Sea Urchin Removal as a Tool for Macroalgal Restoration: A Review on Removing “the Spiny Enemies”
- Leigh Marine Laboratory, University of Auckland, Leigh, New Zealand
Kelp and macroalgal forests provide the ecological foundations of many temperate rocky reef ecosystems, but have regionally declined, often due to sea urchin overgrazing and the formation of urchin barrens. Sea urchin removal has long been used to investigate kelp-sea urchin dynamics and is increasingly being promoted for kelp forest restoration. In this review, we assess the methods and outcomes of sea urchin removal experiments to evaluate their potential use and feasibility as a tool for restoring macroalgal forests. Seventy-nine sea urchin removal projects were reviewed from temperate subtidal rocky reef systems between 1975 and 2020. Removal methods were often not reported (35%), but included manual culling, including crushing (25%) and chemical application (quicklime, 9%), or relocating sea urchins (13%). Only a small percentage of removals were large in scale (16% > 10 ha) and 92% of these utilized culling. Culling is often the most practical method of urchin removal, but all methods can be effective and we encourage development of new approaches that harvest and utilize low-quality urchins. Urchin removal led to an increase in macroalgae in 70% of studies, and a further 21% showed partial increases (e.g., at one or more sites or set of conditions). Restoration effectiveness is increased by removing essentially all sea urchins from discrete areas of urchin barrens. Sea urchin removal provides a simple, relatively cheap, and effective method that promotes kelp recovery within urchin barrens. However, sea urchin removal does not address the underlying cause of elevated sea urchin populations and is unlikely to provide a long-term solution to restore kelp forests and full ecosystem function on its own. We therefore suggest that if sea urchin removal is considered as a tool for kelp forest restoration, it should be incorporated with other management measures that aim to increase kelp forest resilience and biodiversity (e.g., marine protected areas, predator protection or enhancement). This will ensure that kelp restoration efforts have the greatest ecological, socio-economic and cultural outcomes in the long-term.
Introduction
“I will briefly summarize our labors by saying that the aforesaid Quicklime did grievously smite the spiny enemies of Your glorious empire in such a fashion that most of them perished as they so richly deserved.”—Brock Bernstein Letter of Transmittal to Queen Elizabeth II, 30 October 1981, cover letter of Bernstein and Welsford (1982).
Kelp forests frame roughly a quarter of Earth's temperate and subpolar rocky coastlines (Steneck and Johnson, 2014), but these ecosystems can literally be eaten away by sea urchins which create urchin barrens. Reaching up to 60 m long, kelps form the foundation of temperate reef ecosystems by creating complex three-dimensional habitats that support high biodiversity (Graham, 2004; Teagle et al., 2017). They form some of the most productive ecosystems on the planet (Mann, 1973) and provide food and habitat for a plethora of species both within their canopies (Graham, 2004; Christie et al., 2009) and in neighboring areas (Duggins et al., 1989). Kelp forests also provide numerous regulatory, provisionary, and cultural services with large economic value, including carbon sequestration and nutrient remediation, coastal protection, enhanced fisheries and tourism, and harvestable food and materials for sustenance and trade (Bennett et al., 2016; Wernberg et al., 2019). When these macroalgal forests are lost, less productive and structurally simple states such as urchin barrens or algal turfs are frequently left in their wake, with ecological and socio-economic ramifications (Graham, 2004; Filbee-Dexter and Wernberg, 2018).
Declines in kelp and macroalgal forests in recent decades have been well-studied and reviewed (Filbee-Dexter and Scheibling, 2014; Ling et al., 2015; Krumhansl et al., 2016; Wernberg et al., 2019). While kelp has increased in some regions, regional declines, like the 90% kelp loss over 350 km in Northern California, USA, are more prevalent and have had catastrophic local effects (Rogers-Bennett and Catton, 2019). The degradation and loss of kelp forests can be driven by a number of abiotic (e.g., warming, eutrophication, and sedimentation; Airoldi, 2003; Strain et al., 2014; Smale, 2020) and biotic (e.g., herbivory and disease) factors (Cole and Babcock, 1996; Vergés et al., 2014). In mid-latitudes, where kelps are not at the limit of their abiotic tolerances, overabundance and overgrazing by sea urchins are the most important and widely reported cause of kelp forest decline (Steneck et al., 2002; Filbee-Dexter and Scheibling, 2014; Ling et al., 2015; Steneck, 2020). Unlike other kelp herbivores, sea urchins have the unique ability to completely eliminate macroalgae, in some cases from vast areas of reef, and then maintain these areas clear of macroalgae for decades (Norderhaug and Christie, 2009; Watanuki et al., 2010; Filbee-Dexter and Scheibling, 2014). In many cases, the overabundance of sea urchins has been linked to the historic overharvest of their predators (Jackson et al., 2001; Steneck et al., 2002; Steneck, 2020).
Roughly 20 species of sea urchins are known to control macroalgal community composition and influence ecosystem function through grazing (Paine, 1980; Steneck, 2020). High urchin densities have denuded macroalgal forests in Asia, Australasia, Europe, and the Americas (Ling et al., 2015; Krumhansl et al., 2016; Filbee-Dexter and Wernberg, 2018), leading to their epithet “spiny enemies.” For example, in California where purple urchin (Strongylocentrotus purpuratus) densities have increased exponentially (Rogers-Bennett and Catton, 2019) and in Tasmania where the long-spine sea urchin (Centrostephanus rodgersii) has extended its range (Ling, 2008), kelp forests have succumbed to grazing and sea urchins are widely considered undesirable. However, some species of sea urchins are regarded highly, with great commercial and cultural importance as traditional foods and delicacies (Andrew et al., 2002); their roe (“uni”) can fetch market prices as high as USD$100/kg (Sun and Chiang, 2015). Indeed, the “culprit” sea urchin can be seen as both a pest species and a cherished food, and the removal of these sea urchins for kelp restoration purposes can be a sensitive issue.
Once sea urchin densities increase and kelp or macroalgae decrease past critical points, it can be difficult to reverse phase changes. The kelp forest to urchin barrens model is one of the most common examples of phase shifts, documented over the last 50 years (Lawrence, 1975; Filbee-Dexter and Scheibling, 2014). These systems demonstrate “hysteresis,” or an imbalance of the tipping point threshold. Globally averaged, the urchin biomass required to shift from kelp forests to barrens is an order of magnitude greater than the biomass threshold to shift back to kelp forests (668 ± 115 g/m2 compared to 71 ± 20 g/m2; Ling et al., 2015). In other words, once high densities of urchins consume macroalgae and create barrens, small numbers of urchins can maintain the barrens. For kelp to recover, urchin populations must be reduced to very low levels.
One might assume that once the increased sea urchin populations have depleted their locally preferred food source, the population would decrease (through death or emigration). However, sea urchins demonstrate physiological and dietary plasticity, whereby individuals can make metabolic and behavioral adjustments, or switch to alternative foods (e.g., drift algae, turfing algae, invertebrates, detritus), when preferred food is scarce (Lawrence, 1975; Ling and Johnson, 2009; Suskiewicz and Johnson, 2017). Despite survival of sea urchins in depauperate barrens (Andrew, 1989), their general condition is often poorer, as demonstrated by reduced body size, shrunken gonads (roe) and changes in skeletal morphology (Claisse et al., 2013; Spyksma et al., 2017; Pert et al., 2018; Ling et al., 2019). Thus, barren areas may persist for decades (Jackson et al., 2001; Steneck et al., 2002) with high densities of sea urchins unsuitable for harvest.
As urchin barrens have replaced kelp and macroalgal forests in many regions, concern has grown about the transition of a highly productive system to an apparent desert. This has led to a rapid increase in kelp forest restoration initiatives in recent years, which is highlighted in recent reviews (Eger et al., 2020; Layton et al., 2020; Morris et al., 2020) and increasing research efforts (Figure 1). While several approaches can be used for kelp forest restoration (Box 1), where sea urchins are a primary cause of deforestation (Watanuki et al., 2010; Ling et al., 2015; Guarnieri et al., 2020), sea urchin removal provides an obvious approach to kelp restoration.
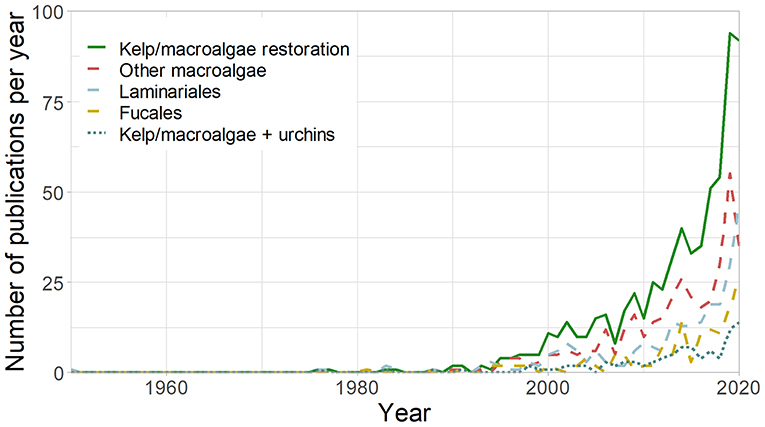
Figure 1. Number of studies published per year on kelp and macroalgal restoration (TOPIC [kelp*OR macroalga*] AND [restor*]), kelp restoration (TOPIC [kelp*OR laminaria*] AND [restor*]), Fucales restoration (TOPIC [fucoid*or fucal*] AND [restor*]), other macroalgal restoration (TOPIC [kelp*OR macroalga*] AND [restor*] NOT [kelp*or laminaria*or fucoid*or fucal*]), and restoration and sea urchins (TOPIC: [kelp*OR macroalga*] AND [restor*] AND [urchin*OR echinoderm*]) in Web of Science between 1950 and 31/12/2020.
Box 1. General methods of active macroalgal restoration.
Herbivore removal. While herbivory by fishes and molluscs can influence macroalgal communities, sea urchins are by far the most destructive grazers of macroalgae and kelp forests (Steneck et al., 2002; Filbee-Dexter and Scheibling, 2014; Ling et al., 2015; Steneck, 2020). Consequently, herbivore removal efforts have focused on sea urchin removal. Dedicated urchin removal to restore kelp forests has been used for over half a century (Leighton et al., 1966; Breen and Mann, 1976) and in many regions (e.g., California, Norway, Canary Islands, Canada, Australia). If overgrazing by urchins is the primary reason for macroalgae decline, active removals (crushing/collecting) or urchin predator addition can allow macroalgae to regrow.
Kelp enhancement. Enhancement includes seeding, transplanting juveniles, adults, fragments, or substrate with attached kelp (Wilson and North, 1983; Westermeier et al., 2016; Fredriksen et al., 2020; Medrano et al., 2020). Transplanting can be quite costly and labor intensive and success is varied (Wilson and North, 1983; Layton et al., 2020), but may be necessary if propagules are limited.
Removal of algal competition. Clearing competing species, such as invasive algae, can allow regrowth of desired species, but effective removal is very difficult (Wilson and North, 1983; Hewitt et al., 2005). Turf (or mat-forming algae) inhibit the kelp recruitment through restricting space and accumulating sediment (Connell et al., 2014; Strain et al., 2014; Filbee-Dexter and Wernberg, 2018). Unlike urchin barrens, large-scale phase shifts from turfs back to kelp are rare (Filbee-Dexter and Wernberg, 2018; Christie et al., 2019).
Artificial habitat addition. Additions of rocks (and more recently concrete) to increase reef habitat for kelp enhancement has been conducted for centuries in Japan (Fujita, 2011). In California, several projects were constructed for environmental mitigation from power plants, with mixed results (Carter et al., 1985; Ambrose, 1994; Reed et al., 2017).
Environmental mitigation or adaptation. Environmental degradation (e.g., temperature, sedimentation, eutrophication) can act additively or synergistically (Strain et al., 2014), and reducing local stressors (e.g., sediment or nutrient loads) may increase resilience to other stressors (Strain et al., 2015). With climate change, restoration to former species assemblages may be impossible and enhancing more temperature tolerant species or “assisted evolution” may be required (Filbee-Dexter and Smajdor, 2019; Coleman et al., 2020).
Urchin populations may be reduced through direct removal or indirect methods (Layton et al., 2020). Direct removals, the focus of this review, are active restoration, whereas indirect (or passive) methods involve management interventions such as marine protected areas that may indirectly lead to declines in sea urchins over time (Box 2). Direct removal of sea urchins may provide a simple and rapid (<2 years) approach to restoring kelp forests (Ling et al., 2010, 2015). However, in some cases additional strategies may also be required to promote kelp recovery (Box 1) and provide longer-term resilience of kelp forests (Box 2). If urchin removal is being considered as part of a kelp restoration plan, it is necessary to understand:
• Can and when does urchin removal restore macroalgal forests?
• Is it feasible and/or practical for large-scale macroalgal restoration?
• What is the potential role of sea urchin removal in macroalgal forest restoration?
Box 2. Natural, indirect or assisted processes to control sea urchin populations.
