Elicitors of plant defenses as a standalone tactic failed to provide sufficient protection to fruits against spotted-wing drosophila
- 1P. E. Marucci Center, Rutgers University, Chatsworth, NJ, United States
- 2IRTA, Sustainable Plant Protection Program, Cabrils, Barcelona, Spain
Spotted-wing drosophila, Drosophila suzukii (Matsumura), is a globally important invasive pest of soft-skinned fruits including raspberries, blueberries, and strawberries. Current control measures for this pest primarily rely on calendar-based insecticide sprays; therefore, more sustainable methods are desirable. Adults of D. suzukii use cues derived from fruits and symbiotic microbes to identify optimal sites for feeding, mating, and oviposition. We hypothesized that modifying any of these cues could influence D. suzukii adult behavior, making them less likely to select suitable fruits. One approach involves applying synthetic elicitors of the salicylic acid (SA) and jasmonic acid (JA) plant defense pathways, which are associated with defenses against pathogens and herbivores, respectively, or the use of crop sterilants, employed for microbial control, to reduce D. suzukii attraction, oviposition, and larval performance in fruits. Here, the preference and performance of D. suzukii was investigated in blueberry fruits treated exogenously with four commercial elicitors of plant defenses – three that primarily activate SA-related defenses (Actigard, LifeGard, and Regalia) and one activator of JA-related defenses (Blush) – and the crop sterilant OxiDate. A series of choice and no-choice experiments were conducted in laboratory, semi-field, and field settings that were performed at various time intervals to assess the residual effects of each treatment. Our results showed no consistent repellent, oviposition deterrent, or larval toxicity activity for any of the products tested. As a result, our findings do not provide sufficient support to recommend the use of these elicitors or the crop sterilant as viable standalone options for D. suzukii management.
1 Introduction
Plants produce secondary metabolites to protect themselves against herbivores and pathogens (Levin, 1976; Rhoades and Cates, 1976; Mithöfer and Boland, 2012). These secondary plant metabolites may be constantly present within the plant (constitutive defenses) or they may be produced in response to attack by an herbivore or pathogen (induced defenses) (Karban and Myers, 1989). Induced defenses rely on signaling compounds known as ‘elicitors’ to initiate the plant’s defense response pathways. Elicitors include a wide variety of molecules, such as proteins, lipids, and carbohydrates found in various plant, microbial, and insect products (Lyon, 2007; Reignault and Walters, 2007; Meena et al., 2022). Once a plant interacts with an elicitor, one or more signal-transduction pathways are activated. Two important signal-transduction pathways involved in plant defenses are regulated by the phytohormones jasmonic acid (JA) and salicylic acid (SA) (Karban and Baldwin, 1997; Smith et al., 2009; War et al., 2012). The JA-mediated pathway is typically induced in response to chewing damage or necrotrophic pathogens (Walling, 2000; Beckers and Spoel, 2006). In contrast, the SA-mediated pathway is associated with immune responses to piercing-sucking insects (War et al., 2011) or biotrophic pathogen infection (Thomma et al., 1998; Glazebrook, 2005).
Recently, there has been significant interest in the potential of exogenously applying naturally found elicitors or their synthetic derivatives to protect plants against herbivores and pathogens (Ruiz-García and Gómez-Plaza, 2013; Bektas and Eulgem, 2015; Moreno-Pérez et al., 2020). Several elicitors of the SA pathway are commercially available as ‘plant bioactivators’ of defenses and are often marketed as biofungicides (Bektas and Eulgem, 2015; Zehra et al., 2021). These synthetic elicitors have been successful at increasing resistance to pathogenic diseases in many crops, such as Pythium damping-off in cucumbers (Benhamou and Bélanger, 1998), powdery mildew in okra (Vimala and Suriachandraselvan, 2008), and fire blight in apples (Brisset et al., 2000). A few studies have also reported reductions in the fitness of herbivorous arthropods that fed on crops treated with an elicitor (Correa et al., 2005; Uefune et al., 2014; Gordy et al., 2015; Sobhy et al., 2015). For example, the application of the SA-elicitor Actigard to potted pear trees reduced the survival of the pear psylla Cacopsylla pyricola (Förster) under laboratory conditions (Cooper and Horton, 2015), but failed to provide sufficient protection under field conditions (Cooper and Horton, 2017). Thus, by inducing host-plant resistance, elicitors of plant defenses may hold promise as environmentally safer alternatives to conventional insecticides employed in pest management programs (Stout et al., 2002; Pickett et al., 2014; Bektas and Eulgem, 2015; Divekar et al., 2022); however, additional studies are required to assess their efficacy under both laboratory and field conditions.
The vinegar fly spotted-wing drosophila, Drosophila suzukii (Matsumura) (Diptera: Drosophilidae), is an invasive pest from Southeast Asia that causes severe damage to soft, thin-skinned fruits such as raspberries, strawberries, and blueberries (Walsh et al., 2011; Lee et al., 2015). While other control methods, such as cultural control, behavioral control, and biological control, are currently under evaluation (Lee et al., 2019; Tait et al., 2021), D. suzukii management continues to depend heavily on frequent insecticide sprays (Diepenbrock et al., 2016). However, due to the unsustainability of continued insecticide use, behavioral manipulation may be more sustainable for D. suzukii management. Adults of D. suzukii utilize several cues from ripe host fruits and symbiotic microbes to detect suitable sites for feeding, mating, and oviposition (Keesey et al., 2015; Cloonan et al., 2018). One potential way of changing these cues is by applying elicitors of plant defenses to fruit crops. Elicitors may directly induce changes in the quality or quantity of volatiles typically emitted from fruits (Pagadala Damodaram et al., 2015; Salifu et al., 2022) or indirectly through their fungicidal effects on associated microbes. For instance, elicitors of the SA pathway may reduce the presence of Hanseniaspora uvarum, a symbiotic yeast closely associated with D. suzukii (Hamby et al., 2012), and thus reduce the attractiveness of fruits to flies (Hamby and Becher, 2016; Rehermann et al., 2022). In fact, fungicides have successfully reduced H. uvarum levels in other fruits, such as grapes (Agarbati et al., 2019). However, the effects of commercially-available elicitors of plant defenses on D. suzukii behavior towards fruits have not yet been studied.
Here, we hypothesized that elicitor-induced responses in fruits may cause D. suzukii adults to avoid these fruits or reduce the performance of larvae within the fruits. To test this hypothesis, we treated highbush blueberries, Vaccinium corymbosum L., fruits exogenously with four elicitors – three that primarily activate SA-related plant defenses (Actigard, LifeGard, and Regalia) and one activator of JA-related defenses (Blush) – to determine their effects on D. suzukii preference and performance. This was achieved through a series of no-choice and choice experiments conducted under laboratory, semi-field, and field conditions. Because Van Timmeren et al. (2020) successfully inhibited H. uvarum growth in the laboratory with a crop sterilant (peroxyacetic acid and hydrogen peroxide) developed for disease control, we also included a crop sterilant (OxiDate) in our study. The findings from our study may contribute to the potential behavior-based management options currently available for D. suzukii.
2 Materials and methods
2.1 Insects
The D. suzukii colony used for all experiments was started in 2013 and was maintained in 50-mL Drosophila polystyrene vials (Genesee Scientific, San Diego, CA, USA) filled to ~1/3 their total volume (15 mL) with a standard Drosophila artificial diet (Jaramillo et al., 2015). Flies were reared in a laboratory at Rutgers University (New Brunswick, NJ) at 22 ± 2°C, 55 ± 5% relative humidity (RH), and 16:8 (L:D) h photoperiod. The flies were 5–10 days old and thus were sexually mature (Revadi et al., 2015). Before each experiment, the flies were given a starvation period of approximately 4 hours.
2.2 Treatments
A total of six treatments were tested, including three elicitors of SA-related defenses: Actigard® 50WG [acibenzolar-S-methyl (active ingredient (a.i.)), Syngenta (Basel, Switzerland)] at 54.8 mL/ha (0.75 oz/acre); LifeGard® WG [Bacillus mycoides (40%) (a.i.), Certis Biologicals USA LLC (Columbia, MD, USA)] at 0.16 L/ha (2.25 oz/acre); and Regalia® [Reynoutria sachalinensis extract (5%) (a.i.), Marrone Bio Innovations Inc. (Davis, CA, USA)] at 5.62 L/ha (2 qt/acre). In addition, Blush® 2X [prohydrojasmon (10%) (a.i.), Fine Americas (Walnut Creek, CA, USA)], an elicitor of JA-related defenses, and the crop sterilant OxiDate® 2.0 [hydrogen peroxide (27.1%) and peroxyacetic acid (2.0%) (a.i.), Biosafe Systems LLC (East Hartford, CN, USA)] were tested; both at a 1:100 (product:water) ratio.
All products used are commercially available. Actigard, LifeGard, and Regalia were selected because, despite all activating the SA pathway, these biofungicides contain different active ingredients, which could affect D. suzukii behavior differently. Moreover, they are commonly utilized for microbial control in various crops (e.g., Louws et al., 2001; Su, 2012; Szymanski et al., 2023). Blush was chosen as it is currently the only commercial activator of the JA pathway. These activators of the SA and JA pathways were anticipated to potentially influence D. suzukii attraction to fruits by directly altering volatile emissions. However, we also hypothesized that they, particularly the SA elicitors, might indirectly affect D. suzukii attraction to fruits by influencing mutualistic microbes such as H. uvarum. Given a previous study’s indication that products like Jet-Ag® (which has the same active ingredients as OxiDate) could reduce H. uvarum (Van Timmeren et al., 2020), we included this treatment as a positive control.
All product treatments were diluted with distilled water and, to maximize their effects, they were applied at the maximum recommended field rate according to the manufacturer’s label. The sixth treatment was a distilled water control. Because our laboratory and 2019 semi-field data showed that Blush had no or minimal activity against D. suzukii (see Results), this elicitor was later removed from testing in 2022 semi-field and field experiments.
2.3 Laboratory experiments
We first conducted olfactory and oviposition laboratory bioassays by applying the elicitors and sterilant directly to diet cups, testing their potential repellent and oviposition deterrent effects on D. suzukii in the absence of fruits. These experiments were conducted at 22 ± 2°C, 55 ± 5% RH, and 16:8 (L:D) h photoperiod.
