Lifespan Intellectual Factors, Genetic Susceptibility, and Cognitive Phenotypes in Aging: Implications for Interventions
- 1Department of Neurology, Shandong Provincial Hospital Affiliated to Shandong University, Jinan, China
- 2Center on Aging Psychology, Institute of Psychology, Chinese Academy of Sciences, Beijing, China
- 3Department of Psychology, University of Chinese Academy of Sciences, Beijing, China
- 4Department of Neurobiology, Care Sciences and Society, Aging Research Center and Center for Alzheimer’s Research, Karolinska Institutet-Stockholm University, Stockholm, Sweden
Along with rapid global population aging, the age-related cognitive disorders such as mild cognitive impairment (MCI) and dementia have posed a serious threat to public health, health care system, and sustainable economic and societal development of all countries. In this narrative review, we seek to summarize the major epidemiological studies from the life-course perspective that investigate the influence of genetic susceptibility [e.g., apolipoprotein (APOE) ε4 allele] and intellectual or psychosocial factors (e.g., educational attainments and leisure activities) as well as their interactions on cognitive phenotypes in aging. Numerous population-based studies have suggested that early-life educational attainments and socioeconomic status, midlife work complexity and social engagements, late-life leisure activities (social, physical, and mentally-stimulating activities), certain personality traits (e.g., high neuroticism and low conscientiousness), and depression significantly affect late-life cognitive phenotypes. Furthermore, certain intellectual or psychosocial factors (e.g., leisure activities and depression) may interact with genetic susceptibility (e.g., APOE ε4 allele) to affect the phenotypes of cognitive aging such that risk or beneficial effects of these factors on cognitive function may vary by carrying the susceptibility genes. Current evidence from the randomized controlled trials that support the cognitive benefits of cognitive training among cognitive healthy older adults remains limited. The cognitive reserve hypothesis has been proposed to partly explain the beneficial effects of lifetime intellectual and psychosocial factors on late-life cognitive function. This implies that, from a life-course perspective, preventive intervention strategies targeting multiple modifiable intellectual and psychosocial factors could interfere with clinical expression of cognitive disorders in old age and delay the onset of dementia syndrome, and thus, may help achieve healthy brain aging.
Introduction
Cognitive aging can be referred to as a process of gradual deteriorations in cognitive function that occur as people age. Phenotypes of cognitive aging as a continuum range from normal age-related cognitive decline through mild cognitive impairment (MCI) to a full stage of dementia; Alzheimer’s disease is the most common form of dementia among older people. The global aging population has increased steadily, especially in low- and middle-income countries such as China and India (Chatterji et al., 2008; Zhang et al., 2012). As a result, the aging-related cognitive disorders such as MCI and dementia have posed tremendous challenges not only for current health care and social welfare system but also for sustainable socioeconomic development of all societies (Wimo et al., 2017; Xu et al., 2017). Dementia, as the most common dementing disorder in older people, is defined as a clinical syndrome characterized by a progressive deterioration in multiple cognitive domains (e.g., memory, attention, executive, and verbal fluency) that are severe enough to interfere with the functioning of daily life. MCI and dementia are the principal causes of functional dependence, poor quality of life, institutionalization, and mortality among older people. Thus, the accelerated cognitive deterioration in aging is the major hamper to achieve successful aging and longevity (Depp et al., 2012). The overall prevalence of dementia among people aged 60 years or older is estimated to be about 6%–8%, and after 60 years of age, the likelihood of dementia occurrence almost doubles every 5–7 years (Prince et al., 2013; Winblad et al., 2016). It was estimated that in 2015, worldwide nearly 47 million people were living with dementia, and the number was projected to reach ~74.7 million by 2030 and ~131.5 million by 2050, with the global costs of dementia in 2015 being estimated at ~$818 billion (Wimo et al., 2017). MCI is even more prevalent than dementia, with the prevalence ranging from 10% to 20% (Petersen et al., 2010; Nie et al., 2011). Since the 1980s, numerous community-based cohort studies of older people (e.g., age ≥60 years) focusing on cognitive aging and dementia have been implemented across the world, especially in Europe and North America (Stanziano et al., 2010). In addition, several observational studies that were initiated among young and middle-aged people in the 1960s and the 1970s have shifted their initial focus from metabolic and cardiovascular disease (e.g., obesity, hypertension, diabetes, and ischemic heart disease) to phenotypes of cognitive aging (e.g., MCI and dementia) as the study cohorts age (Irie et al., 2008; Håkansson et al., 2009; Chang et al., 2010). These long-term observational studies have significantly contributed to the better understanding of multifactorial etiology and the process of cognitive aging and dementia from the life-course perspective (Whalley et al., 2006; Fratiglioni and Qiu, 2011; Qiu, 2012). Whereas it has now been well-established that dementia and cardiovascular disease share common cardiometabolic risk factors from midlife onward, increasing evidence supports the potential role of the life-course intellectual factors (e.g., education, work complexity, and mental activity) in delaying the onset of cognitive disorders (e.g., dementia). In this brief narrative review, we sought to summarize evidence from the systematic reviews and the major up-to-date epidemiological studies concerning the effects of genetic susceptibility, intellectual factors over the lifespan, and their interactions on cognitive phenotypes in old age (e.g., cognitive decline, MCI, and dementia) as well as potential implications for interventions.
The Life-Course Approach in Cognitive Aging
The life-course approach considers biological, physical, and psychosocial environmental factors acting over the lifespan (e.g., gestation, early childhood, adolescence, young adulthood, midlife, and older age) to be relevant for determining the risk of chronic diseases (e.g., cancer, cardiovascular disease, and dementia) occurring later in life (Ben-Shlomo and Kuh, 2002). This approach seeks to identify specific time-windows over the lifespan when exposures have the greatest effect on health outcomes and to determine whether accumulative exposures over the lifespan have interactive (e.g., synergistic, additive or multiplicative) effects on health outcomes in late life (Whalley et al., 2006; Richards and Hatch, 2011). Thus, from the life-course perspective, the late-life cognitive phenotypes are not determined by exposures in any single time-period over the lifespan; rather, they reflect the result of complex interactions of genetic susceptibility, biological factors, and psychosocial environments experienced over the whole life. For instance, the cognitive reserve hypothesis, which is proposed to interpret the disparities between clinical phenotypes of cognitive aging and the load of neuropathologies in the brain (Stern, 2012), can be conceived as the sum of lifetime input of cognitive reserve or intellectual factors (Richards and Deary, 2005; Dekhtyar et al., 2015, 2016). As an example in understanding the etiology of a chronic disease from a life-course perspective, evidence has emerged that late-life risk of dementia is determined by genetic susceptibility and life-long exposures to non-genetic physical, biological, and psychosocial environmental factors as well as their interactions (Qiu, 2012). Furthermore, the life-course model introduces the concept of time window at exposure that is highly relevant for studying the etiology or determinants of chronic diseases with a long-term latent period such as dementia. A factor that increases the risk of a chronic disease if the exposure occurs in a certain time-period may show a differential effect if it occurs in another time-period over the lifespan, owing to various psychosocial and biological mechanisms, interactions with other factors, or selective survival. For instance, using the life-course approach, systematic reviews have identified specific time-windows for certain cardiometabolic risk factors (e.g., high blood pressure, obesity, and high cholesterol) that act as risk factors for late-life cognitive impairment and dementia, mainly when they occur in young adulthood and middle age, but not necessarily in late-life (Qiu et al., 2005; Qiu and Fratiglioni, 2015; Irwin et al., 2018). In this context, it is important to keep the life-course perspective in mind when designing intervention programs to delay clinical expression of cognitive disorders in old age.
Notably, the meta-analysis of population-based neuropathological imaging studies has revealed a 20- to 30-year interval between the first deposit of hallmark Alzheimer pathology in the brain (i.e., amyloid) and the onset of the first clinical symptoms of dementia (Jansen et al., 2015). This implies that owing to the relatively long-term latent (pre-clinical) period of dementia, the potential reverse causality should be kept in mind when interpreting the associations between exposures to environmental factors and the risk of dementia even from the prospective cohort studies.
Genetic Susceptibility
Mutations in amyloid precursor protein, presenilin-1, and presenilin-2 genes cause early-onset familial Alzheimer’s disease, but such cases only account for <3% of all Alzheimer cases (Qiu et al., 2009; Ballard et al., 2011). Late-onset sporadic Alzheimer’s disease accounts for the large majority of Alzheimer cases, which is determined by genetic and environmental factors as well as their interactions over the life-course.
Apolipoprotein E (APOE) ε4 allele is so far the only established genetic factor for sporadic Alzheimer’s disease. The onset age of Alzheimer’s disease is decreased by about 3–4 years for people who carry every APOE ε4 allele (Sando et al., 2008). The risk of Alzheimer’s disease increases with increasing number of the ε4 allele (Qiu et al., 2009), although the risky effect of APOE ε4 allele tends to decrease with advancing age. It is estimated that around 15%–20% of Alzheimer cases in the general elderly population are attributable to carrying the APOE ε4 allele. However, evidence supporting the association of APOE ε4 allele with MCI or cognitive impairment no dementia remains mixed. A meta-analysis suggests that APOE ε4 allele does affect cognitive function in normal aging, but the influence is relatively small and is specific to certain cognitive domains such as episodic memory and executive function (Small et al., 2004). Indeed, data from the Swedish Kungsholmen Project suggested that APOE ε4 allele was associated with global cognitive decline with subsequent progression to dementia, whereas the association with cognitive decline without progression to dementia was less evident (Qiu et al., 2006). Thus, APOE ε4 allele is associated primarily with cognitive decline owing to incipient dementia (Hayden et al., 2009; Qiu and Fratiglioni, 2010). This is in line with the meta-analysis suggesting that APOE ε4 allele is associated with a moderately increased risk for progression from MCI to Alzheimer dementia (Elias-Sonnenschein et al., 2011).
