Hydrogen Sulfide: A Worthwhile Tool in the Design of New Multitarget Drugs
- 1Department of Pharmacy, University of Pisa, Pisa, Italy
- 2School of Pharmaceutical Sciences, Sun Yat-Sen University, Guangzhou, China
- 3International Joint Laboratory (SYSU-PolyU HK) of Novel Anti-Dementia Drugs of Guangdong, Guangzhou, China
- 4Guangdong Province Key Laboratory of Brain Function and Disease, Zhongshan School of Medicine, Sun Yat-sen University, Guangzhou, China
H2S is a gaseous molecule able to trigger a plethora of central physiological and pharmacological effects as antioxidant, pro- and anti-inflammatory, pro- and anti-nociceptive, neuromodulator, and cytoprotective. The polypharmacology of H2S depends on the wide variety of targets implicated, but, despite the efforts, the mechanisms of action that should clarify its activity are still not completely unrevealed. Nevertheless, many attempts to exploit the multifaceted profile of this molecule have already been accomplished and many chemical entities containing an H2S-releasing pharmacophore have been synthetized. Here we discuss recent investigations on multitarget molecules able to release H2S, with a particular focus on the combinations of “native drug” with moieties structurally able to release H2S and their applications as therapeutic tools in bone disease, gastrointestinal system and neurodegenerative disorders.
Introduction
Nitric oxide (NO), carbon monoxide (CO) and more recently hydrogen sulfide (H2S) have emerged as “gasotransmitters” with central physiological and pharmacological effects. In the past three decades, NO has been widely investigated for its role in controlling blood circulation and regulating activities of the brain, lungs, liver, kidneys, stomach and other organs (Marsh and Marsh, 2000). More recently, H2S, another “toxic gas,” also appeared as important regulatory mediator (Moore et al., 2003).
H2S and NO exhibit many common traits like the ability to cross the biological membranes and to penetrate cells without the need of specific membrane receptors. Moreover, these molecules have been involved in the induction of hippocampal long-term potentiation, thus suggesting a key role as neuromodulators in the brain (Qu et al., 2008). Additionally, both mediators are well-known for their capability to regulate the blood pressure both in vitro and in vivo (Ali et al., 2006).
H2S plays the same multiple protective roles in the vascular system exhibited by NO but, in contrast to this latter, H2S is not associated with the harmful production of reactive oxygen species (ROS) (Whiteman et al., 2004). Probably H2S protects against cellular damage through an up-regulation of the nuclear-factor-E2-related factor-2 (Nrf2)-dependent signaling pathway (Calvert et al., 2009, Figure 1).
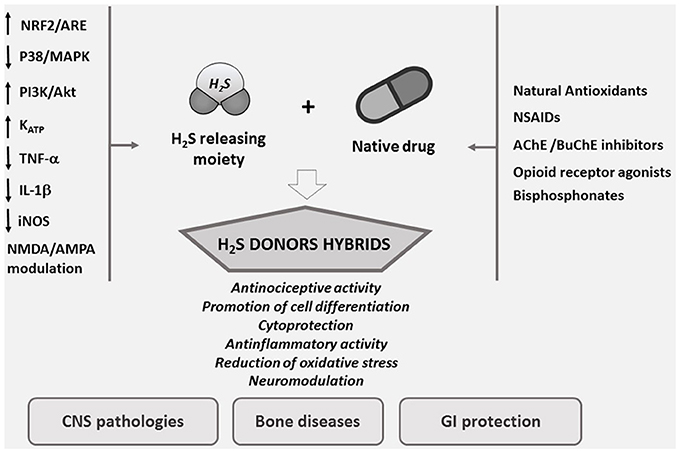
Figure 1. Designing smart agents through the combination of H2S pharmacological properties with old drugs.
In mammals, enzymatic H2S biosynthesis originates primarily from cysteine and homocysteine metabolism due to cystathionine-γ-lyase (CSE), cystathionine-β-synthase (CBS), and 3-mercaptopyruvate sulfurtransferase (3-MST)/cysteine aminotransferase (CAT) (Kimura, 2010). The expression of these enzymes is tissue-specific, with CBS being found predominantly in the brain and nervous system, while CSE is mainly expressed in the liver and in vascular/non-vascular smooth muscle. Conversely, 3MST is ubiquitously localized in many systems such as liver, kidney, heart, lung, thymus, testis, thoracic aorta and brain (Shibuya et al., 2009a,b).
The rapidly expanding literature related to H2S shows that this gasotransmitter triggers cardiovascular protection (Sun et al., 2017b; Wallace et al., 2017), possesses antitumor activity (Ianaro et al., 2016), regulates ion channels function (Naik et al., 2016; Lu et al., 2017), and displays antioxidants effects (Egea et al., 2015). Given these physiological roles, H2S appears to act as a mediator in several functions, thus representing a potential therapeutic agent as well as NO and CO.
Hydrogen Sulfide and Effects on Bone
Recent studies on physiological and pathophysiological roles of H2S, revealed its therapeutic potential in bone diseases (Liu et al., 2014; Zhai et al., 2017).
Osteoporosis is an endemic bone disease of the Western society characterized by an imbalance between bone resorption and bone formation. Physiologically, osteoblasts are responsible for bone formation and osteoclasts are associated with bone degradation. Although the exact mechanism linking H2S to bone formation is still not clarified, it turned out that this gasotransmitter inhibits the differentiation of osteoclasts through a mechanism involving antioxidant response without affecting cell viability (Gambari et al., 2014). Indeed, the study of Xu et al. showed that H2S protects osteoblastic MC3T3-E1 cells against oxidative stress (OS) via inhibition of mitogen-activated protein kinase (MAPK) signaling (Xu et al., 2011). Interestingly, H2S-donors such as GYY4137, stimulates osteogenic differentiation of human mesenchymal stromal cells (h-MSCs) both in vitro (Grassi et al., 2016) and in vivo (Liu et al., 2014). Consequently, the dual activity profile of H2S inspired the development of new molecules based on the multitarget-directed ligands (MTDLs) approach as alternative strategy to design new potential treatments for bone diseases.