2.3.1 Olfactory bioassays
Olfactory paired-choice tests were conducted to determine the repellent effects of the treatments on D. suzukii adults following the methods by Feng et al. (2018) and Urbaneja-Bernat et al. (2021). We employed Drosophila polystyrene vials used for rearing as gated traps, which were filled with ~15 mL of diet (prepared as described above) for these assays. Two vials were placed in a 946.4 mL (32-oz) deli container, one of the vials was treated with an elicitor or sterilant and the other was treated with distilled water. For the treated vials, 2 sprays (approx. 0.28 mL) of each treatment were applied to the top surface of the diet via 59.1 mL plastic fingertip spray bottles (Bottle Crew LLC, West Bloomfield, MI, USA), and the vials were allowed to dry under a fume hood for an hour before testing. The vials were then sealed with Parafilm with a 4 mm-diameter hole in the center to allow flies to enter the vials; once inside, the flies rarely escaped (A.Q., personal observation). Ten flies (5 males and 5 females) were placed in each container (Figure 1A). The containers were positioned under a fume hood, and the flies were given a 48-hour period to make their choice. Subsequently, the number of flies in each vial was recorded. The experiment was replicated five times for each choice combination (N = 5 replicates × 10 flies × 6 combinations = 300 total flies).
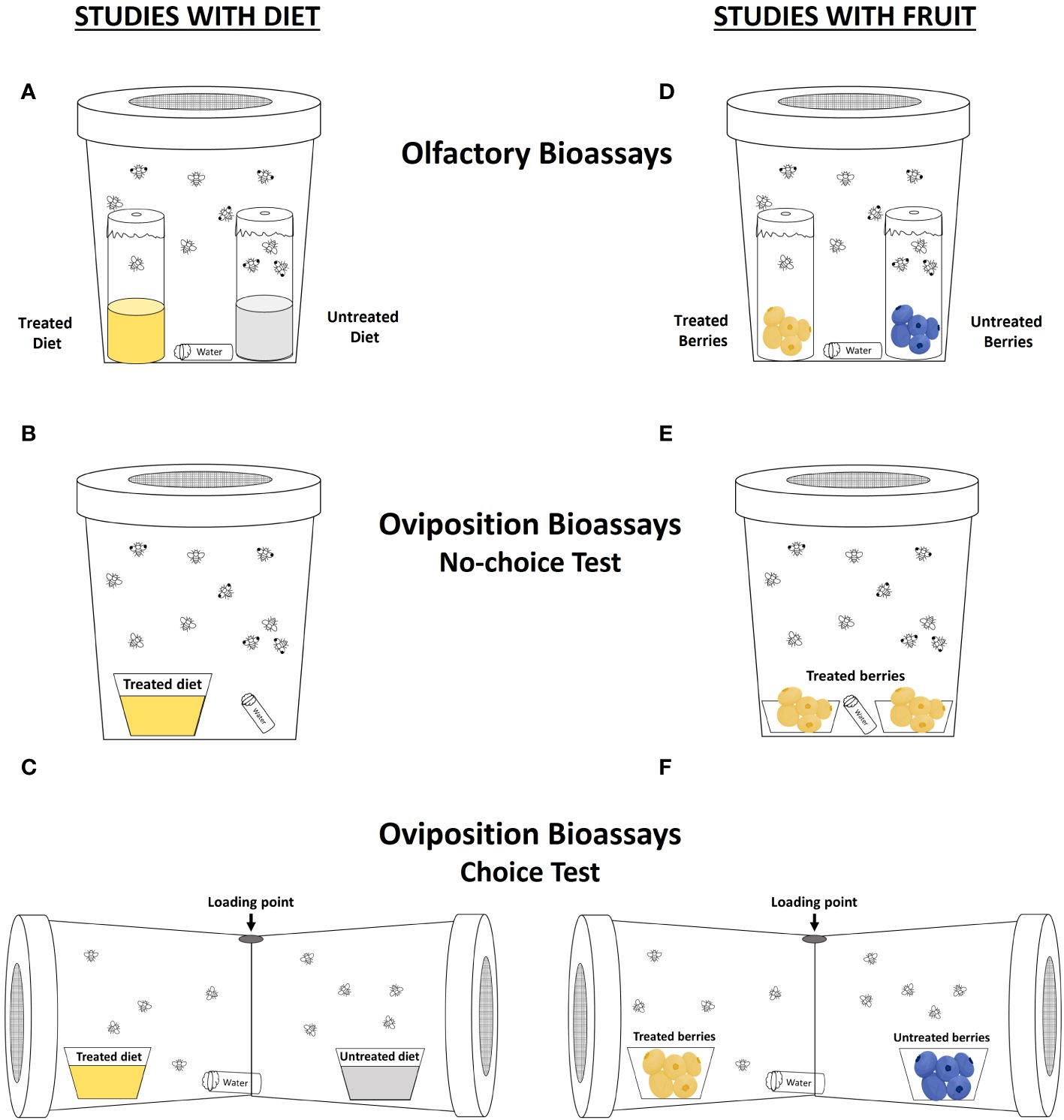
Figure 1 Setup of repellency (A, D), no-choice oviposition (B, E), and choice oviposition (C, F) tests. Tests used either diet (A–C) to assess direct effects of treatment on Drosophila suzukii adult behavior or blueberry fruits (D–F) to assess whether the effects of treatment are indirectly mediated through changes in fruit chemistry.
2.3.2 Oviposition bioassays
To determine oviposition effects, we used both paired-choice and no-choice assays. For these assays, 29.6 mL (1-oz) plastic cups containing 15 mL of diet were used. Treatments were applied to cups (top surface of the diet) using spray bottles as described above and then allowed to dry under a fume hood for an hour before testing.
No-choice tests. For oviposition no-choice tests, a 29.6 mL cup with treated diet was placed in the center of a larger 473.2 mL (16-oz) deli container (Figure 1B). Five sexually mature D. suzukii females were placed in the container, and then the container was sealed with a lid containing small holes for ventilation. These containers were left on a laboratory bench at 22 ± 2°C and 55 ± 5% relative humidity for 48 hours. After 48 hours, the flies were removed, and the cups were inspected for the number of eggs laid in the diet. The cups were further incubated for two weeks to test for adult emergence. Each treatment, including the control (water only), was replicated five times (N = 5 replicates × 5 flies × 6 treatments = 150 total flies).
Choice tests. For the oviposition paired-choice study, choice arenas were constructed by removing the bottoms of two 946.4 mL deli containers and taping the containers together end to end (Figure 1C). One side of the arena contained a diet cup that was treated with an elicitor or sterilant while the other end contained a diet cup that was treated with water. Ten D. suzukii females were added to each arena for 48 hours. These arenas were placed on the laboratory bench under the conditions described above. After 48 hours, the flies were removed, and the diet cups were inspected for the number of eggs laid in the diet. The cups were further incubated for two weeks to test for adult emergence. Each choice combination was replicated five times (N = 5 replicates × 10 flies × 6 combinations = 300 total flies).
2.4 Semi-field experiments
In 2019 and 2022, we conducted semi-field experiments to evaluate whether the influence of the elicitors or sterilant on D. suzukii is mediated by fruits. For these experiments, highbush blueberry (V. corymbosum cv. ‘Bluecrop’) fruits were treated in the field and, subsequently, they were utilized for repellent and oviposition deterrent tests in the laboratory, as described above for tests with diet. Three rows of field-grown highbush blueberry bushes at the Rutgers P.E. Marucci Center (Chatsworth, New Jersey) were selected, and 10 bushes were randomly assigned to each treatment. Before fruit ripening (early June), four terminals per bush containing green berries were bagged with cloth bags to prevent any infestation from resident D. suzukii before the start of the studies. Once the study began (end of June/early July), the bags were opened and the terminals inside the bags were sprayed with a treatment at field rate (see section 2.2) to dripping. After the treatments, the bags were closed again and re-opened as needed for berry sampling. In 2019, berries were collected only once, 24 hours after being sprayed (i.e., at 1 day after treatment (DAT)). However, in 2022, berries were collected from the sprayed terminals to be set up in laboratory assays at four time periods – 1, 3, 7, and 10 DAT – to test for residual effects.
2.4.1 Olfactory bioassays
For olfactory paired-choice tests, similar methods were used as described above for the diet study but with berries instead of a diet. Two gated-trap vials were placed in a 946.4 mL deli container. One of the vials contained five blueberries treated with an elicitor or sterilant, while the other contained untreated berries (Figure 1D). The vials were sealed with Parafilm with a 4 mm-diameter hole in the center to allow the flies to enter the vials. Ten flies (5 males and 5 females) were placed in each container. The containers were positioned in a fume hood under the laboratory conditions described above, and the flies were given a 24-hour period to make their choice. Afterward, the number of flies in each vial was recorded. There were five replicates per choice combination and time interval (N = 5 replicates × 10 flies × 5 combinations × 4-time intervals = 1,000 total flies).
2.4.2 Oviposition bioassays
No-choice tests. For oviposition no-choice tests, 10 treated berries (5 berries in each of two 29.6 mL cups) were placed inside a 473.2 mL deli container (Figure 1E). Ten sexually mature D. suzukii females were placed in the container, which was then sealed with a lid containing fine nylon mesh for ventilation. These cups were left on a bench in the laboratory, under the conditions described above, for 24 hours. After 24 hours, the flies were removed, and the berries were inspected for the number of eggs laid. The berries were then transferred to 118.3 mL plastic cups lined with a cotton pad and incubated for two weeks to measure adult emergence. Each time interval had five replicates per treatment (N = 5 replicates × 10 flies × 5 combinations × 4-time intervals = 1,000 total flies).
Choice tests. For oviposition paired-choice tests, the same choice arenas that were used in the diet study were used in these assays; however, instead of diet, 5 blueberries were placed in 59.1 mL plastic cups (Figure 1F). One side of the arena contained a cup with 5 berries treated with an elicitor or sterilant, while the other end contained a cup with 5 untreated berries. Ten D. suzukii females were added to each arena, and each arena was placed on a bench in the laboratory, under the conditions described above, for 24 hours. After 24 hours, the berries were inspected for eggs laid, and the berries were then kept in 118.3 mL cups for incubation to measure adult emergence. There were five replicates per choice combination and time interval (N = 5 replicates × 10 flies × 5 combinations × 4-time intervals = 1,000 total flies).