In addition, several other candidate genes that are often related to cardiometabolic risk factors have been associated with cognitive phenotypes in aging, such as angiotensin-converting enzyme (ACE) gene, cholesterol 24-hydroxylase gene, fat and obesity-associated FTO gene, and insulin degrading enzyme (IDE) gene (Guerreiro et al., 2012; Reitz et al., 2012; Schrijvers et al., 2012; Zettergren et al., 2017; Haithem et al., 2018). Furthermore, the genome-wide association studies have identified various susceptibility loci or variants that are potentially associated with an elevated risk of Alzheimer’s disease (Harold et al., 2009; Hollingworth et al., 2011; Lambert et al., 2013). However, their associations with late-life cognitive disorders are relatively weak, although a polygenic risk score outside the APOE ε4 locus may help improve risk prediction for MCI and Alzheimer’s disease (Chouraki et al., 2016; Logue et al., 2019).
Intellectual or Psychosocial Factors
Educational Attainments
Since the early 1990s when the Shanghai Aging Study linked illiteracy or low education with late-life sporadic dementia and Alzheimer’s disease (Zhang et al., 1990), numerous epidemiological studies have suggested that early-life low educational attainment is associated with an increased risk of late-life cognitive impairment and dementia, in which the association could not be explained by unhealthy lifestyle and low occupational position associated with low education (Karp et al., 2004; Ngandu et al., 2007; Qiu et al., 2010). The quantitative meta-analyses of prospective cohort studies reported that low education (middle school or below vs. high school or above) was associated with a ~60% increased risk of dementia (Caamaño-Isorna et al., 2006; Norton et al., 2014). A systematic review found that lower education was associated with a greater risk for dementia in many but not all studies and that the effect of early-life educational attainment on dementia risk may be best evaluated within the context of a lifespan developmental model (Sharp and Gatz, 2011). In addition, cognitive ability in childhood, intelligence quotient, and bilingualism also may postpone onset of cognitive impairment and dementia (McGurn et al., 2008; Craik et al., 2010). Furthermore, a higher socioeconomic position over the lifespan was associated with a lower risk of dementia later in life, suggesting that exposures to socioeconomic disadvantage contribute to late-life cognitive phenotypes (Marengoni et al., 2011; Zeki Al Hazzouri et al., 2011). Finally, when early-life educational attainment and occupation-based socioeconomic position were examined simultaneously in association with cognitive impairment and dementia, an independent association existed only with education (Karp et al., 2004), suggesting that education may play a predominant role over occupational position in determining late-life cognitive phenotypes.
Occupational Complexity
Evidence from several population-based studies supports cognitive benefits of occupational complexity. The US Coronary Artery Risk Development in Young Adults study (age 18–30 years) showed that occupational cognitive complexity earlier in adulthood was associated with better white-matter integrity and performance in processing speed and executive function in midlife (Kaup et al., 2018). The Lothian Birth Cohort 1936 study suggested that complexity of work with people and data was associated with better cognitive performance later in life, independent of IQ, education, and social deprivation (Smart et al., 2014). The Swedish Twin Study and the Kungsholmen Project showed that a greater work complexity was associated with a reduced risk of dementia (Andel et al., 2005; Karp et al., 2009). Similarly, using a life-course model of different data sources, additional two Swedish studies suggested that high school performance in childhood and complex occupations in adulthood were both associated with a lower risk of dementia (Dekhtyar et al., 2015, 2016). Finally, the cognitive benefits of more occupational complexity and more leisure activities were also supported even in elderly populations with overall low educational attainments and low socioeconomic position (Darwish et al., 2018). The cognitive reserve hypothesis has been proposed to explain the cognitive benefits of adulthood work complexity (Stern, 2012).
Physical, Social, and Mental Activity
Physical Activity
In 2004, a systematic review found that the majority of longitudinal studies supported an association of physical activity with a reduced risk of dementia (Fratiglioni et al., 2004). Since then, evidence has accumulated to support the potential cognitive benefits of physical activity. Regular physical exercise, even low-intensity activity such as walking, also was associated with a reduced risk of dementia and cognitive decline in older adults (Larson et al., 2006; Tomata et al., 2019). Long-term follow-up studies suggested that physical activity at any time point over the lifespan, especially in early life (e.g., teenagers) and middle age, was associated with a lower likelihood of late-life cognitive impairment and dementia (Andel et al., 2008; Taaffe et al., 2008; Middleton et al., 2010; Zotcheva et al., 2018; Palta et al., 2019). The Rush Memory and Aging Project showed that a higher level of daily physical activity was associated with a lower risk of dementia and global cognitive decline (Buchman et al., 2012). The systematic reviews of prospective studies revealed that physical activity during middle age and later in life might reduce the risk of dementia and cognitive impairment by about 35%–45% (Hamer and Chida, 2009), although the cognitive benefits owing to late-life physical activity may partially reflect a reverse causation (Morgan et al., 2012). A recent systematic review of prospective observational studies found that the majority of studies reported that leisure-time physical activity, but not work-related physical activity, was associated with a reduced risk of Alzheimer’s disease (Stephen et al., 2017). Theoretically, regular physical activity may promote vascular and circulatory health by reducing blood pressure, obesity, and blood glucose, although physical activity often contains components of social and cognitive activities, which may provide cognitive reserve. However, the Whitehall II study showed no evidence for the inverse association between midlife physical activity (age 35–55 years) and late-life risk of cognitive decline and dementia (Sabia et al., 2017). In addition, the Lifestyle Interventions and Independence for Elders Study concluded that a 2-year moderate-intensity physical activity program did not improve global or domain-specific cognitive function (Sink et al., 2015). Finally, the systematic reviews of randomized controlled trials concluded that evidence was largely insufficient that single-component physical activity or aerobic physical activity could prevent cognitive decline or dementia among cognitively healthy older adults (Young et al., 2015), and that multidomain interventions might delay cognitive decline (Brasure et al., 2018).
Social Engagement and Social Network
The systematic review of longitudinal studies in 2004 found evidence suggesting that social disengagement, a poor social network, and social isolation later in life are associated with an elevated risk of dementia (Fratiglioni et al., 2004). Similarly, the updated systematic reviews of longitudinal studies concluded that social relationship factors that represented a lack of social interactions were associated with an increased risk of dementia, in which the strength of the associations was comparable with other well-established risk factors for dementia, such as low educational attainment, physical inactivity, and late-life depression (Kuiper et al., 2015). Additionally, the French PAQUID cohort study showed that late-life social engagement was independently associated with a reduced risk of dementia (Marioni et al., 2015). Similarly, in the Honolulu-Asia Aging Study, low social engagement in late-life and a decline in social engagement from middle age to late-life were associated with a two-fold increased risk of dementia (Saczynski et al., 2006); the association was supported by systematic reviews (Penninkilampi et al., 2018). The Finnish CAIDE study found that being widowed from midlife onwards was associated with a substantial risk of dementia, suggesting that living with a partner might imply cognitive and social challenges that potentially protected against late-life dementia and cognitive decline (Håkansson et al., 2009). Finally, the US Health and Retirement Study suggested that late-life loneliness was associated with a 40% increased, whereas purpose in life was associated with a 30% decreased, risk of dementia independent of social isolation and other genetic and environmental factors (Sutin et al., 2018a,b). Taken together, cumulative evidence supports that more socially active older adults experience less cognitive decline and a reduced risk of dementia in late-life (James et al., 2011).
Mentally-Stimulating Activity
In the past decades, numerous population-based cohort studies have frequently reported that a greater engagement in intellectual or mentally-stimulating activities (e.g., learning, reading, handicrafts, doing crossword puzzle or playing games) from young adulthood through midlife and old age is associated with a lower risk of dementia, as summarized and concluded in several systematic reviews (Fratiglioni et al., 2004; Sajeev et al., 2016; Yates et al., 2016). Some studies showed that their association remains even when these activities are assessed more than 5 years prior to diagnosis of dementia (Akbaraly et al., 2009; Marioni et al., 2015), supporting a potential temporal relationship of intellectual activities to cognitive benefits. Cognitive reserve has been proposed to explain the observed cognitive benefits associated with cognitive or mental activities, although neuroimaging studies also suggested that cognitively engaging activities were associated with greater volumes of cortex and subcortex (e.g., brain reserve; Seider et al., 2016). However, the systematic reviews of randomized controlled trials or intervention studies found little or insufficient evidence that the short-term computerized cognitive training (e.g., ≥12 weeks) or even longer cognitive training exercises (≥6 months) could improve cognitive function or delay cognitive decline or onset of dementia among healthy older adults (Lampit et al., 2014; Butler et al., 2018; Gates et al., 2019). This may suggest that cognitive benefits suggested in observational longitudinal studies might partly reflect an effect of reverse causality.
