Plant Extracts as Potential Acaricides for the Management of Red Spider Mite, Oligonychus coffeae Nietner (Acarina: Tetranychidae), in the Tea Ecosystem: An Eco-Friendly Strategy
- 1North Bengal Regional R & D Centre, Tea Research Association, Nagrakata, India
- 2Bioinformatics Laboratory (DBT-Star College), P.G. Department of Zoology, Darrang College, Tezpur, India
The effects of the application of aqueous extracts of a selection of five traditional plants (Murraya paniculata, Cassia tora, Amphineuron opulentum, Tithonia diversifolia, and Cassia alata) were compared with that of synthetic acaricide in reducing the population of red spider mite (Oligonychus coffeae), a major tea pest, alongside their impact on natural enemies and green leaf yield. Analysis of large-scale field trials showed that all the five plants extract treatments resulted in similar yield; this was analogous to the application of synthetic acaricide. A reduction in the pest population was observed to be on par with the synthetic acaricide, with a higher number of natural enemies treated using the pesticide-plant-treated plot in comparison to the synthetic acaricide-treated plot, which indicated pesticidal plants had a lower impact on natural enemies. A phytotoxicity study on tea leaves indicated that aqueous extracts of selected plants are non-phytotoxic and do not impart any taint to the prepared tea samples. Therefore, the present investigation outlines how plant extracts used as a botanical pesticide display toxicity against red spider mite on tea plants without harming the beneficial insects, increasing the yield and avoiding any lethal consequence for the tea plants or consumers.
Introduction
Tea, Camellia sinensis (L.) O. Kuntze, is a perennial plantation crop and requires warm humid weather for ample growth and production. Such climate conditions also house a diverse range of insect pests and diseases that attack this crop, which turns them into a limiting factor for the production of tea (Hazarika et al., 2009; Majumder et al., 2012). The red spider mite, Oligonychus coffeae Nietner (Acarina: Tetranychidae), is among the foremost tea pests in India (Somchoudhury et al., 1995; Babu, 2010; Barua et al., 2016), and it causes the loss of up to 35–40% of the crop (Sundararaju and Sundara Babu, 1999; Hazarika et al., 2009). The tea plant's mature leaves are attacked by the veins and the mid-rib; finally, the whole leaf is affected. In cases of severe infestation, the tender foliage may also become damaged (Rau, 1965; Jeppson et al., 1975). The red spider mite feeds on the leaf epidermis by constantly puncturing it using their chelicerae (Jeppson et al., 1975; Babu, 2010). This pest remains active throughout the year, and unhindered infestation leads to 100% crop loss if appropriate management strategies are not suitably adopted. In tea-growing areas, planters use different synthetic acaricides to maintain this pest under the economic threshold level (ETL) of 4% (Gurusubramanian et al., 2008; Babu, 2010).
Continuous and non-judicial application of these chemical acaricides leads to certain undesirable issues, such as water pollution, degradation of valuable soil microbes, a decline in biological control agents, resurgence, development of resistance in pest, secondary pest outbreaks, and pesticide residues in manufactured tea (Cranham, 1966; Mobed et al., 1992; Roy et al., 2008, 2012; Hazarika et al., 2009; Babu, 2010). To overcome these problems, tea growers have attempted to find alternative forms of crop protection, which is essential to sustainable tea production. To develop ecologically safe pesticides, identification of toxins or antifeedants from plants is nowadays being highlighted as a prospective process (Wheller et al., 2001; Babu et al., 2004, 2008; Babu, 2010).
Botanicals play an important role in organic tea production and are considered substitute plant protection products, keeping the red spider mite population below the ETL between tea harvests (Babu et al., 2011). The continuous application of plant extracts of the same species for an extended period of time may decrease responses amongst herbivores (Liu et al., 2005). Alteration of plant species or mixing the different plant species used for plant extracts for pest control is therefore essential (Chen et al., 1995), as pest resistance is less likely to occur when using mixtures (Feng and Isman, 1995). If the plant materials are locally available, utilization of crude or raw plant extracts containing a blend of bioactive components is a simpler and more cost-effective approach. Aqueous extracts of different locally available plants in India (viz. Clerodendron infortunatum, Acorus calamus, Aegle marmelos, Xanthium strumarium, Terminalia chebula, Duranta repens, and neem kernel) have been evaluated against the red spider mite in tea, and these show varying degrees of control under field conditions (Babu et al., 2008; Roy et al., 2014, 2016). To ensure a broad choice of protectants, the selection of plants should not be a constrain to a limited choice of plants, and further exploration for other prospective plants is encouraged. It is much more important to conduct various types of bioassays during the screening of a botanical pesticides (Akhtar and Isman, 2004).
In this study, an investigation was undertaken to ascertain the bioefficacy of water extracts of a selected group of plants that commonly exist in the locality of tea-growing areas in northeast India against red spider mites. These plant species were selected because of their ample abundance around tea gardens, bushland, and roadsides, their familiarity to tea planters, and the substantial amount of accessible information that exists on their effectiveness and safety of use (Mollah and Islam, 2005, 2008; Roy et al., 2010; Deka et al., 2017; Green et al., 2017).
Analyses of the selected plant extracts and their effects on the eggs, oviposition deterrence, and adult mortality of red spider mites and Chrysoperla carnea, Oxyopes javanus, and Stethorus gilvifrons, the major predators of red spider mites (Das et al., 2010; Perumalsamy et al., 2010; Babu et al., 2011), were carried out under both laboratory and field conditions. This paper evaluates whether aqueous extracts of selected pesticidal plants have prospective use as biopesticides for the tea plant, and it also highlights the effects of using pesticidal plants.
Materials and Methods
Insect Rearing
Different stages of red spider mites were collected from the Tea Research Association, North Bengal Regional Research and Development Centre (NBRRDC) experimental plot (88° 55′ 0′′ East, 26° 54′ 0′′ North longitude) West Bengal, India. Organic tea is cultivated without the application of synthetic pesticides, chemical fertilizers, and growth regulators. After field collection, these mites were transferred on to 1-year-old potted tea plants (clone TV 1) that were kept in a greenhouse at 26 ± 3°C, 78 ± 4% RH, and an 18L: 7D photoperiod, and they were used for raising the susceptible population without any type of exposure to pesticides. From this stock, red spider mite adults were transferred onto fresh tea leaves (6 cm2) placed on moistened cotton pads (ca. 1.5 cm thick) in plastic trays (42 × 30 × 6.5 cm), which served as rearing chambers. Rearing chambers were kept under controlled conditions (26 ± 3°C, 78 ± 4% RH, and an 18L: 7D photoperiod) in a humidity chamber (Biojenik). Withered and dried leaves were regularly replaced. By adopting this technique, more than 30 generations of susceptible mite populations were maintained and utilized for carrying out the bioassays (Figures 1A,B).
Preparation of Plant Extracts
Fresh and full-grown leaves of Murraya paniculata (L.) Jack (Sapindales: Rutaceae), Cassia tora (L.) (Fabales: Fabaceae), Amphineuron opulentum (Kaulf.) Holt., (Polypodiales: Thelypteridaceae) Tithonia diversifolia (Hemsl.) A. Gray (Asterales: Asteraceae), and Cassia alata (L.) (Fabales: Fabaceae) were collected (Figures 2A–E) locally from nearby areas of NBRRDC, West Bengal. The plant materials (leaves, flower, and succulent stems) were shopped into fine pieces and allowed to dry in the shade. The dried materials were ground using an electric dicer, and they were later passed through a 30 mesh sieve and finally reserved in a 2 kg capability airtight glass jar. The aqueous extract of each selected plant was prepared by following the method of Nagappan (2012). To prepare different concentrations, viz., 2, 4, 6, 8, and 10 gm/l, different quantities of the powder, viz., 20, 40, 60, 80, and 100 gm, were weighed individually into plastic containers containing 1 L of water and then kept for 24 h. The water extracts of each concentration were filtered using a muslin cloth, labeled, and organized for application. All of the spray fluids including control were mixed with 0.1% Teepol AG as an emulsifier.
