Survival and Migration of Rock Ptarmigan in Central Scandinavia
- 1Department for Terrestrial Biodiversity, Norwegian Institute for Nature Research, Trondheim, Norway
- 2Faculty of Biosciences and Aquaculture, Nord University, Steinkjer, Norway
In a world undergoing massive declines in the distribution and abundance of many wildlife species, documenting basic ecological characteristics is often needed to be able to understand and potentially mitigate current and future pressures. Species living in alpine areas might be particularly vulnerable to climate change, in part because they are less likely to be able to migrate to new suitable areas. Here we report from a two year case study of rock ptarmigan (Lagopus muta) in central Scandinavia. Ptarmigan were captured in winter (n = 84), and fitted with radio collars. We estimated the natural survival from mid-winter to late summer to be 0.55 (SE: 0.07), with no distinct differences between juveniles and adults, sex, or between the two years. Natural survival through late winter (February–April) was estimated at 0.77 (SE: 0.05), survival trough breeding season May–July at 0.65 (SE: 0.08), and harvest mortality through the February winter harvest at 9% (SE: 3%). Moreover, we documented large scale movement from the wintering grounds before the breeding season in the spring. The longest recorded movement was 79.5 km, and the mean distance from the capture site for birds still in the sample in May–July was 20.3 (SD: 18) km. We discuss the implications of the results in terms of ongoing climate change.
Introduction
Species inhabiting alpine or polar habitats are expected to be particularly affected by ongoing climate change (Post et al., 2009; Revermann et al., 2012). This is partly because these areas are likely to become much warmer in the future, but also because species inhabiting such areas have less possibilities to find new locations to thrive (Sirami et al., 2017). In general, alpine populations should be expected to move upward, whereas polar tundra species are expected to conduct latitudinal movements (Lehikoinen et al., 2019). Among the few resident birds in these habitats, the rock ptarmigan (Lagopus muta) has a wide, circumpolar distribution across the northern tundra and high-mountain regions (Storch, 2007; Fuglei et al., 2019). Rock ptarmigan as a species is expected to be strongly affected by climate change (Booms et al., 2012; Revermann et al., 2012; Hansen et al., 2013), and is therefore a suitable model species for examining climate change effects on alpine wildlife populations. Globally rock ptarmigan is considered as least concern (LC) in the International Red List of Species (BirdLife_International, 2016), but locally there are concerns about declining populations. In 2015 the species was classified as near threatened (NT) in the Norwegian Red List of Species (Henriksen and Hilmo, 2015).
Compared to other grouse species, the rock ptarmigan are among the least studied species in terms of number of scientific publications (Moss et al., 2010). Therefore, there is still a need for studies documenting the basic biology and life history in many parts of the distributional range. Rock ptarmigan ecology and demography has earlier been studied in e.g., United Kingdom (e.g., Watson et al., 1998), North-America (Wilson and Martin, 2008, 2010), Russia (e.g., Potapov and Potapov, 2012), Japan (e.g., Suzuki et al., 2013), high-alpine areas in the Italian Alps, French Alps and Pyrenees (Scherini et al., 2003; Novoa et al., 2008, 2011), Iceland (e.g., Nielsen, 1999) and the archipelago of Svalbard (e.g., Pedersen et al., 2012; Unander et al., 2016). However, from an important part of their distribution – the mountainous areas across mainland Scandinavia – only aspects relating to population genetics (e.g., Costanzi and Steifetten, 2019), habitat use (Pedersen et al., 2013) and population dynamics based on harvest bag data (Kvasnes et al., 2010) has been studied. Baseline demography and ecology, including spatial behavior, is largely unknown.
