Low Nitrous Oxide Emissions in a Boreal Spruce Forest Soil, Despite Long-Term Fertilization
- Department of Earth Sciences, University of Gothenburg, Gothenburg, Sweden
Nitrogen (N) fertilization can increase stem wood production by several hundred percent in boreal forests. At the same time, there are concerns about the environmental consequences of N fertilization, especially considering losses of the greenhouse gas nitrous oxide (N2O) to the atmosphere. Soils are a large contributor to N2O emissions on a global scale. The aim of this study was to investigate the consequences of long-term nutrient optimization fertilization on N2O emissions in a boreal forest in Northern Sweden. Field N2O flux measurements were conducted during 2 years with manual and automatic chambers, as well as gas probes in the snow. The N2O emissions were generally low during the whole period of measurements, both from the control and fertilized plots. The emissions were generally highest during the winters, as well as the variability in the observed values. Overall, N2O emissions from fertilized plots were about twice the control, which could be explained by changes in the soil carbon-to-nitrogen ratio.
Introduction
Nitrogen (N) is the main limiting nutrient for growth in many boreal forests (Tamm, 1991; Högberg et al., 2017) and fertilization is therefore an effective way to increase the net primary production in this slow growing ecosystem (Axelsson and Axelsson, 1986; Linder, 1987). In Northern Sweden, fertilization can increase stem wood production with more than 350% (Bergh et al., 2005). However, there are concerns about the environmental consequences of intensive forest fertilization practices, primarily due to the drastic changes induced on the ecosystem level. Sustained N addition to initial N-limited ecosystems can in the long-term lead to an N saturation of the ecosystem (Aber et al., 1998), which increases the risk of N losses to water and air (Bremner and Blackmer, 1978; Binkley et al., 1999). Nitrous oxide (N2O) emissions from the forest floor have been shown to be related to N input (Zechmeister-Boltenstern et al., 2002) and to the mineral N content in the soil in general, especially in soils where the dominant form of N is nitrate (NO3–) (Davidson et al., 2000). Soils are, on global scale, a source of N2O to the atmosphere (Ciais et al., 2013) and forests contribute to a significant part of the emissions (Bowden et al., 1990; Ambus and Christensen, 1995; Kesik et al., 2005). Leaching of NO3– is often related to anthropogenic inputs, either N deposition or fertilization (Sponseller et al., 2014). In non-fertilized boreal forests with low N deposition, the majority of the leaching to streams and waters is in the form of dissolved organic nitrogen (Hedin et al., 1995; Stepanauskas et al., 2000).
Generally, forest N fertilization enhances N2O emissions, although a few exceptions exists (Gundersen et al., 2012; Shrestha et al., 2015). There are, though, few data from boreal forests, which are usually characterized by low N2O emissions (Bai et al., 2012), due to its N-limited status. Therefore, N fertilization of this ecosystem can be expected to lead to increased N2O emissions. Indeed, in the review by Gundersen et al. (2012) the largest stimulation of N2O emission by N addition was observed for a wet hemiboreal forest in south-western Sweden, confirming earlier results from the same forest (Klemedtsson et al., 1997). In contrast, Maljanen et al. (2006) did not observe stimulated N2O emission in a boreal forest in southern Finland. The paucity of N2O emission from N-fertilized boreal forests and the contradicting results so far, motivated us to conduct N2O measurements from a N-limited boreal forest in Northern Sweden (Flakaliden) that had been subjected to fertilization (optimal nutrient management, including addition of 50–100 kg N ha–1 year–1) for more than 20 years (Linder, 1995). The leaching of inorganic N from the control and fertilized plots in this forest is less than 1 kg N ha–1 year–1 (Andersson et al., 2002), but so far N2O emissions were unknown. We expected that the unfertilized forest is characterized by low N2O emissions, as generally found for N-limited boreal forests, and that the long-term fertilization led to enhanced N2O emissions, particularly under wet condition. We tested this with field N2O flux measurements during two full years.
Materials and Methods
Site
Flakaliden (64°07′N, 19°24′E) is a Norway spruce [Picea abies (L.) Karst.] forest situated in Vindeln municipality, Northeast Sweden. The altitude is 310–320 m above sea level. The climate is boreal, with short, cold summers with long days and long winters with short days. Mean annual temperature (MAT) and precipitation (MAP) for the period 1990–2019 at the site is 2.4° and 647 mm, respectively (Linder, pers. comm.). One-third of the precipitation falls as snow (Bergh et al., 1999). The annual N deposition in the area is 4.6 kg ha–1 year–1 (Hedwall et al., 2013). The soil texture is sandy and soil type Podzol, with an organic layer between 3 and 6 cm. In 1963, the site was planted with seedlings of Norway spruce of local descent. In 1986, an experiment with different types of fertilization treatments was started (Linder, 1995). In this study, we investigated plots that were annually given a solid fertilizer mix (nutrient optimization) containing among other nutrients an amount of 50–100 kg N ha–1 in the form of ammonium nitrate (NH4NO3, hereafter referred to as fertilized plots), as well as untreated control plots. In the years of N2O measurements, N was added each year in May at a rate of 50 kg N ha–1. The field layer in the fertilized plots was very sparse, while in the control plots it was dominated by dwarf-shrubs (Strengbom et al., 2011).
