Weed control with saturated steam in organic highbush blueberry
- 1Department of Horticulture, Oregon State University, Corvallis, OR, United States
- 2Department of Crop Science, University of São Paulo, Piracicaba, SP, Brazil
Weed management is often a predominant and costly problem in the production of organic blueberries. Geotextile weed fabrics of woven polyethylene are widely used in organic blueberry fields to suppress weeds growing within the rows. Weeds, such as Convolvulus arvensis L., grow at the base of the blueberry plants or through openings and around the edges of the weed fabric, thus requiring hand weeding. This study evaluates the integration of saturated steam (SS), a rotary brush (RB), and organic herbicides for weed control in blueberries. Dose–response studies indicated that SS applied at 121°C and at 7.4 m3 ha−1 of steam (3,655 MJ ha−1) resulted in over 90% control and a reduction in the dry weights of C. arvensis. When treatments were directed to the base of the blueberry plants, SS at 7.4 m3 ha−1 provided 80% control of C. arvensis 28 days after treatment (DAT) and was comparable to hand weeding. Both of these treatments outperformed capric plus caprylic acid (CC) (33.2 kg ai ha−1) or ammonium nonanoate (AN) (24.3 kg ai ha−1) applications, despite C. arversis regrowth being observed. Four repetitive basal applications of SS of up to 29.6 m3 ha−1 over two consecutive years caused minimal and transient damage to new basal shoots of ‘Elliot’ and ‘Duke’ blueberries; basal shoot cross-sectional area compared with the non-treated was unaffected. In contrast, basal application of AN treatments damaged or killed basal shoots. When treatments were applied to the edge of the weed fabric, SS (7.4 m3 ha−1) reduced weed biomass by 42% to 93% at 28 DAT compared with the non-treated. The RB treatment reduced weed biomass from 72% to 99% in all experiments, while CC and AN reduced biomass by 18% to 54%. A partial budget analysis indicated that SS and the RB were 3- and 6.5-fold less expensive than organic herbicides, respectively. Integrating physical (SS) and mechanical (RB) treatments improved weed control. The latter, however, damaged the weed-suppressing fabric where preexisting holes were present, generated dust, and increased the chance of fruit contamination. The SS was safe for the weed-suppressing fabric and the blueberry, but weed regrowth following treatment and copious water requirements hindered its feasibility.
1 Introduction
Highbush blueberry is economically important in the United States, with the production being valued at over US$986 million in 2022 (USDA, 2023). The hectarage in the USA has increased continuously over the past decades, rising by almost 250%, increasing from 16,320 ha in 2000 to nearly 40,000 ha in 2021 (USDA, 2023). The western United States is an important region for the production of blueberries; together Oregon and Washington account for roughly 35% of domestic hectarage. The Pacific Northwest is the world’s largest producer of organic highbush blueberries. Proper weed management is one of the most challenging aspects of organic production, as producer options are limited (Strik and Vance, 2016). Weed management is often identified as a major, costly production problem, hindering the expansion of organic adoption (Strik, 2016). Weed competition can make highbush blueberry economically inviable, as it has been shown to reduce blueberry growth by 38% and yield by up to 92% (Burkhard et al., 2009).
Highbush blueberry plants have a shallow root system located in the top 0.5 m of soil (Valenzuela-Estrada et al., 2008) and, consequently, are sensitive to weed competition. Soil tillage is detrimental to blueberry plants. Blueberries are often grown in raised beds with sawdust mulch to improve soil drainage and plant growth (Strik, 2016). Synthetic geotextile fabrics, commonly called weed mats or synthetic mulches, have become increasingly commonplace in organic blueberry fields in the past decade as they effectively suppress weed growth within the planting rows (Strik, 2016). Synthetic mulches are placed over the sawdust mulch for optimal results (Strik and Davis, 2021). These synthetic mulches require a significant initial investment but are cost-effective as they can last for many years, reduce labor in weeding, and improve crop growth. However, creeping and climbing weed species, such as Convolvulus arvensis L., can grow through the openings at the base of the blueberry plants and around or over the weed fabric’s edges, evading proper control (Figure 1). For this reason, organic producers often rely on labor-intensive hand weeding in those areas to prevent yield losses and interference during crop harvest. The increasing costs and labor scarcity require new weed management approaches in organic blueberry fields (Strik and Vance, 2016).
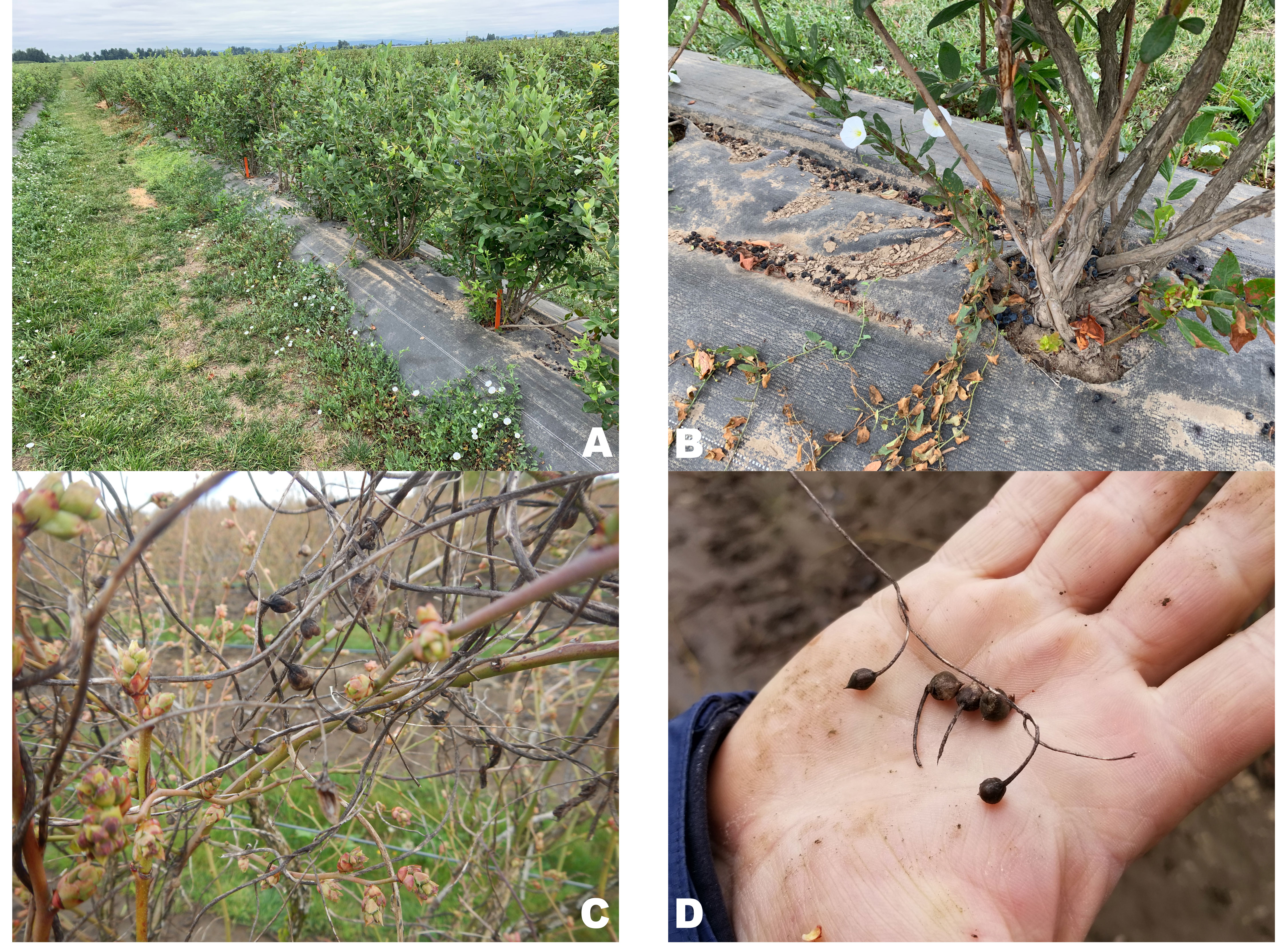
Figure 1 Convolvulus arvensis in organic highbush blueberry (A). The shoots emerge in the spring and can climb the plants when growing at the base of the plant or in adjacent areas (B). The C. arvensis shoots will hinder the mechanical harvest and will set seeds in the fall (C, D).
Sustainable organic blueberry production requires cost-effective weed control methods compatible with blueberry and synthetic mulches. Organic herbicides are compatible with the production systems, but often do not have the efficacy required (Strik and Vance, 2016) and are costly (Dayan and Duke, 2010). Flaming has proven incompatible with synthetic mulches, although weed control with steam and hot water has been successful in other systems (Hansson, 2002; Kristoffersen et al., 2008). Steam transfers thermal energy to the targeted plants, raising tissue temperature and causing cell damage (Bauer et al., 2020). Another approach for thermal weed control is hot foam, which utilizes a biodegradable foaming agent that will trap the heat and improve weed control (Antonopoulos et al., 2023) Rotary brushes are a mechanical weed control option; these brushes rotate along vertical or horizontal axes, uprooting weeds in vegetable systems (Melander, 1997). The literature lacks data about the efficacy of physical and chemical options for weed control in organic blueberries, whether applied alone or in combination, as well as energy demands and associated costs. In this study, we aimed to assess the crop safety and efficacy of organic weed control by saturated steam (SS), a rotary brush (RB), and organic herbicides in blueberries.
2 Materials and methods
Two study protocols were developed to evaluate organic weed control tools in northern highbush blueberry. The first study goal was to assess the impact of steam temperature and the speed of operation on weed control. The second study goal was to compare the efficacy of different weed control tools and their combinations for organic blueberry production.
2.1 The saturated steam equipment
A commercial unit generated saturated steam (SS) (Satusteam™ SW900; Weedtechnics, Terrey Hills, NSW, Australia) for this study (Figure 2). The unit has a diesel-powered boiler operating at 6,205 kPa and generating 0.6 m3 h−1 of SS. The boiler consumes 2 L of diesel per hour. The SS temperature can be regulated from ambient to 121°C, with the highest temperature being recommended by the manufacturer. A gasoline-powered pump moves the water from the reservoir (0.4 m3) using 0.5 L h−1. A circular applicator, with a 0.55-m radius, or a hand-held device 0.5 m wide delivers SS at ambient pressure. A metal nozzle is mounted inside the circular applicator. The equipment is mounted on a 1.2-m trailer and towed by a tractor. The steamer was pulled by a 14-kW tractor. The published information on SS performance on weed control was limited. The first study goal was to assess the impact of steam temperature and application volume on weed control.
