A Methodological Safe-by-Design Approach for the Development of Nanomedicines
- 1Technology and Society Laboratory, Empa – Swiss Federal Laboratories for Materials Science and Technology, St. Gallen, Switzerland
- 2Center for Neuroscience and Cell Biology, Faculty of Pharmacy, University of Coimbra, Coimbra, Portugal
- 3School of Pharmaceutical Sciences Geneva-Lausanne, Geneva, Switzerland
- 4Polymer Engineering Laboratory, Department of Innovative Technologies, Mechanical Engineering and Materials Technology Institute, University of Applied Sciences and Arts of Southern Switzerland, Manno, Switzerland
- 5Institute of Life Technologies, University of Applied Sciences and Arts Western Switzerland (HES-SO Valais-Wallis), Sion, Switzerland
- 6National Institute for Public Health and the Environment, Bilthoven, Netherlands
- 7Particles-Biology Interactions Lab, Empa – Swiss Federal Laboratories for Materials Science and Technology, St. Gallen, Switzerland
Safe-by-Design (SbD) concepts foresee the risk identification and reduction as well as uncertainties regarding human health and environmental safety in early stages of product development. The EU’s NANoREG project and further on the H2020 ProSafe initiative, NanoReg2, and CALIBRATE projects have developed a general SbD approach for nanotechnologies (e.g., paints, textiles, etc.). Based on it, the GoNanoBioMat project elaborated a methodological SbD approach (GoNanoBioMat SbD approach) for nanomedicines with a focus on polymeric nanobiomaterials (NBMs) used for drug delivery. NBMs have various advantages such as the potential to increase drug efficacy and bioavailability. However, the nanoscale brings new challenges to product design, manufacturing, and handling. Nanomedicines are costly and require the combination of knowledge from several fields. In this paper, we present the GoNanoBioMat SbD approach, which allows identifying and addressing the relevant safety aspects to address when developing polymeric NBMs during design, characterization, assessment of human health and environmental risk, manufacturing and handling, and combines the nanoscale and medicine field under one approach. Furthermore, regulatory requirements are integrated into the innovation process.
Introduction
The concept of Safe-by-Design (SbD) was addressed in the field of nanotechnology because of the continuous uncertainty about the potentially harmful effects of nanomaterials on humans and the environment. Its implementation started with the Dutch NanoNextNL program1 and the European NANoREG project and was further developed by the H2020 ProSafe initiative and H2020 NanoReg2 project (Soeteman-Hernandez et al., 2019). Since then, an increasing number of European Union projects focused on SbD for nanomaterials (Lynch, 2017). Even though various concepts of SbD coexist, they share the purpose of assessing safety as early as possible in the innovation process of a nanomaterial or nanoproducts. They aim at reducing adverse effects on human health and the environment by altering nanoproduct design (Soeteman-Hernandez et al., 2019) and by ensuring safety along its lifecycle (Bottero et al., 2017; Kraegeloh et al., 2018). The SbD concept is therefore different from conventional risk assessment approaches, which only consider safety when the product is already fully developed (Schwarz-Plaschg et al., 2017).
Despite being a rather novel concept in the context of nanotechnology, the principle behind SbD is not new and already applied by other industries (Kraegeloh et al., 2018). The medicine field has also long expertise in ensuring safety throughout the drug discovery and development process (Hjorth et al., 2017). However, how to handle safety issues effectively at the very beginning of drug development, to allow the selection of drug candidates, and mitigate toxicity is still being investigated (Kramer et al., 2007; Loiodice et al., 2019). The concept of Quality-by-Design (QbD) is widely used by pharmaceutical industry and its implementation is foreseen by the pharmaceutical development guidelines. The SbD is a new concept for the Pharmaceutical industry and it is not yet included in ICH, EMA, or FDA guidelines. This means that even if safety is considered during the pharmaceutical development, there is no systematic SbD approach yet in place. The concept of QbD presupposes the definition of the critical quality attributes (CQA) that will lead to the achievement of a product with proven effectiveness and the SbD would establish CQA that will lead to a product with high safety.
The application of nanotechnology in the medicine field (nanomedicine) brought new barriers precluding the prediction of potential adverse effects to human health and the environment because of the complexity of nanobiomaterials (NBMs). The unpredictability of nanomedicines’ interaction with biological systems makes it difficult to bring these to the market (Resnik and Tinkle, 2007; Accomasso et al., 2018) and consequently, their potential benefits in medicine are still underexploited (Tinkle et al., 2014; Troiano et al., 2016). The lack of guidelines, standards and tools adapted to nanomedicines for assessing their risks represents one of the causes for this situation (Accomasso et al., 2018).
The development of such products remains therefore challenging. In addition, nanomedicines are costly and based on an interdisciplinary approach. They are at the junction of pharma, medtech, biotech and nanotech companies, and academia, which are important economic and social players in Switzerland and Europe. These companies may have different roles in the value chain of nanomedicines’ development and as they have different backgrounds, they may have various needs to overcome the complexity of nanotechnology for medical applications.