The traditional Chinese medicine Danshensu, also known as DSS (3-(3,4-dihydroxyphenyl) lactic acid), is a natural phenolic acid isolated from Salvia miltiorrhiza root, recognized for its ability to reduce inflammation and suppress ROS formation (Lu et al., 2014; Jiang et al., 2015). In a more recent study, it turned out that DSS protects bone from glucocorticoids-induced bone marrow impairment through the stimulation of osteogenesis; moreover, it depresses adipogenesis in bone marrow stromal cells both in vivo and in vitro (Liao et al., 2009). These observations prompted Bian to develop a multifunctional molecule starting from acetyl-DSS and 5-(4-hydroxyphenyl)-3H-1,2-dithiole-3-thione (ADT-OH), a well characterized molecule known to release H2S (Yan et al., 2017). It has been demonstrated that the newly synthesized hybrid, SDSS (α-3, 4-tris (acetyloxy) benzenepropanoic acid 4-(3-thioxo-3H-1,2-dithiol-5-yl)phenyl ester) (Table 1), after injection into animals, is quickly deacetylated or de-esterified generating ADT-OH, an intermediate capable to induce H2S-release both in vivo and in vitro. The H2S release from SDSS has been confirmed through a fluorescent probe in MC3T3-E1 cells. The new compound also prevents the reduction of the alkaline phosphatase activity, the loss of collagen expression, and the inhibition of bone nodule formation in osteoblasts treated with H2O2. Moreover, SDSS seems to suppress OS and improve mitochondrial function in MC3T3-E1 cells, as well as to inhibit MAPKs and activate the phosphatidylinositol 3-kinase/Akt pathway (Yan et al., 2017). These results suggest that SDSS may protect osteoblasts from OS-induced cell injury and stimulate cell differentiation.
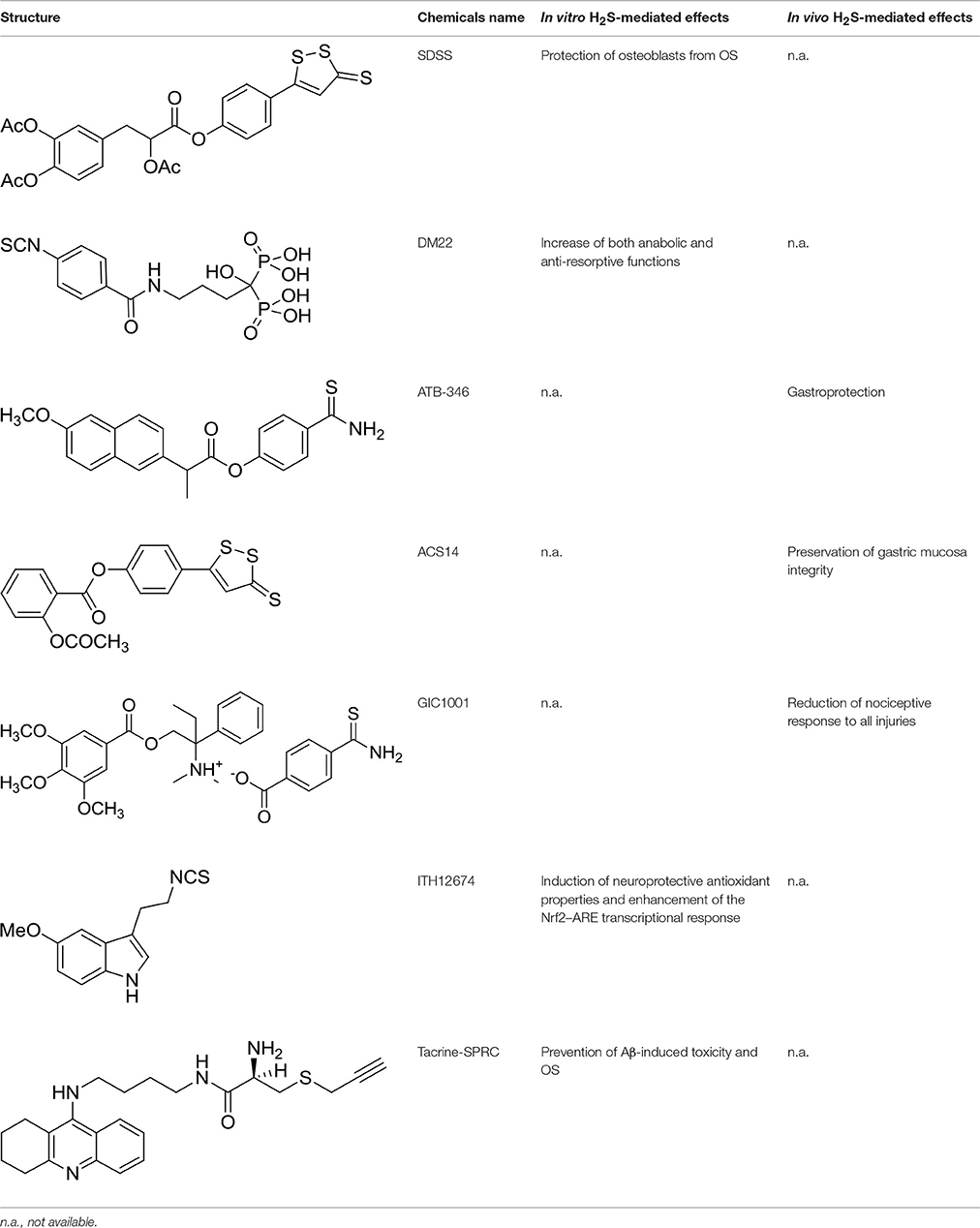
Table 1. Structure of MTDL compounds and their in vitro and in vivo H2S-mediated biological effects.
Nowadays, bisphosphonates (BPs) still represent the first-line and the most prescribed drugs in the treatment of osteoporosis and in the management of metastatic bone disease. BPs have a relatively good safety profile and are tolerated by the majority of patients even though they show several adverse effects among which: gastrointestinal injury, osteonecrosis of the jaws (ONJ) and atypical subtrochanteric femoral fractures (Conte and Guarneri, 2004; Ruggiero, 2011; Kharazmi et al., 2014).
In order to overcome the side effects of BPs, Rapposelli et al. have recently synthesized an innovative molecule called DM-22 (Table 1), obtained by the combination of alendronate (AL) with aryl-isothiocyanate moiety as H2S-releasing group (Lisignoli et al., 2016). Amperometric measurements of H2S generation from DM-22 showed that the hybrid compound is able to release H2S in the presence of organic thiols, such as L-cysteine, with a long-lasting kinetic. Comparing DM-22 with the progenitor molecule (i.e., AL), a substantially improved profile was observed in terms of safety and efficacy on human mesenchymal cell lines (h-MSCs) induced to osteogenic differentiation. Contrary to AL, this new molecule is also able to induce a nearly 3-fold increasing in the mRNA expression of Collagen I and Bone Sialoprotein (BSP) compared to control MSC, thus leading to bone mineralization. This multitarget compound showed, in bone cells in vitro, an increase of both anabolic and anti-resorptive functions compared to the parent drug. In the light of these findings, DM-22 could represent a prototype of a novel family of hybrid molecules useful in treating bone loss.