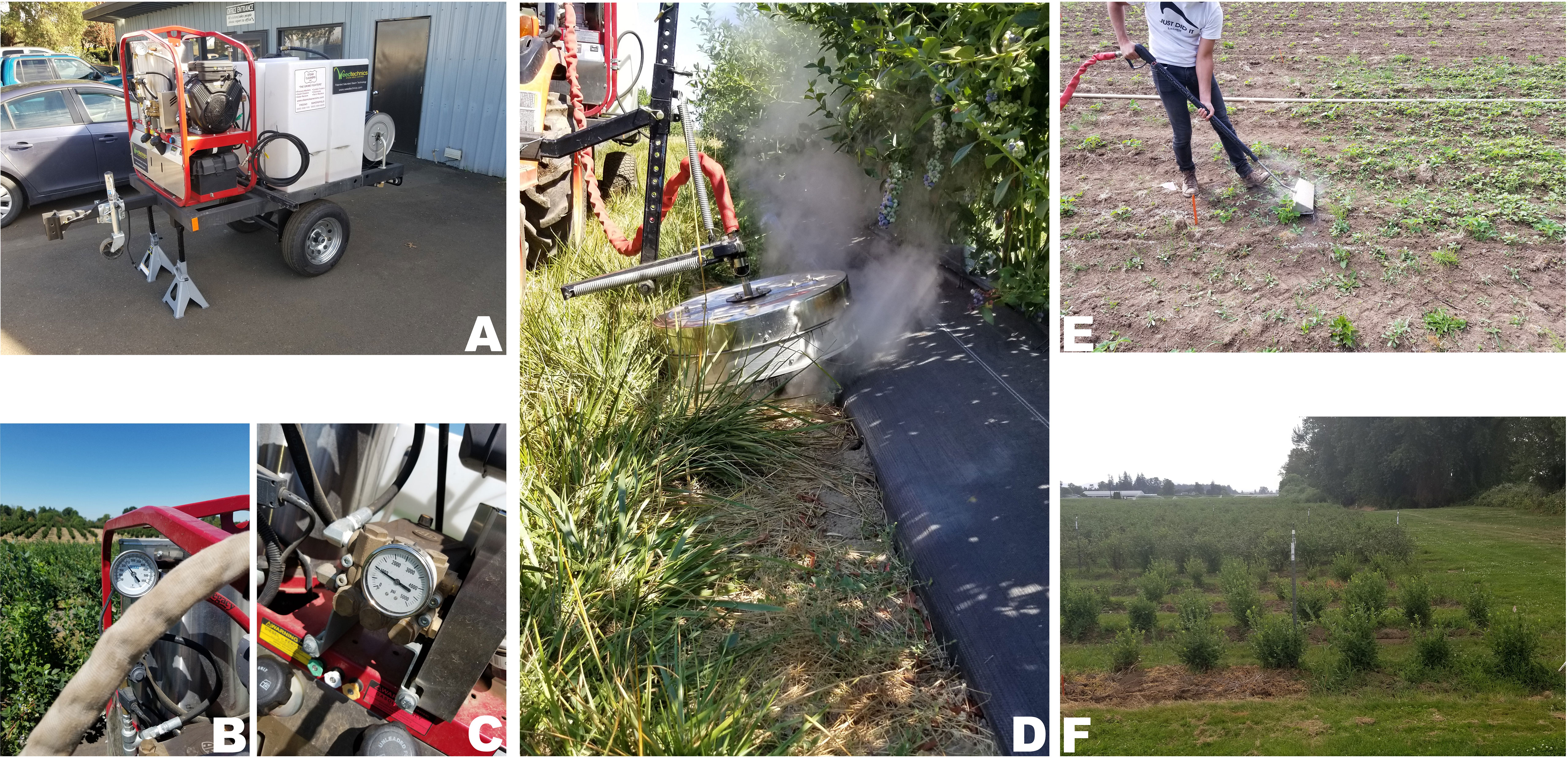
Figure 2 The steamer SW900 (Weedtechnics) was modified to fit a 1.2-m-wide trailer (A). It was operating at 121°C (B) and at 6,205 kPa producing 0.6 m3 h−1 of saturated steam (SS) (C). The SS was delivered by a 0.5-m circular tractor-mounted applicator (D) or a hand-held unit (E). The effects on the weeds included wilting, which was noticeable immediately, with foliage death observed in a few days (F).
2.2 Saturated steam boiler temperature and application volume
Two field experiments were performed to compare boiler temperatures ranging from ambient to 121°C. The sites were infested with C. arvensis (Table 1). Treatments included ambient temperature (~ 26°C ± 6°C), and 65°C, 79°C, 93°C, 107°C, and 121°C boiler temperatures. All treatments were applied by the tractor with the circulator applicator at a constant steam volume of 7.4 m3 ha−1. The experimental plots were 0.5 m wide by 3 m long; treatments were applied with the hand-held unit to ensure a constant boiler temperature.
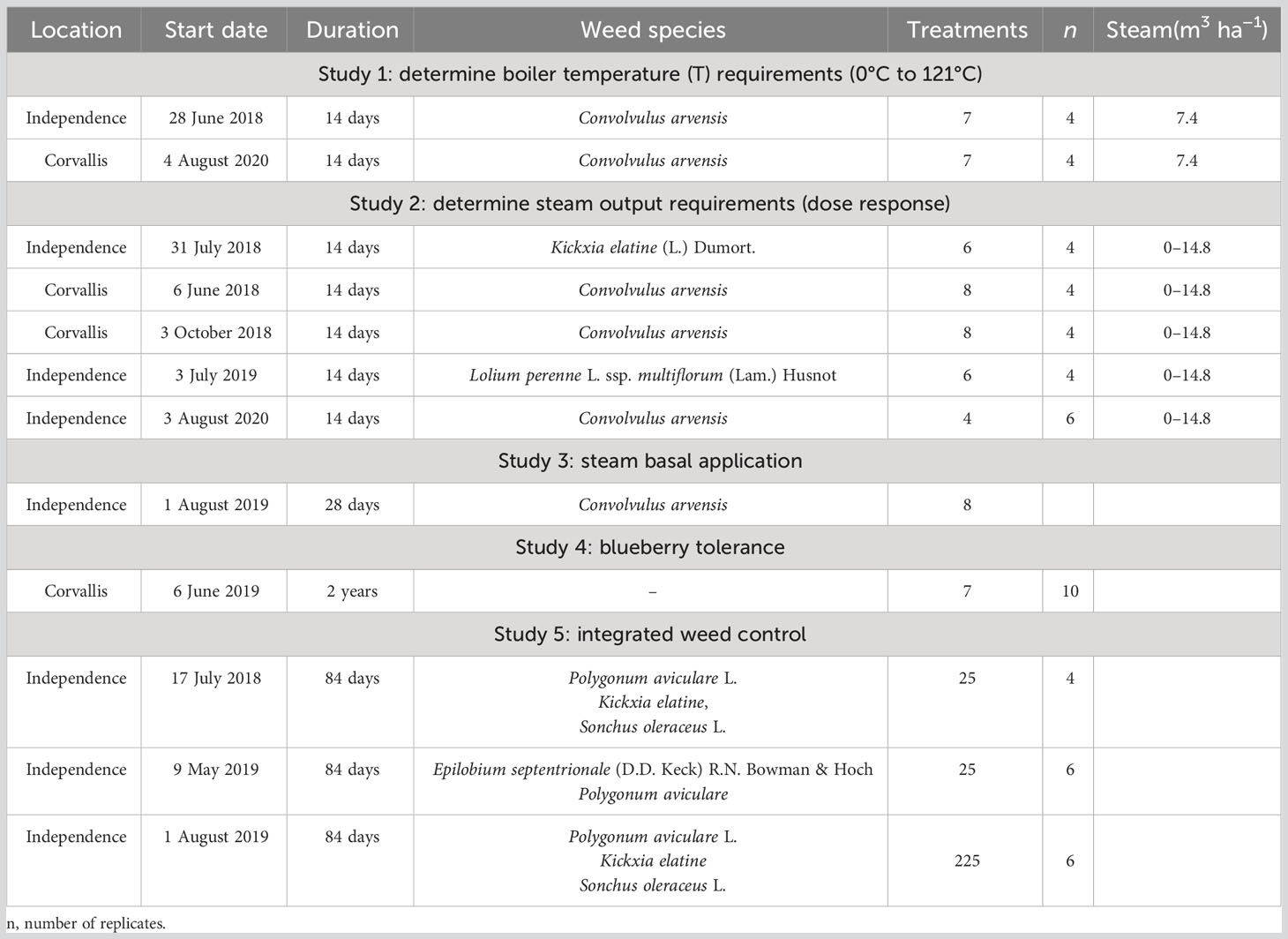
Table 1 Summary of field studies conducted for each research objective by trial location (Corvallis and Independence, OR, USA) and start date.
Six additional studies were conducted to evaluate application volume in different weed species (Table 1). The dosage level was varied by adjusting the travel speed from 0.4 km h−1, 0.8 km h−1, 1.6 km h−1, 2 km h−1, and 4 km h−1. A non-treated control was included as a reference. The experimental plots were 0.5 m by 5 m in the application volume studies. All studies were designed as randomized complete blocks, with four replicates per treatment level. The treatment consisted of a single application of SS. The above-ground biomass was sampled 14 days to 18 days after treatment (DAT). A single quadrat (0.5 m by 0.5 m) per plot was placed in the plot’s center, and the biomass was hand-harvested and dried in an oven at 65.6°C until it reached a constant weight and then it was weighed.
2.3 Basal application of saturated steam in blueberry
2.3.1 Blueberry tolerance
The blueberry shrub consists of several shoots originating from buds at the plant base; the shoots continue to grow for many years. In their second year, shoots, called canes, are surrounded by the periderm, or bark, while the younger shoots are surrounded by a cuticle (Gough, 1993). These 1-year-old shoots are essential to defining blueberry bush architecture and productivity (Strik et al., 2014). Any damage to the young basal shoots can have detrimental long-term effects on blueberry bushes, as new shoots are trained to replace older, less unproductive canes (Strik et al., 2003). Blueberry cultivars can differ in basal shoot number, length, and growth vigor, so the impact of weed management may be cultivar dependent (Strik et al., 2014).
The basal application of SS was evaluated to control weeds growing at the plant’s base; this application was compared with an organic herbicide applied as a spray or with a sponge wiper. Both management strategies were compared for efficacy and crop tolerance. A 2-year study was conducted on the Corvallis, OR OSU Lewis Brown Research farm in a mature highbush blueberry field. The blueberry plants were 3.35 m tall, spaced 0.9 m apart and supported on a “T” trellis system; the raised berms were 0.3 m in height and 1.2 m in width. The cultivars ‘Duke’ and ‘Elliot’ were planted in adjacent field sections. Surface drip irrigation was installed on both sides of the planting row, and the field was mulched with sawdust beneath the synthetic mulch.
The experiments consisted of 10 treatments, which were organized as a randomized complete block replicated four times. The experimental plots included three plants. Each cultivar was an independent study. The treatments were applied to the base of the blueberry plants targeting the lower 0.5 m of the plant, and a non-treated control was included as a reference. The SS was applied at 7.4 m3 ha−1, 14.8 m3 ha−1, and 29.6 m3 ha−1 using the hand-held applicator. The herbicide ammonium nonanoate (AN) (AXXE®; BioSafe Systems, LLC, East Hartford, CT, USA) was applied as a basal-directed spray at 24.3 kg ai ha−1, 48.6 kg ai ha−1, and 97.2 kg ai ha−1, or the equivalent of a field rate, two, and four times the field rate. The approved field rate for blueberry is up to 13% vol/vol of the commercial product in 0.74 m3 ha−1 of carrier volume. The AN treatment used a CO2-pressurized backpack sprayer with three AI 11008 (TeeJet®) nozzles at 275 kPa. The AN was also tested as a sponge-wiper application at one, two, and four times the field rate. The sponge-wiper application was by hand-held sponger wiper (Drift Free Green Sponge Gun Weed Wiper; Smucker, Harrisburg, OR), connected to a pressurized backpack sprayer, and saturated before each application. The sponge-wiper treatments included AN at 13% vol/vol applied for one, two, or four passes; manipulating the spray concentration could affect solution viscosity and delivery. The treatments were applied on 17 June 2019, and reapplied on 29 July 2019, 42 days after the initial treatment (DAIT). The study was repeated in 2020, with the treatments applied on 19 June 2020 (368 DAIT), and reapplied on 30 July 2020 (409 DAIT).
The visual estimates of basal shoot and canopy injury were assessed on a 0% to 100% scale, with 0% being no effect and 100% being plant death. Injury was recorded monthly throughout the experiment. No canopy injury was observed during the study. At the end of the experiment, the diameter of three basal shots per plot was recorded at 0.2 m above the ground and converted to a cross-sectional area. The length of the shoot was also recorded at that time.