As there is no systematic SbD approach in place for nanomedicines and that not all actors (coming from different fields) are experienced in considering safety to reduce risks on human health and the environment, there is a need for a methodological approach enabling to consider all necessary aspects to evaluate the safety of nanomedicines early during product development. This would ultimately improve the efficiency of the innovation process and the collaboration of all involved interdisciplinary actors and thus ensure the development of a safe product from the beginning of the process.
In order to fill this gap, within the GoNanoBioMat project,2 we aimed at elaborating a methodological SbD approach by taking up the principles of the SbD approach developed for nanotechnologies in general and by adapting it to the field of nanomedicines. The developed methodological approach has a focus on polymeric nanocarriers for drug delivery (Som et al., 2019) as they are valuable materials, widely used to prepare nanoparticles and microparticles for the purpose of encapsulating drugs (Etheridge et al., 2013), can be biodegradable, biocompatible, and can be tailored to have targeting abilities (Bennet and Kim, 2014; Moritz and Geszke-Moritz, 2015). Therefore, these materials are expected to increase drug efficacy and safety (Ariën and Stoffels, 2016).
The aim of this paper is (1) to present what we adapted from the SbD concept developed within the EU projects NANoREG and NanoReg2, and the ProSafe initiative (hereafter general SbD approach) for the field of nanomedicines and (2) present the methodological SbD approach (hereafter the GoNanoBioMat SbD approach).
Adaptation of the General SbD Approach to Nanomedicines
The general SbD approach can be applied in many different fields (e.g., paints, textiles, etc.), is addressed to industries, and can be used by regulators as a reference tool (Kraegeloh et al., 2018). Its goal is to “reduce uncertainties and risks of human and environmental safety of nanotechnology, starting as early as possible during the innovation process, on the basis of mandatory and voluntary safety and efficacy compliance requirements” (Soeteman-Hernandez et al., 2019). The main elements of the general SbD approach are: (1) it uses a stage-gate innovation approach, (2) it is based on three pillars, which are Safe materials and products, Safe production, and Safe use and end-of-life; (3) it includes SbD action for maximizing safety while maintaining functionality, and (4) it is integrated into a Safe Innovation Approach (see extensive description in Kraegeloh et al., 2018; Soeteman-Hernandez et al., 2019). Below we show how we changed or adapted these elements of the general SbD approach to nanomedicines (the comparison can be seen in Table 1).
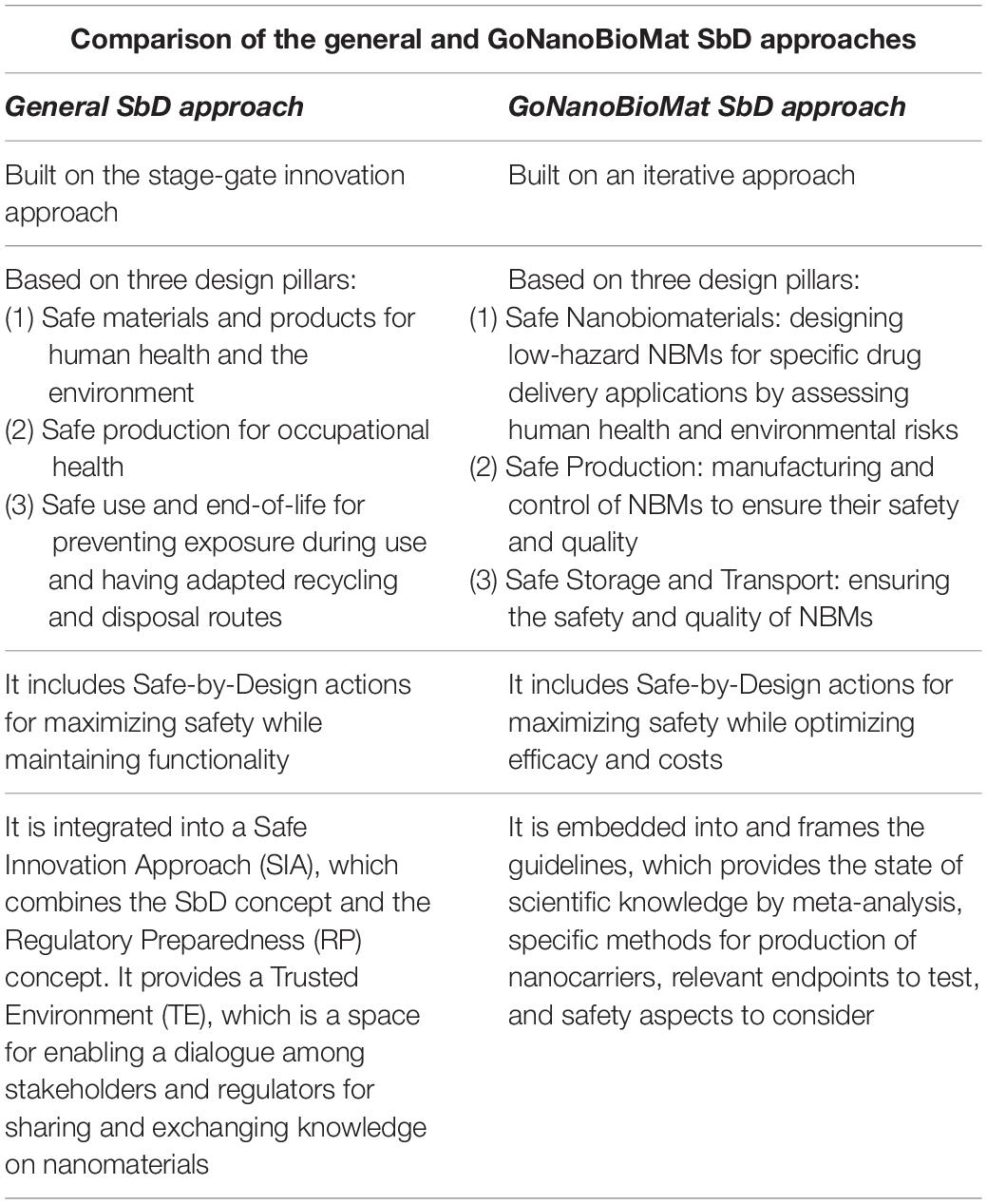
Table 1. Comparison of the general SbD approach developed by NANoREG, NanoReg2, and the ProSafe initiative with the GoNanoBioMat SbD approach.
As can be seen in Table 1, the GoNanoBioMat SbD approach is not based on a stage-gate innovation approach. Instead, it is an iterative approach. This decision was made in order to better represent the reality of “drug discovery and development” field, which also uses an iterative approach (Hjorth et al., 2017). In addition, the iterations are necessary to build up knowledge, as it will be shown in the section “GoNanoBioMat SbD Approach,” on physico-chemical properties and their biological effects. This is because currently, it is not possible to predict these effects only based on literature and modeling.
