 128, 135–146. doi: 10.1016/j.aquatox.2012.11.014
Erftemeijer, P. L. A., Riegl, B., Hoeksema, B. W., and Todd, P. A. (2012). Environmental impacts of dredging and other sediment disturbances on corals: a review. Mar. Poll. Bull. 64, 1737–1765. doi: 10.1016/j.marpolbul.2012.05.008
Estrada-Saldívar, N., Jordán-Dalhgren, E., Rodríguez-Martínez, R. E., Perry, C., and Alvarez-Filip, L. (2019). Functional consequences of the long-term decline of reef-building corals in the Caribbean: evidence of across-reef functional convergence. R. Soc. Open Sci. 6:190298. doi: 10.1098/rsos.190298
Estrada-Saldívar, N., Molina-Hernández, A., Pérez-Cervantes, E., Medellín-Maldonado, F., González-Barrios, F. J., and Alvarez-Filip, L. (2020). Reef-scale impacts of the stony coral tissue loss disease outbreak. Coral Reefs 39, 861–866. doi: 10.1007/s00338-020-01949-z
Florida Coral Disease Response Research, and Epidemiology Team. (2018). Case Definition: Stony Coral Tissue Loss Disease. Available online at: https://floridadep.gov/sites/default/files/Copy%20of%20StonyCoralTissueLossDisease_CaseDefinition%20final%2010022018.pdf (accessed July 24, 2021).
Florida Department Environmental Protection (2012). Environmental Resource Permit, Miami Harbor Phase III Federal Channel Expansion Project - FDEP Permit No. 0305721-001-BI. Available online at: https://www.saj.usace.army.mil/Portals/44/docs/Planning/EnvironmentalBranch/EnviroCompliance/Miami%20Harbor%20Final%20Order%20052212%20508%20compliant.pdf (accessed May 31, 2021)
Fox, J., and Weisberg, S. (2018). An R Companion to Applied Regression. Thousand Oaks, CA: SAGE Publications.
Gignoux-Wolfsohn, S. A., Precht, W. F., Peters, E. C., Gintert, B. E., and Kaufman, L. S. (2020). Ecology, histopathology, and microbial ecology of a white-band disease outbreak in the threatened staghorn coral Acropora cervicornis. Dis. Aqua.Org. 137, 217–237. doi: 10.3354/dao03441
Gilliam, D. S. (2012). Southeast Florida Coral Reef Evaluation and Monitoring Project 2011 Year 9 Final Report. Florida DEP Report #RM085. Miami Beach, FL: Florida Department of Environmental Protection (FDEP).
Gilliam, D. S., Brinkhuis, C. J., Ruzicka, R., and Colella, M. (2015). Southeast Florida Coral Reef Evaluation and Monitoring Project 2014-year 12 Final Report. Florida DEP Report #RM085. Miami Beach, FL: Florida Department of Environmental Protection (FDEP).
Gilliam, D. S., Hayes, N. K., Ruzicka, R., and Colella, M. (2018). Southeast Florida Coral Reef Evaluation and Monitoring Project 2017, Year 15 Executive Summary. Florida DEP Report #RM143. Miami Beach, FL: Florida Department of Environmental Protection (FDEP).
Gilliam, D. S., Walton, C. J., Brinkhuis, V., Ruzicka, R., and Hayes, N. K. (2016). Southeast Florida Coral Reef Evaluation and Monitoring Project 2015, Year 13 Executive Summary. Florida DEP Report #RM143. Miami Beach, FL: Florida Department of Environmental Protection (FDEP).
Ginsburg, R. N. (1956). Environmental relationships of grain size and constituent particles in some South Florida carbonate sediments. AAPG Bull. 40, 2384–2427. doi: 10.1306/5ceae598-16bb-11d7-8645000102c1865d
Gintert, B. E., Precht, W. F., Fura, R., Rogers, K., Rice, M., Precht, L. L., et al. (2019). Regional coral disease outbreak overwhelms impacts from a local dredge project. Environ. Monit. Assess. 191:630. doi: 10.1007/s10661-019-7767-7
Goldberg, W. M. (1973). The ecology of the coral-octocoral communities off the southeast Florida coast: geomorphology, species composition, and zonation. Bull. Mar. Sci. 23, 465–488.