2.3.2 Efficacy of C. arvensis control
A commercial organic blueberry field near Independence, OR, USA, planted with ´Last Call´ blueberries and infested with C. arvensis was used for the study. The blueberry plantings were 3.35 m × 0.90 m apart. The plants were grown on berms, 0.3 m high by 1.2 m wide. The berms were mulched with Douglas fir [Pseudotsuga menziesii (Mirb.) Franco] sawdust covering black geotextile polyethylene landscape fabric. The blueberry bushes were trellised in a two-wire “T” trellis system, with a drip irrigation line placed on both sides of the bushes. The experiment was initiated in May 2019 when the C. arvensis shoots were between 10 cm and 15 cm in length. The plants with C. arvensis were selected for the study, which consisted of seven treatments organized in a randomized complete block design with four replicates. Each experimental unit consisted of three blueberry plants. The treatments included SS, the organic herbicide capric plus caprylic acid (CC) (SUPRRESS®; Westbridge Agricultural Products, Vista, CA, USA), and AN applied as spray or by sponge wiper. Hand weeding and a non-treated control were included as references. The SS treatments were applied using by hand-held application directed to the lower 50 cm of the plant as a single pass delivering 7.4 m3 ha−1 at 121°C and at 6,205 kPa. The spray application of CC and AN were made at 19 kg ai ha−1 and 24.2 kg ai ha−1, the equivalent of 9% vol/vol and 13% vol/vol in 748 L ha−1 of carrier volume, respectively. The sponge wiper applications of CC and AN were made at 9% vol/vol and 13% vol/vol, respectively. The assessments included visual estimates of crop injury and C. arvensis control, made at 7 DAT and 28 DAT on a scale of 0 to 100 representing no control to complete control, respectively. Crop injury was similarly estimated as indicated in the previous section. At 28 DAT, C. arvensis shoots and leaves were collected, dried, and weighed.
2.3.3 Integrated weed control
Three field trials were conducted in 2018 and 2019 in commercial organic highbush blueberry, as described in section 2.2.2. These trials will be referred to as experiment 1, initiated in the summer of 2018; experiment 2, initiated in the spring of 2019; and experiment 3, initiated in the summer of 2019. The cultivars in experiments 1, 2, and 3 were ‘Last Call’, ‘Mega Blue’, and ‘Aurora’, respectively.
The experiments were organized as a 5 × 5 factorial in a randomized complete block design with four replications in experiment 1 and six in experiments 2 and 3. The experimental units were 3.35 m by 25 m and included 26 blueberry plants. Factor A was the first application of SS (7.4 m3 ha−1), a RB, AN (24.2 kg ai ha−1), or CC (19.2 kg ai ha−1), and a non-treated control as reference. Factor B was a second treatment applied 28 days later, resulting in 25 combinations. The retreatment interval was selected based on the optimum for hot water retreatment interval (Hansson and Ascard, 2002). All treatments were applied to a 0.5-m strip of ground running parallel to the weed mat on both sides of the row. The SS treatments were applied by a tractor-mounted circular applicator. The brush weeding was performed by an in-row weeder with a rotary brush attachment (ID David, Murcia, Spain). The brush consists of nylon brushes rotating at 4,000 revolutions per minute (rpm) to 5,000 rpm in the vertical plane in the direction of tractor movement at 1.6 km h−1. The brush was positioned to remove weeds at the soil surface while having minimal contact with the plastic mulch. The AN and CC were applied by CO2-pressurized backpack sprayer using AI11008 nozzles, which were calibrated to deliver 0.74 m3 ha−1.
Weed control and green weed coverage were recorded at 28 DAT and 56 DAT. Weed control was assessed on a scale from 0% to 100%, where 0% represents no effect or control, and 100% was death of all target plants. The green weed coverage was assessed using the online image analysis tool Canopeo (Patrignani and Ochsner, 2015), with red-to-green and the blue-to-green ratios set at 0.95, and excess green set at 20. The pictures were taken at 1.5 m above the soil level using a point-and-shoot digital camera (Nikon Coolpix W300). The above-ground weed biomass was recorded at 28 DAT and 56 DAT in different locations within the experimental unit. Biomass was dried, and weight was recorded.
2.4 Statistical analysis
The SS output per time was converted into volume per area by Equation 1:
where the SS dose is saturated steam in m3 of SS ha−1, the SS flow is the flow rate of SS in m3 SS h−1, s is the operational speed in m h−1,and w is the width of the treated area in m. The result is multiplied by a factor to convert the rate to a per ha basis.
The SS physical properties under isobaric conditions of 6,205 kPa were used to calculate the energy density in MJ ha−1 (NIST, 2023), using Equation 2:
where is the energy density applied (MJ ha−1), Vol is the SS volume (m3 ha−1), density of saturated steam (kg m−3), and H is the enthalpy in MJ kg−1. A similar calculation was performed to calculate the energy under different SS temperature conditions.
The recorded above-ground dry weights were fitted to a three-parameter non-linear regression using the DRC package v 3.0.1 (Ritz et al., 2015) of R software v 4.3.0 (R Core Team, 2023), as seen in Equation 3:
where d is the upper limit of the variable, b is the slope of the curve around the inflection point, x is the energy rate and e is the inflection point for 50% reduction in biomass. The Akaike information criterion was used to select the best model. The ED procedure in DRC package was used to estimate the effective doses causing a 90% and 99% reduction in biomass. The experiments were analyzed separately because of the differences in the weed species present.
Blueberry basal shoot length, shoot caliper, and weed biomass were fitted to a generalized linear mixed model using package lme4 version 1.1–3.4 and the “lmer” procedure. The assumptions of normality and homogeneity of variance were tested by Shapiro–Wilk’s and Levene’s tests, respectively. A log + 1 transformation was applied to the weed biomass data to meet these assumptions; back-transformed data are presented. The weed control and injury data were fitted to a generalized linear mixed model with a logit link distribution using the glmmTMB package version 1.1.7. ANOVA was performed using the glmmTMB function and the means were separated by the Dunnett’s test when compared with the non-treated or Sidak’s test when making multiple comparisons using the emmeans (estimated marginal means) package v 1.8.7.
2.5 Partial budget analysis
A partial budget analysis compared application costs for the different weed control treatments. For the steamer and brush, variable costs were calculated by adding the equipment costs, fuel usage, and labor. For the organic herbicides, variable costs were calculated by adding the herbicide costs, fuel usage, and labor. Diesel charges of US$1.09 L−1 and gasoline charges of US$1.23 L−1 were used to calculate the cost of fuel. Labor was charged at US$19 h−1. Tractor hourly costs were based on methods proposed by Edwards (Edwards, 2023). The fixed costs included machinery and spray equipment. The analysis did not include the purchase prices of the tractor or the sprayer. The final cost was calculated for the treatment of one-fifth of the total field, or the equivalent area adjacent to the geotextile weed fabric.
3 Results
3.1 Saturated steam boiler temperature and application volume
Weed control with SS relies on the transference of thermal energy stored in the SS to the weed foliage. Heat transfer increases with the magnitude of the temperature difference (Ascard et al., 2007). The energy level applied when changing the boiler temperature from 38°C to 121°C was 1,214 MJ ha−1 to 3,586 MJ ha−1 at 7.4 m3 ha−1 of SS (Figure 3). Control and biomass reduction of C. arvensis was only observed at SS temperatures above 65°C (2,018 KJ ha−1). The energy required to provide 50% control or biomass reduction (ED50) was 2,386 MJ ha−1 and 1,563 MJ ha−1, respectively, and 1.6- to 2.3-fold more energy was required to provide 90% control or biomass reduction (ED90), respectively (Table 2).
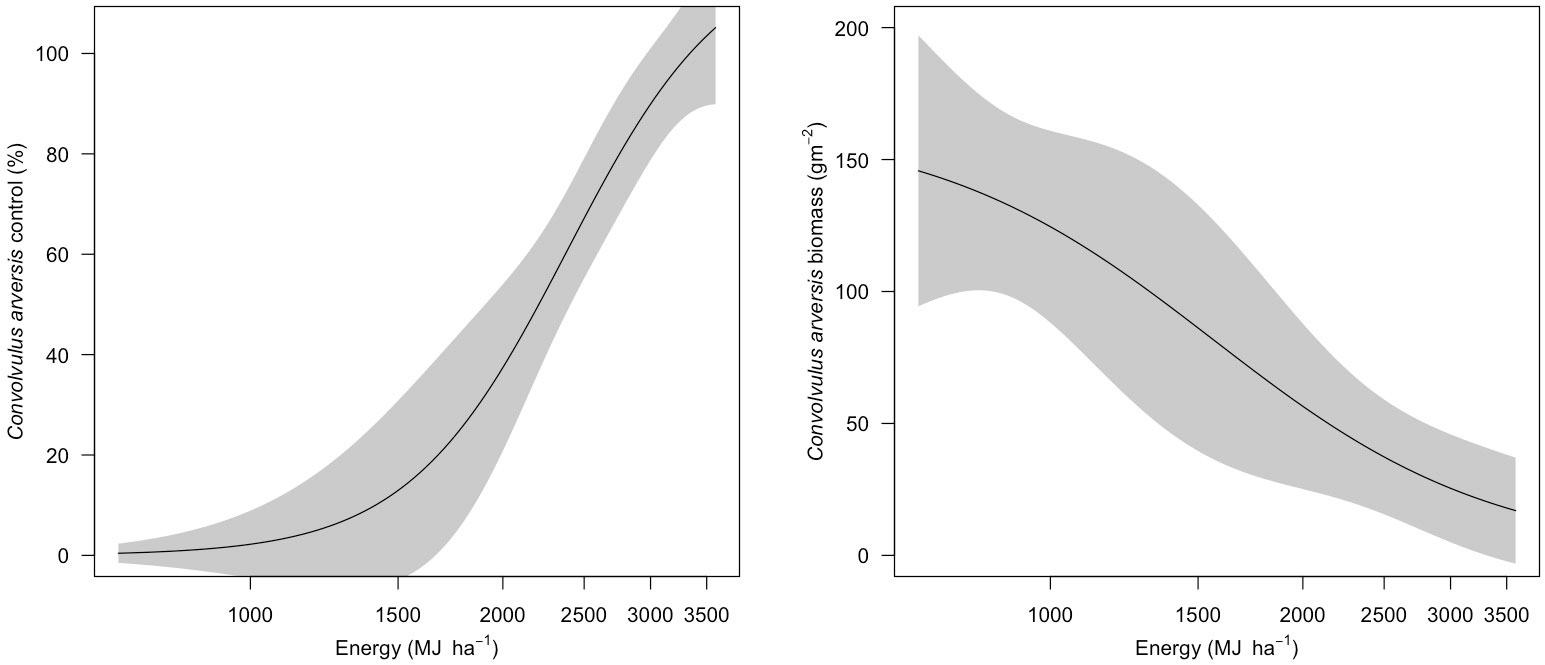
Figure 3 Convolvulus arvensis control (%) and above-ground dry biomass response to the energy level applied by manipulating the saturated steam boiler temperature. The shaded area indicates a 95% confidence interval. The saturated steam was applied at 7.4 m3 ha−1, and data were recorded 28 days after treatment.