Protection of sea urchin predators. Trophic cascades describe the top-down effect of predators on prey species and their associated food resources (Paine, 1980; Ripple et al., 2016). For example, Estes and Palmisano (1974) correlated the reintroduction of sea otters (Enhydra lutris) with declines in sea urchins (Strongylocentrotus spp.) and increases of kelp at the Aleutian Islands. If sea urchin populations are regulated by predation (top-down), then restoring predator populations can decrease urchin densities and restore kelp forests, provided urchin densities fall below the tipping point (Shears and Babcock, 2002, 2003; Ling et al., 2009). Many sea urchin predators are heavily exploited and trophic cascades are linked to their overfishing or harvest. However, because of the socio-economic value of fishing, gaining public and/or political support for protection of these predator species can be challenging. Restoring trophic cascades are considered passive/indirect approaches to restoration and can potentially be achieved through the following measures:
1. Marine protected areas. No-take marine protected areas or marine reserves can restore populations of heavily fished species (Russ and Alcala, 1996; McClanahan et al., 2007; Lester et al., 2009; Stewart et al., 2009). Trophic cascades resulting in kelp restoration occurred in the Cape Rodney-Okakari Point (Goat Island) and Tāwharanui Marine Reserves, in north-eastern New Zealand, after decades of protection. In these reserves, Australasian snapper (Chrysophrys auratus) and spiny lobster (Jasus edwardsii) populations dramatically increased, sea urchin (kina, Evechinus chloroticus) populations declined and kelp canopies re-established, while urchin barrens remained outside of the reserves (Babcock et al., 1999; Shears and Babcock, 2002). Restoration of kelp forests following marine reserve establishment has been demonstrated elsewhere (Behrens and Lafferty, 2004; Edgar et al., 2009), though in some cases of marine protection, kelp restoration has not been an outcome (Sala et al., 1998; Micheli et al., 2005), demonstrating the regionally disparate and variable mechanisms for kelp loss (Shears et al., 2008).
2. Predator enhancement. Restoration of predators can restore trophic cascades and support regrowth of kelp forests (Duggins, 1980; Watson and Estes JStability, 2011). In Tasmania, Johnson et al. (2013) translocated 1,663 large lobsters into barrens; in newly forming (incipient) barrens, a significant increase in macroalgae was seen, but no change was seen in extensive fully-formed barrens after 2.5 years (Johnson et al., 2013).
3. Fishery management. Reduced fishing quotas, spatial exclusion or size restrictions on predator species can increase predator abundance and size. Success of urchin predation depends on predator density and their efficiency at consuming urchins. For example, only very large lobsters can consume large urchins (Ling et al., 2009) and lobster prefer urchins from kelp forests than from barrens (Eurich et al., 2014). Models suggest that reducing fishing pressure may be insufficient to restore existing barrens in Tasmania (Marzloff et al., 2016; Johnson et al., 2017). Due to hysteresis, predator density may need to be impossibly high to induce phase shifts, thus meaning that after urchin populations have skyrocketed, increased predation alone may be unable to solve the issue.
This review assesses the goals, methods, and success of direct sea urchin removal projects in relation to ecological viability and implementation practicality. The aim is to evaluate the effectiveness and utility of different methods and to identify the most useful applications of sea urchin removal to guide future macroalgal restoration projects.
Methods
Web of Science and Google Scholar (1900-31/12/2020) were used to search through literature on urchin and kelp dynamics published in English. The criteria in Web of Science (graz* or herbiv* or barren* or troph*) AND (urchin* or echinod* or echinoid*) AND (kelp* or macroalga* or alga* or seaweed*) AND (remov* or exclus* or restor* or kill*) provided a broad source of published literature (391 records), and “urchin removal restoration” for Google Scholar (17,300 records, first 400 screened) which were examined for relevance, along with their citations and reference lists (Supplementary Figure 1). The search was supplemented with projects in gray literature (e.g., technical reports), sourced through a general Google search (“urchin removal restoration,” first 400 screened) and reference lists in relevant literature. Search criteria included active removals or exclusions of sea urchins from urchin barrens and/or from macroalgal dominated subtidal environments on rocky substrate (e.g., passive indirect restoration such as marine reserves were excluded).
Studies must have measured effects of removal of all or a portion of sea urchins on macroalgae abundance and/or urchin density. Studies that only examined effects of urchin removal on other species (e.g., abalone, sponge) were excluded. Urchin removal studies on coral reefs, soft sediments (e.g., seagrass beds) or artificial substrates (e.g., settlement tiles) were also excluded. Projects involving canopy-forming macroalgae other than kelp (e.g., in the Mediterranean) were included.
In southern California, many urchin removal projects conducted by Wheeler J. North in the 1960s and 1970s are summarized in Wilson and North (1983). For the purposes of this review, these are grouped by method, as individual projects typically had only limited descriptions of relevant details. Additionally, studies from Japan are underrepresented due to many studies being published only in Japanese, which ignores an enormous wealth of information and a large knowledge gap unavailable to non-Japanese speakers. One Japanese literature review reports 1,000 studies conducted between 1970 and 2005 on barren grounds, with 43% of these on algal restoration (Kuwahara et al., 2006). However, Eger et al. (2021b) reviews Japanese literature on kelp restoration more broadly.
Metrics sought from each study included: location, purpose, experiment start date, macroalgal density, maximum plot size, total removal area, duration of monitoring, dependent variables examined, marine protection, time and/or cost estimates, urchin densities or biomass, total number of urchins removed, removal method and personnel details, and removal frequency. One author (KM) extracted and interpreted these data from each report. In cases where metrics were not clearly described and/or there was uncertainty in interpretation of these data, these were discussed and interpreted in consultation with other authors.
The purpose of each project was determined based on the stated research goal and experiments conducted. Five main objectives were considered: (1) ecological role: understanding the role of sea urchin on macroalgae/community composition, (2) multiple stressors (biotic): the relative role of sea urchins + another species on macroalgae/community (e.g., two species of sea urchin or sea urchins and herbivorous fishes), (3) multiple stressors (abiotic): the relative role of sea urchins + abiotic factors (or abiotic and biotic, such as sea urchins + sedimentation or sea urchins + nutrification) on macroalgae/community, (4) fishery effects: studying the effects of urchin removal on urchins or abalone, and (5) restoration purposes (including removal effectiveness).
Success was determined by growth of macroalgae and excluded studies that only tested for changes in urchin densities. Macroalgae regrowth was recorded in different ways (e.g., percent canopy cover, number of plants, biomass) so the response was simplified to: significant macroalgae increase, partial increase, or no increase. These were determined if the statistical tests conducted by researchers showed a significant (p < 0.05) increase in kelp cover in all sites/experiments, partial if significant positive results were only found in some sites or experiments (e.g., depth, different levels of removals, sites, or times), or no statistically significant increase determined in any sites. If no statistical analyses were conducted, positive, partial, or no increase trends were determined from the results. Date refers to start date of removal, and maximum plot size was the largest discrete area of urchin removal, even if this was larger than the monitored site. Summary statistics represent only the number of studies that reported each metric. Analyses and maps were conducted in Microsoft Excel, QGIS Version 3.12.0, and R Version 4.0.0 (QGIS Development Team, 2020; R Core Team, 2020).
Results
Research Location and Purpose
Location
Most sea urchin removal studies (60 of 79 studies) were carried out in just five countries: Australia, USA, Italy, Canada and Japan (Figure 2), and weighted toward the Northern Hemisphere (55 of 79 studies, Supplementary Table 1). Several areas known to have urchin barrens and phase shifts (Filbee-Dexter and Scheibling, 2014) are not represented: Greenland and Iceland, Russia, Greece, and much of the United Kingdom. Japan is underrepresented in this review as many projects were not published in English. Most of the studies were focused in regions where laminarian kelps occur (63 studies), whereas 16 studies were carried out outside the range of shallow water laminarians and focused on habitats dominated by fucoid or other macroalgal species (Mediterranean: 15 studies and Canary Islands: 1 study).
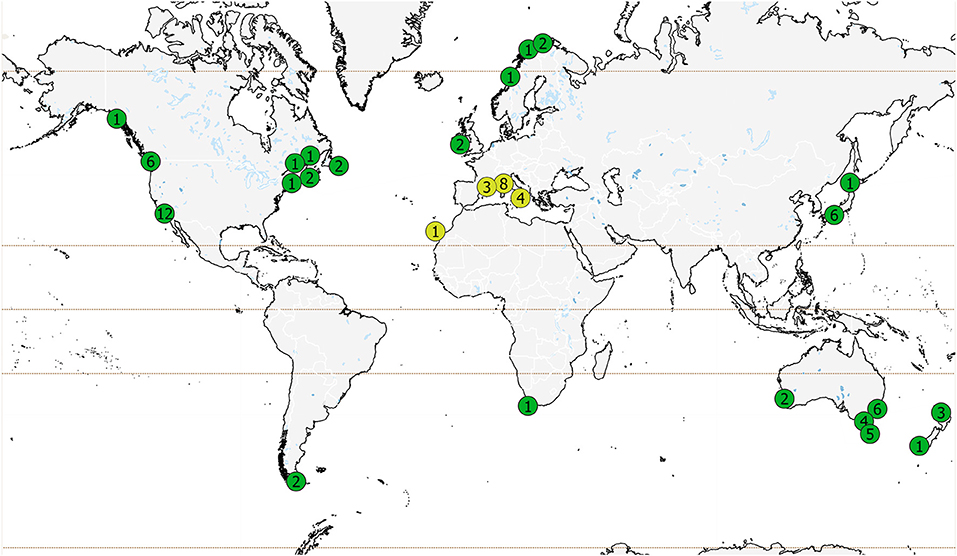
Figure 2. Global distribution of published studies on sea urchin removal. Number of studies within each area are shown; in some cases, multiple studies (differentiated by area, time, or method) could be published within a single report. Green circles represent regions where laminarian kelps occur (63 studies) and yellow circles represent studies outside of the range of shallow water laminarians (dominated by fucoid or other macroalgal species, 16 studies). Note the number of studies in Japan and California is an underrepresentation of the total number of studies in these regions (see text).
The spatial distribution of studies is also indicative of research sites and institutions, not the global distribution of urchin barrens. Twelve studies were conducted at least partially within marine protected areas, nine of which were for experimental purposes and three for restoration.
Research Purpose
The number of sea urchin removal studies published each decade has generally increased since the 1950s, with the primary purpose changing through time (Figure 3). Most studies focused on understanding the ecological effects of sea urchins on macroalgae and benthic communities (49%), followed by restoration (25%). Early studies primarily focused on understanding the ecological role of sea urchins, but the relative proportion of these studies have declined relative to other research interests. In particular, the research into multiple stressors (biotic, relative importance of different herbivores [14%]; abiotic + abiotic/biotic, the relative influence of sea urchins and other factors [7%]) emerged in the 1980s and is increasing. Studies utilizing urchin removal for restoration purposes have occurred through time but increased in recent decades.
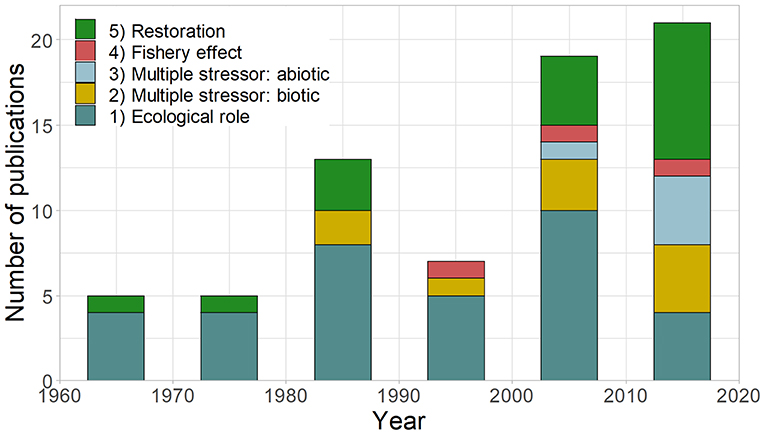
Figure 3. Decadal number and purpose of published urchin removal studies (n = 71, excluding duplicate studies or studies with missing dates): (1) ecological role: understanding the role of sea urchin on macroalgae/community composition, (2) multiple stressors (biotic): the relative role of sea urchins + another species on macroalgae/community, (3) multiple stressors (abiotic): the relative role of sea urchins + abiotic factors on macroalgae/community, (4) fishery effects: studying the effects of urchin removal on urchins or abalone, and (5) restoration purposes (including removal effectiveness). The year used for each study represents the start of urchin removals rather than publication date.
Study Design
Sea Urchin Density Reduction
Almost all studies attempted to completely remove sea urchins within an area, but removal effectiveness varied greatly. Many studies did not report sea urchin density before and after removals, but five studies reported a reduction of <75% and were considered partial removals. Eight studies also compared complete removals with reducing densities of sea urchins to a specific amount (e.g., 33 or 50% reduction).
Size
The maximum plot size and total removal area were generally related to study objectives (Figure 4, n = 73 and n = 74, respectively). Larger removal plots (>1,000 m2) were typically used in restoration projects, whereas smaller plots were used for ecological research (objectives 1–3). The largest scale study removed urchins from 1,000,000 m2 of reef for restoration, but overall area included both incipient barrens (barrens forming within kelp forest) and kelp habitat (Gorfine et al., 2012); the total area of barrens cleared was substantially smaller and not measured.