The GoNanoBioMat SbD approach is also based on three pillars (Table 1), but these were modified to match the scope of the topic at hand. The pillar Safe Nanobiomaterials corresponds to the first pillar of the general SbD approach and has the same aim. In the general and GoNanoBioMat SbD approaches, the second pillar is Safe Production. However, the focus in the GoNanoBioMat SbD approach is not only on the safety of workers but also on ensuring safety and quality of the NBMs and on applying good manufacturing practices (GMP), which are a prerequisite to produce medicines and consequently nanomedicines. On the one hand, the third pillar of the general SbD approach is about Safe use and end-of-life. Its main goal is to prevent exposure during use and to have adapted recycling and disposal routes. On the other hand, the third pillar of the GoNanoBioMat SbD approach is about Safe Storage and Transport in order to ensure the safety and quality of NBMs because they may experience transformations (Cobaleda-Siles et al., 2017), which may affect their safety and quality (USP36–NF31,2012; European Commission, 2013). Storage, and more particularly shelf-life, is an aspect being highly connected to the logistics and costs of the final nanomedicine and therefore its viability on the market (Diven et al., 2015). As can be seen, here the pillar is a bit narrower than in the general approach. This is to better represent the needs for developing nanomedicines.
Both approaches include SbD actions (Table 1). The difference between the two is that the functionality is specified into efficacy in the GoNanoBioMat SbD approach. It was changed into efficacy because efficacy is a measurement of the successful pharmacological effect of a drug and therefore more representative for developing nanomedicines. The goal of these SbD actions is to maximize safety while optimizing efficacy and costs by comparing different forms of NBMs. However, it should be pointed out that sometimes it is not feasible to maximize both efficacy and safety at the same time (Soeteman-Hernandez et al., 2019). Optimization will always require iterations in order to be able to balance efficacy and safety (Hjorth et al., 2017).
Finally, in Table 1 it is possible to see that the general SbD approach is integrated into a Safe Innovation Approach and provides a Trusted Environment (Soeteman-Hernandez et al., 2019). The Safe Innovation Approach combines the SbD concept and the Regulatory Preparedness concept. The Regulatory Preparedness concept being the improvement of anticipation of regulators to keep up with the fast growing knowledge on nanomaterials and thus facilitate the development of adaptable regulations. The Trusted Environment is a space for enabling a dialogue among stakeholders and regulators for sharing and exchanging knowledge on nanomaterials. The GoNanoBioMat SbD approach, however, is embedded into and sets the frame for a document whose title is “Guidelines for implementing a SbD approach for medicinal polymeric nanocarriers” written and published by the GoNanoBioMat project consortium (Som et al., 2019). The guidelines provide the state of scientific knowledge with meta-analyses, decision trees, methods for producing NBMs, relevant endpoints to test, and safety aspects to consider early and throughout the development of polymeric NBMs for drug delivery. The guidelines can be downloaded under this link: www.empa.ch/gonanobiomat.
