Screening and efficacy evaluation of antagonistic fungi against Phytophthora infestans and combination with arbuscular mycorrhizal fungi for biocontrol of late blight in potato
- 1Université catholique de Louvain, Earth and Life Institute, Mycology, Louvain-la-Neuve, Belgium
- 2Microbial Processes and Interactions (MiPI), TERRA Teaching and Research Centre, Gembloux Agro-Bio Tech, University of Liege, Gembloux, Belgium
- 3Medinbio, Wavre, Belgium
Late blight caused by Phytophthora infestans is the most devastating disease of potato crops worldwide. Control practices mostly rely on synthetic fungicides or copper-based products. In recent years, biological control agents have generated significant enthusiasm as eco-friendly sustainable alternatives. Here, the filtrates of 149 filamentous fungi and yeasts were tested in vitro against P. infestans MUCL 54981. The most effective filtrates were then combined in alginate beads with the arbuscular mycorrhizal fungus (AMF) Rhizophagus irregularis MUCL 41833 and tested in vitro for their compatibility, and finally in the greenhouse for their efficacy against P. infestans. The filtrates of Penicillium aurantiogriseum MUCL 47586, Penicillium griseofulvum MUCL 29201, Trichoderma virens MUCL 18139, and Verticillium psalliotae MUCL 18310 totally suppressed conidial germination and growth of P. infestans in vitro. In whole potato plants in vitro, only the filtrate of T. virens significantly suppressed P. infestans plant infestation. With the exception of P. griseofulvum, none of the selected filtrates affected the germination, root colonization, and spore production of R. irregularis following their combined entrapment in alginate beads. According to these results, the filtrate of T. virens was selected for the greenhouse experiment with the potato variety Annabelle. The filtrate was used as leaf spray and/or as root treatment in combination with R. irregularis entrapped in alginate beads. Root treatments with AMF alone as well as with the AMF entrapped with the filtrate of T. virens significantly reduced the area under disease progress curve compared to the non-treated plants. LC-MS analyses of the filtrate showed the production of koninginin D, gliotoxin, and koningic acid, three antifungal compounds, which could have impacted P. infestans. Conversely, foliar application of the filtrate, whether the plant was colonized by the AMF or not, did not reduce symptoms of the disease, possibly because of the greenhouse growth conditions making the potato plants more susceptible to P. infestans and counterbalancing the positive effects of the antimicrobial secondary metabolites or AMF. Our results demonstrated the potential of R. irregularis MUCL 41833 combined or not with a filtrate of T. virens MUCL 18139 into alginate beads as a biological control strategy against P. infestans.
Introduction
Late blight caused by the oomycete Phytophthora infestans is the most devastating disease of potato crops, causing huge economic losses estimated at over 6.7 billion USD worldwide per year (Haverkort et al., 2008; Haas et al., 2009; Fry et al., 2015). Currently, control of this disease is mainly achieved by repeated applications (10–16 per growing season) of chemical fungicides (Haverkort et al., 2009; Alaux et al., 2018; Ivanov et al., 2021), which not only pollute the environment and endanger human health, but also can exert strong selective pressure on the pathogen, leading to the rapid emergence of resistant or tolerant species (Schepers et al., 2018; Gonzalez-Tobon et al., 2019; Pacilly et al., 2019; Wu et al., 2021). For instance, the fight against late blight by copper (Cu) has been used intensively since the 19th century and its efficacy no longer needs to be demonstrated. However, the repeated application of Cu over time resulted in the accumulation of this compound in the soils seriously impacting the microflora (Graham et al., 1986; Brun et al., 1998). The European Commission has thus adopted the regulation (EU) 2018/1981 to restrict the use of this compound. Alternative control measures are hence desired and widely considered in many research programs based, among others, on the utilization of microorganisms [generically called biological control agents (BCAs)] or their metabolites (Wu et al., 2021).
So far, many antagonistic microorganisms (e.g., bacteria and fungi) as well as endophytes/symbionts enhancing plant growth and/or boosting plant defenses mechanisms have been investigated. Several studies have highlighted the antagonistic effects of fungi belonging to the genus Trichoderma, or to non-pathogenic Fusarium, Penicillium, Aspergillus, and Chaetomium genera against P. infestans (Roy et al., 1991; Gupta et al., 2004; Kang and Kim, 2004; Son et al., 2008; Shanthiyaa et al., 2013; Myrchiang et al., 2014; Liu and Chang, 2018), via mechanisms such as mycoparasitism, excretion of antimicrobial compounds, competition for nutrients and/or space, and induction of host plant resistance (Rashad and Moussa, 2020; Raymaekers et al., 2020). However, the direct application of non-indigenous living fungi with potential to control pest and disease could have side effects on the potato growth and yield as well as on the soil environment because it can induce a shift in the microbial community. The application of cell-free extracts/filtrates, which may contain antifungal lytic enzymes, antibiotics, volatile, and nonvolatile antifungal compounds, could thus represent alternatives to the direct application of living fungi.
Arbuscular mycorrhizal fungi (AMF) have also been considered as promising microorganisms to control late blight in potato. These fungi are obligate root symbionts that form associations with the majority of terrestrial plants (Brundrett and Tedersoo, 2018), including crops such as potato, wheat, and maize. In addition to being nutrient suppliers, improving potato yield (O’Herlihy et al., 2003; Hijri, 2016; Buysens et al., 2017), they improve resistance/tolerance of this plant against late blight (Gallou et al., 2011; Alaux et al., 2018) via several mechanisms. For instance, Rhizophagus irregularis MUCL 41833, one of the most widely used AMF in commercial products (Berruti et al., 2014; Buysens et al., 2017) induces the expression of the pathogenesis-related genes PR1 and PR2 (i.e., encoding for the PR1-a protein and for an acid β-1,3-glucanase protein respectively that act as antifungal agents) in the leaves of mycorrhizal potato plants inoculated with the pathogen, which indicates that the AMF-induced systemic resistance/tolerance to this pathogen is associated with the stimulation of these two PR genes (Gallou et al., 2011). In controlled conditions and more importantly in field, the disease progression was delayed in AMF-colonized potato plants, especially when the disease pressure was low (O’Herlihy et al., 2003; Alaux et al., 2018). In other plants, it was reported that an accumulation of different defense compounds, such as phytoalexins, suberin, callose, lignin, phenolic compounds, terpenoids, and sulfur amino acids, was observed in mycorrhizal plants (Hohmann and Messmer, 2017; Jacott et al., 2017; Hill et al., 2018).
Whereas literature has amply documented the biocontrol of late blight of potato using plant or bacterial extracts (Quintanilla et al., 2002; Stephan et al., 2005; Olanya and Larkin, 2006; Dorn et al., 2007; Wang et al., 2007; Hultberg et al., 2010; Moushib et al., 2013; Bengtsson et al., 2015; Hunziker et al., 2015; Rogozhin et al., 2020; Rani et al., 2021), fewer studies have reported biocontrol of potato late blight and control of the pathogen growth with metabolites extracted from fungi. For instance, the application of oosporein, an antifungal metabolite isolated from Verticillium psalliotae, fusaric acid from F. oxysporum, and culture extracts from Trichoderma and Chaetomium species (i.e., C. cochliodes and C. aureum) have been reported to affect the growth of P. infestans in vitro (Nagaoka et al., 2004; Son et al., 2008; Bae et al., 2016; Linkies et al., 2021). Similarly, the viridiofungin A produced by the isolate T23 of T. harzianum suppressed the germination of P. infestans sporangia cultured in vitro (El-Hasan et al., 2009), and more recently, the application of volatile organic compounds emitted by Trichoderma strains T41 and T45 inhibited the in vitro mycelial growth of P. infestans grown on medium and on potato tubers (Elsherbiny et al., 2020). However, to the best of our knowledge, the application of fungal extracts to control late blight in planta was only demonstrated by Shanthiyaa et al. (2013). These authors reported a significant decrease in late blight infection under field conditions via the application of a culture filtrate of C. globosum isolate Cg-6. The combination of culture filtrates of antagonistic fungi with AMF and their application to roots and leaves may also represent a novel approach to control late blight, which has never been considered to date.
The present study aims at investigating the effects of the combination between the AMF R. irregularis MUCL 41833 and filtrates of fungal BCA candidates to protect potato against late blight. First, fungal BCAs whose culture filtrates are efficient against P. infestans were selected out of a collection of 149 candidates. Secondly, the most efficient culture filtrates were tested for their compatibility with the AMF. Finally, the culture filtrate of T. virens (the most efficient of the 149 candidates) was selected and tested with the AMF on late blight symptoms outbreak in the sensitive potato var. Annabelle, under greenhouse conditions.
Materials and methods
Biological material
Biological control agent candidates
A list of 149 fungal BCA candidates (filamentous fungi and yeast) was established (Table S1) based on an extensive literature search of the major fungal BCAs of P. infestans reported to date. One hundred and thirty-nine BCA candidates were obtained from the Mycothèque of the Université catholique de Louvain (MUCL12, Belgium) and 10 from the culture collection of Westerdijk Fungal Biodiversity Institute2 of Utrecht (Netherlands). Most of them were originally isolated from soils, plant debris, and decaying stumps. Some BCA candidates were also selected on the basis of closely related fungal species or genera that were shown to have biological control activities on other major plant fungal pathogens.
The selected BCA candidates were maintained on sterilized (121°C for 15 min) potato dextrose agar (PDA, Oxoid, United Kingdom) medium in Petri plates (90 mm diameter, Sarstedt, Germany) in the dark at 25°C. Each BCA candidate was sub-cultured every 2 months by transferring five plugs of approximately 5 mm diameter from one mother Petri plate that showed the best development to three novel Petri plates.
Phytophthora infestans
P. infestans Mont. De Bary MUCL 54981 was supplied by the MUCL and was maintained on sterilized (121°C for 15 min) PDA medium. It was incubated in 90-mm-diameter Petri plates at 20°C in the dark and produced enough sporangia after 1 month to be used in the antagonism assays with the BCA candidates above.
Arbuscular mycorrhizal fungus
R. irregularis (Blaszk., Wubet, Renker & Buscot; C. Walker & A. Schüßler) MUCL 41833 was provided by the Glomeromycota in vitro collection (GINCO, Belgium) on root organ cultures (ROCs) of carrot (Daucus carota L.) clone DC2.
In vitro production of the AMF—The AMF was cultured on ROCs of carrot clone DC2 in Petri plates (90 mm diameter) containing sterilized (121°C for 15 min) Modified Strullu-Romand (MSR) medium (Declerck et al., 1998) solidified with 3 g L−1 Phytagel (Sigma-Aldrich, St. Louis, USA), following the method described by Cranenbrouck et al. (2005). The Petri plates were incubated in inverted position in the dark at 27°C. After 4 months, several thousands of spores were obtained per Petri plate.
In vivo production of the AMF—The AMF was mass-produced in pots under controlled greenhouse conditions as follows: isolated spores and colonized root fragments of an in vitro culture of the AMF was associated to maize plants (Zea mays L. cultivar ES Ballade) in a 5-L pot containing sterilized lava stone (DCM, Belgium) as growth medium. After 3 months of cultivation at 21°C/18°C (day/night), a relative humidity (RH) varying from 40% to 70%, a photoperiod of 16 h day–1, and a photosynthetic photon flux (PPF) of 120 μmol s–1 m–2, a high production of AMF propagules was obtained in the substrate as well as in the roots of the maize plants.
Potato plants
Solanum tuberosum L. var. Bintje [very sensitive to late blight according to the scale of César and Ryckmans (2021)] was provided by the “Station de Haute Belgique” (Libramont, Belgium) as in vitro plants. The plants were micropropagated every 4 weeks in culture microboxes, sealed with breathing filters in the lid (ref: 0118/120 + OD118, SacO2, Belgium). The plants were grown on sterilized (121°C for 15 min) Murashige and Skoog (MS) (Duchefa, Netherlands) medium supplemented with 20 g L–1 sucrose and solidified with 4.2 g L–1 Gelrite™. The microboxes were placed in a growth chamber (Snijders Scientific B.V., Netherlands), under a temperature of 20/18°C (day/night), an RH of 75%, a photoperiod of 16 h day−1, and a PPF of 50 μmol s–1 m–2.
Solanum tuberosum L. var. Annabelle (sensitive to late blight according to the scale of Arvalis—http://www.fiches.arvalis-infos.fr) was provided by a local organic potato farmer in Belgium as tubers. The tubers were maintained at 22°C in the dark for 2 weeks, the time necessary for their sprouting.
Experimental procedure
Experiment 1: Selection of Fungal BCA candidates whose culture filtrates have antagonistic effects against P. infestans MUCL 54981
Production of fungal filtrates
The BCA candidates were first cultured on solid sterilized (121°C for 15 min) PDA medium at 25°C in the dark during 2 weeks. Three successive sub-cultures were done at 10-day intervals to produce three independent culture batches, with the objective to ascertain the repeatability of results.
For each BCA candidate and batch, five cultures plugs were transferred into 50 ml of liquid Potato Dextrose Broth (PDB, Sigma Aldrich, USA) medium and grown under continuous shaking (80 rpm min−1) at 25°C in the dark, until growth arrest was visually observed (~18 days), theoretically corresponding to the phase of secondary metabolite (SM) production. The liquid culture of each BCA candidate was subsequently blended and grounded twice for 30 s at 20,000 rpm with a sterilized (121°C for 15 min) ultra-turax (Omni Mixer Homogenizer, Omni International, CA). The material was then centrifuged for 3 min at 4,000 rpm. The supernatant was finally collected and filtered through two glass fiber membranes of 1.6 and 0.70 μm porosity (Cytiva Whatman™, USA) to remove cellular debris and through a 0.22-µm filtration membrane (Acrodisc, GelmanSciences, USA) to remove microbial contaminants, before freezing at −20°C until use.
Impact of culture filtrates of selected BCA candidates on the growth inhibition of P. infestans MUCL 54981 measured by absorbance
The culture filtrates of the 149 BCA candidates were tested on growth inhibition of P. infestans by absorbance in 96-well microplates (96-wells-F, sterile, VWR, Radnor, PA, USA). Forty-five microliters of filtrate from each of the three different culture batches was added in the wells of the microplates and covered with 105 μl of sterilized (121°C for 15 min) PDA medium (cooled at 45°C). Sterilized (121°C for 15 min) liquid PDB medium was used as control. The microplate wells were then inoculated with 40 μl of P. infestans suspension (1 × 105 sporangia ml−1 water), and incubated in the dark at 20°C to monitor daily the fungal growth on the medium by measuring the absorbance [optical density (OD)] at 620 nm using a microplate absorbance reader (Multiskan bichromatic, Thermo Labsystems, Helsinki, Finland) until the controls (on each microplates) reached an absorbance of 1.0 ± 0.1.
Percentage of growth inhibition (%GI) of P. infestans in the presence of the filtrates was calculated as follows:
where:
- %GI: percentage inhibition,
- Abs-BCA: absorbance without BCA filtrate (control),
- Abs+BCA: absorbance with BCA filtrate,
- d0: absorbance at day 0,
- dx: absorbance at day x,
- Control: liquid PDB medium control
The filtrates exhibiting a growth inhibition of P. infestans higher than 95% were selected for further testing. In case of total absence of growth, the germination of sporangia was checked under a compound microscope (Olympus BH2, Olympus Optical, GmbH, Hamburg, Germany) at 20–40× magnifications.