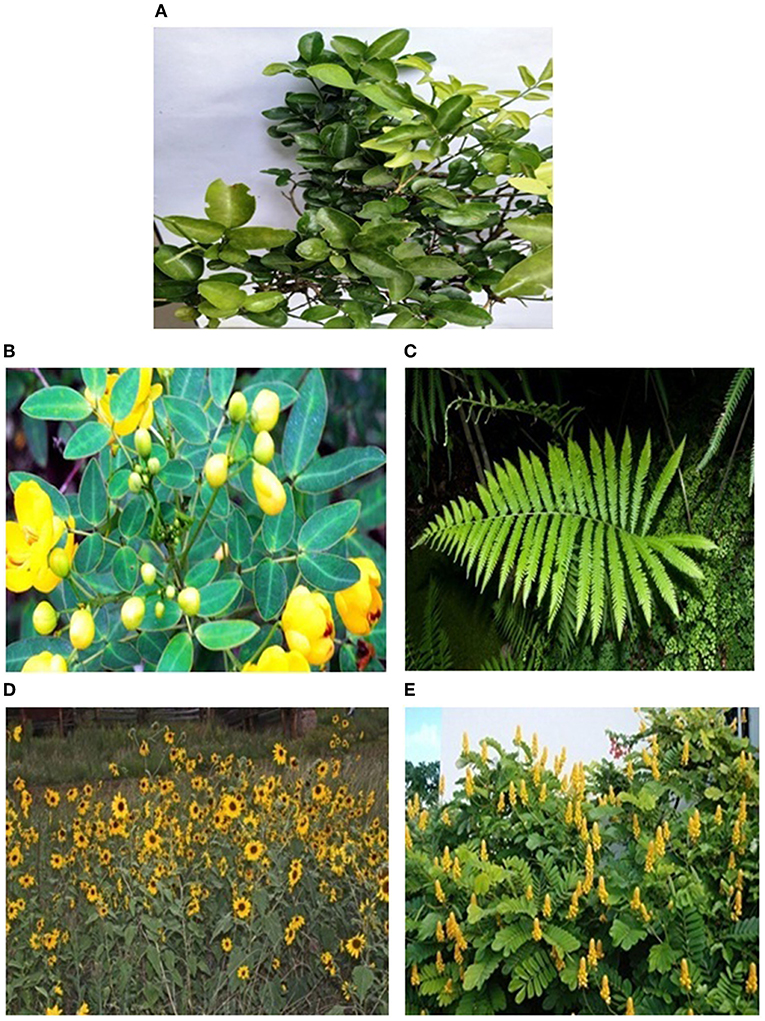
Figure 2. Pesticidal plants evaluated against tea red spider mite. (A) M. paniculata, (B) C. tora, (C) A. opulentum, (D) T. diversifolia, and (E) C. alata (source: North Bengal Regional R and D Centre, Tea Research Association, West Bengal, India).
Acaricidal Activity of Pesticidal Plant Extracts (Leaf Disc Experiment)
Tea leaves collected from the NBRRDC experimental plot were cut into discs 2 cm in diameter. The leaf discs were positioned on soaked cotton set aside in a petri dish, and throughout the study, the wetness in the cotton was prolonged by wetting it using water regularly. A total of 20 red spider mites that were 24 h old were consigned from the stock culture onto each leaf disc. Using a glass atomizer, the spray fluid of the prepared plant extract concentrations were sprayed onto each leaf disc. To ensure the fine droplets fell onto the leaf disc, the distance between the leaf disc and the atomizer was set as constant (1 ft). A control (distilled water mixed with teepol) and Fenpyroximate 5EC (Mitigate) (dose: 0.5 ml/L) were sprayed along with the different concentrations of extracts. The experiment was replicated 30 times. Following the treatments, observations were recorded after 24, 48, and 72 h, and the total numbers of live mites were recorded with a compound microscope at a specified 10X magnification. The percentage mortality of mites was analyzed statistically through analysis of variance to calculate the critical difference (CD at p = 0.05; Snedecor and Cochran, 1989). After significant effects were identified, differences between means were considered significant at a 95% confidence interval based on Tukey's post-hoc Honestly Significant Difference (HSD) test to separate the means.
Ovicidal Action of Pesticidal Plant Extracts
To evaluate the ovicidal action of the selected plant extracts, 15 adult female mites were introduced on a full-grown tea leaf (leaf number four or five from the apex of the tea bush) to lay eggs, and they were kept in a petri dish overnight. To maintain the moisture conditions in the leaves, water-soaked cotton was padded in around the leaves. After hatching the eggs, the mites were removed using a fine camel-hair brush. In each leaf, exactly 30 eggs were chosen for the bioassay; the remaining eggs were carefully detached using a fine needle. For the bioassay, we used 30 eggs per experiment where these were assessed with different concentrations of the pesticidal plant extracts, viz., 2, 4, 6, 8, and 10 gm/l (w/v) sprayed with a fabricated atomizer (made with glass, size: 100 ml and pressure: 30 psi). A control (distilled water mixed with teepol) and Fenpyroximate 5EC (dose: 0.5 ml/L) were sprayed along with the different concentrations of extracts. The experiment was repeated 30 times. After oviposition, the hatchability of the egg was recorded for both control and experimental batches for a period of 12 days. After 12 days, if the eggs did not hatch, the eggs were considered non-feasible (Sarmah et al., 1999). The egg mortality (%) was analyzed statistically through analysis of variance to calculate the critical difference (CD at P = 0.05; Snedecor and Cochran, 1989).
Ovipositional Deterrent and Repellent Activity of Pesticidal Plant Extracts
The ovipositional deterrent and repellent effects of each of the pesticidal plant extracts on adult mites were tested using the choice test method (Roy et al., 2016). The adaxial surface of each leaf disc (2 cm diameter) was positioned facing upward in a petri dish. Using a camel-hair brush, 50% of the leaf disc (either side from the midrib) was painted with each of the tested concentrations from each of the plant extracts (2, 4, 6, 8, and 10 gm/l) and another 50% of the leaf disc was painted with 0.1% soap water (considered as control) and allowed to dry. After drying, 20 gravid red spider mite females were positioned on the center of each leaf disc. After 24 h, the mites left on the treated area were considered as repelled, and the eggs laid on both halves of the leaf disc were recorded up to 4 days after treatment. The experiment was replicated 30 times. Using the following formula, the Discrimination Quotient (DQ) was calculated (Roobakkumar et al., 2010):
[C: eggs (in number) laid on the control area, T: eggs (in number) laid on the treated area. DQ shows a range (0–1), which is an indication of a conclusion showing the consequence of any treatments on the insect's ovipositional behavior].
Large Scale Field Study of Pesticidal Plant Extracts
To evaluate the effectiveness of each selected plant extract against red spider mites in tea plants, this study was conducted at field sites for two consecutive seasons in tea gardens representing two different geographical locations, viz., Zone A: Mission Hill Tea Garden, Darjeeling (27.1° 94′ 5″ N, 87° 17′ 43″ E longitude) and Zone B: the experimental plot at the NBRRDC, Dooars (26.1° 55′ 0″ N, 88.1° 56′ 0″ E longitude) West Bengal, India. The Mission Hill Tea Garden location was at an elevation of 235 m above MSL with a mean maximum temperature of 300 and mean minimum temperature of 6°C, with a mean annual rainfall of 1,300 mm; in the North Bengal Regional R&D Centre experimental plot, the location was at an elevation of 214 m above MSL with a mean maximum temperature of 36° and mean minimum temperature of 10°C and a mean annual rainfall of 1,200 mm. The tea gardens where field trials took place were more than 50 years old. Sections of gardens were planted with mixed clones (mostly TV1, Teenali 17, S3A3, TV25, and TV26). Each experiment was conducted in a randomized block design (RBD) that was replicated for five blocks (Anderson and McLean, 1974). Including the untreated control, each treated plot consisted of 100 bushes that were separated by three buffer rows (8 m spacing). A preliminary study using different doses (viz., 2, 4, 6, 8, and 10 g/l w/v) of plant extract against red spider mite shows that 10 g/l showed better efficacy against this pest. Therefore, a dose of 10 g/l (40 kg/ha; 400 L of spray fluid is required to cover a one-hectare area of the tea plantation) was considered for further field study. In all the trials, the synthetic acaricide Fenpyroximate 5EC, which was applied as per the central insecticide board's (CIB) instructions (@ 200 ml/ha), was used as a positive control, and water was used as a negative control.