2.5 Field experiments
Field cage experiments were conducted in June–July 2022 at the Rutgers P.E. Marucci Research Center using highbush blueberries (V. corymbosum cv. ‘Duke’). The studies were carried out in 5.5 m long × 2.7 m tall cages, which consisted of a PVC pipe frame covered with a No-See-Um mesh (Quest Outfitters Inc., Sarasota, FL, USA). Cages were positioned in separate rows of bushes within a blueberry field, with each bush spaced approximately 0.76 m apart within the rows and 3.05 m between rows. To prevent any potential interference between treatments, cages were alternatively placed in rows, ensuring that no two cages were adjacent to each other. Two 3-m rebars were used to secure the mesh on each of the long sides of the cages, and the shorter ends of the cage were secured shut using clips. Five blueberry bushes were contained within each cage. The elicitors Actigard, LifeGard, and Regalia and the sterilant OxiDate were prepared at a recommended rate according to the label (see section 2.2). Once each treatment was mixed, all five bushes within the cages were sprayed to dripping. Control cages were treated with water. Each treatment was replicated 4 times, and each cage was designated to a single treatment (20 cages total).
Twenty-four hours after treatment, 100 flies (50 males and 50 females) were released into each cage (N = 4 replicates × 100 flies × 5 treatments = 2,000 total flies). Berries were collected at 3, 7, and 10 DAT. Twenty-five berries from the top portion (upper half) of each bush and 25 from the lower portion (bottom half) of each bush in the cage were collected into two separate 236.6 mL (8-oz) deli containers lined with two cotton pads. The blueberries were then incubated on a light bench in the laboratory, as described above, for up to two weeks and adult emergence was recorded.
2.6 Statistical analysis
Data for each year and time interval were analyzed separately. Chi-square tests were conducted for each olfactory paired-choice test, based upon the null hypothesis of an equal probability (50:50) of flies choosing a control versus treated diet or fruit; only flies that made a choice were included in the analysis. For the no-choice oviposition tests (dependent variable = number of eggs laid in diet or fruit), we employed generalized linear models (GLM) assuming a Poisson distribution and a logit link function, with treatment as the independent variable. Data from oviposition choice tests were analyzed using student’s (paired-samples) t-tests to compare differences in number of eggs laid in diet or fruit (dependent variable) between control and elicitor/sterilant treatments.
In the field cage experiment, the independent variables ‘treatment’ (i.e., elicitors, sterilant, and control) and the ‘position’ of fruit on the bush (i.e., top versus bottom) were used as fixed factors, while the number of flies emerging from the berries served as the dependent variable. If the GLM test showed statistical significance, we used the Bonferroni test (P ≤ 0.05) for post-hoc pairwise comparisons. All statistical analysis was performed using SPSS 23.0 software (IBM Corp, 2015).
3 Results
3.1 Laboratory experiments
3.1.1 Olfactory bioassays
There were no effects of the elicitors or sterilant on D. suzukii response to volatiles from the diet (all P values > 0.05; Figure 2A).
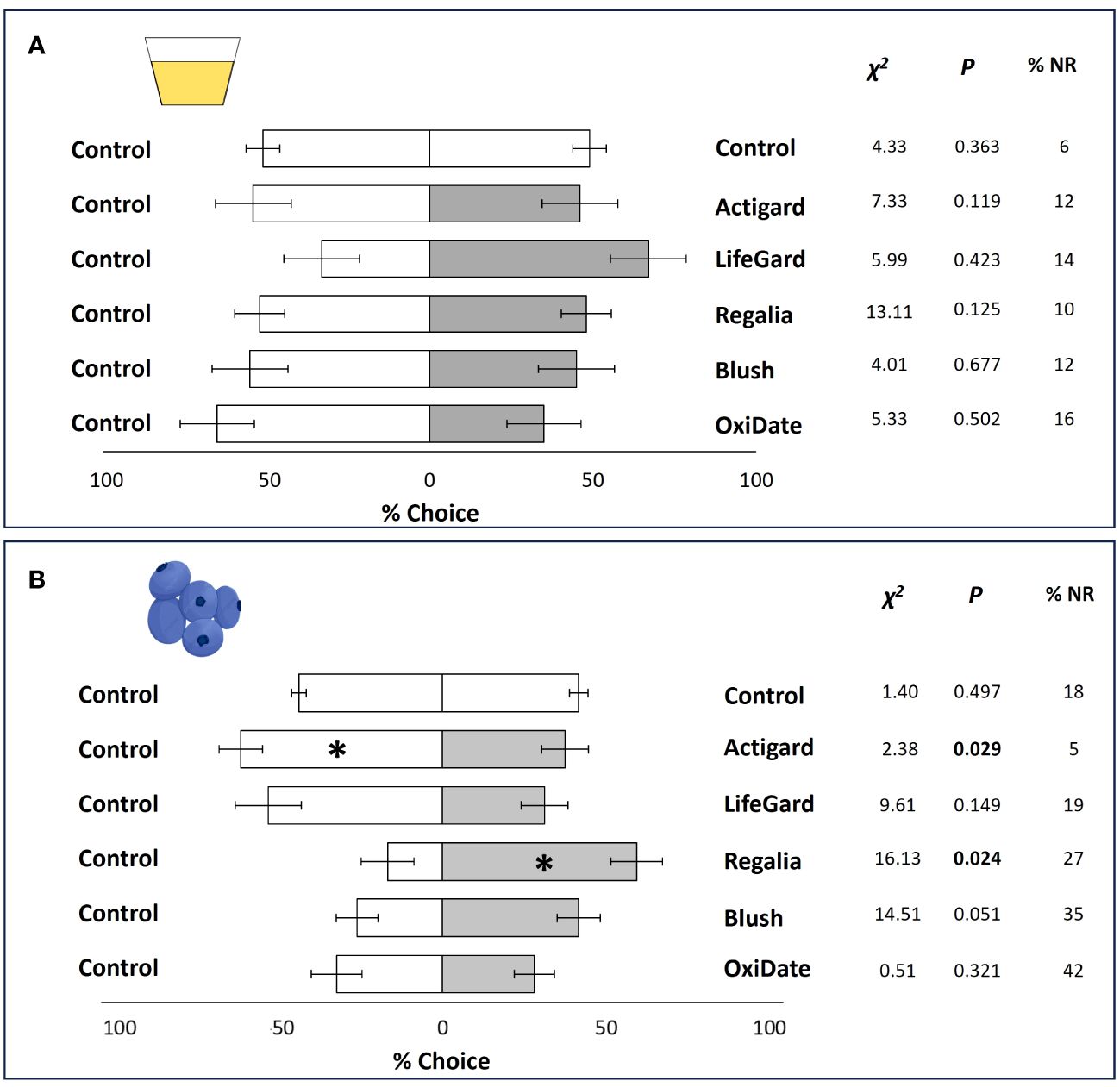
Figure 2 Results from diet and 2019 olfactory tests. Mean percentage (± SE) of Drosophila suzukii flies that made a choice between treated and untreated diet (A). Mean percentage (± SE) of D. suzukii flies that made a choice between treated and untreated fruit (B). Significant P-values according to Chi-square tests are indicated in bold and by an asterisk (P ≤ 0.05). % NR = percent of non-respondents. N = 5.
3.1.2 Oviposition bioassays
3.1.2.1 No-choice tests
In oviposition no-choice assays, we also found no effects of the elicitors or sterilant when treated to diet on the number of eggs laid (P > 0.05; Figure 3A) or number of adults that emerged from the treated diet (P > 0.05; Figure 3B).
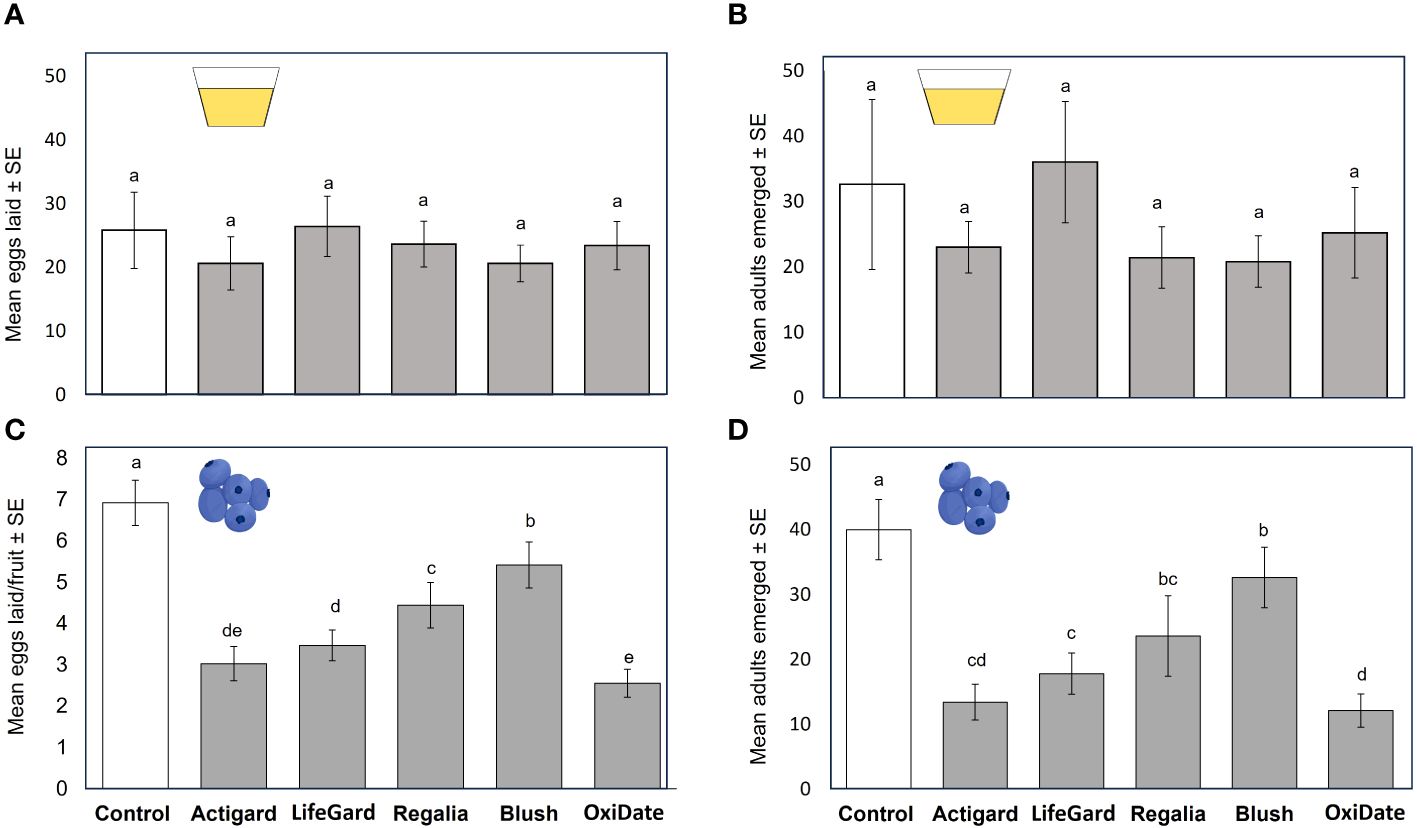
Figure 3 Results from diet and 2019 no-choice oviposition tests. Mean (± SE) number of Drosophila suzukii eggs laid in diet (A) or fruit (C). Mean (± SE) number of D. suzukii adults that emerged from diet (B) or fruit (D). Different letters indicate significant differences among treatments according to Bonferroni tests (P ≤ 0.05). N = 5.