Impact of culture filtrates of selected BCA candidates on the growth inhibition of P. infestans MUCL 54981 measured by colony surface area
The culture filtrates of the 17 BCA candidates selected above (exhibiting a growth inhibition of P. infestans by absorbance higher than 95%) were tested on the growth inhibition of P. infestans by measuring the growth area of the pathogen in comparison to a control without filtrates. A 5-mm-diameter plug of P. infestans was placed in the center of a Petri plate (90 mm diameter, Sarstedt, Germany) containing PDA, and incubated at 20°C in the dark. After 7 days of incubation, the growth area of P. infestans was measured. At that time, 6 ml of filtrate of each BCA candidate grown in PDB was applied on the surface of the colony. Sterilized (121°C for 15 min) liquid PDB medium was used as control. Five replicates were considered per BCA candidate filtrate from a single subculture. The area covered by P. infestans was assessed under a stereomicroscope (Olympus SZ61) at ×10–40 magnification. The percentage of growth inhibition (%GI) of P. infestans was evaluated when the pathogen in the control treatment reached the edge of the Petri plate, as follows:
where:
- GA Pinf-BCA: growth area of P infestans without BCA filtrate (control),
- GA Pinf+BCA: growth area of P infestans with BCA filtrate,
- T0: area at day 0,
- T1: area at day x, i.e., when the control reached the edge of the Petri plate
The filtrates exhibiting a total growth inhibition of P. infestans were selected for further testing.
Impact of culture filtrates of selected BCA candidates on the reduction of leaf symptoms caused by P. infestans MUCL 54981
The culture filtrates of the five BCA candidates selected above (exhibiting a total growth inhibition of P. infestans measured by colony surface area) were tested on the percentage of the leaf area covered by P. infestans symptoms in comparison to a control without filtrates. Potato plants var. Bintje were grown in vitro in culture microboxes and placed in a growth chamber until they reached the six-leaf stage (plants of about 10 cm height), approximately within 4 weeks. The plants were subsequently sprayed with 2 ml of a mixture composed of polysorbate 80 (0.5%, v/v) and each of the five BCA candidate filtrates. The plants were then transferred to a growth chamber (Snijders Scientific B.V., Netherlands), under a temperature of 20/18°C (day/night), an RH of 75%, a photoperiod of 16 h day−1, and a PPF of 50 μmol s–1 m–2. After 24 h of incubation, 0.2 ml of P. infestans suspension (103 sporangia µl−1) was sprayed on the leaves of each plant. The plants were incubated again in the growth chamber under the same growth conditions. Ten replicates were considered per BCA filtrate. After 2 days of incubation, half of the plants was sprayed a second time with 2 ml of the mixture of BCAs filtrates and polysorbate 80 (0.5%, v/v), while the other half did not receive a second spraying. The plants were incubated in the growth chamber under the same culture conditions as described above. Sterilized (121°C for 15 min) liquid PDB medium was used as a control. Five replicates were considered per BCA filtrate.
After 15 days of incubation, four leaves from each plant were randomly selected to assess the percentage of leaf area (%LA) covered by P. infestans symptoms using the assessment keys developed by James (1971) for late blight of potato.
Experiment 2: Effects of culture filtrates of selected BCA candidates on the AMF R. irregularis MUCL 41833
Effect of culture filtrates of selected BCA candidates on propagule germination, root colonization, and extraradical growth of R. irregularis MUCL 41833 entrapped in alginate beads
Five-month-old in vitro produced cultures of the AMF, containing thousands of spores and mycorrhizal roots, were blended twice in 100 ml of sterilized (121°C for 15 min) deionized water for 30 s at 20,000 rpm with a sterilized (121°C for 15 min) ultra-turax. The mixture was subsequently filtered on a sterilized (121°C for 15 min) nylon mesh (41 µm) before being entrapped in alginate beads with each of the five previously selected fungal BCA candidate filtrates as follows: the AMF propagules were mixed in equal volume (v:v, 1:1) with the filtrates in a 4% (w/v) sterilized (121°C for 15 min) sodium alginate solution (Sigma-Aldrich, UK). Alginate droplets of a volume of 100 µl were pipetted manually and dropped in a sterilized (121°C for 15 min) solution of 0.1 M CaCl2 kept under agitation for 30 min. The alginate beads were then rinsed with sterilized (121°C for 15 min) deionized water and stored in 90-mm-diameter Petri plates. Alginate beads containing only AMF propagules in PDB were considered as control. For each of the treatments, 20 alginate beads each containing 100 ± 10 propagules were considered. The percentage of infective beads (%PIB), i.e., beads containing at least one germinated AMF propagule (Declerck et al., 1996), was determined 4 weeks after incubation on sterilized (121°C for 15 min) MSR medium at 27°C in the dark under a stereomicroscope (×6.7–40 magnification).
Beads containing germinated propagules were placed in the vicinity of ROCs of carrot (i.e., two beads per Petri plate) in 90-mm-diameter Petri plates containing 40 ml of MSR medium. The Petri plates were subsequently incubated at 27°C in the dark, and root colonization and spore production were evaluated after 12 weeks. Five Petri plates were set up per treatment. The number of spores was assessed using a 10-mm grid marked on the bottom of each Petri plate to form 10-mm squares, following the method described by Declerck et al. (2004). The measurements were conducted under a dissecting microscope at magnifications of ×6.7 and ×40. The roots were then collected following dissolution of the MSR medium with citrate buffer (Doner and Bécard, 1991) and stained (Phillips and Hayman, 1970) to estimate total colonization (TC%) under a bright-field light microscope (Olympus BH-2, Olympus Optical GmbH) at ×50–250 magnification using the method of McGonigle et al. (1990). For each replicate, 300–350 root intersections were assessed.
Impact of culture filtrates of selected BCA candidates on leek root colonization by R. irregularis MUCL 41833
The effect of increasing concentrations of each of the five previously selected fungal BCA candidate filtrates was evaluated on the ability of R. irregularis propagules to colonize leek roots under greenhouse conditions via a dose–response relationship (adapted from Declerck et al., 1996). Briefly, each selected BCA candidate filtrate was entrapped in 100 µl of alginate beads at increasing concentrations (10, 25, 50, and 75 μl) with in vivo produced AMF propagules (30 ± 5). Alginate beads containing only AMF propagules were considered as the control.
Seeds of leek (Allium porrum L. var. bleu de Solaise) were surface disinfected by soaking in 6% sodium hypochlorite for 2 min and subsequently germinated on sterilized (121°C for 15 min) wet paper. After 10 days, 10 plants were transplanted in plastic multipots (4 cm diameter) containing a mixture (60 g) of sterilized (121°C for 15 min) calcinated clay (Absorb attapulgite 6-30 or 8-16, Oil-Dri, UK), fine quartz (N°4, Euroquartz, Belgium), and coarse quartz (N°1, Euroquartz, Belgium) (w:w:w, 1:1:1) gently mixed with 10 alginate beads with one of the filtrate concentration. Five replicates were considered per BCA filtrate concentration. The multipots were then transferred to the growth chamber (Snijders Scientific B.V., Netherlands), under a temperature of 20/18°C (day/night), an RH of 65%, a photoperiod of 16 h day−1, and a PPF of 24.5 μmol s–1 m–2. Plants were watered with deionized water twice a week. After 5 weeks, the plants were harvested and roots were cleaned and stained with ink according to the protocol described by Phillips and Hayman (1970). Each root system was observed individually for the presence/absence of AMF colonization. Data were expressed as percentage of the mycorrhizal plants (%MP) per pot for each treatment.
Experiment 3: Evaluation of the combined effects of R. irregularis MUCL 41833 and a culture filtrate of Trichoderma virens MUCL 18139 on the disease severity caused by P. infestans MUCL 54981 on potato var. Annabelle under controlled greenhouse conditions
Tubers of potato var. Annabelle were planted in 2-L pots containing a sterilized (121°C for 15 min) substrate composed of potting soil (DCM, Belgium), vermiculite (Pull Rhenen, Netherlands), and sand (quartz N°4, Euroquartz, Belgium) (w:w:w, 1:1:1). A single sprout was kept on each tuber that was placed upwards, 10 cm deep in the substrate. At the start of the experiment (Time 0), 32 tubers were inoculated with R. irregularis propagules (100 ± 10) entrapped in 100 µl of alginate beads (treatment AM). An equivalent number of tubers was similarly inoculated with the AMF supplemented with 10 µl of filtrate of T. virens MUCL 18139 (treatment AM + vir). Forty tubers inoculated with empty alginate beads were included as control (treatment Ctrl—see upper part of Figure 1). The alginate beads were distributed 2 cm around the sprout to facilitate the association of the AMF propagules with the emerging roots. The pots were randomly disposed on tables and maintained under greenhouse conditions [20°C/18°C (day/night), an RH of 70%, a photoperiod of 16 h day–1, and a PPF of 120 μmol m–2 s–1] for a period of 8 weeks. The plants were watered twice a week with 500 ml of deionized water and received 200 ml of Hoagland low-P once a week (see Garcés-Ruiz et al., 2017).
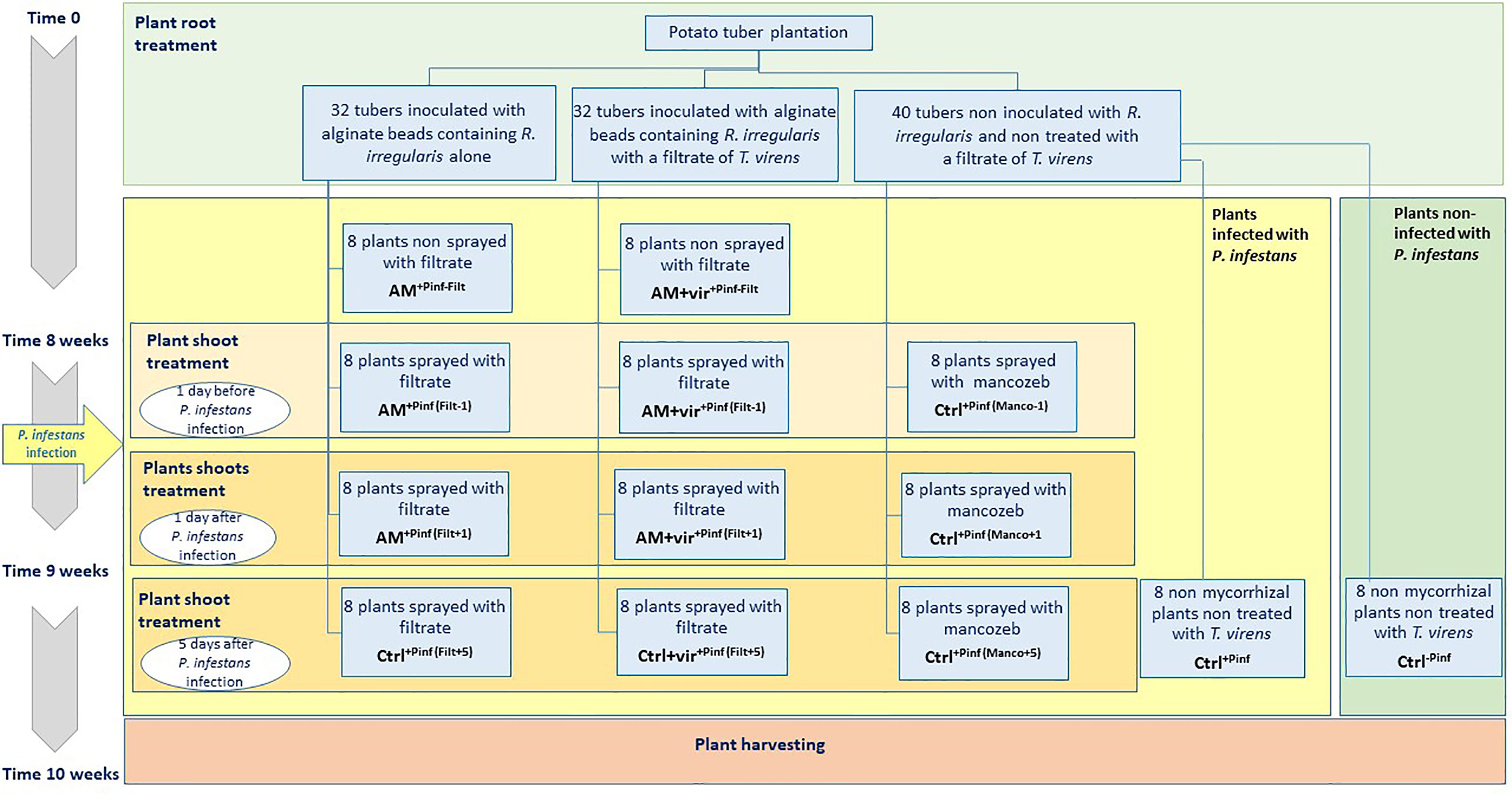
Figure 1 Schematic representation of the experimental design of experiment 3. Evaluation of the combined effects of a culture filtrate of T. virens MUCL 18139 and R. irregularis MUCL 41833 on the disease severity caused by P. infestans MUCL 54981 on potato var. Annabelle under controlled greenhouse conditions.
After 8 weeks of culture, five plants inoculated with the AMF entrapped alone were randomly selected to check the root colonization. At that time, the three treatments above were divided in sub-groups sprayed either with 6 ml (200 L ha−1) of filtrate of the BCA candidate (for the treatments AM and AM+vir) or mancozeb [Dithane Neotec 75 WG (a.s. 75% mancozeb—for the Ctrl treatment), a classical chemical fungicide applied at a concentration of 200 L ha−1].
In detail, the Ctrl treatment was divided into five groups of eight plants as follows: eight plants were not inoculated with P. infestans (Ctrl-Pinf), eight plants were inoculated with P. infestans (Ctrl+Pinf), eight plants were sprayed with mancozeb 1 day before inoculation of P. infestans (Ctrl+Pinf (Manco-1)), eight plants were sprayed with mancozeb 1 day after inoculation by P. infestans (Ctrl+Pinf (Manco+1)), and eight plants were sprayed with mancozeb 5 days after inoculation by P. infestans (Ctrl+Pinf (Manco+5)).
The AM treatment was divided into four groups of eight plants as follows: eight plants were not sprayed with the filtrate (AM+Pinf -Filt), eight plants were sprayed with the filtrate 1 day before inoculation of P. infestans (AM+Pinf (Filt-1)), eight plants were sprayed with the filtrate 1 day after inoculation by P. infestans (AM+Pinf (Filt+1)), and eight plants were sprayed with the filtrate 5 days after inoculation by P. infestans (AM+Pinf (Filt+5)).
The AM+vir treatment was divided into four groups of eight plants as follows: eight plants were not sprayed with the filtrate (AM+vir+Pinf -Filt), eight plants were sprayed with the filtrate 1 day before inoculation of P. infestans (AM+vir+Pinf (Filt-1)), eight plants were sprayed with the filtrate 1 day after inoculation by P. infestans (AM+vir+Pinf (Filt+1)), and eight plants were sprayed with the filtrate 5 days after inoculation by P. infestans (AM+vir+Pinf (Filt+5)).
All the plants were sprayed with 6 ml of a suspension of P. infestans MUCL 54981 sporangia (250 sporangia µl−1 water), except the negative control plants (i.e., treatment Ctrl-Pinf) that were sprayed with 6 ml of PDB medium. At that time, the RH was increased to 90% so that the growing conditions were optimal for P. infestans. The disease development was assessed in each treatment 0, 5, 10, and 15 days post infection by P. infestans by the area under disease progress curve (AUDPC). The AUDPC was calculated according to the equation of Madden and Campbell (1990) as follows:
where:
Yi = disease severity at the ith observation,
Xi = time at the ith observation,
ni = number of times,
i = order index for the times
Fifteen days after inoculation with the pathogen, the plants were harvested. The shoots’ and roots’ fresh weights (SFW and RFW, respectively) and the tubers’ fresh weight (TFW) of each plant were evaluated. Shoots and roots were then oven-dried at 100°C for 2 days until dry weights remained stable and shoots’ and roots’ dry weights (SDW and RDW, respectively) were evaluated.