Green, D., Edmunds, P., and Carpenter, R. (2008). Increasing relative abundance of Porites astreoides on Caribbean reefs mediated by an overall decline in coral cover. Mar. Ecol. Prog. Ser. 359, 1–10. doi: 10.3354/meps07454
Harding, S., van Bochove, J. W., Day, O., Gibson, K., and Raines, P. (2008). “Continued degradation of Tobago’s coral reefs linked to the prevalence of coral disease following the 2005 mass coral bleaching event,” in Proceedings of the 11th International Coral Reef Symposium, (Ft. Lauderdale, FL), 738–741.
Harrell, F. E. Jr. (2021). CRAN - Package Hmisc. CRAN. Available online at: https://cran.r-project.org/web/packages/Hmisc/index.html (accessed May 31, 2021).
Harvell, D., Jordán-Dahlgren, E., Merkel, S., Rosenberg, E., Raymundo, L., Smith, G., et al. (2007). Coral disease, environmental drivers, and the balance between coral and microbial associates. Oceanog. 20, 172–195. doi: 10.5670/oceanog.2007.91
Hawaii Department of Land and Natural Resources (2015). Corals at Kaneohe Bay now Impacted by Disease. Hawaii Department of Land and Natural Resources Press Release, February 12, 2015. Available online at: http://dlnr.hawaii.gov/blog/2015/02/12/nr15-023/. (accessed August 2, 2021)
Hayes, N. K., Walton, C. J., Brinkhuis, V., Ruzika, R., and Gilliam, D. S. (2017). SECREMP Southeast Florida Coral Reef Evaluation and Monitoring Project. FDEP-CRCP 2017. Florida Department of Environmental Protection (FDEP) - Florida Coastal Office. Available online at: https://www.youtube.com/watch?v=3Aj_VIOhJQo&list=PLraw0H6njzME6cabdw1vRg7 (accessed May 31, 2021)
Heres, M. M., Farmer, B. H., Elmer, F., and Hertler, H. (2021). Ecological consequences of Stony Coral Tissue Loss Disease in the Turks and Caicos Islands. Coral Reefs 40, 609–624. doi: 10.1007/s00338-021-02071-4
Heron, S. F., Willis, B. L., Skirving, W. J., Eakin, C. M., Page, C. A., and Miller, I. R. (2010). Summer hot snaps and winter conditions: modelling white syndrome outbreaks on Great Barrier Reef corals. PLoS One 5:e12210. doi: 10.1371/journal.pone.0012210
Hoegh-Guldberg, O., and Bruno, J. F. (2010). The impact of climate change on the world’s marine ecosystems. Science 328, 1523–1528. doi: 10.1126/science.1189930
Intergovernmental Panel on Climate Change (2013). Summary for Policymakers. In: Climate Change 2013: e Physical Science Basis. Contribution of Working Group I to the Fifth Assessment Report of the Intergovernmental Panel on Climate Change. Available online at: https://www.ipcc.ch/site/assets/uploads/2018/03/WG1AR5_SummaryVolume_FINAL.pdf (accessed May 31, 2021).
Iwanowicz, D. D., Schill, W. B., Woodley, C. M., Bruckner, A., Neely, K., and Briggs, K. M. (2020). Exploring the stony coral tissue loss disease bacterial pathobiome. bioRxiv [Preprint] doi: 10.1101/2020.05.27.120469
Jaap, W. C. (1984). The Ecology of the South Florida Coral Reefs: A Community Profile. FWS OBS-82/08 and MMS 84–0038. St. Petersburg, FL: Florida Department of Natural Resources, Marine Research Lab.
Jones, N. P., Kabay, L., Lunz, K. S., and Gilliam, D. S. (2021). Temperature stress and disease drives the extirpation of the threatened pillar coral, Dendrogyra cylindrus, in southeast Florida. Sci. Rep. 11:14113. doi: 10.1038/s41598-021-93111-0
Kassambara, A., Kosinski, M., and Biecek, P. (2021). Package Survminer. CRAN. Available online at: https://cran.r-project.org/web/packages/survminer/index.html (accessed May 31, 2021).