Field Measurements of N2O
Emissions of N2O were measured during two full years from February 2009 to April 2011 using thee different methods: manual chambers in June–December 2009, automatic chambers June–December 2010, and with snow probes during winters (March–April 2009, March–May 2010, and January–April 2011). The study was planned for automatic chambers, which though were delayed and could not started before year 2. The automatic chambers set a limit to the distance between the chambers, due to the maximum length of the tunings between the chambers and analyzer. Therefore, only one fertilized plot and one control plot (50 × 50 m, each) could be measured. In each plot, six metal collars (45 × 45 cm) collars were inserted permanently into the ground to a depth of 2 cm to minimize disturbance during measurements. As previous research found that the spatial dependency of N2O emission is in the range of <1 to ∼70 m (Ambus and Christensen, 1994; Velthof et al., 1996; Turner et al., 2008), our approach likely covered most of the spatial variability in at the study site.
Measurements with manual chambers (n = 6 per treatment) were done weekly in the snow free period 2009 (June–December). The chambers were closed with a lid on top of the permanently installed metal frames. The inside of the lids were lined with a neoprene rubber sealing to avoid air leakage when closed. The volume of each chamber was measured individually, due to differences in topography between the collars. During measurements, gas samples were taken every 10 min during 30 min (four samples), starting 5 min after chamber closure (total chamber closure was 35 min) following the procedure described in Klemedtsson et al. (1997). The sample vials were analyzed on a gas chromatograph and the fluxes were calculated with linear regression, from the concentration changes in the chambers during closure (Klemedtsson et al., 1997).
In June to December 2010, three automatic chambers per treatment were used, on the same collars as for the manual chamber measurements. The chambers, were moved every week between the six collars to avoid long-term chamber effects (e.g., moisture and temperature). The chambers had a lid and sides of transparent polythene plastic, held together by an aluminum frame. The volume of the chamber (including the collar) was about 0.24 m3. The gas sampling system consisted of a tunable diode laser (Campbell Scientific, TGA100A), a valve system, and a data logger (Campbell Scientific, CR-3000). The data logger controlled pressure, flow, opening, and closure of the chambers, which chamber that was connected to the laser, as well as data collection. The chambers were closed for 40 min/h, and sampling air from each chamber was measured for 20 s every other minute. The first 10 s of the measurements were discarded due to remaining air from the previous sample, and the remaining 10 s were used for calculations of concentrations. The concentration increase in the chambers was calculated with linear regression. For more information about the automatic chamber system, see Hedenrud (2015).
During the winter, the concentrations of N2O in the snow profile were measured with gas probes, which were conducted in the near vicinity (0.5–1 m) if the chamber frames, to avoid disturbance of the abiotic conditions underneath the chambers, but representing the same conditions. The gas samples from the probe were transferred into gas vials sealed with a butyl membrane and analyzed using gas chromatography. Snow depth and density were measured at each sampling occasion. The net fluxes of N2O were calculated according to Sommerfeld et al. (1993). The snow probe method is described in detail by Björkman et al. (2010). In 2009, the snow probes could not be used when the snow layer became too thin. New probes were used in 2010, which were adapted to lower snow depths. Björkman et al. (2010) reported that soil respiration measured with snow probes was not different from fluxes measured with chambers on top of the snow. In addition, data from snow probes had lower variability.
Statistics
Flux data were analyzed with the non-parametric Wilcoxon Signed Ranks test for each measured period separately, since the data was obtained with different measurement techniques and with different frequency. Statistical analyses were performed with SPSS (IBM SPSS Statistics 25). In addition, Spearman Rank Order Correlation was conducted using SigmaPlot (version 14.0; Systat Software, Inc.).
Results
The years 2009 and 2010 were rather normal in terms of precipitation (MAP of 673 and 618 mm, respectively; Figure 1B). While 2010 was colder (MAT of 0.5°C) than the long-term average, 2009 was rather normal warm (MAT of 2.0°C; Figure 1B).