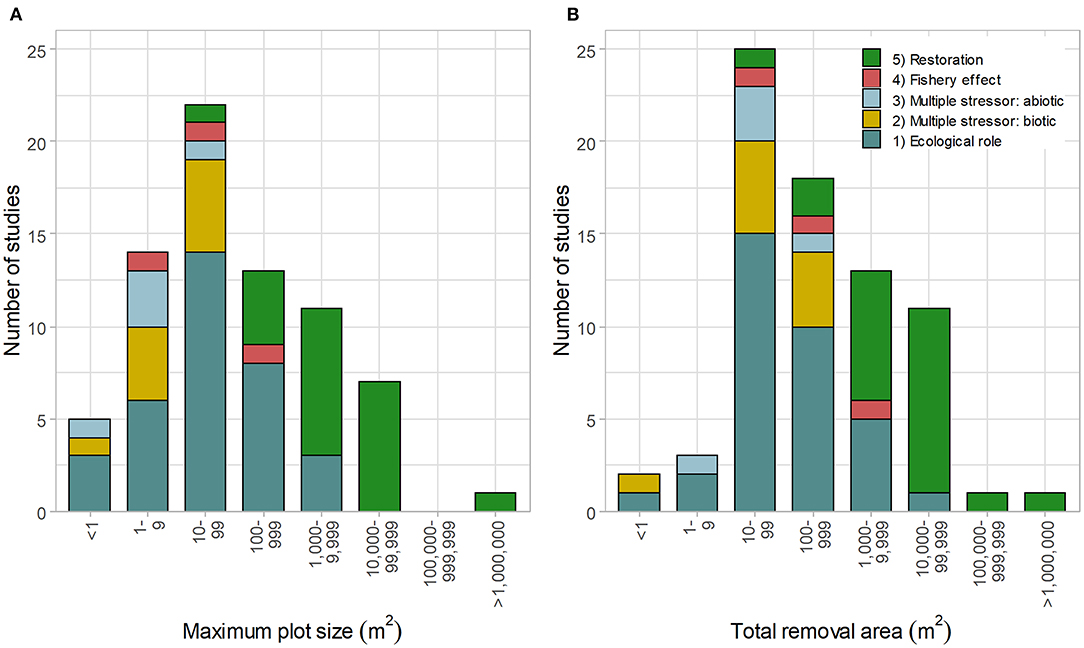
Figure 4. Size of (A) maximum urchin removal plot (n = 73) and (B) total urchin removal area (n = 74) relative to study objectives: (1) ecological role: understanding the role of sea urchin on macroalgae/community composition, (2) multiple stressors (biotic): the relative role of sea urchins + another species on macroalgae/community, (3) multiple stressors (abiotic): the relative role of sea urchins + abiotic factors on macroalgae/community, (4) fishery effects: studying the effects of urchin removal on urchins or abalone, and (5) restoration purposes (including removal effectiveness).
Only 13 of the 79 studies investigated the effects of urchin removal on large-scales (>1 ha [10,000 m2], Figure 4), with five of these projects reported in a single publication (Taino, 2010). In total, only four peer-reviewed papers were published on urchin removal projects larger than one hectare: Taino (2010), Sanderson et al. (2016), Guarnieri et al. (2020), and Williams et al. (2021).
Removal Frequency
Given the majority of studies aimed to examine the effects of removing or reducing densities of urchins, most (54 out of 79) carried out repeated removals to remove reinvading urchins or those missed in initial removals. When reported, the frequency of repeat removals ranged from every few days to annually, and the number of urchins removed was ~10–20% of that initially removed (Low, 1975; Ling, 2008). While in some studies (seven) it could not be concluded if urchins were removed multiple times; in 14 studies, urchins were removed only once (including two which also used barriers to prevent re-entry). Four studies included both single and multiple removals (Andrew et al., 1998; Hereu, 2004; Watanuki et al., 2010; Tracey et al., 2015), with mixed results on the number of sea urchins which remained and kelp recovery. Keats et al. (1990) removed urchins frequently (weekly-monthly) for over 3 years (400 m2), after which removals were stopped and re-invading urchins removed almost all fleshy algae.
Duration of Monitoring
The mean and median duration of monitoring in the studies was 23 and 18 months (n = 76), respectively, with a range from 0.5 months (monitoring urchin density only; Bernstein and Welsford, 1982) to 84 months (House et al., 2018). Only nine projects were monitored for more than 2 years.
Active Removal Methods
Almost half of the studies did not describe the method of removing urchins (Figure 5A). However, many referenced SCUBA or “manual,” suggesting sea urchins were either crushed/pierced in place or collected and removed from plots. The removal methods in the studies reviewed can broadly be broken into three categories, (1) in situ culling, (2) collection, and (3) exclusion, reviewed below.
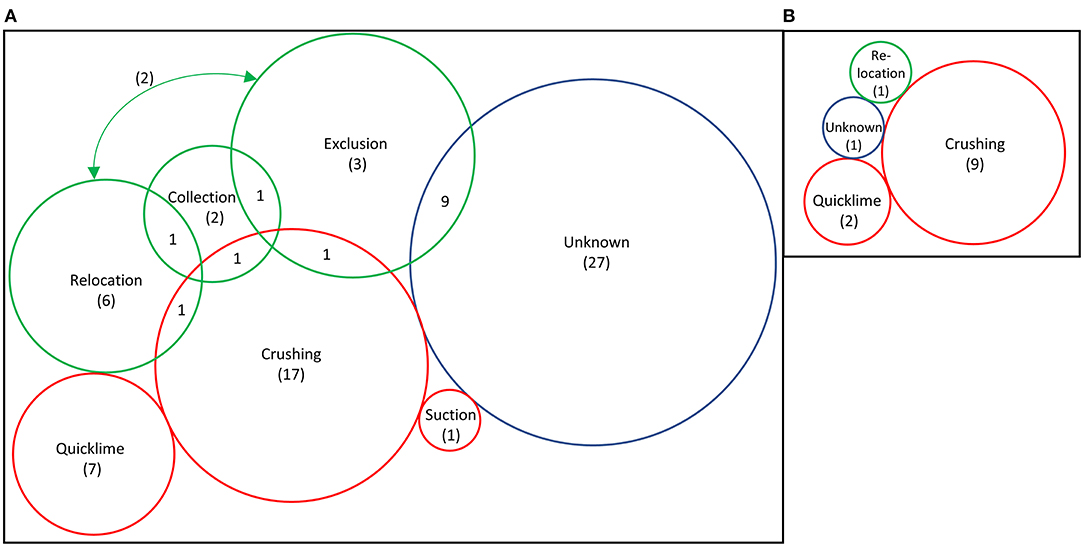
Figure 5. Number of published studies that use the different methods for urchin removal: (A) all studies included in this review (n = 79) and (B) studies that removed urchins from areas >1 hectare (n = 13). Arrow in (A) indicated 2 studies that overlapped between exclusion and translocation. Red circles indicate culling methods, green illustrates collection, relocation, or exclusion methods, blue is unknown. Scale is relative to number of total studies in the category; numbers demonstrate studies that used only that method exclusively.
In situ Culling
Crushing
The most commonly reported method of removal was in situ culling, by crushing or piercing urchins (n = 20), by chemical means (n = 7), or the use of suction dredging (n = 1, Figure 5A). Crushing was particularly common for large-scale removals (Figure 5B), using abalone bars (Sanderson et al., 2016), hammers (Breen and Mann, 1976; Himmelman et al., 1983; Keats et al., 1990; Guarnieri et al., 2020), knives (Guarnieri et al., 2020), or iron rods (Taino, 2010). Essentially all crushing was performed while SCUBA diving, but two studies reported crushing by freediving (Kitching and Ebling, 1961; Taino, 2010). Urchin remains are left underwater and treated as a waste product but provide nutrients for the marine system. This is one of the most simple and thorough methods of removal, with little impact on the environment or other species (Wilson and North, 1983). However, manually crushing each sea urchin is time-consuming, costly, and can be considered wasteful.
Reported crushing rates averaged 19.5 ± 4.6 urchins/min (mean ± SE, n = 9). If rates were given as a range, the mean was used in calculations. Within a single region, rates can vary greatly. Wilson and North (1983) reported the fastest rates (of 2,100–4,200 urchins/h or 35–70 urchins/min), averaging over 3,000 urchins/h for “trained personnel” and sea urchin densities >30 urchins/m2. Leighton et al. (1966) reported the next fastest rates (1,000–2,000 urchins/h, or 17–35 urchins/min). More recently, 3.6 million urchins were removed at a rate of 9.0 urchins/min with urchin densities of ~18 urchins/m2 (House et al., 2018).
Urchin density, size, sea condition, experience of workers, depth, and barren extent affect removal efficiency. Commercial divers estimated the time and cost of working at depths of 15–20 m was more than 3.5 times greater than <10 m (Tracey et al., 2014). In Australia, cull rates increased linearly from 0 to 10 urchins/min as urchin density rises from 0 to 2 urchins/m2, above which point efficiency leveled off (Tracey et al., 2015). The two largest projects were conducted in California with over 18 ha cleared in 6,600 h (House et al., 2018) and in Australia with 50 ha of incipient barrens cleared in 163 h (Gorfine et al., 2012).
Quicklime
Quicklime (calcium oxide, CaO) was first used to kill sea urchins in situ in the 1960s (Leighton et al., 1966). In water, it produces an exothermic reaction and converts to calcium hydroxide (Ca(OH)2) and becomes inert within days (Bernstein and Welsford, 1982). Quicklime was used considerably in California through the 1970s and trialed in the Canadian Atlantic (Bernstein and Welsford, 1982), after which it fell out of fashion for several decades. However, researchers in Norway have resurrected the research and improved effectiveness (Strand et al., 2020). While quicklime is most lethal to echinoderms (including non-targeted starfish), it can also cause mortality in other invertebrates such as abalone (North and Shaefer, 1963), potentially prohibiting the use of this treatment option.
Quicklime application rates are independent of urchin density. The quantity of quicklime applied (low dose [6–7 m2/min] or high dose [2–3 m2/min]) and application method (dumped at surface or hose to seafloor) affect efficiency (Bernstein and Welsford, 1982). Wilson and North (1983) reported 28 m2/min using a directed hose approach with a diver below. This approach can be the most time-efficient and therefore cheapest method for covering large areas (Wilson and North, 1983; Eger et al., 2021b).
Other Culling Technology
Wilson and North (1983) described diver-operated suction dredging for urchin removal, and one fisherman in Northern California uses a vacuum device to remove sea urchins (Barrett, personal communication, 16 April 2020). The impact on other marine life (e.g., bycatch, habitat impacts) were not evaluated, but should be considered. Remotely operated vehicles (ROVs) for culling urchins are being developed (James et al., 2016; Gilbreath et al., 2018; Marauder Robotics, 2021) and may be safer, more efficient, and have access to deeper sites than diving-based methods.
Rate information for these technology are scarce. Wilson and North (1983) reported a rate of 6,000 urchins/h (100 urchins/min) for suction dredging. A prototype machine devised by Gilbreath et al. (2018) had a theoretical rate of 1,000 urchins/h (17 urchins/min), and they also describe a system of hand picking with a vacuum that removes 200–600 urchins/h (3–10 urchins/min).
Collection/Relocation
In 15 reviewed studies, sea urchins were manually removed by divers (Figure 5A). At least a portion of the sea urchins were either relocated (10 studies), collected with an unknown fate, or taken to a laboratory for analysis (Kain and Jones, 1966; Jones and Kain, 1967) or experimentation (Carlsson and Christie, 2019).
Rather than culling or harvesting, relocation is a simple non-destructive method. Most studies were small scale (<1,000 m2), with two exceptions. Mooney (2001) transplanted or crushed ~25,000 sea urchins depending on sea conditions; Watanuki et al. (2010) had divers relocate 32,900 urchins. While rarely described, sea urchins were typically moved into nearby areas of reef.
No studies in this review reported collection rates, but all estimates show it to be several times slower than culling. A trial by a commercial scallop diver indicated culling is 2.4 times faster than collection (Lisson, 2018). Cresswell et al. (2019) estimated culling to be 2.7 times faster based on catch data and fishermen estimates, and Rootsaert (personal communication, 3 June 2020) estimated culling to be 4 times faster than collection. In addition, culling requires fewer resources (e.g., large boats) than collection.
Exclusion
Sixteen projects used exclusion devices to minimize urchin re-invasion, with varying effectiveness. The primary aim of these studies was to investigate ecological effects of urchin removal, with only two studies restoration focused. Natural barriers can be effective, including sand (Kriegisch et al., 2016) or octocorals (Ling et al., 2020). Fences and cages were typically only used in small (<50 m2) removals.
Personnel
Most studies (60/79) did not specifically state who did the removals and it was assumed to be the researchers with limited assistance (Figure 6). These were mostly smaller projects whereas large projects (>10 ha) typically employed the help of commercial abalone divers and volunteers (Taino, 2010; Gorfine et al., 2012; Sanderson et al., 2016; House et al., 2018). Sanderson et al. (2016) assessed the feasibility of commercial abalone fishermen crushing urchins when fishing was slow, but based on 14 dives, it showed little promise. Early trials of quicklime were conducted by a collaboration between California Department of Fish and Game and the Kelco Inc., which held a lease for kelp harvesting (Wilson and North, 1983).