Kennedy, C. (2014). 2014 Surprisingly Rough on Coral Reefs, and El Niño Looms in 2015 | NOAA Climate.gov. Climate.gov. Available online at: https://www.climate.gov/news-features/featured-images/2014-surprisingly-rough-coral-reefs-and-el-ni%C3%B1o-looms-2015 (accessed June 1, 2021)
Kline, D., and Vollmer, S. (2011). White band disease (Type I) of endangered Caribbean acroporid corals is caused by pathogenic bacteria. Sci. Rep. 1:7. doi: 10.1038/srep00007
Kolodziej, G., Studivan, M. S., Gleason, A. C. R., Langdon, C., Enochs, I. C., and Manzello, D. P. (2021). Impacts of stony coral tissue loss disease (SCTLD) on coral community structure at an inshore patch reef of the upper Florida Keys using photomosaics. Front. Mar. Sci. 8:682163. doi: 10.3389/fmars.2021.682163
Kourafalou, V. H., and Kang, H. (2012). Florida Current meandering and evolution of cyclonic eddies along the Florida Keys Reef Tract: are they interconnected? J. Geophys. Res. 117:5028. doi: 10.1029/2011jc007383
Lamb, J. B., Wenger, A. S., Devlin, M. J., Ceccarelli, D. M., Williamson, D. H., and Willis, B. L. (2016). Reserves as tools for alleviating impacts of marine disease. Phil. Trans. R. Soc. B 371:20150210. doi: 10.1098/rstb.2015.0210
Landsberg, J. H., Kiryu, Y., Peters, E. C., Wilson, P. W., Perry, N., Waters, Y., et al. (2020). Stony coral tissue loss disease in Florida is associated with disruption of host–zooxanthellae physiology. Front. Mar. Sci. 7:576013. doi: 10.3389/fmars.2020.576013
Lesser, M. P., Bythell, J. C., Gates, R. D., Johnstone, R. W., and Hoegh-Guldberg, O. (2007). Are infectious diseases really killing corals? Alternative interpretations of the experimental and ecological data. J. Exp. Mar. Biol. Ecol. 346, 36–44. doi: 10.1016/j.jembe.2007.02.015
Lewis, C. L., Neely, K. L., Richardson, L. L., and Rodriguez-Lanetty, M. (2017). Temporal dynamics of black band disease affecting pillar coral (Dendrogyra cylindrus) following two consecutive hyperthermal events on the Florida Reef Tract. Coral Reefs 36, 427–431. doi: 10.1007/s00338-017-1545-1
Lighty, R. G., Macintyre, I. G., and Stuckenrath, R. (1978). Submerged early Holocene barrier reef south-east Florida shelf. Nature 276, 59–60. doi: 10.1038/276059a0
Lirman, D., and Fong, P. (2007). Is proximity to land-based sources of coral stressors an appropriate measure of risk to coral reefs? An example from the Florida Reef Tract. Mar. Poll. Bull. 54, 779–791. doi: 10.1016/j.marpolbul.2006.12.014
Lunz, K., Landsberg, J., Kiryu, Y., and Brinkhuis, V. (2017). Investigation of the Coral Disease Outbreak Affecting Scleractinian Coral Species Along the Florida Reef Tract. Florida Department of Environmental Protection (FDEP). Available online at: https://floridadep.gov/sites/default/files/FWRI-Diseases-Report.pdf (accessed May 31, 2021).
Manzello, D. P. (2015). Rapid recent warming of coral reefs in the Florida Keys. Sci. Rep. 5:16762. doi: 10.1038/srep16762
Manzello, D. P., Berkelmans, R., and Hendee, J. C. (2007). Coral bleaching indices and thresholds for the Florida reef tract, Bahamas, and St. Croix, US Virgin Islands. Mar. Poll. Bull. 54, 1923–1931. doi: 10.1016/j.marpolbul.2007.08.009
Mao-Jones, J., Ritchie, K. B., Jones, L. E., and Ellner, S. P. (2010). How microbial community composition regulates coral disease development. PLoS Biol. 8:e1000345. doi: 10.1371/journal.pbio.1000345
Marszalek, D. S. (1982). “Impact of dredging on a subtropical reef community, southeast Florida, U.S.A,” in Proceedings of the 4th International Coral Reef Symposium, (Manilla), 147–154.
Marszalek, D. S., Babashoff, G., Noel, M. R., and Worley, D. R. (1977). “Reef distribution in south Florida,” in Proceedings of the 3rd International Coral Reef Symposium, (Miami), 223–229.