In this case study, we provide insight into some key aspects of rock ptarmigan demography and ecology in a study area in central Scandinavia (Figure 1), based on a small radio telemetry project. Although our study is completely descriptive and does not intend to test any specific hypothesis about rock ptarmigan ecology or demography, there are two specific aspects that we focus on:
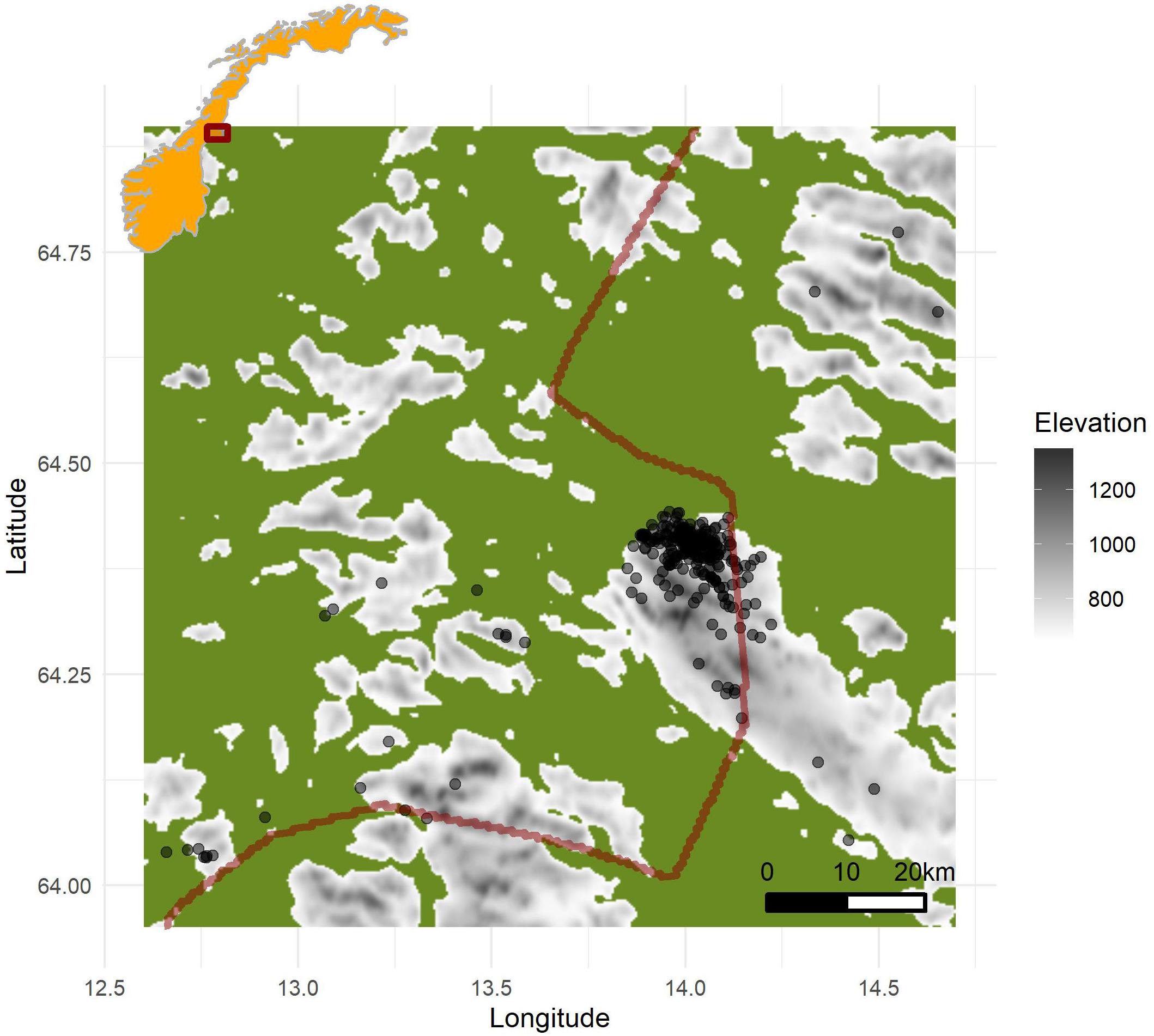
Figure 1. Map of the study area. Inset map: Norway marked in orange, and study area marked with a red rectangle. In the main map, areas below 650 masl is marked in green, and areas above 650 (corresponding roughly to habitats used by rock ptarmigan) is gray shaded by elevation. The border between Norway (west) and Sweden (east) is marked with a red line. Captures and relocations of rock ptarmigan is marked with black dots.
1. First, we use known fate models to estimate survival probabilities. We investigate to which extent survival probability differs between years, age (juvenile vs. adult) and sex (females vs. males). We estimate survival probabilities during a 6 month period from February–July, as well as for late winter (settlement period) and in the breeding period. We also report harvest mortality rates for the winter harvest season in February.
2. Second, we estimate movement rates away from the wintering grounds, using mean displacement rates as our estimator of interest. The rationale for this was to identify to which extent the birds captured at their wintering grounds were stationary or moved to other areas in the breeding season.
Materials and Methods
Study Area
The current study was conducted in central Norway, in the municipality Lierne (central location for our core study area: 64°25′N, 13°59′E), partly within the Lierne National Park (Figure 1). The study area cover both the northern boreal, low- and mid-alpine ecoregions. The lower parts is dominated by willow (Salix spp.) and scattered forests of mountain birch (Betula pubescens), the mid areas sedges, grasses, patches of dwarf birch (B. nana) and snowbed communities, while the highest parts lack continuous vegetation cover. Yearly precipitation normal is equivalent to 675 millimeter per year, while the temperature normal is –10°C for January and 12°C for July. Snow depth at the study area is 1–3 m deep during winter and snow cover persists from early October to late May. Based on field observations and previous studies, important predator species on juvenile and adult rock ptarmigan in the study area include gyrfalcon (Falco rusticolus) and golden eagle (Aquila chrysaetos), red foxes (Vulpes vulpes), arctic foxes (V. lagopus) and to a limited extent wolverine (Gulo gulo). Potential additional predators on eggs and chicks includes raven (Corvus corax) and hooded crow (C. cornix).
Field Data Collection
Rock ptarmigan were captured at night in the winter in 2012 and 2013, with handheld spotlights and long-handled dip nets from snowmobiles. Similar approach has been used in previous studies on willow ptarmigan (L. lagopus) in Norway (Sandercock et al., 2011). At capture, birds were aged as juveniles or adults and sexed, and morphometric measures [weight (g) and wing length (mm)] were taken. We sexed and aged (juvenile: born the proceeding summer, adult: all older birds) the birds based on plumage coloration, wing length, and patterns of pigmentation of the three outermost primaries (Bergerud et al., 1963). In total, we captured and radio collared 84 rock ptarmigan (n = 44 males, n = 40 females, n = 50 juveniles, n = 34 adults) across the two seasons. Note that there was a marked difference in the proportion of juveniles among captured birds in 2012 (78%) and 2013 (24%), corresponding well with a large difference in breeding success in 2011 (high) and 2012 (low) respectively (E.B. Nilsen, pers com, based on line transect data on willow ptarmigan from the study area).