Personality Traits
Personality traits encompass a range of behaviors that can be typically summarized into various patterns or dimensions that are generally stable during adulthood. Different personality traits may be associated with various behavioral and cognitive disorders. A cross-sectional study showed that high agreeableness and openness were correlated with poorer performance in executive function independent of demographics, depression, and cortisol levels (Ouanes et al., 2017). A population-based cohort study of people aged ≥75 years in Stockholm suggested that low neuroticism in combination with high extraversion was associated with the reduced risk of dementia (Wang et al., 2009). A systematic review found that the association of various personality traits with Alzheimer’s disease and dementia was highly consistent across several well-established cohort studies (Terracciano et al., 2014). The population-based surveys and the meta-analysis indicated that certain types of personality traits (e.g., high neuroticism, low conscientiousness, low extraversion, low agreeableness, and low openness) were associated with an increased risk of Alzheimer’s disease and dementia; it was estimated that neuroticism and conscientiousness could account for 13%–11%, respectively, of all Alzheimer cases in the population (Terracciano et al., 2014, 2017b). This suggests that the estimated population attributable fractions for dementia due to these personality traits are generally comparable to those of well-established clinical and lifestyle risk factors such as midlife hypertension, diabetes, and depression (Norton et al., 2014). Finally, personality traits may change along with the development of MCI or dementia such as decreases in conscientiousness and extraversion, and increases in neuroticism (Islam et al., 2019), although a large-scale study showed no evidence for preclinical change in personality prior to the onset of MCI or clinical dementia (Terracciano et al., 2017a). It was proposed that the personality profile for dementia is characterized by high neuroticism and low openness and extraversion, although the personality traits can also be interpreted as an early indicator of subsequent development of dementia (D’Iorio et al., 2018; Yoneda et al., 2018).
Depression or Depressive Symptomatology
The relationship of depression and depressive symptomatology with dementia has been investigated in numerous studies from a life-course perspective, as previously reviewed (Byers and Yaffe, 2011). Several population-based cohort studies have reported an elevated risk of dementia and cognitive impairment associated with a history of depression (Dotson et al., 2010; Ritchie et al., 2010; Lenoir et al., 2011). A Swedish study of middle-aged women found that self-reported stress in midlife was associated with an increased risk of dementia developed two decades later (Johansson et al., 2010). The meta-analysis of prospective cohort studies reported a double-increased risk for dementia associated with depression (Ownby et al., 2006; Cherbuin et al., 2015), where an increased interval between diagnoses of depression and onset of dementia was associated with an increased risk of subsequent dementia, supporting the notion that depression may be a risk factor for dementia. Although long-term follow-up studies also support a temporal relationship of depression to subsequent cognitive outcomes, it remains debatable regarding whether late-life depression or depressive symptomatology is a preclinical symptom or a causal risk factor for dementia and cognitive impairment (Saczynski et al., 2010; Li et al., 2011). Taken together, earlier-life depression or high depressive symptomatology has often been linked with an over two-fold increased risk of dementia, whereas most population-based cohort studies of late-life depression and dementia risk have supported an association, yet the temporal nature of their association (i.e., whether depression or high depressive symptomatology represents a prodrome or consequence of or a risk factor for dementia) remains to be clarified.
Lifespan Cumulative Effects of Multiple Intellectual Factors
Evidence from multidisciplinary research has been accumulating that various intellectual or psychosocial factors over the lifespan such as early-life educational attainment and socioeconomic position, midlife greater work complexity, certain personality traits, and more leisure activities in late-life are associated with a reduced risk of cognitive impairment and dementia later in life. Lifespan various intellectual or psychosocial factors are often correlated and their effects may be cumulative. Indeed, several population-based studies using the life-course approaches suggested that cumulative exposures to or composite measures of various cognitive reserve-enhancing factors over the lifespan (e.g., early-life education, midlife work complexity, and late-life leisure activities) were associated with higher cognitive function and a reduced risk of dementia in late-life (González et al., 2013; Dekhtyar et al., 2015, 2016; Wang et al., 2017). The UK Medical Research Council Cognitive Function and Ageing Study suggested that an active cognitive lifestyle (e.g., high education, occupational complexity, and frequent social engagement) in either middle age or late-life was associated with a more favorable cognitive trajectory and a decreased risk of dementia (Valenzuela et al., 2011; Marioni et al., 2012). In addition, the Kungsholmen Project showed that leisure activities containing more than one of the three components (i.e., physical, social, and mental components) seemed to be more beneficial in reducing dementia risk than to be engaged in only one type of activity, suggesting a cumulative effect of multiple activities (Karp et al., 2006). Finally, cognitive reserve capacity could partly mediate the association of leisure-time social, mental, and physical activities with late-life cognitive function (Clare et al., 2017). Notably, we cannot rule out the potential that the observed associations from most of the follow-up studies might be partially affected by unmeasured potential confounders or by reverse causality owing to the long-term latent period (around 20–30 years) of dementia (Jansen et al., 2015; Sajeev et al., 2016).
Interactions of Genetic Susceptibility With Intellectual Factors
Evidence from epidemiological studies suggested that certain intellectual or psychosocial factors may interact with genetic susceptibility (e.g., APOE ε4 allele) to affect late-life cognitive phenotypes, i.e., the effects of these factors on cognitive phenotypes in aging may vary by genetic susceptibility. For example, several studies have shown that APOE ε4 allele may magnify the risk of dementia and cognitive impairment associated with midlife psychosocial factors such as physical inactivity and cognitive inactivity, such that people with these factors are more vulnerable to dementia and cognitive decline when carrying the ε4 allele (Andel et al., 2008; Carlson et al., 2008; Kivipelto et al., 2008). On the other hand, intellectual or psychosocial factors such as high educational attainment in early life and leisure-time active engagement in physical, social, and mental-stimulating activities later in life could diminish the increased risk of dementia owing to the APOE ε4 allele, even among very old people (Ferrari et al., 2013). In addition, the Singapore Longitudinal Aging Study showed that the association of more leisure activities with a reduced risk of cognitive decline was stronger among carriers of the APOE ε4 allele than non-carriers (Niti et al., 2008). Also, population-based prospective studies showed that cognitive activity and regular physical activity at middle age were associated with a lower likelihood of dementia and poor cognitive performance in later life, in which such protective effect was stronger among carriers than non-carriers of the APOE ε4 allele (Carlson et al., 2008; Chang et al., 2010). Furthermore, several population-based studies have suggested that APOE ε4 allele may modify the association of depression with risk of dementia and cognitive decline, such that people with both depression and the ε4 allele have a markedly increased risk of cognitive decline and dementia (Irie et al., 2008; Niti et al., 2009; Köhler et al., 2010; Kim et al., 2011; Pink et al., 2015). The Finnish CAIDE study of middle-aged cohort revealed that being widowed or divorced from midlife onwards could interact with the APOE ε4 allele to dramatically increase the risk of late-life cognitive impairment and dementia (Håkansson et al., 2009). These studies imply that certain psychosocial factors could interact with genetic susceptibility to affect late-life cognitive phenotypes.
In addition, certain vulnerability personality traits may act interactively with genetic or environmental factors to magnify the deleterious effects of cognitive function in older adults. Data from the Ginkgo Evaluation of Memory study showed evidence that extraversion and neuroticism could potentially modify the association of the APOE ε4 allele with cognitive decline and Alzheimer’s disease, such that the associations of high neuroticism with faster cognitive decline and an increased risk of Alzheimer’s disease were evident only among the ε4 allele carriers and that higher extraversion magnified the deleterious effect of the APOE ε4 allele on cognitive function and the risk of Alzheimer’s disease (Dar-Nimrod et al., 2012). The Victoria Longitudinal Study revealed that the effects of certain personality traits (e.g., neuroticism, extraversion, and openness) on declarative memory were moderated by APOE ε4 allele such that the ε4 allele could magnify the association of lower openness with worse performance in episodic and semantic memory (Sapkota et al., 2016). Similarly, data from this project also demonstrated that the cognitive benefits of complex cognitive activities were greater in non-carriers than carriers of the APOE ε4 allele (Runge et al., 2014). Notably, the Baltimore Longitudinal Study of Aging detected interactions of APOE ε4 allele with openness and agreeableness on the risk of Alzheimer’s disease, in which high openness was protective among the ε4 allele carriers, whereas high agreeableness was protective among non-carriers of the ε4 allele (Terracciano et al., 2014). These studies suggest that the interactions between APOE genotype and personality on cognitive aging may vary by traits and across cohorts. Finally, a follow-up study of middle-aged and older adults (age ≥50 years) suggested that a greater polygenic risk score for Alzheimer’s disease was associated with faster cognitive decline partially via higher neuroticism and lower conscientiousness (Stephan et al., 2018). This suggests that the genetic vulnerability for Alzheimer’s dementia may contribute to cognitive phenotypes in part through its association with personality traits. Exploring the interaction of personality traits with genetic susceptibility may help identify older adults with certain characters who are at a substantial risk of accelerated cognitive decline and dementia for early interventions.