Martin, C. (2019). A Mysterious Coral Disease Is Ravaging Caribbean Reefs. Science News. Available online at: https://www.sciencenews.org/article/mysterious-coral-disease-ravaging-caribbean-reefs (accessed May 30, 2021)
Maynard, J., van Hooidonk, R., Eakin, C. M., Puotinen, M., Garren, M., Williams, G., et al. (2015). Projections of climate conditions that increase coral disease susceptibility and pathogen abundance and virulence. Nat. Clim. Change 5, 688–694. doi: 10.1038/nclimate2625
Meiling, S., Muller, E. M., Smith, T. B., and Brandt, M. E. (2020). 3D photogrammetry reveals dynamics of stony coral tissue loss disease (SCTLD) lesion progression across a thermal stress event. Front. Mar. Sci. 7:597643. doi: 10.3389/fmars.2020.597643
Merselis, D. G., Lirman, D., and Rodriguez-Lanetty, M. (2018). Symbiotic immuno-suppression: is disease susceptibility the price of bleaching resistance? PeerJ 6:e4494. doi: 10.7717/peerj.4494
Meyer, J. L., Castellanos-Gell, J., Aeby, G. S., Häse, C. C., Ushijima, B., and Paul, V. J. (2019). Microbial community shifts associated with the ongoing stony coral tissue loss disease outbreak on the Florida reef tract. Front. Microbiol. 10:2244. doi: 10.3389/fmicb.2019.02244
Miami-Dade County Department Environmental Resource Management (2016). 24-Month Monitoring of Scleractinians Relocated from Government Cut to A Natural Reef Recipient Site In Association with the Phase 3 Port of Miami/Government Cut Deepening Project. Resolution R-422-12, Schedule A: Monitoring Project 1. Miami-Dade County Department of Regulatory and Economic Resources, Division of Environmental Resources Management (DERM), Restoration and Enhancement Section. Miami, FL: Division of Environmental Resources Management (DERM).
Miller, C. V., May, L. A., Moffitt, Z. J., and Woodley, C. M. (2020). Exploratory treatments for stony coral tissue loss disease: pillar coral (Dendrogyra cylindrus). Charleston, SC: NOAA Technical Memorandum NOS NCCOS 245 and CRCP 37, 78. doi: 10.7289/V5/TM-NOS-NCCOS-245
Miller, J., Muller, E., Rogers, C., Waara, R., Atkinson, A., Whelan, K. R. T., et al. (2009). Coral disease following massive bleaching in 2005 causes 60% decline in coral cover on reefs in the US Virgin Islands. Coral Reefs 28, 925–937. doi: 10.1007/s00338-009-0531-7
Miller, M. W., Karazsia, J., Groves, C. E., Griffin, S., Moore, T., Wilber, P., et al. (2016). Detecting sedimentation impacts to coral reefs resulting from dredging the Port of Miami, Florida USA. PeerJ 4:e2711. doi: 10.7717/peerj.2711
Muller, E. M., Bartels, E., and Baums, I. B. (2018). Bleaching causes loss of disease resistance within the threatened coral species Acropora cervicornis. eLife 7:e35066. doi: 10.7554/elife.35066.028
Muller, E. M., Rogers, C. S., Spitzack, A. S., and van Woesik, R. (2008). Bleaching increase likelihood of disease on Acropora palmata (Lamarck) in Hawksnest Bay, St. John, US Virgin Islands. Coral Reefs 27, 191–195. doi: 10.1007/s00338-007-0310-2
Muller, E. M., Sartor, C., Alcaraz, N. I., and van Woesik, R. (2020). Spatial epidemiology of the Stony-Coral-Tissue-Loss Disease in Florida. Front. Mar. Sci. 7:163. doi: 10.3389/fmars.2020.00163
Mydlarz, L. D., Couch, C. S., Weil, E., Smith, G., and Harvell, C. D. (2009). Immune defenses of healthy, bleached and diseased Montastraea faveolata during a natural bleaching event. Dis. Aquat. Org. 87, 67–78. doi: 10.3354/dao02088
National Marine Fisheries Service (2016). Examination of Sedimentation Impacts to Coral Reef Along the Port of Miami Entrance Channel, December 2015 – NOAA’s National Marine Fisheries Service Final Report. St. Petersburg, FL: NOAA National Marine Fisheries Service, Southeast Region.
National Oceanic Atmospheric Administration (2014). Coral Reef Watch Satellite Monitoring and Modeled Outlooks. NOAA Coral Reef Watch. Available online at: https://www.coralreefwatch.noaa.gov/satellite/index.php (accessed June 1, 2021)
National Oceanic Atmospheric Administration (2015a). 2014-16 Bleaching Event Continues June 2015 Update. NOAA Coral Watch. Available online at: https://coralreefwatch.noaa.gov/satellite/analyses_guidance/global_bleaching_update_20150602.php (accessed June 1, 2021).