Percentage of root colonization was assessed following staining (Phillips and Hayman, 1970). Total colonization (TC%) and proportion of root length colonized by vesicles/spores (V%) and arbuscules (A%) were estimated under a bright-field light microscope (Olympus BH-2, Olympus Optical GmbH) at ×50–250 magnification using the method of McGonigle et al. (1990). For each replicate, 300–350 root intersections were assessed.
LCMS analysis of T. virens MUCL 18139 filtrate
Culture filtrates of T. virens were analyzed for their content in antimicrobial compounds by UPLC-mass spectrometry using an Agilent 1290 Infinity II HPLC system coupled to a mass detector (Jet Stream ESI‐qTOF 6530, Agilent) set up in positive mode and with the following parameters: capillary voltage: 3.5 kV; nebulizer pressure: 35 psi; drying gas: 8 L min−1; drying gas temperature: 300°C; flow rate of sheath gas: 11 L min−1; sheath gas temperature: 350°C; fragmentor voltage: 175 V; skimmer voltage: 65 V; octopole RF: 750 V. Accurate mass spectra were recorded in the range of m/z =50–1,700. Analyses were performed using an C18 Acquity UPLC BEH column (2.1 × 50 mm × 1.7 μm; Waters) and 0.1% formic acid (solvent A)/acetonitrile acidified with 0.1% formic acid (solvent B) as mobile phase with constant flow rate at 0.6 ml min−1 and column temperature set at 40°C. First, 2% solvent B was applied during 3 min before increasing to 70% in 10 min. Then, 100% solvent B was applied for 3 min before going back to initial conditions. Masshunter Qualitative Analysis software (Agilent) was used for data analysis.
Statistical analysis
Statistical analyses were performed with JMP 16.0 and SAS 9.4 statistical software (SAS Institute Inc., Cary, NC, United States). Levene and Shapiro–Wilk tests were run prior to the analysis to confirm homogeneity of variance and normality of distribution. To fulfill the assumptions of the method, data were log10 or root transformed, prior to the analysis. If data remained non-normal after transformation, they were analyzed by Kruskal–Wallis. Dunn test, a non-parametrical test for pairwise or with a control comparison, was used to identify significant differences (p ≤ 0.05) between treatments.
Data for growth inhibition percentage of P. infestans MUCL 54981 were analyzed by Kruskal–Wallis non-parametrical test followed by a pairwise comparison Dunn test (p ≤ 0.05). Data for in vitro potato plant infection percentage were analyzed using one-way ANOVA followed by a pairwise comparison post-hoc Tukey HSD test (p ≤ 0.05). Data for R. irregularis MUCL 41833 spore production as well as carrot root colonization percentages were analyzed using a one-way ANOVA followed by comparison with the control using a Dunnett-HSD test. Finally, data for plant infection (i.e., AUDPC), plant growth parameters [i.e., SDW, RDW, total dry weight (TDW), and TFW], and root colonization were analyzed using one-way ANOVA followed by a pairwise comparison post-hoc Tukey HSD or Dunnett’s test (p ≤ 0.05).
Results
Experiment 1: Selection of fungal BCA candidates whose culture filtrates have antagonistic effects against P. infestans MUCL 54981
In a first test, the impact of culture filtrates of different BCA candidates was evaluated on the growth of P. infestans by absorbance in 96-well microplates. The measurement was taken when the absorbance in the control (i.e., without filtrate) reached a value of 1.0 ± 0.1. Culture filtrates of the 149 BCA candidates were tested (Figure S1) and 17 of them (i.e., Aspergillus flavus MUCL 35049, Aspergillus niger MUCL 52577, Chaetomium globosum MUCL 20345, Emericella nidulans MUCL 35515, Fusarium proliferatum MUCL 53645, Laetiporus sulphureus MUCL 55875, Penicillium aurantiogriseum MUCL 47586, Penicillium frequentans MUCL 13766, Penicillium funiculosum MUCL 40813, Penicillium griseofulvum MUCL 29201, Penicillium purpurogenum MUCL 47592, Sporothrix insectorum MUCL 19334, Talaromyces flavus MUCL 14302, Trichoderma arundinaceum CBS 113214, Trichoderma pseudokoningii MUCL 11426, T. virens MUCL 18139, and V. psalliotae MUCL 18310) inhibited the growth of P. infestans by more than 95% in three independent batches. In contact with those filtrates, most of the sporangia of P. infestans did not germinate and were often empty (Figure 2).
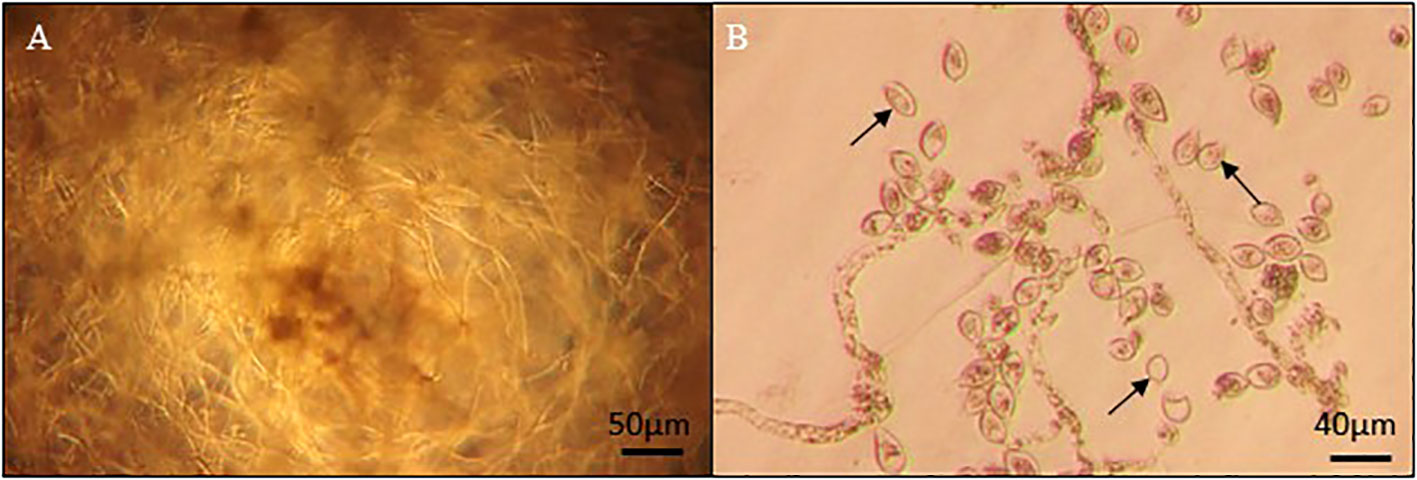
Figure 2 Phytophthora MUCL 54981 culture grown in 96-well microplates (A) on PDB medium without culture filtrate, showing a dense growing hyphae (B) on PDA with culture filtrate of P. aurantiogriseum MUCL 47586, showing the absence of hyphal growth and presence of empty sporangia (arrow).
In a second test, the culture filtrates of the 17 BCA candidates above were evaluated on surface area inhibition of 7-day-old cultures of P. infestans. After 15 days of incubation, a significant effect (p ≤ 0.001) of the different culture filtrates was noticed on the growth of P. infestans. Ten out of the 17 culture filtrates inhibited by more than 80% the growth of P. infestans (Figure 3). Interestingly, 10 days later, we observed that the colonies of P. infestans treated with a culture filtrate of A. flavus MUCL 35049, L. sulphureus MUCL 55875, P. purpurogenum MUCL 47592, T. arundinaceum CBS 113214, and T. flavus MUCL 14302 resumed their growth. Conversely, the growth of P. infestans treated with a culture filtrate of A. niger MUCL 52577, P. aurantiogriseum MUCL 47586, P. griseofulvum MUCL 29201, T. virens MUCL 18139, and V. psalliotae MUCL 18310 was completely inhibited and no regrowth was observed thereafter. The culture filtrate of those five BCA candidates were selected for further tests.
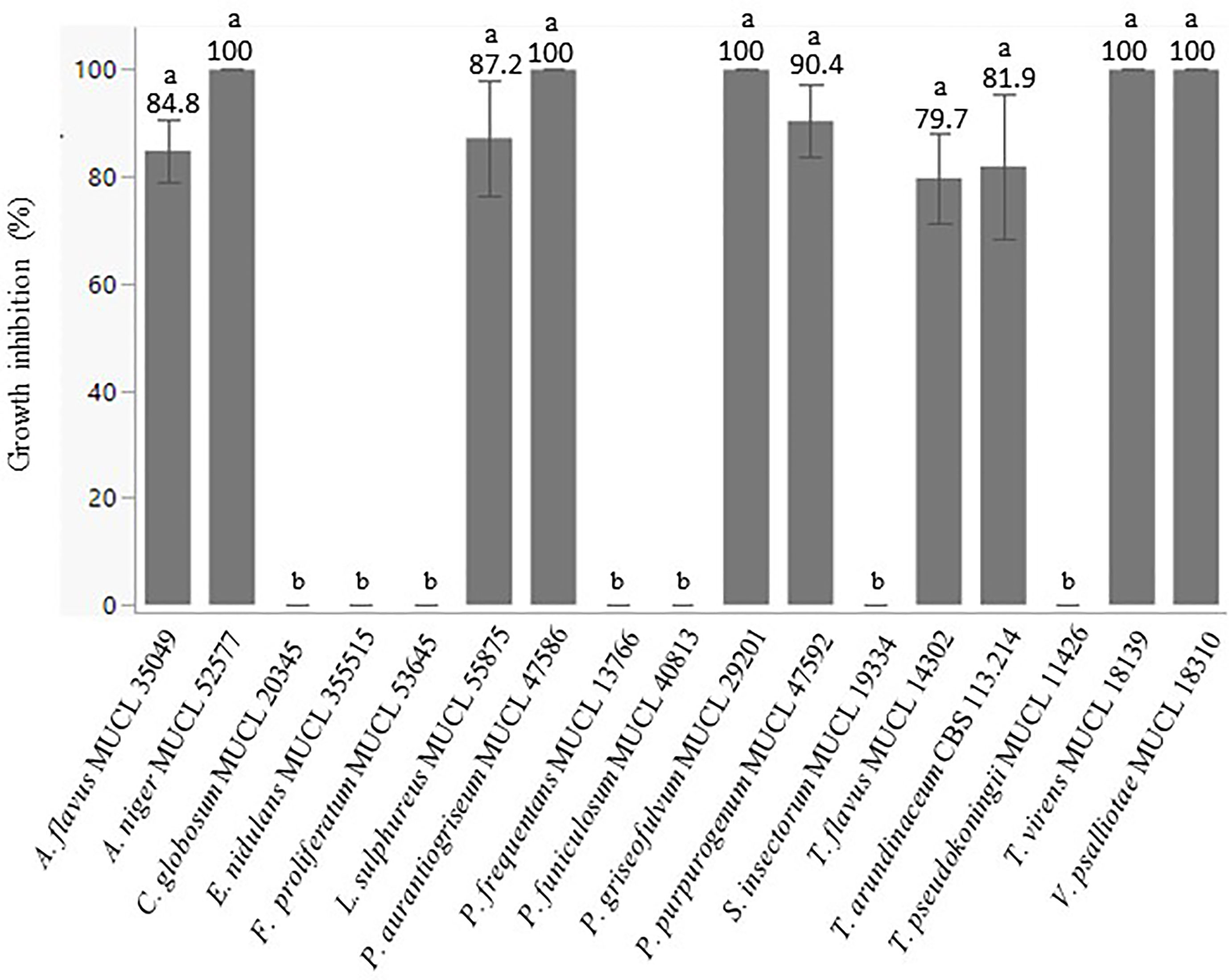
Figure 3 Percentage of growth inhibition of P. infestans MUCL 54981 colonies induced by culture filtrates of different fungal BCA candidates. Values are expressed as the mean ± SD (n = 5). Different lowercase letters show significant differences between the treatments (p ≤ 0.05; Dunn test).
In a third test, the impact of culture filtrates of the selected BCA candidates on the reduction of potato leaf symptoms caused by P. infestans was evaluated. Potato plants grown in vitro were sprayed with the culture filtrates of the five selected BCA candidates 1 day before and 2 days after inoculation of P. infestans. The percentage of leaf area (%LA) covered by symptoms of P. infestans was evaluated in the presence/absence of the culture filtrates (Figure 4). No plant survived with the filtrate of A. niger MUCL 52577. After 15 days of incubation, a significant effect (p = 0.003) of the culture filtrates of the different BCA candidates was noticed. The culture filtrate of P. griseofulvum MUCL 29201, applied prior to inoculation of the pathogen, decreased by 70% the %LA covered by symptoms as compared to the control, while the second spraying (2 days after inoculation of P. infestans) killed the plants. The culture filtrates of P. aurantiogriseum MUCL 47586 and V. psalliotae MUCL 18310 did not show any difference of %LA covered by symptoms as compared to the control, whatever the time of application. The culture filtrate of T. virens MUCL 18139 applied prior to inoculation of the pathogen did not impact the %LA covered by symptoms, even if the symptoms of the pathogen were reduced by 58%, while at the second spraying (i.e., after inoculation by the pathogen), a significant decrease was noticed in %LA covered by symptoms of the pathogen compared to the control.