Hydrogen Sulfide and Effects on Gastrointestinal System
H2S is closely linked with the gastrointestinal (GI) system and it is also implicated in its regulation (Bala et al., 2014; Brzozowski et al., 2017; Souza et al., 2017). Beside the host production, H2S can be produced exogenously during the digestive processes or by microbes. Its pro- and anti-inflammatory, smooth muscle relaxant, pro-secretory, and pro- and anti-nociceptive actions are well documented in literature, even though its activity is not clarified as a whole (Linden, 2014).
H2S is involved in the cytoprotection of gastric mucosa; gastric mucosa is constantly exposed to exogenous substances, some of which, such as ethanol, nicotine and non-steroidal anti-inflammatory drugs (NSAIDs), can affect its integrity. Therefore, the mucosa possesses various protective mechanisms including the release of gaseous mediators such as NO and H2S, generally recognized to be implicated in the maintenance of gastrointestinal integrity and in the mechanism of gastroduodenal protection (Magierowski et al., 2015).
In this regard, GI ulceration and bleeding associated with the use of conventional NSAIDs still represent the major issues to overcome. Considerable efforts have been done in the recent past to develop innovative anti-inflammatory and analgesic drugs with minor side effects.
ATB-346 [2-(6-methoxy-napthalen-2-yl)-propionic acid 4-thiocarbamoyl-phenyl ester] (Table 1) is an H2S-releasing derivative of naproxen (Wallace et al., 2010). Naproxen is one of the most used NSAIDs, since some evidence suggested that its use might be associated with fewer cardiovascular side effects than selective COX-2 inhibitors and other NSAIDs. The molecule contains a 4-hydroxyphenyl-thioamide (Martelli et al., 2013), an H2S-releasing moiety used for the first time with this purpose. Activity of ATB-346 was evaluated in healthy animals, in several models characterized by impaired mucosal defense and in a model of gastric ulcer healing. In terms of gastric injury, ATB-346 showed to be approximately 100-fold safer than naproxen in healthy animals, exerting also comparable or superior effects respect to those of naproxen in two models of inflammation. Additionally, in opposition to selective COX-2 inhibitors, ATB-346 did not produce significant gastric damage in rats with compromised mucosal defense; it also enhances healing of pre-existing gastric ulcers. Importantly, ATB-346 exhibited a better cardiovascular profile than conventional NSAIDs.
Similarly, ACS14 [2-acetyloxybenzoic acid 4-(3-thioxo-3H-1, 2-dithiol-5-yl) phenyl ester] (Table 1) is a H2S releasing compound derived from the conjunction of acetylsalicylic acid with ADT-OH (Liu et al., 2012). ACS14 proved to be an effective and safe molecule, with significant advantages if compared to the native drug. Kinetic and metabolic experiments have shown that, after in vivo administration, ACS14 is transformed mostly by generating salicylic acid and ADTOH, the H2S-donating moiety. In addition to maintaining the thromboxane-suppressing activity, the aspirin-H2S releasing hybrid preserves the gastric mucosa integrity through the increase of H2S/GSH formation, thus affecting the redox imbalance processes (Sparatore et al., 2009). Additionally, a recent study revealed that ACS14 is also able to protect gastric mucosa against the aspirin induced damage through the inhibition of OS and a stimulation of local blood flow, maybe due to the involvement of KATP channels (Sun et al., 2017a).
As regards the smooth muscle physiology in GI system, H2S demostrated beneficial effects since H2S is able to inhibit muscle contraction, via the inhibition of Rho kinase and PKC activities and stimulation of MLCP activity, which lead to dephosphorylation of the 20-kDa regulatory light chain of myosin II (MLC20) (Nalli et al., 2015).
Different studies investigated the effect of H2S on nociception in GI. Even though endogenous H2S produced by CBS seems to contribute to visceral hypersensitivity in rats (Xu et al., 2009), exogenous H2S is able to decrease colorectal distension-induced nociception via activation of ATP-dependent K+ (KATP) channels. Anti-nociceptive effects of H2S seem to involve also an AKT-dependent transactivation and internalization of μ-opioid receptors (Distrutti et al., 2010).
These results led to the hypothesis that the combination of an opioid receptor agonist (such as trimebutine) with an H2S-releasing moiety could theoretically provide an additive or even synergistic analgesic effect. Therefore GIC-1001 (Table 1), a new and improved trimebutine derivative able to release H2S in vivo, has been developed: this drug is a salt composed of trimebutine functionalized with the H2S-releasing counter ion 3-thiocarbamoylbenzoate (3TCB) (Cenac et al., 2015). GIC-1001 has been tested in mouse model of colorectal distension. It showed to significantly reduce, in a dose-dependent manner, nociceptive response to all injuries; this effect is considerably improved if compared to equimolar administration of its parent trimebutine maleate salt. Taking into account the better efficacy in animal model and the safety for its use in humans (phase I study completed; ClinicalTrials.gov Identifier: NCT01738425), GIC-1001 was subjected to phase II study, demonstrating a clinically significant pain reduction (ClinicalTrials.gov Identifier: NCT01926444).
Summarizing, the H2S release evoked by GIC-1001 potentiates the μ-opioid receptor agonistic activity of trimebutine in mouse model. Therefore, the orally administered drug salt GIC-1001 represents an alternative to i.v. sedation during full colonoscopy. Moreover, GIC-1001 could also be potentially useful as a new treatment for the irritable bowel syndrome (IBS) associated to the visceral hypersensitivity.
To date, further studies are still required to shed light on how better exploit H2S polyhedral activity at the GI level.
Hydrogen Sulfide and Effects Induced on CNS
A rapid increase in the knowledge on H2S biological functions suggests that defects in H2S metabolism may be involved in CNS diseases (Ji et al., 2017; Shefa and Yeo, 2017). These amount of data prompted many researchers to deeply investigate the pharmacological effects of this gasotransmitter as neuromodulator, neuroprotective and anti-inflammatory agent.