3.1.2.2 Choice tests
In oviposition paired-choice assays, D. suzukii laid fewer eggs (t = 2.48, df = 8, P = 0.038) and had lower adult emergence (t = 2.61, df = 8, P = 0.031) in the Actigard-treated diet compared with the control diet, while all other choice combinations were non-significant (P values > 0.05) (Figures 4A, B).
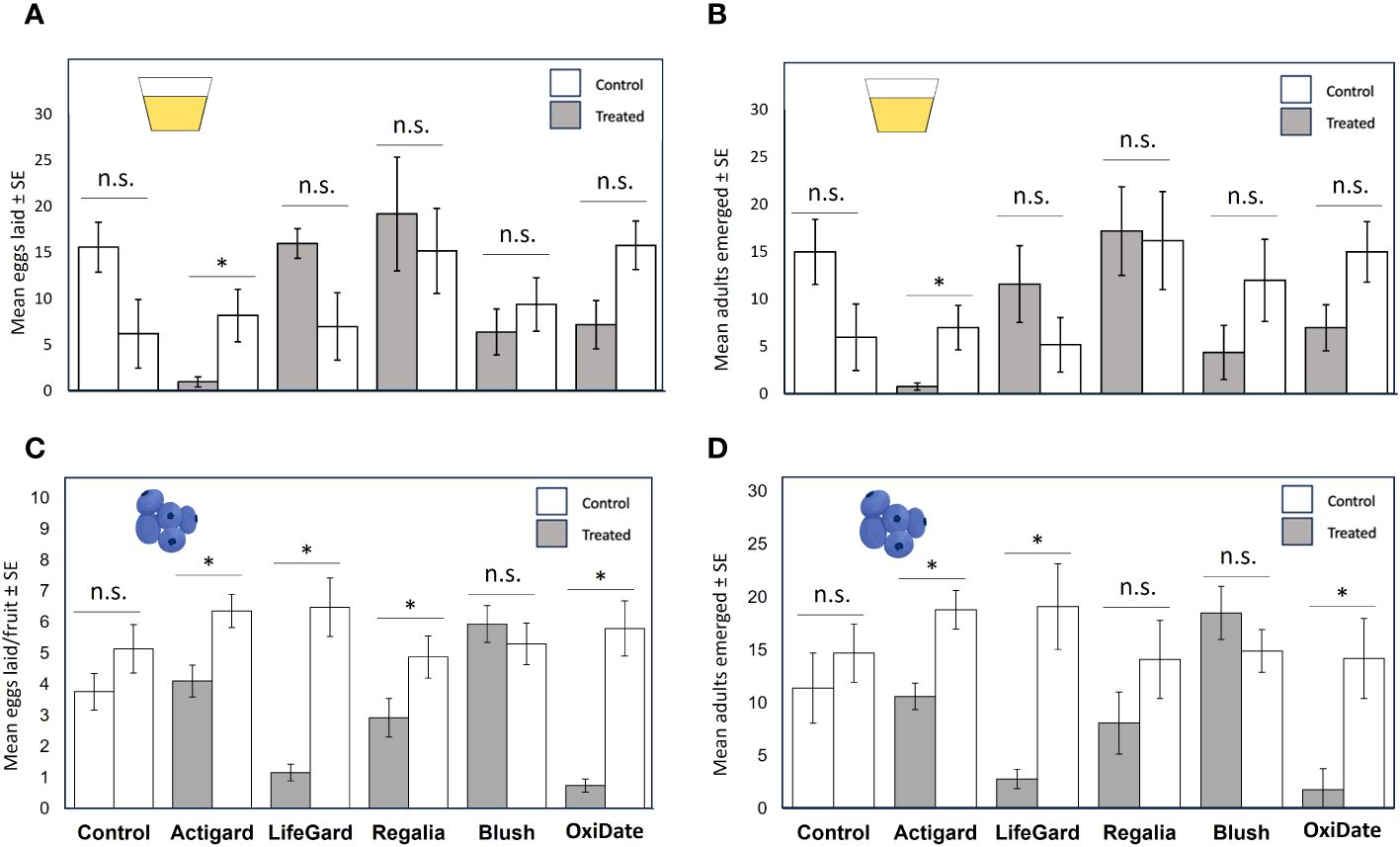
Figure 4 Results from diet and 2019 choice oviposition tests. Mean (± SE) number of Drosophila suzukii eggs laid between treated and untreated diet (A) and between treated and untreated fruit (C). Mean (± SE) number of D. suzukii adults that emerged from treated or untreated diet (B) and treated or untreated fruit (D). An asterisk indicates a significant difference between treatments according to t-tests (P ≤ 0.05). n.s. = not significant (P > 0.05). N = 5.
3.2 Semi-field experiments
3.2.1 Olfactory bioassays
In 2019, fruit treated with Actigard repelled D. suzukii flies, while fruit treated with Regalia was attractive to flies at 1 DAT (Figure 2B). All other treatments were not significant (P > 0.05).
In 2022, at 1 DAT and 7 DAT, fruit treated with Regalia were again more attractive to flies than control fruit (Figures 5A,C). At 3 DAT, nearly three times more flies chose the control fruits than the OxiDate-treated fruits (Figure 5B). At 7 DAT, 40% more flies also chose the control fruits over Actigard-treated fruits (Figure 5C). At 10 DAT, more than twice as many flies chose control fruits than LifeGard-treated fruits (Figure 5D). All other treatment combinations were not significant (P > 0.05) (Figure 5).
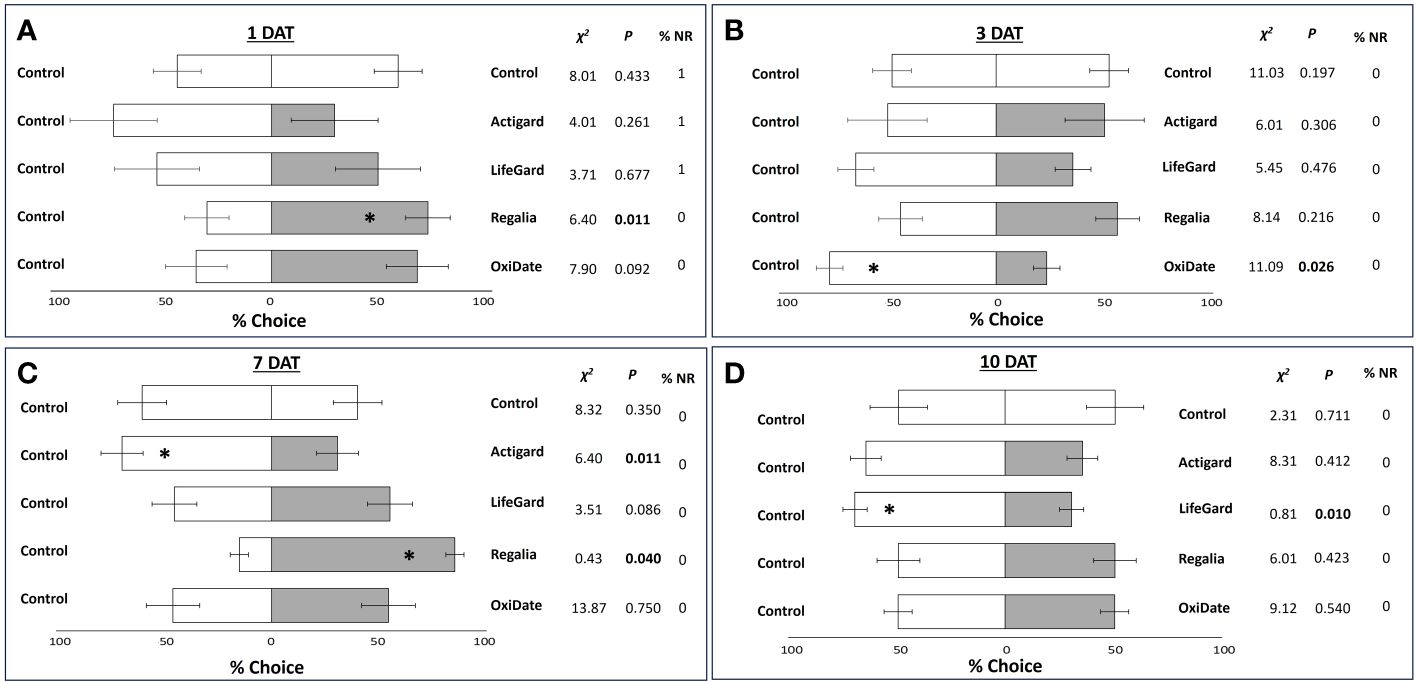
Figure 5 Results from 2022 olfactory tests. Mean percentage (± SE) of Drosophila suzukii flies that made a choice between treated and untreated fruit at 1 (A), 3 (B), 7 (C), and 10 (D) days after treatment (DAT). Significant P-values according to Chi-square tests are indicated in bold and by an asterisk (P ≤ 0.05). % NR = percent of non-respondents. N = 5.
3.2.2 Oviposition bioassays
No-choice tests. In 2019 oviposition no-choice assays, all elicitor and sterilant treatments reduced the number of eggs laid (F = 288.45, df = 5, P < 0.001) and the number of adults that emerged from fruits (F = 14.68, df = 5, P = 0.023) compared to untreated (control) fruit at 1 DAT (Figures 3C, D). However, the SA-related elicitors (Actigard, LifeGard, and Regalia) and the sterilant OxiDate had a stronger negative effect on D. suzukii oviposition and adult emergence than the JA-related elicitor Blush (Figures 3C, D).