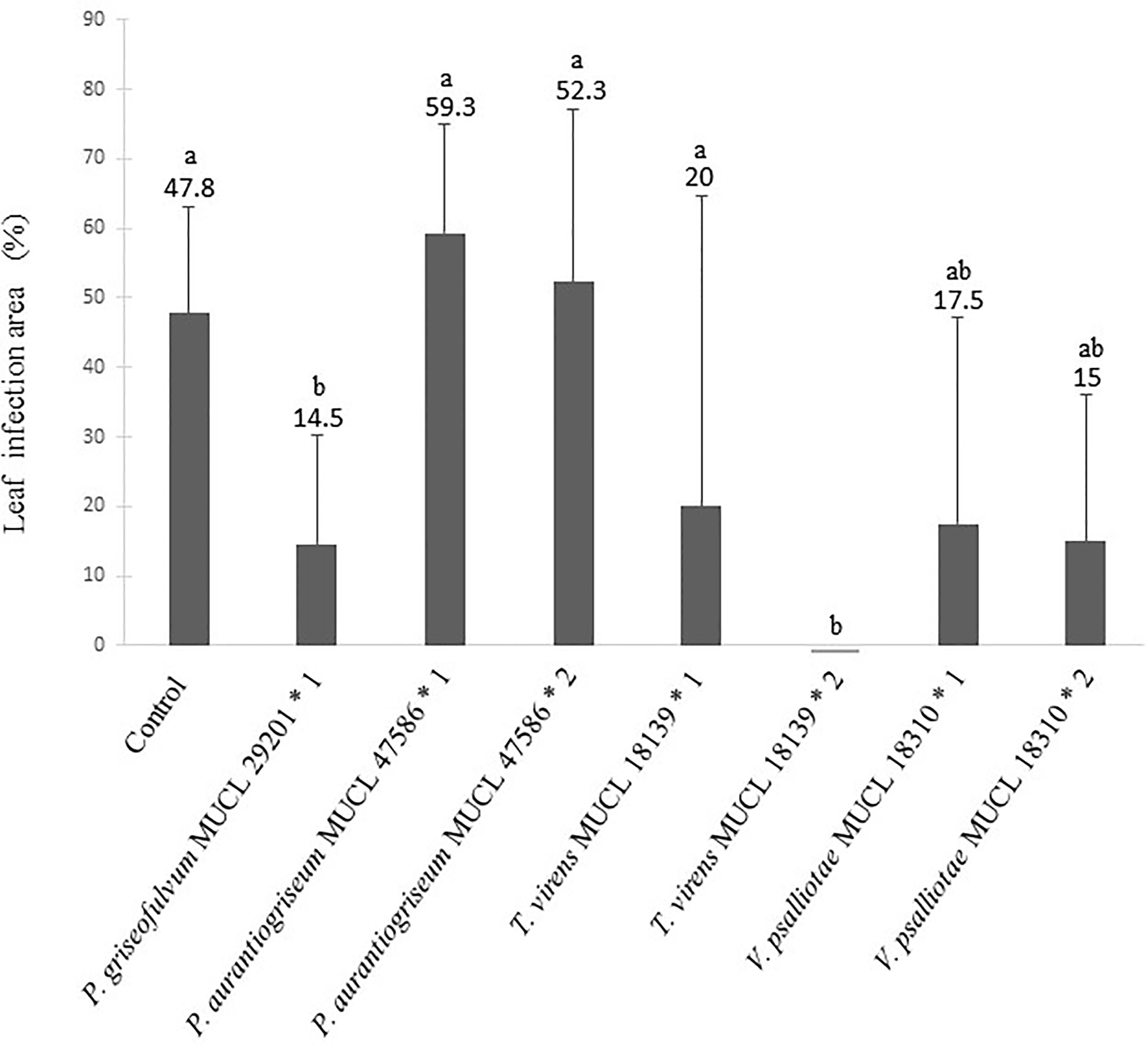
Figure 4 Percentage of leaf infection area (%LA) of potato plants treated or not with culture filtrates of fungal BCA candidates applied 1 day prior (* 1) or 1 day after (* 2) inoculation with P. infestans MUCL 54981. Values are expressed as the mean +SD (n = 5). Different lowercase letters show significant difference between the treatments (p ≤ 0.05; Tukey HSD).
Experiment 2: Effects of culture filtrates of selected BCA candidates on the AMF R. irregularis MUCL 41833
In a first test, the effect of culture filtrates of four fungal BCA candidates selected from Experiment 1 was evaluated on propagule (i.e., spores and colonized root fragments) germination, root colonization, and extraradical spore production of R. irregularis entrapped in alginate beads. Whatever the culture filtrate entrapped with the AMF propagules, the percentage of infective beads (%PIB) was always 100% (Table S2). After 12 weeks of association with carrot roots in vitro, root colonization and spore production were observed (Table 1). A significant effect of the treatment was noticed on the total colonization (TC%) (p = 0.0001). Compared to the control, only the in vitro culture of R. irregularis initiated from AMF propagules entrapped with the culture filtrate of P. griseofulvum MUCL 29201 presented a significantly lower TC% (Table 1). Conversely, no significant effect of the culture filtrate was noticed on the number of spores produced (p = 0.061). Interestingly, the culture filtrate of T. virens MUCL 18139 that showed the highest effect on P. infestans (Experiment 1, test 3) did not impact root colonization and spore production of R. irregularis compared to the control.
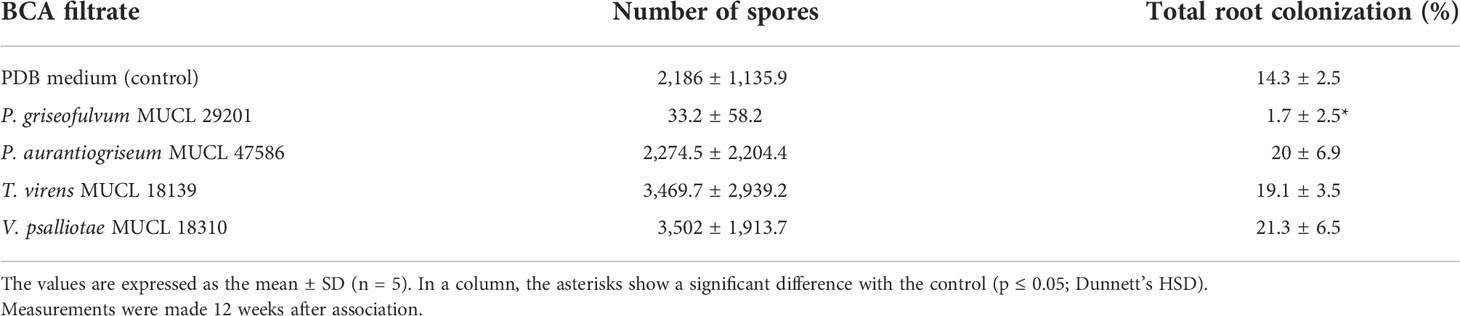
Table 1 Number of spores and percentage of total root colonization of carrot root organ cultures associated with R. irregularis MUCL 41833 propagules entrapped in alginate beads with PDB medium (control) or with culture filtrates of different fungal BCA candidates.
In a second test, a dose–response assessment was conducted to evaluate the dose of filtrate required to impact the colonization of a population of plants, estimated by the percentage of the mycorrhizal plants (%MP) (Table 2). After 5 weeks of culture, the %MP reached 88.55% in the control treatment (i.e., the beads containing only the AMF). The %MP did not differ significantly between the control treatment and the treatment containing different doses of culture filtrates of P. aurantiogriseum MUCL 47586. For the culture filtrate of T. virens MUCL 18139, only the 75-µl dose significantly reduced the %MP as compared to the control. In contrast, for the culture filtrate of V. psalliotae MUCL 18310, only the 10-µl dose significantly reduced the %MP as compared to the control. Finally, for the culture filtrate of P. griseofulvum MUCL 29201, the 50-µl and 75-µl dose significantly reduced the %MP as compared to the control.
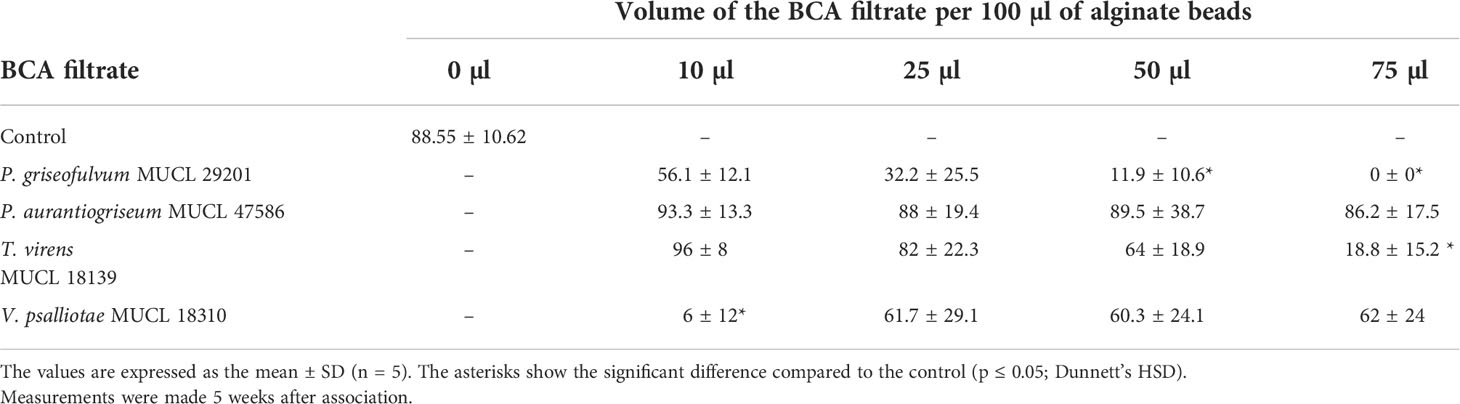
Table 2 Percentage of mycorrhizal plants (%MP) inoculated with alginate beads containing propagules of R. irregularis MUCL 41833 alone (control) or with different concentrations of culture filtrates of the fungal BCA candidates.
Experiment 3: Evaluation of the combined effects of R. irregularis MUCL 41833 and culture filtrate of T. virens MUCL 18139 on the disease severity caused by P. infestans MUCL 54981 on potato var. Annabelle under controlled greenhouse conditions
Plant growth parameters
Throughout the experiment, the potato plants developed normally. At the end of the experiment (i.e., 2 weeks after plant infection by P. infestans), a significant effect of the treatments was noticed on SDW, RDW, TDW, and TFW (p< 0.0001, p = 0.0132, p< 0.0001, and p = 0.0012, respectively). Compared to the Ctrl-Pinf treatment, only the SDW of the plants in the treatments AM+Pinf -Filt, AM+Pinf (Filt-1), and AM+vir+Pinf (Filt+1) were significantly lower (Table S3). The RDW of the potato plants were significantly lower for AM+Pinf-Filt and AM+Pinf (Filt-1) treatments compared to the Ctrl+Pinf(Manco-1) treatment. Regarding the TDW, no significant difference was observed between the Ctrl-Pinf and the Ctrl+Pinf treatments as well as between the Ctrl-Pinf treatment and all the other treatments. No significant difference was noticed for TFW between the Ctrl-Pinf and Ctrl+Pinf treatments (Table S3). Compared to the Ctrl-Pinf treatment, the AM+Pinf(Filt+1), AM+vir+Pinf(Filt-1), and AM+vir+Pinf(Filt+1) treatments had a significantly higher TFW.
Root colonization by the AMF
Root colonization was estimated at the end of the experiment (i.e., 10 weeks after AMF plant inoculation and 2 weeks after plant infection by the pathogen) (Table 3). At the end of the experiment, a significant positive effect of the treatment was noticed for the percentage of root arbuscules (A%) (i.e., p = 0.0176), but not for the percentage root vesicles/spores (V%) and the TC% (p = 0.1403 and 0.0698, respectively). Compared to the AM+Pinf-Filt treatment, except for the AM+Pinf(Filt+1) treatment, all the other treatments significantly enhanced the A% colonization of the potato roots.
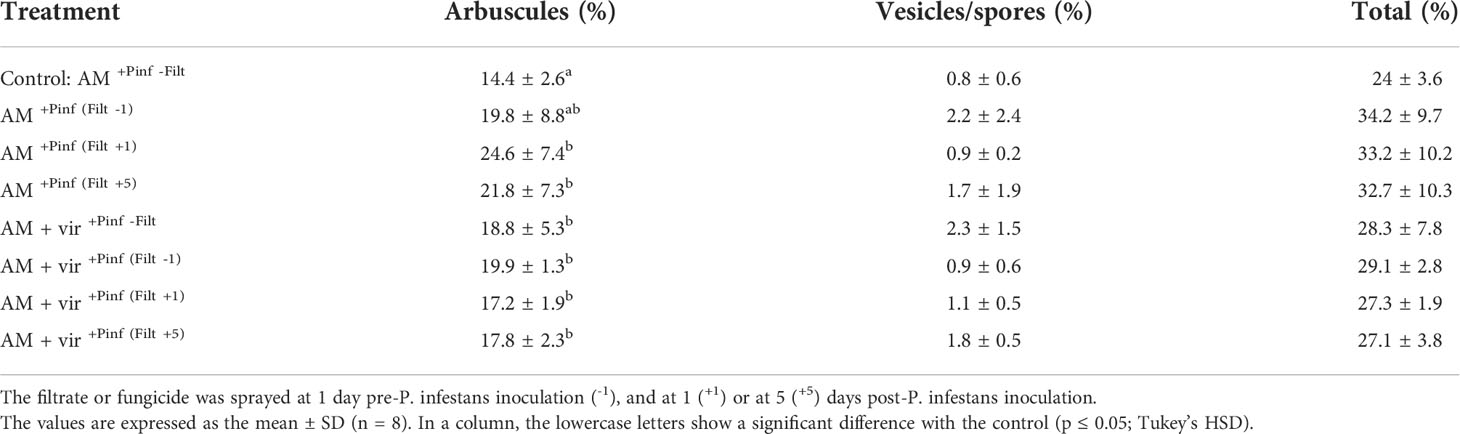
Table 3 Percentages of arbuscules, intraradical vesicles/spores, and total colonization of roots of potato plants grown under greenhouse conditions after 10 weeks of association with R. irregularis MUCL 41833 propagules, entrapped (AM + vir) or not with a culture filtrate of T. virens MUCL 18139 and sprayed (+Filt) or not (-Filt) with a culture filtrate of T. virens MUCL 18139 or with Mancozeb (Manco) fungicide estimated 15 days post-infection with P. infestans MUCL 54981.
Leaf symptoms caused by Phytophthora infestans
Leaf symptoms caused by P. infestans MUCL 54981 are presented in Figure 5. All potato plants inoculated with P. infestans 54981 showed leaf symptoms 15 days after inoculation and a significant effect of the treatment was observed on the AUDPC (p ≤ 0.001). The AUDPC ranged between ~40 and ~242. The application of Mancozeb prior to the inoculation with P. infestans did not have any effect on the disease progression as compared to the Ctrl+Pinf treatment. On the other hand, Mancozeb sprayed 1 and 5 days post-inoculation with P. infestans decreased the AUDPC. Compared to the Ctrl+Pinf treatments, only the plants associated with R. irregularis MUCL 41833 propagules entrapped alone or with T. virens MUCL 18139 filtrate but non-sprayed with T. virens MUCL 18139 filtrate (i.e., AM+Pinf-Filt and AM+vir+Pinf-Filt treatments, respectively) significantly reduced the plant infection by the pathogen. Interestingly, the values did not significantly differ from those of the Ctrl-Pinf treatment and from those of the plants treated with Mancozeb [Ctrl+Pinf (Manco+1) and Ctrl+Pinf (Manco+5)].