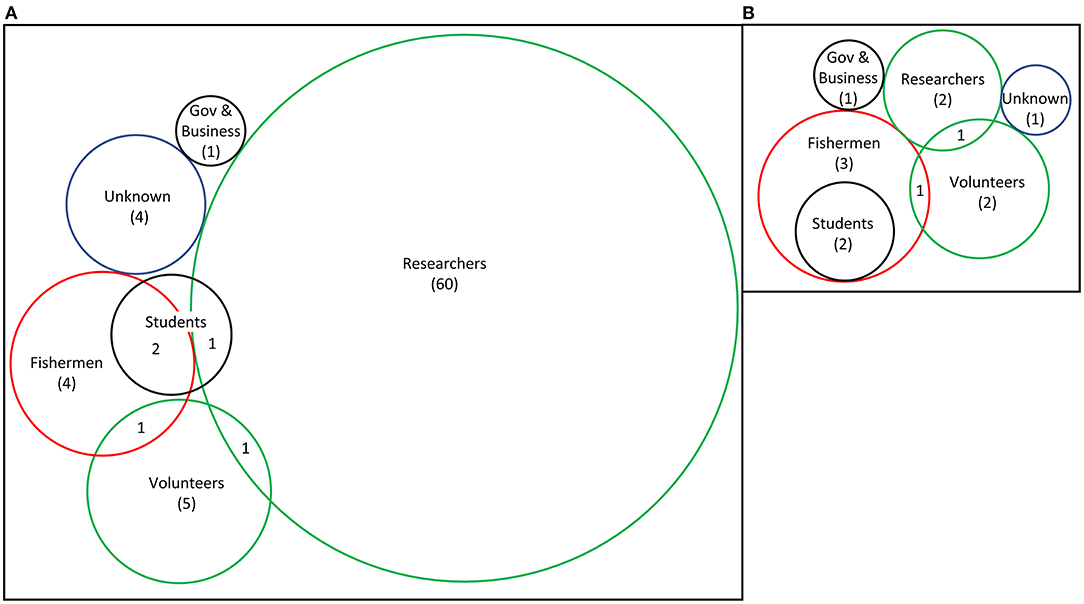
Figure 6. Number of studies with the different personnel that removed urchins in published urchin removal studies: (A) all studies included in this review (n = 79) and (B) only studies that removed more than 1 hectare (n = 13). Scale is relative to number of total studies in the category; numbers demonstrate studies that used only that method exclusively. If not otherwise stated, it was reasonably assumed researchers conducted removals with limited assistance. Fishermen were typically commercial abalone or sea urchin divers.
Macroalgal Recovery Following of Urchin Removal
In this review, success of urchin removal was determined simply as macroalgal growth. Differing categories of algae, such as “fleshy,” “foliose,” “erect,” “large and small perennial and annuals,” or “non-encrusting algae,” made comparisons difficult, restricting the ability to reach more specific conclusions about the relative effect on the dominant algal taxa (such as between laminaria and fucoids). For example, in one study “foliose algae” included laminarian and fucoid kelps, seasonal reds and browns, and articulated coralline algae (Andrew and Underwood, 1993). Of the studies that statistically tested changes in macroalgae density (n = 43), 61% showed significant increases in macroalgae (n = 26) and a further 28% (n = 12) showed partial increases (e.g., at one site or set of conditions). Of studies that did not statistically test for increases (n = 27), the proportions are similar: 85% exhibited increases and 11% partial increases. Only six (of 70) studies showed no increase in macroalgae. The remaining nine studies did not examine macroalgae results. Partial reductions in urchin density (e.g., by 50 or 33%) generally did not allow macroalgal regrowth (Andrew and Underwood, 1993; Hill et al., 2003). Similarly, methods can vary in their effectiveness (proportion of sea urchins eliminated) of removal (e.g., quicklime; Bernstein and Welsford, 1982; Strand et al., 2020); low removal effectiveness can prevent macroalgae regrowth (Strand et al., 2020).
While we could not examine success rate based on taxonomic differences, we were able to compare how the smaller fucoid or other macroalgal dominated canopies found in the Mediterranean and Canary Islands responded, compared to the wider study results which were generally focused on laminarian-dominated systems. Fifteen of the 16 studies in these areas (one study included re-seeding efforts and was therefore excluded) showed either partial or full success. Two studies showed positive but not statistically tested macroalgal growth, nine studies (69%) showed significant increases, and four (31%) showed significant partial success. This is a higher success rate than that seen across all studies combined. However, the limited number of studies and diversity of approaches used make it difficult to assess if this is a function of study design or ecological differences between these broad forest types. Likewise, due to the variety of methods in how and where urchins were removed across studies, it was not possible to explicitly examine how effectiveness related to size of urchin removal plots. For example, positive results were observed in relatively small plots when sea urchins were successfully excluded through the use of cages or regular ongoing urchin removal. Similarly, when urchins were removed from small discrete patches of barrens surrounded by algal forest there was rapid recovery (Ling, 2008; Bulleri, 2013). In contrast, when only small areas were cleared of urchins from within a larger barren there was little recovery due to continued reinvasion (Shears and Babcock, 2002). Large removal plots within extensive barrens can also be effective with limited sea urchin immigration and may require less frequent removals to maintain densities (Low, 1975; Villouta et al., 2001; Guarnieri et al., 2020). Comparisons of effectiveness between studies of plot size (and barren size) are confounded by varying sea urchin removal frequency.
While most studies removed sea urchins multiple times, differing study designs did not permit between-study comparisons to evaluate the importance of removal frequency. However, a few studies explicitly compared the effects of removal frequency on algal growth, i.e., single removal vs. ongoing removals. Hereu (2004) found single eradication was sufficient for macroalgal growth, but the majority of studies found it was necessary to remove sea urchins continuously (e.g., Shears and Babcock, 2002). In two other studies, urchin densities were higher in the single removal compared to multiple removal sites. While one study showed reduced algal regrowth in the single removal (Watanuki et al., 2010), algal regrowth in the other study was indistinguishable between single and multiple removals (Andrew et al., 1998).
Twenty-two studies reported the effects of urchin removal on species other than kelps, primarily focusing on sessile and mobile invertebrates. Two studies examined fish response, one showed a positive and one a mixed effect (Mooney, 2001; Williams et al., 2021). Sessile invertebrates were less likely than fish to show a partial or positive response (50%, n = 14). Abalone showed a positive increase in both studies when macroalgae increased (n = 2). Other mobile invertebrates (examined separately) typically showed an increase in abundance and/or diversity following urchin removal (64%, n = 11), except for limpets, which decreased in two studies.
Assessing the overall success of sea urchin removals to restore macroalgal forests was often difficult, owing to a lack of comparison to pristine, forested reference/control sites. In most cases, the recovery of macroalgae in removal sites was compared only to barren control sites. Furthermore, given the variation in how macroalgal species regrowth was reported, this review did not attempt to evaluate if recovery followed a transitional succession. Twelve studies noted a sequential progression of different species through time and though this may have occurred in other studies it was not clearly reported. Of the twelve studies reporting successional patterns, eight reported establishment of a laminarian/fucoid canopy following an initial assemblage of fast-growing opportunistic species.
Discussion
This review found sea urchin removal to be generally effective in promoting recovery of macroalgal forests, and that several removal methods are feasible to conduct on large-scales for restoration purposes. However, these findings are nuanced and discussed in greater detail below. Similarly, the potential roles of sea urchin removal in kelp forest restoration are described with suggestions on how it can best be utilized.
Can Urchin Removal Restore Macroalgal Forests?
Overall, most studies support sea urchins as important top-down controllers of the reef system; their removal led to some degree of macroalgal regrowth in 91% (including statistically tested or not, positive or partial success of cases). Most of the research reviewed here found that essentially all accessible urchins needed to be removed in order to promote macroalgal regrowth. This is consistent with other reviews on urchin barrens and kelp forest dynamics, which included urchin declines resulting from manual removals, storms, disease die-offs, and marine reserve establishment (Filbee-Dexter and Scheibling, 2014; Ling et al., 2015). In contrast, removing only a portion of sea urchins from barrens is generally not sufficient to promote macroalgal recovery (Andrew and Underwood, 1993; Prince, 1995; Hill et al., 2003; Carnell and Keough, 2016; Sanderson et al., 2016). Similarly, in cases where removal methods were not sufficiently effective or there was continued reinvasion of sea urchins into removal areas there was limited macroalgal recovery (Shears and Babcock, 2002; Strand et al., 2020). These examples support the non-linearity of the urchin-kelp dynamic and demonstrate the need to reduce and maintain urchin populations below the threshold density that maintains barrens (Ling et al., 2015).
While most studies showed at least partial success, results of sea urchin removals appear to be context-dependent, even when almost all sea urchins were removed. The majority of urchin removal studies were undertaken in urchin barrens, where urchins can be expected to be the main factor limiting kelp growth. In cases where kelp cover was already high or densities of sea urchins were initially low, there was generally little effect of urchin removal on total macroalgal cover (e.g., Fricke, 1979; Castilla and Fernandez, 1998; Vanderklift and Kendrick, 2005; Carter et al., 2007). For example, in Washington, no macroalgal differences were seen following removals from rock walls with initially low urchin densities (<0.25 urchins/m2) and macroalgal cover (5%), suggesting low initial algae cover was not due to urchin grazing (Elahi and Sebens, 2013). Even within a study, the effect of urchin removal varied with environmental context and among different urchin species. In southern New Zealand, depth range was a critical predictor of macroalgal recovery (Villouta et al., 2001) and in two other studies, control sites showed increased macroalgal cover in tandem with removal sites, suggesting an extraneous recovery mechanism (Leinaas and Christie, 1996; Carnell and Keough, 2016). In the Mediterranean, removal of one species of urchin (Arbacia lixula) showed a significant effect on algal regrowth, while another species (Paracentrotus lividus) did not (Bulleri et al., 1999; Bonaviri et al., 2011).
For macroalgae to regrow, sea urchins must be effectively removed from discrete areas of urchin barrens to minimize reinvasion. While small-scale experimental plots within larger barrens could be maintained with the use of exclusion cages or frequent removals, these are not practical for large-scale restoration. Successful algal regrowth was achieved where urchins were removed from discrete areas, whether small incipient barrens (Ling, 2008; Bulleri, 2013) or larger well-defined areas within extensive barrens (House et al., 2018; Guarnieri et al., 2020; Williams et al., 2021). Under these conditions there is minimal reinvasion of urchins from adjacent barrens and therefore recovered kelp may be more resilient. However, there is potential within very large barrens that the availability of kelp propagules is reduced which would result in a slower recovery time (see importance of proximity to existing kelp forest for restoration success; Eger et al. (2021b)). To date, no studies have examined the effect of plot size on reinvasion within extensive barrens (but see Andrew and Underwood, 1993 regarding shape and area:edge ratio), but larger removal areas are expected to be more resilient to reinvasion (Leighton et al., 1966; Shears and Babcock, 2002). Similarly, selecting removal areas with natural barriers, such as constrictions and habitat features (e.g., rocky points with strong currents, depths, sand) can minimize immigration of urchins. Greater results and longer-term effectiveness can be achieved if removal areas are selected to minimize reinvasion (i.e., removing sea urchins from an entire or discrete area of barrens).
Is Urchin Removal a Feasible Method for Kelp Forest Restoration?
Practicality
Urchin removal appears to be an effective means of increasing macroalgae cover, but its use in large-scale kelp forest restoration requires an assessment of the practicality on ecologically relevant scales. A few projects have demonstrated urchin removals can be carried out on very large (>100,000 m2) areas (Taino, 2010; Gorfine et al., 2012; House et al., 2018; Williams et al., 2021). Overall, several factors demonstrate high feasibility: high success rate (e.g., this review), rapid restoration (Ling et al., 2015), and a broad array of potential benefits (enhanced productivity, biodiversity, socio-economic services). It may be faster, cheaper (see below), and more effective than restoration of other marine ecosystems, such as coral reefs, seagrass beds, or oyster reefs (Bayraktarov et al., 2016). As such, it seems relatively pragmatic to implement under some circumstances. Nevertheless, urchin removal still requires high levels of labor and encompasses challenges inherent to working in marine environments. Finding sufficient divers (regardless of cost) may be challenging and in some cases depths may exceed practicable limits. Removals across extensive barrens (e.g., 100s of km) may be unrealistic, but addressing smaller areas or incipient barrens may be easier and more cost-effective. Similarly, sites can be prioritized for environmentally important areas or ease of access (Tracey et al., 2014). Lastly, as sea urchins are frequently managed under fisheries regulations, their removal may not be legal without specific permits. In summary, it may not be the most practical or long-term method, but it is feasible. Cost-benefit analyses would be useful to aid in determining if sea urchin removal is warranted, and if so, which methods to use.
Ethics and Cultural Considerations
The methods and use of sea urchin removal must also be evaluated from a cultural perspective to determine if removal, and in particular culling, is an acceptable form of restoration. In general, large-scale ecosystem manipulation can have far-reaching or unexpected societal and cultural effects. In New Zealand, the common barren-forming sea urchin, kina, is a taonga (a treasured or sacred) species and an important kaimoana (traditional food source) for Māori. Thus, culling must be considered with respect to both environment and culture and should be conducted in cooperation with indigenous peoples. As sea urchins are an important food traditionally, commercially, and recreationally, the loss of sea urchins can have significant socio-economic impacts (Steneck et al., 2013). Overall, large-scale culling of animals is a rather desperate means of restoration, but in some cases may be the best option to begin restoring ecosystem services. As outlined below, if a single culling event is combined with additional measures (sea urchin harvest or marine protection), this may be more palatable and beneficial for communities.
Cost
Over large-scales (>1,000 m2), the practicality of manual removal has been questioned (Keane et al., 2019; Ling et al., 2019) due to high costs from labor and boat rates (Tracey et al., 2014). However, actual cost estimates are scarce (but see Larby, 2020). In Tasmania, Australia, commercial divers quoted ~US$9,805/ha based on 1.5 urchin/m2 (Tracey et al., 2014). In Victoria, Australia, costs were estimated at AUD$35,000 for 163 h and culling just over 200,000 urchins (Gorfine et al., 2012). In the Mediterranean, urchin removal and macroalgal re-seeding was ~US$62,000/ha (Medrano et al., 2020). A review by Eger et al. (2021b) found urchin culling (quicklime [$1,300 USD/ha 2010], then crushing [$43,000/ha]) to be the cheapest kelp restoration methods by over an order of magnitude. Both are considerably less than the estimated global average cost of marine restoration of USD$80,000-$160,000/ha (Bayraktarov et al., 2016).