National Oceanic Atmospheric Administration (2015b). NOAA Coral Reef Watch – 2014 annual summaries of thermal conditions related to coral bleaching for U.S. National Coral Reef Monitoring Program (NCRMP) jurisdictions. Available online at: https://coralreefwatch.noaa.gov/satellite/analyses_guidance/2014_annual_summaries_thermal_stress_conditions_NCRMP.pdf (accessed May 31, 2021).
National Oceanic Atmospheric Administration (2021a). Station VAKF1 – Virginia Key Station, Virginia Key, FL, Coastal Marine Automated Network, National Data Buoy Center. Available online at: http://www.ndbc.noaa.gov/station_page.php?station=vakf1. (accessed June 21, 2021)
National Oceanic Atmospheric Administration (2021b). NOAA. Station FWYF1 – Fowey Rock, FL, Coastal Marine Automated Network, National Data Buoy Center. Available online at: https://www.ndbc.noaa.gov/station_page.php?station=fwyf1. (accessed June 21, 2021)
Neely, K. (2018). Surveying the Florida Keys Southern Coral Disease Boundary. Miami, FL: Florida Department of Environmental Protection (FDEP).
Neely, K. L., Lewis, C. L., Lunz, K. S., and Kabay, L. (2021a). Rapid population decline of the pillar coral Dendrogyra cylindrus along the Florida Reef Tract. Front. Mar. Sci. 8:656515. doi: 10.3389/fmars.2021.656515
Neely, K. L., Macaulay, K. A., Hower, E. K., and Dobler, M. A. (2020). Effectiveness of topical antibiotics in treating corals affected by Stony Coral Tissue Loss Disease. PeerJ 8:e9289. doi: 10.7717/peerj.9289
Neely, K. L., Shea, C., Macaulay, K. A., Hower, E. K., and Dobler, M. A. (2021b). Short and long-term effectiveness of coral disease treatments. Front. Mar. Sci. 8:675349. doi: 10.3389/fmars.2021.675349
Palmer, C. V., McGinty, E. S., Cummings, D. J., Smith, S. M., Bartels, E., and Mydlarz, L. D. (2011). Patterns of coral ecological immunology: variation in the responses of Caribbean corals to elevated temperature and a pathogen elicitor. Jour. Exp. Biol. 214, 4240–4249. doi: 10.1242/jeb.061267
Peters, E. C., and Fogarty, N. D. (2016). Data Collection for the Southeast Florida Action Network (SEAFAN) to Assess Reef Conditions Before and During the 2014-2015 Coral Disease Outbreak. Florida Department of Environmental Protection (FDEP) Available online at: https://repository.library.noaa.gov/view/noaa/14351 (accessed May 31, 2021).
Pinzón, J. H., Kamel, B., Burge, C. A., Harvell, C. D., Medina, M., Weil, E., et al. (2015). Whole transcriptome analysis reveals changes in expression of immune-related genes during and after bleaching in a reef-building coral. R. Soc. Open Sci. 2:140214. doi: 10.1098/rsos.140214
Porter, J. W., Dustan, P., Jaap, W. C., Patterson, K. L., Kosmynin, V., Meier, O. W., et al. (2001). Patterns of spread of coral disease in the Florida Keys. Hydrobiol. 460, 1–24.
Precht, L., Thanner, S., Peters, E., Rogers, K., Kaufman, L., Aronson, B., et al. (2018). Emerging Coral Disease Threatens Ailing Caribbean Reefs. ECO Environment, Coastal and Marine. Available online at: https://www.ecomagazine.com/news/coasts/emerging-coral-disease-threatens-ailing-caribbean-reefs (accessed May 31, 2021)
Precht, W. F. (2021). Failure to respond to a coral disease epizootic in Florida: causes and consequences. ReEco 6, 1–47. doi: 10.3897/rethinkingecology.6.56285
Precht, W. F., and Aronson, R. B. (2004). Climate flickers and range shifts of reef corals. Front. Ecol. Env. 2:307–314. doi: 10.1890/1540-9295(2004)002[0307:cfarso]2.0.co;2
Precht, W. F., and Miller, S. L. (2007). “Ecological Shifts along the Florida reef tract: the past as a key to the future,” in Geological Approaches to Coral Reef Ecology, ed. R. B. Aronson (New York, NY: Springer), 237–312. doi: 10.1007/978-0-387-33537-7_9
Precht, W. F., Fura, R., Croop, J., and Robbart, M. L. (2021). Corals survive the burial stress of seasonally shifting sands. Reef Encoun. 36, 51–54.