Each bird was marked with a uniquely numbered leg ring, and equipped with a necklace VHF-radio transmitter (Holohil Systems Ltd., 10/15 g) with a 24 months expected battery life. The collars had mortality switches, so that we could detect when a bird was dead. Previous studies have shown that necklace radios of this size have little to no effect on the demographic parameters or movements of ptarmigan under natural conditions (see Sandercock et al., 2011 and citations therein).
Radio collared birds were tracked from the ground or air at irregular intervals. When tracking from ground (using ski or snowmobile during winter, and on foot during spring/summer), we either conducted radio-triangulation at relatively close distances (from 50 m – to a few hundred meters) to obtain precise positions, or obtained just one signal so we could determine the state of the bird based on the mortality switch. When tracking from the air, we used either a small winged aircraft or a small helicopter. Whenever we obtained a mortality signal, we tried to locate the bird on the ground to determine the cause of death. In addition, we were notified by small game hunters in the region when a marked bird was shot (hunting is allowed from August/September–February in this part of Scandinavia). Hunters cannot normally see the collars during a hunting situation, and there were no particular restrictions for hunters regarding shooting marked birds. We believe that all or most shot birds were reported, but do not have any independent data to back up this statement.
At the onset of the study, we opted to follow the birds throughout their full annual cycle, as has been done in previous studies on willow ptarmigan (Sandercock et al., 2011). However, because of the high combined loss-rates (i.e., combination of censoring and mortality), and the fact that the birds were spread across a huge area (see Results section) during summer and fall/early winter, we obtained relatively few locations in that period. We therefore here restrict our analysis to the time between February 1st and July 31st each year. In the study periods, we conducted three and four flights in the years 2012 and 2013, respectively. For each individual, we had between zero and 16 relocations. Birds that were never relocated after marking (n = 2) were not included in the analysis.
All data used in this study is published and openly accessible through GBIF, located here:1 (Nilsen et al., 2017). The animal study was reviewed and approved by the Norwegian Food Safety Authority (Application ID 3960).
Statistical Analysis
Based on the data described above, we examined the two aspects of rock ptarmigan ecology and demography as outlined above. To examine survival during late winter, spring and summer (February 1st–July 31st), we first determined the entry – and exit time for each bird into the sample, at a weekly basis for each of the two years of the study period. Entry point was determined as either (i) the week of capture, or (ii) first week of February for birds that survived the first year and entered into their second study year. Exit from the sample were again determined on a weekly basis, and were coded as either mortality or censored. Censored birds were either (i) those that we lost contact with, or (ii) those that survived until the end of the annual study period. Because we did not have continuous follow-up times, we adjusted our data to a monthly schedule. For birds of which mortality was recorded, exit was defined to occur in the mid-point between the last live contact and the first mortality signal. For birds that were censored because we lost contact, we censored the birds the first month after the last detection. We used Kaplan–Meyer models (Pollock et al., 1989; Murray, 2006) to estimate survival probabilities, the non-parametric cumulative incidence function (NPCIFE) to estimate cause-specific mortality (Heisey and Patterson, 2006), and cox-proportional hazard models (Murray, 2006; Murray and Patterson, 2006) to test for differences among years (2012 vs. 2013), age (juveniles vs. adults) and sex (males vs. females). We could not consider interactions between independent variables, because of relatively low sample sizes. We used Akaike’s Information Criterion corrected for small sample sizes (AICc) to guide the model selection procedures (Burnham and Anderson, 2002). All analysis were conducted in program R version 3.6.0 (R Development Core Team, 2019), and survival analysis were conducted using add-on library survival (Therneau, 2015).
The R-code for performing analysis are available as a registered archive at Open Science Framework, through an add-on connection with GitHub (Nilsen, 2020)2.
Results
Out of 84 radio marked rock ptarmigan, 9 were shot by hunters in the study area, and 33 were recorded as dead due to other causes (mostly predation). The birds were marked in February and March, and we estimated the harvest mortality during the four weeks of February to be 0.09 (SE: 0.03).
Based on cox proportional hazard models, we did not find any clear evidence that survival differed between years, or between age- and sex categories (Table 1). Moreover, we did not detect any effects of weight (at capture) on the mortality risk, whether we controlled for potential confounding effects of age- and sex or not (Table 1). For all models, the proportional hazards assumption was met (year-model: chi.sq = 0.5, p = 0.48; sex-model: chi.sq = 0.02, p = 0.9; age-model: chi.sq = 0.93, p = 0.33: weight-model: chi.sq = 0.94, p = 0.33; weight-sex model: global p = 0.58; weight-age model: global p = 0.58). Based on the pooled sample, overall probability to survive from February 1st and through July was estimated at 0.45 (SE: 0.07) (Figure 2), with natural survival (i.e., disregarding birds that were shot by hunters during the harvest season) estimated at 0.55 (SE: 0.07). Survival probability for late winter to start of the breeding season (February 1st–April 30th) was estimated at 0.7 (SE: 0.05), with natural survival estimated at 0.77 (SE: 0.05). Survival probability for the breeding season (May 1st–July 31st) was estimated at 0.65 (SE: 0.08).