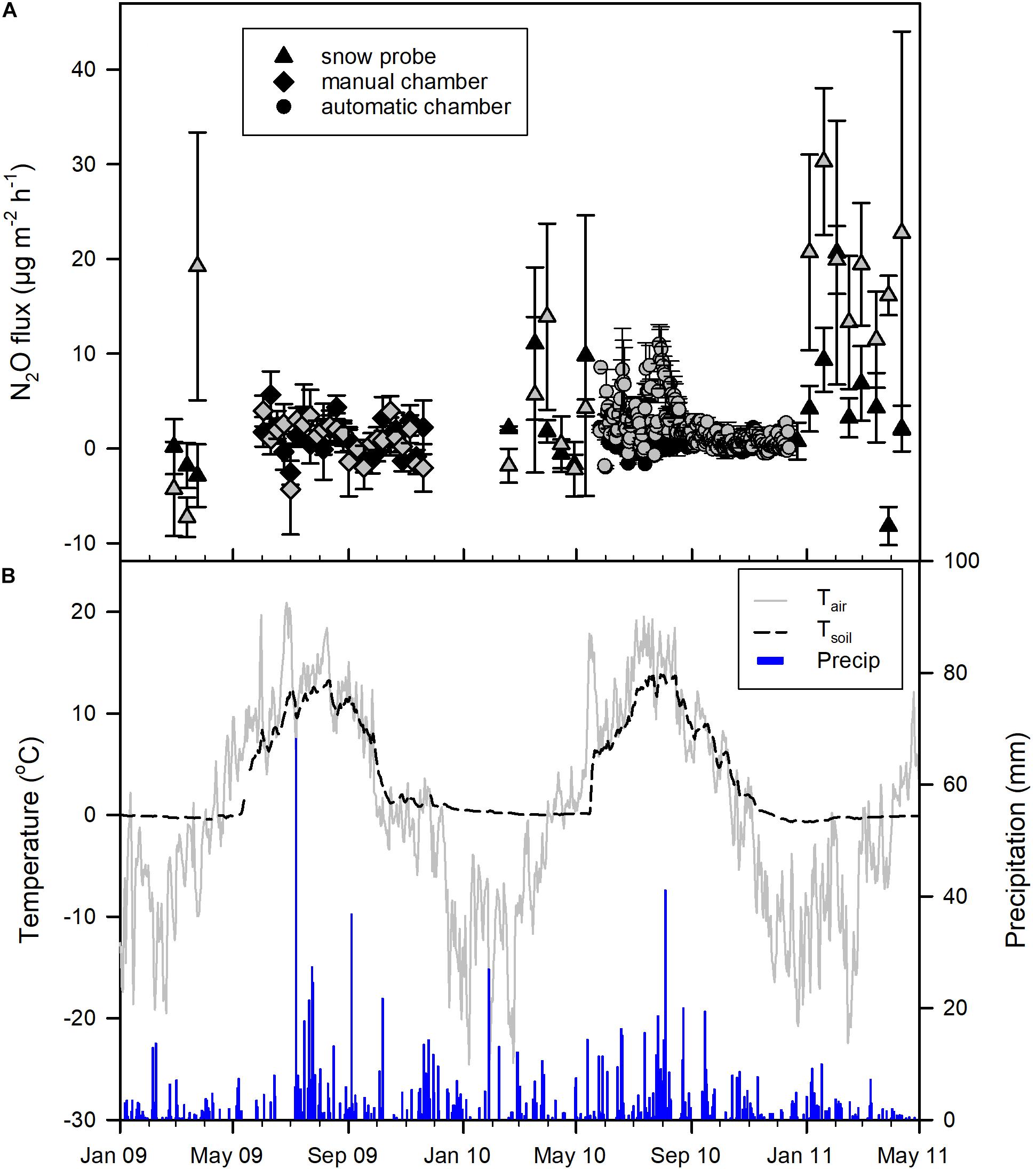
Figure 1. (A) Soil emissions of N2O from a Norway spruce forest at Flakaliden from non-fertilized control (black symbols) and fertilized plots (gray symbols). Data are means and standard deviation. (B) Air and soil (20 cm depth) temperature as well as precipitation at Flakaliden.
The N2O emissions were low from both the control and fertilized plot at the site. The mean (±SD) N2O flux for the entire measurement period was 0.97 (±2.15) μg N2O m–2 h–1 for the control plot and 2.67 (±4.30) μg N2O m–2 h–1 for the fertilized plot (Figure 1A). During the first year of measurements (March 2009–February 2010) there was no statistical significant difference between control and fertilized plots, but during summer and fall 2010, when fluxes were measured with automatic chambers, the emissions from the fertilized plot were significantly higher compared to the control plot (p < 0.001, see Figure 2). Fertilization was conducted at the end of May 2010 and the significantly higher fluxes were primarily detected from June to September. During the rest of the period measured with automatic chambers (October–December) the emissions were low and there was no significant difference between control and fertilized plots (Figure 2). When measurements were conducted with snow probes, the emissions were both high and had high variability (Figure 1A). Winter measurements showed significant differences between the control and the fertilized plot only in 2011 (January–April), where the fertilized plot had higher emissions compared to the control (Figure 1A).