Precht, W. F., Gintert, B. E., Robbart, M. L., Fura, R., and van Woesik, R. (2016). Unprecedented disease-related coral mortality in Southeastern Florida. Sci. Rep. 6:31374. doi: 10.1038/srep31374
Precht, W. F., Gintert, B., Fura, R., Rogers, K., Robbart, M., and Dial, S. (2019). “Miami harbor deep dredge project: a reappraisal reveals same results,” in Proceedings of the WEDA Dredging Summit & Expo ‘19, (Chicagi, IL).
Randall, C. J., Jordan-Garza, A. G., Muller, E. M., and van Woesik, R. (2014). Relationships between the history of thermal stress and the relative risk of diseases of Caribbean corals. Ecology 95, 1981–1994. doi: 10.1890/13-0774.1
Reed, K., Muller, E., and van Woesik, R. (2010). Coral immunology and resistance to disease. Dis. Aquat. Org. 90, 85–92. doi: 10.3354/dao02213
Richardson, L. L., and Aronson, R. B. (2002). “Infectious diseases of reef corals,” in Proceedings of the 9th International Coral Reef Symposium, (Bali), 1225–1230.
Rippe, J. P., Kriefall, N. G., Davies, S. W., and Castillo, K. D. (2019). Differential disease incidence and mortality of inner and outer reef corals of the upper Florida Keys in association with a white syndrome outbreak. Bull. Mar. Sci. 95, 305–316. doi: 10.5343/bms.2018.0034
Rogers, C. S., Muller, E., Spitzack, T., and Miller, J. (2009). Extensive coral mortality in the US Virgin Islands in 2005/2006: a review of the evidence for synergy among thermal stress, coral bleaching and disease. Caribb. J. Sci. 45, 204–214. doi: 10.18475/cjos.v45i2.a8
Rosales, S. M., Clark, A. S., Huebner, L. K., Ruzicka, R. R., and Muller, E. M. (2020). Rhodobacterales and Rhizobiales are associated with stony coral tissue loss disease and its suspected sources of transmission. Front. Microbiol. 11:681. doi: 10.3389/fmicb.2020.00681
Rubin, E. T., Enochs, I. C., Foord, C., Mayfield, A. B., Kolodziej, G., Basden, I., et al. (2021). Molecular mechanisms of coral persistence within highly urbanized locations in the Port of Miami, Florida. Front. Mar. Sci. 8:695236. doi: 10.3389/fmars.2021.695236
Ruzicka, R. (2018). Brief Overview and Preliminary Synopsis of Coral Loss Due to the Coral Disease Outbreak in SE FL and Florida Keys – CREMP & SECREMP Results. Available online at: https://floridadep.gov/sites/default/files/FRT-Coral-Disease-Call-10-Summary.pdf. (accessed August 18, 2021).
Searle, S. R., Speed, F. M., and Milliken, G. A. (1980). Population marginal means in the linear model: an alternative to least squares means. Am. Statist. 34, 216. doi: 10.2307/2684063
Sharp, W. C., Shea, C. P., Maxwell, K. E., Muller, E. M., and Hunt, J. H. (2020). Evaluating the small-scale epidemiology of the stony-coral -tissue-loss-disease in the middle Florida Keys. PLoS One 15:e0241871. doi: 10.1371/journal.pone.0241871
Shilling, E. N., Combs, I. R., and Voss, J. D. (2021). Assessing the effectiveness of two intervention methods for stony coral tissue loss disease on Montastraea cavernosa. Sci. Rep. 11:8566. doi: 10.1038/s41598-021-86926-4
Sinigalliano, C. D., Enochs, I. C., Stamates, S. J., Jones, P. R., Featherstone, C. M., Gidley, M. L., et al. (2019). Water Quality and Coral Reef Monitoring Along the Southeast Florida Coast. NOAA Technical Report, OAR-AOML-47. Available online at: https://repository.library.noaa.gov/view/noaa/22011 (accessed May 31, 2021).