Comparing these coarse cost estimates to those for kelp forest benefits may offer sufficient support for restoration. Estimates of kelp benefits ranged from AUD$1,400/ha (Bennett et al., 2016) to USD$28,619/ha (Costanza et al., 2014) to USD$156,700/ha annually (Eger et al., 2021a). Restoration typically provides net gains, and may have high returns on investment from ecosystem benefits, tourism, fisheries, or potential pharmaceutical breakthroughs (De Groot et al., 2013; Duarte et al., 2020) with the breakeven point in only 2–7 years (Eger et al., 2021b). The “restoration economy” can also support high numbers of jobs per monetary investment, with considerable economic and employment multipliers (BenDor et al., 2015), and be an opportunity for green job creation and/or retraining.
While funding sources for removals were not specifically investigated in this study, governments, environmental agencies, commercial and recreational fishermen, divers, or other stakeholder groups and volunteers may contribute time or money to restoration projects. Increased fisheries quotas, subsidies or other opportunities for profit may promote harvest, but may require monitoring to ensure harvest is from within barrens. Incentivizing harvest could help ameliorate costs of sea urchin removals and therefore increase the feasibility of restoration.
Utilizing Urchins From Barrens
Developing uses for sea urchins removed from barrens would provide additional opportunities and incentives for their harvest and provide a less wasteful option than culling. A few possibilities are outlined below:
Harvest for Consumption
Commercial harvest of some species of sea urchins has been shown to reduce densities and promote kelp recovery (Wilson and North, 1983), and prevent barren formation (Keane et al., 2019). However, the roe of urchins from barrens is typically of poor quality, and thus of limited value for food or market (Claisse et al., 2013; Pert et al., 2018; Ling et al., 2019). As a result, fishermen typically do not harvest urchins from barrens, preferring to harvest urchins from within or on the borders of kelp forests to optimize quality of product (Pert et al., 2018; Byrne and Andrew, 2020 and references therein). Thus, in many cases harvest from urchin barrens may be an unlikely solution for kelp restoration. However, harvest at kelp restoration sites could play a role in keeping urchin numbers down if size and roe condition is initially marketable or improved following initial urchin removals. Fishermen have reported better quality in areas that have been “thinned out” to lower densities (Miller and Abraham, 2011), lending support for rotational harvest (Andrew et al., 2002; Carter et al., 2007; Lisson, 2018) or spatial based management (Mooney, 2001; Andrew et al., 2002; Miller and Nolan, 2008).
Sea Urchin Translocation
Relocating sea urchins from barrens to kelp forests can improve roe condition (James and Herbert, 2009; Kino, 2010; Thomas, 2011; Agatsuma, 2020 and articles within, Blount et al., 2017). However, transplanting excessive urchin densities could harm new sites and expand overgrazing issues (Agatsuma, 2020). This technique would only be successful if there is a small ratio of barrens to kelp habitat; it would also incur additional costs. Thus, translocation may be ineffective for large-scale kelp forest restoration, but may be useful on small scales to improve roe quality.
Urchin Conditioning
Urchin conditioning or “ranching” has recently been proposed to utilize sea urchins from barrens. Sea urchins are harvested in poor condition and then “fattened” in laboratory or field settings for sale as food (Pert et al., 2018). Urchinomics, Inc. has recently received considerable press for proposing a business model for urchin conditioning (Urchinomics, 2020). Economic feasibility will depend on marketability and the relative natural availability, as conditioning may not be cost-effective as it relies on expensive infrastructure.
Other Uses
Without roe enhancement, sea urchins may be utilized for other purposes. Sea urchins can be used to ameliorate soils for agriculture (Garau et al., 2012) with further research underway at the University of Tasmania. Potential pharmaceuticals, bioactive compounds, and animal feed may provide broad applications; jewelry, dyes, and decorations could supply small markets.
Role of Sea Urchin Removal in Kelp Forest Restoration
Sea urchins are a major factor limiting kelp forests in many parts of the world (Filbee-Dexter and Scheibling, 2014) and overfishing of sea urchin predators is one of the major causes of this imbalance (Steneck et al., 2002; Steneck, 2020). Marine protection has been shown to be a viable but passive approach to restoring kelp forests (Shears and Babcock, 2002; Behrens and Lafferty, 2004; Edgar et al., 2009), but this can take decades following recovery of previously fished predators within MPAs (Babcock et al., 2010; Malakhoff and Miller, 2021). In temperate regions where sea urchin barrens are common, sea urchin removal could be a valuable tool for kelp forest restoration, both in isolation and in conjunction with other management approaches such as MPAs (Figure 7). The application of urchin removal as a restoration tool and approach used will depend on the objectives of kelp restoration projects. If the restoration goal is simply to restore kelp to rocky reefs, then this can likely be achieved solely through ongoing sea urchin removal. Sea urchin removal is also expected to be beneficial for some species that are strongly habitat dependent, but increasing habitat alone will not increase abundances of species that are primarily limited by high fishing pressure. Therefore, while urchin removal is effective at promoting kelp recovery, it will have limited potential on its own for restoring the original ecosystem state and function (see Bayraktarov et al., 2016). Kelp forests with only the “ghosts of missing animals” (Dayton et al., 1998) may not be considered a restoration success. Consequently, for urchin removal to provide a wider role in kelp forest restoration it would be best used as a component of a larger restoration plan.
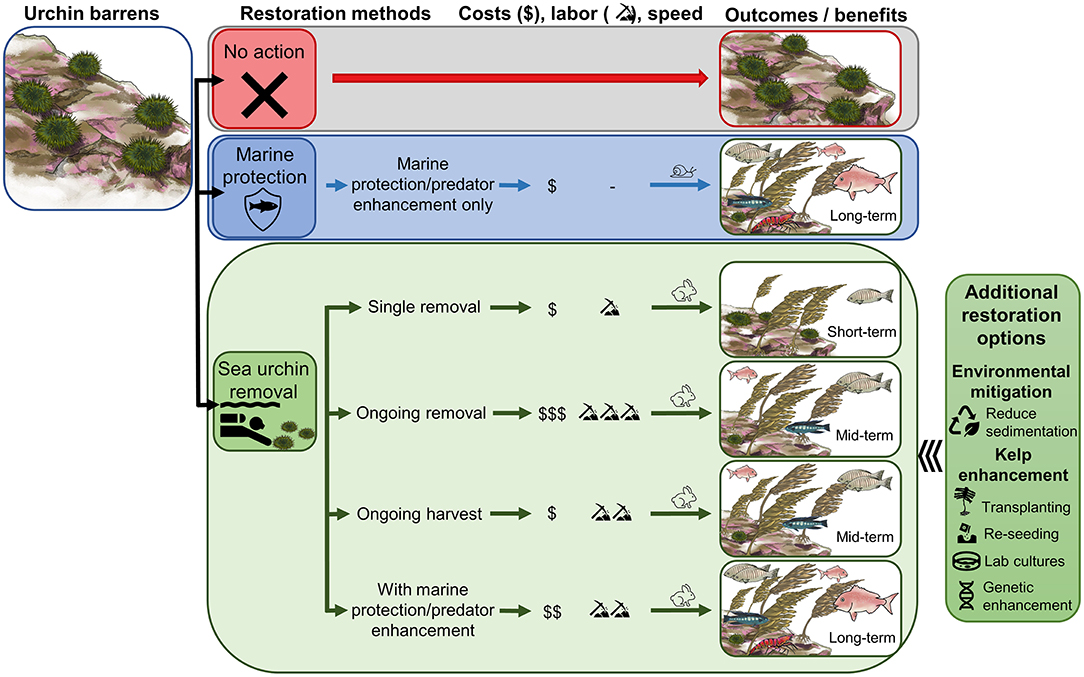
Figure 7. Approaches to restoration of kelp forests in urchin barrens. Marine protection provides a passive approach to kelp forest restoration, whereas sea urchin removal can be used in several ways to actively promote kelp forest ecosystem recovery. The costs, labor, speed of kelp recovery, biodiversity benefits and long-term resilience will depend on the urchin removal approach used and integration with other management measures (e.g., urchin harvest and marine protection). If other stressors limit kelp recovery following sea urchin removal, additional restoration methods may be necessary to actively promote kelp recovery (right). Illustrations by M. Perocheau and S. Ruste.
Restoration objectives should be clearly defined and the methods used should reflect these aims. Single sea urchin removal will incur only low to moderate labor and costs and may promote rapid kelp recovery, but effects may be temporary as kelp forests will be vulnerable to reinvasion of sea urchins, and biodiversity benefits will be limited (Figure 7). Ongoing sea urchin removals can improve kelp resilience and biodiversity (Figure 7) and enhance the quality of remaining sea urchins, creating opportunities for ongoing harvest of sea urchins from restoration sites (Claisse et al., 2013). These benefits will be dependent on continuation of removal and harvest; once this stops, the restored site can revert back to sea urchin barrens. Ongoing removals will require additional labor effort and costs; however, costs may be neutralized if sea urchins are of marketable quality and can be harvested. While ongoing urchin removals or harvest can provide some ecological and economic benefits, ongoing fishing pressure at restoration sites for other species is likely to prevent restoration of full ecosystem function (e.g., biodiversity, predator abundance).
Combining sea urchin removal with other management tools (Figure 7), such as marine protected areas (McClanahan et al., 2002) or predator protection and enhancement programs (Johnson et al., 2013), provides an opportunity to speed up kelp recovery and maximize long-term biodiversity benefits. Marine protection strategies can most effectively restore trophic cascades and ecosystem function and with minimal cost and no labor, but on their own are slow and context-dependent (Shears et al., 2008). When urchins are removed, the rate of macroalgal recovery (canopy species) on temperate reefs is on average 18.5 ± 2.0 months (Ling et al., 2015), close to an order of magnitude faster than the potential decadal time spans for trophic cascades to occur through marine protection alone (Shears and Babcock, 2003). Thus, urchin removal with marine protection provides both rapid kelp restoration with long-lasting kelp forest resilience, restoring maximum ecosystem services (Figure 7).
In cases where additional stressors inhibit kelp growth in urchin barrens, other restoration approaches may be needed to facilitate kelp forest restoration following urchin removal (Box 1, Figure 7). While sea urchins initially created barrens, changing environmental conditions (e.g., increased temperature or storms, eutrophication, pollution, invasive species, lack of kelp propagules, other herbivores) may prohibit kelp recovery following sea urchin removal or create different responses in the future (Strain et al., 2015). In some cases, environmental mitigation strategies can be implemented to reduce these stressors but may be slow to enact or require more resources than a restoration plan allows. Active kelp reseeding and enhancement methods provide additional tools that can be used to promote kelp recovery following sea urchin removals (Guarnieri et al., 2014; Medrano et al., 2020); see reviews in Eger et al. (2020) and Morris et al. (2020). These include transplanting or re-seeding of kelps from wild or cultured stocks, with the potential to develop novel strains that can cope with changing environmental conditions.
Globally, research to improve kelp forest restoration techniques is growing, yet sea urchin removal may provide some of the most effective and cheapest means within urchin barrens. If it is determined to be an appropriate approach for promoting kelp forests, sea urchin removal should be done in a manner to maximize effectiveness and likelihood for success (Box 3). With increasing loss of kelp forests globally, effective restoration strategies are needed to mitigate their declines and improve long-term resilience. As kelp forests support many of our temperate coastal ecosystems, many species (including humans) depend on their vitality. The vast array of kelp forest benefits (e.g., high productivity, ecosystem foundation, carbon sequestration, support of coastal fisheries) suggest the use of sea urchin removal, in combination with other management and restoration approaches methods, is warranted in many situations.
Box 3. Guidance for projects using sea urchin removal from barrens.
If urchin removal is to be used for kelp restoration, one must consider the best approach to remove them quickly and cheaply, with the fewest negative externalities. The aims of a project need to be specified, the root causes of urchin barrens need to be addressed, and the costs and benefits of different removal methods need to be weighed. Single removals may be sufficient to prevent or slow barren formation, but removals alone will typically have little long-term benefit for meeting biodiversity objectives. Greatest restoration outcomes will be achieved if combined with other measures.
Six main considerations will help to plan and implement urchin removal restoration projects:
1. The objectives and goals of restoration need to be clearly defined. These will determine sites, methods used and the importance of scale, costs, availability of labor, environmental impacts, and utilization of urchins.
2. Discrete and/or large areas must be cleared to minimize reinvasion. Sites should be selected to minimize reinvasion (e.g., complete removal from barrens, minimize edge effects, natural barriers to entry). Removals are most effective when barrens are small and urchins can be removed from discrete patches of barrens; where barrens are extensive, removals need to be large to reduce reinvasion.
3. Removals, regardless of method, need to reduce sea urchin densities close to zero. Partial removals are not effective due to hysteresis; thus, sea urchin densities must be below the threshold which can maintain barrens.
4. Sites need to be monitored for urchin reinvasion and kelp growth, and more than one removal may be required. Densities must be kept low until kelp is restored. If kelp propagules are limited, additional enhancement approaches may be necessary.
5. Sea urchins may be utilized. Harvesting urchins for consumption or other uses creates incentives and ensures sea urchins are not wasted. If initial harvest is not viable due to poor roe quality, restoration plans may include subsequent harvest if roe quality improves.
6. Urchin removal may be only a temporary solution unless a part of a larger restoration plan. Urchin removal does not address the root cause of urchin population growth, and thus additional measures will likely be necessary to increase biodiversity and ecosystem resilience. For example, it can be used in conjunction with:
a. Urchin harvest (removals can improve remaining sea urchin quality);
b. Restoration of predators through reintroduction or reduced harvest (predators control);
c. Implementation of marine protected areas (jump-start kelp recovery and increase; biodiversity and resilience);
d. Other kelp restoration approaches (transplanting, seeding, competition removal); and
e. General improvement of environmental conditions (addressing abiotic or biotic stressors).
