Plant protection consequences in Hungary of the withdrawal of systemic active substances from sugar beet seed treatment
- Department of Agronomy, Institute of Agronomy, Hungarian University of Agriculture and Life Sciences, Kaposvár Campus, Kaposvár, Hungary
After decades of development, neonicotinoids were widely used in Hungarian sugar beet production and seeds included thiamethoxam treatment by 2019 increasing protection against early spring pests. In Hungary, neonicotides’ influence on bee health led to their removal of emergency authorization. In the absence of thiamethoxam in 2020, experience showed that multiple treatments were needed to protect beet from pests early spring pests. Repeated applications of mostly pyrethroids have proved suitable; however, in sugar beet, this has resulted in a multiple increase in environmental stress and honey bee mortality, as well as increased input values. The aim of this work is to explore the current state of production and collect new alternative options for applying effectively in Hungarian sugar beet production technology, against early spring pests.
1 Introduction
Prior to the introduction of neonicotinoids in the early 1990s, insecticidal seed treatment in sugar beet was often supplemented with granules or sprays applied at sowing and insect pest control sprays during the growing season (Elbert et al., 2008). This was done using various organophosphates, carbamates or pyrethroids that were replaced by neonicotinoid seed treatment in recent decades and due to their high efficacy in controlling a wide range of pests and maintaining high yield stability, neonicotinoids became generally used on sugar beet seeds in most European countries (International Confederation of European Beet Growers, 2018). Since neonicotinoids provided adequate protection, in most cases, no further insecticide applications were necessary (Hauer et al., 2017; Viric Gasparic et al., 2021).
Alternatives to neonicotinoids are less studied, for reasons that include the delayed ban on non-flowering crops and the efficacy of thiamethoxam on the main pests. Research has focused on other crops such as rapeseed and maize (Scott and Bilsborrow, 2019). Neonicotinoids are hardly substitute against the most harmful pests, as there are no effective alternatives (Hauer et al., 2017). Thiamethoxam emergency authorization has been granted for sugar beet seeds in Hungary for the last 3 years, but this will not be implemented next year (National Food Chain Safety Office, 2023).
Considering the current situation, pest control is solved by in-crop treatment (Jactel et al., 2019), posing additional environmental impact. The aim of this work is to explore current options for the control of sugar beet pests, taking into account the impact on the environment and pollinators, and to collect new alternatives that may be used in sugar beet production technology against the early spring pests.
2 Intensity of sugar beet production in Hungary
Sugar beet played a vital role in Hungarian crop production until the end of the 20th century (Radics, 2012). As a result of the 2006 reform of the European sugar regime, four of the five sugar factories operating in Hungary closed down (Borbély, 2007) and the cultivation area reduced from 100,000 hectares to around 10,000 hectares in the last decade (Hungarian Central Statistical Office, 2022).
Hungarian sugar beet production is currently concentrated mainly in the region of the only sugar factory in operation (Figure 1). Sugar beet requires one of the most intensive technologies among crops grown in Hungary (Radics, 2012), and the withdrawal of pesticide active substances indicate complications of early pest control (Viric Gasparic et al., 2021).
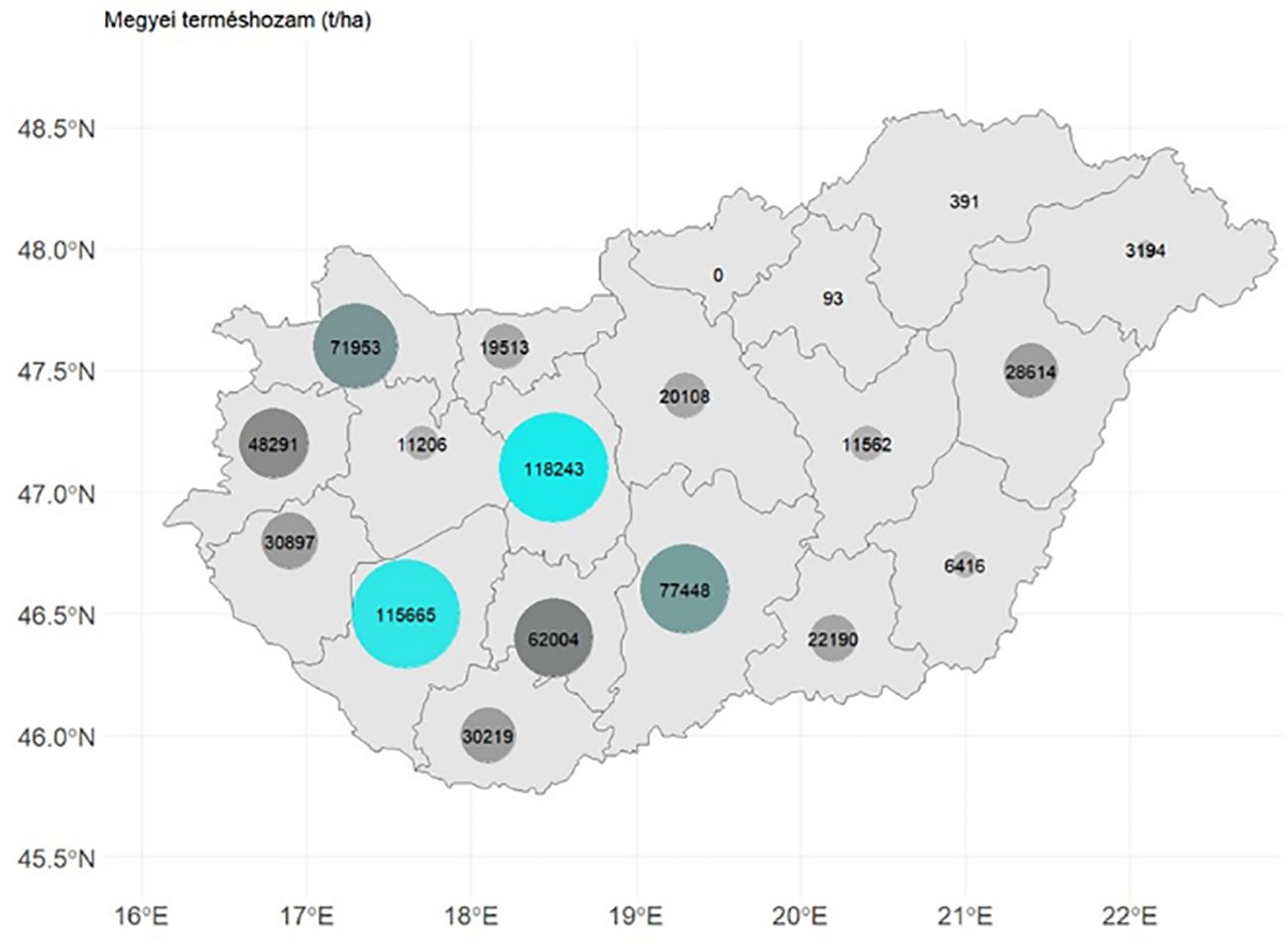
Figure 1 Sugar beet overall yield/county (t) in Hungary, in 2021. Based on Hungarian Central Statistical Office (2022).