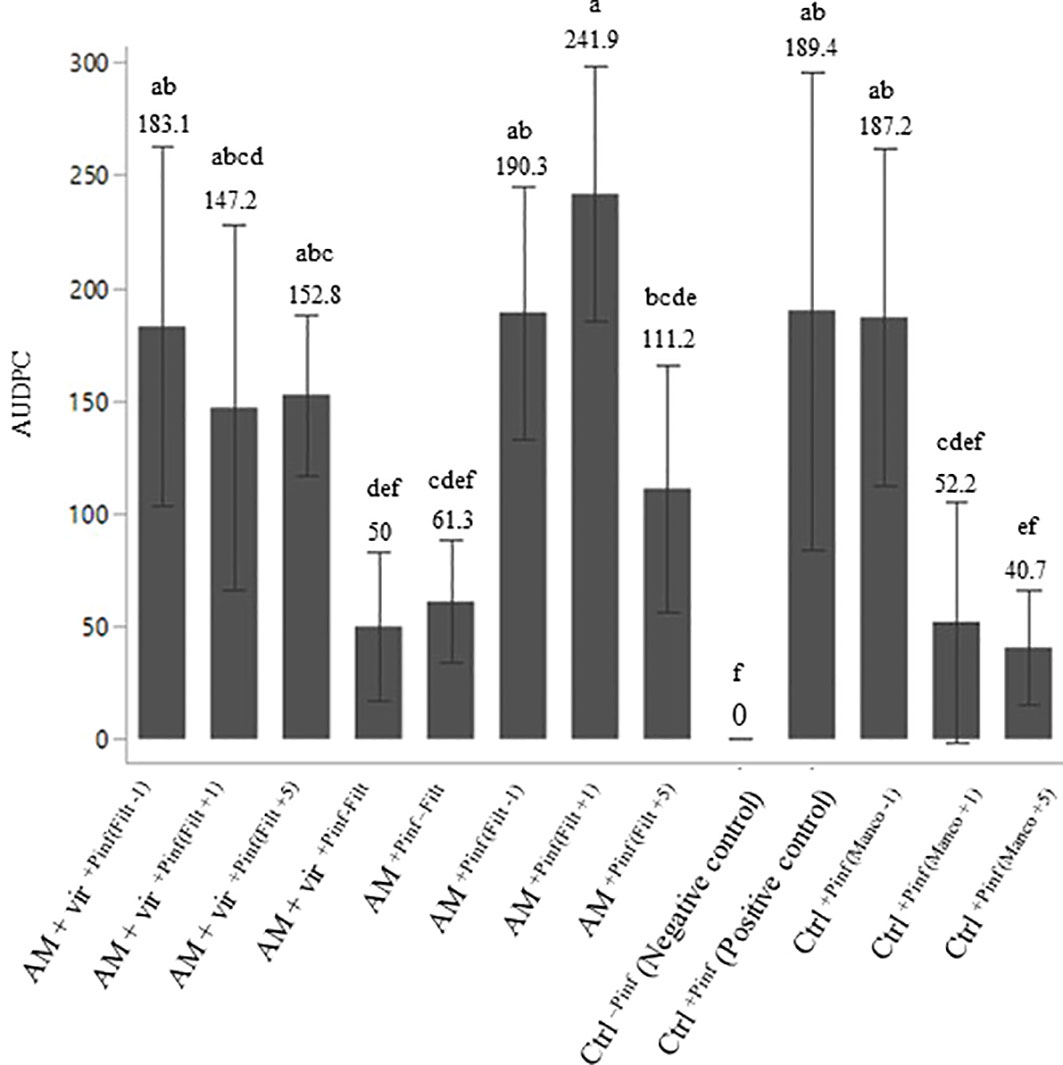
Figure 5 Area under disease progress curve (AUDPC) of potato plants grown 10 weeks in greenhouse in association (AM) or not (Ctrl) with R. irregularis MUCL 41833 propagules, entrapped (AM + vir) or not with a culture filtrate of T. virens MUCL 18139 and sprayed (+Filt) or not (-Filt) with a culture filtrate of T. virens MUCL 18139 or with Mancozeb (Manco) fungicide at different spraying times (-1,+1,+5) estimated 15 days post-infection with P. infestans MUCL 54981. The filtrate or fungicide was sprayed at 1 day pre-P. infestans inoculation (-1), at 1 (+1) or at 5 (+5) days post-P. infestans inoculation. Values are expressed as the mean ± SD (n = 8). Values followed by identical letters did not differ significantly at p ≤ 0.05 (one-way ANOVA and Tukey’s HSD).
Bioactive metabolites production by Trichoderma virens MUCL 18139
LCMS analysis of T. virens MUCL 18139 filtrate was performed in order to identify the metabolites potentially involved in the antifungal activity of the strain. The results of LCMS analyses were compared to an “in-house database” composed of the antimicrobial compounds produced by Trichoderma species described in literature so far. Based on molecular ion masses, we could identify three metabolites (i.e., koninginin D, gliotoxin, and koningic acid) that have antifungal activity (Reino et al., 2008) in this filtrate (Figure 6). This analysis also illustrates that besides these known molecules, other unidentified compounds are also efficiently produced by this fungus, but their structures and their roles in the antifungal potential of the strain remain undetermined so far.
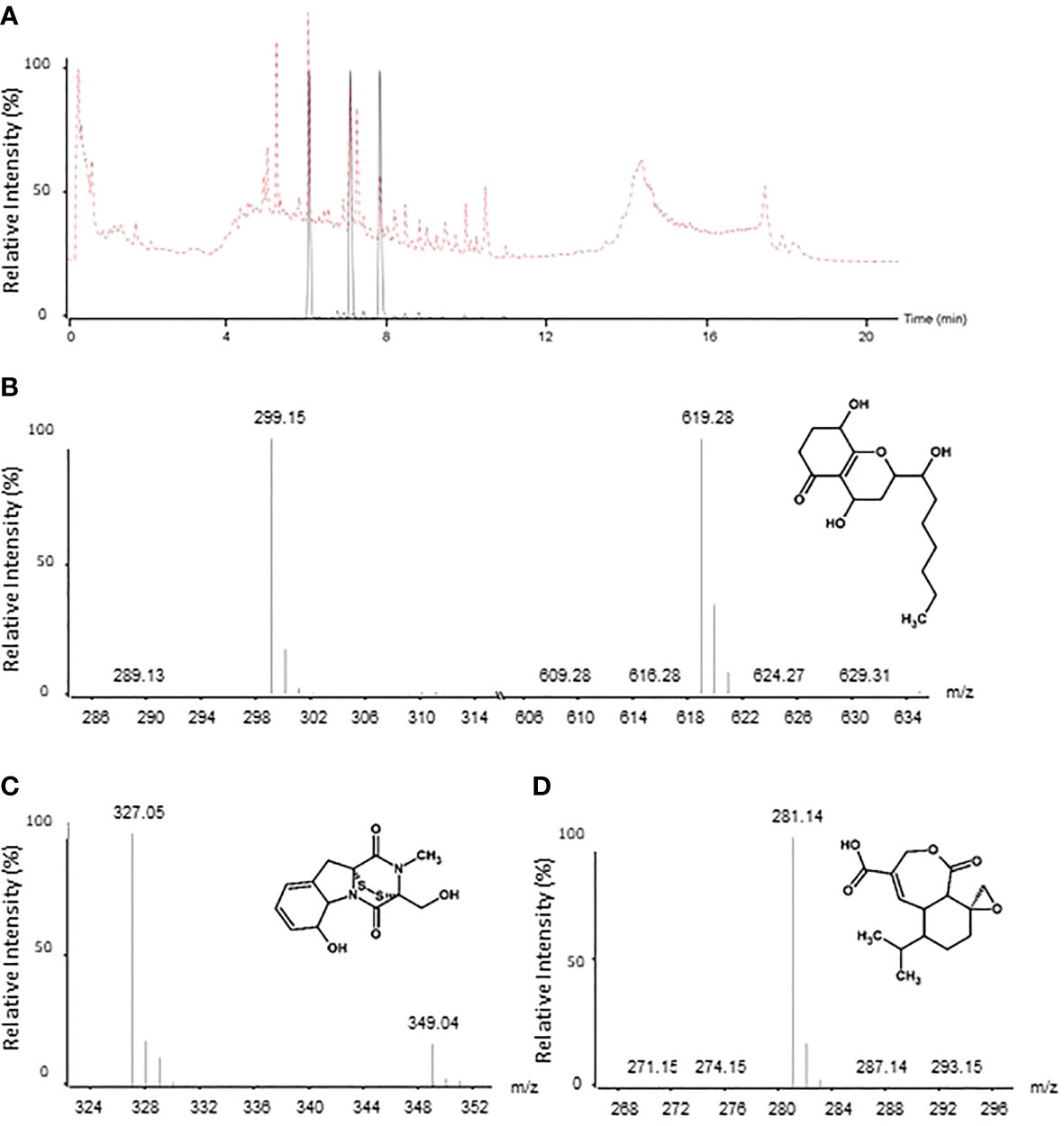
Figure 6 LC-MS analysis of T. virens MUCL 18139 filtrate. Overlay of extracted ion chromatograms (EIC) of three potential molecules involved in the bioactivity of T. virens MUCL 18139 (black). Total ion current (TIC) is represented in the dotted red line (A). Mass spectra and structures of the tree potential metabolites detected in filtrate: koninginin D (RT = 6.1 min): [M+H] +=299.15; [2M+Na]+=619.28; gliotoxin (RT = 7.2 min): [M+H]+= 327.05; [M+Na]+=349.04 and koningic acid (RT = 7.9 min): [M+H]+=281.14 (B–D, respectively).
Discussion
In the present study, the filtrates of 149 fungal strains were tested for their inhibitory activity against P. infestans. The results revealed that five strains (i.e., A. niger, P. aurantiogriseum, P. griseofulvum, T. virens, and V. psalliotae) totally inhibited the germination and growth of P. infestans. Their compatibility with the AMF R. irregularis was evaluated in alginate beads and, with the exception of P. griseofulvum, no negative impact was noticed on the AMF germination and root colonization. The filtrate of T. virens combined the most interesting properties and was therefore tested with the AMF on the disease incidence of P. infestans in the susceptible potato variety Annabelle. AMF alone or AMF with the filtrate of T. virens applied to the roots significantly reduced the area under disease progress curve (by ~69% and 77%, respectively) of the disease, while foliar application of the filtrate led to similar disease spread as in the non-treated control, even in the presence of the AMF, which, without filtrate, conferred significant protection.
Screening for anti-oomycete activity
To evaluate anti-oomycete activity, the culture filtrates of the 149 BCA candidates were tested in vitro on the inhibition of growth of P. infestans by measuring (1) the absorbance in 96-well microplates, (2) the colony surface area in Petri plates, and (3) the leaf symptoms in potato plants var. Bintje grown in culture microboxes. Five of the 149 BCA candidates (i.e., A. niger, P. aurantiogriseum, P. griseofulvum, T. virens, and V. psalliotae) totally inhibited the germination and growth of P. infestans, while their impact on disease symptoms in potato plants was more variable.
A. niger has been shown to reduce disease severity of late blight and Fusarium wilt of tomato (Júnior et al., 2006; Sharma et al., 2011). This reduction has been attributed to a reduced frequency of infection and the prevention of host penetration by a “biofilm” formed by the fungus (Júnior et al., 2006). Interestingly, several studies (e.g., Nielsen et al., 2009; Brzezinska and Jankiewicz, 2012) reported the presence of antibacterial and antifungal metabolites such as Naphtho-γ-pyrones, ochratoxin, and fumonisins, which can have an impact on fungal/oomycete pathogens. A. niger has also been reported to excrete significant amounts of plant degrading enzymes such as xylanases, amylases, cellulases, endoxylanases, β-xylosidases, and pectinases (Sakthi et al., 2012; Benoit-Gelber et al., 2017; Bellaouchi et al., 2021; Budenkova et al., 2021) in its extracellular environment (Budenkova et al., 2021). Although we did not verify the excretion of these enzymes in the PDB medium, it is not excluded that some of them were produced by A. niger, thus impacting the growth of potato plants as observed in our experiment. Indeed, the plants sprayed with the culture filtrate of A. niger withered completely, suggesting a phytotoxic effect.
Penicillium species are frequently reported to control plant diseases. For instance, P. aurantiogriseum isolated from soil samples has been shown to reduce the incidence of Fusarium wilt of sesame in a pot experiment and to excrete extracellular enzymes such as proteases, cellulase, and β-1,3-glucanase on agar plates (Zhao et al., 2021), which could also explain the growth reduction of P. infestans in our study. An antagonistic effect on late blight has also been reported both in vitro and in situ with P. aurantiogriseum (Jindal et al., 1988). P. griseofulvum is known to produce griseofulvin (Frisvad et al., 2004), an SM efficient against Botrytis cinerea, Sclerotinia sclerotiorum, Magnaporthe grisea, Corticium sasaki, Puccinia recondita, Blumeria graminis f. sp. Hordei, Alternaria solani, Fusarium solani, and Colletotrichum gloeosporioides (Stierle and Stierle, 2015; Tang et al., 2015; Ribeiro et al., 2018; Bai et al., 2019; Valente et al., 2020). While a significant reduction of growth of P. infestans was measured by direct interaction in the 96-well microplates and in the Petri plates with both Penicillum species, only P. griseofulvum induced a significant decrease in symptoms of late blight following leaf spraying with the filtrates. Curiously, the second spraying was detrimental to the plants, with the same withered symptoms as observed with A. niger. This could be related to the high potential of P. griseofulvum to produce lignocellulolytic enzymes (Ribeiro et al., 2018; Soeka and Ilyas, 2018; Guan et al., 2021).
V. psalliotae is known to parasite fungi as Thanatephorus cucumeris (CABI, 2019) and Phakopsora pachyrhizi Syd, responsible for the soybean rust (Saksirirat and Hoppe, 1991). This fungus was also shown to excrete β-l,3-glucanase, chitinase, and protease into Czapek-Dox medium in the presence of autoclaved uredospores of the soybean rust fungus as sole carbon source (Saksirirat and Hoppe, 1991; Gan et al., 2007), which could impact the growth of plant pathogens. More recently, Nagaoka et al. (2004) reported the presence of oosporein, an antifungal metabolite in a culture filtrate of V. psalliotae. As in our study, results showed that the growth of P. infestans in Petri plate was completely inhibited by the metabolite of V. psalliotae. Curiously, in our study, the application of the filtrates on the leaves of potato plants did not reduce late blight infection.
Trichoderma species have often been reported as endophytes in several plant species and to impact pathogens via several mechanisms such as antibiosis, parasitism, competition, and induction of host plant resistance (Reino et al., 2008). In our study, the growth of P. infestans was impaired by the application of the filtrate either by direct contact (in the 96-well microplates and Petri plates) or indirectly following application on the leaves, without affecting the plant development as observed for A. niger and P. griseofulvum. This fungus was reported to produce nearly 40 SMs with biocontrol activity (Reino et al., 2008). An LCMS analysis of the culture filtrates of T. virens MUCL 18139 demonstrated the presence of three SMs: koninginin D, koningic acid (synonym: hepteiidic acid), and gliotoxin with anti-fungal/oomycetes activities. Koninginin D is a pyrane metabolite that has been shown to reduce the growth of soil-borne fungi and oomycetes such as Rhizoctonia solani, Phytophthora cinnamomic, Pythium middletonii, Fusarium oxysporum, and Bipolaris sorokiniana (Reino et al., 2008). Koningic acid is an antibacterial and antifungal sesquiterpene lactone active against R. solani and anaerobic bacteria (Itoh et al., 1980; Reino et al., 2008). Finally, gliotoxin has been shown to affect R. solani and Pythium ultimum (Weindling, 1934; Reino et al., 2008; Vargas et al., 2014). In addition to the potential of gliotoxin for biological control of pathogens, this molecule has also some toxicity effects on humans and animals (Scharf et al., 2016; da Silva, 2019), which needs to be considered via toxicological studies before field application. However, unlike T. virens (as living organism), the use of the gliotoxin molecule in the field might not lead to major health risks because it degrades rapidly (Scharf et al., 2016; Jayalakshmi et al., 2021). Indeed, Jayalakshmi et al. (2021) found that gliotoxin stability is influenced by the soil wetness, soil microbial community, and pH conditions. For instance, they noticed that degradation of gliotoxin occurred after 5 days in alkaline soil compared to 10 days in acidic soil.
Since our study was conducted strictly with filtrates, potential mechanisms such as mycoparasitism could be excluded. It is therefore likely that the impacts observed in the 96-well microplates and Petri plates are linked to the SMs, possibly those cited above, and that the reduction in the symptoms observed on in vitro potato leaves is linked to a direct interaction of the filtrates with the pathogen or to an indirect effect via the stimulation of plant defense mechanisms. Indeed, it has been previously reported that Trichoderma SMs, particularly peptaibols, are directly implicated in the induction of plant defense genes (Viterbo et al., 2007; Vinale et al., 2012), and consequently the production of plant defensive compounds such as phytoalexins, flavonoids, terpenoids, phenolic by-products, and additional antimicrobial compounds (Sood et al., 2020).