Observations of the red spider mite population were recorded from both surfaces (adaxial and abaxial) of randomly collected tea leaves from each treated and control plot (Babu et al., 2008). Before spraying, 100 leaves were randomly collected from each treatment, and the red spider mite population there was counted for pretreatment assessment. Using a hand-operated knapsack sprayer (droplet diameter: 1.6 mm, hollow cone NMD 60450 nozzle, discharge rate: 450 ml/min at 40 psi pressure, and droplet size: 140 mL), spraying was carried out, the bushes were drenched for better coverage and control. Prior to being re-filled with another formulation for application, the spraying machine was cleaned thoroughly with soap and water. Similar to the pre-treatment assessment, post-treatment assessments were also carried out at 7-day intervals up to the fourth week, and, accordingly, the decrease in mites (mean) during each treatment was analyzed with the following formula:
Here, X indicates the pre-treatment population count and Y the post-treatment population count.
All the data were angularly transformed prior to the statistical analysis. The differences among the population density of mites before and after each treatment were assessed through Tukey's post-hoc Honestly Significant Difference (HSD) test to separate the means at a 95% confidence interval, and the critical difference (CD, p = 0.05) was analyzed accordingly (Snedecor and Cochran, 1989).
Sampling for the Presence of Non-target Beneficial Insects
To find out the effects of pesticidal plant extracts on non-target beneficial insects, a direct spray test was conducted by following the methodology of Leatemia and Isman (2004). For the bioassay, the study was conducted for two consecutive seasons under field conditions in tea gardens representing two different geographical locations, viz., Zone A: Mission Hill Tea Garden, Darjeeling and Zone B: the experimental plot at the NBRRDC, Dooars West Bengal, India. Each of the plant extracts were sprayed (dose: 4 kg/ha) with Fenpyroximate 5EC (@ 200 ml/ha) and a control (distilled water mixed with teepol). The populations of non-target beneficial organisms like C. carnea, O. javanus, and S. gilvifrons were recorded on day 0 (pre-spray) and day 21 (post-spray; a total of two rounds of spraying was carried out; the first spray on day 0 and the second spray on day 7). Visual observations were made from 30 randomly selected bushes per replication to estimate the population of C. carnea nymphs and O. javanus adults. A total of 50 leaves were collected at random per replication, and these were observed under a binocular microscope to estimate the larval population of S. gilvifrons.
Phytotoxicity, Tainting, and Organoleptic Tests
To evaluate the phytotoxic effect of each plant extract (at X, 2X, and 4X concentrations) on tea leaves, a field experiment was carried out. Four treatments were used: (1) 10 gm/l, (2) 20 gm/l, (3) 40 gm/l of water, and (4) a control (water spray), and three replications were maintained at 84 square meters per replication. Similar to the experiment large scale field study, spraying was carried out, and observations were recorded on day 0 (pre-treatment) and days 3, 7, and 14 (post-treatment) on yellowing, stunting, necrosis, epinasty, hyponasty etc. The injury levels were graded with the following Phytotoxicity Rating Scale (PRS):
To find out whether the pesticidal plant extracts resulted in any taint (odor and foreign taste) to the black tea, on days 7 and 14 after spraying, tea shoots were harvested and processed in a mini CTC (crush, tear, curl) machine. The prepared samples of tea were assessed by a professional tea taster to check whether there was a taint or not. For the organoleptic test, liquor strength and leaf infusions were considered and scored (1–2: poor quality, 3–5: moderate quality, 6–8: good quality, and 10: very good quality; Roy et al., 2014).
Effect of Pesticidal Plant Extracts on Green Leaf Yield
In order to find out the effects of aqueous plant extracts on reducing the mite infestation, the yield (green leaf) was also recorded during the bioassay carried out in the field conditions by maintaining a standard plucking round of 7-day intervals, and the yield (green tea leaf) was recorded for the first six rounds of plucking. The average yield was expressed in kg/plot. The yield recorded at each plucking was converted into made tea for one hectare as described by Ponmurugan and Baby (2007) using the formula
Results
Acaricidal Activity of Pesticidal Plant Extracts (Leaf Disc Experiment)
Findings of the leaf disc experiment indicated that, amongst the five plant extracts, T. diversifolia and C. alata @ 10 gm/l concentrations were more effective than the other three plants even after 24 h of treatment, showing maximum mortality (Table 1). After 72 h, both plants offered more than 80% mortality at 10 g/l concentration, which was similar to the synthetic acaricide Fenpyroximate 5EC, while in the other three plants, 52–70% mortality of the red spider mite was recorded.
Ovicidal Action of Pesticidal Plant Extracts
Ovicidal action of the extracts was reliant on concentrations, i.e., percent mortality increased with a rising concentration of each plant extract. In comparison to the control, the aqueous extract of all of the selected plants affected the hatchability of the eggs. The experimental findings showed that the highest mortality was recorded against T. diversifolia at the highest concentration (10 gm/l) among the tested concentrations of the selected plant extract to the level of 66.7%, followed by 58.4, 57.4, and 48.5% egg mortality, which was recorded in highest concentrations against C. alata, A. opulentum, and M. paniculata, respectively. This is comparable to the market sample Fenpyroximate 5EC, where around 70% egg mortality was recorded. The lowest mortality against the highest concentrations was recorded against C. tora (34.15%) (Table 2).
Ovipositional Deterrent and Repellent Activity of Pesticidal Plant Extracts
The number of eggs laid was considerably lower on the treated side of the leaf disc where plant extracts (2, 4, 6, 8, and 10 gm/l) were sprayed than on the control side. Regarding the number of eggs laid, adult mites showed discrimination in terms of their egg laying when the leaves were treated with the pesticidal plant extracts. In this experiment, the DQ value was high (range 0.432–0.819) in all tested plants at superior concentrations, i.e., 10 g/extract. The DQ value was highest (0.819) in T. diversifolia, followed by C. alata, A. opulentum, M. paniculata, and C. tora, respectively (Table 3).
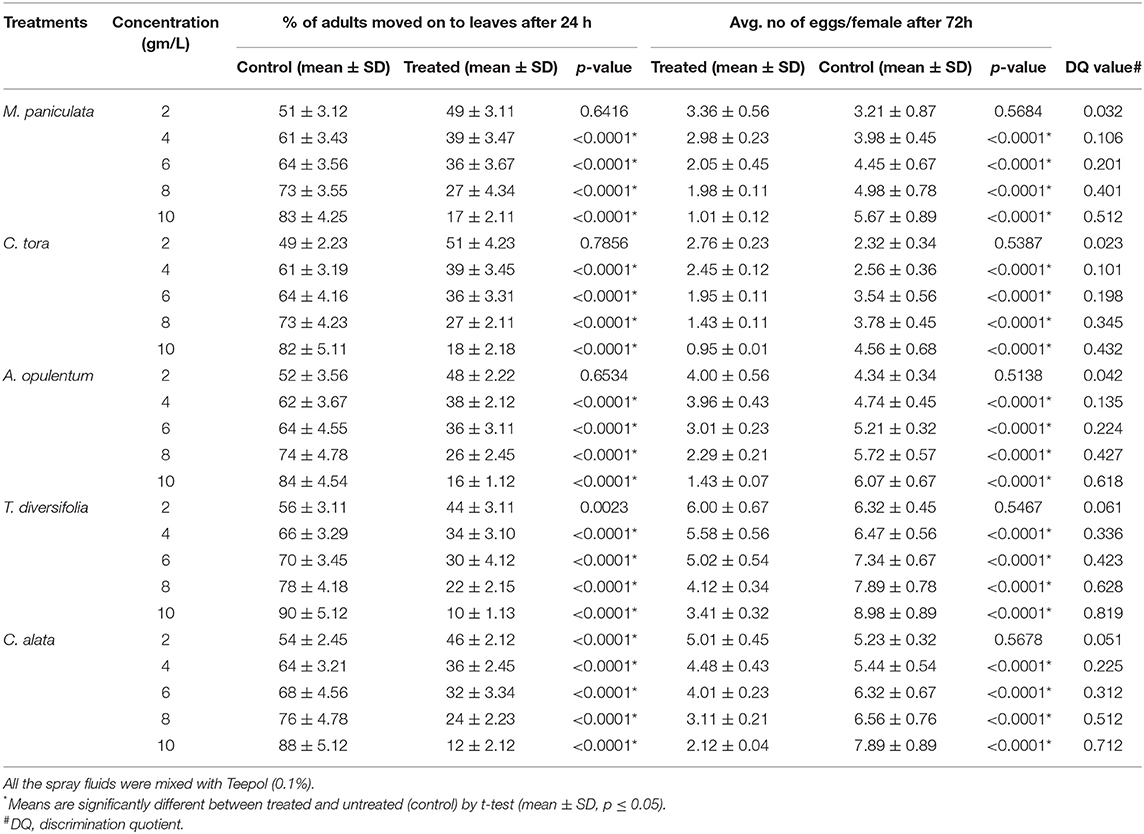
Table 3. The percentage of female red spider mites that remained on the leaves where introduced and the numbers of eggs oviposited on the control (untreated) or treated tea leaves with pesticidal plant extracts in choice tests.