The primary physiological source of H2S in the brain is CBS, a cytoplasm Pyridoxal-5′-phosphate (PLP)-dependent enzyme (Abe and Kimura, 1996). Noteworthy, in the brain of Alzheimer's disease (AD) patients has been observed a dramatic decrease of CBS activity and a consequent severe reduction in H2S levels (about 55%) (Eto et al., 2002a). Likewise, endogenous H2S production was found to decrease during the development of Parkinson disease (PD) (Kida et al., 2011). In CNS, H2S, at physiological concentrations, acts mainly as neuromodulator (Kimura, 2002; Zhang and Bian, 2014) via the involvement of at least two classes of ionotropic glutamate receptors, N-methyl-D-aspartate (NMDA) and α-amino-3-hydroxy-5-methyl-4-isoxazolepropionic acid (AMPA) receptors, which play critical roles in synaptic plasticity (Eto et al., 2002b; Kimura, 2002). Even if the mechanism of action need to be further elucidated, H2S seems to acts both directly on NMDA receptor (via sulfhydrating cysteine residues) (Kimura, 2013) and indirectly, through the regulation of intracellular Ca+2 levels (Nagai et al., 2004).
Further studies showed that H2S provides protection to neurons against OS in both extracellular and intracellular microenvironments. H2S protects the brain through the enhancement of γ-glutamylcysteine synthetase (γ-GCS) activity and cystine transport, which lead to an increase of intracellular glutathione (GSH) levels (Kimura and Kimura, 2004). Another mechanism involved in cytoprotection is the stabilization of membrane potentials. Kimura et al. demonstrated that KATP and Cl− (CFTR) channels are activated by H2S as protective mechanism from OS in an immortalized mouse hippocampal cell line (HT22) (Kimura et al., 2006).
H2S has also been found to exert both pro- and anti-inflammatory effects. It is now clear that microglia and astrocytes contribute to neuroinflammatory processes through the production of ROS and pro-inflammatory mediators. Notwithstanding, Lee et al. showed that, in presence of H2S, this process could be inhibited. It seems that H2S narrows the release of pro-inflammatory factors, including tumor necrosis factor-alpha (TNF-α), IL-1β, and nitric oxide (NO), and, at the same time, up-regulates the production of anti-inflammatory cytokines such as IL-4 and IL-10 (Lee et al., 2010; Huang et al., 2016).
Additionally, H2S may regulate neuroinflammation through the inhibition of LPS-stimulated inducible NO synthase (iNOS) and p38 mitogen-activated protein kinase (p38-MAPK) signaling pathways (Hu et al., 2007; Liu et al., 2015).
As a whole, these findings corroborate the functional involvement of H2S in neurodegenerative diseases (He et al., 2016; Yuan et al., 2017). Therefore, the restoration of correct levels of endogenous H2S is an appealing challenge for the development of new potential therapies for CNS disorders. Although the advantages of multi-target strategy are clear, the discovery of new multi-target drugs endowed of H2S-releasing properties is still in its infancy, at least in the field of neurodegenerative diseases.
A recent paper of Egea et al. (2015) described the first multitarget compound provided of neuroprotective and antioxidant properties. The molecule, named ITH12674 (Table 1), was synthesized through the combination of two pharmacophore moieties belonging to sulforaphane and melatonin. Sulforaphane is part of many members of the Brassicaceae family, such as cabbages and broccoli, and it showed a significant neuroprotective profile in OS models of neurodegenerative diseases such as AD, PD, and inflammation (Innamorato et al., 2008). The neuroprotective effect of sulforaphane, which bears an isothiocyanate function, is due to an Nrf2-mediated antioxidant response (Tebay et al., 2015). Melatonin is an endogenous whose levels decreases with aging. The neuroprotection elicited by melatonin is mainly related to its potent antioxidant and scavenger activity (Reiter et al., 2009). Thus, ITH12674 was obtained by the replacement of the amine-group of melatonin with the isothiocyanate of sulforaphane. In vitro assays showed that ITH12674 elicited better neuroprotective effects when compared to parent drugs (Egea et al., 2015).
The main drawback in the administration of gaseous H2S is the difficulty to ensure an accurate dosage thus avoiding the risk of overdose (with dramatic consequences due to H2S toxicity). Therefore, the development of “sulfide-precursors” or “prodrugs” able to produce H2S as a result of endogenous metabolism, is currently being the most investigated strategy. Actually, medicinal chemistry is exploring new natural (Citi et al., 2014) and synthetic (Martelli et al., 2013; Zheng et al., 2016; Barresi et al., 2017; Zhao et al., 2017) H2S-releasing agents as worthwhile tools for the development of new MTDL with H2S-related pharmacological properties.
As well as Brassicaceae, Allium sativum is another natural source of organic sulfur-containing compounds. In particular, S-Allylcysteine (SAC), one of the major water-soluble transformation product from garlic, turned out to be active in preventing the damage associated with OS (Rojas et al., 2011) and cancer (Nicastro et al., 2015). Moreover, it elicited cardioprotective effects in a rat model of acute myocardial infarction (Chuah et al., 2007). However, it still remains uncertain whether SAC acts as a H2S precursor or as a pharmacological activator of H2S-synthesized enzymes (Guo et al., 2013).
Recently, a structural analog of SAC, S-propargyl-cysteine (SPRC) (ZYZ-802), has been identified as a new sulfur-containing amino acid (Wang et al., 2009). SPRC represents a new H2S-donor agent able to reduce deleterious effects of OS since it showed to be able to prevent the decrease of H2S levels in rat hippocampus subjected to lipopolysaccharide (LPS) insult and inhibit TNF-α, TNF-α receptor 1 (TNFR1), and Aβ generation (Gong et al., 2011).
Santos et al. explored a set of natural-based hybrids obtained by the conjunction of SAC or SPRC moiety with tacrine, the first cholinesterase inhibitor approved for the treatment of AD. Among the new series of compounds synthesized, Tacrine-SPRC (Table 1) showed to prevent Aβ-induced toxicity and H2O2-induced OS in SH-SY5Y cells (Keri et al., 2016). Even though Tacrine-SPRC was synthesized aiming to develop a hybrid molecule between a native drug and a natural antioxidant compound, SPRC could be classified as a H2S-releasing agent, thus leading us to consider Tacrine-SPRC as promising scaffold for the development of new H2S-releasing/cholinesterase inhibitor drugs for AD therapy.
Conclusions
Latest investigations focused on pleiotropic activity of endogenous H2S led to recognize it as a key mediator implicated in many physiological aspects in human body.
The multiple effects induced by H2S resulted from the wide variety of targets involved. Despite the efforts, the mechanisms of action that should clarify the pharmacological effects are still not completely unrevealed. Nevertheless, many attempts in exploiting the multifaceted profile of this molecule have already been accomplished by medicinal chemists and several chemical entities containing an H2S-releasing moiety have been synthetized.