3 Soil-dwelling and juvenile sugar beet arthropod pests
The most important early spring pests in sugar beet cultivation are the follows:
Scarabaeidae larvae, cause damage by chewing roots, destroying the root surface, manifesting in early wilting symptoms (Lange, 1987). Tenebrionidae – such as Opatrum sabulosum L. and Omophlus proteus Kirsch – larvae, cause damage by chewing the underground organs, may result in wilting and subsequent death especially in drought (Sigareva et al., 2019). Elateridae larvae cause the death of young plants by masticating their immediate underground parts, such as Agriotes sputator L., Agriotes lineatus L., Agriotes obscurus L. and Agriotes ustulatus Schaller (Furlan et al., 2021). Asproparthenis punctiventris Germar, Chromoderus fasciatus Müller Asproparthenis affinis Schrank, Otiorhychus ligustici L. and Psallidium maxillosum Fabricius pose a significant threat to sugar beet production both as larvae and adults. Larvae feed on underground tissues, while imagoes masticate the stigma or first leaflets, reducing leaf surface area, or consuming the apical meristem. A. punctiventris and C. fasciatus are species that complete their life cycle within a year and exist in the adult form during the winter (Lemic et al., 2016). Conversely, P. maxillosum undergoes a larval stage in its first year and maturing into an adult in its second year, as well as Tanymecus palliatus Fabricius and Tanymecus dilaticollis Gyllenhal, which mostly inflicts substantial harm on monocots, however it also induces damage to juvenile sugar beet in spring (Kereši et al., 2006). T. palliatus is also dangerous for juvenile sugar beet Tanymecus species, due to its dicotyledonous host plant preference (Keszthelyi et al., 2008).
Chaetocnema tibialis Illiger is the most widespread pest in Hungary, but it causes complete eradication less frequently than the A. punctiventris. They masticate the leaves during the juvenile period and the adults peel in the leaf surface. The imagos overwinter and leave their wintering places at average temperatures of 8–10°C and then seek out beet leaves (Kereši et al., 2006).
Aphis fabae Scopoli exhibit puncture wounds on the phloem side with their piercing-sucking mouthparts (Hurej and Werf, 1993). In Hungary, their population can undergo up to 14 generations, leading to potential harm from March to September. Beet necrotic yellow vein virus (BNYVV) is a notable viral disease that affects sugar beet. Its primary carriers are aphids. The National Association of Sugar Beet Growers (CTOSZ) has proactively advocated for the use of thiamethoxam as an emergency treatment for beet seeds in the past 3 years driven by the necessity to effectively defend against vectors (National Association of Sugar Beet Growers, 2020; Keszthelyi et al., 2022).
The period of damage by soil-dwelling and juvenile sugar beet arthropod pests is shown in Figure 2.
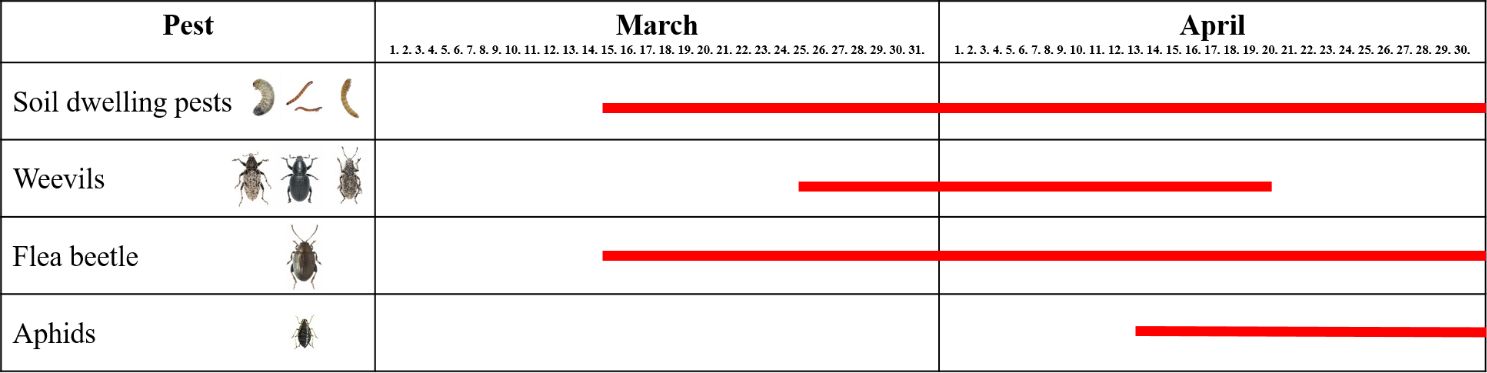
Figure 2 Soil-dwelling and juvenile sugar beet arthropod pests damage periods in Hungary. Based on Keszthelyi et al. (2022).