Smith, T. B., Brandt, M. E., Calnan, J. M., Nemeth, R. S., Blondeau, J., Kadison, E., et al. (2013). Convergent mortality responses of Caribbean coral species to seawater warming. Ecosphere 4:art87. doi: 10.1890/es13-00107.1
Soffer, N., Brandt, M. E., Correa, A. M., Smith, T. B., and Thurber, R. V. (2014). Potential role of viruses in white plague coral disease. ISME J. 8, 271–283. doi: 10.1038/ismej.2013.137
Sokolow, S. (2009). Effects of a changing climate on the dynamics of coral infectious disease: a review of the evidence. Dis. Aquat. Org. 87, 5–18. doi: 10.3354/dao02099
Staletovich, J. (2017). Miami’s Sewage is Supposed to be Pumped Offshore but the Pipe has Sprung a Leak. Miami Herald – Environment (July 31, 2017). Available online at: https://www.miamiherald.com/news/local/environment/article164655777.html (accessed August 8, 2021)
Staley, C., Kaiser, T., Gidley, M. L., Enochs, I. C., Jones, P. R., Goodwin, K. D., et al. (2017). Differential impacts of land-based sources of pollution on the microbiota of Southeast Florida coral reefs. Appl. Env. Microb. 83, e3378–e3316.
Stathakopoulos, A., and Riegl, B. M. (2015). Accretion history of mid-Holocene coral reefs from the southeast Florida continental reef tract, USA. Coral Reefs 34, 173–187. doi: 10.1007/s00338-014-1233-3
Stoddart, J., Jones, R., Page, C., Marnane, M., De Lestang, P., and Elsdon, T. (2019). No effect of dredging on the prevalence of coral disease detected during a large dredging program. Mar. Poll. Bull. 140, 353–363. doi: 10.1016/j.marpolbul.2019.01.047
Sweet, M. J., Cróquer, A., and Bythell, J. C. (2014). Experimental antibiotic treatment identifies potential pathogens of white band disease in the endangered Caribbean coral Acropora cervicornis. Proc. R. Soc. B 281:20140094. doi: 10.1098/rspb.2014.0094
Talbot, R. (2019). As Disease Ravages Coral Reefs, Scientists Scramble for Solutions. Yale E360. Available online at: https://e360.yale.edu/features/as-disease-ravages-coral-reefs-scientists-scramble-for-solutions (accessed May 31, 2021).
Therneau, T. M., and Lumley, T. (2021). Package Survival. CRAN. Available online at: https://cran.r-project.org/web/packages/survival/index.html (accessed May 31, 2021).
Thome, P. E., Rivera-Ortega, J., Rodríguez-Villalobos, J. C., Cerqueda-García, D., Guzmán-Urieta, E. O., García-Maldonado, J. Q., et al. (2021). Local dynamics of a white syndrome outbreak and changes in the microbial community associated with colonies of the scleractinian brain coral Pseudodiploria strigosa. PeerJ 9:e10695. doi: 10.7717/peerj.10695
Toth, L. T., Precht, W. F., Modys, A., Stathakopoulos, A., Robbart, M. L., Hudson, J. H., et al. (2021). Climate and the latitudinal limits of subtropical reef development. Sci. Rep. 11:13044. doi: 10.1038/s41598-021-87883-8
Toth, L. T., Stathakopoulos, A., Kuffner, I. B., Ruzicka, R. R., Colella, M. A., and Shinn, E. A. (2019). The unprecedented loss of Florida’s reef−building corals and the emergence of a novel coral−reef assemblage. Bull. Ecol. Soc. Am. 100:e01615. doi: 10.1002/bes2.1615
Toth, L. T., van Woesik, R., Murdoch, T. J. T., Smith, S. R., Ogden, J. C., Precht, W. F., et al. (2014). Do no-take reserves benefit Florida’s corals? 14 years of change and stasis in the Florida Keys National Marine Sanctuary. Coral Reefs 33, 565–577. doi: 10.1007/s00338-014-1158-x
US Army Corps of Engineers (2021). Miami Harbor Deepening. Available online at: https://www.saj.usace.army.mil/Missions/Civil-Works/Navigation/Navigation-Projects/Miami-Harbor-Deepening/ (accessed August 18, 2021)
Ushijima, B., Meyer, J. L., Thompson, S., Pitts, K., Marusich, M. F., Tittl, J., et al. (2020). Disease diagnostics and potential coinfections by Vibrio coralliilyticus during an ongoing coral disease outbreak in Florida. Front. Microbiol. 11:569354. doi: 10.3389/fmicb.2020.569354
van Woesik, R., Scott, W. J., and Aronson, R. B. (2014). Lost opportunities: Coral recruitment does not translate to reef recovery in the Florida Keys. Mar. Poll. Bull. 88, 110–117. doi: 10.1016/j.marpolbul.2014.09.017
Vaughan, T. W. (1914). Investigations of the geology and geologic processes of the reef tracts and adjacent areas of the Bahamas and Florida. Carnegie Inst. Washington Yearb. 12, 1–183.