Biological Plausibility: Cognitive Reserve
Intellectual or psychosocial factors may influence cognitive phenotypes in aging through cognitive reserve and its interplay with overall load of brain pathologies (e.g., microvascular and degenerative pathologies), in which genetic susceptibility may play a part (Figure 1). The concept of cognitive reserve has been proposed to explain the diversities between pathological burden of the brain and clinical phenotypes of cognitive aging (Stern, 2012). More specifically, people with higher cognitive reserve (e.g., higher educational attainments, greater work complexity, and more frequent participation in mental activity) are able to tolerate more pathology in the brain than those with lower reserve prior to expressing cognitive symptoms. This hypothesis is supported by neuroimaging studies, in which the effect of cognitive reserve on the relationship between brain pathology and cognitive function was investigated in vivo using neuroimaging markers of neurodegeneration (Brayne et al., 2010; Reed et al., 2010; Murray et al., 2011; Vemuri et al., 2011). Similarly, clinicopathological studies also have shown that cognitive function remains higher in people with a heavier burden of brain degenerative pathology if they also have high education or rich social networks (Bennett et al., 2006; Roe et al., 2007) or have a resilient personality profile (e.g., high conscientiousness and low neuroticism personality traits; Terracciano et al., 2013). Epidemiological evidence also supports the role of cognitive reserve in moderating the longitudinal association between late-life social isolation and poor global cognitive function (Evans et al., 2018). These studies imply that, at a given level of clinical severity of dementia or Alzheimer’s disease, the degree of brain pathology will be greater in individuals with higher cognitive reserve than those with lower cognitive reserve. Of note, there is evidence suggesting that genetic factors (e.g., APOE ε4 allele) and cognitive reserve capacity could interplay to affect cognitive phenotypes in old age, even among very old people, possibly through influencing structural and functional brain networks (Ferrari et al., 2013; Pietzuch et al., 2019).
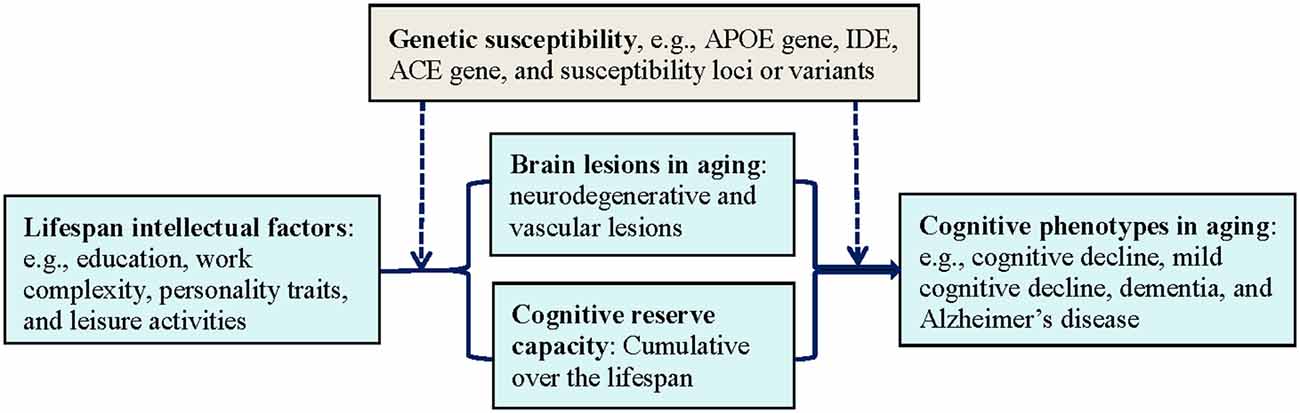
Figure 1. The interplay of lifespan intellectual factors, cognitive reserve capacity, brain pathologies, and genetic susceptibility in cognitive phenotypes of aging. Abbreviations: ACE, angiotensin-converting enzyme gene; APOE, apolipoprotein E gene; IDE, insulin degrading enzyme gene.
The underlying neural mechanisms for cognitive reserve are not fully understood. Neural reserve and neural compensation mechanisms have been proposed for cognitive reserve (Stern, 2012). Neural reserve means that cognitive reserve is associated with individual differences in the resilience of pre-existing cognitive networks, whereas neural compensation refers to the idea that some individuals are better than others in using compensatory mechanisms to maintain cognitive function. The concept of cognitive reserve is useful in the clinical assessment of cognitive phenotypes as well as in preventive interventions to delay clinical expression of cognitive outcomes in aging (Qiu and Fratiglioni, 2018).
Implications for Interventions and Conclusions
Along with rapid global population aging, cognitive impairment and dementia as the major cognitive disorders in aging have posed tremendous economic and societal burden to the modern societies. Epidemiological research has from the life-course perspective provided sufficient evidence suggesting that intellectual factors over the lifespan such as early-life high education, midlife work complexity, and late-life socially-integrative lifestyles (e.g., active engagements in social, physical, and mental activities) may delay the clinical onset of cognitive disorders in aging by providing cognitive reserve, whereas depression or depressive symptoms may confer risk for adverse cognitive outcomes in old age, in which the beneficial or risky effects may vary by genetic susceptibility (e.g., APOE ε4 allele). In this regard, a potential implication of these findings in dementia prevention is to conduct multimodal interventions targeting those intellectual and psychosocial factors together with other modifiable risk factors (e.g., cardiovascular risk factors). However, evidence from randomized controlled trials or intervention studies is still limited. Thus, one of the priorities in future dementia research should be given to testing effects of the multimodal intervention approaches in delaying cognitive decline and the clinical onset of dementia among different populations in the world. The global coordinated effort toward this goal is ongoing, stimulated by the Finnish FINGER multidomain intervention models (see World Wide FINGER Initiative1). This approach may help achieve the overall goal of successful aging, especially with regard to healthy brain aging, and thus, may reduce the huge economic and societal burden of cognitive disorders in our aging society.
Contribution to the Field
As population ages, the age-related cognitive disorders (e.g., MCI or dementia) have posed a serious threat to global public health, health care system, and sustainable economic and societal development. In this narrative review, we summarize the major epidemiological studies from the life-course perspective that investigate the influence of genetic susceptibility (e.g., APOE genotype) and intellectual or psychosocial factors (e.g., education, work complexity, and leisure activities) as well as their interactions on cognitive phenotypes in aging. Population-based studies have suggested that early-life educational attainments, midlife work complexity and social engagements, late-life social, physical, and mental activities, and certain personality traits may affect late-life cognitive phenotypes. Furthermore, certain intellectual or psychosocial factors may interact with genetic susceptibility to affect the phenotypes of cognitive aging such that risk or beneficial effects of these factors on cognitive function may vary by carrying the susceptibility genes. The cognitive reserve hypothesis may partly explain the beneficial effects of lifespan intellectual and psychosocial factors on late-life cognitive function. This implies that, from a life-course perspective, intervention strategies targeting modifiable intellectual or psychosocial factors could interfere with clinical expression of cognitive disorders in old age and delay dementia onset, and thus, may help achieve healthy brain aging and reduce the burden of dementia.
Author Contributions
CQ and YW contributed to conceptualization and structure of the manuscript, reviewed the literature, and wrote the initial version of the manuscript. YD and JL provided critical comments and revisions. All authors have read and approved the final version of the manuscript.
Funding
This work was supported in part by grants from the National R&D Program of the Ministry of Science and Technology of China (grant no.: 2017YFC1310100), the National Nature Science Foundation of China (grants no.: 31711530157 and 8171101298), and the Taishan Scholar Program of Shandong Province, China and by grants from the Swedish Research Council (Vetenskapsrådet; grant nos.: 2017-00740 and 2017-05819) and the Swedish Council for Working Life and Social Research (grant no.: 2014-01382), Stockholm, Sweden. The funding agency had no role in the writing of this manuscript and in the decision to submit the work for publication.
Conflict of Interest Statement
The authors declare that the research was conducted in the absence of any commercial or financial relationships that could be construed as a potential conflict of interest.