4 The effectiveness of neonicotinoid seed treatment in sugar beet production in the past decades
Neonicotinoids are a group of modern pesticides that are used to control various pest species in sugar beet production and were originally used as alternatives to halogenated hydrocarbons because of their systemic properties, which allow them to be absorbed and translocated in plants and were found to be highly effective (Bass and Field, 2018).
Neonicotinoids are substances that play an agonistic role at nicotinic acetylcholine receptors (nAChR), thereby opening ion channels (Casida and Durkin, 2013). In addition, their insecticidal action is also partially manifested in voltage-gated calcium channels (Jepson et al., 2006). Differences between arthropod and mammalian nAChRs, particularly in the properties and structure of the subunits, may explain the high selectivity of neonicotinoids for arthropods and their presumed relatively low toxicity in mammals (Tomizawa and Casida, 2003, 2005). Insecticide spraying was only necessary in cases, where A. punctiventris pressure was high, so neonicotinoid seed treatment contributed to considerable reduction in the amount of insecticides (Bažok et al., 2012; Potyondi et al., 2014; Viric Gasparic et al., 2021). This was further enhanced with the emergence of neonicotinoid-treated seeds, such as banding, film coating, pelleting or multi-layer coating, are effective in protecting young plants from insect damage (Altmann, 1991; Meredith and Morris, 2003; Elbert and Nauen, 2004). Neonicotinoids have been widely used for seed treatment from the 1990s in including sugar beet (Viric Gasparic et al., 2021). Neonicotinoids have a significant advantage over previous soil-based insecticides because they have a wide range of target organisms and are effective for long periods against early spring pests (Figure 3). This method replaced the older, high-dose, full-area or broadcast applications. As a result, the environmental impact has been reduced (Altmann, 1991).
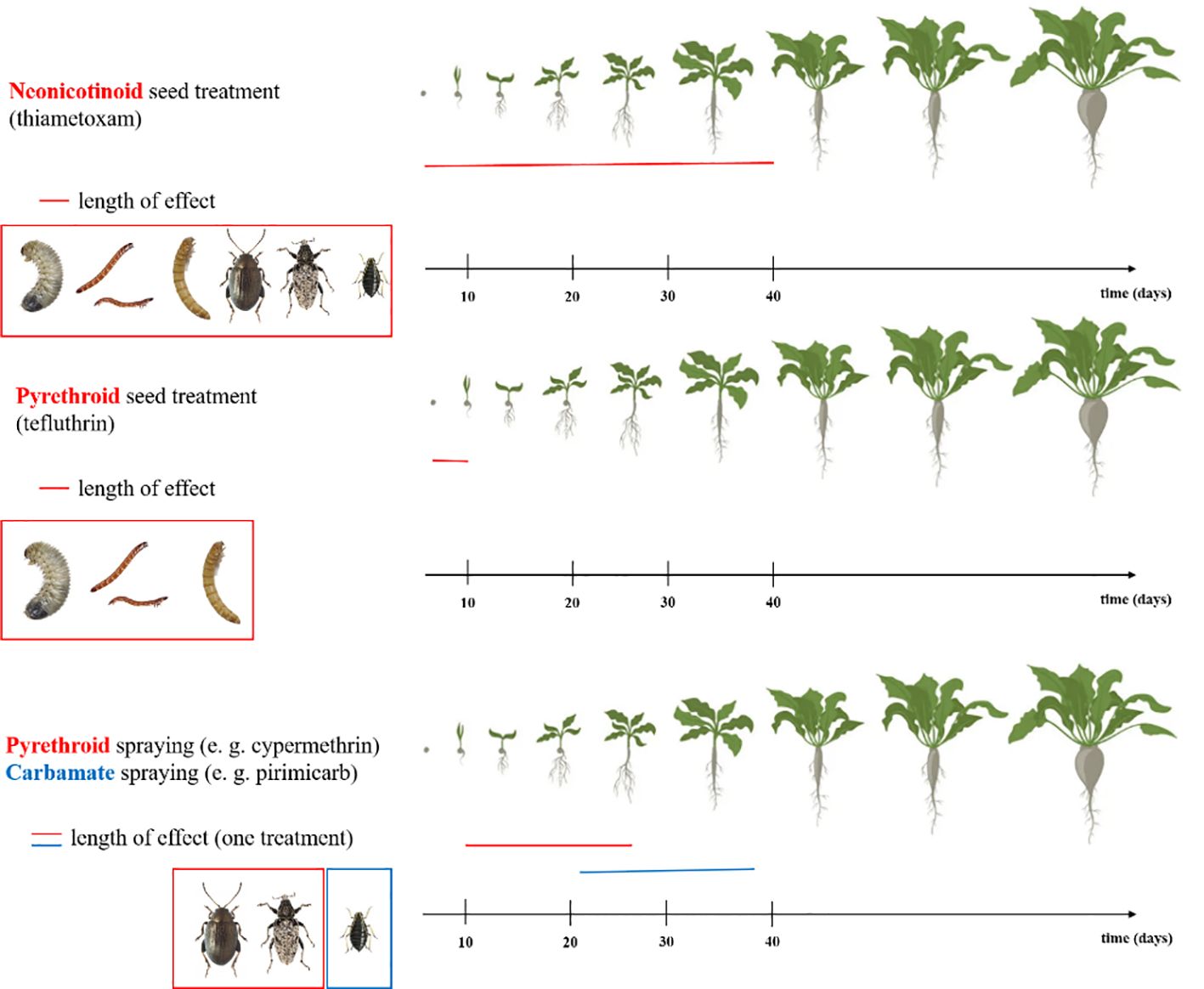
Figure 3 Duration of action of active substances thiametoxam (Syngenta Seedcare, 2023a), tefluthrin (Syngenta Seedcare, 2023b), cypermethrin (World Health Organization et al., 1989) and pirimicarb (International Programme on Chemical Safety and World Health Organization, 1986) used in sugar beet crop protection.