Conclusions
A variety of methods have been used to remove sea urchins over the last 60 years and typically urchin removal was found to lead to macroalgal regrowth within urchin barrens. Consequently, when urchin grazing is the main factor limiting kelp growth, urchin removal provides a simple and relatively cheap approach to promoting and initiating kelp forest restoration. The method and scale of urchin removal will depend on site- and region-specific conditions and what is considered the most practical, culturally appropriate, and effective approach to meet kelp restoration objectives. While urchin removal may promote rapid macroalgal regrowth within urchin barrens, in order to provide long-term ecosystem benefits (including productivity, complexity and diverse ecosystem services), urchin removal will need to be combined with other management strategies that increase biodiversity and kelp forest resilience such as marine protection or ongoing urchin harvest. Kelp forest restoration initiatives are increasing globally with the aim of restoring kelp forests that are resilient to current stressors and stressors associated with a changing climate. Based on our review, we provide recommendations on how sea urchin removal can most effectively be used to control sea urchin densities and promote the long-term resilience of kelp forests with their associated biodiversity and ecosystem services. Unbridled, these “spiny enemies” can raze kelp forests, but at lower densities they remain as an integral part of the ecosystem and provide important food and cultural resources.
Data Availability Statement
The original contributions presented in the study are included in the article/Supplementary Materials, further inquiries can be directed to the corresponding author.
Author Contributions
KM and NS conceived the initial idea for the review. KM conducted the literature review, extracted the data, and prepared the manuscript with input from NS and CB. All authors contributed to manuscript revision. All authors contributed to the article and approved the submitted version.
Funding
This work was supported in part by University of Auckland Doctoral Scholarship to KM, funding from Foundation North: GIFT and Sustainable Seas (1.1 Ecological responses to cumulative effects) to NS, and from the George Mason Centre for the Natural Environment to CB.
Conflict of Interest
The authors declare that the research was conducted in the absence of any commercial or financial relationships that could be construed as a potential conflict of interest.
Publisher's Note
All claims expressed in this article are solely those of the authors and do not necessarily represent those of their affiliated organizations, or those of the publisher, the editors and the reviewers. Any product that may be evaluated in this article, or claim that may be made by its manufacturer, is not guaranteed or endorsed by the publisher.
Acknowledgments
Many thank to Richard Taylor, Celia Balemi, Evanne Miller and two reviewers for improvements to the manuscript. Thank to Millie Perocheau and Shawn Ruste for assistance with illustrations.
Supplementary Material
The Supplementary Material for this article can be found online at: https://www.frontiersin.org/articles/10.3389/fmars.2022.831001/full#supplementary-material
References
Agatsuma, Y.. (2020). “Stock enhancement of regular sea urchins,” in Developments in Aquaculture and Fisheries Science, ed J. M. Lawrence (London: Elsevier), 299–313. doi: 10.1016/B978-0-12-819570-3.00017-2
Airoldi, L.. (2003). “The effects of sedimentation on rocky coast assemblages,” in Oceanography and Marine Biology, an Annual Review, eds R. N. Gibson and R. J. A. Atkinson (London: CRC Press), Vol. 41. 169–171.
Ambrose, R. F.. (1994). Mitigating the effects of a coastal power plant on a kelp forest community: rationale and requirements for an artificial reef. Bull. Mar. Sci. 55, 694–708.
Andrew, N. L.. (1989). Contrasting ecological implications of food limitation in sea urchins and herbivorous gastropods. Mar. Ecol. Prog. Ser. 51, 189–193. doi: 10.3354/meps051189
Andrew, N. L., Agatsuma, Y., Ballesteros, E., Bazhin, A. G., Creaser, E. P., Barnes, D. K. A., et al. (2002). “Status and management of world sea urchin fisheries,” in Oceanography and Marine Biology, Annual Review, Vol. 40, eds R. N. Gibson, Margaret Barnes, and R. J. A. Atkinson (London: CRC Press), 351–438.
Andrew, N. L., and Underwood, A. J. (1993). Density-dependent foraging in the sea urchin Centrostephanus rodgersii on shallow subtidal reefs in New South Wales, Australia. Mar. Ecol. Prog. Ser. 99, 89–89. doi: 10.3354/meps099089
Andrew, N. L., Worthington, D. G., Brett, P. A., Bentley, N., Chick, R. C., and Blount, C. (1998). Interactions between the Abalone Fishery and Sea Urchins in New South Wales. Cronulla, NSW: NSW Fisheries Research Institute. Available online at: https://www.dpi.nsw.gov.au/__data/assets/pdf_file/0004/545593/FFRS-12_Andrew-1998.pdf
Babcock, R. C., Kelly, S., Shears, N. T., Walker, J. W., and Willis, T. J. (1999). Changes in community structure in temperate marine reserves. Mar. Ecol. Prog. Ser. 189, 125–134. doi: 10.3354/meps189125
Babcock, R. C., Shears, N. T., Alcala, A. C., Barrett, N. S., Edgar, G. J., Lafferty, K. D., et al. (2010). Decadal trends in marine reserves reveal differential rates of change in direct and indirect effects. Proc. Natl. Acad. Sci. U.S.A. 107, 18256–18261. doi: 10.1073/pnas.0908012107
Bayraktarov, E., Saunders, M. I., Abdullah, S., Mills, M., Beher, J., Possingham, H. P., et al. (2016). The cost and feasibility of marine coastal restoration. Ecol. Appl. 26, 1055–1074. doi: 10.1890/15-1077
Behrens, M. D., and Lafferty, K. D. (2004). Effects of marine reserves and urchin disease on Southern Californian rocky reef communities. Mar. Ecol. Prog. Ser. 279, 129–139. doi: 10.3354/meps279129
BenDor, T., Lester, T. W., Livengood, A., Davis, A., and Yonavjak, L. (2015). Estimating the size and impact of the ecological restoration economy. PLoS ONE 10, e0128339. doi: 10.1371/journal.pone.0128339
Bennett, S., Wernberg, T., Connell, S. D., Hobday, A. J., Johnson, C. R., and Poloczanska, E. S. (2016). The ‘great southern reef': social, ecological and economic value of Australia's neglected kelp forests. Mar. Freshwat. Res. 67, 47–56. doi: 10.1071/MF15232
Bernstein, B. B., and Welsford, R. W. (1982). An Assessment of Feasibility of Using High-Calcium Quicklime as an Experimental Tool for Research into Kelp Bed-Sea Urchin Ecosystems in Nova Scotia. Halifax, NS.
Blount, C., Chick, R. C., and Worthington, D. G. (2017). Enhancement of an underexploited fishery - improving the yield and colour of roe in the sea urchin Centrostephanus rodgersii by reducing density or transplanting individuals. Fish. Res. 186, 586–597. doi: 10.1016/j.fishres.2016.08.022
Bonaviri, C., Vega Fernandez, T., Fanelli, G., Badalamenti, F., and Gianguzza, P. (2011). Leading role of the sea urchin Arbacia Lixula in maintaining the barren state in southwestern mediterranean. Mar. Biol. 158, 2505–2513. doi: 10.1007/s00227-011-1751-2
Breen, P. A., and Mann, K. H. (1976). Destructive grazing of kelp by sea-urchins in eastern Canada. J. Fish. Res. Board Canada 33, 1278–1283. doi: 10.1139/f76-164
Bulleri, F.. (2013). Grazing by sea urchins at the margins of barren patches on mediterranean rocky reefs. Mar. Biol. 160, 2493–2501. doi: 10.1007/s00227-013-2244-2
Bulleri, F., Benedetti-Cecchi, L., and Cinelli, F. (1999). Grazing by the sea urchins Arbacia Lixula L. and Paracentrotus lividus lam. In the Northwest Mediterranean. J. Exp. Mar. Biol. Ecol. 241, 81–95. doi: 10.1016/S0022-0981(99)00073-8
Byrne, M., and Andrew, N. L. (2020). “Centrostephanus rodgersii and Centrostephanus tenuispinus,” in Developments in Aquaculture and Fisheries Science, ed J. M. Lawrence (London: Elsevier), 379–396.
Carlsson, P. M., and Christie, H. C. (2019). Regrowth of Kelp After Removal of Sea Urchins (Strongylocentrotus droebachiensis). Oslo: Norwegian Institute for Water Research.
Carnell, P. E., and Keough, M. J. (2016). The influence of herbivores on primary producers can vary spatially and interact with disturbance. Oikos 125, 1273–1283. doi: 10.1111/oik.02502
Carter, J. W., Carpenter, A. L., Foster, M. S., and Jessee, W. N. (1985). Benthic succession on an artificial reef designed to support a kelp-reef community. Bull. Mar. Sci. 37, 86–113.
Carter, S. K., VanBlaricom, G. R., and Allen, B. L. (2007). Testing the generality of the trophic cascade paradigm for sea otters: a case study with kelp forests in Northern Washington, USA. Hydrobiologia 579, 233–249. doi: 10.1007/s10750-006-0403-x
Castilla, J. C., and Fernandez, M. (1998). Small-scale benthic fisheries in Chile: on co-management and sustainable use of benthic invertebrates. Ecol. Appl. 8, S124–S132. doi: 10.2307/2641370
Christie, H., Andersen, G. S., Bekkby, T., Fagerli, C. W., Gitmark, J. K., Gundersen, H., et al. (2019). Shifts between sugar kelp and turf algae in norway: regime shifts or fluctuations between different opportunistic seaweed species?. Front. Mar. Sci. 6, 72. doi: 10.3389/fmars.2019.00072
Christie, H., Norderhaug, K. M., and Fredriksen, S. (2009). Macrophytes as habitat for fauna. Mar. Ecol. Prog. Ser. 396, 221–233. doi: 10.3354/meps08351
Claisse, J. T., Williams, J. P., Ford, T., Pondella, D. J., Meux, B., and Protopapadakis, L. (2013). Kelp forest habitat restoration has the potential to increase sea urchin gonad biomass. Ecosphere 4, art38. doi: 10.1890/ES12-00408.1
Cole, R. G., and Babcock, R. C. (1996). Mass mortality of a dominant kelp (Laminariales) at Goat Island, North-Eastern New Zealand. Mar. Freshwat. Res. 47, 907–911. doi: 10.1071/MF9960907
Coleman, M. A., Wood, G., Filbee-Dexter, K., Minne, A. J. P., Goold, H. D., Vergés, A., et al. (2020). Restore or redefine: future trajectories for restoration. Front. Mar. Sci. 7, 237. doi: 10.3389/fmars.2020.00237
Connell, S. D., Foster, M. S., and Airoldi, L. (2014). What are algal turfs? Towards a better description of turfs. Mar. Ecol. Prog. Ser. 495, 299–307. doi: 10.3354/meps10513
Costanza, R., de Groot, R., Sutton, P., van der Ploeg, S., Anderson, S. J., Kubiszewski, I., et al. (2014). Changes in the global value of ecosystem services. Global Environ. Change 26, 152–158. doi: 10.1016/j.gloenvcha.2014.04.002
Cresswell, K., Keane, J., Ogier, E., and Yamazaki, S. (2019). Centrostephanus Subsidy Program: Initial Evaluation. Hobart: Institute for Marine and Antarctic Studies; University of Tasmania.