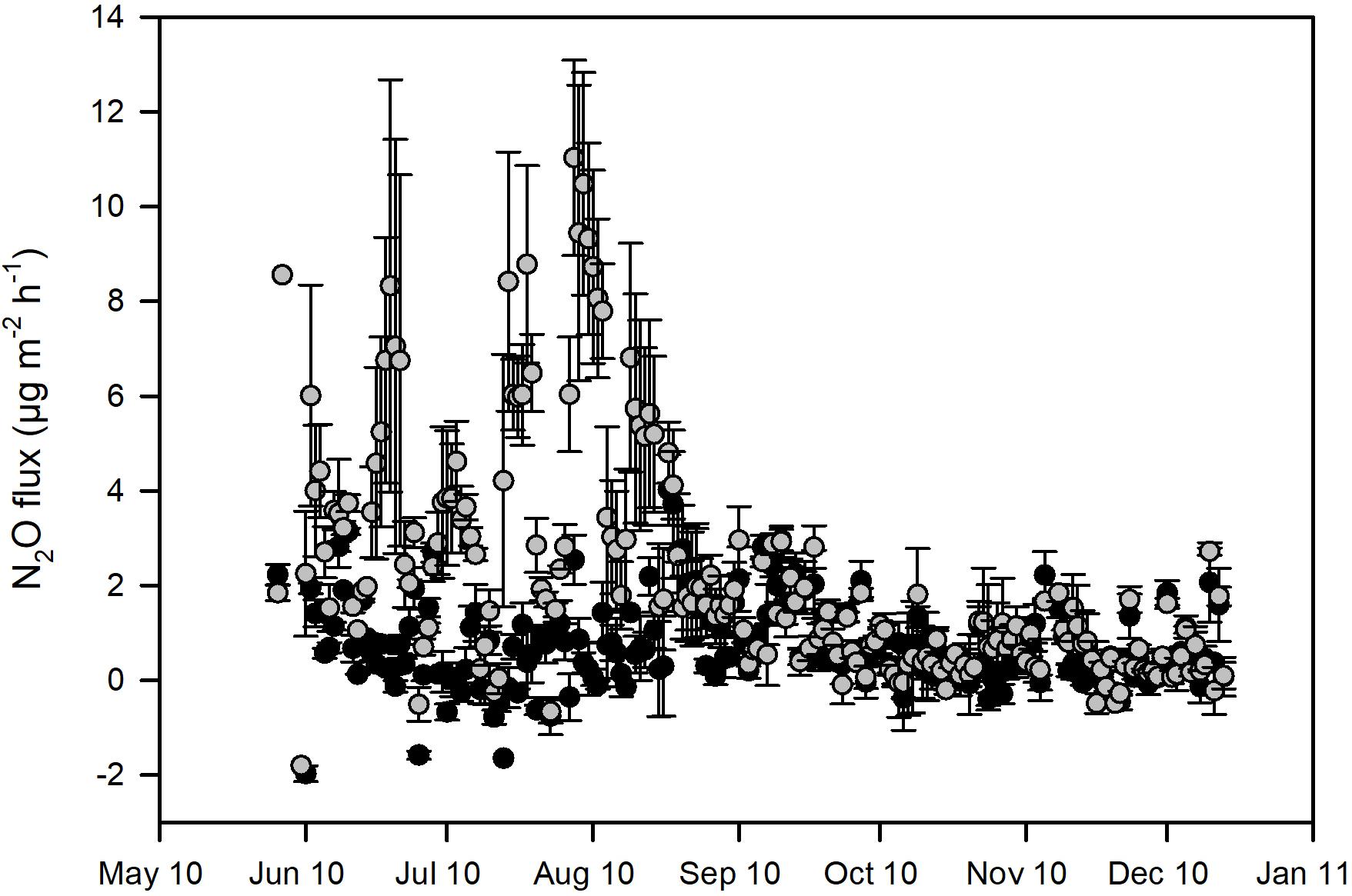
Figure 2. Detail of the soil N2O emissions from a Norway spruce forest at Flakaliden measured with automatic chambers at daily resolution during the summer and fall 2010, from non-fertilized control (black symbols) and fertilized plots (gray symbols).
The mean of the emissions is biased by sampling frequency, due to the use of manual and automatic chambers during years 1 and 2, respectively. We therefore in addition integrated fluxes by linear interpolation between subsequent measurement days, but not for gaps between chamber and snow probe measurements. The mean of these integrated fluxes was 1.98 and 4.79 μg N2O m–2 h–1 for control and fertilized plot, respectively, hence about twice the average daily fluxes. This difference is due to the less frequent measurements of winter emission, which, though, often were higher than summer and fall emissions (Figure 1A).
Correlations with climatic variables were conducted for the period of N2O flux measurements by automatic chambers and for the period with all chamber measurements (manual and automatic). While no significant correlations were detected for the fluxes from the control plot (Table 1), N2O emissions from the fertilized plot was positively correlated with both, soil and air temperature, explaining 34–41% of the observed variability (Table 1). In addition, the correlation with precipitation was significant for the fertilized plot, but with much lower explained variability (2–3%; Table 1).