However, a growing number of studies have described that neonicotinoids may have adverse effects not only on the target species but also on others, in particular pollinators, such as bees (Godfray et al., 2014; Woodcock et al., 2016, 2017). As a consequence, concerns about neonicotinoids have increased significantly and public awareness of these issues has also increased.
5 Effects on pollinators and the environment
Neonicotinoids have potential impact on non-target organisms, including pollinators, such as bees (Lundin et al., 2015) as they accumulate in the pollen of treated plants and have toxic effects on pollinators, consequently classified by the Environmental Protection Agency (EPA) as II and III class toxicity agents (Buszewski et al., 2019). However, this classification does not apply to sugar beet in relation to bee populations, since sugar beet is a biannual crop, however it is harvested in the autumn of the first year, before flowering. It is important to mention that most of the studies (Krupke et al., 2012; Botías et al., 2017) have focused on flowering plants, as bees can readily gather pollen from them.
Regarding neonicotinoids or other active substances employed in sugar beet production, the insecticidal treatment affects pollinators indirectly through the accumulation in soil and through plant residues. Moreover, the soil type is significant for the leaching dynamics in the residual section. For example, concerning the elution profiles, neonicotinoids exhibit high mobility and are prone to leaching in sandy soil. However, their mobility is only moderate in loam soil, as demonstrated by Mörtl et al. (2016).
Similarly to neonicotinoids, questions have been raised about the environmental impact of pyrethroids, particularly on pollinators. Pyrethroids are derived from pyrethrins, which are naturally occurring component of chrysanthemum (Palmquist et al., 2012). They disrupt the regular functioning of the nervous system of insects by altering of voltage-sensitive sodium channels, which results in the cessation of their normal activity (Soderlund, 2010). A three-year investigation of pyrethroid residues in China revealed contamination of nectar-producing plants due to pyrethroid insecticides (He et al., 2023) that is, as it was the case with neonicotinoids, does not directly affect pollinators in sugar beet production.
Therefore, neither neonicotinoids, nor pyrethroids pose a direct risk to bees, it rather affects the next years’ annual plant cultures (Krupke et al., 2012) via the uptake of their residues from soil (Schaafsma et al., 2015). Moreover, the methodology of investigation may also need consideration. In a systematic review conducted by Lundin et al. (2015), it was proposed that in addition to laboratory experiments using high dosages, which were commonly used to study the effects of neonicotinoids on honey bees (Apis mellifera L.), future research should focus on field studies that involve realistic exposure to neonicotinoids.
6 Impact of thiamethoxam withdrawal, current state of crop protection
In 2018, the European Union Commission banned the use of neonicotinoid insecticides imidacloprid, clothianidin and thiamethoxam in arable crops, because of their potential adverse effects on honey bee populations. However, this decision was taken with some exceptions, such as allowing the use of these active substances in permanent greenhouses (Official Journal of the European Union, 2018a, 2018b, 2018c). As a result, arable crops, including sugar beet, remained unprotected against pests, while the pressure of harmful organisms has continued to increase (Viric Gasparic et al., 2021). Nevertheless, the authorizing authority rejected the emergency use authorization for the insecticide Cruiser SB (600 g/L thiamethoxam) for the treatment of sugar beet seed as well as in 2020 initiated by the National Association of Sugar Beet Growers (2020). On the basis of the judgment of the European Court of Justice of 19 January 2023 in Case C-162/21, it is unlawful to grant an emergency authorization for the marketing of seed treatment products and for the marketing and use of seeds treated with those products where the marketing and use of seeds treated with those products is expressly prohibited by an implementing regulation (InfoCuria, 2023). Accordingly, alternative solutions for sugar beet protection should be explored in the next season in Hungary.
7 Pending and currently marketed systemic active substances
However, due to the withdrawal of neonicotinoid, seed treatment is currently achieved with contact pyrethroids, which do not always provide sufficient protection against severe pest presence, and therefore the use of soil disinfection as an additional treatment against juvenile pests of sugar beet becomes justified. This is also carried out with pyrethroid active substances, which may pose a higher insecticide pressure on the environment compared to previous practice. Considering this gap in knowledge, the study of Romanowski and Blake in 2023 looked into whether this was due to an absence of research, or whether alternatives to neonicotinoids are ineffective in this system (Romanowski and Blake, 2023). Therefore, it would be worthwhile to consider the possible inclusion of other new insecticides with systemic action in the seed treatment of sugar beet protection technology.
Alternatives to neonicotinoids in the sugar beet system have been less studied. Reasons for this are the delayed ban on non-flowering crops and the efficacy of thiamethoxam in this pest system. Much of the focus has been on flowering systems such as rape, where the negative consequences of the withdrawal were considered, and the potential alternatives became permitted as seed treatment (Scott and Bilsborrow, 2019). A 2017 study compared the use of thiamethoxam and alternative strategies on sugar beet as a preventive measure prior to the expected ban (Hauer et al., 2017).