GoNanoBioMat SbD Approach
As mentioned, the GoNanoBioMat SbD approach is a methodological approach for developing nanomedicines with a focus on polymeric NBMs for drug delivery and is presented in Figure 1. It contains the following steps: Material Design, Characterization, Human Health and Environmental Risks (first pillar), Manufacturing and Control (second pillar), and Storage and Transport (third pillar). The regulatory framework for developing nanomedicines is also included within the approach starting at the end of the Material Design step. The bullet points inside the boxes correspond to methods and tools that can be used or endpoints that should be considered and tested in each step. The blue arrows represent the flow of polymeric NBMs from their design until their storage and transport. The red arrows are feedback loops (iterations) going back to the Material Design step.
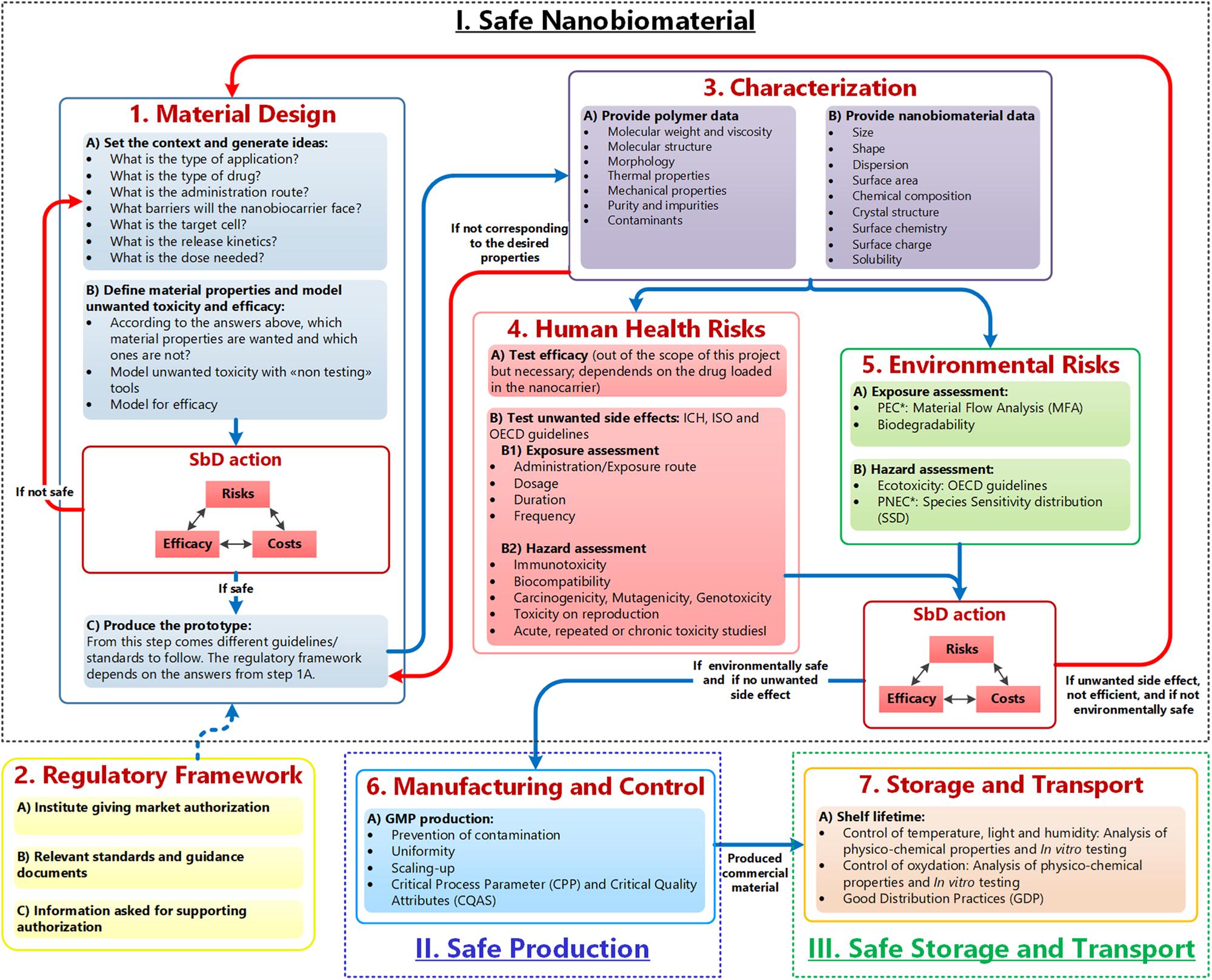
Figure 1. GoNanoBioMat SbD approach. The blue arrows correspond to the flow of polymeric NBMs from design to storage and transport. The red arrows are feedback loops used whenever the NBM is unsafe, or inefficient. Adapted from Som et al. (2019). *PEC, predicted environmental concentration; PNEC, predicted no effect concentration.