Large-Scale Field Study
The field evaluation results of each selected plant extract against red spider mite at Zone A (Mission Hill Tea Garden, Darjeeling) and Zone B (NBRRDC experimental plot, Dooars) during season I and season II are presented in Table 4.
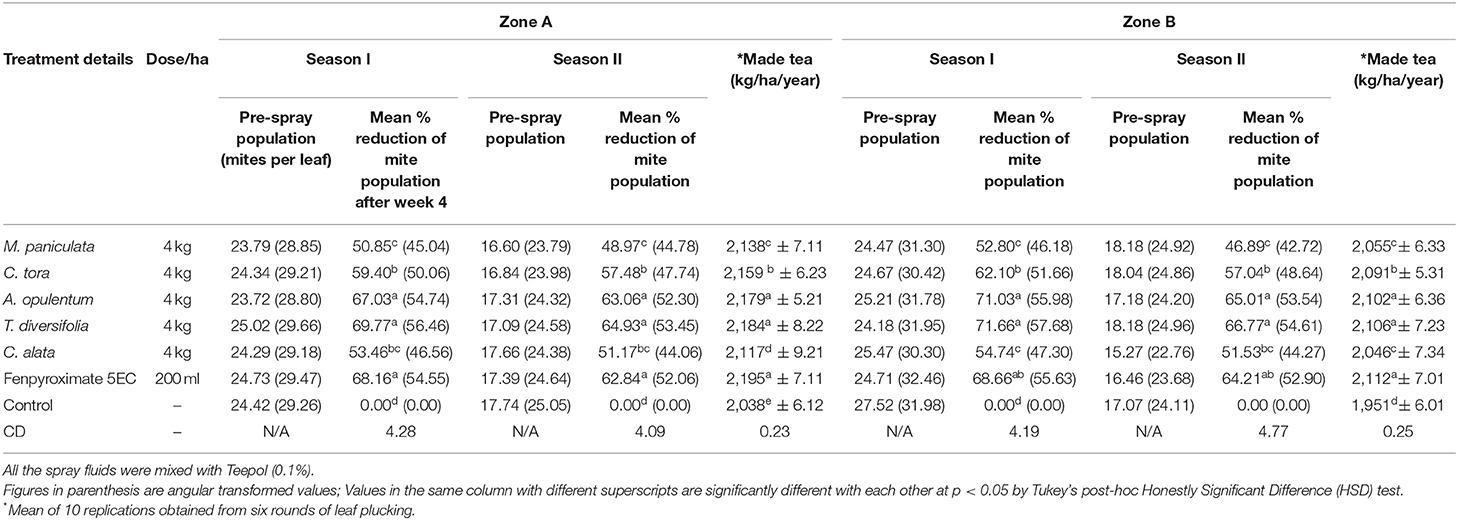
Table 4. Efficacy of pesticidal plant extracts on red spider mite in field conditions and effect on yield.
In Zone A, during season I, pre-treatment observation of the red spider mite population ranged from 23.72 to 25.02%. Results indicated that all the treatments were found to be significantly superior to untreated checks in minimizing the population of mites (Table 4). The mean percent reductions in the red spider mite population in the cases of A. opulentum (67.03%) and T. diversifolia (69.77%) at doses of 4 kg /ha were similar to Fenpyroximate 5EC (68.16%). The reduction in the mean red spider mite population was slightly inferior in plots treated with M. paniculata (50.85%), C. tora (59.40%), and C. alata (53.46%) compared to plots treated with Fenpyroximate 5EC.
During season II also, both A. opulentum (63.06%) and T. diversifolia (64.93%) minimized the mite population in a manner similar to the Fenpyroximate 5EC (62.84%), which were significantly superior when compared to M. paniculata (48.97%), C. tora (57.48%), and C. alata (51.17%; Table 4).
In Zone B, during season I, the incidence of red spider mite infestation was significantly reduced in plots treated with various insecticides as compared to untreated control (Table 4). Among all the tested pesticidal plants, T. diversifolia (71.66%) and A. opulentum (71.03%) significantly reduce the number of red spider mites followed by C. tora (62.10%), C. alata (54.74%), and M. paniculata (52.80%). However, there was no significant difference in mean percent control of red spider mite among C. tora, A. opulentum, T. diversifolia, and Fenpyroximate 5% EC after 21 days. Similar to season I, during season II, the plots treated with pesticidal plant extracts were found to be the most effective treatments for the management of red spider mites. While comparing the overall mean data recorded during the study period, the mean infestation incidence was significantly on par in plots treated T. diversifolia and A. opulentum at 4 kg/ha and Fenpyroximate 5% EC at 200 ml/ha (Table 4). Treatments using C. tora, C. alata, and M. paniculata extracts were found to be inferior to treatments using Fenpyroximate 5% EC at 200 ml/ha but superior to untreated checks in reducing the mean percent reduction of the red spider mite.
Effects on Non-target Beneficial Insects
The population level of non-target beneficial organisms was recorded on day 0 (pre-treatment) and day 21 after two rounds of spray (post-treatment). The most common insect predators in the tea plant ecosystem, viz., C. carnea, O. javanus, and S. gilvifrons, were recorded during the trial period. The present results indicate that the tested pesticidal plants did not have any adverse effect on these three non-target beneficial organisms (Figures 3A,B). The numbers of beneficial insects in tea plants treated with plants extracts were similar to that noticed in the control. In both the seasons, among all the treatments, the synthetic acaricide (Fenpyroximate 5EC) significantly reduced numbers of non-target beneficial insects compared to all other treatments (P < 0.05; Figures 3A,B).
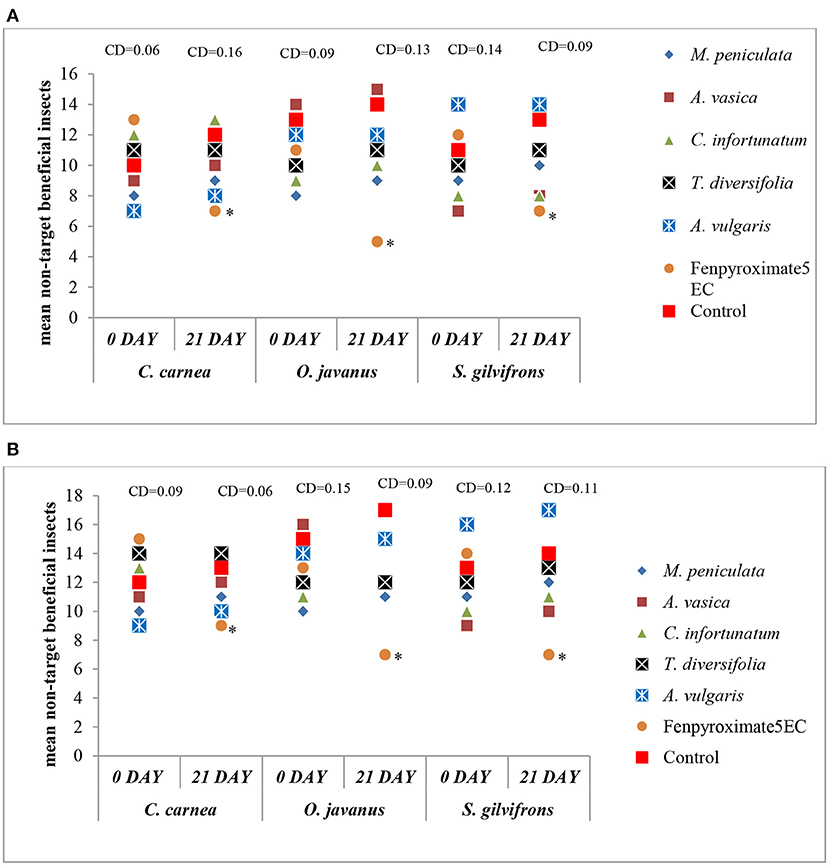
Figure 3. Effect of aqueous extracts of selected plants on non-target beneficial organisms before treatment (0 day) and after 21 days. (A) during season-I and (B) during season-II. *Values are significantly different between day 1 and 21 by t-test (p < 0.05).