However, biological control involved in Integrated Pest Management (IPM), have not been considered for pests of sugar beet (Romanowski and Blake, 2023), only aphids have been studied recently. Laurent tested non-neonicotinoid-based treatments against sugar beet aphids, and while some other insecticides were effective, biopesticides (non-chemical pesticides derived from natural products) were much less effective (Laurent et al., 2023). Biological control strategies for aphids and related virus transmission were investigated in a 2022 study. It concluded that the use of semiochemical agents, entomopathogenic fungi (EPF) and Plant Growth Promoting Rhizobacteria (PGPRs) in combination with resistant beet varieties is the most promising, and that this requires synergies to be created from experimental data provided by different partners (national research centers, universities, private companies and associations in the sugar beet industry) (Francis et al., 2022). According to one of the first studies to evaluate the use of virus prediction in this system based on public opinion, when viable alternatives to thiamethoxam seed treatment were evaluated, alternative pest management strategies were limited, particularly systems against sugar beet aphid (Aphis fabae L.), such as biological control and crop rotation, have proven to be weak responses to the threat of virus yellows (Romanowski and Blake, 2023). In a study by Furlan and Kreutzweiser (2015), they concluded that IPM has potential, but it requires time and investment both in research and public outreach. Jactel et al. (2019) studied alternatives to neonicotinoids against soil-dwelling Agriotes species and found that the most commonly used, highly effective alternative to neonicotinoids were pyrethroids; however, because of their mode of action and increased application occasions and environmental impact, this group may not provide an effective plant protection solution to replace neonicotinoids (Furlan et al., 2018), therefore alternative solutions would worth considering to include as seed treatment. Additional risk of the unilateral utilization of this group of insecticides can trigger further serious phytosanitary concern. The superior translocation of this active ingredient makes it well suited for seed treatment and there is less risk of cross-resistance, because of the different mode of action (Zeng et al., 2021), furthermore this substance is already in use in rape (Brassica napus L.) (Scott and Bilsborrow, 2019).
Diamides (IRAC MoA: 28) are one of the most advanced insecticides with specific target site activity that may be considered as an alternative to neonicotinoids. They are compounds with a broad pest spectrum and a systemic as well as a broad toxicological profile. Diamides activate the insect’s ryanodine receptors (RyRy), which results in uncontrolled calcium release and inhibits muscle contraction (paralysis). This renders the insect immobile, unable to feed (Selby et al., 2013). Among them, cyantranilipole besides basipetal and translaminar translocation, it also translocates acropetally, and therefore successfully used in arable crops (oilseed rape, maize, soybean, rice) compared to other diamides. It is effective against several species of both chewing and sucking pests in plant cultivation (Mandal, 2012; Rath and Nayak, 2013; Keszthelyi et al., 2023). The currently developed diamides, as cyclaniliprole and tetraniliprole are systemic active substances have been available since 2015, but currently not yet permitted for sugar beet in Hungary (Keszthelyi et al., 2023).
Flupyradifurone, butenolide (IRAc MoA: 4D) derivatives have the similar mode of action as neonicotinoids and a related chemical structure. Butenolides are typical neurotoxins that induce paralysis by continuous stimulation of the nerve endings following muscle overactivity, especially in sucking pests (Shuai et al., 2022). It is characterized by systemic, good translaminar plant tissue spread (Nauen et al., 2015). However, some degree of cross-resistance has been shown for this active substance in Bemisia tabaci (Smith et al., 2016; Wang et al., 2020).
Spirotetramat, tetramic acid (IRAC MoA: 23) derivative, has a wide range of uses due to its bidirectional (basipetal and acropetal), systemic translocation, with fatty acid biosynthesis inhibition properties. It has favorable environmental and ecotoxicological properties but is also not yet available (Nauen et al., 2008).
Triflumuron (IRAC MoA: 15) is also a systemic compound and plays an important role in IPM programs. It acts by blocking chitin synthase of developing insect and it is effective against chewing mouth-breathing insects, also it has an egg-destroying effect (Belinato et al., 2013).
8 Conclusions
Neonicotinoids were widely used in sugar beet technology by 2010, and all seed treatments by 2019 that increased protection against early spring sugar beet pests, eliminating one of the most costly and serious beet production challenge. However, in Hungary, due to the environmental influence of neonicotides, especially on honey bee health, led to the removal from emergency authorization. Experience has showed that in order to overcome the less effective applications, additional plant protection steps are needed to be included into the technology.
Therefore, future study into pest management alternatives to neonicotinoids seems to be inevitable. Cyantraniliprole, which has not been used in sugar beet, may be a good alternative, since current crop treatment methods pose a higher overall environmental impact than neonicotinoids, also the application of systemic substances in sugar beet production has less direct environmental impact compared to other flowering crops and pose less risk of resistance and cross-resistance. This would certainly be of great impact on solving the plant protection problems of sugar beet production.
Author contributions
IJ: Conceptualization, Visualization, Writing – original draft, Writing – review & editing, Supervision. FC: Conceptualization, Writing – original draft, Writing – review & editing, Methodology, Resources, Visualization. KS-T: Conceptualization, Writing – original draft, Writing – review & editing, Visualization. FP-F: Conceptualization, Writing – original draft, Writing – review & editing. SK: Conceptualization, Supervision, Writing – original draft, Writing – review & editing.
Funding
The author(s) declare that no financial support was received for the research, authorship, and/or publication of this article.
Conflict of interest
The authors declare that the research was conducted in the absence of any commercial or financial relationships that could be construed as a potential conflict of interest.
Publisher’s note
All claims expressed in this article are solely those of the authors and do not necessarily represent those of their affiliated organizations, or those of the publisher, the editors and the reviewers. Any product that may be evaluated in this article, or claim that may be made by its manufacturer, is not guaranteed or endorsed by the publisher.
References
Altmann R. G. (1991). Ein neues Insektizid zur Bekampfung “von Rubensch” adlingen. Pflanzenschutz-Nachrichten Bayer (German edition) 44, 159–174.