It is important to note, that most of these steps also apply to other NBMs and other type of nanomedicine applications. For example, the Human Health and Environmental Risks steps could be applied to any type of NBMs. However, in the Material Design step and in the Characterization step, specific questions (e.g., what is the type of drug and what is the release kinetics) for drug delivery and specific parameters to characterize polymers are provided, respectively. Therefore, the total of questions only applies to polymeric NBMs for drug delivery, even if many questions also apply to other NBMs or other applications.
As can be seen in Figure 1, the GoNanoBioMat SbD approach starts with the Material Design step. This step is divided into three sub-steps, which are (a) set the context and generate ideas, (b) define material properties and screen for unwanted toxicity and efficacy, and (c) produce the prototype.
In the first sub-step, a set of questions can be used to guide the conceptual process for developing NBMs for drug delivery, and searching for the relevant literature. The questions include the type of application, type of drug (possibility of chemical interaction between drug and polymer), administration route, the biological barriers, target cells, release kinetics, and dose needed. All these aspects influence the design of nanocarriers (Elsabahy and Wooley, 2012), in other words, its physicochemical properties to be efficient as a drug delivery system and lining up for safe application. An important consideration to bear in mind is that the properties of the polymer (particles larger than 1 micron) may not be equal to the properties of the polymer when the size of its particles is reduced to the nanoscale. Once the data from literature are collected, the data can be used to screen for efficacy but also toxicity and to define the wished material properties of the nanocarriers (second sub-step) by using modeling tools (i.e., non-testing tools), such as quantitative structure–activity relationship tools (OECD, 2007). These tools have for aim to find a correlation between NBMs properties and their corresponding effect (e.g., cell internalization, cytotoxicity) and may enable to assess whether a material is safe for medical purposes. However, it has to be noted that such methods still need to be further developed.
As aspects of safety and functionality should be taken into account at the very beginning of the project’s conception (Cobaleda-Siles et al., 2017), these two sub-steps based on literature and modeling are facilitating their consideration. However, assessing the human health risks in an early stage of innovation only based on data found in the literature is currently not adequate. This may be a result of the lack of standardized assays, which lead to a high variation in reported studies (Hofmann-Amtenbrink et al., 2015). Also some studies have no proper characterization and lack appropriate controls specific to the nanoscale (Jesus et al., 2019), which makes comparisons between toxicity outcomes difficult. Therefore, experimental studies are still needed.
After these two sub-steps, comes the first SbD action. Its goal is to compare different possible NBMs for the intended use/application, which was defined in the beginning of the Material Design step, and to select the NBMs having a good balance between, safety, efficacy, and costs. After this, the selected NBMs should be produced as prototypes.
These prototypes should be then characterized in order to be able to find relationships between physicochemical properties of NBMs and their biological effects, and thus apply the concept of SbD. As can be seen in Figure 1, the properties attributed to the polymer itself (e.g., molecular weight) and the properties attributed to the nanosize (e.g., size) should be characterized. If the desired properties of the prototypes do not correspond to the measured properties, the prototypes should go back to the prototype production sub-step in order to optimize the production process. One criterion in SbD requires understanding the variables contributing to undesired side effects (Lin et al., 2018). Therefore, to have a thorough characterization of polymeric NBMs, the Characterization step includes specific parameter to be tested for polymers NMBs, such as molecular weight, size and surface area. This step is also essential to determine later the CQAs, which are defined as “physical, chemical, biological, or microbiological properties or characteristics that should be within an appropriate limit, range, or distribution to ensure the desired product quality” (ICH Q8 (R2), 2009).
The next two steps are experimental steps to evaluate the human health and the environmental risks of the selected NBMs. For both, the exposure and the hazard should be evaluated. For the Human Health Risks step, the route of administration/exposure, the dosage, the duration and frequency should be determined as safety of NBMs depends on the route of administration/exposure and the resulting respective pharmacokinetic profiles (Jesus et al., 2019). For the hazard, the following endpoints should be tested: immunotoxicity, biocompatibility, carcinogenicity, mutagenicity, genotoxicity, toxicity on reproduction, acute, repeated, or chronic toxicity studies. All tested endpoints should as well include appropriate controls for the nanoscale. The proposed endpoints are in line with current regulation (Jesus et al., 2019).
In parallel, the assessment of the environmental risks should be performed. To do so, the predicted environmental concentration and the predicted no effect concentration have to be calculated (Hauser et al., 2019). The former can be assessed via a material flow analysis and the latter via performing a (probabilistic) species sensitivity distribution. For this, ecotoxicity data are needed, which can be obtained either via literature or experimentally by following OECD guidelines.