In 2022 oviposition no-choice assays, at 1 DAT, females laid 66% fewer eggs in Actigard-treated fruits than in untreated fruits (F = 70.04, df = 4, P < 0.001) (Figure 6A), resulting in 69% fewer emerged adults (F = 68.68, df = 4, P < 0.001) (Figure 6E). At 3 DAT, there were no significant effects of the elicitors and sterilant on D. suzukii oviposition or adult emergence (Ps > 0.05) (Figures 6B, F). However, at 7 DAT, females laid 64% fewer number of eggs (F = 137.87, df = 4, P < 0.001), and fewer number of adults emerged (F = 82.76, df = 4, P < 0.001), in fruits treated with Oxidate than those treated with LifeGard (Figures 6C, G). There were no significant effects of treatments on D. suzukii oviposition or adult emergence at 10 DAT (Ps > 0.05) (Figures 6D, H).
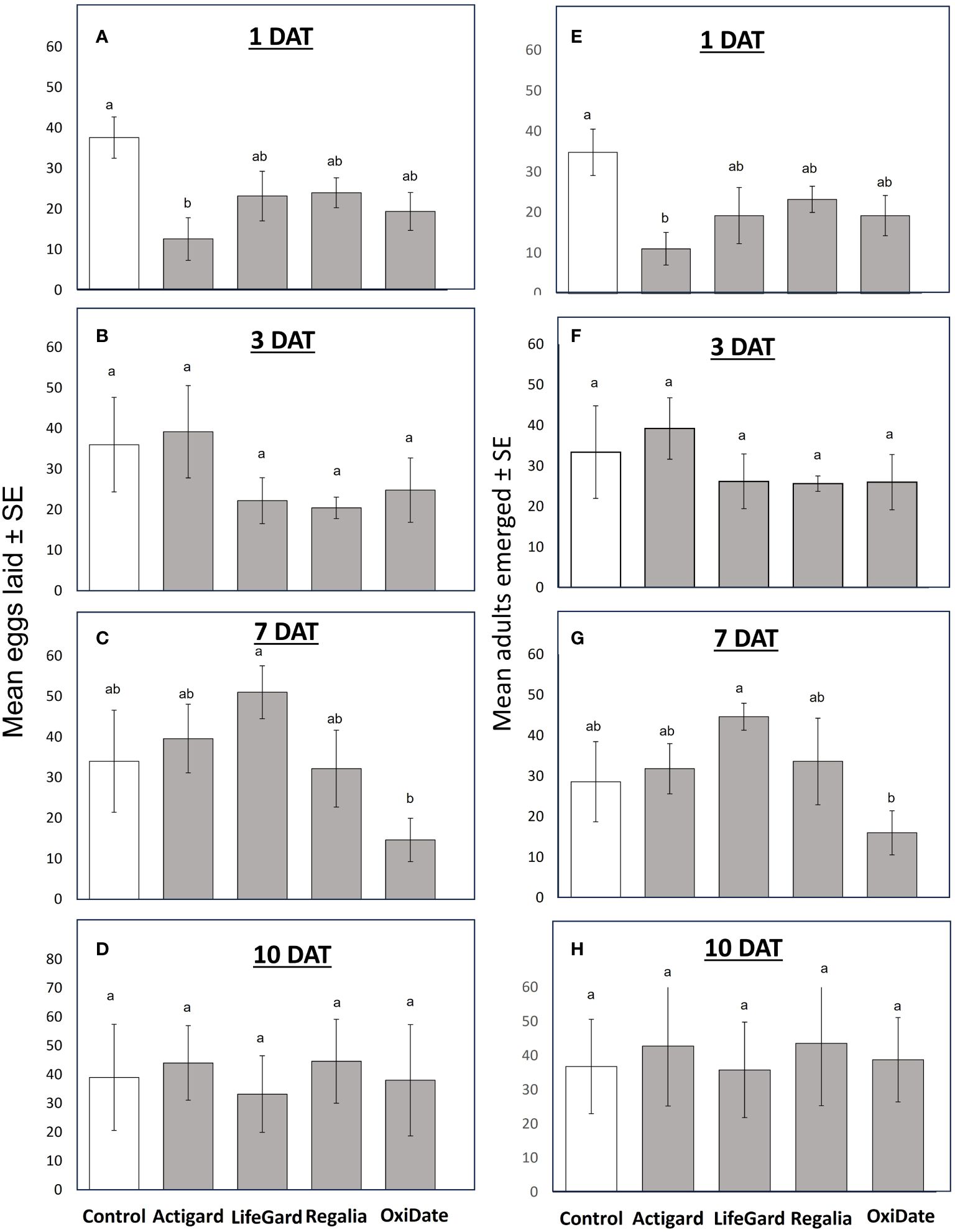
Figure 6 Results from 2022 no-choice oviposition tests. Mean (± SE) number of Drosophila suzukii eggs laid in fruit at 1 (A), 3 (B), 7 (C), and 10 (D) days after treatment (DAT). Mean (± SE) number of D. suzukii adults that emerged from fruit at 1 (E), 3 (F), 7 (G), and 10 (H) DAT. Different letters indicate significant differences among treatments according to Bonferroni tests (P ≤ 0.05). N = 5.
Choice tests. In 2019, when given a choice, D. suzukii females laid fewer eggs in fruit treated with Actigard (t = 3.45, df = 18, P = 0.003), LifeGard (t = 2.89, df = 18, P = 0.010), Regalia (t = 2.21, df = 18, P = 0.040), and OxiDate (t = 2.63, df = 18, P = 0.017) compared to the control, whereas Blush had no effect (t = 0.55, df = 18, P = 0.588) (Figure 4C). Also, significantly fewer number of adults emerged from fruit treated with Actigard (t = 0.179, df = 18, P = 0.001), LifeGard (t = 4.15, df = 18, P = 0.001), and OxiDate (t = 354, df = 18, P = 0.002) compared to the untreated diet, while Regalia and Blush had no effects (Ps > 0.05) (Figure 4D).
In 2022 oviposition paired-choice assays, there was no effect of elicitors or sterilant on number of eggs laid by D. suzukii females in fruits (Figures 7A–D) or the number of adults that emerged from fruits (Figures 7E–H) at any of the time intervals (all P values > 0.05).
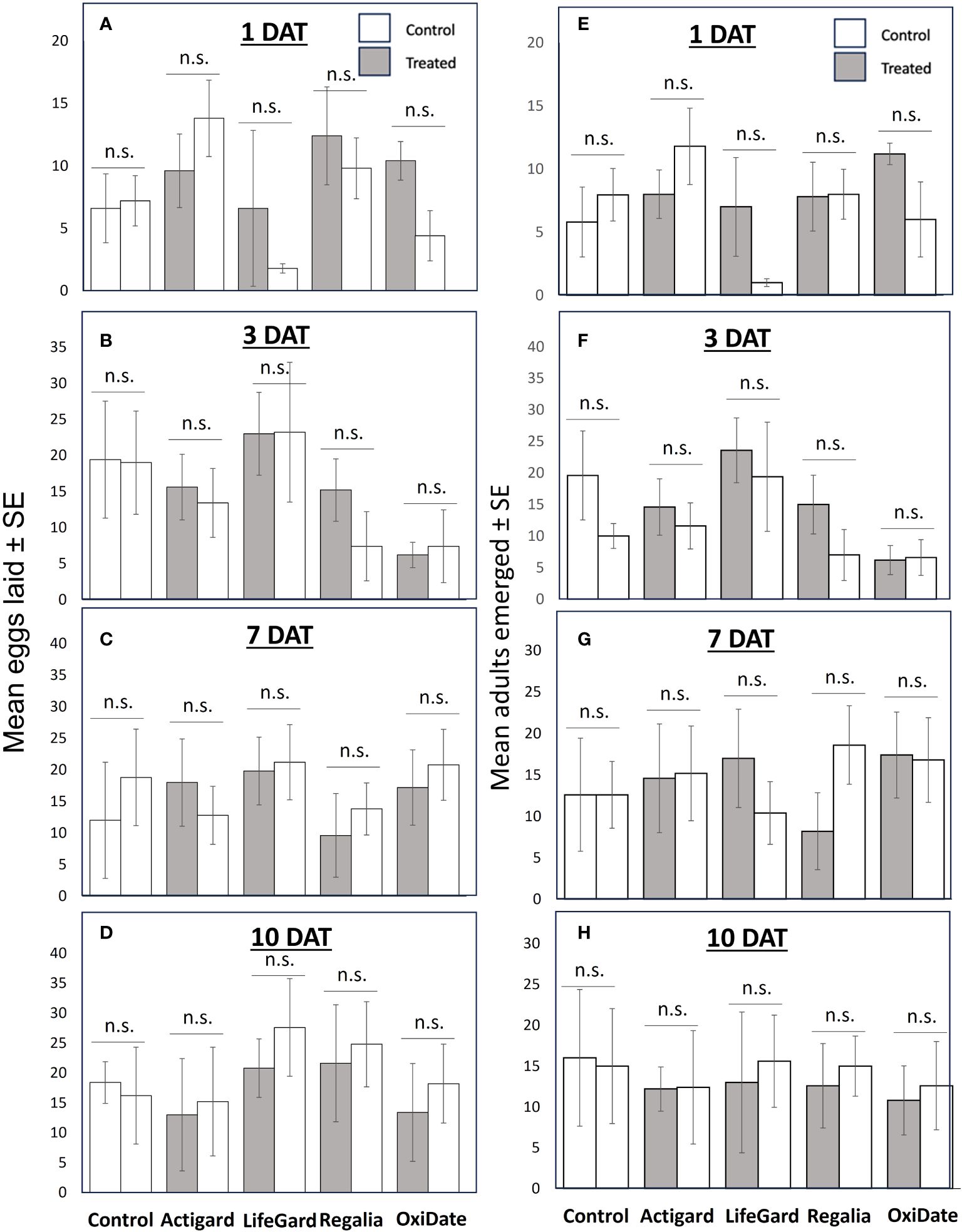
Figure 7 Results from 2022 choice oviposition tests. Mean (± SE) number of Drosophila suzukii eggs laid in fruit at 1 (A), 3 (B), 7 (C), and 10 (D) days after treatment (DAT). Mean (± SE) number of D. suzukii adults that emerged from fruit at 1 (E), 3 (F), 7 (G), and 10 (H) DAT. An asterisk indicates a significant difference between treatments according to t-tests (P ≤ 0.05). n.s. = not significant (P > 0.05). N = 5.
3.3 Field experiments
There was a significant effect of ‘position’ at 3 DAT (F = 828.45, df = 1, P < 0.001), 7 DAT (F = 903.82, df = 1, P < 0.001), and 10 DAT (F = 748.75, df = 1, P < 0.001) (Figure 8), with significantly more eggs laid in fruits at the bottom than the top of the bushes. However, there was a significant ‘treatment’ × ‘position’ interaction at 3 DAT (F = 22.67, df = 4, P < 0.001), where D. suzukii females laid fewer eggs in Actigard-treated fruits collected from the top of the bushes than in control fruits collected from the same position (P = 0.030; Figure 8A).