The energy applied was also manipulated by altering the application volume; however, as SS volume increases, the cost of treatment increases because of the greater consumption of water, fuel, and labor. The efficacy of the SS was weed species dependent (Table 3, Figure 4), when increasing energy (and volumes) from 0 MJ ha−1 to 7,173 MJ ha−1 (0 m3 ha−1 to 14.8 m3 ha−1) at a constant temperature of 121°C. The ED50 values of dicotyledonous species Kickxia elatine (L.) Dumort., C arvensis, and Amaranthus retroflexus L. were 827 MJ ha−1, 1,563 MJ ha−1, and 1,767 MJ ha−1, respectively. The ED90 values for the same species ranged from 1,853 M J ha−1 to 3,643 M J ha−1, which were below or similar to the energy delivered when applying 7.4 m3 ha−1 of SS. By contrast, the ED50 for the monocots Festuca rubra L. and Lolium perenne ssp. multiflorum (syn. Lolium multiflorum Lam.) ranged from 1,769 MJ ha−1 to 2,429 MJ ha−1. The ED50 could not be calculated for L. multiflorum because the energy required to impact it was > 7,173 MJ ha−1. Similarly, the ED90 and ED99 for the monocot species were beyond the tested rates, and thus not calculated. These results confirmed that operating the boiler at the maximum temperature more effectively controlled C. arvensis and applying SS at 7.4 m3 ha−1 controlled most dicotyledonous plants for 14 days.
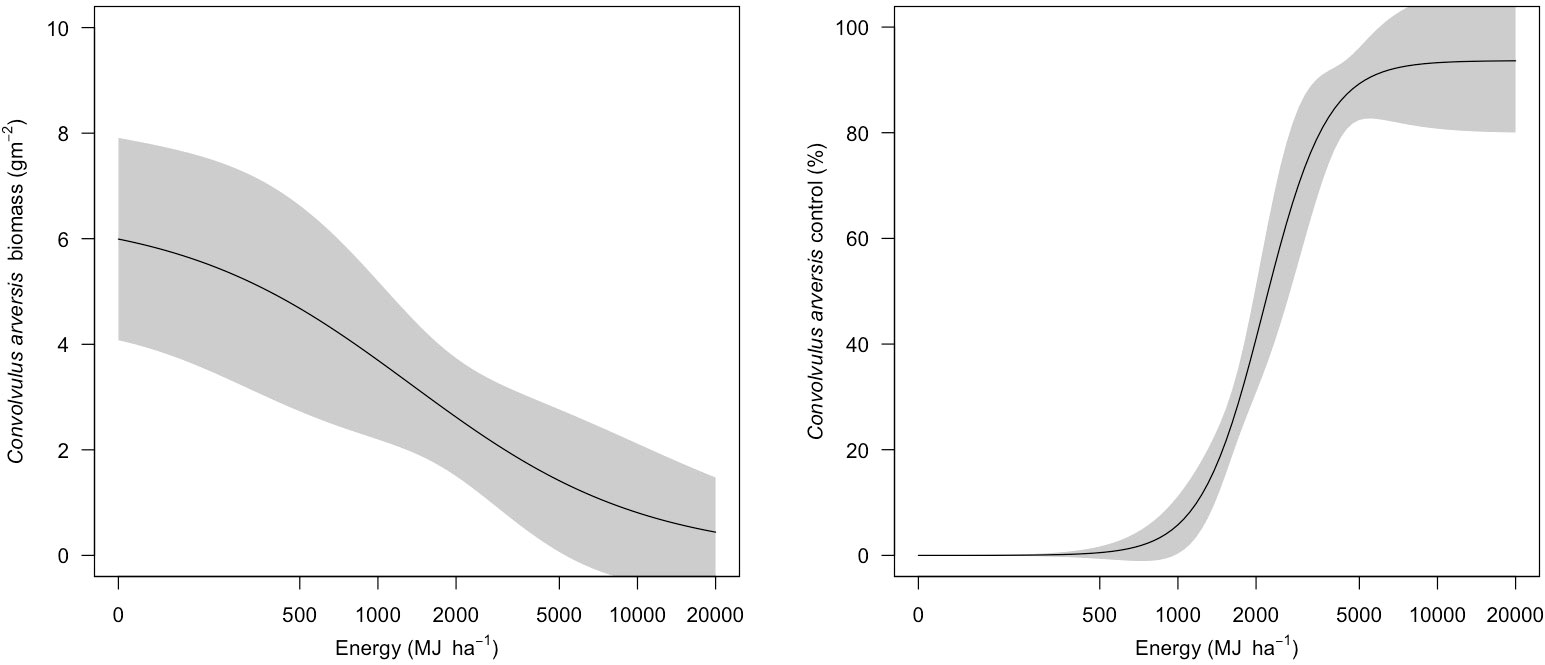
Figure 4 Convolvulus arvensis above-ground dry biomass and the control in response to increasing energy levels applied with saturated steam. The energy levels were applied by changing the amount of saturated steam applied per area at 121°C and at 6,205 kPa. The shaded area indicates a 95% confidence interval. The data were recorded 28 days after treatment.
3.2 Basal application of saturated steam in blueberry
3.2.1 Blueberry tolerance
The basal applications of SS at 7.4 m3 ha−1 and 14.8 m3 ha−1 resulted in 10% to 15% injury to cultivar ‘Duke’ at 14 DAIT, while nearly 50% injury was observed with SS at 29.6 m3 ha−1 (Figure 5). At all the tested rates, the injury due to SS was limited to the shoot’s lower foliage, and SS injury to basal shoots increased after SS but later diminished regardless of the SS rate. The spray treatment of AN at 24.3 kg ai ha−1 caused 63% injury at 14 DAIT and reduced to 50% at 28 DAIT, but injury rates of 30% remained by the end of the study. The injury rate increased with AN rate and remained at 50% to 75% at 480 DAIT with the higher rates. The AN applications with the sponger wiper were as injurious to 1-year-old shoots as was an AN spray application. The ‘Elliot’ blueberry response to the treatments was comparable to ‘Duke’, with AN treatments causing marginally higher injury levels in ‘Elliot’.
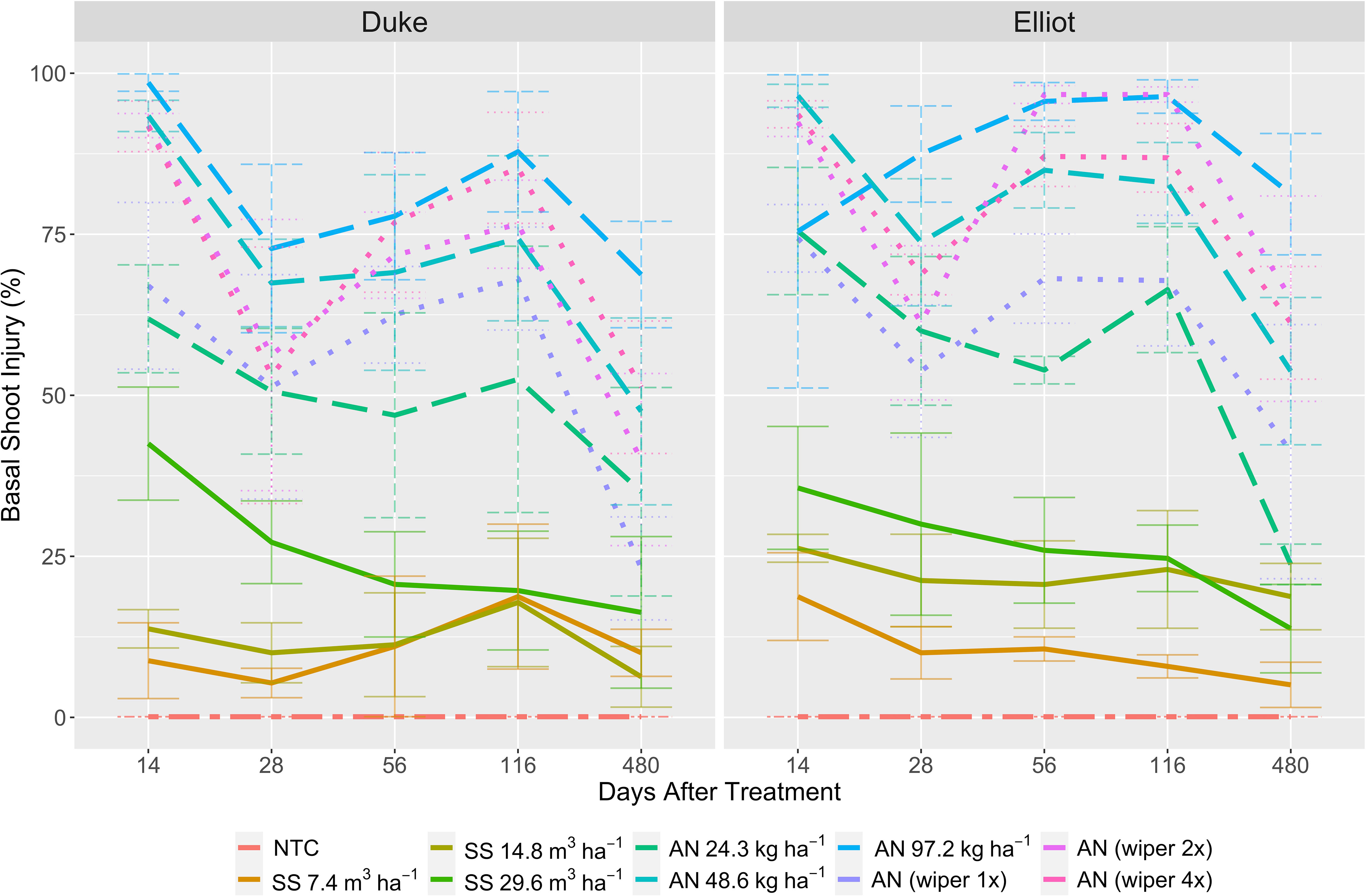
Figure 5 Highbush blueberry basal shoot injury response to increasing levels of saturated steam or ammonium nonanoate. The mature plants of cultivars ‘Duke’ and ‘Elliot’ were used in this study. The treatments were applied to the lower 50 cm of the blueberry bush. The treatments presented on the graph are non-treated (NTC) (short–long–dashed line), saturated steam (SS; solid line), and ammonium nonanoate, which was applied as a spray (long dashed line) or as a wiper treatment (dotted line). The treatments were applied in June 2019 and reapplied 42 days later. The study was repeated in 2020 with treatments applied 368 days and 409 days after the initial treatment. The means and standard errors are presented (n = 4).
Cross-sectional area and length of non-treated blueberry averaged 70 mm2 and 71.8 cm in ‘Duke’ and 72.5 mm2 and 85.4 cm in ‘Elliot’ (Figure 6), respectively. The treatment with SS up to 29.3 m3 ha−1 did not affect basal shoot cross-sectional area or length compared with non-treated based on Dunnett’s test regardless of cultivar. AN sprayed at 97.2 kg ai ha−1 reduced the cross-sectional areas and lengths of basal shoots of ‘Duke’ plants by 57% and 62%, respectively, compared with non-treated plants. In the cultivar ‘Elliot’, AN reduced cross-sectional area and length by 45% and 52%, respectively. The detrimental effects of AN applied with a sponge-wiper were noticed in the ‘Elliot’ two- and fourfold treatments.