Footnotes
References
Akbaraly, T. N., Portet, F., Fustinoni, S., Dartigues, J. F., Artero, S., Rouaud, O., et al. (2009). Leisure activities and the risk of dementia in the elderly: results from the Three-City Study. Neurology 73, 854–861. doi: 10.1212/WNL.0b013e3181b7849b
Andel, R., Crowe, M., Pedersen, N. L., Fratiglioni, L., Johansson, B., and Gatz, M. (2008). Physical exercise at midlife and risk of dementia three decades later: a population-based study of Swedish twins. J. Gerontol. A Biol. Sci. Med. Sci. 63, 62–66. doi: 10.1093/gerona/63.1.62
Andel, R., Crowe, M., Pedersen, N. L., Mortimer, J., Crimmins, E., Johansson, B., et al. (2005). Complexity of work and risk of Alzheimer’s disease: a population-based study of Swedish twins. J. Gerontol. B Psychol. Sci. Soc. Sci. 60, P251–P258. doi: 10.1093/geronb/60.5.p251
Ballard, C., Gauthier, S., Corbett, A., Brayne, C., Aarsland, D., and Jones, E. (2011). Alzheimer’s disease. Lancet 377, 1019–1031. doi: 10.1016/S0140-6736(10)61349-9
Bennett, D. A., Schneider, J. A., Tang, Y., Arnold, S. E., and Wilson, R. S. (2006). The effect of social networks on the relation between Alzheimer’s disease pathology and level of cognitive function in old people: a longitudinal cohort study. Lancet Neurol. 5, 406–412. doi: 10.1016/S1474-4422(06)70417-3
Ben-Shlomo, Y., and Kuh, D. (2002). A life course approach to chronic disease epidemiology: conceptual models, empirical challenges and interdisciplinary perspectives. Int. J. Epidemiol. 31, 285–293. doi: 10.1093/intjepid/31.2.285
Brasure, M., Desai, P., Davila, H., Nelson, V. A., Calvert, C., Jutkowitz, E., et al. (2018). Physical activity interventions in preventing cognitive decline and Alzheimer-type dementia: a systematic review. Ann. Intern. Med. 168, 30–38. doi: 10.7326/m17-1528
Brayne, C., Ince, P. G., Keage, H. A., McKeith, I. G., Matthews, F. E., Polvikoski, T., et al. (2010). Education, the brain and dementia: neuroprotection or compensation? Brain 133, 2210–2216. doi: 10.1093/brain/awq185
Buchman, A. S., Boyle, P. A., Yu, L., Shah, R. C., Wilson, R. S., and Bennett, D. A. (2012). Total daily physical activity and the risk of AD and cognitive decline in older adults. Neurology 78, 1323–1329. doi: 10.1212/wnl.0b013e3182535d35
Butler, M., McCreedy, E., Nelson, V. A., Desai, P., Ratner, E., Fink, H. A., et al. (2018). Does cognitive training prevent cognitive decline?: a systematic review. Ann. Intern. Med. 168, 63–68. doi: 10.7326/m17-1531
Byers, A. L., and Yaffe, K. (2011). Depression and risk of developing dementia. Nat. Rev. Neurol. 7, 323–331. doi: 10.1038/nrneurol.2011.60
Caamaño-Isorna, F., Corral, M., Montes-Martínez, A., and Takkouche, B. (2006). Education and dementia: a meta-analytic study. Neuroepidemiology 26, 226–232. doi: 10.1159/000093378
Carlson, M. C., Helms, M. J., Steffens, D. C., Burke, J. R., Potter, G. G., and Plassman, B. L. (2008). Midlife activity predicts risk of dementia in older male twin pairs. Alzheimers Dement. 4, 324–331. doi: 10.1016/j.jalz.2008.07.002
Chang, M., Jonsson, P. V., Snaedal, J., Bjornsson, S., Saczynski, J. S., Aspelund, T., et al. (2010). The effect of midlife physical activity on cognitive function among older adults: AGES—reykjavik study. J. Gerontol. A Biol. Sci. Med. Sci. 65, 1369–1374. doi: 10.1093/gerona/glq152
Chatterji, S., Kowal, P., Mathers, C., Naidoo, N., Verdes, E., Smith, J. P., et al. (2008). The health of aging populations in China and India. Health Aff. 27, 1052–1063. doi: 10.1377/hlthaff.27.4.1052
Cherbuin, N., Kim, S., and Anstey, K. J. (2015). Dementia risk estimates associated with measures of depression: a systematic review and meta-analysis. BMJ Open 5:e008853. doi: 10.1136/bmjopen-2015-008853
Chouraki, V., Reitz, C., Maury, F., Bis, J. C., Bellenguez, C., Yu, L., et al. (2016). Evaluation of a genetic risk score to improve risk prediction for Alzheimer’s disease. J. Alzheimers Dis. 53, 921–932. doi: 10.3233/JAD-150749
Clare, L., Wu, Y. T., Teale, J. C., MacLeod, C., Matthews, F., Brayne, C., et al. (2017). Potentially modifiable lifestyle factors, cognitive reserve, and cognitive function in later life: a cross-sectional study. PLoS Med. 14:e1002259. doi: 10.1371/journal.pmed.1002259
Craik, F. I., Bialystok, E., and Freedman, M. (2010). Delaying the onset of Alzheimer disease: bilingualism as a form of cognitive reserve. Neurology 75, 1726–1729. doi: 10.1212/wnl.0b013e3181fc2a1c
Dar-Nimrod, I., Chapman, B. P., Franks, P., Robbins, J., Porsteinsson, A., Mapstone, M., et al. (2012). Personality factors moderate the associations between apolipoprotein genotype and cognitive function as well as late onset alzheimer disease. Am. J. Geriatr. Psychiatry 20, 1026–1035. doi: 10.1097/jgp.0b013e318267016b
Darwish, H., Farran, N., Assaad, S., and Chaaya, M. (2018). Cognitive reserve factors in a developing country: education and occupational attainment lower the risk of dementia in a sample of Lebanese older adults. Front. Aging Neurosci. 10:277. doi: 10.3389/fnagi.2018.00277
Dekhtyar, S., Wang, H. X., Fratiglioni, L., and Herlitz, A. (2016). Childhood school performance, education and occupational complexity: a life-course study of dementia in the Kungsholmen Project. Int. J. Epidemiol. 45, 1207–1215. doi: 10.1093/ije/dyw008
Dekhtyar, S., Wang, H. X., Scott, K., Goodman, A., Koupil, I., and Herlitz, A. (2015). A life-course study of cognitive reserve in dementia–from childhood to old age. Am. J. Geriatr. Psychiatry 23, 885–896. doi: 10.1016/j.jagp.2015.02.002
Depp, C. A., Harmell, A., and Vahia, I. V. (2012). Successful cognitive aging. Curr. Top. Behav. Neurosci. 10, 35–50. doi: 10.1007/7854_2011_158
D’Iorio, A., Garramone, F., Piscopo, F., Baiano, C., Raimo, S., and Santangelo, G. (2018). Meta-analysis of personality traits in Alzheimer’s disease: a comparison with healthy subjects. J. Alzheimers Dis. 62, 773–787. doi: 10.3233/jad-170901
Dotson, V. M., Beydoun, M. A., and Zonderman, A. B. (2010). Recurrent depressive symptoms and the incidence of dementia and mild cognitive impairment. Neurology 75, 27–34. doi: 10.1212/wnl.0b013e3181e62124
Elias-Sonnenschein, L. S., Viechtbauer, W., Ramakers, I. H., Verhey, F. R., and Visser, P. J. (2011). Predictive value of APOE-ε4 allele for progression from MCI to AD-type dementia: a meta-analysis. J. Neurol. Neurosurg. Psychiatry 82, 1149–1156. doi: 10.1136/jnnp.2010.231555
Evans, I. E. M., Llewellyn, D. J., Matthews, F. E., Woods, R. T., Brayne, C., Clare, L., et al. (2018). Social isolation, cognitive reserve, and cognition in healthy older people. PLoS One 13:e0201008. doi: 10.1371/journal.pone.0201008
Ferrari, C., Xu, W. L., Wang, H. X., Winblad, B., Sorbi, S., Qiu, C., et al. (2013). How can elderly apolipoprotein E ε4 carriers remain free from dementia? Neurobiol. Aging 34, 13–21. doi: 10.1016/j.neurobiolaging.2012.03.003
Fratiglioni, L., Paillard-Borg, S., and Winblad, B. (2004). An active and socially integrated lifestyle in late life might protect against dementia. Lancet Neurol. 3, 343–353. doi: 10.1016/s1474-4422(04)00767-7
Fratiglioni, L., and Qiu, C. (2011). Possibilities of prevention in cognitive ageing: dementia as target, delayed onset as goal. Lancet Neurol. 10, 778–779. doi: 10.1016/s1474-4422(11)70145-4
Gates, N. J., Rutjes, A. W., Di Nisio, M., Karim, S., Chong, L. Y., March, E., et al. (2019). Computerised cognitive training for maintaining cognitive function in cognitively healthy people in late life. Cochrane Database Syst. Rev. 3:CD012277. doi: 10.1002/14651858.cd012277.pub2
González, H. M., Tarraf, W., Bowen, M. E., Johnson-Jennings, M. D., and Fisher, G. G. (2013). What do parents have to do with my cognitive reserve? Life course perspectives on twelve-year cognitive decline. Neuroepidemiology 41, 101–109. doi: 10.1159/000350723
Guerreiro, R. J., Gustafson, D. R., and Hardy, J. (2012). The genetic architecture of Alzheimer’s disease: beyond APP, PSENs and APOE. Neurobiol. Aging 33, 437–456. doi: 10.1016/j.neurobiolaging.2010.03.025
Haithem, H., Ons, A., Salma, N., Jihène, R., Mariam, A., Mariem, M., et al. (2018). Association between dementia and vascular disease-associated polymorphisms in a Tunisian population. Int. J. Neurosci. 128, 32–41. doi: 10.1080/00207454.2017.1348353
Håkansson, K., Rovio, S., Helkala, E. L., Vilska, A. R., Winblad, B., Soininen, H., et al. (2009). Association between mid-life marital status and cognitive function in later life: population based cohort study. BMJ 339:b2462. doi: 10.1136/bmj.b2462
Hamer, M., and Chida, Y. (2009). Physical activity and risk of neurodegenerative disease: a systematic review of prospective evidence. Psychol. Med. 39, 3–11. doi: 10.1017/s0033291708003681
Harold, D., Abraham, R., Hollingworth, P., Sims, R., Gerrish, A., Hamshere, M. L., et al. (2009). Genome-wide association study identifies variants at CLU and PICALM associated with Alzheimer’s disease. Nat. Genet. 41, 1088–1093. doi: 10.1038/ng.440