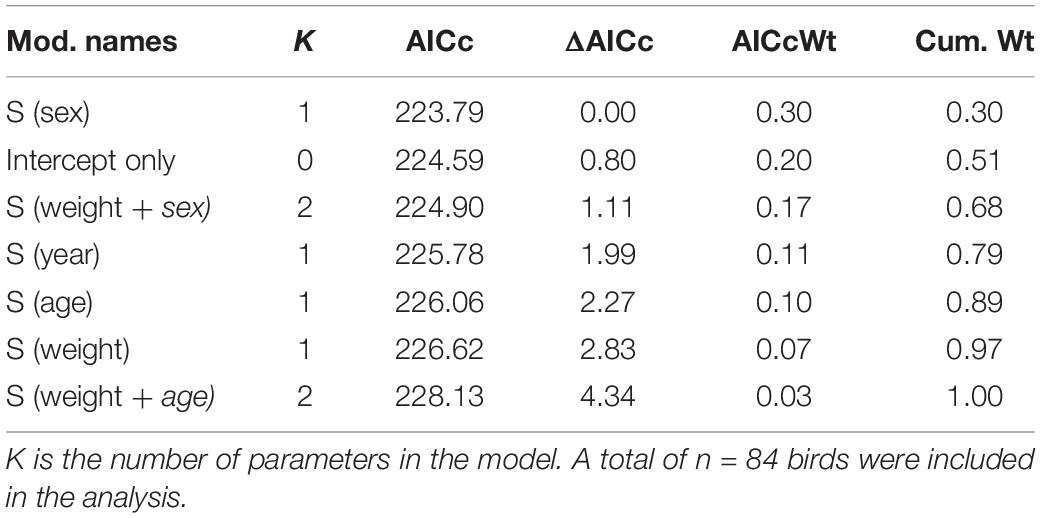
Table 1. Results from cox-proportional hazard models used to test for differences in survival between sex- and age-classes, as well as between years.
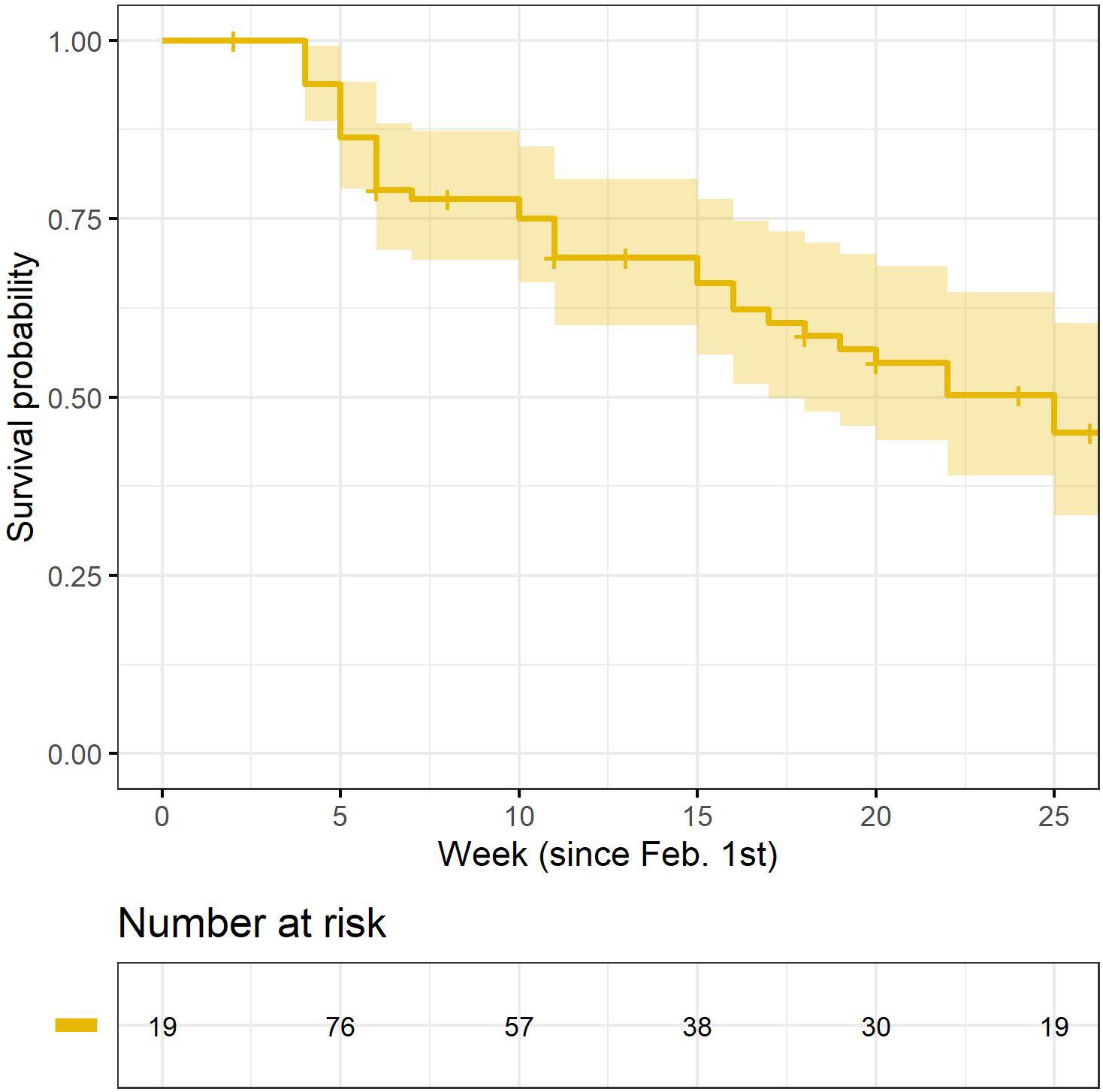
Figure 2. Kaplan–Meyer survival curve for a sample of rock ptarmigan radio collared in Central Norway in 2012–2013. The time on the x-axis is shifted so that week 1 represent the 1st week in February each year, whereas week 26 is the last week in July. Lower table depicts the number at risk (i.e., time-specific sample sizes) throughout the study period.