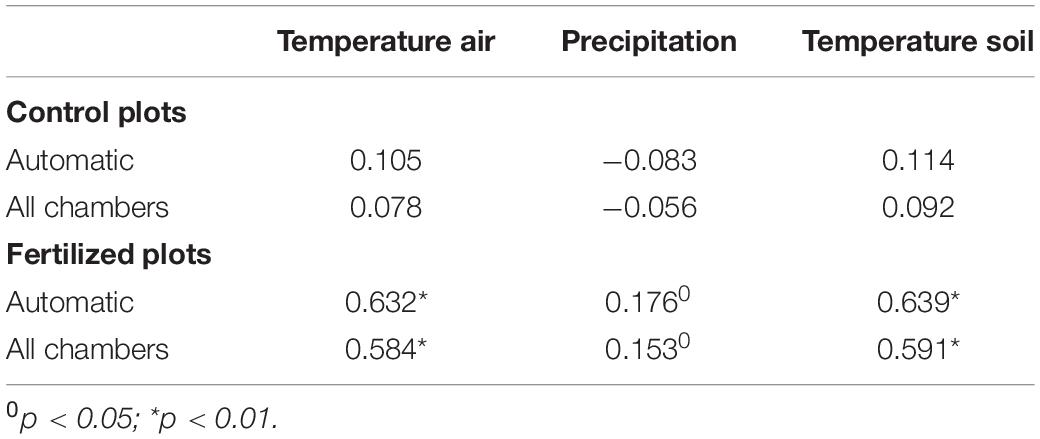
Table 1. Spearman Rank Order Correlation coefficient between N2O fluxes and climatic factors: air and soil (20 cm depth) temperatures as well as precipitation for the period with automatic chambers and period including all chamber measurements (manual and automatic).
Discussion
The N2O emissions were mostly low during the whole series of measurements, both from the control and fertilized plots, which is a general observation for boreal forests (Klemedtsson et al., 1997; Kim and Tanaka, 2003; Matson et al., 2009; Ullah et al., 2009). The N2O emissions were highest during the winter, which has been reported before for forest ecosystems (Sommerfeld et al., 1993; Brooks et al., 1997; Groffman et al., 2006). Snow insulates the ground, which means that the soil temperature can be above zero degrees although the air temperature is lower, allowing N2O production to continue under the snow (Enanga et al., 2016). The large variability in the winter measurements is connected to the sampling method with gas probes, where the variation between the different probes was much higher than for the chambers. This may be due to variations in snow density, which strongly affects the emissions from the snow (Björkman et al., 2010).
Overall, the N2O emissions from the fertilized plot was about twice the control, but statistical significance was only observed during the second year, when measurements were conducted with automatic chambers. It is a common observation that N2O fluxes have high temporal variability (Groffman et al., 2009) and with more frequent sampling, there is a better chance of capturing this variability. Indeed, a recent study concluded that at least two flux measurements per day are required to achieve robust annual emission estimates and to detect differences between treatments in field trials (Lammirato et al., unpublished data). We therefore can conclude with confidence that fertilization of the boreal forest at Flakaliden did enhance N2O emissions. While the measurements were conducted in a nutrient optimization experiment, we assign most of the enhanced N2O emission to N addition, as it is well known that N is the main limiting nutrient for growth in boreal forests (Tamm, 1991; Högberg et al., 2017). We, though, cannot fully rule out that other nutrients might have stimulated microbial growth and, potentially, N2O production and emission.
Two review papers (Gundersen et al., 2012; Shrestha et al., 2015) concluded that N fertilization generally enhances N2O emission from forests, although exceptions exist. However, few of the reviewed studies have been conducted in boreal forest. While Maljanen et al. (2006) did not observe enhanced N2O emission due to fertilization in a boreal forest in Finland, N fertilization turned a hemi-boreal forest in south-western Sweden from a sink into a significant source of N2O [reported in Gundersen et al. (2012)]. Large stimulation of N2O emission by fertilization was also reported for a subalpine coniferous forest after 8 years of yearly N addition (Li et al., 2019).
The difference between fertilized and control fluxes in our study can largely be explained by the C/N ratio of the soil, which is a good proxy for N2O emissions from forest soils (Klemedtsson et al., 2005; Pilegaard et al., 2006). Björsne (2018) measured several soil properties in the investigated forests plots in 2016 and found significantly lower C/N ratio in the fertilized than control plots (26.4 and 32.2, respectively. In addition, other changes in soil properties could have contributed to the higher N2O fluxes from fertilized plots. Björsne (2018) also observed numerically lower soil pH in the fertilized plots, which could lead to higher N2O emissions as well (Weslien et al., 2009).
Earlier studies have estimated N2O emissions derived from fertilization in forests to be 0.5–1% of the added N (Macdonald et al., 1997; Papen and Butterbach-Bahl, 1999; Maljanen et al., 2006). The comparable number for the present study is 0.3%, based on the integrated fluxes over the entire measurement period. In a pan-European study (Gundersen et al., 2012) the increase in mean N2O emissions due to fertilization ranges from 2 to 230 mg N m–2 year–1, and our study would range at the lower end (9 mg N m–2 year–1). Overall, we conclude that N is even in the fertilized plot relatively tightly cycled and efficiently used, pointing to prevailing N limitation even after long-term N fertilization.