Herein, we focused on recent investigations on multitarget molecules able to release H2S. A drug that potentially “hits” more that one targets offers the possibility to increase the efficacy, limiting at the same the potential drawbacks generally arising from monotherapy with a single-target drug or a combination regimen of multiple drugs. Even if a new (H2S-releasing) hybrid could show improved PD and PK properties than “native drug,” the main drawback for this kind of combination is to foresee a “tissue-specific” H2S-release and thus avoiding systemic effects. The strategy discussed here revealed that the right combination of a “native drug” with moieties structurally able to release H2S could improve the toxicity profile (i.e., alendronate) or ameliorate the pharmacological effects of “old” drugs (i.e., tacrine-SPRC).
Even though this mediator has been widely investigated in the cardiovascular field, recent efforts in the search of new chemical structures to control H2S release are paving the way to the exploitation of this pleiotropic gasotrasmitter in other therapeutic fields, such as bone diseases and neurodegenerative disorders.
Author Contributions
SS and GN contributed equally. SS, GN, and SR wrote the manuscript. RP and MM revised the content of the work. SR conceived the idea. All authors agree to be accountable for the content of the work.
Conflict of Interest Statement
SR is the inventor of one patent discussed in this work.
The other authors declare that the research was conducted in the absence of any commercial or financial relationships that could be construed as a potential conflict of interest.
Acknowledgments
We would like to thank the International Society of Drug Discovery (ISDD srl, Milan, Italy) to fund fellowship to SS. The authors also thank the COST action CA15135 (Multitarget Paradigm for Innovative Ligand Identification in the Drug Discovery Process MuTaLig) for support.
References
Abe, K., and Kimura, H. (1996). The possible role of hydrogen sulfide as an endogenous neuromodulator. J. Neurosci. 16, 1066–1071.
Ali, M. Y., Ping, C. Y., Mok, Y. Y., Ling, L., Whiteman, M., Bhatia, M., et al. (2006). Regulation of vascular nitric oxide in vitro and in vivo; a new role for endogenous hydrogen sulphide? Br. J. Pharmacol. 149, 625–634. doi: 10.1038/sj.bjp.0706906
Bala, V., Rajagopal, S., Kumar, D. P., Nalli, A. D., Mahavadi, S., Sanyal, A. J., et al. (2014). Release of GLP-1 and PYY in response to the activation of G protein-coupled bile acid receptor TGR5 is mediated by Epac/PLC-ε pathway and modulated by endogenous H2S. Front. Physiol. 5:420. doi: 10.3389/fphys.2014.00420
Barresi, E., Nesi, G., Citi, V., Piragine, E., Piano, I., Taliani, S., et al. (2017). Iminothioethers as hydrogen sulfide donors: from the gasotransmitter release to the vascular effects. J. Med. Chem. 60, 7512–7523. doi: 10.1021/acs.jmedchem.7b00888
Brzozowski, T., Magierowska, K., Magierowski, M., Ptak-Belowska, A., Pajdo, R., Kwiecien, S., et al. (2017). Recent advances in the gastric mucosal protection against stress-induced gastric lesions. Importance of renin-angiotensin vasoactive metabolites, gaseous mediators and appetite peptides. Curr. Pharm. Des. doi: 10.2174/1381612823666170220160222. [Epub ahead of print].
Calvert, J. W., Jha, S., Gundewar, S., Elrod, J. W., Ramachandran, A., Pattillo, C. B., et al. (2009). Hydrogen sulfide mediates cardioprotection through Nrf2 signaling. Circ. Res. 105, 365–374. doi: 10.1161/CIRCRESAHA.109.199919
Cenac, N., Castro, M., Desormeaux, C., Colin, P., Sie, M., Ranger, M., et al. (2015). A novel orally administered trimebutine compound (GIC-1001) is anti-nociceptive and features peripheral opioid agonistic activity and hydrogen sulphide-releasing capacity in mice. Euro. J. Pain. 20, 723–730. doi: 10.1002/ejp.798
Chuah, S. C., Moore, P. K., and Zhu, Y. Z. (2007). S-allylcysteine mediates cardioprotection in an acute myocardial infarction rat model via a hydrogen sulfide-mediated pathway. Am. J. Physiol. Heart Circ. Physiol. 293, H2693–H2701. doi: 10.1152/ajpheart.00853.2007
Citi, V., Martelli, A., Testai, L., Marino, A., Breschi, M. C., and Calderone, V. (2014). Hydrogen sulfide releasing capacity of natural isothiocyanates: is it a reliable explanation for the multiple biological effects of Brassicaceae? Plant. Med. 80, 610–613. doi: 10.1055/s-0034-1368591
Conte, P., and Guarneri, V. (2004). Safety of intravenous and oral bisphosphonates and compliance with dosing regimens. Oncologist 9(Suppl. 4), 28–37. doi: 10.1634/theoncologist.9-90004-28
Distrutti, E., Cipriani, S., Renga, B., Mencarelli, A., Migliorati, M., Cianetti, S., et al. (2010). Hydrogen sulphide induces μ opioid receptor-dependent analgesia in a rodent model of visceral pain. Mol. Pain 6:36. doi: 10.1186/1744-8069-6-36
Egea, J., Buendia, I., Parada, E., Navarro, E., Rada, P., Cuadrado, A., et al. (2015). Melatonin–sulforaphane hybrid ITH12674 induces neuroprotection in oxidative stress conditions by a ‘drug–prodrug’ mechanism of action. Br. J. Pharmacol. 172, 1807–1821. doi: 10.1111/bph.13025
Eto, K., Asada, T., Arima, K., Makifuchi, T., and Kimura, H. (2002a). Brain hydrogen sulfide is severely decreased in Alzheimer's disease. Biochem. Biophys. Res. Commun. 293, 1485–1488. doi: 10.1016/S0006-291X(02)00422-9
Eto, K., Ogasawara, M., Umemura, K., Nagai, Y., and Kimura, H. (2002b). Hydrogen sulfide is produced in response to neuronal excitation. J. Neurosci. 22, 3386–3391.