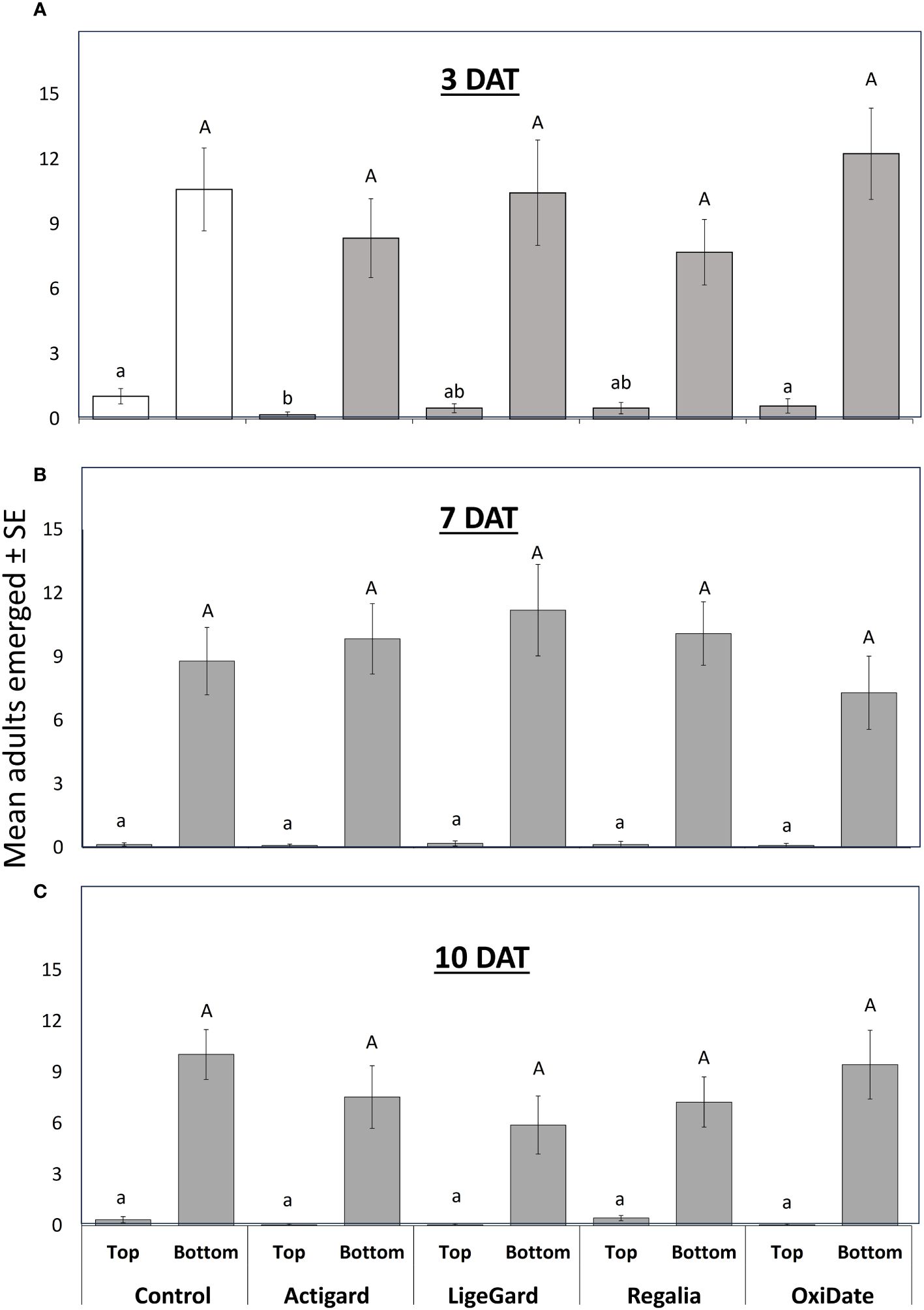
Figure 8 Results from field cage study. Mean (± SE) number of Drosophila suzukii adults that emerged from fruits at 3 (A), 7 (B), and 10 (C) days after treatment (DAT). Fruits were collected from the bottom or top of bushes. Different lowercase and uppercase letters indicate significant differences among treatments in the number of flies that emerged from fruits collected from the top and bottom of bushes, respectively, according to Bonferroni tests (P ≤ 0.05). N = 4.
4 Discussion
In this study, four elicitors and a crop sterilant were assessed as potential behavior-modifying products for D. suzukii management under the hypothesis that they would induce changes in the fruit, either directly or indirectly by influencing associated microbes, making them less attractive to this pest. However, our findings showed inconsistent repellency or oviposition deterrent effects of these products, posing challenges in drawing definitive conclusions regarding their long-lasting effectiveness under field conditions. While Actigard reduced D. suzukii oviposition in berries at 1 DAT in both no-choice and choice tests, its effect was short-lived; thus, it would necessitate multiple sprays within a week to make it effective, which may be impractical as a control option. In some cases, flies were even more attracted to the treated berries. For instance, although the specific mechanism(s) remain unknown, Regalia-treated berries were more attractive to the flies at 1 DAT and 7 DAT, and more flies chose LifeGard-treated berries at 10 DAT. Furthermore, none of the tested products showed significant larval toxicity effects, as evidenced by the similar correlation between egg counts and adult emergence across most assays. This suggests that these products, while not consistently, could potentially influence adult behaviors such as attraction and oviposition under certain circumstances.
While the elicitors and sterilant treatments used in our study did not consistently produce the expected repellent or oviposition deterrent effects on D. suzukii, products similar to those employed here effectively enhanced plant resistance against other insect pests. For example, various studies have demonstrated that application of SA induces defenses in plants, leading to reduced feeding (Stella de Freitas et al., 2019; Wei et al., 2021) and oviposition (Shahabinejad et al., 2014) by insect herbivores. However, most of these studies focused on folivorous insects, unlike our study, which examined a frugivore. An exception is the study by Damodaram et al. (2015), where the application of acibenzolar-S-methyl, the active ingredient in Actigard, to mangoes resulted in increased levels of phenols and flavonoids in the fruits. This, in turn, led to reduced oviposition, larval performance, and adult emergence of the oriental fruit fly Bactrocera dorsalis (Hendel). Recently, Hussain et al. (2023) also reported an increase in the levels of phenols, tannin, and flavonoid content and a decrease in the number of eggs laid by D. suzukii females in wine grapes treated with JA and SA. In addition, they found that SA-treated diet reduced D. suzukii oviposition in a dose-dependent manner. Among all the elicitor and sterilant treatments we employed, the SA-related Actigard demonstrated in some assays repellency and oviposition deterrent effects on D. suzukii when applied to blueberry fruits; however, the underlying mechanism(s) behind these effects remain unexplored. Like the findings by Hussain et al. (2023), Actigard also exhibited a reduction in egg laying in choice tests when applied to the diet. Thus, it remains unclear to what extent the observed effects of Actigard on D. suzukii resulted from directly influencing adult behavior or indirectly through alterations in fruit chemistry.
Van Timmeren et al. (2020) conducted studies using the crop sterilant Jet-Ag, whose active ingredients are the same as OxiDate, and found that it does not reduce D. suzukii oviposition in treated berries; however, it reduced the number of larvae infesting the berries. When directly applied to H. uvarum colonies in the laboratory, the sterilant inhibited yeast growth in a dose-dependent manner (Van Timmeren et al., 2020). In our study, the crop sterilant OxiDate did not elicit strong aversion from the flies or reduce fruit infestation. It is possible that, under field conditions, applying a crop sterilant to blueberries does not provide sufficient coverage to inhibit H. uvarum growth. Van Timmeren et al. (2020) also noted that Jet-Ag breaks down quickly under field conditions, resulting in limited residual activity. Moreover, recent research by Schöneberg et al. (2024) revealed that, under high pest pressure, Jet-Ag does not offer adequate standalone control against D. suzukii under field conditions, even when it occasionally reduced fruit yeast levels. Given these current findings, incorporating elicitors of plant defenses or crop sterilants as a standalone control option for D. suzukii may be challenging.
Our earlier studies revealed that Blush, an elicitor of JA-related defenses, has either no or minimal activity on D. suzukii and was consequently excluded as a treatment in subsequent studies. Initially, we included Blush based on findings from prior research suggesting that applications of JA could be effective for crop protection against insect herbivores (Uefune et al., 2014; Wei et al., 2021). However, these earlier studies primarily focused on foliar feeders, leaving the effects of JA and related elicitors of the JA pathway on frugivores unclear. Several studies have shown that applications of JA increase volatile emissions in plants that attract natural enemies and thus could enhance biological control of crop pests (Lou et al., 2005; Bruinsma et al., 2009). Consequently, applications of JA elicitors may have positive impacts on natural enemies of D. suzukii, such as the larval parasitoids Ganaspis brasiliensis (Ihering) and Leptopilina japonica (Novković and Kimura) (Wang et al., 2018), by enhancing their attraction to infested berries. It is also possible that SA and JA elicitors might reduce D. suzukii larval developmental times, a parameter not measured in our study, rendering them more susceptible to parasitism as stated by the ‘slow-growth high-mortality’ hypothesis (Feeny, 1976; Benrey and Denno, 1997). Indeed, reductions in development times were observed in stinkbug, Oebalus pugnax (F.), nymphs that fed on SA-induced plants (Stella de Freitas et al., 2019) and larvae of the fall armyworm, Spodoptera frugiperda (J. E. Smith), that fed on JA-induced plants (Gordy et al., 2015). Future studies examining elicitor treatments within the context of tri-trophic interactions could yield valuable insights for enhancing biological control efforts against D. suzukii.
Numerous abiotic and biotic factors, including environment, plant nutrition, genotype, and baseline resistance levels, can influence induced resistance in plants (Walters and Fountaine, 2009). Consequently, achieving efficacy in utilizing plant defense elicitors and crop sterilants in the field can be challenging. In our field cage experiments, nearly all D. suzukii adults emerged from fruits collected from the lower parts of the bushes. This observation aligns with previously reported D. suzukii adult behaviors, which prefer to target berries closer to the ground and within the interior of bushes, benefiting from cooler temperatures and higher humidity (Rice et al., 2017). It is conceivable that certain areas of the bushes were not thoroughly sprayed, potentially hindering the induction of a strong defense response. Weather conditions could have also influenced our field results. Notably, rain was experienced on the morning of the third day after treatment for replicates 1 and 2, which could have diminished the residual activity of the treatments. Future experiments should also consider the timing of the elicitor/sterilant treatments. In our study, non-infested berries were treated only once and when they were already ripe. Investigating whether it might be more effective to treat them before ripening and continuously thereafter is warranted. For instance, exposure to the elicitors or sterilants before ripening could potentially induce a more rapid increase in defenses after oviposition or larval feeding, a phenomenon referred to as ‘priming’ (Goellner and Conrath, 2008). It is also possible that these products could have ovicidal or larvicidal effects on D. suzukii post-infestation, as evidenced by the curative activity of some insecticides (Wise et al., 2015).