Dayton, P. K., Tegner, M. J., Edwards, P. B., and Riser, K. L. (1998). Sliding baselines, ghosts, and reduced expectations in kelp forest communities. Ecol. Appl. 8, 309–322. doi: 10.1890/1051-0761(1998)008[0309:SBGARE]2.0.CO;2
De Groot, R. S., Blignaut, J., Van Der Ploeg, S., Aronson, J., Elmqvist, T., and Farley, J. (2013). Benefits of investing in ecosystem restoration. Conserv. Biol. 27, 1286–1293. doi: 10.1111/cobi.12158
Duarte, C. M., Agusti, S., Barbier, E., Britten, G. L., Castilla, J. C., Gattuso, J.-P., et al. (2020). Rebuilding marine life. Nature 580, 39–51. doi: 10.1038/s41586-020-2146-7
Duggins, D. O.. (1980). Kelp beds and sea otters: an experimental approach. Ecology 61, 447–453. doi: 10.2307/1937405
Duggins, D. O., Simenstad, C. A., and Estes, J. A. (1989). Magnification of secondary production by kelp detritus in coastal marine ecosystems. Science 245, 170–173. doi: 10.1126/science.245.4914.170
Edgar, G. J., Barrett, N. S., and Stuart-Smith, R. D. (2009). Exploited reefs protected from fishing transform over decades into conservation features otherwise absent from seascapes. Ecol. Appl. 19, 1967–1974. doi: 10.1890/09-0610.1
Eger, A., Marzinelli, E., Baes, R., Blain, C., Blamey, L., Carnell, P., et al. (2021a). The economic value of fisheries, blue carbon, and nutrient cycling in global marine forests. EcoEvoRxiv [Preprint]. doi: 10.32942/osf.io/n7kjs
Eger, A., Marzinelli, E., Christie, H., Fujita, D., Hong, S., Kim, J. H., et al. (2021b). Global kelp forest restoration: past lessons, status, future goals. EcoEvoRxiv [Preprint]. doi: 10.32942/osf.io/emaz2
Eger, A. M., Marzinelli, E., Gribben, P., Johnson, C. R., Layton, C., Steinberg, P. D., et al. (2020). Playing to the positives: using synergies to enhance kelp forest restoration. Front. Mar. Sci. 7, 544. doi: 10.3389/fmars.2020.00544
Elahi, R., and Sebens, K. P. (2013). Experimental removal and recovery of subtidal grazers highlights the importance of functional redundancy and temporal context. PLoS ONE 8, e78969. doi: 10.1371/journal.pone.0078969
Estes, J. A., and Palmisano, J. F. (1974). Sea otters: their role in structuring nearshore communities. Science 185, 1058–1060. doi: 10.1126/science.185.4156.1058
Eurich, J. G., Selden, R. L., and Warner, R. R. (2014). California spiny lobster preference for urchins from kelp forests: implications for urchin barren persistence. Mar. Ecol. Prog. Ser. 498, 217–225. doi: 10.3354/meps10643
Filbee-Dexter, K., and Scheibling, R. E. (2014). Sea urchin barrens as alternative stable states of collapsed kelp ecosystems. Mar. Ecol. Prog. Ser. 495, 1–25. doi: 10.3354/meps10573
Filbee-Dexter, K., and Smajdor, A. (2019). Ethics of assisted evolution in marine conservation. Front. Mar. Sci. 6, 20. doi: 10.3389/fmars.2019.00020
Filbee-Dexter, K., and Wernberg, T. (2018). Rise of turfs: a new battlefront for globally declining kelp forests. Bioscience 68, 64–76. doi: 10.1093/biosci/bix147
Fredriksen, S., Filbee-Dexter, K., Norderhaug, K. M., Steen, H., Bodvin, T., Coleman, M. A., et al. (2020). Green gravel: a novel restoration tool to combat kelp forest decline. Sci. Rep. 10, 1–7. doi: 10.1038/s41598-020-60553-x
Fricke, A. H.. (1979). Kelp grazing by the common sea urchin Parechinus angulosus leske in false bay, cape. S. Afr. J. Zool. 14, 143–148. doi: 10.1080/02541858.1979.11447664
Fujita, D.. (2011). Management of kelp ecosystem in Japan. Cah. Biol. Mar. 52, 499. doi: 10.21411/CBM.A.A3047D24
Garau, G., Castaldi, P., Deiana, S., Campus, P., Mazza, A., Deiana, P., et al. (2012). Assessment of the use potential of edible sea urchins (Paracentrotus lividus) processing waste within the agricultural system: influence on soil chemical and biological properties and bean (Phaseolus Vulgaris) and Wheat (Triticum Vulgare) growth in an amended acidic soil. J. Environ. Manage. 109, 12–18. doi: 10.1016/j.jenvman.2012.05.001
Gilbreath, W., Goss, C., and Marshall, K. (2018). Purple Urchin Removal. San Luis Obispo, CA: Mechanical Engineering Department, California Polytechnic State University.
Gorfine, H., Bell, J., Mills, K., and Lewis, Z. (2012). Removing Sea Urchins (Centrostephanus rodgersii) to Recover Abalone (Haliotis rubra) Habitat. Queenscliff, VIC: Fisheries Victoria, Department of Primary Industries.
Graham, M. H.. (2004). Effects of local deforestation on the diversity and structure of Southern California giant kelp forest food webs. Ecosystems 7, 341–357. doi: 10.1007/s10021-003-0245-6
Guarnieri, G., Bevilacqua, S., Figueiras, N., Tamburello, L., and Fraschetti, S. (2020). Large-scale sea urchin culling drives the reduction of subtidal barren grounds in the mediterranean sea. Front. Mar. Sci. 7, 519. doi: 10.3389/fmars.2020.00519
Guarnieri, G., Bevilacqua, S., Vignes, F., and Fraschetti, S. (2014). Grazer removal and nutrient enrichment as recovery enhancers for overexploited rocky subtidal habitats. Oecologia 175, 959–970. doi: 10.1007/s00442-014-2944-4
Hereu, B.. (2004). The role of trophic interactions between fishes, sea urchins and algae in the northwestern mediterranean rocky infralittoral (Doctoral dissertation). Barcelona: Universitat de Barcelona.
Hewitt, C. L., Campbell, M. L., McEnnulty, F., Moore, K. M., Murfet, N. B., Robertson, B., et al. (2005). Efficacy of physical removal of a marine pest: the introduced kelp Undaria pinnatifida in a Tasmanian marine reserve. Biol. Invasions 7, 251–263. doi: 10.1007/s10530-004-0739-y
Hill, N. A., Blount, C., Poore, A. G. B., Worthington, D., and Steinberg, P. D. (2003). Grazing effects of the sea urchin Centrostephanus rodgersii in two contrasting rocky reef habitats: effects of urchin density and its implications for the fishery. Mar. Freshwat. Res. 54, 691–700. doi: 10.1071/MF03052
Himmelman, J. H., Cardinal, A., and Bourget, E. (1983). Community development following removal of urchins, Strongylocentrotus droebachiensis, from the rocky subtidal zone of the St. Lawrence Estuary, Eastern Canada. Oecologia 59, 27–39. doi: 10.1007/BF00388068
House, P., Barilotti, A., Burdick, H., Ford, T., Williams, J., and Williams, C. (2018). Palos Verdes Kelp Forest Restoration Project. Los Angeles, CA: The Bay Foundation; Vantuna Research Group. Available online at: http://www.santamonicabay.org/wp-content/uploads/2018/02/Kelp-Restoration-Year-4-Annual-Report.pdf
Jackson, J. B., Kirby, M. X., Berger, W. H., Bjorndal, K. A., Botsford, L. W., Bourque, B. J., et al. (2001). Historical overfishing and the recent collapse of coastal ecosystems. Science 293, 629–637. doi: 10.1126/science.1059199
James, P., and Herbert, P. (2009). Kina Roe Enhancement by Translocation. NIWA Client Report. Wellington: Seafood Innovations Ltd.
James, P., Noble, C., Siikavuopio, S., Sloan, R., Hannon, C., Þ*órarinsdóttir, G., et al. (2016). Sea Urchin Fishing Techniques Report. Tromso: Nofima.
Johnson, C. R., Chabot, R. H., Marzloff, M. P., and Wotherspoon, S. (2017). Knowing when (not) to attempt ecological restoration. Restor. Ecol. 25, 140–147. doi: 10.1111/rec.12413
Johnson, C. R., Ling, S. D., Sanderson, C., Dominguez, J., Flukes, E., Frusher, S., et al. (2013). Rebuilding Ecosystem Resilience: Assessment of Management Options to Minimise Formation of ‘barrens' Habitat by the Long-Spined Sea Urchin (Centrostephanus rodgersii) in Tasmania, Project Number 2007/045. Hobart: Institute for Marine and Antarctic Studies, University of Tasmania. Available online at: https://www.imas.utas.edu.au/__data/assets/pdf_file/0011/977591/Assessment_Management_Options_Centrostephanus.pdf
Jones, N. S., and Kain, J. M. (1967). Subtidal algal colonization following the removal of Echinus. Helgoland. Wiss. Meer. 15, 460–466. doi: 10.1007/BF01618642
Kain, J. M., and Jones, N. S. (1966). Algal Colonization after Removal of Echinus. Paper presented at the Proceedings of the Fifth International Seaweed Symposium, Halifax, August 25–28 1965.
Keane, J. P., Mundy, C., Porteus, M., and Johnson, O. (2019). Can Commercial Harvest of Long-Spined Sea Urchins Reduce the Impact of Urchin Grazing on Abalone and Lobster Fisheries?, Project No 2013/026. Hobart: Institute for Marine and Antarctic Studies, University of Tasmania. Available online at: https://www.frdc.com.au/sites/default/files/products/2013-026-DLD.pdf
Keats, D. W., South, G. R., and Steele, D. H. (1990). Effects of an experimental reduction in grazing by green sea-urchins on a benthic macroalgal community in eastern newfoundland. Mar. Ecol. Prog. Ser. 68, 181–193. doi: 10.3354/meps068181
Kino, S.. (2010). Transplantation of the sea urchin Loxechinus albus in Chiloé Island, Chile. Aquacult. Sci. 58, 55–63. doi: 10.11233/AQUACULTURESCI.58.55
Kitching, J. A., and Ebling, F. J. (1961). The ecology of lough ine. J. Anim. Ecol. 373–83. doi: 10.2307/2304
Kriegisch, N., Reeves, S., Johnson, C. R., and Ling, S. D. (2016). Phase-shift dynamics of sea urchin overgrazing on nutrified reefs. PLoS ONE 11, e0168333. doi: 10.1371/journal.pone.0168333
Krumhansl, K. A., Okamoto, D. K., Rassweiler, A., Novak, M., Bolton, J. J., Cavanaugh, K. C., et al. (2016). Global patterns of kelp forest change over the past half-century. Proc. Natl. Acad. Sci. U.S.A. 113, 13785–13790. doi: 10.1073/pnas.1606102113
Kuwahara, H., Watanuki, A., Aota, T., Ando, W., Kawai, T., Terawaki, T., et al. (2006). Trends in Literature on Seaweed Restoration Techniques on Barren Grounds in Japan Fish. Eng. (Japan)/Suisan Kogaku (Japan).
Larby, S.. (2020). 'Take All' Harvest Trial of Longspined Sea Urchin (Centrostephanus rodgersii). Marion Bay to Cape Hauy.
Lawrence, J. M.. (1975). On the relationship between marine plants and sea urchins. Oceanogr. Mar. Biol. Annu. Rev. 13, 213–286.
Layton, C., Coleman, M. A., Marzinelli, E. M., Steinberg, P. D., Swearer, S. E., Vergés, A., et al. (2020). Kelp forest restoration in Australia. Front. Mar. Sci. 7, 74. doi: 10.3389/fmars.2020.00074
Leighton, D. L., Jones, L. G., and North, W. J. (1966). Ecological relationships between giant kelp and sea urchins in Southern California. Paper presented at the Proceedings of the Fifth International Seaweed Symposium, Halifax, August 25–28 1965.
Leinaas, H. P., and Christie, H. (1996). Effects of removing sea urchins (Strongylocentrotus droebachiensis): stability of the barren state and succession of kelp forest recovery in the east atlantic. Oecologia 105, 524–536. doi: 10.1007/BF00330016
Lester, S. E., Halpern, B. S., Grorud-Colvert, K., Lubchenco, J., Ruttenberg, B. I., Gaines, S. D., et al. (2009). Biological effects within no-take marine reserves: a global synthesis. Mar. Ecol. Prog. Ser. 384, 33–46. doi: 10.3354/meps08029
Ling, S. D.. (2008). Range expansion of a habitat-modifying species leads to loss of taxonomic diversity: a new and impoverished reef state. Oecologia 156, 883–894. doi: 10.1007/s00442-008-1043-9
Ling, S. D., Ibbott, S., and Sanderson, J. C. (2010). Recovery of canopy-forming macroalgae following removal of the enigmatic grazing sea urchin Heliocidaris erythrogramma. J. Exp. Mar. Biol. Ecol. 395, 135–146. doi: 10.1016/j.jembe.2010.08.027
Ling, S. D., and Johnson, C. R. (2009). Population dynamics of an ecologically important range-extender: kelp beds versus sea urchin barrens. Mar. Ecol. Prog. Ser. 374, 113–125. doi: 10.3354/meps07729
Ling, S. D., Johnson, C. R., Frusher, S. D., and Ridgway, K. R. (2009). Overfishing reduces resilience of kelp beds to climate-driven catastrophic phase shift. Proc. Natl. Acad. Sci. U.S.A. 106, 22341–22345. doi: 10.1073/pnas.0907529106
Ling, S. D., Kriegisch, N., Woolley, B., and Reeves, S. E. (2019). Density-dependent feedbacks, hysteresis, and demography of overgrazing sea urchins. Ecology 100, e02577. doi: 10.1002/ecy.2577
Ling, S. D., Reeves, S. E., and Kriegisch, N. (2020). Octocoral barrier to grazing sea urchins allows macroalgal recovery on barrens ground. J. Exp. Mar. Biol. Ecol. 524, 151292. doi: 10.1016/j.jembe.2019.151292
Ling, S. D., Scheibling, R. E., Rassweiler, A., Johnson, C. R., Shears, N., Connell, S. D., et al. (2015). Global regime shift dynamics of catastrophic sea urchin overgrazing. Philos. Trans. R. Soc. Ser. B Biol. Sci. 370, 20130269. doi: 10.1098/rstb.2013.0269
Lisson, D.. (2018). Maintaining Healthy Abalone Reef Systems on Tasmania's East Coast, Version 4.0. North Hobart, TAS: Tasmanian Abalone Council Ltd.
Low, C. J.. (1975). The effect of grouping of strongylocentrotus franciscanus, the giant red sea urchin, on its population biology (Doctoral dissertation). Vancouver: University of British Columbia.
Malakhoff, K. D., and Miller, R. J. (2021). After 15 years, no evidence for trophic cascades in marine protected areas. Proc. R. Soc. Ser. B Biol. Sci. 288, 20203061. doi: 10.1098/rspb.2020.3061
Mann, K. H.. (1973). Seaweeds: their productivity and strategy for growth. Science 182, 975–981. doi: 10.1126/science.182.4116.975
Marauder Robotics (2021). Bringing Balance to the Oceans. Available online at: http://balancedoceans.com/ (accessed July 13, 2021).
Marzloff, M. P., Little, L. R., and Johnson, C. R. (2016). Building resilience against climate-driven shifts in a temperate reef system: staying away from context-dependent ecological thresholds. Ecosystems 19, 1–15. doi: 10.1007/s10021-015-9913-6
McClanahan, T., Arthur, R., Kaunda-Arara, B., Kiambo, R., Machano, H., Mangi, S., et al. (2002). Sea urchin reduction as a restoration technique in a new marine park. Paper presented at the Proceedings of the Ninth International Coral Reef Symposium, Bali, 23–27 October 2000.