Gambari, L., Lisignoli, G., Cattini, L., Manferdini, C., Facchini, A., and Grassi, F. (2014). Sodium hydrosulfide inhibits the differentiation of osteoclast progenitor cells via NRF2-dependent mechanism. Pharmacol. Res. 87, 99–112. doi: 10.1016/j.phrs.2014.06.014
Gong, Q.-H., Wang, Q., Pan, L.-L., Liu, X.-H., Xin, H., and Zhu, Y.-Z. (2011). S-propargyl-cysteine, a novel hydrogen sulfide-modulated agent, attenuates lipopolysaccharide-induced spatial learning and memory impairment: involvement of TNF signaling and NF-κB pathway in rats. Brain Behav. Immun. 25, 110–119. doi: 10.1016/j.bbi.2010.09.001
Grassi, F., Tyagi, A. M., Calvert, J. W., Gambari, L., Walker, L. D., Yu, M., et al. (2016). Hydrogen sulfide is a novel regulator of bone formation implicated in the bone loss induced by estrogen deficiency. J. Bone Miner. Res. 31, 949–963. doi: 10.1002/jbmr.2757
Guo, W., Cheng, Z.-Y., and Zhu, Y.-Z. (2013). Hydrogen sulfide and translational medicine. Acta Pharmacol. Sin. 34, 1284–1291. doi: 10.1038/aps.2013.127
He, X. L., Yan, N., Chen, X. S., Qi, Y. W., Yan, Y., and Cai, Z. (2016). Hydrogen sulfide down-regulates BACE1 and PS1 via activating PI3K/Akt pathway in the brain of APP/PS1 transgenic mouse. Pharmacol. Rep. 68, 975–982. doi: 10.1016/j.pharep.2016.05.006
Hu, L. F., Wong, P. T. H., Moore, P. K., and Bian, J. S. (2007). Hydrogen sulfide attenuates lipopolysaccharide-induced inflammation by inhibition of p38 mitogen-activated protein kinase in microglia. J. Neurochem. 100, 1121–1128. doi: 10.1111/j.1471-4159.2006.04283.x
Huang, C. W., Feng, W., Peh, M. T., Peh, K., Dymock, B. W., and Moore, P. K. (2016). A novel slow-releasing hydrogen sulfide donor, FW1256, exerts anti-inflammatory effects in mouse macrophages and in vivo. Pharmacol. Res. 113(Pt A), 533–546. doi: 10.1016/j.phrs.2016.09.032
Ianaro, A., Cirino, G., and Wallace, J. L. (2016). Hydrogen sulfide-releasing anti-inflammatory drugs for chemoprevention and treatment of cancer. Pharmacol. Res. 111, 652–658. doi: 10.1016/j.phrs.2016.07.041
Innamorato, N. G., Rojo, A. I., Garcia-Yague, A. J., Yamamoto, M., de Ceballos, M. L., and Cuadrado, A. (2008). The transcription factor Nrf2 is a therapeutic target against brain inflammation. J. Immunol. 181, 680–689. doi: 10.4049/jimmunol.181.1.680
Ji, J., Xiang, P., Li, T., Lan, L., Xu, X., Lu, G., et al. (2017). NOSH-NBP, a novel nitric oxide and hydrogen sulfide- releasing hybrid, attenuates ischemic stroke-induced neuroinflammatory injury by modulating microglia polarization. Front. Cell. Neurosci. 11:154. doi: 10.3389/fncel.2017.00154
Jiang, X., Lv, B., Li, P., Ma, X., Wang, T., Zhou, Q., et al. (2015). Bioactivity-integrated UPLC/Q-TOF–MS of Danhong injection to identify NF-κB inhibitors and anti-inflammatory targets based on endothelial cell culture and network pharmacology. J. Ethnopharmacol. 174, 270–276. doi: 10.1016/j.jep.2015.08.026
Keri, R. S., Quintanova, C., Chaves, S., Silva, D. F., Cardoso, S. M., and Santos, M. A. (2016). New tacrine hybrids with natural-based cysteine derivatives as multitargeted drugs for potential treatment of Alzheimer's disease. Chem. Biol. Drug Des. 87, 101–111. doi: 10.1111/cbdd.12633
Kharazmi, M., Hallberg, P., Warfvinge, G., and Michaëlsson, K. (2014). Risk of atypical femoral fractures and osteonecrosis of the jaw associated with alendronate use compared with other oral bisphosphonates. Rheumatology 53, 1911–1913. doi: 10.1093/rheumatology/keu286
Kida, K., Yamada, M., Tokuda, K., Marutani, E., Kakinohana, M., Kaneki, M., et al. (2011). Inhaled hydrogen sulfide prevents neurodegeneration and movement disorder in a mouse model of Parkinson's disease. Antioxid. Redox Signal. 15, 343–352. doi: 10.1089/ars.2010.3671
Kimura, H. (2002). Hydrogen sulfide as a neuromodulator. Mol. Neurobiol. 26, 13–19. doi: 10.1385/MN:26:1:013
Kimura, H. (2010). Hydrogen sulfide: from brain to gut. Antioxid. Redox Signal. 12, 1111–1123. doi: 10.1089/ars.2009.2919
Kimura, H. (2013). Physiological role of hydrogen sulfide and polysulfide in the central nervous system. Neurochem. Int. 63, 492–497. doi: 10.1016/j.neuint.2013.09.003
Kimura, Y., Dargusch, R., Schubert, D., and Kimura, H. (2006). Hydrogen sulfide protects HT22 neuronal cells from oxidative stress. Antioxid. Redox Signal. 8, 661–670. doi: 10.1089/ars.2006.8.661
Kimura, Y., and Kimura, H. (2004). Hydrogen sulfide protects neurons from oxidative stress. FASEB J. 18, 1165–1167. doi: 10.1096/fj.04-1815fje
Lee, M., Sparatore, A., Del Soldato, P., Mcgeer, E., and McGeer, P. L. (2010). Hydrogen sulfide-releasing NSAIDs attenuate neuroinflammation induced by microglial and astrocytic activation. Glia 58, 103–113. doi: 10.1002/glia.20905
Liao, C., Liu, Y. -Y., Tie, W., Ai, C.-M., and Chen, H.-Q. (2009). Osteogenic effects of D(+) β-3, 4-dihydroxyphenyl lactic acid (salvianic acid A, SAA) on osteoblasts and bone marrow stromal cells of intact and prednisone-treated rats. Acta Pharmacol. Sin. 30, 321–332. doi: 10.1038/aps.2009.9
Linden, D. R. (2014). Hydrogen sulfide signaling in the gastrointestinal tract. Antioxid. Redox Signal. 20, 818–830. doi: 10.1089/ars.2013.5312
Lisignoli, G., Grassi, F., Calderone, V., and Rapposelli, S. (2016). New Molecules for Bone Tissue Regeneration. Google Patents. EP20150808792.