After the Human Health and Environmental risks steps comes the second SbD action. As for the first one, the goal is to compare the selected NBMs and choose the one maximizing safety, while optimizing efficacy and costs. At this point, either one NBM is selected as the final candidate or if no NBMs have a good balance between benefits and risks, the developer should go back to the Material Design step. The results of these two steps can help to build up a useful database. In other words, with iterations, a database with the experimental results could be established and these data could be used for modeling. Ultimately, it would enable better predictions of NMBs’ efficacy and toxicity.
If one final candidate has been selected, the developer of NBMs should go to the Manufacturing and Control step. The goal of this step is to scale-up the production by applying GMP, preventing contamination and ensuring uniformity between the batches. In this step, CQAs of NBMs must be identified as well as Critical Process Parameters. These are defined as the “process parameters that influence CQAs and therefore should be monitored or controlled to ensure the process produces the desired quality” (ICH Q8 (R2), 2009). It can be noted that this step is typically valid for any type of NBMs.
After scale-up, usually the nanocarrier and their encapsulated drug system would go to clinical trials. However, as we did not include clinical trials in the approach because it was out of the scope of the project, the next step is Storage and Transport. The (nano)medicine stability studies have to be performed (SME Office, 2016; MDR, 2017), because nanocarriers and encapsulated drug, both, or just one of them, might experience degradation process during their life cycle, which might affect the quality and safety of the nanomedicine (Cobaleda-Siles et al., 2017).
Finally, the Swiss and European regulatory frameworks for the marketing authorization of nanomedicine is embedded within the GoNanoBioMat SbD approach. More information on this aspect can be directly found in the GoNanoBioMat guidelines.3
Discussion
In case of nanomedicines, SbD approaches should be included in the International Council for Harmonisation of Technical Requirements for Pharmaceuticals for Human Use (ICH) guidelines and relevant OECD guidance and guidelines. ICH is unique in bringing together the regulatory authorities and pharmaceutical industry to discuss scientific and technical aspects of drug registration and thus to discuss what should be included within guidelines concerning the safety of the NBMs and nanomedicines.
The GoNanoBioMat SbD approach is methodological, contains all important elements to consider in order to integrate safety early and throughout the development of polymeric NBMs for drug delivery. It can as well to a certain extent be applied to other types of NBMS and nanomedicine applications. Including safety in the design of NBMs is an important aspect, especially for nanomedicines, which are highly regulated, cost and time consuming, and complex. However, the approach should not be seen as a warranty of complete safety, because absolute safety is unreachable (Cobaleda-Siles et al., 2017; Hjorth et al., 2017; van de Poel and Robaey, 2017), and should therefore be considered as a design strategy (van de Poel and Robaey, 2017) since the past showed that each nanomedicine has to be taken as case-by-case (McNeil, 2009). As for the general SbD concept, it has no legal binding and does not replace regulatory requirements (Soeteman-Hernandez et al., 2019).
The GoNanoBioMat SbD approach was focusing only on the safety of nanocarriers (polymeric NBMs) and not on the nanocarriers and its encapsulated drug. For regulatory purpose, it is necessary to test the safety of the nanocarrier alone in addition to the nanocarrier/drug system. Therefore, this GoNanoBioMat SbD approach is a first step toward the integration of safety early in the development of such products. Efficacy, which is closely related to the drug used, could not be included in the approach and therefore must be evaluated case-by-case. In a future development of the GoNanoBioMat SbD approach adding steps for clinical trials and use will be developed.
Finally, we believe that the GoNanoBioMat SbD approach as presented here may facilitate the implementation of the general SbD concept and to find a balance between benefits and risks by comparing different nanocarrier candidates in terms of their respective safety, efficacy, and costs. For instance, the GoNanoBioMat SbD approach provides all relevant steps for developing polymeric NBMs; provides methodology and endpoints to test human health and environmental risks, which are in line with current regulations; is an iterative process; and combines the nanoscale and medicine field under one methodological approach. In addition, the approach may bring the different actors of the value chain on a common ground. Ultimately, the approach may enable to move toward safe and efficient NBMs, safe production, and safe storage and transport.
Author COntributions
MS created the GoNanoBioMat SbD approach in collaboration with CS, OB, SJ, PW, GB, GP, ÄS, and LS-H. MS wrote the manuscript. CS wrote some part of the manuscript. OB, SJ, PW, GB, GP, ÄS, and LH read and reviewed the manuscript. All authors approved the submitted version.
Funding
This study is part of the GoNanoBioMat project and has received funding from the Horizon 2020 framework program of the European Union, ProSafe Joint Transnational Call 2016; CTI (1.1.2018 Innosuisse), under grant agreement Number 19267.1 PFNM-NM; and FCT Foundation for Science and Technology under the project PROSAFE/0001/2016.