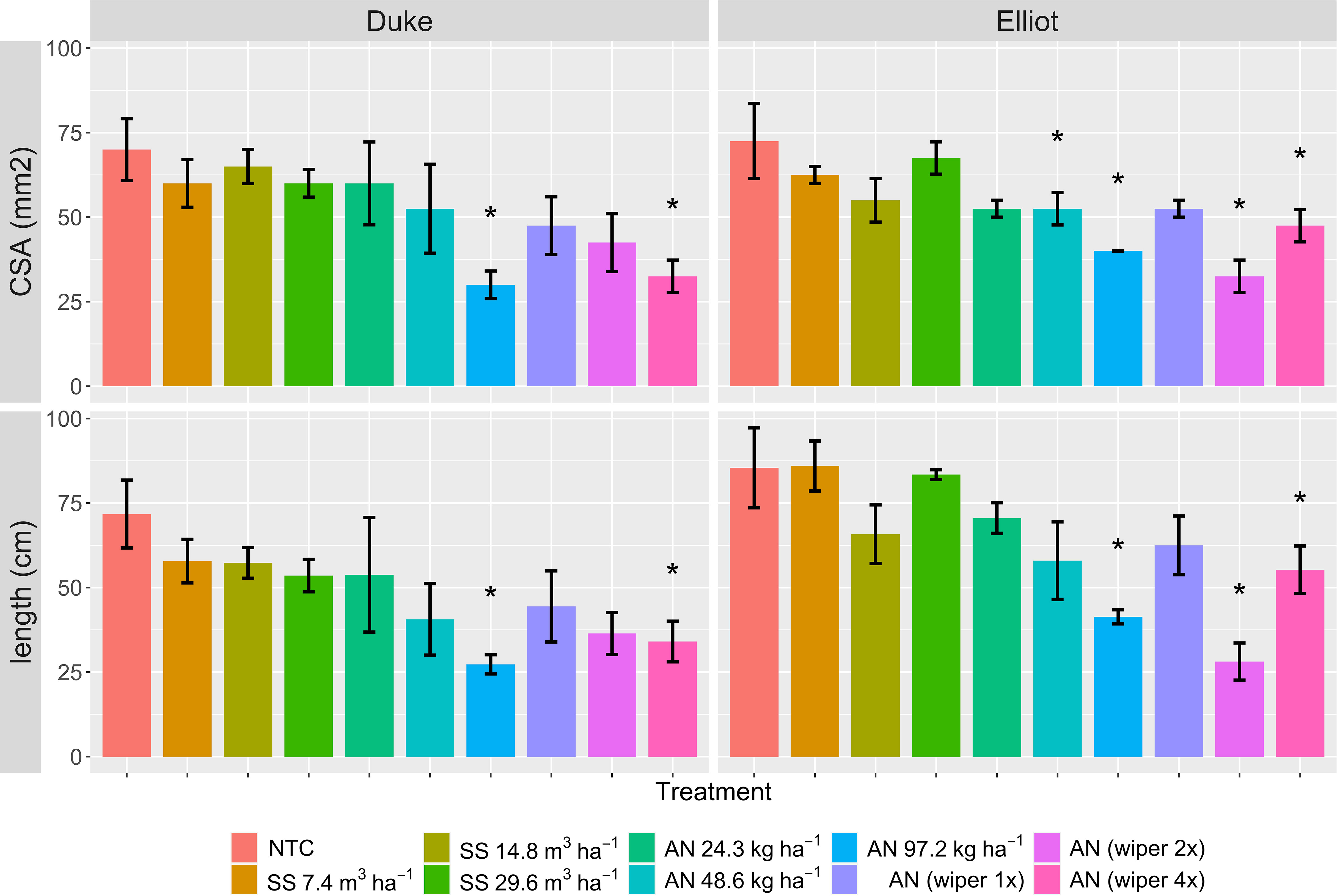
Figure 6 Highbush blueberry basal shoot cross-sectional area (CSA) (mm2) and length (cm) in response to increasing levels of saturated steam (SS) or ammonium nonanoate (AN) and nontreated control (NTC). The mature plants of cultivars ‘Duke’ and ‘Elliot’ were used in this study. The SS was applied to the lower 50 cm of the blueberry bush at 7,407 L ha−1, 14,814 L ha−1, or 29,628 L ha−1. The AN was applied as a spray or as a sponge wiper treatment. The spray treatments were delivered at 24.3 kg ai ha−1, 48.6 kg ai ha−1, or 97.2 kg ai ha−1. The wiper treatments were applied at 13% vol/vol at one, two, or four passes for each application to achieve the targeted rate. The treatments were first applied in June 2019 and reapplied 6 weeks later. The study was repeated in 2020. The means and standard errors are presented (n = 4). An asterisk (*) indicates that the means are significantly different from the non-treated controls based on the Dunnett’s test (p ≤ 0.05) within a cultivar and measurement type.
3.2.2 Efficacy of C. arvensis control
Manual removal controlled 93% of C. arvensis at 7 DAT, and was no different than SS 77% (Table 4). Both treatments performed better than AN (45%–48%) or CC spray, or with the wiper (6%). C. arvensis regrew in all treatments; however, manual removal and SS provided better control of C. arvensis with 83% and 60% control at 28 DAT, respectively. C. arvensis dry weight was lowest with SS, with 6.4 g plant−1, or 56% less biomass than the control (14.7 g plant−1). CC reduced C. arvensis biomass compared with the control, whereas AN did not affect C. arvensis biomass. The AN spray caused 47% injury to young blueberry shoots at 7 DAT, which lessened to 20% at 28 DAT. However, AN applied by the wiper caused significantly less injury, with 11% and 3% injury observed at 7 DAT and 28 DAT, respectively. The SS caused 29% injury at 7 DAT, although shoots had recovered by 28 DAT (2%). C. arvensis regrowth, likely from root buds, occurred even when the entire above-ground part of C. arvensis was treated with SS, AN, or CC, or then it was manually removed.
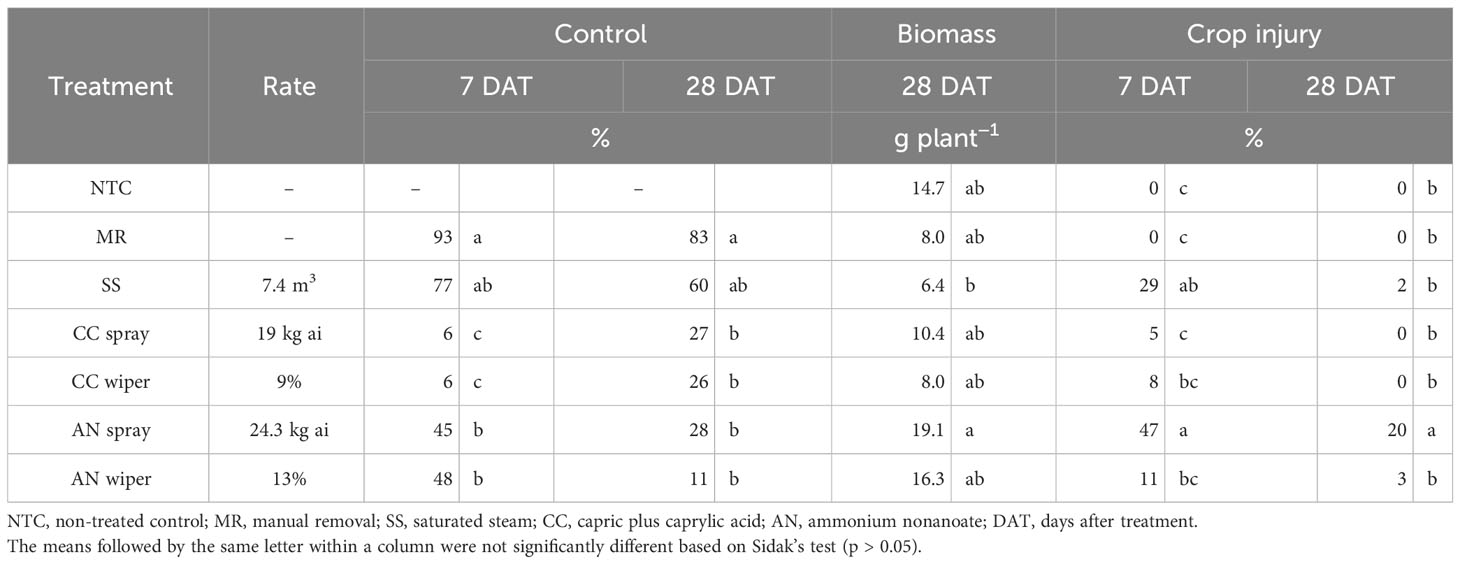
Table 4 Convolvulus arvensis control, biomass, and highbush blueberry response to saturated steam and organic herbicides applied as a spray or with a sponge wiper in a certified organic field near Independence, OR, USA in the summer of 2019.
3.3 Integrated weed control
Because a significant effect of assessment timing was observed, each assessment was analyzed separately. At 28 DAT, only the effect of factor A was present for weed control, coverage, and biomass, as treatment B was imposed subsequently (Table 5). There was a significant effect of factors A, B and their interactions for weed control in all experiments, and in experiments 1 and 3 for coverage was observed at 56 DAT. There were no interactions of factors in weed biomass. Data were analyzed by interacting factors A and B at 56 DAT for consistency.
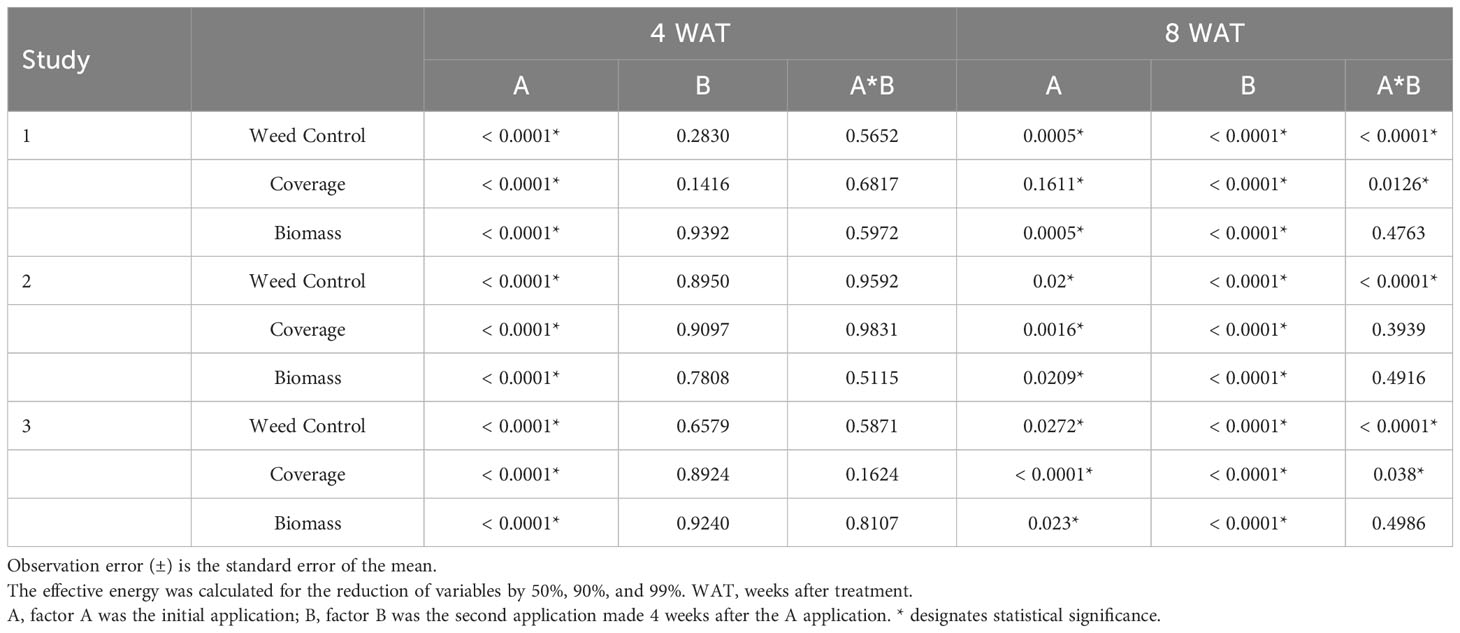
Table 5 Summary of the main effects and interactions for weed control, ground coverage, and weed biomass at three studies in commercial organic highbush blueberry fields near Independence, OR, USA, between 2018 and 2019.