Liu, H., Deng, Y., Gao, J., Liu, Y., Li, W., Shi, J., et al. (2015). Sodium hydrosulfide attenuates beta-amyloid-induced cognitive deficits and neuroinflammation via modulation of MAPK/NF-kappaB pathway in rats. Curr. Alzheimer Res. 12, 673–683. doi: 10.2174/1567205012666150713102326
Liu, L., Cui, J., Song, C.-J., Bian, J.-S., Sparatore, A., Del Soldato, P., et al. (2012). H (2) S-releasing aspirin protects against aspirin-induced gastric injury via reducing oxidative stress. PLoS ONE 7:e46301. doi: 10.1371/journal.pone.0046301
Liu, Y., Yang, R., Liu, X., Zhou, Y., Qu, C., Kikuiri, T., et al. (2014). Hydrogen sulfide maintains mesenchymal stem cell function and bone homeostasis via regulation of Ca2+ channel sulfhydration. Cell Stem Cell 15, 66–78. doi: 10.1016/j.stem.2014.03.005
Lu, G., Xu, C., Tang, K., Zhang, J., Li, Q., Peng, L., et al. (2017). H2S inhibits angiotensin II-induced atrial Kv1.5 upregulation by attenuating Nox4-mediated ROS generation during atrial fibrillation. Biochem. Biophys. Res. Commun. 483, 534–540. doi: 10.1016/j.bbrc.2016.12.110
Lu, H., Tian, A., Wu, J., Yang, C., Xing, R., Jia, P., et al. (2014). Danshensu inhibits β-adrenergic receptors-mediated cardiac fibrosis by ROS/P38 MAPK axis. Biol.Pharm. Bull. 37, 961–967. doi: 10.1248/bpb.b13-00921
Magierowski, M., Magierowska, K., Kwiecien, S., and Brzozowski, T. (2015). Gaseous mediators nitric oxide and hydrogen sulfide in the mechanism of gastrointestinal integrity, protection and ulcer healing. Molecules 20, 9099–9123. doi: 10.3390/molecules20059099
Marsh, N., and Marsh, A. (2000). A short history of nitroglycerine and nitric oxide in pharmacology and physiology. Clin. Exp. Pharmacol. Physiol. 27, 313–319. doi: 10.1046/j.1440-1681.2000.03240.x
Martelli, A., Testai, L., Citi, V., Marino, A., Pugliesi, I., Barresi, E., et al. (2013). Arylthioamides as H2S donors: l-cysteine-activated releasing properties and vascular effects in vitro and in vivo. ACS Med. Chem. Lett. 4, 904–908. doi: 10.1021/ml400239a
Moore, P. K., Bhatia, M., and Moochhala, S. (2003). Hydrogen sulfide: from the smell of the past to the mediator of the future? Trends Pharmacol. Sci. 24, 609–611. doi: 10.1016/j.tips.2003.10.007
Nagai, Y., Tsugane, M., Oka, J.-I., and Kimura, H. (2004). Hydrogen sulfide induces calcium waves in astrocytes. FASEB J. 18, 557–559. doi: 10.1096/fj.03-1052fje
Naik, J. S., Osmond, J. M., Walker, B. R., and Kanagy, N. L. (2016). Hydrogen sulfide-induced vasodilation mediated by endothelial TRPV4 channels. Am. J. Physiol. Heart Circ. Physiol. 311, H1437–H1444. doi: 10.1152/ajpheart.00465.2016
Nalli, A. D., Rajagopal, S., Mahavadi, S., Grider, J. R., and Murthy, K. S. (2015). Inhibition of RhoA-dependent pathway and contraction by endogenous hydrogen sulfide in rabbit gastric smooth muscle cells. Am. J. Physiol.Cell Physiol. 308, C485–C495. doi: 10.1152/ajpcell.00280.2014
Nicastro, H. L., Ross, S. A., and Milner, J. A. (2015). Garlic and onions: their cancer prevention properties. Cancer Prev. Res. 8, 181–189. doi: 10.1158/1940-6207.CAPR-14-0172
Qu, K., Lee, S. W., Bian, J. S., Low, C. M., and Wong, P. T. (2008). Hydrogen sulfide: neurochemistry and neurobiology. Neurochem. Int. 52, 155–165. doi: 10.1016/j.neuint.2007.05.016
Reiter, R. J., Paredes, S. D., Manchester, L. C., and Tan, D. X. (2009). Reducing oxidative/nitrosative stress: a newly-discovered genre for melatonin. Crit. Rev. Biochem. Mol. Biol. 44, 175–200. doi: 10.1080/10409230903044914
Rojas, P., Serrano-García, N., Medina-Campos, O. N., Pedraza-Chaverri, J., Maldonado, P. D., and Ruiz-Sánchez, E. (2011). S-Allylcysteine, a garlic compound, protects against oxidative stress in 1-methyl-4-phenylpyridinium-induced parkinsonism in mice. J. Nutr. Biochem. 22, 937–944. doi: 10.1016/j.jnutbio.2010.08.005
Ruggiero, S. L. (2011). Bisphosphonate-related osteonecrosis of the jaw: an overview. Ann. N.Y. Acad. Sci. 1218, 38–46. doi: 10.1111/j.1749-6632.2010.05768.x
Shefa, U., and Yeo, S. G. (2017). Role of gasotransmitters in oxidative stresses, neuroinflammation, and neuronal repair. BioMed. Res. Int. 2017:1689341. doi: 10.1155/2017/1689341
Shibuya, N., Mikami, Y., Kimura, Y., Nagahara, N., and Kimura, H. (2009a). Vascular endothelium expresses 3-mercaptopyruvate sulfurtransferase and produces hydrogen sulfide. J. Biochem. 146, 623–626. doi: 10.1093/jb/mvp111
Shibuya, N., Tanaka, M., Yoshida, M., Ogasawara, Y., Togawa, T., Ishii, K., et al. (2009b). 3-Mercaptopyruvate sulfurtransferase produces hydrogen sulfide and bound sulfane sulfur in the brain. Antioxid. Redox Signal. 11, 703–714. doi: 10.1089/ARS.2008.2253
Souza, L. K., Araujo, T. S., Sousa, N. A., Sousa, F. B., Nogueira, K. M., Nicolau, L. A., et al. (2017). Evidence that d-cysteine protects mice from gastric damage via hydrogen sulfide produced by d-amino acid oxidase. Nitric Oxide 64, 1–6. doi: 10.1016/j.niox.2017.01.010
Sparatore, A., Perrino, E., Tazzari, V., Giustarini, D., Rossi, R., Rossoni, G., et al. (2009). Pharmacological profile of a novel H 2 S-releasing aspirin. Free Rad. Biol. Med. 46, 586–592. doi: 10.1016/j.freeradbiomed.2008.11.013