Vaughan, T. W. (1918). The temperature of the Florida coral reef tract. Carnegie Inst. Washington Publ. 213, 321–339.
Walker, B. K. (2012). Spatial analyses of benthic habitats to define coral reef ecosystem regions and potential biogeographic boundaries along a latitudinal gradient. PLoS One 7:e30466. doi: 10.1371/journal.pone.0030466
Walker, B. K. (2018). Southeast Florida Reef-Wide Post-Irma Coral Disease Surveys. Florida DEP. Miami. Available online at: https://floridadep.gov/sites/default/files/Southeast-Florida-Reef-Wide-Post-Irma-Coral-Disease-Surveys_0.pdf (accessed May 31, 2021).
Walker, B. K., Turner, N. R., Noren, H. K. G., Buckley, S. F., and Pitts, K. A. (2021). Optimizing stony coral tissue loss disease (SCTLD) intervention treatments on Montastraea cavernosa in an endemic zone. Front. Mar. Sci. 8:666224. doi: 10.3389/fmars.2021.666224
Walton, C. J., Hayes, N. K., and Gilliam, D. S. (2018). Impacts of a regional, multi-year, multi-species coral disease outbreak in southeast Florida. Front. Mar. Sci. 5:323. doi: 10.3389/fmars.2018.00323
Ward, J., Kim, K., and Harvell, C. (2007). Temperature affects coral disease resistance and pathogen growth. Mar. Ecol. Prog. Ser. 329, 115–121. doi: 10.3354/meps329115
Warrender, T., McCoy, C., Panton, C., Fletcher, C., Ebanks, K., Precht, W. F., et al. (2021). Monitoring the progression of stony coral tissue loss disease (SCTLD) in the Cayman Islands. Front. Mar. Sci (in-press),Google Scholar
Wei, T., and Simko, V. (2021). Package Corrplot. CRAN. Available online at: https://cran.r-project.org/web/packages/corrplot/index.html (accessed May 31, 2021).
Whitall, D., Bricker, S., Cox, D., Baez, J., Stamates, J., Gregg, K., et al. (2019). Southeast Florida Reef Tract Water Quality Assessment. NOAA Technical Memoradum NOS NCCOS 271. Silver Spring. Available online at: https://repository.library.noaa.gov/view/noaa/22999 (accessed August 18, 2021).
Williams, S. D., Walter, C. S., and Muller, E. M. (2021). Fine scale temporal and spatial dynamics of the stony coral tissue loss disease outbreak within the lower Florida Keys. Front. Mar. Sci. 8:631776. doi: 10.3389/fmars.2021.631776
Work, T. M. (2021). Final Report on Electron Microscopy of Florida Corals Affected with Stony Coral Tissue Loss Disease (SCTLD). Final Report, Florida Department of Environmental Protection, Coral Protection and Restoration Program (PO# B8E83B). Tallahassee, FL.
World Meterological Organization. (2020). State of the Global Climate 2020 – Provisional Report. Available online at: https://library.wmo.int/index.php?lvl=notice_display&id=21804#.YRa9DYhKhPY (accessed August 18, 2021).
Keywords: bleaching, white plague disease, stony coral tissue loss disease, sedimentation, anthropogenic stressors, climate change, ocean warming
Citation: Spadafore R, Fura R, Precht WF and Vollmer SV (2021) Multi-Variate Analyses of Coral Mortality From the 2014–2015 Stony Coral Tissue Loss Disease Outbreak Off Miami-Dade County, Florida. Front. Mar. Sci. 8:723998. doi: 10.3389/fmars.2021.723998
Received: 11 June 2021; Accepted: 20 August 2021;
Published: 20 September 2021.
Edited by:
Aldo Cróquer, The Nature Conservancy, Dominican RepublicReviewed by:
Karen Lynn Neely, Nova Southeastern University, United StatesPedro L. Ardisson, Center for Research and Advanced Studies – Mérida Unit, Mexico
Copyright © 2021 Spadafore, Fura, Precht and Vollmer. This is an open-access article distributed under the terms of the Creative Commons Attribution License (CC BY). The use, distribution or reproduction in other forums is permitted, provided the original author(s) and the copyright owner(s) are credited and that the original publication in this journal is cited, in accordance with accepted academic practice. No use, distribution or reproduction is permitted which does not comply with these terms.
*Correspondence: Rachele Spadafore, Rachele.spadafore@gmail.com