Phytotoxic Effect, Tainting, and Organoleptic Test
The phytotoxicity study was carried out separately after the application of aqueous extracts of selected plants at 10, 20, and 40 gm/l water. Observations recorded on phytotoxicity symptoms indicate that none of the concentrations showed any type of phytotoxic effect on tea leaves. There was no visible injury on the tip or the surface of the tea leaves, nor was there wilting, necrosis, vein clearing, hyponasty, or epinasty. Likewise, after the application of pesticidal plant extracts, tea shoots (two leaves and a bud) were plucked on days 1, 3, 5, 7, 10, and 14, and manufactured samples were tested by professional tea tasters which revealed that the made tea did not show any taint and scored 6.5–7 in organoleptic test, which represented excellent color, liquor, quality, and potency.
Effect of Pesticidal Plant Extracts on Green Leaf Yield
The average yield recorded from the first six pluckings (at 7-day intervals) from both the experimental locations during the trial period is presented in Table 4. In spite of there being more insect pest populations on tea plants being treated with selected pesticides than with the synthetic pesticides, harvested yields acquired from the pesticidal plant treatments were shown to be as good as those from the synthetic pesticides. The yield (green leaf in kg/plot) was significantly low in the untreated control, confirming the better efficacy of the different tested plant extracts (Table 4). This is most prominent with the use of T. diversifolia and A. opulentum where the yields were statistically comparable in zone A (2,184 and 2,179 kg/ha, respectively) and zone B (2,106 and 2,102 kg/ha, respectively) with Fenpyroximate 5EC (2,195 kg/ha in zone A and 2,112 kg/ha in zone B) and was statistically higher when compared to the control (2,038 kg/ha in zone A and 1,951 kg/ha in zone B). The synthetic pesticides (Fenpyroximate 5EC) showed the highest increase in yield in both locations. In the case of other pesticidal plants, viz., M. paniculata, C. tora, and C. alata, the yields were slightly lower (not statistically significant) than with T. diversifolia, A. opulentum, and Fenpyroximate 5EC in both the locations. In comparison to the yields recorded in the control plot, the pesticidal plant treatments showed an ability to considerably enhance the percentage of the yield.
Discussion
Earlier works on the pesticidal plant species that are being used to control different tea pests have reported bioactivities against insects, bacteria, fungi, and parasites (Sarmah et al., 1999; Singh, 2000; Weinzierl, 2000; Raja et al., 2003; Isman, 2006; Roy et al., 2010, 2011; Roy and Mukhopadhyay, 2012; Vasanthakumar et al., 2012; Deka et al., 2017). Conversely, none of these works have dealt with the studies on the impact on field crop performance or tri-trophic interactions.
The findings obtained from the leaf disc experiment show that all of the selected plant extracts showed acaricidal activity where 52–82% mortality of adult red spider mites were recorded with the highest dose (10 g/l) after 72 h (Table 1). Similar properties of indigenous plant extracts Linostoma decandrum, Ageratum houstonianum, A. haustonianum Bidens pilosa, Allamanda catharitica, B. pilosa, Crassocephalum crepidioides, Casuarina equisetifolia, Gliricidia sepium, Conyza bonariensis, Lantana camara, and Ocimum basilicum, which were recorded by Bora et al. (1998) and Radhakrishnan and Prabhakaran (2014). Pesticidal plants as insecticides (botanical insecticides) are gaining importance since numerous plants show insecticidal properties. Currently, botanical insecticides comprise only 1% of the world insecticide trade, however, its annual sales growth in near about 15% is utterly promising (Wink, 1993). In the house and backyard sector, the impact of botanical insecticides is most prominent (Isman, 1995). Plant products that have prospective use as insecticidal compounds have gained astonishing importance in recent years (Nattudurai et al., 2015). Therefore, based on the finding of our laboratory studies, large-scale field trials were carried out using the extracts of the same plants.
The aqueous extracts of different plants were sprayed on the eggs of red spider mite, and all the pesticidal plants influenced the hatchability of the eggs and showed the ovicidal activity of the extracts and at higher concentrations, all of which was an effect similar to that of synthetic acaricides. Chemical substances present in the host plants possibly block the micropyle region of the egg, preventing the gaseous exchanges that finally destroy the embryo in the egg. Raja et al. (2003) screened nine different plants using different solvent extracts against Spodoptera litura as ovicidal and ovipositional deterrents, and they noticed efficacy irrespective of the solvents and concentrations used for the extraction. The curtailed blastokinesis and anomalous rupture of additional embryonic membranes in the embryo, or irregular diffusion of extracts through the egg chorion to remarkable parts of eggs at unusual times of the perceptive time, could also be related to an explanation of the inconsistency of morphological possessions (Slama, 1974). Vasanthakumar et al. (2012) studied the acaricidal action of the leaf extracts of Gliricidia maculate, Vitex negundo, Wedelia chinensis, Pongamia glabra, and Morinda tinctoria on red spider mites and reported that the aqueous extracts of P. glabra and M. tinctoria showed utmost ovicidal activity, ovipositional anticipation, and 100% adult mortality. The current findings also showed similar results with reference to the ovicidal action of the selected pesticidal plant extracts against the red spider mites.
Along with the ovicidal and adulticidal properties, the aqueous extracts of five selected pesticidal plants also indicated a repellency action against adult red spider mites. This indicates the selected pesticidal plants have repellent effects that depress the feeding behavior and movement of the mites. Roy et al. (2016) and Handique et al. (2017) also reported similar activity when they sprayed leaf extracts of D. repens, Nyctanthes arbor-tristis, Phlogacanthus thyrsiformis, and Sapindus mukorossi against red spider mites. Similar to the red spider mites on tea, the repellent properties of different plant extracts were studied and established against different pests, viz., A. aegypti (Yang et al., 2004), Culex tritaeniorhynchus (Karunamoorthi et al., 2008), and whitefly, Bemisia tabaci (Al-mazra'awi and Ateyyat, 2009). The aqueous extracts of M. paniculata, C. tora, A. opulentum, T. diversifolia, and C. alata also exerted ovipositional deterrent effects by preventing the red spider mites from laying eggs on the treated leaves. The ovipositional deterrence property of ethanol extract of N. arbor-tristis was also evaluated and confirmed against Helicoverpa armigera (Chauhan et al., 2008). Our findings also agree with those of Roobakkumar et al. (2010) and Handique et al. (2017) in terms of the use of plant extracts against the red spider mites.
In lower-economic countries, synthetic pesticides are frequently used, which leads to several harmful impacts on the ecosystems as well as human health (Ecobichon, 2001). In these countries, the application of pesticidal plant extracts as biocontrol options has been argued for extensively as sustainable options suitable for smallholder farmers (Isman, 2006; Sola et al., 2014), and our experimental findings also confirmed this and indicated that application of extracts of pesticidal plant extract can control pests effectively. It has also been demonstrated that pesticidal plants can minimize the population of red spider mites and can support yield increase comparable to those where synthetic pesticides were used. The relatively regular application of pesticidal plant extracts highlights their use as synthetic pesticide substitutes; they are active compounds that break down rapidly and they show little persistence (Casida, 1980). This means that, during the preparations of the water extracts of these plants, the labor inputs might increase, however, for the commercialization of these products, integrating photostabilizers and sticking agents may perhaps lengthen their effectiveness on crops and hence reduce the frequency of application. This kind of substitution for synthetic pesticides is commonly accepted by the small tea growers due to the high cost of synthetic pesticides; the use of pesticidal plants minimally requires labor costs for harvesting and processing. A cost-effective analysis of the application of pesticidal plants shows that these of more cost-benefit than synthetic pesticides (Amoabeng et al., 2014; Mkenda et al., 2015). By contrast, the lower persistence of these plants indicates that the consumer's health is at a minimum risk because of the reduced exposure to the plant's bioactive compounds that decompose into harmless natural products, unlike with the synthetic pesticides, which stick to the plants for few days or remain in the soil for weeks or months or even years. It indicates that leaves can be harvested without the risk of leftover residue as exposure to UV light and microorganisms present in that environment helps to quicken the breakdown of naturally occurring compounds (Isman, 2000; Angioni et al., 2005; Caboni et al., 2006). The findings of this research indicated another important transaction when comparing pesticidal plant and synthetic plant protection and their impact on non-target beneficial organisms. Toxicity and the perseverance of synthetic pesticides as anticipated their effect on predators, parasitoids, and pollinators is generally extremely high (Potts et al., 2010; Stanley and Preetha, 2016). In fact, our findings indicated that synthetic pesticides regularly used in the tea ecosystem for the control of red spider mites resulted in the non-existence of predators/parasitoids observed over the cropping season. In general, it leads to the occurrence of pest resurgence after the application of synthetic pesticides enabling populations of pest species to spread out in the non-existence of beneficial insects (Roubos et al., 2014; Welch and Harwood, 2014). On the other hand, pesticidal plant extracts showed less of an impact on predators/parasitoids. A marginal difference was noticed (statistically not significant) amongst the pesticidal plant extracts and the control (water spray) in terms of non-target beneficial insects as compared to the reductions noticed in the synthetic treatment.