Most of the birds captured and marked with radio collars left the area where they had been captured before the breeding season started in the spring (Figure 3). Based on the maximum displacement for birds still alive and in the sample (n = 36), mean displacement distance was estimated at 7.8 (SD: 12.2) km in April. In a pooled sample for May–July, the displacement for birds still alive and in the sample (n = 25) was estimated at 20.3 (SD: 18) km. We note that this is probably an underestimation, because we are more likely to have lost contact with birds that moved long distances. The maximum recorded movement from the capture site was 79.5 km. A total of 6 birds moved longer than 50 km, of which four were males and two were females. For 16 birds, we detected movements longer than 25 km from the capture site, including seven males and nine females.
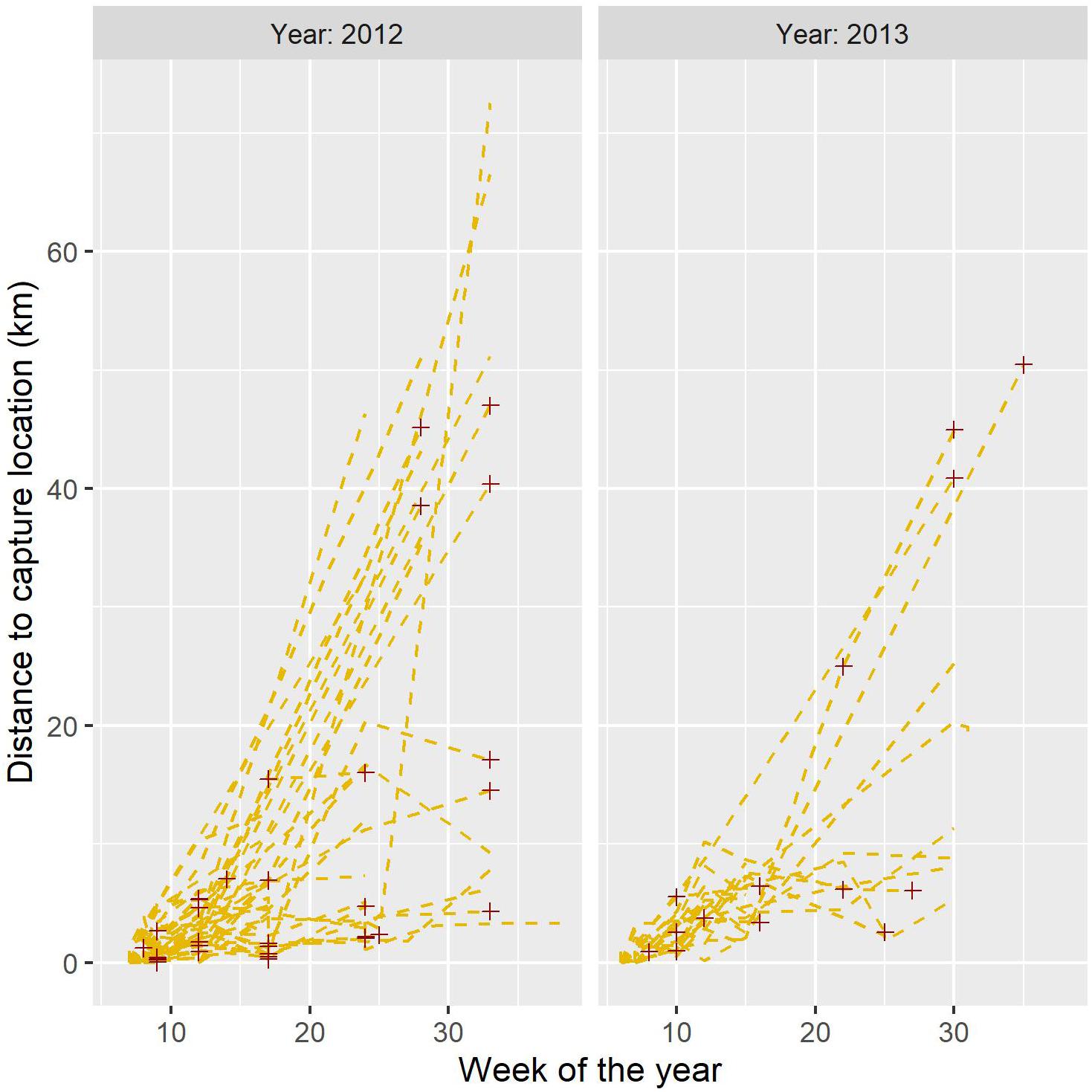
Figure 3. Linear displacement from the capture site plotted agains week. Each line represent the trajectory for one individual bird (n = 82 birds, for which we had at least two positions), and red crosses indicates death events.