Hayden, K. M., Zandi, P. P., West, N. A., Tschanz, J. T., Norton, M. C., Corcoran, C., et al. (2009). Effects of family history and apolipoprotein E ε4 status on cognitive decline in the absence of Alzheimer dementia: the Cache County Study. Arch. Neurol. 66, 1378–1383. doi: 10.1001/archneurol.2009.237
Hollingworth, P., Harold, D., Sims, R., Gerrish, A., Lambert, J. C., Carrasquillo, M. M., et al. (2011). Common variants at ABCA7, MS4A6A/MS4A4E, EPHA1, CD33 and CD2AP are associated with Alzheimer’s disease. Nat. Genet. 43, 429–435. doi: 10.1038/ng.803
Irie, F., Masaki, K. H., Petrovitch, H., Abbott, R. D., Ross, G. W., Taaffe, D. R., et al. (2008). Apolipoprotein E ε4 allele genotype and the effect of depressive symptoms on the risk of dementia in men: the Honolulu-Asia Aging Study. Arch. Gen. Psychiatry 65, 906–912. doi: 10.1001/archpsyc.65.8.906
Irwin, K., Sexton, C., Daniel, T., Lawlor, B., and Naci, L. (2018). Healthy aging and dementia: two roads diverging in midlife? Front. Aging Neurosci. 10:275. doi: 10.3389/fnagi.2018.00275
Islam, M., Mazumder, M., Schwabe-Warf, D., Stephan, Y., Sutin, A. R., and Terracciano, A. (2019). Personality changes with dementia from the informant perspective: new data and meta-analysis. J. Am. Med. Dir. Assoc. 20, 131–137. doi: 10.1016/j.jamda.2018.11.004
James, B. D., Wilson, R. S., Barnes, L. L., and Bennett, D. A. (2011). Late-life social activity and cognitive decline in old age. J. Int. Neuropsychol. Soc. 17, 998–1005. doi: 10.1017/s1355617711000531
Jansen, W. J., Ossenkoppele, R., Knol, D. L., Tijms, B. M., Scheltens, P., Verhey, F. R., et al. (2015). Prevalence of cerebral amyloid pathology in persons without dementia: a meta-analysis. JAMA 313, 1924–1938. doi: 10.1001/jama.2015.4668
Johansson, L., Guo, X., Waern, M., Ostling, S., Gustafson, D., Bengtsson, C., et al. (2010). Midlife psychological stress and risk of dementia: a 35-year longitudinal population study. Brain 133, 2217–2224. doi: 10.1093/brain/awq116
Karp, A., Andel, R., Parker, M. G., Wang, H.-X., Winblad, B., and Fratiglioni, L. (2009). Mentally stimulating activities at work during midlife and dementia risk after age 75: follow-up study from the Kungsholmen Project. Am. J. Geriatr. Psychiatry 17, 227–236. doi: 10.1097/jgp.0b013e318190b691
Karp, A., Kåreholt, I., Qiu, C., Bellander, T., Winblad, B., and Fratiglioni, L. (2004). Relation of education and occupation-based socioeconomic status to incident Alzheimer’s disease. Am. J. Epidemiol. 159, 175–183. doi: 10.1093/aje/kwh018
Karp, A., Paillard-Borg, S., Wang, H. X., Silverstein, M., Winblad, B., and Fratiglioni, L. (2006). Mental, physical and social components in leisure activities equally contribute to decrease dementia risk. Dement. Geriatr. Cogn. Disord. 21, 65–73. doi: 10.1159/000089919
Kaup, A. R., Xia, F., Launer, L. J., Sidney, S., Nasrallah, I., Erus, G., et al. (2018). Occupational cognitive complexity in earlier adulthood is associated with brain structure and cognitive health in midlife: the CARDIA study. Neuropsychology 32, 895–905. doi: 10.1037/neu0000474
Kim, J. M., Stewart, R., Kim, S. Y., Kim, S. W., Bae, Y., Yang, S. J., et al. (2011). Synergistic associations of depression and apolipoprotein E genotype with incidence of dementia. Int. J. Geriatr. Psychiatry 26, 893–898. doi: 10.1002/gps.2621
Kivipelto, M., Rovio, S., Ngandu, T., Kåreholt, I., Eskelinen, M., Winblad, B., et al. (2008). Apolipoprotein E ε4 magnifies lifestyle risks for dementia: a population-based study. J. Cell. Mol. Med. 12, 2762–2771. doi: 10.1111/j.1582-4934.2008.00296.x
Köhler, S., van Boxtel, M. P., van Os, J., Thomas, A. J., O’Brien, J. T., Jolles, J., et al. (2010). Depressive symptoms and cognitive decline in community-dwelling older adults. J. Am. Geriatr. Soc. 58, 873–879. doi: 10.1111/j.1532-5415.2010.02807.x
Kuiper, J. S., Zuidersma, M., Oude Voshaar, R. C., Zuidema, S. U., van den Heuvel, E. R., Stolk, R. P., et al. (2015). Social relationships and risk of dementia: a systematic review and meta-analysis of longitudinal cohort studies. Ageing Res. Rev. 22, 39–57. doi: 10.1016/j.arr.2015.04.006
Lambert, J. C., Ibrahim-Verbaas, C. A., Harold, D., Naj, A. C., Sims, R., Bellenguez, C., et al. (2013). Meta-analysis of 74,046 individuals identifies 11 new susceptibility loci for Alzheimer’s disease. Nat. Genet. 45, 1452–1458. doi: 10.1038/ng.2802
Lampit, A., Hallock, H., and Valenzuela, M. (2014). Computerized cognitive training in cognitively healthy older adults: a systematic review and meta-analysis of effect modifiers. PLoS Med. 11:e1001756. doi: 10.1371/journal.pmed.1001756
Larson, E. B., Wang, L., Bowen, J. D., McCormick, W. C., Teri, L., Crane, P., et al. (2006). Exercise is associated with reduced risk for incident dementia among persons 65 years of age and older. Ann. Intern. Med. 144, 73–81. doi: 10.7326/0003-4819-144-2-200601170-00004
Lenoir, H., Dufouil, C., Auriacombe, S., Lacombe, J. M., Dartigues, J. F., Ritchie, K., et al. (2011). Depression history, depressive symptoms, and incident dementia: the 3C Study. J. Alzheimers Dis. 26, 27–38. doi: 10.3233/JAD-2011-101614
Li, G., Wang, L. Y., Shofer, J. B., Thompson, M. L., Peskind, E. R., McCormick, W., et al. (2011). Temporal relationship between depression and dementia: findings from a large community-based 15-year follow-up study. Arch. Gen. Psychiatry 68, 970–977. doi: 10.1001/archgenpsychiatry.2011.86
Logue, M. W., Panizzon, M. S., Elman, J. A., Gillespie, N. A., Hatton, S. N., Gustavson, D. E., et al. (2019). Use of an Alzheimer’s disease polygenic risk score to identify mild cognitive impairment in adults in their 50s. Mol. Psychiatry 24, 421–430. doi: 10.1038/s41380-018-0030-8
Marengoni, A., Fratiglioni, L., Bandinelli, S., and Ferrucci, L. (2011). Socioeconomic status during lifetime and cognitive impairment no-dementia in late life: the population-based aging in the Chianti area (InCHIANTI) study. J. Alzheimers Dis. 24, 559–568. doi: 10.3233/JAD-2011-101863
Marioni, R. E., Proust-Lima, C., Amieva, H., Brayne, C., Matthews, F. E., Dartigues, J. F., et al. (2015). Social activity, cognitive decline and dementia risk: a 20-year prospective cohort study. BMC Public Health 15:1089. doi: 10.1186/s12889-015-2426-6
Marioni, R. E., van den Hout, A., Valenzuela, M. J., Brayne, C., and Matthews, F. E. (2012). Active cognitive lifestyle associates with cognitive recovery and a reduced risk of cognitive decline. J. Alzheimers Dis. 28, 223–230. doi: 10.3233/jad-2011-110377
McGurn, B., Deary, I. J., and Starr, J. M. (2008). Childhood cognitive ability and risk of late-onset Alzheimer and vascular dementia. Neurology 71, 1051–1056. doi: 10.1212/01.wnl.0000319692.20283.10
Middleton, L. E., Barnes, D. E., Lui, L. Y., and Yaffe, K. (2010). Physical activity over the life course and its association with cognitive performance and impairment in old age. J. Am. Geriatr. Soc. 58, 1322–1326. doi: 10.1111/j.1532-5415.2010.02903.x
Morgan, G. S., Gallacher, J., Bayer, A., Fish, M., Ebrahim, S., and Ben-Shlomo, Y. (2012). Physical activity in middle-age and dementia in later life: findings from a prospective cohort of men in Caerphilly, South Wales and a meta-analysis. J. Alzheimers Dis. 31, 569–580. doi: 10.3233/jad-2012-112171
Murray, A. D., Staff, R. T., McNeil, C. J., Salarirad, S., Ahearn, T. S., Mustafa, N., et al. (2011). The balance between cognitive reserve and brain imaging biomarkers of cerebrovascular and Alzheimer’s diseases. Brain 134, 3687–3696. doi: 10.1093/brain/awr259
Ngandu, T., von Strauss, E., Helkala, E. L., Winblad, B., Nissinen, A., Tuomilehto, J., et al. (2007). Education and dementia: what lies behind the association? Neurology 69, 1442–1450. doi: 10.1212/01.wnl.0000277456.29440.16
Nie, H., Xu, Y., Liu, B., Zhang, Y., Lei, T., Hui, X., et al. (2011). The prevalence of mild cognitive impairment about elderly population in China: a meta-analysis. Int. J. Geriatr. Psychiatry 26, 558–563. doi: 10.1002/gps.2579
Niti, M., Yap, K. B., Kua, E. H., and Ng, T. P. (2009). APOE-ε4, depressive symptoms, and cognitive decline in Chinese older adults: singapore Longitudinal Aging Studies. J. Gerontol. A Biol. Sci. Med. Sci. 64, 306–311. doi: 10.1093/gerona/gln013
Niti, M., Yap, K. B., Kua, E. H., Tan, C. H., and Ng, T. P. (2008). Physical, social and productive leisure activities, cognitive decline and interaction with APOE-ε4 genotype in Chinese older adults. Int. Psychogeriatr. 20, 237–251. doi: 10.1017/s1041610207006655
Norton, S., Matthews, F. E., Barnes, D. E., Yaffe, K., and Brayne, C. (2014). Potential for primary prevention of Alzheimer’s disease: an analysis of population-based data. Lancet Neurol. 13, 788–794. doi: 10.1016/S1474-4422(14)70136-X