It is due to the lower persistence of the pesticidal plant extracts and the various modes of accomplishment where the pesticidal plant treatments may perhaps act against pests as anti-feedants and repellents through toxicity post-ingestion (Tembo et al., 2018). The lower toxic effect and the persistence of the pesticidal plant extracts are supported by their condensed effects on beneficial insects. Compared to the synthetic pesticide, pests are less affected by the pesticidal plants extracts; however, it would facilitate the natural pest directive. As a result, controlling pest populations is additionally effective as regulation of pests is more effective when the ratio between the pests and predators in numbers is less (Arditi and Ginzburg, 1989; Rusch et al., 2010). Even though all the selected plant extracts could reduce a similar or lower number of pests in comparison to the synthetic pesticide, crop yields were repeatedly comparable with that of the synthetic chemicals. This may be due to the increased decline in the pest population through natural enemies, and perhaps the bush can tolerate a definite amount of damage and can physiologically compensate to maintain overall yield (Rubia et al., 1996; Brown, 2005). We may see forms of crop protection via the direct control of fungal or bacterial pathogens or indirect physiological support by acting as a topical green fertilizer, foliar feed, biostimulants, or the phytotonic effects of the plant extracts (Jama et al., 2000; Shaaban, 2001; Soylu et al., 2010; Marei et al., 2012; Rasoul et al., 2012; Pretali et al., 2016).
Interpretation of the phytotoxicity on leaves of tea plants indicated that the selected plants are non-phytotoxic to tea, and the teas made from the leaves sprayed with these extracts when evaluated by professional tea tasters revealed that prepared tea had no taint. Thus, the present study suggests that plant extracts used as a pesticide showed toxicity against red spider mites on tea plants without harming the beneficial insects, increasing the yield without being accompanied by toxic consequences for the part of the tea plants and consumers.
Data Availability Statement
The original contributions presented in the study are included in the article/supplementary material, further inquiries can be directed to the corresponding author/s.
Author Contributions
BD performed laboratory and field trials, analyzed and interpreted the data of the work, and prepared the original manuscript. AB designed and guided the laboratory as well as the field trials and reviewed and edited the writing. CB collected plant materials, prepared the extracts, and assisted in field trials. SS assisted in conducting of laboratory and field trials. All authors read and approved the final manuscript.
Conflict of Interest
The authors declare that the research was conducted in the absence of any commercial or financial relationships that could be construed as a potential conflict of interest.
Publisher's Note
All claims expressed in this article are solely those of the authors and do not necessarily represent those of their affiliated organizations, or those of the publisher, the editors and the reviewers. Any product that may be evaluated in this article, or claim that may be made by its manufacturer, is not guaranteed or endorsed by the publisher.
Acknowledgments
The authors are thankful to the Director, Tea Research Association, Tocklai Tea Research Institute, Jorhat, Assam, India, for proving all necessary facilities and support for the successful completion of this study.
References
Akhtar, Y., and Isman, M. B. (2004). Comparative growth inhibitory and antifeedant effects of plant extracts and pure allelochemicals on four phytophagous insect species. J. Appl. Entomol. 128, 32–38. doi: 10.1046/j.1439-0418.2003.00806.x
Al-mazra'awi, M. S., and Ateyyat, M. (2009). Insecticidal and repellent activities of medicinal plant extracts against the sweet potato whitefly, Bemisia tabaci (Hom.: Aleyrodidae) and its parasitoid Eretmocerus mundus (Hym.: Aphelinidae). J. Pest Sci. 82, 149–154. doi: 10.1007/s10340-008-0233-x
Amoabeng, B. W., Gurr, G. M., Gitau, C. W., and Stevenson, P. C. (2014). Cost: benefit analysis of botanical insecticide use in cabbage: implications for smallholder farmers in developing countries. Crop Prot. 57, 71–76. doi: 10.1016/j.cropro.2013.11.019
Anderson, V. L., and McLean, R. A. (1974). Design of Experiments: A Realistic Approach. New York, NY: M. Dekker.
Angioni, A., Dedola, F., Minelli, E. V., Barra, A., Cabras, P., and Caboni, P. (2005). Residues and half-life times of pyrethrins on peaches after field treatments. J. Agric. Food Chem. 53, 4059–4063. doi: 10.1021/jf0477999
Arditi, R., and Ginzburg, L. R. (1989). Coupling in predator-prey dynamics: ratio-Dependence. J. Theor. Biol. 139, 311–326. doi: 10.1016/S0022-5193(89)80211-5
Babu, A. (2010). Pest management in tea: the south Indian Scenario. Bull. UPASI Tea Res. Found. 55, 23–30.
Babu, A., Perumalsamy, K., Sankara, R. S. M., and Muraleedharan, N. (2008). Use of neem kernel aqueous extract for the management of red spider mite infesting tea in south India. J. Plant. Crops 36, 393–397.
Babu, A., Selvasundaram, R., Muraleedharan, N., and Sasidhar, R. (2004). A new predator of red spider mites. News UPASI Tea Res. Found. 14:1.
Babu, A., Vasanthakumar, D., Rahman, J., Roobak Kumar, A., and Sundaravadivelan, C. (2011). Potential of Malladaboninensis okamoto (Neuroptera: Chrysophidae), as a biocontrol agent of Oligonychus coffeae nietner (Acarina: Tetranychidae) infesting tea. J. Plant. Crops 39, 193–195.
Barua, A., Babu, A., and Rajkhowa, R. C. (2016). Seasonal abundance and predatory potential of Stethorusaptus kapur (Coleoptera: Coccinellidae): a biocontrol agent of tea red spider mite Oligonychus coffeae nietner (Acarina: Tetranychidae). Proc. Zool. Soc. 71, 224–228. doi: 10.1007/s12595-016-0197-4
Bora, H. R., Hazarika, L. K., and Dutta, N. (1998). “Green pesticides, crop protection and safety evaluation,” in Botanicals for Forest and Tea Pest Management, eds N. P. Agrihotri, S. Walia, and V. T. Gajbhiye (New Delhi: Society of Pesticide Science, India; Division of Agricultural Chemicals; IARI), 101–106.
Brown, P. R. (2005). The effect of simulated house mouse damage to wheat in Australia. Crop Prot. 24, 101–109. doi: 10.1016/j.cropro.2004.06.012
Caboni, P., Sarais, G., Angioni, A., Garcia, A. J., Lai, F., Dedola, F., et al. (2006). Residues and persistence of neemformulations on strawberry after field treatment. J. Agric. Food Chem. 54, 10026–10032. doi: 10.1021/jf062461v
Casida, J. E. (1980). Pyrethrum flowers and pyrethroid insecticides. Environ. Health Perspect. 34, 189–202. doi: 10.1289/ehp.8034189
Chauhan, P., Sharma, H. B., Sharma, R., and Bhadoria, N. S. (2008). Ovipositional deterrent effect of some plant extracts against gram pod borer, Helicoverpa armigera (Huber). Plant Arch. 8, 991–993.
Chen, W., Isman, M. B., and Chiu, S. F. (1995). Antifeedant and growth inhibitory effects of the limonoid toosendanin and Melia toosendan extracts on the variegated cutworm, Peridromasaucia (Lep., Noctuidae). J. Appl. Entomol. 119, 367–370. doi: 10.1111/j.1439-0418.1995.tb01302.x
Cranham, J. E. (1966). Tea pests and their control. Annu. Rev. Entomol. 11, 491–514 doi: 10.1146/annurev.en.11.010166.002423
Das, S., Roy, S., and Mukhopadhyay, A. (2010). Diversity of arthropod natural enemies in the tea plantations of North Bengal with emphasis on their association with tea pests. Curr. Sci. 99, 1457–1463.