Discussion
A main contribution of our study is to provide updated information about some central aspect of rock ptarmigan biology within a central part of its distribution. Although our study is purely exploratory in nature, we assessed some basic ecological aspects of a rock ptarmigan population in Norway. We conclude that (i) overwinter survival is relatively low but with no marked differences between years, age classes or sexes, and (ii) that there are distinct movements between winter and summer areas, and that few birds remained resident in the wintering area.
Survival is a key demographic rate, and variation in survival rates will influence both short- and long term population growth rate (Caswell, 2001). In our study, overwinter (February–July) natural survival was estimated at 55%. Compared to annual survival probabilities for rock ptarmigan in Japan [estimated at 44–74% for birds of different ages: Suzuki et al. (2013)], France [61% and 70% in Haut Giffre and Canigou Massif, respectively: Novoa et al. (2011)], and Svalbard [40–50% for males and females, respectively: Unander et al. (2016)] this is very low survival, bearing in mind that we only estimated survival for a part of the year. At Island, rock ptarmigan survival was shown to be highly variable, varying between 36–65% for adult birds and constant at 19% for juveniles (Sturludottir et al., 2018). Because our study only lasted two years, we are not able to estimate robustly any between year variation due to stochastic environmental factors or variation in harvest pressure. In addition, recent studies of rock ptarmigan throughout their range has shown that cyclic dynamics is a common feature (Fuglei et al., 2019), suggesting that also demographic rates are likely to fluctuate temporarily. Often, populations inhabiting alpine areas (i.e., high altitudes) have higher survival and lower reproduction compared to populations/species at lower elevations (Sandercock et al., 2005a, b). In our study area, this model would predict that rock ptarmigan should have higher survival and lower reproductive output than the willow ptarmigan inhabiting lower elevations. Our results from this short term study is not consistent with a “high survival strategy” for rock ptarmigan in our study area, but we can not conclude if this inconsistency arise due to the short term nature of the field study, or represent a more general life history strategy for rock ptarmigan in Scandinavian mountains. It is however important to note that part of the winter mortality reported in our case study was caused by harvest, which is previously shown to be at least partially additive to other mortality sources in willow ptarmigan (Sandercock et al., 2011).
Most birds that were captured during winter moved out of the wintering area before the breeding season in the spring. Such movements have been discussed in the literature for willow ptarmigan in central Scandinavia, with slightly different conclusions from different study areas (Brøseth et al., 2005; Hornell-Willebrand et al., 2014). For rock ptarmigan we have no comparable data from Scandinavia, but long distance movements have been documented (Gardarsson and Bossert, 1997) with flights >300 km. Examining the genetic structure of rock ptarmigan in southern Scandinavia, Costanzi (2019) reported low genetic differentiation among rock ptarmigan in a large contiguous mountain habitat in southern Norway, but with considerable differentiation between neighboring populations separated by unsuitable rock ptarmigan habitat. Because climate change is likely to result in reduced area occupancy and suitable habitat for rock ptarmigan in alpine areas (see e.g., Revermann et al., 2012 for an example from the Swizz alps), a plausible prediction is that the alpine islands that rock ptarmigan inhabit in the Scandinavian landscape will become both smaller and more isolated in the future. Our study document that long-distance seasonal movements are common among rock ptarmigan in our alpine study area, but a further understanding of their spatial behavior will be key when designing future conservation plans for rock ptarmigan in Scandinavia.
Data Availability Statement
Publicly available datasets were analyzed in this study. This data can be found here: https://www.gbif.org/dataset/b848f1f3-3955-4725-8ad8-e711e4a9e0ac (Nilsen et al., 2017).
Ethics Statement
The animal study was reviewed and approved by the Norwegian Food Safety Authority (Application ID 3960).
Author Contributions
EN, PM, HB, BH, and HP contributed to design and field data collection. EN conducted the statistical analysis of the data, produced the graphical displays, and wrote the manuscript. All authors contributed to the writing and approved the final version of manuscript.
Conflict of Interest
The authors declare that the research was conducted in the absence of any commercial or financial relationships that could be construed as a potential conflict of interest.
Acknowledgments
We would like to thank the Norwegian Environment Agency for funding this study. Several students helped us in the field, and Lierne Fjellstyre provided invaluable help with capture and radio tracking of birds.
Footnotes
References
Bergerud, A. T., Peters, S. S., and McGrath, R. (1963). Determining sex and age of willow ptarmigan in Newfoundland. J. Wildlife Manag. 27, 700–711. doi: 10.2307/3798486
BirdLife_International (2016). Lagopus Muta (Errata Version published in 2017). The IUCN Red List of Threatened Species 2016 [Online]. Cambridge, UK: BirdLife International.