Compatibility, within beads, of effective BCA filtrates with the AMF R. irregularis
In soils, AMFs interact with many microorganisms whose exudates can, for some, stimulate spore germination, mycelial growth, root colonization, or sporulation (Frey-Klett et al., 2007), while others, on the contrary, can impact the establishment of an effective symbiosis by mechanisms such as antagonism (Green et al., 1999; Ravnskov et al., 2006; Van Wees et al., 2008). It is thus tempting to speculate that fungal filtrates having an inhibitory effect on P. infestans, as in our experiment, can potentially also have an impact on AMFs, hence the need to study their compatibility.
With the exception of filtrates of P. griseofulvum, the co-entrapment in alginate beads of filtrates of P. aurantiogriseum, T. virens, and V. psalliotae with R. irregularis did not impact spore germination and colonization of carrot roots in vitro or leek root colonization in pot cultures. These results are in agreement with the study of Fracchia et al. (2004) on the effect of P. restrictum and T. harzianum exudates on Funneliformis mosseae and Gigaspora rosea. In their study, neither stimulation nor inhibition was noticed on the germination and hyphal growth of spores in vitro and on root colonization of soybean in pots with both AMF species. Conversely, with A. niger exudates, the germination and hyphal growth of F. mosseae and G. rosea spores and root colonization of soybean was increased. Such stimulating effects were also reported by Della Mónica et al. (2014). These authors noticed that growth and branching of hyphae arising from germinating spores of G. rosea cultured in vitro and root colonization of wheat grown in the greenhouse were observed with exudates of T. flavus.
A number of studies have reported that the growth and root colonization of AMF are impacted by concentrations of exudates in a dose-dependent manner (Fracchia et al., 2004; Scervino et al., 2008; Nagahashi and Douds, 2011; Lanfranco et al., 2018). For instance, Fracchia et al. (2004) found that the application of 1–2.5 µl ml−1 exudates of A. niger stimulated the hyphal growth of F. mosseae, while for G. rosea, this increase was observed at concentrations above 2.5 µl ml−1. The same observation was made by Scervino et al. (2008). These authors reported that the effects of exudates of Rhodotorula mucilaginosa on the hyphal length of G. rosea and G. margarita spores grown in vitro were concentration-dependent. In our study, increasing dose of filtrates of P. aurantiogriseum did not affect the percentage of colonized leek plants, while with the filtrate of T. virens, V. psallioteae, and P. griseofulvum, this percentage changed according to the dose of filtrates. Indeed, at the highest dose (i.e., 50 and 75 µl per bead) of filtrate of P. griseofulvum, the percentage of colonized leek plants decreased. A similar decrease was observed at the highest dose (i.e., 75 µl per bead) of the filtrate of T. virens, while at doses of 10, 25, and 50 µl per bead, no inhibitory effect was noticed. Interestingly, only the lowest filtrate dose of V. psalliotae (i.e., 10 µl per bead) induced a decrease in the percentage of colonized leek plants, whereas higher doses (i.e., 50 and 75 µl per bead) did not impact the percentage of colonized leek plants, suggesting a hormetic effect.
Effects of combination of T. virens filtrate with the AMF R. irregularis on late blight
From the results above, it appeared that T. virens offers the highest guarantees of success due to its strong direct antagonism against P. infestans and its compatibility with AMF in beads at low dose (i.e., 10 µl per bead). The co-entrapment of T. virens filtrates (10 µl per bead) and AMF applied belowground, combined with the foliar application of T. virens filtrates before and after infection by P. infestans, was thus evaluated on the development of leaf symptoms in the susceptible potato variety Annabelle.
Belowground treatment with beads containing the AMF alone or the AMF combined with the culture filtrate of T. virens reduced the AUDPC by ~69% and 77%, respectively, as compared to the non-treated plants infected with P. infestans. The decrease in symptoms could be related to a mycorrhizal-induced resistance providing a systemic protection against P. infestans, as earlier reported with the potato variety Bintje colonized with the same AMF species and infected by P. infestans (Pozo and Azcón-Aguilar, 2007; Gallou et al., 2011; Alaux et al., 2018).
All treatments with culture filtrate of T. virens applied in the beads or sprayed on the aerial part showed a systematic higher total root colonization by the AMF and, with the exception of the treatment AM+Pinf (Filt-1), a significantly higher percentage of arbuscules compared to the treatments with the AMF only. This suggests a probable stimulating effect of the filtrate of T. virens either directly on the AMF via root application or indirectly via spraying on the aboveground plant part. For AMF, a number of studies have reported an increasing root colonization in the presence of Trichoderma spp. (e.g., Fracchia et al., 1998; Metwally, 2020), while indirect effects via the plants were more rare. For instance, Moutassem et al. (2020) revealed a strong accumulation of polyphenols and flavonoids in chickpea plants treated with SMs of various Trichoderma spp., such as T. virens. Interestingly, plant phenolic compounds such as flavonoids have often been reported to stimulate AMF root colonization (Akiyama et al., 2002; Mandal et al., 2010). For example, Fries et al. (1997) demonstrated the stimulatory effect of p-coumaric acid, p-hydroxybenzoic acid, or quercetin on the growth and colonization of clover and sorghum roots by Rhizophagus intraradices. Therefore, it is not excluded that the significant increase in arbuscules noticed in the potato plants sprayed with T. virens filtrate was due to a possible increase in phenolic compound accumulated in the roots of potato.
Curiously, independently of the presence of AMF, which reduced symptoms in the absence of the foliar T. virens filtrate application, all plants sprayed with the filtrate of T. virens developed foliar symptoms equivalent to those of the control treatment (Ctrl +Pinf plants). This suggests that foliar application of a filtrate of T. virens made potato plants more susceptible to infection by P. infestans. T. virens is known for its production of hydrolytic enzymes such as xylanase, cellulase, endoglucanase, β-glucosidase, and exoglucanase (Amira et al., 2011; Ngikoh et al., 2017; Sood et al., 2020) that mediate several processes such as plant signaling and interaction, plant growth and resistance to pests and diseases, and cell wall dissolution of microbes (Druzhinina et al., 2011; Ahluwalia et al., 2015; Meng et al., 2019; Sood et al., 2020). In the present study, the culture filtrate of T. virens was obtained by growing the fungus on PDB medium, a rich source of cellulose, hemicellulose, lignin, and pectin of potato origin (Lesiecki et al., 2012). Thus, it is not excluded that in addition to the antimicrobial metabolites produced, the filtrate of T. virens contained lytic enzymes affecting the potato leaves, hence increasing their susceptibility to infection by P. infestans and neutralizing the positive effect of the antimicrobial metabolites or AMF on this pathogen, observed in the treatments with beads containing the AMF alone or combined with the culture filtrate of T. virens.
Curiously, these results are contrasting with those obtained in vitro where the second foliar spraying of the filtrate of T. virens significantly reduced the percentage of leaf area infection of potato plants var. Bintje. The differences may be attributed to several factors, among which are the higher relative humidity in the greenhouse (90% versus 75% in the growth chamber) and temperature (reaching up to 26°C during the day versus 20°C in the growth chamber), increasing the sporulation of P. infestans and the development of the disease (Magarey et al., 2005; Singh and Sharma, 2013; Escuredo et al., 2019). It is also possible that solar irradiation, UV light, and higher temperature in greenhouse have led to chemical degradation of antimicrobial molecules produced in the filtrate of T. virens, resulting in a reduced effectiveness on the control of P. infestans. This was supported by the study of Najdabbasi et al. (2020), showing that the essential oil cinnamon impacted germination of sporangia and mycelial growth of P. infestans on detached leaf bioassays, but was less effective under greenhouse conditions on plants grown in pots. Liu et al. (2018) reported that roflamycoin, an antifungal metabolite produced by Streptomyces roseoflavus, was degraded under the sunlight as well as under fluorescent light. Photo-oxidation of natural compounds by UV, including biocontrol products, has been reported by Ahonsi et al. (2006) and Romdhane et al. (2018). Plant parameters may also be involved in the differences observed between both experiments. Stewart (1990) reported that plants in bud or early stages of flowering expressed more resistance than older plants. Similarly, Fry and Apple (1986) found that the rate of plant infestation was influenced by the age of the plants, and disease progression did not occur as fast in intermediate-aged plants when compared to older plants. In the present study, the plants in the greenhouse were at the late stage of growth (4 weeks older than those in vitro) and could possibly be more sensitive to P. infestans infestation. It is also not excluded that the higher density of plants in the greenhouse as compared to the growth chamber (one single plant per microbox) could have played a role in sporangia production and spread, enhancing the probability of infection of the plants in the greenhouse as suggested by Stewart et al. (1983) and Michalska et al. (2011). Finally, Bintje was micropropagated in vitro, and thus grown in the presence of only the filtrate of T. virens, while in the greenhouse, the cultivar Annabelle was confronted to a population of microorganisms, potentially degrading some of the metabolites produced by T. virens, thus decreasing/annihilating their potential effects on P. infestans.
In conclusion, this is the first study on the combination of fungal filtrates and AMF for the biocontrol of P. infestans. The filtrates of several fungal BCA candidates were tested in vitro and in planta. Among them, the filtrate of T. virens MUCL 18139 reduced the P. infestans potato symptoms under in vitro conditions. Under greenhouse conditions, this filtrate applied to the roots stimulated root colonization by the AMF, and in combination with the AMF propagules in alginate beads, it strongly reduced the infection of the sensitive potato variety Annabelle, similar to the AMF treatment. Conversely, foliar application of the filtrate, whether the plant was colonized by the AMF or not, did not reduce symptoms of the disease, possibly because of the production of lytic enzymes, making the potato plant more susceptible to P. infestans and counterbalancing the positive effects of the antimicrobial SMs (e.g., koninginin D, gliotoxin, and koningic acid) or AMF. The strong beneficial effects observed with root application of filtrates of T. virens and AMF opens the door for further testing under field conditions, in a context of multi-interactions with the local microbial communities and under physicochemical and climatic diverse conditions, in an integrated approach allowing to reduce the amount of pesticides or their frequency of use.
Data availability statement
The original contributions presented in the study are included in the article/supplementary material. Further inquiries can be directed to the corresponding author.
Author contributions
SD contributed to conception and design of the study. IL, MC-S, FN and AA organized the database. IL and MC-S performed the statistical analysis. IL, MC-S and AA wrote the first draft of the manuscript. SD, IL, MC-S, AA and MO wrote sections of the manuscript. All authors contributed to manuscript revision, read, and approved the submitted version.
Funding
This work was made possible by financial support of the Walloon region (Belgium) in the frame of WALINNOV research program (project Biofungi—Convention n° 1810004) and was partly funded by the EU Interreg V France-Wallonie-Vlaanderen portfolio SmartBiocontrol (Bioscreen project), avec le soutien du Fonds européen de développement régional - Met steun van het Europees Fonds voor Regionale Ontwikkeling.
Conflict of interest
Author TP was employed by company Medinbio.
The remaining authors declare that the research was conducted in the absence of any commercial or financial relationships that could be construed as a potential conflict of interest.
Publisher’s note
All claims expressed in this article are solely those of the authors and do not necessarily represent those of their affiliated organizations, or those of the publisher, the editors and the reviewers. Any product that may be evaluated in this article, or claim that may be made by its manufacturer, is not guaranteed or endorsed by the publisher.
Supplementary material
The Supplementary Material for this article can be found online at: https://www.frontiersin.org/articles/10.3389/fagro.2022.948309/full#supplementary-material
Footnotes
- ^ Part of the Belgian Coordinated Collections of Microorganisms (BCCM).
- ^ 2 Part of the Royal Netherlands Academy of Arts and Sciences.
References
Ahluwalia V., Kumar J., Rana V. S., Sati O. P., Walia S. (2015). Comparative evaluation of two Trichoderma harzianum strains for major secondary metabolite production and antifungal activity. Natural Product Res. 29 (10), 914–920. doi: 10.1080/14786419.2014.958739
Ahonsi M. O., Boss D., Maurhofer M., Défago G. (2006). Potential environmental fate of elsinochrome a, a perylenequinone toxin produced in culture by bindweed biocontrol fungus Stagonospora convolvuli LA39. Environmentalist 26, 183–193. doi: 10.1007/s10669-006-7830-0
Akiyama K., Matsuoka H., Hayashi H. (2002). Isolation and identification of a phosphate deficiency-induced c-glycosylflavonoid that stimulates arbuscular mycorrhiza formation in melon roots. Mol. Plant-Microbe Interact. 15 (4), 334–340. doi: 10.1094/MPMI.2002.15.4.334
Alaux P. L., César V., Naveau F., Cranenbrouck S., Declerck S. (2018). ). impact of Rhizophagus irregularis MUCL 41833 on disease symptoms caused by Phytophthora infestans in potato grown under field conditions. Crop Prot. 107, 26–33. doi: 10.1016/j.cropro.2018.01.003
Amira R. D., Roshanida A. R., Rosli M. I., Zahrah M. S. F., Anuar J. M., Adha C. N. (2011). Bioconversion of empty fruit bunches (EFB) and palm oil mill effluent (POME) into compost using Trichoderma virens. Afr. J. Biotechnol. 10 (81), 18775–18780. doi: 10.5897/AJB11.2751
Bae S.-J., Mohanta T. K., Chung J. Y., Ryu M., Park G., Shim S., et al. (2016). Trichoderma metabolites as biological control agents against phytophthora pathogens. Biol. Control 92, 128–138. doi: 10.1016/j.biocontrol.2015.10.005
Bai M., Huang G. L., Mei R. Q., Wang B., Luo Y. P., Nong X. H., et al. (2019). Bioactive lactones from the mangrove-derived fungus penicillium sp. TGM112. Mar. Drugs 17 (8), 433. doi: 10.1111/jph.12819
Bellaouchi R., Abouloifa H., Rokni Y., Hasnaoui A., Ghabbour N., Hakkou A., et al. (2021). Characterization and optimization of extracellular enzymes production by aspergillus niger strains isolated from date by-products. J. Genet. Eng. Biotechnol. 19 (1), 1–8. doi: 10.1186/s43141-021-00145-y
Bengtsson T., Holefors A., Liljeroth E., Hultberg M., Andreasson E. (2015). Biosurfactants have the potential to induce defence against Phytophthora infestans in potato. Potato Res. 58 (1), 83–90. doi: 10.1007/s11540-015-9286-4
Benoit-Gelber I., Gruntjes T., Vinck A., Van Veluw J. G., Wösten H. A., Boeren S., et al. (2017). Mixed colonies of aspergillus niger and Aspergillus oryzae cooperatively degrading wheat bran. Fungal Genet. Biol. 102, 31–37. doi: 10.1186/s12864-017-4164-x
Berruti A., Borriello R., Orgiazzi A., Barbera A. C., Lumini E., Bianciotto V. (2014). “Arbuscular mycorrhizal fungi and their value for ecosystem management,” in Biodiversity, The Dynamic Balance of the Planet (Intech: Rijeka, Croatia), 159–191.