Deka, B., Babu, A., and Sarmah, M. (2017). Bio-efficacy of certain indigenous plant extracts against red spider mite, Oligonychus coffeae, Nietner (Tetranychidae: Acarina) infesting tea, J. Tea Sci. Res. 7, 28–33. doi: 10.5376/jtsr.2017.07.0004
Ecobichon, D. J. (2001). Pesticide use in developing countries. Toxicology 160, 27–33. doi: 10.1016/S0300-483X(00)00452-2
Feng, R., and Isman, M. B. (1995). Selection for resistance to azadirachtin in the green peach aphid, Myzuspersicae. Experientia 51, 831–833 doi: 10.1007/BF01922438
Green, P. W. C., Belmain, S. R., Ndakidemi, P. A., Farrell, I. W., and Stevenson, P. C. (2017). Insecticidal activity of Tithonia diversifolia and Vernonia amygdalina. Ind. Crops Prod. 110, 15–21. doi: 10.1016/j.indcrop.2017.08.021
Gurusubramanian, G., Rahman, A., Sarmah, M., Roy, S., and Bora, S. (2008). Pesticide usage pattern in tea ecosystem, their retrospects and alternative measures. J. Environ. Biol. 29, 813–826.
Handique, G., Roy, S., Rahman, A., Bora, F. R., and Barua, A. (2017). Use of some plant extracts for management of red spider mite, Oligonychus coffeae (Acarina: Tetranychidae) in tea plantations. Int. J. Trop. Insect Sci. 37, 234–242. doi: 10.1017/S1742758417000169
Hazarika, L. K., Bhuyan, M., and Hazarika, B. N. (2009). Insect pests of tea and their management. Annu. Rev. Entomol. 54, 267–284. doi: 10.1146/annurev.ento.53.103106.093359
Isman, M. B. (1995). Leads and prospects for the development of new botanical insecticides, Rev. Pestic. Toxicol 3, 1–20.
Isman, M. B. (2000). Plant essential oils for pest and disease management. Crop Prot. 19, 603–608. doi: 10.1016/S0261-2194(00)00079-X
Isman, M. B. (2006). Botanical insecticides, deterrents and repellents in modern agriculture and an increasingly regulated world. Annu. Rev. Entomol. 51, 45–66. doi: 10.1146/annurev.ento.51.110104.151146
Jama, B., Palm, C. A., Buresh, R. J., Niang, A., Gachengo, C., Nziguheba, G., et al. (2000). Tithonia diversifolia as a green manure for soil fertility improvement in western Kenya: a review. Agrofor. Syst. 49, 201–221. doi: 10.1023/A:1006339025728
Jeppson, L. R., Keifer, H. H., and Baker, E. W. (1975). Mites Injurious to Economic Plants. Berkeley, CA: University of California Press. doi: 10.1525/9780520335431
Karunamoorthi, K., Ramanujam, S., and Rathinasamy, R. (2008). Evaluation of leaf extracts of Vitex negundo L. (Family: Verbenaceae) against larvae of Culex tritaeniorhynchus and repellent activity on adult vector mosquitoes. Parasitol. Res. 103, 545–550. doi: 10.1007/s00436-008-1005-5
Leatemia, J. A., and Isman, M. B. (2004). Insecticidal activity of crude seed extracts of Annona spp., Lansium domesticum and Sandoricum koetjape against lepidopteran larvae. Phytoparasitica 32, 30–37. doi: 10.1007/BF02980856
Liu, S. S., Li, Y. H., Liu, Y., and Zalucki, M. P. (2005). Experience-induced preference for oviposition repellents derived from a nonhost plant by a specialist herbivore. Ecol. Lett. 8, 722–729. doi: 10.1111/j.1461-0248.2005.00776.x
Majumder, A. B., Pathak, S. K., and Hath, T. K. (2012). Evaluation of some bio-rational insecticides against the looper complex, Hyposidra spp. in tea plantations of Dooars, West Bengal. J. Biopest. 5, 91–95.
Marei, G. I. K., Abdel Rasoul, M. A., and Abdelgaleil, S. A. M. (2012). Comparative antifungal activities and biochemical effects of monoterpenes on plant pathogenic fungi. Pestic. Biochem. Physiol. 103, 56–61. doi: 10.1016/j.pestbp.2012.03.004
Mkenda, P., Mwanauta, R., Stevenson, P. C., Ndakidemi, P., Mtei, K., and Belmain, S. R. (2015). Extracts from field margin weeds provide economically viable and environmentally benign pest control compared to synthetic pesticides. PLoS ONE 10:e0143530. doi: 10.1371/journal.pone.0143530
Mobed, K., Gold, E. B., and Scgenker, M. B. (1992). Occupational health problems among migrants and seasonal farm workers. Western J. Med. 157, 367–373.
Mollah, J. U., and Islam, W. (2005). Effect of Murraya paniculata (L.) Jack extract on the adult mortality of Callosobruchus maculatus F. (Coleoptera: Bruchidae). Front. Nat. Prod. Chem. 1, 209–214. doi: 10.2174/1574089054583614
Mollah, J. U., and Islam, W. (2008). Toxicity of Murraya paniculata (L.) Jack leaf-derived materials against Callosobruchus maculatus (f.) (coleoptera: bruchidae). Pak. Entomol. 30, 61–64.
Nagappan, R. (2012). Evaluation of aqueous and ethanol extract of bioactive medicinal plant, Cassia didymobotrya (Fresenius) Irwin and Barne by against immature stages of filarial vector, Culex quinquefasciatus Say (Diptera: Culicidae). Asian Pac. J. Trop. Biomed. 2, 707–711. doi: 10.1016/S2221-1691(12)60214-7
Nattudurai, G., Irudayaraj, S. S., Paulraj, M. G., Baskar, K., and Ignacimuthu, S. (2015). Insecticidal and repellent activities of Toddalia asiatica (L.) Lam, extracts against three major stored product pests. Entomol. Ornithol. Herpetol. 4:148.
Perumalsamy, K., Selvasundaran, R., Roobakkumar, A., Rahman, V. J., and Muraleedharan, N. (2010). Life table and predatory efficiency of Stethorus gilvifrons (Coleoptera: coccinellidae), an important predator of the red spider mite, Oligonychus coffeae (Acari: Tetranychidae), infesting tea. Exp. Appl. Acarol. 50, 141–150. doi: 10.1007/s10493-009-9290-y
Ponmurugan, P., and Baby, U. I. (2007). Evaluation of fungicides and bio-control agents against Phomopsis canker of tea under field conditions. Austr. Plant Pathol. 36, 68–72. doi: 10.1071/AP06084
Potts, S. G., Biesmeijer, J. C., Kremen, C., Neumann, P., Schweiger, O., and Kunin, W. E. (2010). Global pollinator declines: trends, impacts and drivers. Trends Ecol. Evol. 25, 345–353. doi: 10.1016/j.tree.2010.01.007
Pretali, L., Bernardo, L., Butterfield, T. S., Trevisan, M., and Lucini, L. (2016). Botanical and biological pesticides elicit a similar induced systemic response in tomato (Solanum lycopersicum) secondary metabolism. Phytochemistry 130, 56–63. doi: 10.1016/j.phytochem.2016.04.002
Radhakrishnan, B., and Prabhakaran, P. (2014). Biocidal activity of certain indigenous plant extracts against red spider mite, Oligonychus coffeae (Nietner) infesting tea. Biopest. J. 7, 29–34.
Raja, N., Elumalai, K., Jayakumar, M., Jeyasankar, A., Muthu, C., and Ignacimuthu, F. (2003). Biological activity of different plant extracts against Armyworm, Spodoptera litura (Fab.) (Lepidoptera: Noctuidae). J. Ent. Res. 27, 281–292.
Rasoul, M. A. A., Marei, G. I. K., and Abdelgaleil, S. A. M. (2012). Evaluation of antibacterial properties and biochemical effects of monoterpenes on plant pathogenic bacteria. Afr. J. Microbiol. Res. 6, 3667–3672. doi: 10.5897/AJMR12.118
Roobakkumar, A., Mariappan, S. R., Subramaniam, B.abu A., and Muraleedharan, N. (2010). Bioefficacy of certain plant extracts against the red spider mite, Oligonychus coffeae (Nietner) (Acarina: Tetranychidae) infesting tea in Tamil Nadu, India. Int. J. Acarol. 36, 255–258. doi: 10.1080/01647951003652592
Roubos, C. R., Rodriguez-Saona, C., and Isaacs, R. (2014). Mitigating the effects of insecticides on arthropod biological control at field and landscape scales. Biol. Control 75, 28–38. doi: 10.1016/j.biocontrol.2014.01.006
Roy, S., Handique, G., Muraleedharan, N., Dashora, K., Roy, S. M., Mukhopadhyay, A., et al. (2016). Use of plant extracts for tea pest management in India. Appl. Microbiol. Biotechnol. 100, 4831–4844. doi: 10.1007/s00253-016-7522-8
Roy, S., and Mukhopadhyay, A. (2012). Bioefficacy assessment of Melia azedarach (L.) seed extract on tea red spider mite, Oligonychus coffeae (Nietner) (Acari, Tetranychidae). Int J Acarol. 38, 79–86. doi: 10.1080/01647954.2011.594811
Roy, S., Mukhopadhyay, A., and Gurusubramanian, G. (2008). Susceptibility status of Helopeltis theivora Warerhouse (Heteroptera: Miridae) to the commonly applied insecticides in the tea plantation of the Sub-Himalayan Dooars area of North Bengal India. Resist. Pest Manage. Newslett. 18, 10–17.