Bass C., Field L. M. (2018). Neonicotinoids. Curr. Biol. 28, 772–773. doi: 10.1016/j.cub.2018.05.061
Bažok R., Buketa M., Lopatko D., Ljikar K. (2012). Suzbijanje štetnika še´cerne repe nekad i danas. Glas. Biljn. Zašt. 12, 414–428. doi: 10.17221/86/2015-PPS
Belinato T. A., Martins A. J., Lima J. B. P., Valle D. (2013). Effect of triflumuron, a chitin synthesis inhibitor, on Aedes aEgypti, Aedes albopictus and Culex quinquefasciatus under laboratory conditions. Parasites Vectors 6, 1–7. doi: 10.1186/1756-3305-6-83
Borbély Á. (2007). Az Európai Unió cukoripari reformjának eloszlási és strukturális hatásai. Ph.D. dissertation. Corvinus University of Budapest, Doctoral School of Business and Management.
Botías C., David A., Hill E. M., Goulson D. (2017). Quantifying exposure of wild bumblebees to mixtures of agrochemicals in agricultural and urban landscapes. Environ. pollut. 222, 73–82. doi: 10.1016/j.envpol.2017.01.001
Buszewski B., Bukowska M., Ligor M., Staneczko-Baranowska I. (2019). A holistic study of neonicotinoids neuroactive insecticides—properties, applications, occurrence, and analysis. Environ. Sci. pollut. Res. 26, 34723–34740. doi: 10.1007/s11356-019-06114-w
Casida J. E., Durkin K. A. (2013). Neuroactive insecticides: targets, selectivity, resistance, and secondary effects. Annu. Rev. Entomol. 58, 99–117. doi: 10.1146/annurev-ento-120811-153645
Elbert A., Haas M., Springer B., Thielert W., Nauen R. (2008). Applied aspects of neonicotinoid uses in crop protection. Pest Manage. Sci. 64, 1099–1105. doi: 10.1002/ps.1616
Elbert A., Nauen R. (2004). “New applications for neonicotinoid insecticides using imidacloprid as an example,” in Insect Pest Management, Field and Protected Crops. Eds. Horowitz A. R., Ishaaya I. (Springer, Berlin–Heidelberg–New York), 29–44.
Francis F., Then C., Francis A., Gbangbo Y. A. C., Iannello L., Ben Fekih I. (2022). Complementary strategies for biological control of aphids and related virus transmission in sugar beet to replace neonicotinoids. Agriculture 12, 1663. doi: 10.3390/agriculture12101663
Furlan L., Kreutzweiser D. (2015). Alternatives to neonicotinoid insecticides for pest control: case studies in agriculture and forestry. Environ. Sci. Pollut. Res. 22 (1), 145–147.
Furlan L., Contiero B., Tóth M. (2021). Assessment of the attraction range of sex pheromone traps to Agriotes (Coleoptera, Elateridae) male click beetles in South-Eastern Europe. Insects 12, 733. doi: 10.3390/insects12080733
Furlan L., Pozzebon A., Duso C., Simon-Delso N., Sánchez-Bayo F., Marchand P. A., et al. (2018). An update of the worldwide integrated assessment (WIA) on systemic insecticides. Part 3: alternatives to systemic insecticides. Environ. Sci. pollut. Res. 28, 1–23. doi: 10.1007/s11356-017-1052-5
Godfray H. C. J., Blacquiere T., Field L. M., Hails R. S., Petrokofsky G., Potts S. G., et al. (2014). A restatement of the natural science evidence base concerning neonicotinoid insecticides and insect pollinators. Proc. R. Soc. B P Roy Soc. B-Biol Sci. 281, 20140558. doi: 10.1098/rspb.2014.0558
Hauer M., Hansen A. L., Manderyck B., Olsson Å., Raaijmakers E., Hanse B., et al. (2017). Neonicotinoids in sugar beet cultivation in Central and Northern Europe: Efficacy and environmental impact of neonicotinoid seed treatments and alternative measures. Crop Prot. 1, 132–142. doi: 10.1016/j.cropro.2016.11.034
He Q., Zhang S., Yin F., Liu Q., Gao Q., Xiao J., et al. (2023). Risk assessment of honeybee larvae exposure to pyrethroid insecticides in beebread and honey. Ecotoxicol. Environ. Saf. 267, 115591. doi: 10.1016/j.ecoenv.2023.115591
Hungarian Central Statistical Office. (2022). Available online at: https://www.ksh.hu/stadat_files/mez/hu/mez0081.html?fbclid=IwAR0cMOEA2j780_M-qdLrOZXDoPAXkVFJmQWRumEErCtxLEkeciNlLFpQ43s.
Hurej M., Werf W. V. D. (1993). The influence of black bean aphid, Aphis fabae Scop., and its honeydew on leaf growth and dry matter production of sugar beet. Ann. Appl. Biol. 122, 201–214. doi: 10.1111/j.1744-7348.1993.tb04027.x
InfoCuria. (2023). Judgment - 19/01/2023 - Pesticide Action Network Europe and Others Case C-162/21. Available online at: https://curia.europa.eu/juris/document/document.jsf?text=&docid=269405&pageIndex=0&doclang=EN&mode=lst&dir=&occ=first&part=1&cid=7397235Acknowledgments.
International Confederation of European Beet Growers. (2018). The case for neonicotinoids in pelleted sugar beet seeds. D.004/19.1.2018. Available online at: https://www.cibe-europe.eu/PlantProtectionProducts.
International Programme on Chemical Safety, World Health Organization. (1986). Carbamate pesticides: a general introduction (Geneva: World Health Organization). Available at: https://iris.who.int/handle/10665/38687.