Ouanes, S., Castelao, E., von Gunten, A., Vidal, P. M., Preisig, M., and Popp, J. (2017). Personality, cortisol, and cognition in non-demented elderly subjects: results from a population-based study. Front. Aging Neurosci. 9:63. doi: 10.3389/fnagi.2017.00063
Ownby, R. L., Crocco, E., Acevedo, A., John, V., and Loewenstein, D. (2006). Depression and risk for Alzheimer disease: systematic review, meta-analysis, and metaregression analysis. Arch. Gen. Psychiatry 63, 530–538. doi: 10.1001/archpsyc.63.5.530
Palta, P., Sharrett, A. R., Deal, J. A., Evenson, K. R., Gabriel, K. P., Folsom, A. R., et al. (2019). Leisure-time physical activity sustained since midlife and preservation of cognitive function: the Atherosclerosis Risk in Communities study cohort. Alzheimers Dement. 15, 273–281. doi: 10.1016/j.jalz.2018.08.008
Penninkilampi, R., Casey, A. N., Singh, M. F., and Brodaty, H. (2018). The association between social engagement, loneliness and risk of dementia: a systematic review and meta-analysis. J. Alzheimers Dis. 66, 1619–1633. doi: 10.3233/jad-180439
Petersen, R. C., Roberts, R. O., Knopman, D. S., Geda, Y. E., Cha, R. H., Pankratz, V. S., et al. (2010). Prevalence of mild cognitive impairment is higher in men: the Mayo Clinic Study of Aging. Neurology 75, 889–897. doi: 10.1212/WNL.0b013e3181f11d85
Pietzuch, M., King, A. E., Ward, D. D., and Vickers, J. C. (2019). The influence of genetic factors and cognitive reserve on structural and functional resting-state brain networks in aging and Alzheimer’s disease. Front. Aging Neurosci. 11:30. doi: 10.3389/fnagi.2019.00030
Pink, A., Stokin, G. B., Bartley, M. M., Roberts, R. O., Sochor, O., Machulda, M. M., et al. (2015). Neuropsychiatric symptoms, APOE ε4 and the risk of incident dementia: a population-based study. Neurology 84, 935–943. doi: 10.1212/WNL.0000000000001307
Prince, M., Bryce, R., Albanese, E., Wimo, A., Ribeiro, W., and Ferri, C. P. (2013). The global prevalence of dementia: a systematic review and metaanalysis. Alzheimers Dement. 9, 63.e2–75.e2. doi: 10.1016/j.jalz.2012.11.007
Qiu, C. (2012). Preventing Alzheimer’s disease by targeting vascular risk factors: hope and gap. J. Alzheimers Dis. 32, 721–731. doi: 10.3233/JAD-2012-120922
Qiu, C., and Fratiglioni, L. (2010). Apolipoprotein E ε4 status and cognitive decline with and without dementia. Arch. Neurol. 67:1036. doi: 10.1001/archneurol.2010.163
Qiu, C., and Fratiglioni, L. (2015). A major role for cardiovascular burden in age-related cognitive decline. Nat. Rev. Cardiol. 12, 267–277. doi: 10.1038/nrcardio.2014.223
Qiu, C., and Fratiglioni, L. (2018). Aging without dementia is achievable: current evidence from epidemiological research. J. Alzheimers Dis. 62, 933–942. doi: 10.3233/jad-171037
Qiu, C., Kivipelto, M., and von Strauss, E. (2009). Epidemiology of Alzheimer’s disease: occurrence, determinants, and strategies toward intervention. Dialogues Clin. Neurosci. 11, 111–128.
Qiu, C., Winblad, B., and Fratiglioni, L. (2005). The age-dependent relation of blood pressure to cognitive function and dementia. Lancet Neurol. 4, 487–499. doi: 10.1016/s1474-4422(05)70141-1
Qiu, C., Winblad, B., and Fratiglioni, L. (2006). Cerebrovascular disease, APOE ε4 allele and cognitive decline in a cognitively normal population. Neurol. Res. 28, 650–656. doi: 10.1179/016164106X130443
Qiu, C., Xu, W. L., and Fratiglioni, L. (2010). Vascular and psychosocial factors in Alzheimer’s disease: epidemiological evidence towards intervention. J. Alzheimers Dis. 20, 689–697. doi: 10.3233/JAD-2010-091663
Reed, B. R., Mungas, D., Farias, S. T., Harvey, D., Beckett, L., Widaman, K., et al. (2010). Measuring cognitive reserve based on the decomposition of episodic memory variance. Brain 133, 2196–2209. doi: 10.1093/brain/awq154
Reitz, C., Tosto, G., Mayeux, R., and Luchsinger, J. A. (2012). Genetic variants in the Fat and Obesity Associated (FTO) gene and risk of Alzheimer’s disease. PLoS One 7:e50354. doi: 10.1371/journal.pone.0050354
Richards, M., and Deary, I. J. (2005). A life course approach to cognitive reserve: a model for cognitive aging and development? Ann. Neurol. 58, 617–622. doi: 10.1002/ana.20637
Richards, M., and Hatch, S. L. (2011). A life course approach to the development of mental skills. J. Gerontol. B Psychol. Sci. Soc. Sci. 66, i26–i35. doi: 10.1093/geronb/gbr013
Ritchie, K., Carrière, I., Ritchie, C. W., Berr, C., Artero, S., and Ancelin, M. L. (2010). Designing prevention programmes to reduce incidence of dementia: prospective cohort study of modifiable risk factors. BMJ 341:c3885. doi: 10.1136/bmj.c3885
Roe, C. M., Xiong, C., Miller, J. P., and Morris, J. C. (2007). Education and Alzheimer disease without dementia: support for the cognitive reserve hypothesis. Neurology 68, 223–228. doi: 10.1212/01.wnl.0000251303.50459.8a
Runge, S. K., Small, B. J., McFall, G. P., and Dixon, R. A. (2014). APOE moderates the association between lifestyle activities and cognitive performance: evidence of genetic plasticity in aging. J. Int. Neuropsychol. Soc. 20, 478–486. doi: 10.1017/s1355617714000356
Sabia, S., Dugravot, A., Dartigues, J. F., Abell, J., Elbaz, A., Kivimäki, M., et al. (2017). Physical activity, cognitive decline, and risk of dementia: 28 year follow-up of Whitehall II cohort study. BMJ 357:j2709. doi: 10.1136/bmj.j2709
Saczynski, J. S., Beiser, A., Seshadri, S., Auerbach, S., Wolf, P. A., and Au, R. (2010). Depressive symptoms and risk of dementia: the Framingham Heart Study. Neurology 75, 35–41. doi: 10.1212/WNL.0b013e3181e62138
Saczynski, J. S., Pfeifer, L. A., Masaki, K., Korf, E. S., Laurin, D., White, L., et al. (2006). The effect of social engagement on incident dementia: the Honolulu-Asia Aging Study. Am. J. Epidemiol. 163, 433–440. doi: 10.1093/aje/kwj061
Sajeev, G., Weuve, J., Jackson, J. W., VanderWeele, T. J., Bennett, D. A., Grodstein, F., et al. (2016). Late-life cognitive activity and dementia: a systematic review and bias analysis. Epidemiology 27, 732–742. doi: 10.1097/ede.0000000000000513
Sando, S. B., Melquist, S., Cannon, A., Hutton, M. L., Sletvold, O., Saltvedt, I., et al. (2008). APOE ε4 lowers age at onset and is a high risk factor for Alzheimer’s disease; a case control study from central Norway. BMC Neurol. 8:9. doi: 10.1186/1471-2377-8-9
Sapkota, S., Wiebe, S. A., Small, B. J., and Dixon, R. A. (2016). Apolipoprotein E and Clusterin can magnify effects of personality vulnerability on declarative memory performance in non-demented older adults. Int. J. Geriatr. Psychiatry 31, 502–509. doi: 10.1002/gps.4355
Schrijvers, E. M., Schürmann, B., Koudstaal, P. J., van den Bussche, H., Van Duijn, C. M., Hentschel, F., et al. (2012). Genome-wide association study of vascular dementia. Stroke 43, 315–319. doi: 10.1161/STROKEAHA.111.628768
Seider, T. R., Fieo, R. A., O’Shea, A., Porges, E. C., Woods, A. J., and Cohen, R. A. (2016). Cognitively engaging activity is associated with greater cortical and subcortical volumes. Front. Aging Neurosci. 8:94. doi: 10.3389/fnagi.2016.00094
Sharp, E. S., and Gatz, M. (2011). Relationship between education and dementia: an updated systematic review. Alzheimer Dis. Assoc. Disord. 25, 289–304. doi: 10.1097/wad.0b013e318211c83c
Sink, K. M., Espeland, M. A., Castro, C. M., Church, T., Cohen, R., Dodson, J. A., et al. (2015). Effect of a 24-month physical activity intervention vs. health education on cognitive outcomes in sedentary older adults: The LIFE randomized trial. JAMA 314, 781–790. doi: 10.1001/jama.2015.9617
Small, B. J., Rosnick, C. B., Fratiglioni, L., and Bäckman, L. (2004). Apolipoprotein E and cognitive performance: a meta-analysis. Psychol. Aging 19, 592–600. doi: 10.1037/0882-7974.19.4.592
Smart, E. L., Gow, A. J., and Deary, I. J. (2014). Occupational complexity and lifetime cognitive abilities. Neurology 83, 2285–2291. doi: 10.1212/WNL.0000000000001075
Stanziano, D. C., Whitehurst, M., Graham, P., and Roos, B. A. (2010). A review of selected longitudinal studies on aging: past findings and future directions. J. Am. Geriatr. Soc. 58, S292–S297. doi: 10.1111/j.1532-5415.2010.02936.x
Stephan, Y., Sutin, A. R., Luchetti, M., Caille, P., and Terracciano, A. (2018). Polygenic Score for Alzheimer Disease and cognition: the mediating role of personality. J. Psychiatr. Res. 107, 110–113. doi: 10.1016/j.jpsychires.2018.10.015
Stephen, R., Hongisto, K., Solomon, A., and Lönnroos, E. (2017). Physical activity and Alzheimer’s disease: a systematic review. J. Gerontol. A Biol. Sci. Med. Sci. 72, 733–739. doi: 10.1093/gerona/glw251
Stern, Y. (2012). Cognitive reserve in ageing and Alzheimer’s disease. Lancet Neurol. 11, 1006–1012. doi: 10.1016/S1474-4422(12)70191-6
Sutin, A. R., Stephan, Y., Luchetti, M., and Terracciano, A. (2018a). Loneliness and risk of dementia. J. Gerontol. B Psychol. Sci. Soc. Sci. doi: 10.1093/geronb/gby112 [Epub ahead of print].