5 Conclusion
While this study did not find strong evidence supporting the use of the tested elicitors of the SA and JA defense pathways or crop sterilants as effective long-lasting behavior modifiers for D. suzukii in blueberries, these or similar products may still find utility in managing this pest for other purposes. For instance, although we cannot recommend them as standalone tools in a D. suzukii management program, future research could explore alternative applications, such as enhancing biological control or incorporating them into trap cropping or push-pull systems. Furthermore, it is plausible that elicitors may show greater efficacy against D. suzukii in other crop systems. Host-plant resistance remains a relatively unexplored tool for D. suzukii management, and multiple interacting factors can influence how a plant responds to elicitors of defenses or crop sterilants. With further refinement through future studies, there is potential to integrate induced host-plant resistance into D. suzukii management programs, offering a safer and more sustainable approach to protect crops against this invasive pest.
Data availability statement
The raw data supporting the conclusions of this article will be made available by the authors, without undue reservation.
Ethics statement
The manuscript presents research on animals that do not require ethical approval for their study.
Author contributions
AQ: Conceptualization, Data curation, Formal Analysis, Methodology, Writing – original draft. PU-B: Conceptualization, Data curation, Formal Analysis, Methodology, Writing – review & editing. RH: Methodology, Writing – review & editing. CR-S: Conceptualization, Funding acquisition, Resources, Supervision, Validation, Writing – review & editing.
Funding
The author(s) declare financial support was received for the research, authorship, and/or publication of this article. This study was funded by a USDA Sustainable Agriculture Research and Education (SARE) grant (LNE22-455R), a USDA Specialty Crops Research Initiative (Award # 2020-51181-32140), a USDA Organic Agriculture Research and Extension Initiative (Award # 2018-51300-28434), The Sridhar Polavarapu Memorial Endowment for Vaccinium Research, and the Hatch project No. NJ08550.
Acknowledgments
The authors thank Beth Ferguson, Stephanie Aponte, Jennifer Frake, Sophie Olsen, and other members of the Entomology Lab. at the Rutgers P.E. Marucci Center for providing valuable assistance with laboratory and field experiments and with the mass rearing of D. suzukii. We also thank Yahel Ben-Zvi for helpful comments on an earlier version of the manuscript and the various agrochemical companies that kindly contributed samples of the products used in the experiments.
Conflict of interest
The authors declare that the research was conducted in the absence of any commercial or financial relationships that could be construed as a potential conflict of interest.
The author(s) declared that they were an editorial board member of Frontiers, at the time of submission. This had no impact on the peer review process and the final decision
Publisher’s note
All claims expressed in this article are solely those of the authors and do not necessarily represent those of their affiliated organizations, or those of the publisher, the editors and the reviewers. Any product that may be evaluated in this article, or claim that may be made by its manufacturer, is not guaranteed or endorsed by the publisher.
References
Agarbati A., Canonico L., Ciani M., Comitini F. (2019). The impact of fungicide treatments on yeast biota of Verdicchio and Montepulciano grape varieties. PloS One 14, e0217385. doi: 10.1371/journal.pone.0217385
Beckers G. J., Spoel S. H. (2006). Fine-tuning plant defence signalling: salicylate versus jasmonate. Plant Biol. 8, 1–10. doi: 10.1055/s-2005-872705
Bektas Y., Eulgem T. (2015). Synthetic plant defense elicitors. Front. Plant Sci. 5. doi: 10.3389/fpls.2014.00804
Benhamou N., Bélanger R. R. (1998). Induction of systemic resistance to Pythium damping-off in cucumber plants by benzothiadiazole: ultrastructure and cytochemistry of the host response. Plant J. 14, 13–21. doi: 10.1046/j.1365-313X.1998.00088.x
Benrey B., Denno R. F. (1997). The slow-growth–high-mortality hypothesis: a test using the cabbage butterfly. Ecology 78, 987–999. doi: 10.1890/0012-9658(1997)078[0987:TSGHMH]2.0.CO;2
Brisset M.-N., Cesbron S., Thomson S., Paulin J.-P. (2000). Acibenzolar-S-methyl induces the accumulation of defense-related enzymes in apple and protects from fire blight. Euro J. Plant Pathol. 106, 529–536. doi: 10.1023/A:1008728119087
Bruinsma M., Posthumus M. A., Mumm R., Mueller M. J., van Loon J. J. A., Dicke M. (2009). Jasmonic acid-induced volatiles of Brassica oleracea attract parasitoids: effects of time and dose, and comparison with induction by herbivores. J. Exp. Bot. 60, 2575–2587. doi: 10.1093/jxb/erp101
Cloonan K. R., Abraham J., Angeli S., Syed Z., Rodriguez-Saona C. (2018). Advances in the chemical ecology of the spotted wing drosophila (Drosophila suzukii) and its applications. J. Chem. Ecol. 44, 922–939. doi: 10.1007/s10886-018-1000-y
Cooper W. R., Horton D. R. (2015). ). Effects of elicitors of host plant defenses on pear psylla, Cacopsylla pyricola. Entomol Exp. Appl. 157, 300–306. doi: 10.1111/eea.12360
Cooper W. R., Horton D. R. (2017). Elicitors of host plant defenses partially suppress Cacopsylla pyricola (Hemiptera: Psyllidae) populations under field conditions. J. Insect Sci. 17, 49. doi: 10.1093/jisesa/iex020
Correa R. S., Moraes J. C., Auad A. M., Carvalho G. A. (2005). Silicon and acibenzolar-S-methyl as resistance inducers in cucumber, against the whitefly Bemisia tabaci (Gennadius) (Hemiptera: Aleyrodidae) biotype B. Neotrop Entomol 34, 429–433. doi: 10.1590/S1519-566X2005000300011
Damodaram K. J. P., Aurade R. M., Kempraj V., Roy T. K., Shivashankara K. S., Verghese A. (2015). Salicylic acid induces changes in mango fruit that affect oviposition behavior and development of the oriental fruit fly, Bactrocera dorsalis. PloS One 10, e0139124. doi: 10.1371/journal.pone.0139124
Diepenbrock L. M., Rosensteel D. O., Hardin J. A., Sial A. A., Burrack H. J. (2016). Season-long programs for control of Drosophila suzukii in southeastern U.S. blueberries. Crop Prot 81, 76–84. doi: 10.1016/j.cropro.2015.12.012
Divekar P. A., Narayana S., Divekar B. A., Kumar R., Gadratagi B. G., Ray A., et al. (2022). Plant secondary metabolites as defense tools against herbivores for sustainable crop protection. Int. J. Mol. Sci. 23, 2690. doi: 10.3390/ijms23052690
Feeny P. (1976). “Plant apparency and chemical defense,” in Biochemical interaction between plants and insects. Eds. Wallace J. W., Mansell R. L. (Springer, Boston, MA), 1–40. doi: 10.1007/978-1-4684-2646-5_1
Feng Y., Bruton R., Park A., Zhang A. (2018). Identification of attractive blend for spotted wing drosophila, Drosophila suzukii, from apple juice. J. Pest Sci. 91, 1251–1267. doi: 10.1007/s10340-018-1006-9
Glazebrook J. (2005). Contrasting mechanisms of defense against biotrophic and necrotrophic pathogens. Annu. Rev. Phytopathol. 43, 205–227. doi: 10.1146/annurev.phyto.43.040204.135923
Goellner K., Conrath U. (2008). Priming: it’s all the world to induced disease resistance. Eur. J. Plant Pathol. 121, 233–242. doi: 10.1007/s10658-007-9251-4
Gordy J. W., Leonard B. R., Blouin D., Davis J. A., Stout M. J. (2015). Comparative effectiveness of potential elicitors of plant resistance against Spodoptera frugiperda (J. E. Smith) (Lepidoptera: Noctuidae) in four crop plants. PloS One 10, e0136689. doi: 10.1371/journal.pone.0136689
Hamby K. A., Becher P. G. (2016). Current knowledge of interactions between Drosophila suzukii and microbes, and their potential utility for pest management. J. Pest Sci. 89, 621–630. doi: 10.1007/s10340-016-0768-1
Hamby K. A., Hernández A., Boundy-Mills K., Zalom F. G. (2012). Associations of yeasts with spotted-wing drosophila (Drosophila suzukii; Diptera: Drosophilidae) in cherries and Raspberries. Appl. Environ. Microbiol. 78, 4869–4873. doi: 10.1128/AEM.00841-12
Hussain B., War A. R., Pfeiffer D. G. (2023). Jasmonic acid and salicylic acid induced defensive response in wine grapes against Drosophila suzukii (Diptera: Drosophilidae). Heliyon 9, e16505. doi: 10.1016/j.heliyon.2023.e16505
Jaramillo S. L., Mehlferber E., Moore P. J. (2015). Life-history trade-offs under different larval diets in Drosophila suzukii (Diptera: Drosophilidae). Physiol. Entomol 40, 2–9. doi: 10.1111/phen.12082
Karban R., Baldwin I. T. (1997). Induced responses to herbivory (Chicago, IL: University of Chicago Press), 330. doi: 10.7208/chicago/9780226424972.001.0001
Karban R., Myers J. H. (1989). Induced plant responses to herbivory. Annu. Rev. Ecol. Syst. 20, 331–348. doi: 10.1146/annurev.es.20.110189.001555
Keesey I. W., Knaden M., Hansson B. S. (2015). Olfactory specialization in Drosophila suzukii supports an ecological shift in host preference from rotten to fresh fruit. J. Chem. Ecol. 41, 121–128. doi: 10.1007/s10886-015-0544-3