Jactel H., Verheggen F., Thiéry D., Escobar-Gutiérrez A. J., Gachet E., Desneux N. (2019). Alternatives to neonicotinoids. Environ. Int. 129, 423–429. doi: 10.1016/j.envint.2019.04.045
Jepson J. E. C., Brown L. A., Sattelle D. B. (2006). The actions of the neonicotinoid imidacloprid on cholinergic neurons of Drosophila melanogaster. Invert Neurosci. 6, 33–40. doi: 10.1007/s10158-005-0013-8
Kereši T., Sekulić R. R., Čačić N. J., Forgić G. Đ., Marić V. R. (2006). Control of sugar beet pests at early season by seed treatment with insecticides. Zbornik Matice srpske za prirodne nauke 110), 195–204. doi: 10.2298/ZMSPN0610195K
Keszthelyi S., Gerbovits B., Jócsák I. (2023). Impact analysis of different applications of cyantraniliprole on control of horse chestnut leaf miner (Cameraria ohridella) larvae supported by biophoton emission. Biol. Futura 74, 1–11. doi: 10.1007/s42977-023-00169-0
Keszthelyi S., Kurucsai P., Szabó T., Pál-Fám F., Marczali Zs. (2008). “Chemical protections against corn weevil (Tanymecus dilaticollis Gyll.) on different soil types,” in Cereal Research Communications; Vol. 36, June 2008, Supplement: Proceedings of the VII. Alps-Adria Scientific Workshop, Springer, Stara Lesna, Slovakia.
Keszthelyi S., Lukács H., Kazinczi G., Varga Zs. (2022). A cukorrépa védelme. Növényvédelem 83 [N. S. 58], 1.
Krupke C. H., Hunt G. J., Eitzer B. D., Andino G., Given K. (2012). Multiple routes of pesticide exposure for honey bees living near agricultural fields. PloS One 7, e29268. doi: 10.1371/journal.pone.0029268
Lange W. H. (1987). Insect pests of sugar beet. Annu. Rev. Entomol. 32, 341–360. doi: 10.1146/annurev.en.32.010187.002013
Laurent A., Favrot A., Maupas F., Royer C., Makowski D. (2023). Assessment of non-neonicotinoid treatments against aphids on sugar beets. Crop Prot 164, 106140. doi: 10.1016/j.cropro.2022.106140
Lemic D., Benítez H. A., Püschel T. A., Gašparić H. V., Šatvar M., Bažok R. (2016). Ecological morphology of the sugar beet weevil Croatian populations: Evaluating the role of environmental conditions on body shape. Zoologischer Anzeiger-A J. Comp. Zool. 260, 25–32. doi: 10.1016/j.jcz.2015.11.003
Lundin O., Rundlöf M., Smith H. G., Fries I., Bommarco R. (2015). Neonicotinoid insecticides and their impacts on bees: a systematic review of research approaches and identification of knowledge gaps. PloS One 10, e0136928. doi: 10.1371/journal.pone.0136928
Mandal S. (2012). Bio-efficacy of Cyazypyr 10% OD, a new anthranilic diamide insecticide, against the insect pests of tomato and its impact on natural enemies and crop health. Acta Phytopath Entomol Hung 47, 233–249. doi: 10.1556/APhyt.47.2012.2.6
Meredith R. H., Morris D. B. (2003). Clothianidin on sugar beet: field trial results from Northern Europe. PflanzenschutzNachrichten Bayer (English edition) 56, 111–126.
Mörtl M., Kereki O., Darvas B., Klátyik S., Vehovszky Á., Győri J., et al. (2016). Study on soil mobility of two neonicotinoid insecticides. J. Chem 2016, 4546584. doi: 10.1155/2016/4546584
National Association of Sugar Beet Growers. (2020). Application for the granting of an emergency authorisation for the use of the neonicotinoid seed treatment (thiamethoxam) in industrial sugar beet in Hungary for 2021. (Sopronhorpács: National Association of Sugar Beet Growers (CTOSZ), Beta Research Institute Nonprofit Ltd.).
National Food Chain Safety Office. (2023). Cruiser SB insecticide refusal of an application for emergency use (official procedure). (Budapest: National Food Chain Safety Office, Directorate for Plant Protection and Wine).
Nauen R., Jeschke P., Velten R., Beck M. E., Ebbinghaus-Kintscher U., Thielert W., et al. (2015). Flupyradifurone: a brief profile of a new butenolide insecticide. Pest Manage. Sci. 71, 850–862. doi: 10.1002/ps.3932
Nauen R., Reckmann U., Thomzik J., Thielert W. (2008). Biological profile of spirotetramat (Movento®)–a new two-way systemic (ambimobile) insecticide against sucking pest species. Bayer CropSci. J. 61, 245–278.
Official Journal of the European Union. (2018a). Commission Implementing Regulation (EU) 2018/785. Available online at: https://eur-lex.europa.eu/legal-content/EN/TXT/?uri=CELEX%3A32018R0785.
Official Journal of the European Union. (2018b). Commission Implementing Regulation (EU) 2018/784. Available online at: https://eur-lex.europa.eu/legal-content/EN/TXT/?uri=CELEX%3A32018R0784.
Official Journal of the European Union. (2018c). Commission Implementing Regulation (EU) 2018/783. Available online at: https://eur-lex.europa.eu/legal-content/EN/TXT/?uri=CELEX%3A32018R0783.
Palmquist K., Salatas J., Fairbrother A. (2012). “Pyrethroid insecticides: use, environmental fate, and ecotoxicology,” in Insecticides-advances in integrated pest management. (Rijeka: InTech), 251–278.
Potyondi L., Kimmel J., Csima F., Takács É. (2014). “The effect of climate change on sugar beet pests and diseases in Hungary,” in 74th IIRB Congress, Dresden: Poster presentations - Abstracts and proceedings [online] p. 101.
Radics L. (2012). Fenntartható szemléletű szántóföldi növénytermesztéstan 2. Agroinform kiadó Budapest 2012, 171–191.
Rath L. K., Nayak U. S. (2013). Field evaluation of cyazypyr against yellow stem borer and gall midge infesting rice in western Odisha. ORYZA Int. J. Rice 50, 375–437.