Conflict of Interest
The authors declare that the research was conducted in the absence of any commercial or financial relationships that could be construed as a potential conflict of interest.
Footnotes
- ^ www.nanonextnl.nl
- ^ The GoNanoBioMat project was initiated by the ProSafe initiative
- ^ www.empa.ch/gonanobiomat
References
Accomasso, L., Cristallini, C., and Giachino, C. (2018). Risk assessment and risk minimization in nanomedicine: a need for predictive, alternative, and 3Rs Strategies. Front. Pharmacol. 9:228. doi: 10.3389/fphar.2018.00228
Ariën, A., and Stoffels, P. (2016). “History: potential, challenges, and future development in nanopharmaceutical research and industry,” in Pharmaceutical Nanotechnology: Innovation and Production, eds J. Cornier, A. Owen, A. Kwade, and M. H. Vander Voorde (Hoboken, NJ: Wiley), 3–12.
Bennet, D., and Kim, S. (2014). “Polymer nanoparticles for smart drug delivery,” in Application of Nanotechnology in Drug Delivery, ed. A. D. Sezer (London: IntechOpen), 257–310.
Bottero, J. Y., Rose, J., Garidel, C., De, Masion, A., Deutsch, T., et al. (2017). Serenade: safer and ecodesign research and eductaion applied to nanomaterial development, the new generation of materials safer by design. Environ. Sci. Nano 4, 526–538. doi: 10.1039/c6en00282j
Cobaleda-Siles, M., Guillamon, A. P., Delpivo, C., Vázquez-Campos, S., and Puntes, V. F. (2017). Safer by design strategies. J. Phys. Conf. Ser. 838:012016. doi: 10.1088/1742-6596/838/1/012016
Diven, D. G., Bartenstein, D. W., and Carroll, D. R. (2015). Extending shelf life just makes sense. Mayo Clin. Proc. 90, 1471–1474. doi: 10.1016/j.mayocp.2015.08.007
Elsabahy, M., and Wooley, K. L. (2012). Design of polymeric nanoparticles for biomedical delivery applications. Chem. Soc. Rev. 41, 2545–2561. doi: 10.1039/c2cs15327k
Etheridge, M. L., Campbell, S. A., Erdman, A. G., Haynes, C. L., Wolf, S. M., and McCullough, J. (2013). The big picture on nanomedicine: the state of investigational and approved nanomedicine products. Nanomed. Nanotechnol. Biol. Med. 9, 1–14. doi: 10.1016/j.nano.2012.05.013
European Commission. (2013). Information from European Union institutions, bodies, offices and agencies, european commission guidelines of 5 november 2013 on good distribution practice of medicinal products for human use (Text with EEA relevance) (2013/C 343/01). Offi. J. Eur. Union 2013, C343/1–14.
Hauser, M., Li, G., and Nowack, B. (2019). Environmental hazard assessment for polymeric and inorganic nanobiomaterials used in drug delivery. J. Nanobiotechnol. 17, 1–10.
Hjorth, R., Hove, L., and Van, Wickson, F. (2017). What can nanosafety learn from drug development? The feasibility of “safety by design”. Nanotoxicology 11, 305–312. doi: 10.1080/17435390.2017.1299891
Hofmann-Amtenbrink, M., Grainger, D. W., and Hofmann, H. (2015). Nanoparticles in medicine: current challenges facing inorganic nanoparticle toxicity assessments and standardizations. Nanomedicine Nanotechnology Biol. Med. 11, 1689–1694. doi: 10.1016/j.nano.2015.05.005
ICH Q8 (R2) (2009). Requirements for Registration of Pharmaceuticals for Human Use - Guidlines for Elemental Impurities. Guidel: ICH Harmon.
Jesus, S., Schmutz, M., Som, C., Borchard, G., Wick, P., and Borges, O. (2019). Hazard assessment of polymeric nanobiomaterials for drug delivery: what can we learn from literature so far. Front. Bioeng. Biotechnol. 7:261. doi: 10.3389/fbioe.2019.00261
Kraegeloh, A., Suarez-merino, B., Sluijters, T., and Micheletti, C. (2018). Implementation of Safe-by-Design for nanomaterial development and safe innovation: why we need a comprehensive approach. Nanomaterials 8:239. doi: 10.3390/nano8040239
Kramer, J. A., Sagartz, J. E., and Morris, D. L. (2007). The application of discovery toxicology and pathology towards the design of safer pharmaceutical lead candidates. Nat. Rev. Drug Discov. 6, 636–649. doi: 10.1038/nrd2378
Lin, S., Yu, T., Yu, Z., Hu, X., and Yin, D. (2018). Nanomaterials safer-by-design: an environmental safety perspective. Adv. Mater. 30:1705691. doi: 10.1002/adma.201705691
Loiodice, S., Nogueira, da Costa, A., and Atienzar, F. (2019). Current trends in silico, in vitro toxicology, and safety biomarkers in early drug development. Drug Chem. Toxicol. 42, 113–121. doi: 10.1080/01480545.2017.1400044
Lynch, I. (2017). Compendium of Projects in the European NanoSafety Cluster. Birmingham: University of Birmingham, 259.