Sun, H. Z., Zheng, S., Lu, K., Hou, F. T., Bi, J. X., Liu, X. L., et al. (2017a). Hydrogen sulfide attenuates gastric mucosal injury induced by restraint water-immersion stress via activation of KATP channel and NF-kappaB dependent pathway. World J. Gastroenterol. 23, 87–92. doi: 10.3748/wjg.v23.i1.87
Sun, X., Wang, W., Dai, J., Jin, S., Huang, J., Guo, C., et al. (2017b). A Long-term and slow-releasing hydrogen sulfide donor protects against myocardial ischemia/reperfusion injury. Sci. Rep. 7:3541. doi: 10.1038/s41598-017-03941-0
Tebay, L. E., Robertson, H., Durant, S. T., Vitale, S. R., Penning, T. M., Dinkova-Kostova, A. T., et al. (2015). Mechanisms of activation of the transcription factor Nrf2 by redox stressors, nutrient cues, and energy status and the pathways through which it attenuates degenerative disease. Free Radic. Biol. Med. 88(Pt B), 108–146. doi: 10.1016/j.freeradbiomed.2015.06.021
Wallace, J. L., Caliendo, G., Santagada, V., and Cirino, G. (2010). Markedly reduced toxicity of a hydrogen sulphide-releasing derivative of naproxen (ATB-346). Br. J. Pharmacol. 159, 1236–1246. doi: 10.1111/j.1476-5381.2009.00611.x
Wallace, J. L., Vaughan, D., Dicay, M., MacNaughton, W. K., and de Nucci, G. (2017). Hydrogen sulfide-releasing therapeutics: translation to the clinic. Antioxid. Redox Signal. doi: 10.1089/ars.2017.7068. [Epub ahead of print].
Wang, Q., Liu, H.-R., Mu, Q., Rose, P., and Zhu, Y. Z. (2009). S-propargyl-cysteine protects both adult rat hearts and neonatal cardiomyocytes from ischemia/hypoxia injury: the contribution of the hydrogen sulfide-mediated pathway. J. Cardiovasc. Pharmacol. 54, 139–146. doi: 10.1097/FJC.0b013e3181ac8e12
Whiteman, M., Armstrong, J. S., Chu, S. H., Jia-Ling, S., Wong, B. S., Cheung, N. S., et al. (2004). The novel neuromodulator hydrogen sulfide: an endogenous peroxynitrite ‘scavenger’? J. Neurochem. 90, 765–768. doi: 10.1111/j.1471-4159.2004.02617.x
Xu, G.-Y., Winston, J. H., Shenoy, M., Zhou, S., Chen, J. D., and Pasricha, P. J. (2009). The endogenous hydrogen sulfide producing enzyme cystathionine-β synthase contributes to visceral hypersensitivity in a rat model of irritable bowel syndrome. Mol. Pain 5:44. doi: 10.1186/1744-8069-5-44
Xu, Z.-S., Wang, X.-Y., Xiao, D.-M., Hu, L.-F., Lu, M., Wu, Z.-Y., et al. (2011). Hydrogen sulfide protects MC3T3-E1 osteoblastic cells against H 2 O 2-induced oxidative damage—implications for the treatment of osteoporosis. Free Radic. Biol. Med. 50, 1314–1323. doi: 10.1016/j.freeradbiomed.2011.02.016
Yan, X., Wu, H., Wu, Z., Hua, F., Liang, D., Sun, H., et al. (2017). The new synthetic H2S-releasing SDSS protects MC3T3-E1 osteoblasts against H2O2-induced apoptosis by suppressing oxidative stress, inhibiting MAPKs, and activating the PI3K/Akt pathway. Front.pharmacol. 8:07. doi: 10.3389/fphar.2017.00007
Yuan, Y. Q., Wang, Y. L., Yuan, B. S., Yuan, X., Hou, X. O., Bian, J. S., et al. (2017). Impaired CBS-H2S signaling axis contributes to MPTP-induced neurodegeneration in a mouse model of Parkinson's disease. Brain Behav. Immun. S0889-1591(17)30388-4. doi: 10.1016/j.bbi.2017.07.159. [Epub ahead of print].
Zhai, Y., Tyagi, S. C., and Tyagi, N. (2017). Cross-talk of MicroRNA and hydrogen sulfide: a novel therapeutic approach for bone diseases. Biomed. Pharmacother. 92, 1073–1084. doi: 10.1016/j.biopha.2017.06.007
Zhang, X., and Bian, J.-S. (2014). Hydrogen sulfide: a neuromodulator and neuroprotectant in the central nervous system. ACS Chem. Neurosci. 5, 876–883. doi: 10.1021/cn500185g
Zhao, Y., Bolton, S. G., and Pluth, M. D. (2017). Light-activated COS/H2S donation from photocaged thiocarbamates. 19, 2278–2281. doi: 10.1021/acs.orglett.7b00808
Keywords: hydrogen sulfide, multitarget-directed ligands, bone disease, hybrid molecules, H2S-donors, multifunctional compounds, antioxidants, neurodegenerative disorders
Citation: Sestito S, Nesi G, Pi R, Macchia M and Rapposelli S (2017) Hydrogen Sulfide: A Worthwhile Tool in the Design of New Multitarget Drugs. Front. Chem. 5:72. doi: 10.3389/fchem.2017.00072
Received: 12 August 2017; Accepted: 15 September 2017;
Published: 27 September 2017.
Edited by:
Antonio Randazzo, University of Naples Federico II, ItalyReviewed by:
Alla B. Salmina, Krasnoyarsk State Medical University Named After Prof. V. F. Voino-Yasenetsky, RussiaSenthilkumar Rajagopal, Rayalaseema University, India
Copyright © 2017 Sestito, Nesi, Pi, Macchia and Rapposelli. This is an open-access article distributed under the terms of the Creative Commons Attribution License (CC BY). The use, distribution or reproduction in other forums is permitted, provided the original author(s) or licensor are credited and that the original publication in this journal is cited, in accordance with accepted academic practice. No use, distribution or reproduction is permitted which does not comply with these terms.
*Correspondence: Simona Rapposelli, simona.rapposelli@unipi.it