Data Availability Statement
The raw data supporting the conclusions of this article will be made available by the authors, without undue reservation.
Author Contributions
LK designed the experiment. PW conducted measurements and flux analysis. All authors discussed and interpreted results. A-KB and TR drafted the manuscript, to which all authors contributed.
Funding
This work was financially supported by the Swedish Energy Agency, with additional funding by the Swedish Research Council (VR; grant number 2010-3795) and the Swedish Research Council Formas (grant number 2010-3795).
Conflict of Interest
The authors declare that the research was conducted in the absence of any commercial or financial relationships that could be construed as a potential conflict of interest.
Publisher’s Note
All claims expressed in this article are solely those of the authors and do not necessarily represent those of their affiliated organizations, or those of the publisher, the editors and the reviewers. Any product that may be evaluated in this article, or claim that may be made by its manufacturer, is not guaranteed or endorsed by the publisher.
Acknowledgments
This study has been made possible by the Swedish Infrastructure for Ecosystem Science (SITES) in Svartberget. Sune Linder (SLU) is thanked for answering questions about the field experiment. This manuscript is a contribution to the Strategic Research Area BECC (Biodiversity and Ecosystem Services in a Changing Climate; www.becc.lu.se).
References
Aber, J., McDowell, W., Nadelhoffer, K., Magill, A., Berntson, G., Kamakea, M., et al. (1998). Nitrogen saturation in temperate forest ecosystems. BioScience. 48, 921–934. doi: 10.2307/1313296
Ambus, P., and Christensen, S. (1994). Measurement of N2O emission from a fertilzed grassland: an analysis of spatial variability. J. Geophys. Res. 99, 16549–16555. doi: 10.1029/94jd00267
Ambus, P., and Christensen, S. (1995). Spatial and seasonal nitrous oxide and methane fluxes in Danish forest ecosystems, grassland ecosystems, and agroecosystems. J. Environ. Qual. 24, 993–1001. doi: 10.2134/jeq1995.00472425002400050031x
Andersson, P., Berggren, D., and Nilsson, I. (2002). Indices for nitrogen status and nitrate leaching from Norway spruce (Picea abies (L.) Karst.) stands in Sweden. For. Ecol. Manag. 157, 39–53. doi: 10.1016/s0378-1127(00)00651-4
Axelsson, E., and Axelsson, B. (1986). Changes in carbon allocation patterns in spruce and pine trees following irrigation and fertilization. Tree Physiol. 2, 189–204. doi: 10.1093/treephys/2.1-2-3.189
Bai, E., Houlton, B. Z., and Wang, Y. P. (2012). Isotopic identification of nitrogen hotspots across natural terrestrial ecosystems. Biogeosciences 9, 3287–3304. doi: 10.5194/bg-9-3287-2012
Bergh, J., Linder, S., and Bergström, J. (2005). Potential production of Norway spruce in Sweden. For. Ecol. Manag. 204, 1–10. doi: 10.1016/j.foreco.2004.07.075
Bergh, J., Linder, S., Lundmark, T., and Elfving, B. (1999). The effect of water and nutrient availability on the productivity of Norway spruce in northern and southern Sweden. Forest Ecol. Manag. 119, 51–62. doi: 10.1016/S0378-1127(98)00509-X
Binkley, D., Burnham, H., and Allen, H. L. (1999). Water quality impacts of forest fertilization with nitrogen and phosphorus. For. Ecol. Manag. 121, 191–213. doi: 10.1016/s0378-1127(98)00549-0
Björkman, M. P., Morgner, E., Björk, R. G., Cooper, E. J., Elberling, B., and Klemedtsson, L. (2010). A comparison of annual and seasonal carbon dioxide effluxes between sub-Arctic Sweden and High-Arctic Svalbard. Polar Res. 29, 75–84. doi: 10.1111/j.1751-8369.2010.00150.x
Björsne, A.-K. (2018). The Nitrogen Cycle In Soil-Climate impact and Methodological Challenges in Natural Ecosystems. Ph.D. thesis, University of Gothenburg, Sweden.
Bowden, R. D., Steudler, P. A., Melillo, J. M., and Aber, J. D. (1990). Annual nitrous oxide fluxes from temperate forest soils in the Northeastern United-States. J. Geophys. Res. Atmos. 95, 13997–14005. doi: 10.1029/jd095id09p13997
Bremner, J. M., and Blackmer, A. M. (1978). Nitrous oxide-Emission from soils during nitrification of fertilizer nitrogen. Science 199, 295–296. doi: 10.1126/science.199.4326.295
Brooks, P. D., Schmidt, S. K., and Williams, M. W. (1997). Winter production of CO2 and N2O from Alpine tundra: environmental controls and relationship to inter-system C and N fluxes. Oecologia 110, 403–413. doi: 10.1007/pl00008814
Ciais, P., Chris, S., Govindasamy, B., Bopp, L., Chhabra, A., Defries, R. S., et al. (2013). “Carbon and other biogeochemical cycles,” in Climate Change 2013: The Physical Science Basis. Contribution of Working Group I to the Fifth Assessment Report of the Intergovernmental Panel on Climate Change, eds T. F. Stocker, et al. (Cambridge: Cambridge University Press).