McClanahan, T. R., Graham, N. A., Calnan, J. M., and MacNeil, M. A. (2007). Toward pristine biomass: reef fish recovery in coral reef marine protected areas in Kenya. Ecol. Appl. 17, 1055–1067. doi: 10.1890/06-1450
Medrano, A., Hereu, B., Cleminson, M., Pagès-Escolà, M., Rovira, G.l, Solà, J., and Linares, C. (2020). From marine deserts to algal beds: Treptacantha elegans revegetation to reverse stable degraded ecosystems inside and outside a no-take marine reserve. Restor. Ecol. 28, 632–644. doi: 10.1111/rec.13123
Micheli, F., Benedetti-Cecchi, L., Gambaccini, S., Bertocci, I., Borsini, C., Osio, G. C., et al. (2005). Cascading human impacts, marine protected areas, and the structure of mediterranean reef assemblages. Ecol. Monogr. 75, 81–102. doi: 10.1890/03-4058
Miller, R. J., and Nolan, S. C. (2008). Management methods for a sea urchin dive fishery with individual fishing zones. J. Shellfish Res. 27, 929–938. doi: 10.2983/0730-8000(2008)27[929:MMFASU]2.0.CO;2
Miller, S., and Abraham, E. (2011). Characterisation of New Zealand Kina Fisheries. Wellington: Ministry of Fisheries.
Mooney, R. C.. (2001). The effects of variable removal levels of the sea urchin, strongylocentrotus franciscanus, on near-shore rocky communities in the traditional territory of the hesquiat first nation (Doctoral dissertation), Vancouver: University of British Columbia.
Morris, R. L., Hale, R., Strain, E. M. A., Reeves, S. E., Vergés, A., Marzinelli, E. M., et al. (2020). Key principles for managing recovery of kelp forests through restoration. Bioscience 70, 688–698. doi: 10.1093/biosci/biaa058
Norderhaug, K. M., and Christie, H. C. (2009). Sea urchin grazing and kelp re-vegetation in the ne atlantic. Mar. Biol. Res. 5, 515–528. doi: 10.1080/17451000902932985
North, W. J., and Shaefer, M. B. (1963). Kelp Habitat Improvement Project. Final Report. San Diego: University of California; Institute of Marine Research.
Paine, R. T.. (1980). Food webs: linkage, interaction strength and community infrastructure. J. Anim. Ecol. 49, 667–685. doi: 10.2307/4220
Pert, C. G., Swearer, S. E., Dworjanyn, S., Kriegisch, N., Turchini, G. M., Francis, D. S., et al. (2018). Barrens of gold: gonad conditioning of an overabundant sea urchin. Aquacult. Environ. Interact. 10, 345–361. doi: 10.3354/aei00274
Prince, J.. (1995). Limited effects of the sea urchin Echinometra mathaei (De Blainville) on the recruitment of benthic algae and macroinvertebrates into intertidal rock platforms at ROTTNEST Island, Western Australia. J. Exp. Mar. Biol. Ecol. 186, 237–258. doi: 10.1016/0022-0981(94)00161-6
QGIS Development Team (2020). Qgis Geographic Information System. Open Source Geospatial Foundation Project.
R Core Team (2020). R: A Language and Environment for Statistical Computing. Vienna: Foundation for Statistical Computing.
Reed, D., Schroeter, S., and Page, M. (2017). Annual Report of the Status of Condition C: Kelp Reef Mitigation. San Diego, CA: University of California.
Ripple, W. J., Estes, J. A., Schmitz, O. J., Constant, V., Kaylor, M. J., Lenz, A., et al. (2016). What is a trophic cascade?. Trends Ecol. Evol. 31, 842–849. doi: 10.1016/j.tree.2016.08.010
Rogers-Bennett, L., and Catton, C. A. (2019). Marine heat wave and multiple stressors tip bull kelp forest to sea urchin barrens. Sci. Rep. 9, 1–9. doi: 10.1038/s41598-019-51114-y
Russ, G. R., and Alcala, A. C. (1996). Marine reserves: rates and patterns of recovery and decline of large predatory fish. Ecol. Appl. 6, 947–961. doi: 10.2307/2269497
Sala, E., Boudouresque, C. F., and Harmelin-Vivien, M. (1998). Fishing trophic cascades, and the structure of algal assemblages: evaluation of an old but untested paradigm. Oikos 82, 425–439. doi: 10.2307/3546364
Sanderson, J. C., Ling, S. D., Dominguez, J. G., and Johnson, C. R. (2016). Limited effectiveness of divers to mitigate 'barrens' formation by culling sea urchins while fishing for abalone. Mar. Freshwat. Res. 67, 84–95. doi: 10.1071/MF14255
Shears, N. T., and Babcock, R. C. (2002). Marine reserves demonstrate top-down control of community structure on temperate reefs. Oecologia 132, 131–142. doi: 10.1007/s00442-002-0920-x
Shears, N. T., and Babcock, R. C. (2003). Continuing trophic cascade effects after 25 years of no-take marine reserve protection. Mar. Ecol. Prog. Ser. 246, 1–16. doi: 10.3354/meps246001
Shears, N. T., Babcock, R. C., and Salomon, A. K. (2008). Context-dependent effects of fishing: variation in trophic cascades across environmental gradients. Ecol. Appl. 18, 1860–1873. doi: 10.1890/07-1776.1
Smale, D. A.. (2020). Impacts of ocean warming on kelp forest ecosystems. New Phytol. 225, 1447–1454. doi: 10.1111/nph.16107
Spyksma, A. J. P., Shears, N. T., and Taylor, R. B. (2017). Predators indirectly induce stronger prey through a trophic cascade. Proc. R. Soc. Ser. B Biol. Sci. 284, 20171440. doi: 10.1098/rspb.2017.1440
Steneck, R. S.. (2020). “Regular sea urchins as drivers of shallow benthic marine community structure,” in Developments in Aquaculture and Fisheries Science, ed J. M. Lawrence (London: Elsevier), 255–279.
Steneck, R. S., Graham, M. H., Bourque, B. J., Corbett, D., Erlandson, J. M., Estes, J. A., et al. (2002). Kelp forest ecosystems: biodiversity, stability, resilience and future. Environ. Conserv. 29, 436–459. doi: 10.1017/S0376892902000322
Steneck, R. S., and Johnson, C. R. (2014). “Kelp forests: dynamic patterns, processes, and feedbacks,” in Marine Community Ecology, eds M. D. Bertness, J. Bruno, B. R. Silliman, and J. J. Stachowicz (Sunderland, MA: Sinauer Associates, Inc), 315–336.
Steneck, R. S., Leland, A., McNaught, D. C., and Vavrinec, J. (2013). Ecosystem flips, locks, and feedbacks: the lasting effects of fisheries on maine's kelp forest ecosystem. Bull. Mar. Sci. 89, 31–55. doi: 10.5343/bms.2011.1148
Stewart, G. B., Kaiser, M. J., Côté, I. M., Halpern, B. S., Lester, S. E., Bayliss, H. R., et al. (2009). Temperate marine reserves: global ecological effects and guidelines for future networks. Conserv. Lett. 2, 243–253. doi: 10.1111/j.1755-263X.2009.00074.x
Strain, E. M., Thomson, R. J., Micheli, F., Mancuso, F. P., and Airoldi, L. (2014). Identifying the interacting roles of stressors in driving the global loss of canopy-forming to mat-forming algae in marine ecosystems. Global Change Biol. 20, 3300–3312. doi: 10.1111/gcb.12619
Strain, E. M., van Belzen, J., van Dalen, J., Bouma, T. J., and Airoldi, L. (2015). Management of local stressors can improve the resilience of marine canopy algae to global stressors. PLoS ONE 10, e0120837. doi: 10.1371/journal.pone.0120837
Strand, H. K., Christie, H., Fagerli, C. W., Mengede, M., and Moy, F. (2020). Optimizing the use of quicklime (Cao) for sea urchin management—a lab and field study. Ecol. Eng. X. 143:100018. doi: 10.1016/j.ecoena.2020.100018
Sun, J., and Chiang, F. S. (2015). “Use and exploitation of sea urchins,” in Echinoderm Aquaculture, eds N.P. Brown and S.D. Eddy (Hoboken, NJ: John Wiley and Sons), p. 25–45. doi: 10.1002/9781119005810.ch2
Suskiewicz, T. S., and Johnson, L. E. (2017). Consumption rates of a key marine herbivore: a review of the extrinsic and intrinsic control of feeding in the green sea urchin. Mar. Biol. 164, 131. doi: 10.1007/s00227-017-3159-0
Taino, S.. (2010). Different effects on seaweed succession after sea urchin removal at several coastal waters in Tosa Bay, Southern Japan. Bull. Fisheries Res. Agency 32, 61–67.
Teagle, H., Hawkins, S. J., Moore, P. J., and Smale, D. A. (2017). The role of kelp species as biogenic habitat formers in coastal marine ecosystems. J. Exp. Mar. Biol. Ecol. 492, 81–98. doi: 10.1016/j.jembe.2017.01.017
Thomas, S. F.. (2011). Translocation of Evechinus Chloroticus in the Sur 5 Fishery and the Reproductive Potential of Large Urchins. Dunedin: University of Otago.
Tracey, S., Mundy, C., Baulch, T., Marzloff, M., Hartmann, K., Ling, S., et al. (2014). Trial of an Industry Implemented, Spatially Discrete Eradication/Control Program for Centrostephanus rodgersii in Tasmania. Institute for Marine and Antarctic Studies. Canberra, TAS: Fisheries Research and Development Corporation.
Tracey, S. R., Baulch, T., Hartmann, K., Ling, S. D., Lucieer, V., Marzloff, M. P., et al. (2015). Systematic culling controls a climate driven, habitat modifying invader. Biol. Invasions 17, 1885–1896. doi: 10.1007/s10530-015-0845-z
Urchinomics (2020). Urchinomics Impact. Available online at: https://www.urchinomics.com/impact (accessed April 21, 2020).
Vanderklift, M. A., and Kendrick, G. A. (2005). Contrasting influence of sea urchins on attached and drift macroalgae. Mar. Ecol. Prog. Ser. 299, 101–110. doi: 10.3354/meps299101
Vergés, A., Steinberg, P. D., Hay, M. E., Poore, A. G. B., Campbell, A. H., Ballesteros, E., et al. (2014). The tropicalization of temperate marine ecosystems: climate-mediated changes in herbivory and community phase shifts. Proc. R. Soc. Ser. B Biol. Sci. 281, 20140846. doi: 10.1098/rspb.2014.0846
Villouta, E., Chadderton, W. L., Pugsley, C. W., and Hay, C. H. (2001). Effects of sea urchin (Evechinus chloroticus) grazing in dusky sound, Fiordland, New Zealand. N. Z. J. Mar. Freshwat. Res. 35, 1007–1024. doi: 10.1080/00288330.2001.9517060
Watanuki, A., Aota, T., Otsuka, E., Kawai, T., Iwahashi, Y., Kuwahara, H., et al. (2010). Restoration of kelp beds on an urchin barren: removal of sea urchins by citizen divers in Southwestern Hokkaido. Bull. Fish. Res. Agency 32, 83–87.
Watson, J., and Estes JStability, A. (2011). Stability, Resilience, and phase shifts in rocky subtidal communities along the west coast of Vancouver Island, Canada. Ecol. Monogr. 81, 215–239. doi: 10.1890/10-0262.1
Wernberg, T., Krumhansl, K., Filbee-Dexter, K., and Pedersen, M. F. (2019). “Status and trends for the world's kelp forests,” in World Seas: An Environmental Evaluation, ed C. Sheppard (London: Elsevier), 57–78.
Westermeier, R., Murúa, P., Patiño, D. J., Muñoz, L., and Müller, D. G. (2016). Holdfast fragmentation of Macrocystis pyrifera (Integrifolia Morph) and Lessonia berteroana in Atacama (Chile): a novel approach for kelp bed restoration. J. Appl. Phycol. 28, 2969–2977. doi: 10.1007/s10811-016-0827-2
Williams, J. P., Claisse, J. T., Pondella, D. J. I. I., Williams, C. M., Robart, M. J., et al. (2021). Sea urchin mass mortality rapidly restores kelp forest communities. Mar. Ecol. Prog. Ser. 664, 117–131. doi: 10.3354/meps13680
Keywords: urchin barrens, trophic cascade, phase-shift, kelp restoration, habitat restoration, ecosystem management, rocky reef, canopy-forming algae
Citation: Miller KI, Blain CO and Shears NT (2022) Sea Urchin Removal as a Tool for Macroalgal Restoration: A Review on Removing “the Spiny Enemies”. Front. Mar. Sci. 9:831001. doi: 10.3389/fmars.2022.831001
Received: 07 December 2021; Accepted: 20 January 2022;
Published: 24 February 2022.
Edited by:
Cataldo Pierri, University of Bari Aldo Moro, ItalyReviewed by:
Mariachiara Chiantore, University of Genoa, ItalyGiovanni Fanelli, National Research Council, Italy
Copyright © 2022 Miller, Blain and Shears. This is an open-access article distributed under the terms of the Creative Commons Attribution License (CC BY). The use, distribution or reproduction in other forums is permitted, provided the original author(s) and the copyright owner(s) are credited and that the original publication in this journal is cited, in accordance with accepted academic practice. No use, distribution or reproduction is permitted which does not comply with these terms.
*Correspondence: Kelsey I. Miller, kmil284@aucklanduni.ac.nz