Romanowski H., Blake L. (2023). Neonicotinoid seed treatment on sugar beet in England: a qualitative analysis of the controversy, existing policy and viability of alternatives. J. Environ. Stud. Sci. 13, 453–472. doi: 10.1007/s13412-023-00830-z
Schaafsma A., Limay-Rios V., Baute T., Smith J., Xue Y. (2015). Neonicotinoid insecticide residues in surface water and soil associated with commercial maize (corn) fields in southwestern Ontario. PloS One 10, e0118139. doi: 10.1371/journal.pone.0118139
Scott C., Bilsborrow P. E. (2019). The impact of the EU neonicotinoid seed-dressing ban on oilseed rape production in England. Pest Manag. Sci. 75, 125–133. doi: 10.1002/ps.5189
Selby T. P., Lahm G. P., Stevenson T. M., Hughes K. A., Cordova D., Annan I. B., et al. (2013). Discovery of cyantraniliprole, a potent and selective anthranilic diamide ryanodine receptor activator with cross-spectrum insecticidal activity. Bioorg. Med. Chem. Lett. 23, 6341–6345. doi: 10.1016/j.bmcl.2013.09.076
Shuai J., Wang X., Li G., Kong Y., Li W., Li Z., et al. (2022). Study on the mode of action between Apis mellifera (α8) 2 (β1) 3 nAChR and typical neonicotinoids versus flupyradifurone with different bee-toxic levels. J. Mol. Graphics Model. 114, 108177. doi: 10.1016/j.jmgm.2022.108177
Sigareva D. D., Kovtun A. M., Kornyushin V. V. (2019). Occurrence of entomopathogenic nematodes (Rhabditida: Steienernematidae, Heterorhabditidae) from agricultural ecosystems in Forest (Polissya) and Forest-Steppe natural zones of Ukraine. Vestnik Zoologii 53, 285–296. doi: 10.2478/vzoo-2019-0028
Smith H. A., Nagle C. A., MacVean C. A., McKenzie C. L. (2016). Susceptibility of Bemisia tabaci MEAM1 (Hemiptera: Aleyrodidae) to imidacloprid, thiamethoxam, dinotefuran and flupyradifurone in south Florida. Insects 7, 57. doi: 10.3390/insects7040057
Soderlund D. M. (2010). “Toxicology and mode of action of pyrethroid insecticides,” in Hayes’ handbook of pesticide toxicology (London: Academic Press), 1665–1686.
Syngenta Seedcare. (2023a). cruiser. Available online at: https://syngentaseedcare.com/cruiser.
Syngenta Seedcare. (2023b). force. Available online at: https://www.syngentaseedcare.com/force.
Tomizawa M., Casida J. E. (2003). Selective toxicity of neonicotinoids attributable to specificity of insect and mammalian nicotinic receptors. Annu. Rev. Entomol 48, 339–364. doi: 10.1146/annurev.ento.48.091801.112731
Tomizawa M., Casida J. E. (2005). Neonicotinoid insecticide toxicology: mechanisms of selective action. Annu. Rev. Pharmacol. Toxicol. 45, 247–268. doi: 10.1146/annurev.pharmtox.45.120403.095930
Viric Gasparic H. V., Lemic D., Drmic Z., Cacija M., Bazok R. (2021). The efficacy of seed treatments on major sugar beet pests: possible consequences of the recent neonicotinoid ban. Agronomy 11, 1277. doi: 10.3390/agronomy11071277
Wang R., Wang J., Zhang J., Che W., Feng H., Luo C. (2020). Characterization of flupyradifurone resistance in the whitefly Bemisia tabaci Mediterranean (Q biotype). Pest Manage. Sci. 76, 4286–4292. doi: 10.1002/ps.5995
Woodcock B. A., Bullock J. M., Shore R. F., Heard M. S., Pereira M. G., Redhead J., et al. (2017). Country-specific effects of neonicotinoid pesticides on honey bees and wild bees. Science 356, 1393–1395. doi: 10.1126/science.aaa1190
Woodcock B. A., Isaac N. J., Bullock J. M., Roy D. B., Garthwaite D. G., Crowe A., et al. (2016). Impacts of neonicotinoid use on long-term population changes in wild bees in England. Nat. Commun. 7, 12459. doi: 10.1038/ncomms12459
World Health Organization, International Programme on Chemical Safety, WHO Task Group Meeting on Environmental Health Criteria for Cypermethrin. (1989). Cypermethrin/published under the joint sponsorship of the United Nations Environment Programme, the International Labour Organisation, and the World Health Organization (Geneva: World Health Organization). Available at: https://iris.who.int/handle/10665/40017.
Keywords: insecticides, crop protection, in-crop treatment, juvenile arthropod pest, Beta vulgaris production, systemic mode of action
Citation: Jócsák I, Csima F, Pál-Fám F, Somfalvi-Tóth K and Keszthelyi S (2024) Plant protection consequences in Hungary of the withdrawal of systemic active substances from sugar beet seed treatment. Front. Agron. 6:1363950. doi: 10.3389/fagro.2024.1363950
Received: 31 December 2023; Accepted: 25 March 2024;
Published: 10 April 2024.
Edited by:
Rachid Lahlali, Ecole Nationale d’Agriculture de Meknès, MoroccoReviewed by:
Spiridon Mantzoukas, University of Ioannina, GreeceYuguang Wang, Heilongjiang University, China
Copyright © 2024 Jócsák, Csima, Pál-Fám, Somfalvi-Tóth and Keszthelyi. This is an open-access article distributed under the terms of the Creative Commons Attribution License (CC BY). The use, distribution or reproduction in other forums is permitted, provided the original author(s) and the copyright owner(s) are credited and that the original publication in this journal is cited, in accordance with accepted academic practice. No use, distribution or reproduction is permitted which does not comply with these terms.
*Correspondence: Ildikó Jócsák, jocsak.ildiko@uni-mate.hu
†These authors have contributed equally to this work and share first authorship