Booms, T. L., Lindgren, M., and Huettmann, F. (2012). “Linking Alaska’s predicted Climate, Gyrfalcon, and Ptarmigan distribution in space and time: A unique 200-year perspective,” in Gyrfalcons and Ptarmigans in a Changing World, eds R. T. Watson, T. J. Cade, M. R. Fuller, G. Hunt, and E. Potapov, (Idaho: The Peregrin Fund), 1.
Brøseth, H., Tufto, J., Pedersen, H. C., Steen, H., and Kastdalen, L. (2005). Dispersal patterns in a harvested willow ptarmigan population. J. Appl. Ecol. 42, 453–459. doi: 10.1111/j.1365-2664.2005.01031.x
Burnham, K. P., and Anderson, D. R. (2002). Model selection and multimodel inference: a practical information-theoretic approach. New York: Springer-Verlag.
Caswell, H. (2001). Matrix population Models: Construction, analysis and interpretation, 2 Edn. Massachusetts: Sinauer Associates Inc.
Costanzi, J.-M. (2019). Habitat isolation in an alpine landscape: effects on population genetics and adult sex ratios in the rock ptarmigan Lagopus muta. Norway. Ph.D.thesis University of South-Eastern Norway.
Costanzi, J.-M., and Steifetten, Ø. (2019). Island biogeography theory explains the genetic diversity of a fragmented rock ptarmigan (Lagopus muta) population. Ecol. Evol. 9, 3837–3849. doi: 10.1002/ece3.5007
Fuglei, E., Henden, J. A., Callahan, C. T., Gilg, O., Hansen, J., Ims, R. A., et al. (2019). Circumpolar status of Arctic ptarmigan: population dynamics and trends Ambio 49, 749–761. doi: 10.1007/s13280-019-01191-0
Hansen, B. B., Grotan, V., Aanes, R., Saether, B. E., Stien, A., Fuglei, E., et al. (2013). Climate Events Synchronize the Dynamics of a Resident Vertebrate Community in the High Arctic. Science 339, 313–315. doi: 10.1126/science.1226766
Heisey, D. M., and Patterson, B. R. (2006). A review of methods to estimate cause-specific mortality in presence of competing risks. J. Wildlife Manag. 70, 1544–1555. doi: 10.2193/0022-541x(2006)70[1544:aromte]2.0.co;2
Hornell-Willebrand, M., Willebrand, T., and Smith, A. A. (2014). Seasonal Movements and Dispersal Patterns: implications for Recruitment and Management of Willow Ptarmigan (Lagopus lagopus). J. Wildlife Manag. 78, 194–201. doi: 10.1002/jwmg.650
Kvasnes, M. A. J., Storaas, T., Pedersen, H. C., Bjork, S., and Nilsen, E. B. (2010). Spatial dynamics of Norwegian tetraonid populations. Ecol. Res. 25, 367–374. doi: 10.1007/s11284-009-0665-7
Lehikoinen, A., Brotons, L., Calladine, J., Campedelli, T., Escandell, V., Flousek, J., et al. (2019). Declining population trends of European mountain birds. Glob. Change Biol. 25, 577–588. doi: 10.1111/gcb.14522
Moss, R., Storch, I., and Muller, M. (2010). Trends in grouse research. Wildlife Biol. 16, 1–11. doi: 10.2981/09-055
Murray, D. L. (2006). On improving telemetry-based survival estimation. J. Wildlife Manag. 70, 1530–1543. doi: 10.2193/0022-541X200670
Murray, D. L., and Patterson, B. R. (2006). Wildlife survival estimation: recent advances and future directions. J. Wildlife Manag. 70, 1499–1503. doi: 10.2193/0022-541x(2006)70[1499:wseraa]2.0.co;2
Nielsen, O. K. (1999). Gyrfalcon predation on ptarmigan: numerical and functional responses. J. Anim. Ecol. 68, 1034–1050. doi: 10.1046/j.1365-2656.1999.00351.x
Nilsen, E. B., Pedersen, H. C., Brøseth, H., and Moa, P. F. (2017). Rock Ptarmigan (Lagopus muta) Radio Telemetry in Lierne, Norway. v1.2. Trondheim: Norwegian Institute for Nature Research. doi: 10.15468/o4zcd3
Novoa, C., Besnard, A., Brenot, J. F., and Ellison, L. N. (2008). Effect of weather on the reproductive rate of Rock Ptarmigan Lagopus muta in the eastern Pyrenees. Ibis 150, 270–278. doi: 10.1111/j.1474-919X.2007.00771.x
Novoa, C., Desmet, J.-F., Brenot, J.-F., Muffat-Joly, B., Arvin-Bérod, M. A., and Tran, B. (2011). “Demographic traits of two alpine populations of rock ptarmigan,” in Ecology, conservation and management of grouse, eds B. K. Sandercock, K. Martin, and G. Segelbacher, (Berkeley: University of California Press), 267–280.
Pedersen, Å, Blanchet, M.-A., Hörnell-Willebrand, M., Jepsen, J., Biuw, M., and Fuglei, E. (2013). Rock Ptarmigan (Lagopus muta) breeding habitat use in northern Sweden. J. Ornithol. 115, 195–209. doi: 10.1007/s10336-013-1001-0
Pedersen, A. O., Bardsen, B. J., Yoccoz, N. G., Lecomte, N., and Fuglei, E. (2012). Monitoring Svalbard rock ptarmigan: distance sampling and occupancy modeling. J. Wildlife Manag. 76, 308–316. doi: 10.1002/jwmg.276
Pollock, K. H., Winterstein, S. R., Bunck, C. M., and Curtis, P. D. (1989). Survival analysis in telemetry studies - the staggered entry design. J. Wildlife Manag. 53, 7–15.