Sutin, A. R., Stephan, Y., and Terracciano, A. (2018b). Psychological well-being and risk of dementia. Int. J. Geriatr. Psychiatry 33, 743–747. doi: 10.1002/gps.4849
Taaffe, D. R., Irie, F., Masaki, K. H., Abbott, R. D., Petrovitch, H., Ross, G. W., et al. (2008). Physical activity, physical, function and incident dementia in elderly men: the Honolulu-Asia Aging Study. J. Gerontol. A Biol. Sci. Med. Sci. 63, 529–535. doi: 10.1093/gerona/63.5.529
Terracciano, A., An, Y., Sutin, A. R., Thambisetty, M., and Resnick, S. M. (2017a). Personality change in the preclinical phase of Alzheimer disease. JAMA Psychiatry 74, 1259–1265. doi: 10.1001/jamapsychiatry.2017.2816
Terracciano, A., Stephan, Y., Luchetti, M., Albanese, E., and Sutin, A. R. (2017b). Personality traits and risk of cognitive impairment and dementia. J. Psychiatr. Res. 89, 22–27. doi: 10.1016/j.jpsychires.2017.01.011
Terracciano, A., Iacono, D., O’Brien, R. J., Troncoso, J. C., An, Y., Sutin, A. R., et al. (2013). Personality and resilience to Alzheimer’s disease neuropathology: a prospective autopsy study. Neurobiol. Aging 34, 1045–1050. doi: 10.1016/j.neurobiolaging.2012.08.008
Terracciano, A., Sutin, A. R., An, Y., O’Brien, R. J., Ferrucci, L., Zonderman, A. B., et al. (2014). Personality and risk of Alzheimer’s disease: new data and meta-analysis. Alzheimers Dement. 10, 179–186. doi: 10.1016/j.jalz.2013.03.002
Tomata, Y., Zhang, S., Sugawara, Y., and Tsuji, I. (2019). Impact of time spent walking on incident dementia in elderly Japanese. Int. J. Geriatr. Psychiatry 34, 204–209. doi: 10.1002/gps.5011
Valenzuela, M., Brayne, C., Sachdev, P., Wilcock, G., and Matthews, F. (2011). Cognitive lifestyle and long-term risk of dementia and survival after diagnosis in a multicenter population-based cohort. Am. J. Epidemiol. 173, 1004–1012. doi: 10.1093/aje/kwq476
Vemuri, P., Weigand, S. D., Przybelski, S. A., Knopman, D. S., Smith, G. E., Trojanowski, J. Q., et al. (2011). Cognitive reserve and Alzheimer’s disease biomarkers are independent determinants of cognition. Brain 134, 1479–1492. doi: 10.1093/brain/awr049
Wang, H.-X., Karp, A., Herlitz, A., Crowe, M., Kåreholt, I., Winblad, B., et al. (2009). Personality and lifestyle in relation to dementia incidence. Neurology 72, 253–259. doi: 10.1212/01.wnl.0000339485.39246.87
Wang, H.-X., MacDonald, S. W., Dekhtyar, S., and Fratiglioni, L. (2017). Association of lifelong exposure to cognitive reserve-enhancing factors with dementia risk: a community-based cohort study. PLoS Med. 14:e1002251. doi: 10.1371/journal.pmed.1002251
Whalley, L. J., Dick, F. D., and McNeill, G. (2006). A life-course approach to the aetiology of late-onset dementias. Lancet Neurol. 5, 87–96. doi: 10.1016/s1474-4422(05)70286-6
Wimo, A., Guerchet, M., Ali, G. C., Wu, Y. T., Prina, A. M., Winblad, B., et al. (2017). The worldwide costs of dementia 2015 and comparisons with 2010. Alzheimers Dement. 13, 1–7. doi: 10.1016/j.jalz.2016.07.150
Winblad, B., Amouyel, P., Andrieu, S., Ballard, C., Brayne, C., Brodaty, H., et al. (2016). Defeating Alzheimer’s disease and other dementias: a priority for European science and society. Lancet Neurol. 15, 455–532. doi: 10.1016/S1474-4422(16)00062-4
Xu, J., Wang, J., Wimo, A., Fratiglioni, L., and Qiu, C. (2017). The economic burden of dementia in China, 1990–2030: implications for health policy. Bull. World Health Organ. 95, 18–26. doi: 10.2471/BLT.15.167726
Yates, L. A., Ziser, S., Spector, A., and Orrell, M. (2016). Cognitive leisure activities and future risk of cognitive impairment and dementia: systematic review and meta-analysis. Int. Psychogeriatr. 28, 1791–1806. doi: 10.1017/s1041610216001137
Yoneda, T., Rush, J., Graham, E. K., Berg, A. I., Comijs, H., Katz, M., et al. (2018). Increases in neuroticism may be an early indicator of dementia: a coordinated analysis. J. Gerontol. B Psychol. Sci. Soc. Sci. doi: 10.1093/geronb/gby034 [Epub ahead of print].
Young, J., Angevaren, M., Rusted, J., and Tabet, N. (2015). Aerobic exercise to improve cognitive function in older people without known cognitive impairment. Cochrane Database Syst. Rev. 4:CD005381. doi: 10.1002/14651858.cd005381.pub4
Zeki Al Hazzouri, A., Haan, M. N., Kalbfleisch, J. D., Galea, S., Lisabeth, L. D., and Aiello, A. E. (2011). Life-course socioeconomic position and incidence of dementia and cognitive impairment without dementia in older Mexican Americans: results from the Sacramento area Latino study on aging. Am. J. Epidemiol. 173, 1148–1158. doi: 10.1093/aje/kwq483
Zettergren, A., Kern, S., Gustafson, D., Gudmundsson, P., Sigström, R., Östling, S., et al. (2017). The ACE gene is associated with late-life major depression and age at dementia onset in a population-based cohort. Am. J. Geriatr. Psychiatry 25, 170–177. doi: 10.1016/j.jagp.2016.06.009
Zhang, N. J., Guo, M., and Zheng, X. (2012). China: awakening giant developing solutions to population aging. Gerontologist 52, 589–596. doi: 10.1093/geront/gns105
Zhang, M. Y., Katzman, R., Salmon, D., Jin, H., Cai, G. J., Wang, Z. Y., et al. (1990). The prevalence of dementia and Alzheimer’s disease in Shanghai, China: impact of age, gender, and education. Ann. Neurol. 27, 428–437. doi: 10.1002/ana.410270412
Keywords: psychosocial factors, genetic susceptibility, interaction, cognitive aging, cognitive reserve, life-course epidemiology
Citation: Wang Y, Du Y, Li J and Qiu C (2019) Lifespan Intellectual Factors, Genetic Susceptibility, and Cognitive Phenotypes in Aging: Implications for Interventions. Front. Aging Neurosci. 11:129. doi: 10.3389/fnagi.2019.00129
Received: 20 February 2019; Accepted: 16 May 2019;
Published: 31 May 2019.
Edited by:
Guido Gainotti, Università Cattolica del Sacro Cuore, ItalyReviewed by:
Antonio Terracciano, Florida State University, United StatesCamillo Marra, Catholic University of the Sacred Heart, Rome, Italy
Copyright © 2019 Wang, Du, Li and Qiu. This is an open-access article distributed under the terms of the Creative Commons Attribution License (CC BY). The use, distribution or reproduction in other forums is permitted, provided the original author(s) and the copyright owner(s) are credited and that the original publication in this journal is cited, in accordance with accepted academic practice. No use, distribution or reproduction is permitted which does not comply with these terms.
*Correspondence: Chengxuan Qiu, chengxuan.qiu@ki.se