The SS significantly affected weed control, coverage, and weed biomass in all experiments, but the effect varied among experiments and with evaluation timing. The primary weed species in experiment 1 were Polygonum aviculare L., K. elatine, and Sonchus oleraceus L. The average air temperature was 29°C, and no rainfall was recorded during the study. The SS treatment provided 48% weed control at 28 DAT in experiment 1, and was not different from the RB (61%) (Figure 7A). Both treatments provided better control than organic herbicides AN and CC, which provided 33% and 24% control, respectively. All treatments halved weed coverage compared with the control in experiment 1 (Figure 7B); the differences among the treatments were not significant. The SS, RB, and AN treatments reduced weed biomass significantly in experiment 1 (by 82%–93%), and CC reduced biomass by 45% compared with the control. Experiment 2 was initiated in the spring season; Epilobium ciliatum Raf., S. oleraceus, and P. aviculare were the dominant weed species. The average air temperature was 23°C and 68 mm of rainfall was recorded during the study. None of the treatments attained a high weed control in experiment 2, with SS controlling 15% of weeds at 28 DAT, while other treatments resulted in 6% to 9% control. The SS and CC provided the lowest coverage levels, while the RB and AN treatments did not differ from the non-treated control. Weed biomass reduction was evident in SS and the RB at 56 DAT in experiment 2, whereas AN and CC did not reduce biomass compared with non-treated. In experiment 3, the average air temperature was 28°C for the first month, and 22°C for the second month of the study. A total of 82 mm of rainfall was recorded in the final 15 days of the study. The RB provided the greatest level of control (62%), followed by SS (44%). AN provided 40% control and was not different from SS or CC (29%). Weed coverage ranged from 13% to 18%, compared with 26% coverage in the control. The SS reduced weed biomass by 68% in experiment 3, and the RB, AN, and CC reduced it by 38%–44%, compared with the control.
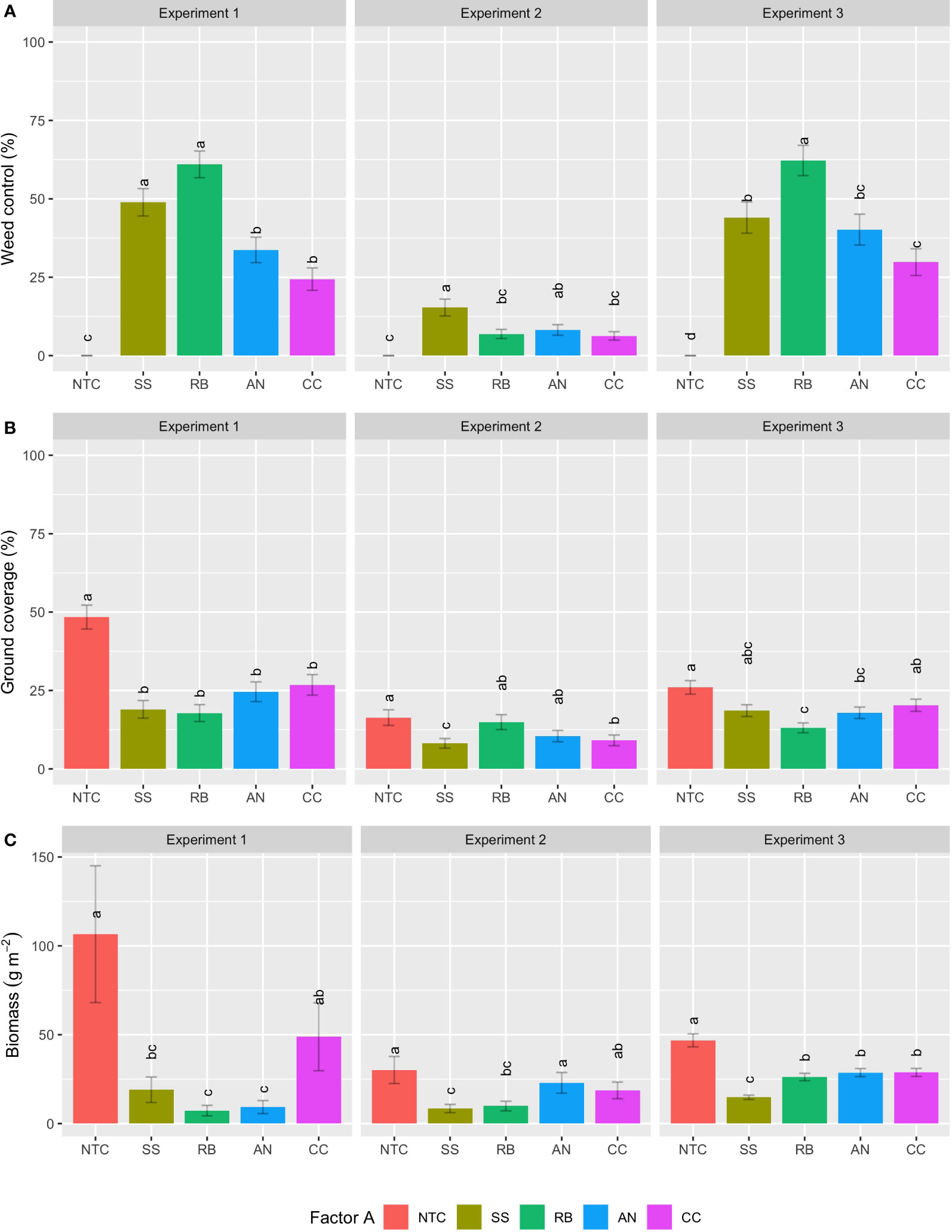
Figure 7 Weed control (A), weed coverage (B), and weed biomass (C) 4 weeks after treatment A application in three organic highbush blueberry field studies near Independence, OR, USA, in 2018 and 2019. The treatments included a non-treated control (NTC), saturated steam (SS) applied at 7,407 l ha−1, a rotary brush weeder (RB), ammonium nonanoate (AN) applied at 24.3 kg ai ha−1, and capric plus caprylic acid (CC) applied at 19.3 kg ai ha−1. The bars labeled with different letters within each study and panel are significantly different based on Sidak’s test (p ≤ 0.05).
Weed control efficacy provided by SS improved with retreatment. At 56 DAT, the SS treatments provided 81% to 93% control in experiment 1, the highest control regardless of treatment (Figure 8A). The RB provided control from 58% to 88%, with the highest control when following brush in factor A. Weed control with organic herbicides at factor B was dependent on factor A; lower control was observed in treatments that included CC in factor A. Weed coverage following treatment with SS (7%–19%) was lowest in most cases (Figure 8B), and highest following treatment with CC. The weed biomass differed significantly among treatments, with the resultant biomass following SS in factor B ranging from 0 g m−2 to 8 g m−2, following the RB ranging from 3 g m−2 to 18 g m−2, and following CC ranging from 0 g m−2 to 102 g m−2 (Figure 8C). Weed control levels were lower in experiment 2 relative to experiment 1. The SS controlled 40% to 70% of the weeds in experiment 2, and the brush weeder controlled 21% to 48%. However, the RB in factor B resulted in lower weed coverage and biomass in experiment 2. The results in experiment 3 indicate that SS and the RB provided the greatest weed control, that is, 82%–95%, and 74%–94% control, respectively. Similarly, the weed biomass was lowest in factor B following SS and RB treatments.
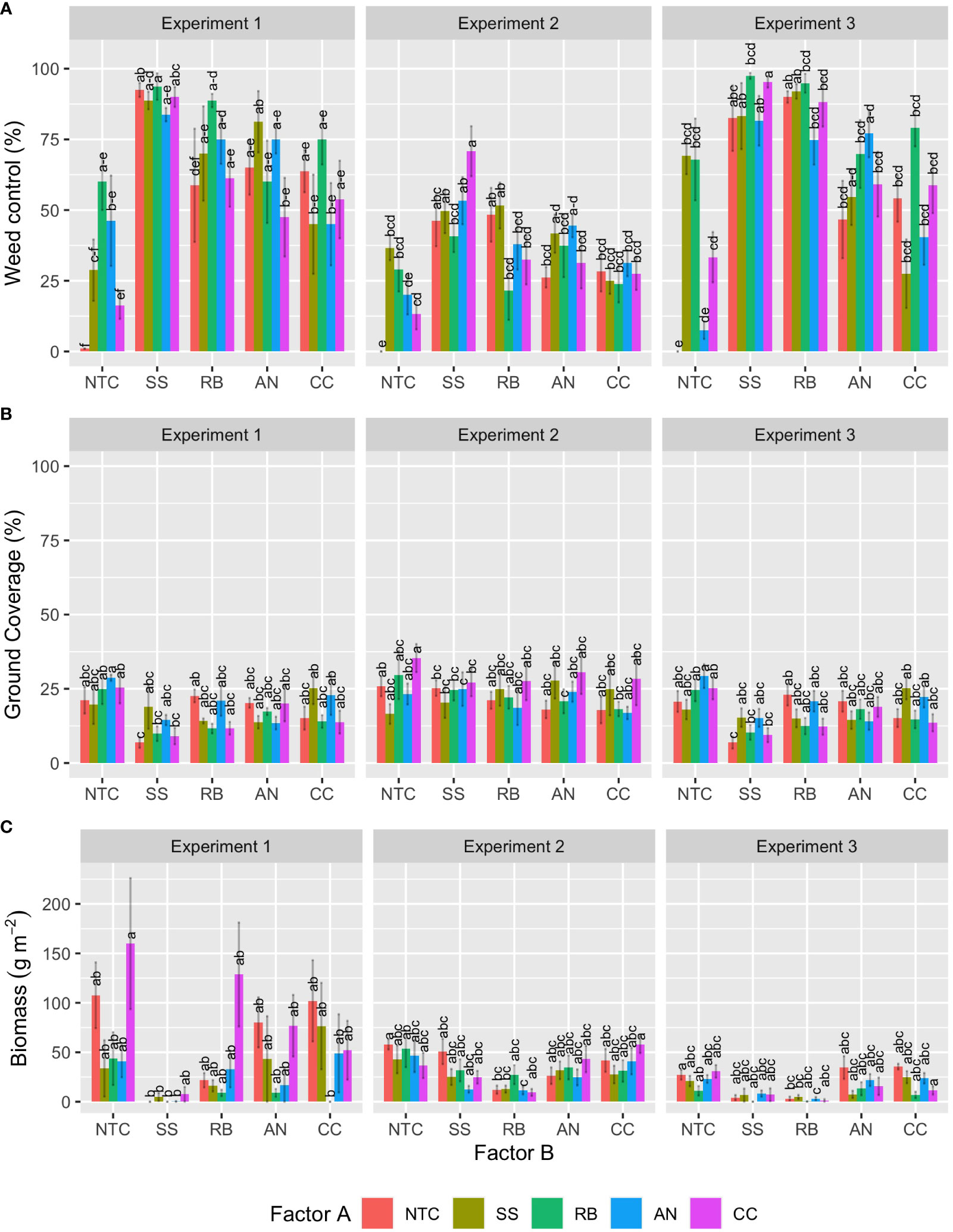
Figure 8 Weed control (A), weed coverage (B), and weed biomass (C) 8 weeks after treatment A application and 4 weeks after treatment B application in three organic highbush blueberry field studies near Independence, OR, USA, in 2018 and 2019. Factor B in the horizontal axis includes the non-treated control (NTC), saturated steam (SS) applied at 7,407 L ha−1, a rotary brush weeder (RB), ammonium nonanoate (AN) applied at 24.3 kg ai ha−1, and capric plus caprylic acid (CC) applied at 19.3 kg ai ha−1. The bars labeled with different letters within each study and panel are significantly different based on Sidak’s test (p ≤ 0.05).
The non-chemical treatments SS and the RB improved weed control by 22%, reduced weed cover by 15%, and biomass by 48.3 g m−2, compared with AN and CC on 28 DAT (Table 6). Treatments including SS also performed better than treatments without SS for weed control (+12.3%), weed coverage (−7.9%), and weed biomass (−32.4 g m−2). However, SS was not different from the RB at 28 DAT. Similar responses were observed at 56 DAT. There was no difference between treatments integrating SS and the RB compared with those only treated with SS or the RB.