McNeil, S. E. (2009). Nanoparticle therapeutics: a personal perspective. Wiley Interdiscip. Rev. Nanomed. Nanobiotechnol. 1, 264–271. doi: 10.1002/wnan.6
MDR (2017). Regulation (EU) 2017/745 of the European Parliament and of the Council of 5 April 2017 on Medical Devices, Amending Directive 2001/83/EC, Regulation (EC) No 178/2002 and Regulation (EC) No 11223/2009 and Repealing Council Directives 90/385/EEC and 93/42/EEC. Available online at: https://eur-lex.europa.eu/legal-content/EN/ALL/?uri (accessed October 29, 2019).
Moritz, M., and Geszke-Moritz, M. (2015). Recent developments – In the application of polymeric nanoparticles as drug carriers. Adv. Clin. Exp. Med. 24, 749–758. doi: 10.17219/acem/31802
OECD (2007). Guidance Document on the Validation of (quantitative) Structure-Activity Relationship [(Q)SAR] models. OECD Environment, Health and Safety Publications. Series on Testing and Assessment No. 69. ENV/JM/MONO (2007) 2. Paris: Organisation for Economic Co-operation and Development.
Resnik, D. B., and Tinkle, S. S. (2007). Ethics in nanomedicine. Nanomedicine 2, 345–350. doi: 10.2217/17435889.2.3.345
Schwarz-Plaschg, C., Kallhoff, A., and Eisenberger, I. (2017). Making nanomaterials safer by design? Nanoethics 11, 277–281. doi: 10.1007/s11569-017-0307-4
SME Office (2016). User Guide for Micro, Small and Medium-Sized Enterprises (SMEs). Amsterdam: European Medicines Agency, 44.
Soeteman-Hernandez, L. G., Apostolova, M. D., Bekker, C., Dekkers, S., Grafström, R. C., Groenewold, M., et al. (2019). Safe innovation approach: towards an agile system for dealing with innovations. Mater. Today Commun. 20:100548. doi: 10.1016/j.mtcomm.2019.100548
Som, C., Schmutz, M., Borges, O., Jesus, S., Borchard, G., Nguyen, V., et al. (2019). Guidelines for Implementing a Safe-by-Design Approach for Medicinal Polymeric Nanocarriers. Available online at: www.empa.ch/gonanobiomat
Tinkle, S., Mcneil, S. E., Mühlebach, S., Bawa, R., Borchard, G., Barenholz, Y. C., et al. (2014). Nanomedicines: addressing the scientific and regulatory gap. Ann. N. Y. Acad. Sci. 1313, 35–56. doi: 10.1111/nyas.12403
Troiano, G., Nolan, J., Parsons, D., Van Geen Hoven, C., and Zale, S. (2016). A quality by design approach to developing and manufacturing polymeric nanoparticle drug products. AAPS J. 18, 1354–1365. doi: 10.1208/s12248-016-9969-z
USP36–NF31 (2012). USP36–NF31, G. C 1079 Good Storage and Distribution Practices for Drug Products Rockville, MD: The United States Pharmacopeial Convention.
Keywords: Safe-by-Design, polymeric nanobiomaterials, nanocarriers, drug delivery, nanomedicine
Citation: Schmutz M, Borges O, Jesus S, Borchard G, Perale G, Zinn M, Sips ÄAJAM, Soeteman-Hernandez LG, Wick P and Som C (2020) A Methodological Safe-by-Design Approach for the Development of Nanomedicines. Front. Bioeng. Biotechnol. 8:258. doi: 10.3389/fbioe.2020.00258
Received: 31 October 2019; Accepted: 12 March 2020;
Published: 02 April 2020.
Edited by:
Michele Iafisco, Italian National Research Council, ItalyReviewed by:
Diana Boraschi, Istituto di Biochimica delle Proteine (IBP), ItalyIvana Fenoglio, University of Turin, Italy
Henriqueta Louro, Instituto Nacional de Saúde Doutor Ricardo Jorge, Portugal
Copyright © 2020 Schmutz, Borges, Jesus, Borchard, Perale, Zinn, Sips, Soeteman-Hernandez, Wick and Som. This is an open-access article distributed under the terms of the Creative Commons Attribution License (CC BY). The use, distribution or reproduction in other forums is permitted, provided the original author(s) and the copyright owner(s) are credited and that the original publication in this journal is cited, in accordance with accepted academic practice. No use, distribution or reproduction is permitted which does not comply with these terms.
*Correspondence: Claudia Som, claudia.som@empa.ch