Roy, S., Mukhopadhyay, A., and Gurusubramanian, G. (2010). Field efficacy of a biopesticide prepared from Clerodendrum viscosum Vent. (Verbenaceae) against two major tea pests in the sub Himalayan tea plantation of North Bengal, India. J. Pest Sci. 83, 371–377. doi: 10.1007/s10340-010-0306-5
Roy, S., Mukhopadhyay, A., and Gurusubramanian, G. (2011). Anti-mite activities of Clerodendrum viscosum Ventenat (Verbenaceae) extracts on tea red spider mite, Oligonychus coffeae Nietner (Acarina: Tetranychidae). Arch. Phytopathol. Plant Prot. 44, 1550–1559. doi: 10.1080/03235408.2010.510827
Roy, S., Mukhopadhyay, A., and Gurusubramanian, G. (2012). Chemical-based integrated approaches for the management of tea red spider mite, Oligonychus coffeae Nietner (Acari: Tetranychidae) in tea plantations of sub-himalayan north bengal, India. Int. J. Acarol. 38, 74–78. doi: 10.1080/01647954.2011.590154
Roy, S., Rahman, A., Phukan, A. K., and Muraleedharan, N. N. (2014). Terminalia chebula Retz. (Combretaceae): source of a botanical acaricide against Oligonychus coffeae Nietner (Acarina: Tetranychidae). nt. J. Acarol. 40, 138–144. doi: 10.1080/01647954.2014.888095
Rubia, E. G., Heong, K. L., Zalucki, M., Gonzales, B., and Norton, G. A. (1996). Mechanisms of compensation of rice plants to yellow stem borer Scirpophaga incertulas (Walker) injury. Crop Prot. 15, 335–340. doi: 10.1016/0261-2194(95)00102-6
Rusch, A., Valantin-Morison, M., Sarthou, J. P., and Roger-Estrade, J. (2010). Biological control of insect pests in agroecosystems: effects of crop management, farming systems, and seminatural habitats at the landscape scale: a review. Adv. Agron. 109, 219–259. doi: 10.1016/B978-0-12-385040-9.00006-2
Sarmah, M., Basit, A., and Hazarika, L. K. (1999). Effect of Polygonum hydropiper L. and Lantana camara L. on tea red spider mite, Oligonychus coffeae. Two Bud 46, 20–22.
Shaaban, M. M. (2001). Green microalgae water extract as foliar feeding to wheat plants. Pak. J. Biol. Sci. 4, 628–632. doi: 10.3923/pjbs.2001.628.632
Singh, R. P. (2000). “Botanicals in pest management: an ecological perspective,” in Pesticides and Environment, eds G. S. Dhaliwal and B. Singh (New Delhi: Commonwealth Publishers), 279–343.
Slama, K. (1974). “Physiological and biochemical effects of juvenoids,” in Insect Hormones and Bioanalogues, ed A. Kumar (New York, NY : Springer-Verlag), 217–281.
Snedecor, G. W., and Cochran, W. G. (1989). Statistical Methods, 8th Edn. Ames, IA: Iowa State University Press, 503.
Sola, P., Mvumi, B. M., Ogendo, J. O., Mponda, O., Kamanula, J. F., Nyirenda, S. P., et al. (2014). Botanical pesticide production, trade and regulatory mechanisms in sub-Saharan Africa: making a case for plant-based pesticidal products. Food Secur. 6, 369–384. doi: 10.1007/s12571-014-0343-7
Somchoudhury, A. K., Saha, K., Sarkar, P. K., Choudhary, A., and Bhattacharya, A. (1995). “Approaches to integrated control of red spider mite, Oligonychus coffeae (Nietner) on tea,” in Proceedings of 1995 International Tea Quality-Human Health Symposium (Shanghi).
Soylu, E. M., Kurt, S., and Soylu, S. (2010). In vitro and in vivo antifungal activities of the essential oils of various plants against tomato grey mould disease agent Botrytis cinerea. Int. J. Food Microbiol. 143, 183–189. doi: 10.1016/j.ijfoodmicro.2010.08.015
Stanley, J., and Preetha, G. (2016). Pesticide Toxicity to Non-Target Organisms. Dordrecht: Springer Netherlands. doi: 10.1007/978-94-017-7752-0
Sundararaju, D., and Sundara Babu, P. C. (1999). Helopeltis spp. (Heteroptera: Miridae) and their management in plantation and horticultural crops of India. J. Plant. Crop 27, 155–174.
Tembo, Y., Mkindi, A. G., Mkenda, P. A., Mpumi, N., Mwanauta, R., Stevenson, P. C., et al. (2018). Pesticidal plant extracts improve yield and reduce insect pests on legume crops without harming beneficial arthropods. Front. Plant Sci. 9:1425. doi: 10.3389/fpls.2018.01425
Vasanthakumar, D., Roobakkumar, A., Subramaniam, M. S. R., Kumar, P., Sundaravadivelan, C., and Babu, A. (2012). Evaluation of certain leaf extracts against red spider mite, Oligonychus coffeae Nietner (Acarina: Tetranychidae) infesting tea. Int. J. Acarol. 38, 135–137. doi: 10.1080/01647954.2011.622293
Weinzierl, R. A. (2000). “Botanical insecticides, soaps and oils,” in Biological and Biotechnological Control of Insect Pests, eds J. E. Rechcigl and N. A. Rechcigl (Washington, DC: Lewis Publishers; CRC Press; LLC), 101–120. doi: 10.1201/9781439822692.ch4
Welch, K. D., and Harwood, J. D. (2014). Temporal dynamics of natural enemy-pest interactions in a changing environment. Biol. Control 75, 18–27. doi: 10.1016/j.biocontrol.2014.01.004
Wheller, D. A., Isman, M. B., Sanches-Vindas, P. E., and Arnason, J. T. (2001). Screening of Costa Rican Trichilia species for biological activity against the larvae of Spodoptera litura (Lepidoptera: Noctuidae). Biochem. Syst. Ecol. 29, 347–358. doi: 10.1016/S0305-1978(00)00070-3
Wink, M. (1993). “Production and application of phytochemicals from an agricultural perspective,” in Phytochemistry and Agriculture, eds T. A. van Beek and H. Breteler (Oxford, UK: Clarendon Press), 171–213.
Keywords: pesticidal plants, tea, red spider mite, phytotoxic effect, natural enemies
Citation: Deka B, Babu A, Baruah C and Sarkar S (2022) Plant Extracts as Potential Acaricides for the Management of Red Spider Mite, Oligonychus coffeae Nietner (Acarina: Tetranychidae), in the Tea Ecosystem: An Eco-Friendly Strategy. Front. Agron. 4:685568. doi: 10.3389/fagro.2022.685568
Received: 25 March 2021; Accepted: 10 February 2022;
Published: 22 March 2022.
Edited by:
Jouda Mediouni-Ben Jemâa, Institut National de la Recherche Agronomique de Tunisie (INRAT), TunisiaReviewed by:
Vasanthakumar Duraikkannu, UPASI Tea Research Foundation, IndiaAbir Soltani, Institut National de la Recherche Agronomique de Tunisie (INRAT), Tunisia
Copyright © 2022 Deka, Babu, Baruah and Sarkar. This is an open-access article distributed under the terms of the Creative Commons Attribution License (CC BY). The use, distribution or reproduction in other forums is permitted, provided the original author(s) and the copyright owner(s) are credited and that the original publication in this journal is cited, in accordance with accepted academic practice. No use, distribution or reproduction is permitted which does not comply with these terms.
*Correspondence: Bhabesh Deka, bhabesh.deka@gmail.com