Davidson, E. A., Keller, M., Erickson, H. E., Verchot, L. V., and Veldkamp, E. (2000). Testing a conceptual model of soil emissions of nitrous and nitric oxides. BioScience 50, 667–680. doi: 10.1641/0006-3568(2000)050[0667:tacmos]2.0.co;2
Enanga, E. M., Creed, I. F., Fairweather, T., and Casson, N. J. (2016). Snow-covered soils produce N2O that is lost from forested catchments. J. Geophys. Res. Biogeo. 121, 2356–2368. doi: 10.1002/2016jg003411
Groffman, P. M., Butterbach-Bahl, K., Fulweiler, R. W., Gold, A. J., Morse, J. L., Stander, E. K., et al. (2009). Challenges to incorporating spatially and temporally explicit phenomena (hotspots and hot moments) in denitrification models. Biogeochemistry 93, 49–77. doi: 10.1007/s10533-008-9277-5
Groffman, P. M., Hardy, J. P., Driscoll, C. T., and Fahey, T. J. (2006). Snow depth, soil freezing, and fluxes of carbon dioxide, nitrous oxide and methane in a northern hardwood forest. Glob. Change Biol. 12, 1748–1760. doi: 10.1111/j.1365-2486.2006.01194.x
Gundersen, P., Christiansen, J. R., Alberti, G., Brüggemann, N., Castaldi, S., Gasche, R., et al. (2012). The response of methane and nitrous oxide fluxes to forest change in Europe. Biogeosciences 9, 3999–4012. doi: 10.5194/bg-9-3999-2012
Hedenrud, A. (2015). Nitrous Oxide Emissions From A Willow Plantation In Southwestern Sweden. Licentiate thesis. University of Gothenburg, Sweden.
Hedin, L. O., Armesto, J. J., and Johnson, A. H. (1995). Patterns of nutrient loss from unpolluted, old-growth temperate forests: evaluation of biogeochemical theory. Ecology 76, 493–509. doi: 10.2307/1941208
Hedwall, P.-O., Nordin, A., Strengbom, J., Brunet, J., and Olsson, B. (2013). Does background nitrogen deposition affect the response of boreal vegetation to fertilization? Oecologia 310, 382–392.
Högberg, P., Näsholm, T., Franklin, O., and Högberg, M. N. (2017). Tamm review: on the nature of the nitrogen limitation to plant growth in Fennoscandian boreal forests. For. Ecol. Manag. 403, 161–185. doi: 10.1016/j.foreco.2017.04.045
Kesik, M., Ambus, P., Baritz, R., Brüggemann, N., Butterbach-Bahl, K., Damm, M., et al. (2005). Inventories of N2O and NO emissions from European forest soils. Biogeosciences 2, 353–375.
Kim, Y., and Tanaka, N. (2003). Effect of forest fire on the fluxes of CO2, CH4 and N2O in boreal forest soils, interior Alaska. J. Geophys. Res. Atmos. 108:8154. doi: 10.1029/2001JD000663
Klemedtsson, L., Klemedtsson, A. K., Moldan, F., and Weslien, P. (1997). Nitrous oxide emission from Swedish forest soils in relation to liming and simulated increased N-deposition. Biol. Fertil. Soils 25, 290–295. doi: 10.1007/s003740050317
Klemedtsson, L., von Arnold, K., Weslien, P., and Gundersen, P. (2005). Soil CN ratio as a scalar parameter to predict nitrous oxide emissions. Glob. Change Biol. 11, 1142–1147. doi: 10.1111/j.1365-2486.2005.00973.x
Li, D., Liu, Q., Yin, H., Luo, Y., and Hui, D. (2019). Differential responses and controls of soil CO2 and N2O fluxes to experimental warming and nitrogen fertilization in a subalpine coniferous spruce (Picea asperata Mast.) plantation forest. Forests 10:808. doi: 10.3390/f10090808
Linder, S. (1987). “Responses to water and nutrition in coniferous ecosystems,” in Potentials and Limitations of Ecosystem Analysis, ed. E.-D. Schulze (Berlin: Springer).
Linder, S. (1995). Foliar analysis for detecting and correcting nutrient imbalances in Norway spruce. Ecol. Bull. 178–190.