Brundrett M. C., Tedersoo L. (2018). Evolutionary history of mycorrhizal symbioses and global host plant diversity. New Phytol. 220 (4), 1108–1115. doi: 10.1111/nph.14976
Brun L. A., Maillet J., Richarte J., Herrmann P., Remy J. C. (1998). Relationships between extractable copper, soil properties and copper uptake by wild plants in vineyard soils. Environ. pollut. 102 (2-3), 151–161. doi: 10.1016/S0269-7491(98)00120-1
Brzezinska M. S., Jankiewicz U. (2012). Production of antifungal chitinase by Aspergillus niger LOCK 62 and its potential role in the biological control. Curr. Microbiol. 65 (6), 666–672. doi: 10.1007/s00284-012-0208-2
Budenkova E., Sukhikh S., Ivanova S., Babich O., Dolganyuk V., Michaud P., et al. (2021). Improvement of enzymatic saccharification of cellulose-containing raw materials using Aspergillus niger. Processes 9 (8), 1360. doi: 10.3390/pr9081360
Buysens C., Alaux P.-L., César V., Huret S., Declerck S., Cranenbrouck S. (2017). Tracing native and inoculated Rhizophagus irregularis in three potato cultivars (Charlotte, Nicola and bintje) grown under field conditions. Appl. Soil Ecol. 115, 1–9. doi: 10.1016/j.apsoil.2017.03.007
CABI (2019). Available at: Verticillium psalliotae (brown spot: mushrooms) (cabi.org).
César V., Ryckmans D. (2021) La sensibilité variétale au mildiou de la pomme de terre sous l’œil des experts en wallonie (Le Sillon Belge). Available at: https://www.sillonbelge.be/7233/article/2021-03-11/la-sensibilite-varietale-au-mildiou-de-la-pomme-de-terre-sous-loeil-des-experts (Accessed March 11, 2021).
Cranenbrouck S., Voets L., Bivort C., Renard L., Strullu D. G., Declerck S. (2005). “Methodologies for in vitro cultivation of arbuscular mycorrhizal fungi with root organs,” in In in vitro culture of mycorrhizas (Springer: Berlin, Heidelberg), (pp. 341–375). doi: 10.1007/3-540-27331-X_18
da Silva M. R. B. L. (2019). “Gliotoxin and bis-methyl-glitoxin production by trichoderma spp. as biocontrol agents,” in Human risk potential by using trichoderma spp. metabolites (Instituto Politecnico do Porto (Portugal: Doctoral dissertation).
Declerck S., Dimitri D. O., Bivort C., de Souza F. A. (2004). Development of extraradical mycelium of Scutellospora reticulata under root-organ culture: spore production and function of auxiliary cells. Mycological Res. 108 (1), 84–92. doi: 10.1017/S0953756203008761
Declerck S., Strullu D. G., Plenchette C. (1998). Monoxenic culture of the intraradical forms of Glomus sp. isolated from a tropical ecosystem: a proposed methodology for germplasm collection. Mycologia 90 (4), 579–585. doi: 10.1080/00275514.1998.12026946
Declerck S., Strullu D. G., Plenchette C., Guillemette T. (1996). Entrapment of in vitro produced spores of Glomus versiforme in alginate beads: in vitro and in vivo inoculum potentials. J. Biotechnol. 48 (1-2), 51–57. doi: 10.1016/0168-1656(96)01396-X
Della Mónica I. F., Stefanoni Rubio P. J., Cina R. P., Recchi M., Godeas A. M., Scervino J. M. (2014). Effects of the phosphate-solubilizing fungus Talaromyces flavus on the development and efficiency of the Gigaspora rosea-Triticum aestivum symbiosis. Symbiosis 64 (1), 25–32. doi: 10.1007/s13199-014-0299-6
Doner L. W., Bécard G. (1991). Solubilization of gellan gels by chelation of cations. Biotechnol. Techniques 5 (1), 25–28. doi: 10.1007/BF00152749
Dorn B., Musa T., Krebs H., Fried P. M., Forrer H. R. (2007). Control of late blight in organic potato production: evaluation of copper-free preparations under field, growth chamber and laboratory conditions. Eur. J. Plant Pathol. 119 (2), 217–240. doi: 10.1007/s10658-007-9166-0
Druzhinina I. S., Seidl-Seiboth V., Herrera-Estrella A., Horwitz B. A., Kenerley C. M., Monte E., et al. (2011). Trichoderma: the genomics of opportunistic success. Nat. Rev. Microbiol. 9 (10), 749–759. doi: 10.1038/nrmicro2637
El-Hasan A., Walker F., Schöne J., Buchenauer H. (2009). Detection of viridiofungin a and other antifungal metabolites excreted by Trichoderma harzianum active against different plant pathogens. Eur. J. Plant Pathol. 124 (3), 457–470. doi: 10.1007/s10658-009-9433-3
Elsherbiny E. A., Amin B. H., Aleem B., Kingsley K. L., Bennett J. W. (2020). Trichoderma volatile organic compounds as a biofumigation tool against late blight pathogen Phytophthora infestans in postharvest potato tubers. J. Agric. Food Chem. 68 (31), 8163–8171. doi: 10.1021/acs.jafc.0c03150
Escuredo O., Seijo-Rodríguez A., Rodríguez-Flores M. S., Seijo M. C. (2019). Decision support systems for detecting aerial potato Phytophthora infestans sporangia in northwestern Spain. Agron. J. 111 (1), 354–361. doi: 10.2134/agronj2018.02.0124
Fracchia S., Mujica M. T., García-Romera I., Garcia-Garrido J. M., Martin J., Ocampo J. A., et al. (1998). Interactions between Glomus mosseae and arbuscular mycorrhizal sporocarp-associated saprophytic fungi. Plant Soil 200 (2), 131–137. doi: 10.1023/A:1004349426315
Fracchia S., Sampedro I., Scervino J. M., Garcia-Romera I., Ocampo J. A., Godeas A. (2004). Influence of saprobe fungi and their exudates on arbuscular mycorrhizal symbioses. Symbiosis 36 (2), 169–182.
Frey-Klett P., Garbaye J., Tarkka M. (2007). The mycorrhiza helper bacteria revisited. New Phytol. 176 (1), 22–36. doi: 10.1111/j.1469-8137.2007.02191.x
Fries L. L., Pacovsky R. S., Safir G. R., Siqueira J. O. (1997). Plant growth and arbuscular mycorrhizal fungal colonization affected by exogenously applied phenolic compounds. J. Chem. Ecol. 23 (7), 1755–1767. doi: 10.1023/B:JOEC.0000006449.09141.cd
Frisvad J. C., Smedsgaard J., Larsen T. O., Samson R. A. (2004). Mycotoxins, drugs and other extrolites produced by species in Penicillium subgenus Penicillium. Stud. Mycology 49, 201–241.
Fry W. E., Apple A. E. (1986). Disease management implications of age-related changes in susceptibility of potato foliage to Phytophthora infestans. Am. Potato J. 63 (1), 47–56. doi: 10.1007/BF02855299
Fry W. E., Birch P. R. J., Judelson H. S., Grünwald N. J., Danies G., Everts K. L., et al. (2015). Five reasons to consider Phytophthora infestans a reemerging pathogen. Phytopathology 105 (7), 966–981. doi: 10.1094/PHYTO-01-15-0005-FI
Gallou A., Mosquera H. P. L., Cranenbrouck S., Suárez J. P., Declerck S. (2011). Mycorrhiza induced resistance in potato plantlets challenged by Phytophthora infestans. Physiol. Mol. Plant Pathol. 76 (1), 20–26. doi: 10.1016/j.pmpp.2011.06.005
Gan Z., Yang J., Tao N., Liang L., Mi Q., Li J., et al. (2007). Cloning of the gene Lecanicillium psalliotae chitinase Lpchi1 and identification of its potential role in the biocontrol of root-knot nematode Meloidogyne incognita. Appl. Microbiol. Biotechnol. 76 (6), 1309–1317. doi: 10.1007/s00253-007-1111-9
Garcés-Ruiz M., Calonne-Salmon M., Plouznikoff K., Misson C., Navarrete-Mier M., Cranenbrouck S., et al. (2017). Dynamics of short-term phosphorus uptake by intact mycorrhizal and non-mycorrhizal maize plants grown in a circulatory semi-hydroponic cultivation system. Front. Plant Sci. 8. doi: 10.3389/fpls.2017.01471
Gonzales-Tobon J., Rodriguez-Jaramillo A., Forero L. M., González L. N., Danies G., Restrepo S. (2019). Evaluation of small ncRNAs as a possible epigenetic mechanism mediating the transition from biotrophy to necrotrophy in the life cycle of Phytophthora infestans. Res. Square. doi: 10.21203/rs.2.12907/v2
Graham J. H., Timmer L. W., Fardelmann D. (1986). Toxicity of fungicidal copper in soil to citrus seedlings and vesicular-arbuscular mycorrhizal fungi. Phytopathology 76 (1), 66–70. doi: 10.1094/Phyto-76-66
Green H., Larsen J., Olsson P. A., Jensen D. F., Jakobsen I. (1999). Suppression of the biocontrol agent Trichoderma harzianum by mycelium of the arbuscular mycorrhizal fungus Glomus intraradices in root-free soil. Appl. Environ. Microbiol. 65 (4), 1428–1434. doi: 10.1128/AEM.65.4.1428-1434.1999
Guan F., Liu J., Cai J., Qi C., Wang C., Cui Y., et al. (2021). Cellulase production by a newly isolated soil fungus Penicillium griseofulvum A2. Res. Square. doi: 10.21203/rs.3.rs-549434/v1
Haas B. J., Kamoun S., Zody M. C., Jiang R. H., Handsaker R. E., Cano L. M., et al. (2009). Genome sequence and analysis of the Irish potato famine pathogen Phytophthora infestans. Nature 461(7262), 393–398. doi: 10.1038/nature08358
Haverkort A. J., Boonekamp P. M., Hutten R., Jacobsen E. J. P. R., Lotz L. A. P., Kessel G. J. T., et al. (2008). Societal costs of late blight in potato and prospects of durable resistance through cisgenic modification. Potato Res. 51 (1), 47–57. doi: 10.1007/s11540-008-9089-y
Haverkort A. J., Struik P. C., Visser R. G. F., Jacobsen E. J. P. R. (2009). Applied biotechnology to combat late blight in potato caused by Phytophthora infestans. Potato Res. 52 (3), 249–264. doi: 10.1007/s11540-009-9136-3
Hijri M. (2016). Analysis of a large dataset of mycorrhiza inoculation field trials on potato shows highly significant increases in yield. Mycorrhiza 26 (3), 209–214. doi: 10.1007/s00572-015-0661-4
Hill E. M., Robinson L. A., Abdul-Sada A., Vanbergen A. J., Hodge A., Hartley S. E. (2018). Arbuscular mycorrhizal fungi and plant chemical defence: effects of colonisation on aboveground and belowground metabolomes. J. Chem. Ecolology 44, 198–208. doi: 10.1007/s10886-017-0921-1
Hohmann P., Messmer M. M. (2017). Breeding for mycorrhizal symbiosis: focus on disease resistance. Euphytica 213, 113. doi: 10.1007/s10681-017-1900-x
Hultberg M., Bengtsson T., Liljeroth E. (2010). Late blight on potato is suppressed by the biosurfactant-producing strain Pseudomonas koreensis 2.74 and its biosurfactant. BioControl 55 (4), 543–550. doi: 10.1007/s10526-010-9289-7
Hunziker L., Bönisch D., Groenhagen U., Bailly A., Schulz S., Weisskopf L. (2015). Pseudomonas strains naturally associated with potato plants produce volatiles with high potential for inhibition of Phytophthora infestans. Appl. Environ. Microbiol. 81 (3), 821–830. doi: 10.1128/AEM.02999-14
Itoh Y., Kodama K., Furuya K., Takahashi S., Haneishi T., Takiguchi Y., et al. (1980). A new sesquiterpene antibiotic, heptelidic acid producing organisms, fermentation, isolation and characterization. J. Antibiotics 33 (5), 468–473. doi: 10.7164/antibiotics.33.468
Ivanov A. A., Ukladov E. O., Golubeva T. S. (2021). Phytophthora infestans: an overview of methods and attempts to combat late blight. J. Fungi 7 (12), 1071. doi: 10.3390/jof7121071
Jacott C., Murray J., Ridout C. (2017). Trade-offs in arbuscular mycorrhizal symbiosis: disease resistance, growth responses and perspectives for crop breeding. Agronomy 7, 75. doi: 10.3390/agronomy7040075
James W. C. (1971). A manual of assessment keys for plant diseases (American Phytopathological Society, St. Paul, MN). doi: 10.1146/annurev.py.12.090174.000331
Jayalakshmi R., Oviya R., Premalatha K., Mehetre S. T., Paramasivam M., Kannan R., et al. (2021). Production, stability and degradation of trichoderma gliotoxin in growth medium, irrigation water and agricultural soil. Sci. Rep. 11 (1), 1–14. doi: 10.1038/s41598-021-95907-6
Jindal K. K., Singh H., Meeta M. (1988). Biological control of Phytophthora infestans on potato. Indian J. Plant Pathol. 6 (1), 59–62.
Júnior V. L., Maffia L. A., da Silva Romeiro R., Mizubuti E. S. (2006). Biocontrol of tomato late blight with the combination of epiphytic antagonists and rhizobacteria. Biol. Control 38 (3), 331–340. doi: 10.1016/j.biocontrol.2006.04.005
Kang S., Kim S. (2004). New antifungal activity of penicillic acid against Phytophthora species. Biotechnol. Lett. 26, 695–698. doi: 10.1023/B:BILE.0000024090.96693.a4
Lanfranco L., Fiorilli V., Gutjahr C. (2018). Partner communication and role of nutrients in the arbuscular mycorrhizal symbiosis. New Phytol. 220 (4), 1031–1046. doi: 10.1111/nph.15230
Lesiecki M., Białas W., Lewandowicz G. (2012). Enzymatic hydrolysis of potato pulp. Acta Scientiarum Polonorum Technologia Alimentaria 11 (1), 53–59.
Linkies A., Jacob S., Zink P., Maschemer M., Maier W., Koch E. (2021). Characterization of cultural traits and fungicidal activity of strains belonging to the fungal genus Chaetomium. J. Appl. Microbiol. 131 (1), 375–391. doi: 10.1111/jam.14946
Liu C., Chang Z. (2018). Identification of the biocontrol strain LB-2 and determination of its antifungal effects on plant pathogenic fungi. J. Plant Pathol. 100 (1), 25–32. doi: 10.1007/s42161-018-0005-2
Liu Y., Han X., Zhen D. M., Chi G. T., Li Y. N., Liu D. Q. (2018). Photolysis of the active metabolite roflamycoin produced by Steptomyces roseoflavus men-myco-93-63. J. Plant Prot. 45 (6), 1260–1266.