Post, E., Forchhammer, M. C., Bret-Harte, M. S., Callaghan, T. V., Christensen, T. R., Elberling, B., et al. (2009). Ecological Dynamics Across the Arctic Associated with Recent Climate Change. Science 325, 1355–1358. doi: 10.1126/science.1173113
Potapov, R., and Potapov, E. (2012). Willow and Rock Ptarmigan monitoring in Russia: An historic overview. Boise: Peregrine Fund.
R Development Core Team (2019). R: A language and environment for statistical computing. Vienna: R Foundation for Statistical Computing.
Revermann, R., Schmid, H., Zbinden, N., Spaar, R., and Schröder, B. (2012). Habitat at the mountain tops: how long can Rock Ptarmigan (Lagopus muta helvetica) survive rapid climate change in the Swiss Alps? A multi-scale approach. J. Ornithol. 153, 891–905. doi: 10.1007/s10336-012-0819-1
Sandercock, B. K., Martin, K., and Hannon, S. J. (2005a). Demographic consequences of age-structure in extreme environments: population models for arctic and alpine ptarmigan. Oecologia 146, 13–24. doi: 10.1007/s00442-005-0174-5
Sandercock, B. K., Martin, K., and Hannon, S. J. (2005b). Life history strategies in extreme environments: comparative demography of Arctic and alpine Ptarmigan. Ecology 86, 2176–2186. doi: 10.1890/04-0563
Sandercock, B. K., Nilsen, E. B., Broseth, H., and Pedersen, H. C. (2011). Is hunting mortality additive or compensatory to natural mortality? Effects of experimental harvest on the survival and cause-specific mortality of willow ptarmigan. J. Anim. Ecol. 80, 244–258. doi: 10.1111/j.1365-2656.2010.01769.x
Scherini, G. C., Tosi, G., and Wauters, L. A. (2003). Social behaviour, reproductive biology and breeding success of alpine Rock Ptarmigan Lagopus mutus helveticus in northern Italy. Ardea 91, 11–23.
Sirami, C., Caplat, P., Popy, S., Clamens, A., Arlettaz, R., Jiguet, F., et al. (2017). Impacts of global change on species distributions: obstacles and solutions to integrate climate and land use. Glob. Ecol. Biogeogr. 26, 385–394. doi: 10.1111/geb.12555
Sturludottir, E., Nielsen, O. K., and Stefansson, G. (2018). Evaluation of Ptarmigan Management with a Population Reconstruction Model. J. Wildlife Manag. 82, 958–965. doi: 10.1002/jwmg.21458
Suzuki, A., Kobayashi, A., Nakamura, H., and Takasu, F. (2013). Population viability analysis of the Japanese rock ptarmigan Lagopus muta japonica in Japan. Wildlife Biol. 19, 339–346. doi: 10.2981/13-021
Therneau, T. (2015). A Package for Survival Analysis in S. https://CRAN.R-project.org/package=survival.
Unander, S., Pedersen, A. O., Soininen, E. M., Descamps, S., Hornell-Willebrand, M., and Fuglei, E. (2016). Populations on the limits: survival of Svalbard rock ptarmigan. J. Ornithol. 157, 407–418. doi: 10.1007/s10336-015-1282-6
Watson, A., Moss, R., and Rae, S. (1998). Population dynamics of Scottish Rock Ptarmigan cycles. Ecology 79, 1174–1192. doi: 10.1890/0012-9658(1998)079[1174:PDOSRP]2.0.CO;2
Wilson, S., and Martin, K. (2008). Breeding habitat selection of sympatric White-tailed, Rock and Willow Ptarmigan in the southern Yukon Territory, Canada. J. Ornithol. 149, 629–637. doi: 10.1007/s10336-008-0308-8
Keywords: alpine wildlife, population ecology, hunting mortality, tetraonids, survival
Citation: Nilsen EB, Moa PF, Brøseth H, Pedersen HC and Hagen BR (2020) Survival and Migration of Rock Ptarmigan in Central Scandinavia. Front. Ecol. Evol. 8:34. doi: 10.3389/fevo.2020.00034
Received: 18 September 2019; Accepted: 06 February 2020;
Published: 25 February 2020.
Edited by:
Miguel Lurgi, Swansea University, United KingdomReviewed by:
Davide Scridel, Museo delle Scienze, ItalyRolf A. Ims, UiT The Arctic University of Norway, Norway
Copyright © 2020 Nilsen, Moa, Brøseth, Pedersen and Hagen. This is an open-access article distributed under the terms of the Creative Commons Attribution License (CC BY). The use, distribution or reproduction in other forums is permitted, provided the original author(s) and the copyright owner(s) are credited and that the original publication in this journal is cited, in accordance with accepted academic practice. No use, distribution or reproduction is permitted which does not comply with these terms.
*Correspondence: Erlend B. Nilsen, erlend.nilsen@nina.no
†These authors share first authorship