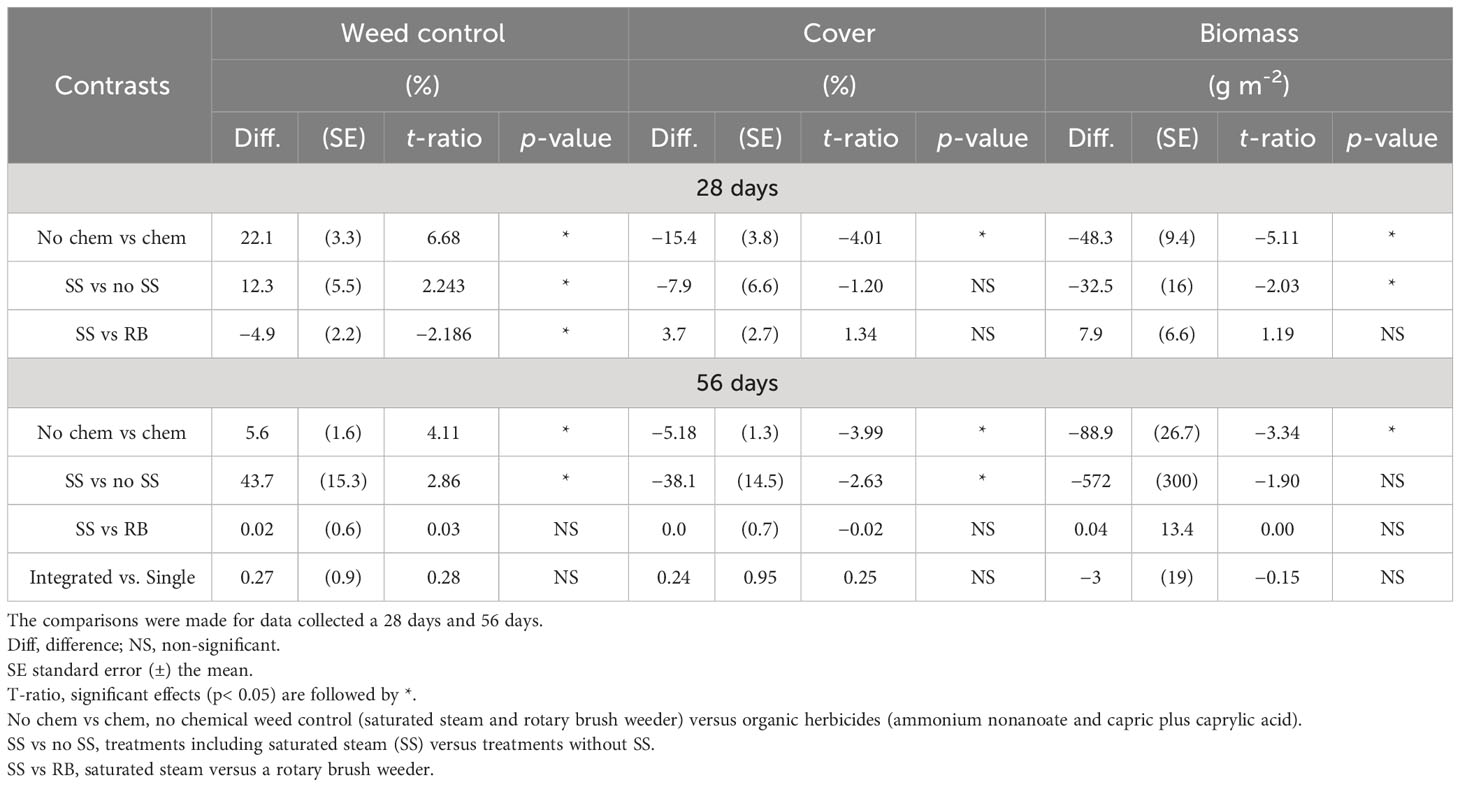
Table 6 Summary of selected contrasts to examine the effects of treatments for weed control, ground coverage, and weed biomass in a combined analysis for three studies in commercial organic highbush blueberry fields near Independence, OR, USA, between 2018 and 2019.
3.4 Partial budget analysis
The costs for treating one-fifth of the field were calculated for all treatments; the RB (US$86.61) and SS (US$135 ha−1) provided an economic advantage (Table 7). AN and CC were 2.0 to 3.5 times costlier than SS. The RB costs are likely overestimated in this study because we used the same operational capacity for SS and the RB in the calculations. However, the RB can operate at greater speeds than the SS, and operates in the field without large quantities of water, which must be transported. The RB was the least costly treatment in the study.
4 Discussion
This study evaluated how SS temperature and application volume affected weed control. SS efficacy against C. arvensis increased with boiler temperature (Figure 2). This was expected because SS energy density increases with temperature, reaching 0.512 MJ kg−1 at 121°C and 6,205 kPa (NIST, 2023). Furthermore, the magnitude of heat transfer is directly proportional to the difference in temperature, thus improving energy transfer from the SS to plant leaves. In this study, SS effects were only observed at temperatures of 65°C or greater, which is similar to the previously reported temperature (Hansson and Mattsson, 2003; de Cauwer et al., 2015). A non-linear relationship between the SS application energy (volume) and biomass reduction was confirmed, with the ED90 for dicotyledonous weeds at 3,643 MJ ha−1 (7.4 m3 ha−1) or lower, whereas the ED90 for monocotyledonous weeds was greater than 7,400 MJ ha−1 (Table 3). Although the 7.4 m3 ha−1 volume of SS required to achieve adequate weed control is high, it is significantly lower than the volume required for hot foam ranging from 87 m3 ha−1 to 133.3 m3 ha−1 (Martelloni et al., 2019; Antonopoulos et al., 2023). The greater tolerance of monocots is attributed to protected growing points at or below the soil surface making these plants less fragile (Bauer et al., 2020), and their upright leaf architecture likely reduces heat transfer efficiency (de Cauwer et al., 2015). In L. multiflorum, the SS efficacy was reduced by 8.5-fold in 25-cm-tall plants, compared with 7-cm-tall plants, based on a ED50 of 2,429 MJ ha−1 (Table 3). The L. multiflorum ED50 is lower than the value reported for L. perenne (7,500 MJ ha−1) with hot water at 98°C (de Cauwer et al., 2016). Previous studies reported that across species, smaller plants need three- to fivefold less energy than older ones (Hansson and Ascard, 2002; de Cauwer et al., 2015); a similar response is observed following flame weeding (Ascard, 1994; Ascard, 1995).
This study confirms that SS can be safely applied to blueberry plants. The SS did not affect the growth of young blueberry shoots after multiple applications at rates of up to 29.3 m3 ha−1, with damage being restricted to shoot foliage (Figures 5 and 6). The greater tolerance of shoots to SS than foliage can be attributed to reduced heat transfer due to the vertical position of the shoots, their uneven bark surface, and xylem water flux dissipating heat (Chatziefstratiou et al., 2013). Conversely, damage was observed with AN applied as a spray or sponge wiper. AN is a non-selective foliar herbicide (Dayan and Duke, 2010; Webber et al., 2010), so directed spray treatment was expected to damage young shoots. The wiper was in contact with the young shoots to simulate an application targeting C. arvensis climbing in the plant. The AN damage with sponge application damage was probably due to young shoots not having bark; AN moved through the shoot’s cuticle, much as if it moved through the leaf cuticle. The injury levels were much lower (i.e.,< 11%) when the treatments were to plants infested with C. arvensis (Table 4). Although no report is available in blueberry, non-selective herbicides with sponge wipers have damaged other crops (Harrington and Ghanizadeh, 2017; Moyo et al., 2022).
The SS at 7.4 m3 ha−1 consistently controlled weeds in organic blueberry fields, for up to 28 days. The optimum results were observed with the SS applied twice, as SS weed control was 50% or less 28 days after a single application (Figure 8), but increased up to 93% control after a second application (Figure 8). The SS improved weed control 12%–43% compared with treatments without SS (Table 6). SS efficacy was reduced in experiment 2 compared with experiments 1 and 3. This is likely a result of continuous weed emergence in response to rainfall observed during experiment 2 (spring), and not in experiments 1 and 3 (summer). The drought-stressed plants in experiments 1 and 3 may be more sensitive to SS. Previous studies documented that hot water better controlled drought-stressed plants, while air temperature had little effect on hot water efficacy (Hansson and Mattsson, 2003). The SS treatment costs were significantly lower than organic herbicides (Table 6). It is important to consider that the costs assumed that one-fifth of the field was treated and does not include the cost of transporting water and the expected reduction in operational capacity resulting from refilling the water tank. SS consumes a significant amount of water (7.4 m3 ha−1), has a low speed of operation (≤ 1.6 km h−1), and coupled with the short-lived weed control effect (< 28 days), would create a logistic challenge to ensuring prompt treatment of large areas. SS was a viable control only if used with the synthetic mulch, greatly reducing the size of the treated area. An important detail was that SS did not damage the weed mat (Moretti, personal observation). In our studies, we observed frequent adjustments and maintenance needed to maintain the operation of the steamer. Adjustments included regulating fuel pressure to control boiler temperature and steam flow, and maintenance done to the water tank, boiler, and thermostat, which were damaged primarily by vibration, dust, and moisture, respectively. The current steamer design is not adequate for commercial farm use under inclement weather conditions.
Weed control efficacy achieved by the RB was comparable to that of the SS in this study (Table 6), with the highest weed control in experiments 1 and 3 (up to 93%), and the lowest in experiment 2 (20%–50%). Similar to SS, the RB control was short-lived. The nylon brushes remove only the above-ground portion of the plants, so regrowth was observed shortly after treatment under moist conditions. The efficacy of the RB is improved with reduced operation speed; the nylon brushes provide greater rotation per unit area and deliver increased work intensity (Bond and Grundy, 2001). In this study, we operated the RB at 1.6 km h−1, to be consistent with SS and to minimize damage to the synthetic mulch. Lower speeds immediately tore the synthetic mulch. At the speed adopted, damage to the synthetic mulch only occurred where preexisting damage was present. Proper equipment positioning and an experienced tractor operator would likely eliminate this damage. In dry conditions, the RB was the most effective and least costly option (US$86.61 ha−1). However, the RB produced significant dust. The dust can disperse pathogenic microorganisms to humans and onto the fruit (Kumar et al., 2018), and can create a significant source of contamination. The RB would not be compatible in a system without synthetic mulch because the nylon brushes would damage the shallow blueberry roots and scatter the organic mulches.
The organic herbicides AN and CC did not provide consistent weed control in this study (Figures 7, 8, Table 6), and were the most expensive treatments (Table 7). The erratic performance of AN and CC observed in this study is consistent with previous studies reporting poor performance in cool-season vegetables (Johnson and Davis, 2014; Johnson and Luo, 2018) and cover crops (Lewis et al., 2020). It is unlikely that the assessment interval (28 days) contributed to the poor performance of these herbicides. Previous studies have reported 70%–75% control with pelargonic acid, a similar fatty acid herbicides, when assessed 7–14 DAT (Travlos et al., 2020). Others reported excellent control with AN, with 91% control at 33 DAT (Parkash et al., 2022). The erratic performance of fatty acid herbicides has been documented previously (Johnson and Luo, 2018), and their performance is affected by the weed species in the sites, as the efficacy of fatty acid herbicides depends on weed species, density, and developmental stage (Loddo et al., 2023). The lower efficacy and higher costs suggest that organic herbicides are not cost-effective in organic highbush blueberries at this time.