Madden L. V., Campbell C. L. (1990). ““Nonlinear disease progress curves” in epidemics of plant diseases,” in Ecological studies (Analysis and synthesis), vol. 13 . Ed. Kranz J. (Berlin, Heidelberg: Springer), 181–229. doi: 10.1007/978-3-642-75398-5_6
Magarey R. D., Sutton T. B., Thayer C. L. (2005). A simple generic infection model for foliar fungal plant pathogens. Phytopathology 95 (1), 92–100. doi: 10.1094/PHYTO-95-0092
Mandal S. M., Chakraborty D., Dey S. (2010). Phenolic acids act as signaling molecules in plant-microbe symbioses. Plant Signaling Behav. 5 (4), 359–368. doi: 10.4161/psb.5.4.10871
McGonigle T. P., Miller M. H., Evans D. G., Fairchild G. L., Swan J. A. (1990). A new method which gives an objective measure of colonization of roots by vesicular–arbuscular mycorrhizal fungi. New Phytol. 115 (3), 495–501. doi: 10.1111/j.1469-8137.1990.tb00476.x
Meng X., Miao Y., Liu Q., Ma L., Guo K., Liu D., et al. (2019). TgSWO from Trichoderma guizhouense NJAU4742 promotes growth in cucumber plants by modifying the root morphology and the cell wall architecture. Microbial Cell Factories 18 (1), 1–15. doi: 10.1186/s12934-019-1196-8
Metwally R. A. (2020). Arbuscular mycorrhizal fungi and Trichoderma viride cooperative effect on biochemical, mineral content, and protein pattern of onion plants. J. Basic Microbiol. 60 (8), 712–721. doi: 10.1002/jobm.202000087
Michalska A. M., Zimnoch-Guzowska E., Sobkowiak S., Plich J. (2011). Resistance of potato to stem infection by Phytophthora infestans and a comparison to detached leaflet and field resistance assessments. Am. J. Potato Res. 88 (4), 367–373. doi: 10.1007/s12230-011-9202-7
Moushib L. I., Witzell J., Lenman M., Liljeroth E., Andreasson E. (2013). Sugar beet extract induces defence against Phytophthora infestans in potato plants. Eur. J. Plant Pathol. 136 (2), 261–271. doi: 10.1007/s10658-012-0160-9
Moutassem D., Belabid L., Bellik Y. (2020). Efficiency of secondary metabolites produced by Trichoderma spp. in the biological control of fusarium wilt in chickpea. J. Crop Prot. 9 (2), 217–231.
Myrchiang P., Dkhar M. S., Devi H. R. (2014). Studies on endophytic fungi associated with medicinally important aromatic plant Artemisia nilagirica (CB Clarke) pamp. and their antagonistic activity against Phytophthora infestans. J. Advenced Lab. Res. 5 (4), 112–119.
Nagahashi G., Douds J. D.D. (2011). The effects of hydroxy fatty acids on the hyphal branching of germinated spores of AM fungi. Fungal Biol. 115 (4-5), 351–358. doi: 10.1016/j.funbio.2011.01.006
Nagaoka T., Nakata K., Kouno K. (2004). Antifungal activity of oosporein from an antagonistic fungus against Phytophthora infestans. Z. für Naturforschung C 59 (3-4), 302–304. doi: 10.1515/znc-2004-3-432
Najdabbasi N., Mirmajlessi S. M., Dewitte K., Landschoot S., Mänd M., Audenaert K., et al. (2020). Biocidal activity of plant-derived compounds against Phytophthora infestans: An alternative approach to late blight management. Crop Prot. 138, 105315. doi: 10.1016/j.cropro.2020.105315
Ngikoh B., Karim N. A. A., Jahim J., Bakar F. D. A., Murad A. M. A. (2017). Characterisation of cellulases and xylanase from Trichoderma virens UKM1 and its potential in oil palm empty fruit bunch (OPEFB) saccharification. J. Phys. Sci. 28, 171. doi: 10.21315/jps2017.28.s1.11
Nielsen K. F., Mogensen J. M., Johansen M., Larsen T. O., Frisvad J. C. (2009). Review of secondary metabolites and mycotoxins from the Aspergillus niger group. Anal. Bioanal. Chem. 395 (5), 1225–1242. doi: 10.1007/s00216-009-3081-5
O’Herlihy E. A., Duffy E. M., Cassells A. C. (2003). The effects of arbuscular mycorrhizal fungi and chitosan sprays on yield and late blight resistance in potato crops from microplants. Folia Geobotanica 38 (2), 201–207. doi: 10.1007/BF02803152
Olanya O. M., Larkin R. P. (2006). Efficacy of essential oils and biopesticides on Phytophthora infestans suppression in laboratory and growth chamber studies. Biocontrol Sci. Technol. 16 (9), 901–917. doi: 10.1080/09583150600827918
Pacilly F. C. A., Lammerts van Bueren E. T., Groot J. C. J., Hofstede G. J. (2019). Moving perceptions on potato late blight control: workshops with model-based scenarios. Crop Prot. 199, 76–87. doi: 10.1016/j.cropro.2019.01.010
Phillips J. M., Hayman D. S. (1970). Improved procedures for clearing roots and staining parasitic and vesicular-arbuscular mycorrhizal fungi for rapid assessment of infection. Trans. Br. Mycological Soc. 55 (1), 158–IN18. doi: 10.1016/s0007-1536(70)80110-3
Pozo M. J., Azcón-Aguilar C. (2007). Unraveling mycorrhiza-induced resistance. Curr. Opin. Plant Biol. 10 (4), 393–398. doi: 10.1016/j.pbi.2007.05.004
Quintanilla P., Rohloff J., Iversen T. H. (2002). Influence of essential oils on Phytophthora infestans. Potato Res. 45 (2), 225–235. doi: 10.1007/BF02736117
Rani A., Upadhyay S. K., Shukla G., Singh C., Singh R. (2021). Efficacy of bacterial isolates against causal agent of late blight of potato, Phytophthora infestans. Indian J. Agric. Res. 55 (4), 403–409. doi: 10.18805/IJARe.A-5548
Rashad Y. M., Moussa T. A. (2020). “Biocontrol agents for fungal plant diseases management”,” in Cottage industry of biocontrol agents and their applications. Eds. El-Wakeil N., Saleh M., Abu-hashim M. (Cham: Springer), 337–363. doi: 10.1007/978-3-030-33161-0_11
Ravnskov S., Jensen B., Knudsen I. M., Bødker L., Jensen D. F., Karliński L., et al. (2006). Soil inoculation with the biocontrol agent Clonostachys rosea and the mycorrhizal fungus Glomus intraradices results in mutual inhibition, plant growth promotion and alteration of soil microbial communities. Soil Biol. Biochem. 38 (12), 3453–3462. doi: 10.1016/j.soilbio.2006.06.003
Raymaekers K., Ponet L., Holtappels D., Berckmans B., Cammue B. P. (2020). Screening for novel biocontrol agents applicable in plant disease management–a review. Biol. Control 144, 104240. doi: 10.1016/j.biocontrol.2020.104240
Reino J. L., Guerrero R. F., Hernandez-Galan R., Collado I. G. (2008). Secondary metabolites from species of the biocontrol agent Trichoderma. Phytochem. Rev. 7 (1), 89–123. doi: 10.1007/s11101-006-9032-2
Ribeiro A. I., Costa E. S., Thomasi S. S., Brandã;o D. F. R., Vieira P. C., Fernandes J. B., et al. (2018). Biological and chemical control of Sclerotinia sclerotiorum using Stachybotrys levispora and its secondary metabolite griseofulvin. J. Agric. Food Chem. 66 (29), 7627–7632. doi: 10.1021/acs.jafc.7b04197
Rogozhin E. A., Vasilchenko A. S., Barashkova A. S., Smirnov A. N., Zavriev S. K., Demushkin V. P. (2020). Peptide extracts from seven medicinal plants discovered to inhibit oomycete Phytophthora infestans, a causative agent of potato late blight disease. Plants 9 (10), 1294. doi: 10.3390/plants9101294
Romdhane S., Devers-Lamrani M., Martin-Laurent F., Jrad A. B., Raviglione D., Salvia M. V., et al. (2018). Evidence for photolytic and microbial degradation processes in the dissipation of leptospermone, a natural β-triketone herbicide. Environ. Sci. pollut. Res. 25 (30), 29848–29859. doi: 10.1007/s11356-017-9728-4
Roy S., Singh B. P., Bhattacharyya S. K. (1991). Biocontrol of late blight of potato. Phytophthora News Lett. 17, 18.
Saksirirat W., Hoppe H. H. (1991). Secretion of extracellular enzymes by Verticillium psalliotae treschow and Verticillium lecanii (Zimm.) viegas during growth on uredospores of the soybean rust fungus (Phakopsora pachyrhizi syd.) in liquid cultures. J. Phytopathol. 131 (2), 161–173. doi: 10.1111/j.1439-0434.1991.tb04741.x
Sakthi S. S., Kanchana D., Saranraj P., Usharani G. (2012). Evaluation of amylase activity of the amylolytic fungi Aspergillus niger using cassava as substrate. Int. J. Appl. Microbiol. Sci. 1, 24–34.
Scervino J. M., Sampedro I., Ponce M. A., Rodriguez M. A., Ocampo J. A., Godeas A. (2008). Rhodotorulic acid enhances root colonization of tomato plants by arbuscular mycorrhizal (AM) fungi due to its stimulatory effect on the pre-symbiotic stages of the AM fungi. Soil Biol. Biochem. 40 (9), 2474–2476. doi: 10.1016/j.soilbio.2008.05.020
Scharf D. H., Brakhage A. A., Mukherjee P. K. (2016). Gliotoxin–bane or boon? Environ. Microbiol. 18 (4), 1096–1109. doi: 10.1111/1462-2920.13080
Schepers H. T. A. M., Kessel G. J. T., Lucca F., Förch M. G., Van Den Bosch G. B. M., Topper C. G., et al. (2018). Reduced efficacy of fluazinam against Phytophthora infestans in the Netherlands. Eur. J. Plant Pathol. 151 (4), 947–960. doi: 10.1007/s10658-018-1430-y
Shanthiyaa V., Saravanakumar D., Rajendran L., Karthikeyan G., Prabakar K., Raguchander T. (2013). Use of Chaetomium globosum for biocontrol of potato late blight disease. Crop Prot. 52, 33–38. doi: 10.1016/j.cropro.2013.05.006
Sharma B. K., Loganathan M., Singh R. P., Bag T. K., Rai R. K., Rai A. B., et al. (2011). Aspergillus niger, a potential biocontrol agent for controlling fusarial wilt of tomato. J. Mycopathol Res. 49 (1), 115–118.
Singh B. P., Sharma S. (2013). Forecasting of potato late blight. Int. J. Innovative Horticult. 2 (1), 1–11.
Soeka Y. S., Ilyas M. (2018). “Ability of Penicillium griseofulvum inacc f 14 in producing cellulase enzyme for composting media plant of white oyster mushroom (Pleurotus ostreatus jacq. ex fr.) p. kumm and ear mushrooms (Auricularia auricula j.),” in In IOP Conference Series: Earth and Environmental Science (Bristol, UK: IOP Publishing), Vol. 166. 012026. doi: 10.1088/1755-1315/166/1/012026
Son S. W., Kim H. Y., Choi G. J., Lim H. K., Jang K. S., Lee S. O., et al. (2008). Bikaverin and fusaric acid from Fusarium oxysporum show antioomycete activity against Phytophthora infestans. J. Appl. Microbiol. 104 (3), 692–698. doi: 10.1111/j.1365-2672.2007.03581.x
Sood M., Kapoor D., Kumar V., Sheteiwy M. S., Ramakrishnan M., Landi M., et al. (2020). Trichoderma: The “secrets” of a multitalented biocontrol agent. Plants 9 (6), 762. doi: 10.3390/plants9060762
Stephan D., Schmitt A., Carvalho S. M., Seddon B., Koch E. (2005). Evaluation of biocontrol preparations and plant extracts for the control of Phytophthora infestans on potato leaves. Eur. J. Plant Pathol. 112 (3), 235–246. doi: 10.1007/s10658-005-2083-1
Stewart H. E. (1990). Effect of plant age and inoculum concentration on expression of major gene resistance to Phytophthora infestans in detached potato leaflets. Mycological Res. 94 (6), 823–826. doi: 10.1016/S0953-7562(09)81385-3
Stewart H. E., Flavelle P. H., McCalmont D. C., Wastie R. L. (1983). Correlation between glasshouse and field tests for resistance to foliage blight caused by Phytophthora infestans. Potato Res. 26 (1), 41–48. doi: 10.1007/BF02357372
Stierle A. A., Stierle D. B. (2015). Bioactive secondary metabolites produced by the fungal endophytes of conifers. Natural Product Commun. 10, (10). doi: 10.1177/1934578X1501001012
Tang H. Y., Zhang Q., Li H., Gao J. M. (2015). Antimicrobial and allelopathic metabolites produced by Penicillium brasilianum. Natural Product Res. 29 (4), 345–348. doi: 10.1080/14786419.2014.940347
Valente S., Cometto A., Piombo E., Meloni G. R., Ballester A. R., González-Candelas L., et al. (2020). Elaborated regulation of griseofulvin biosynthesis in Penicillium griseofulvum and its role on conidiation and virulence. Int. J. Food Microbiol. 328, 108687. doi: 10.1016/j.ijfoodmicro.2020.108687
Van Wees S. C., van der Ent S., Pieterse C. M. (2008). Plant immune responses triggered by beneficial microbes. Curr. Opin. Plant Biol. 11 (4), 443–448. doi: 10.1016/j.pbi.2008.05.005
Vargas W. A., Mukherjee P. K., Laughlin D., Wiest A., Moran-Diez M. E., Kenerley C. M. (2014). Role of gliotoxin in the symbiotic and pathogenic interactions of Trichoderma virens. Microbiology 160 (10), 2319–2330. doi: 10.1099/mic.0.079210-0
Vinale F., Sivasithamparam K., Ghisalberti E. L., Ruocco M., Woo S., Lorito M. (2012). Trichoderma secondary metabolites that affect plant metabolism. Natural Product Commun. 7 (11), 1545–1550. doi: 10.1177/1934578X1200701133
Viterbo A. D. A., Wiest A. R. I. C., Brotman Y., Chet I. L. A. N., Kenerley C. (2007). The 18mer peptaibols from trichoderma virens elicit plant defence responses. Mol. Plant Pathol. 8 (6), 737–746. doi: 10.1111/j.1364-3703.2007.00430.x
Wang S., Hu T., Zhang F., Forrer H. R., Cao K. (2007). Screening for plant extracts to control potato late blight. Front. Agric. China 1 (1), 43–46. doi: 10.1007/s11703-007-0007-x
Weindling R. (1934). Studies on a lethal principle effective in the parasitic action of Trichoderma lignorum on Rhizoctonia solani and other soil fungi. Phytopathology 24 (11), 1153–1179.
Wu Z. H., Ma Q., Sun Z. N., Cui H. C., Liu H. R. (2021). Biocontrol mechanism of Myxococcus fulvus B25-I-3 against Phytophthora infestans and its control efficiency on potato late blight. Folia Microbiologica 66, 555–567. doi: 10.1007/s12223-021-00865-1
Keywords: biological control agent (BCA), late blight, Bintje and Annabelle, fungal filtrate, antagonism, alginate beads, secondary metabolites, induced resistance
Citation: Lalaymia I, Naveau F, Arguelles Arias A, Ongena M, Picaud T, Declerck S and Calonne-Salmon M (2022) Screening and efficacy evaluation of antagonistic fungi against Phytophthora infestans and combination with arbuscular mycorrhizal fungi for biocontrol of late blight in potato. Front. Agron. 4:948309. doi: 10.3389/fagro.2022.948309
Received: 19 May 2022; Accepted: 04 July 2022;
Published: 02 August 2022.
Edited by:
David Ezra, Agricultural Research Organization (ARO), IsraelReviewed by:
Laure Weisskopf, Université de Fribourg, SwitzerlandSaif Ullah, University of Agriculture, Faisalabad, Pakistan
Copyright © 2022 Lalaymia, Naveau, Arguelles Arias, Ongena, Picaud, Declerck and Calonne-Salmon. This is an open-access article distributed under the terms of the Creative Commons Attribution License (CC BY). The use, distribution or reproduction in other forums is permitted, provided the original author(s) and the copyright owner(s) are credited and that the original publication in this journal is cited, in accordance with accepted academic practice. No use, distribution or reproduction is permitted which does not comply with these terms.
*Correspondence: Stéphane Declerck, stephan.declerck@uclouvain.be