Macdonald, J. A., Skiba, U., Sheppard, L. J., Ball, B., Roberts, J. D., Smith, K. A., et al. (1997). The effect of nitrogen deposition and seasonal variability on methane oxidation and nitrous oxide emission rates in an upland spruce plantation and moorland. Atmos. Environ. 31, 3693–3706. doi: 10.1016/s1352-2310(97)00265-3
Maljanen, M., Jokinen, H., Saari, A., Strömmer, R., and Martikainen, P. J. (2006). Methane and nitrous oxide fluxes, and carbon dioxide production in boreal forest soil fertilized with wood ash and nitrogen. Soil Use Manag. 22, 151–157. doi: 10.1111/j.1475-2743.2006.00029.x
Matson, A., Pennock, D., and Bedard-Haughn, A. (2009). Methane and nitrous oxide emissions from mature forest stands in the boreal forest, Saskatchewan, Canada. For. Ecol. Manag. 258, 1073–1083. doi: 10.1016/j.foreco.2009.05.034
Papen, H., and Butterbach-Bahl, K. (1999). A 3-year continuous record of nitrogen trace gas fluxes from untreated and limed soil of a N-saturated spruce and beech forest ecosystem in Germany: 1. N2O emissions. J. Geophys. Res. Atmos. 104, 18487–18503. doi: 10.1029/1999jd900293
Pilegaard, K., Skiba, U., Ambus, P., Beier, C., Brüggemann, N., Butterbach-Bahl, K., et al. (2006). Factors controlling regional differences in forest soil emission of nitrogen oxides (NO and N2O). Biogeosciences 3, 651–661. doi: 10.5194/bg-3-651-2006
Shrestha, R. K., Strahm, B. D., and Sucre, E. B. (2015). Greenhouse gas emissions in response to nitrogen fertilization in managed forest ecosystems. New Forests 46, 167–193. doi: 10.1007/s11056-014-9454-4
Sommerfeld, R. A., Mosier, A. R., and Musselman, R. C. (1993). CO2. CH4 and N2O flux through a Wyoming snowpack and implications for global budgets. Nature 361:140. doi: 10.1038/361140a0
Sponseller, R. A., Temnerud, J., Bishop, K., and Laudon, H. (2014). Patterns and drivers of riverine nitrogen (N) across alpine, subarctic, and boreal Sweden. Biogeochemistry 120, 105–120. doi: 10.1007/s10533-014-9984-z
Stepanauskas, R., Laudon, H., and Jørgensen, N. O. G. (2000). High DON bioavailability in boreal streams during a spring flood. Limnol. Oceanogr. 45, 1298–1307. doi: 10.4319/lo.2000.45.6.1298
Strengbom, J., Dahlberg, A., Larsson, A., Lindelöw, Å, Sandström, J., Widenfalk, O., et al. (2011). Introducing intensively managed spruce plantations in Swedish: forest landscapes will impair biodiversity decline. Forests 2, 610–630. doi: 10.3390/f2030610
Tamm, C. O. (1991). Nitrogen in Terrestrial Ecosystems: Questions of Productivity, Vegetational Changes, and Ecosystem Stability. Berlin: Springer.
Turner, D. A., Chen, D., Galbally, I. E., Leuning, R., Edis, R. B., Li, Y., et al. (2008). Spatial variability of nitrous oxide emissions from an Australian irrigated dairy pasture. Plant Soil 309, 77–88. doi: 10.1007/s11104-008-9639-8
Ullah, S., Frasier, R., Pelletier, L., and Moore, T. R. (2009). Greenhouse gas fluxes from boreal forest soils during the snow-free period in Quebec, Canada. Can. J. For. Res. 39, 666–680. doi: 10.1139/x08-209
Velthof, G. L., Jarvis, S. C., Stein, A., Allen, A. G., and Oenema, O. (1996). Spatial variability of nitrous oxide fluxes in mown and grazed grasslands on a poorly drained clay soil. Soil Biol. Biochem. 28, 1215–1225. doi: 10.1016/0038-0717(96)00129-0
Weslien, P., Kasimir Klemedtsson, Å, Börjesson, G., and Klemedtsson, L. (2009). Strong pH influence on N2O and CH4 fluxes from forested organic soils. Eur. J. Soil Sci. 60, 311–320. doi: 10.1111/j.1365-2389.2009.01123.x
Keywords: forest fertilization, nitrous oxide emissions, boreal forest soil, Norway spruce, carbon-to-nitrogen ratio
Citation: Rütting T, Björsne A-K, Weslien P, Kasimir Å and Klemedtsson L (2021) Low Nitrous Oxide Emissions in a Boreal Spruce Forest Soil, Despite Long-Term Fertilization. Front. For. Glob. Change 4:710574. doi: 10.3389/ffgc.2021.710574
Received: 16 May 2021; Accepted: 26 July 2021;
Published: 26 August 2021.
Edited by:
Junbin Zhao, Norwegian Institute of Bioeconomy Research (NIBIO), NorwayReviewed by:
Brian D. Strahm, Virginia Tech, United StatesHaofei Yu, University of Central Florida, United States
Copyright © 2021 Rütting, Björsne, Weslien, Kasimir and Klemedtsson. This is an open-access article distributed under the terms of the Creative Commons Attribution License (CC BY). The use, distribution or reproduction in other forums is permitted, provided the original author(s) and the copyright owner(s) are credited and that the original publication in this journal is cited, in accordance with accepted academic practice. No use, distribution or reproduction is permitted which does not comply with these terms.
*Correspondence: Tobias Rütting, Tobias.rutting@gu.se