5 Conclusion
This study is the first to evaluate the use of SS for weed control in organic highbush blueberries. When SS was applied at 121°C and at 7.4 m3 ha−1 of steam, or the equivalent of 3,655 MJ ha−1, it controlled and reduced dry weights of C. arvensis by 90%. SS can be safely used when applied to the base of highbush blueberry up to 29.3 m3 ha−1. Importantly, SS is compatible with systems using synthetic mulches. Despite SS efficacy in controlling both dicotyledonous and monocotyledonous weed species at 7.4 m3 ha−1 practical challenges surfaced. Weed regrowth post treatment and the demand for copious amounts of water constrained the feasibility of employing SS in commercial blueberry production. Overcoming these challenges demands developing strategies to curtail water use and extend the duration of weed control. This is crucial for making SS a viable weed management option in commercial highbush blueberry.
Data availability statement
The raw data supporting the conclusions of this article will be made available by the authors upon request, without undue reservation.
Author contributions
MM: Conceptualization, Data curation, Formal analysis, Funding acquisition, Investigation, Methodology, Project administration, Resources, Software, Supervision, Validation, Visualization, Writing – original draft, Writing – review & editing. RP: Data curation, Formal analysis, Visualization, Writing – original draft, Writing – review & editing.
Funding
The author(s) declare financial support was received for the research, authorship, and/or publication of this article. This study was funded by the USDA Organic Transition award 2017–51106–27004, the USDA Northwest Small Fruit Research award 2072–21000–055–001-G, and the Oregon State University Agricultural Research Foundation.
Acknowledgments
The authors would like to thank the staff at Agricare Inc. in Independence, OR, USA, the OSU Lewis Brown Research Farm staff, and the Oregon State University Horticulture Weed Science research group for their assistance in establishing the studies.
Conflict of interest
The authors declare that the research was conducted in the absence of any commercial or financial relationships that could be construed as a potential conflict of interest.
Publisher’s note
All claims expressed in this article are solely those of the authors and do not necessarily represent those of their affiliated organizations, or those of the publisher, the editors and the reviewers. Any product that may be evaluated in this article, or claim that may be made by its manufacturer, is not guaranteed or endorsed by the publisher.
References
Antonopoulos N., Kanatas P., Gazoulis I., Tataridas A., Ntovakos D., Ntaoulis V. N., et al. (2023). Hot foam: Evaluation of a new, non-chemical weed control option in perennial crops. Smart Agric. Technol. 3, 100063. doi: 10.1016/j.atech.2022.100063
Ascard J. (1994). Dose-response models for flame weeding in relation to plant size and density. Weed Res. 34, 377–385. doi: 10.1111/j.1365-3180.1994.tb02007.x
Ascard J. (1995). Effects of flame weeding on weed species at different developmental stages. Weed Res. 35, 397–411. doi: 10.1111/j.1365-3180.1995.tb01636.x
Ascard J., Hatcher P., Melander B., Upadhyaya M. (2007). “Thermal weed control,” in Non-chemical weed management: principles, concepts, and technology. Eds. Upadhyaya M. K., Blackshaw. R. E. (Cambridge, MA, USA: CABI), 155.
Bauer M. V., Marx C., Bauer F. V., Flury D. M., Ripken T., Streit B. (2020). Thermal weed control technologies for conservation agriculture-a review. Weed Res. 60, 241–250. doi: 10.1111/wre.12418
Bond W., Grundy A. (2001). Non-chemical weed management in organic farming systems. Weed Res. 41, 383–405. doi: 10.1046/j.1365-3180.2001.00246.x
Burkhard N., Lynch D., Percival D., Sharifi M. (2009). Organic mulch impact on vegetation dynamics and productivity of highbush blueberry under organic production. Hortscience 44, 688–696. doi: 10.21273/Hortsci.44.3.688
Chatziefstratiou E. K., Bohrer G., Bova A. S., Subramanian R., Frasson R. P., Scherzer A., et al. (2013). Firestem2d–a two-dimensional heat transfer model for simulating tree stem injury in fires. PLoS One 8, e70110. doi: 10.1371/journal.pone.0070110
Dayan F. E., Duke S. O. (2010). Natural products for weed management in organic farming in the USA. Outlooks Pest Manage. 21, 156–160. doi: 10.1564/21aug02
de Cauwer B., Bogaert S., Claerhout S., Bulcke R., Reheul D. (2015). Efficacy and reduced fuel use for hot water weed control on pavements. Weed Res. 55, 195–205. doi: 10.1111/wre.12132
de Cauwer B., de Keyser A., Biesemans N., Claerhout S., Reheul D. (2016). Impact of wetting agents, time of day and periodic energy dosing strategy on the efficacy of hot water for weed control. Weed Res. 56, 323–334. doi: 10.1111/wre.12212
Edwards W. M. (2023) Machinery management: estimating farm machinery costs [Online] (Iowa State University, Department of Economics). Available at: https://www.extension.iastate.edu/agdm/crops/html/a3-29.html (Accessed January 10 2023).
Hansson D. (2002). “Hot water weed control on hard surface areas,” in 5th EWRS Workshop on Physicla and Cultural Weed Control. Ed. Cloutier D. C. (Pisa, Italy: European Weed Research Society), 307–316.
Hansson D., Ascard J. (2002). Influence of developmental stage and time of assessment on hot water weed control. Weed Res. 42, 307–316. doi: 10.1046/j.1365-3180.2002.00290.x
Hansson D., Mattsson J. E. (2003). Effect of air temperature, rain and drought on hot water weed control. Weed Res. 43, 245–251. doi: 10.1046/j.1365-3180.2003.00339.x
Harrington K. C., Ghanizadeh H. (2017). Herbicide application using wiper applicators- a review. Crop Prot. 102, 56–62. doi: 10.1016/j.cropro.2017.08.009
Johnson W. C. III, Davis J. W. (2014). Pelargonic acid for weed control in organic Vidalia® sweet onion production. HortTechnology 24, 696–701. doi: 10.21273/HORTTECH.24.6.696
Johnson W. C. III, Luo X. (2018). Cool-season weed control using ammonium nonanoate and cultivation in organic Vidalia® sweet onion production. Weed Technol. 32, 90–94. doi: 10.1017/wet.2017.91
Kristoffersen P., Rask A. M., Larsen S. U. (2008). Non-chemical weed control on traffic islands: a comparison of the efficacy of five weed control techniques. Weed Res. 48, 124–130. doi: 10.1111/j.1365-3180.2007.00612.x
Kumar G. D., Ravi S., Micallef S. A., Brown E. W., Macarisin D. (2018). Aeolian contamination of fruits by enteric pathogens: an unexplored paradigm. Curr. Opin. Food Sci. 19, 138–144. doi: 10.1016/j.cofs.2017.12.003
Lewis D. G., Cutulle M. A., Schmidt-Jeffris R. A., Blubaugh C. K. (2020). Better together? combining cover crop mulches, organic herbicides, and weed seed biological control in reduced-tillage systems. Environ. Entomology 49, 1327–1334. doi: 10.1093/ee/nvaa105
Loddo D., Jagarapu K. K., Strati E., Trespidi G., Nikolić N., Masin R., et al. (2023). Assessing herbicide efficacy of pelargonic acid on several weed species. Agronomy 13, 1511. doi: 10.3390/agronomy13061511
Martelloni L., Frasconi C., Sportelli M., Fontanelli M., Raffaelli M., Peruzzi A. (2019). The use of different hot foam doses for weed Control. Agronomy 9, 490. doi: 10.3390/agronomy9090490
Melander B. (1997). Optimization of the adjustment of a vertical axis rotary brush weeder for intra-row weed control in row crops. J. Agric. Eng. Res. 68, 39–50. doi: 10.1006/jaer.1997.0178
Moyo C., Harrington K. C., Kemp P. D., Eerens J. P., Ghanizadeh H. (2022). Wiper application of herbicides to Cirsium arvense. Agronomy 12, 1–11. doi: 10.3390/agronomy12102262
National Institute of Standards and Technology (NIST) (2023) Saturation Properties of Water - Temperature Increments (US Secretary of Commerce). Available at: https://webbook.nist.gov/chemistry/fluid/ (Accessed February 5, 2023).
Parkash V., Saini R., Singh M., Singh S. (2022). Comparison of the effects of ammonium nonanoate and an essential oil herbicide on weed control efficacy and water use efficiency of pumpkin. Weed Technol. 36, 64–72. doi: 10.1017/wet.2021.89
Patrignani A., Ochsner T. E. (2015). Canopeo: A powerful new tool for measuring fractional green canopy cover. Agron. J. 107, 2312–2320. doi: 10.2134/agronj15.0150
R Core Team (2023). “R: a language and environment for statistical computing,” in R Foundation for Statistical Computing, 4.3.0 ed(Vienna, Austria). Available at: https://ropensci.org/blog/2021/11/16/how-to-cite-r-and-r-packages/.
Ritz C., Baty F., Streibig J. C., Gerhard D. (2015). Dose-response analysis using R. PloS One 10, e0146021. doi: 10.1371/journal.pone.0146021
Strik B. C. (2016). A review of optimal systems for organic production of blueberry and blackberry for fresh and processed markets in the northwestern United States. Scientia Hortic. 208, 92–103. doi: 10.1016/j.scienta.2015.11.044
Strik B., Buller G., Hellman E. (2003). Pruning severity affects yield, berry weight, and hand harvest efficiency of highbush blueberry. HortScience 38, 196–199. doi: 10.21273/Hortsci.38.2.196
Strik B. C., Buller G., Tarara J. M. (2014). Grow tubes reduce root and crown growth but not early production during establishment of highbush blueberry. HortScience 49, 581–588. doi: 10.21273/HORTSCI.49.5.581
Strik B. C., Davis A. J. (2021). Individual and combined use of sawdust and weed mat mulch in a new planting of northern highbush blueberry. III. Yield, fruit quality, and costs. HortScience 56, 363–367. doi: 10.21273/Hortsci15659-20
Strik B., Vance A. (2016). “Weed management strategies in long-term organic blueberry production systems-impact of mulch type and weed control methods on economics,” in XI International Vaccinium Symposium. Ed. Olmstead J. W. (Acta Horticulturae), 347–354. doi: 10.17660/ActaHortic.2017.1180.47
Travlos I., Rapti E., Gazoulis I., Kanatas P., Tataridas A., Kakabouki I., et al. (2020). The herbicidal potential of different pelargonic acid products and essential oils against several important weed species. Agronomy 10, 1687. doi: 10.3390/agronomy10111687
United States Department of Agriculture (USDA) (2023) National Agricultural Statistics Service (NASS) Agricultural Chemical Use. Available at: https://www.nass.usda.gov/ (Accessed September 5 2023).
Valenzuela-Estrada L. R., Vera-Caraballo V., Ruth L. E., Eissenstat D. M. (2008). Root anatomy, morphology, and longevity among root orders in Vaccinium corymbosum (Ericaceae). Am. J. Bot. 95, 1506–1514. doi: 10.3732/ajb.0800092
Keywords: non-chemical, Vaccinium corymbosum L., Convolvulus arvensis L., no-till, organic herbicides, mechanical weed control, synthetic mulch, thermal weed control
Citation: Moretti ML and Pedroso RM (2023) Weed control with saturated steam in organic highbush blueberry. Front. Agron. 5:1297979. doi: 10.3389/fagro.2023.1297979
Received: 20 September 2023; Accepted: 22 November 2023;
Published: 11 December 2023.
Edited by:
Rick Llewellyn, Commonwealth Scientific and Industrial Research Organisation (CSIRO), AustraliaReviewed by:
Stephen Christopher Marble, University of Florida, United StatesIoannis Gazoulis, Agricultural University of Athens, Greece
Copyright © 2023 Moretti and Pedroso. This is an open-access article distributed under the terms of the Creative Commons Attribution License (CC BY). The use, distribution or reproduction in other forums is permitted, provided the original author(s) and the copyright owner(s) are credited and that the original publication in this journal is cited, in accordance with accepted academic practice. No use, distribution or reproduction is permitted which does not comply with these terms.
*Correspondence: Marcelo L. Moretti, marcelo.moretti@oregonstate.edu