Lee J. C., Dreves A. J., Cave A. M., Kawai S., Isaacs R., Miller J. C., et al. (2015). Infestation of wild and ornamental noncrop fruits by Drosophila suzukii (Diptera: Drosophilidae). Ann. Entomol Soc. Am. 108, 117–129. doi: 10.1093/aesa/sau014
Lee J. C., Wang X., Daane K. M., Hoelmer K. A., Isaacs R., Sial A. A., et al. (2019). Biological control of spotted-wing drosophila (Diptera: Drosophilidae) current and pending tactics. J. Integr. Pest Manag 10, 13. doi: 10.1093/jipm/pmz012
Levin D. A. (1976). The chemical defenses of plants to pathogens and herbivores. Ann. Rev. Ecol. Syst. 7, 121–159. doi: 10.1146/annurev.es.07.110176.001005
Lou Y.-G., Du M.-H., Turlings T. C. J., Cheng J.-A., Shan W.-F. (2005). Exogenous application of jasmonic acid induces volatile emissions in rice and enhances parasitism of Nilaparvata lugens eggs by the parasitoid Anagrus nilaparvatae. J. Chem. Ecol. 31, 1985–2002. doi: 10.1007/s10886-005-6072-9
Louws F. J., Wilson M., Campbell H. L., Cuppels D. A., Jones J. B., Shoemaker P. B., et al. (2001). Field control of bacterial spot and bacterial speck of tomato using a plant activator. Plant Dis. 85, 481–488. doi: 10.1094/PDIS.2001.85.5.481
Lyon G. (2007). “Agents that can elicit induced resistance,” in Induced resistance for plant defence: A sustainable approach to crop protection. Eds. Walters D., Newton A., Lyon G. (Blackwell Publishing, Oxford, UK), 11–40. doi: 10.1002/9781118371848.ch2
Meena M., Yadav G., Sonigra P., Nagda A., Mehta T., Zehra A., et al. (2022). “Role of microbial bioagents as elicitors in plant defense regulation,” in Transcription factors for biotic stress tolerance in plants. Eds. Wani S. H., Nataraj V., Singh G. P. (Springer, Cham, Switzerland), 103–128. doi: 10.1007/978-3-031-12990-2_6
Mithöfer A., Boland W. (2012). Plant defense against herbivores: chemical aspects. Annu. Rev. Plant Biol. 63, 431–450. doi: 10.1146/annurev-arplant-042110-103854
Moreno-Pérez A., Martínez-Ferri E., Pliego-Alfaro F., Pliego C. (2020). Elicitors and plant defence. JOJ Hortic. Arboric. 2, 555600. doi: 10.19080/JOJHA.2020.02.555600
Pagadala Damodaram K. J., Aurade R. M., Kempraj V., Roy T. K., Shivashankara K. S., Verghese A. (2015). Salicylic acid induces changes in mango fruit that affect oviposition behavior and development of the Oriental fruit fly, Bactrocera dorsalis. PloS One 10, e0139124. doi: 10.1371/journal.pone.0139124
Pickett J. A., Aradottír G. I., Birkett M. A., Bruce T. J. A., Hooper A. M., Midega C. A. O., et al. (2014). Delivering sustainable crop protection systems via the seed: exploiting natural constitutive and inducible defence pathways. Philos. Trans. R Soc. Lond B Biol. Sci. 369, 20120281. doi: 10.1098/rstb.2014.0053
Rehermann G., Spitaler U., Sahle K., Cossu C. S., Donne L. D., Bianchi F., et al. (2022). Behavioral manipulation of Drosophila suzukii for pest control: high attraction to yeast enhances insecticide efficacy when applied on leaves. Pest Manag Sci. 78, 896–904. doi: 10.1002/ps.6699
Reignault P., Walters D. (2007). “Topical application of inducers for disease control,” in Induced resistance for plant defence: A sustainable approach to crop protection. Eds. Walters D., Newton A., Lyon G. (Blackwell Publishing, Oxford, UK), 179–200. doi: 10.1002/9780470995983.ch10
Revadi S., Lebreton S., Witzgall P., Anfora G., Dekker T., Becher P. G. (2015). Sexual behavior of Drosophila suzukii. Insects. 6, 183–196. doi: 10.3390/insects6010183
Rhoades D. F., Cates R. G. (1976). “Toward a general theory of plant antiherbivore chemistry,” in Biochemical interaction between plants and insects, recent advances in phytochemistry. Eds. Wallace J. W., Mansell R. L. (Springer US, Boston, MA), 168–213. doi: 10.1007/978-1-4684-2646-5_4
Rice K. B., Jones S. K., Morrison W., Leskey T. C. (2017). Spotted wing drosophila prefer low hanging fruit: insights into foraging behavior and management strategies. J. Insect Behav. 30, 645–661. doi: 10.1007/s10905-017-9646-9
Ruiz-García Y., Gómez-Plaza E. (2013). Elicitors: a tool for improving fruit phenolic content. Agriculture 3, 33–52. doi: 10.3390/agriculture3010033
Salifu R., Chen C., Sam F. E., Jiang Y. (2022). Application of elicitors in grapevine defense: impact on volatile compounds. Horticulturae 8, 451. doi: 10.3390/horticulturae8050451
Schöneberg T., Tewey M., Hamby K. A. (2024). Peroxyacetic acid and hydrogen peroxide crop sanitizer efficacy against fungi and Drosophila suzukii in blackberries. Crop Prot 175, 106458. doi: 10.1016/j.cropro.2023.106458
Shahabinejad M., Shojaaddini M., Maserti B., Arvin S. M. J., Seyedi S. M. (2014). Exogenous application of methyl jasmonate and salicylic acid increases antioxidant activity in the leaves of pistachio (Pistacia vera L. cv. Fandoughi) trees and reduces the performance of the phloem-feeding psyllid Agonoscena pistaciae. Arthropod-Plant Interact. 8, 525–530. doi: 10.1007/s11829-014-9334-z
Smith J. L., De Moraes C. M., Mescher M. C. (2009). Jasmonate- and salicylate-mediated plant defense responses to insect herbivores, pathogens and parasitic plants. Pest Manag Sci. 65, 497–503. doi: 10.1002/ps.1714
Sobhy I. S., Mandour N. S., Sarhan A. A. (2015). Tomato treatment with chemical inducers reduces the performance of Spodoptera littoralis (Lepidoptera: Noctuidae). Appl. Entomol Zool 50, 175–182. doi: 10.1007/s13355-014-0319-2
Stella de Freitas T. F., Stout M. J., Sant’Ana J. (2019). Effects of exogenous methyl jasmonate and salicylic acid on rice resistance to Oebalus pugnax. Pest Manag Sci. 75, 744–752. doi: 10.1002/ps.5174
Stout M. J., Zehnder G. W., Baur M. E. (2002). Potential for the use of elicitors of plant resistance in arthropod management programs. Arch. Insect Biochem. Physiol. 51, 222–235. doi: 10.1002/arch.10066
Su H. (2012). Regalia® bioprotectant in plant disease management. Outlooks Pest Manag 23, 30–34. doi: 10.1564/23feb09
Szymanski S., Longley R., Hatlen R. J., Heger L., Sharma N., Bonito G., et al. (2023). The blueberry fruit mycobiome varies by tissue type and fungicide treatment. Phytobiomes J. 7, 208–219. doi: 10.1094/PBIOMES-04-22-0028-FI
Tait G., Mermer S., Stockton D., Lee J., Avosani S., Abrieux A., et al. (2021). Drosophila suzukii (Diptera: Drosophilidae): A decade of research towards a sustainable integrated pest management program. J. Econ Entomol 114, 1950–1974. doi: 10.1093/jee/toab158
Thomma B. P. H. J., Eggermont K., Penninckx I. A. M. A., Mauch-Mani B., Vogelsang R., Cammue B. P. A., et al. (1998). Separate jasmonate-dependent and salicylate-dependent defense-response pathways in Arabidopsis are essential for resistance to distinct microbial pathogens. Proc. Natl. Aca Sci. U.S.A. 95, 15107–15111. doi: 10.1073/pnas.95.25.15107
Uefune M., Ozawa R., Takabayashi J. (2014). Prohydrojasmon treatment of lima bean plants reduces the performance of two-spotted spider mites and induces volatiles. J. Plant Interact. 9, 69–73. doi: 10.1080/17429145.2012.763146
Urbaneja-Bernat P., Cloonan K., Zhang A., Salazar-Mendoza P., Rodriguez-Saona C. (2021). Fruit volatiles mediate differential attraction of Drosophila suzukii to wild and cultivated blueberries. J. Pest Sci. 94, 1249–1263. doi: 10.1007/s10340-021-01332-z
Van Timmeren S., Fanning P. D., Schöneberg T., Hamby K., Lee J., Isaacs R. (2020). Exploring the efficacy and mechanisms of a crop sterilant for reducing infestation by spotted-wing drosophila (Diptera: Drosophilidae). J. Econ Entomol 113, 288–298. doi: 10.1093/jee/toz245
Vimala R. V., Suriachandraselvan M. (2008). Induced resistance in bhendi against powdery mildew by foliar application of salicylic acid. J. Biopesticides 2, 111–114.
Walling L. L. (2000). The myriad plant responses to herbivores. J. Plant Growth Regul. 19, 195–216. doi: 10.1007/s003440000026
Walsh D. B., Bolda M. P., Goodhue R. E., Dreves A. J., Lee J., Bruck D. J., et al. (2011). Drosophila suzukii (Diptera: Drosophilidae): Invasive pest of ripening soft fruit expanding its geographic range and damage potential. J. Integr. Pest Manag 2, G1–G7. doi: 10.1603/IPM10010
Walters D. R., Fountaine J. M. (2009). Practical application of induced resistance to plant diseases: an appraisal of effectiveness under field conditions. J. Agric. Sci. 147, 523–535. doi: 10.1017/S0021859609008806
Wang X.-G., Nance A. H., Jones J. M. L., Hoelmer K. A., Daane K. M. (2018). Aspects of the biology and reproductive strategy of two Asian larval parasitoids evaluated for classical biological control of Drosophila suzukii. Biol. Control 121, 58–65. doi: 10.1016/j.biocontrol.2018.02.010
War A. R., Paulraj M. G., Ahmad T., Buhroo A. A., Hussain B., Ignacimuthu S., et al. (2012). Mechanisms of plant defense against insect herbivores. Plant Signal Behav. 7, 1306–1320. doi: 10.4161/psb.21663
War A. R., Paulraj M. G., War M. Y., Ignacimuthu S. (2011). Role of salicylic acid in induction of plant defense system in chickpea (Cicer arietinum L.). Plant Signal Behav. 6, 1787–1792. doi: 10.4161/psb.6.11.17685
Wei X., Vrieling K., Kim H. K., Mulder P. P. J., Klinkhamer P. G. L. (2021). Application of methyl jasmonate and salicylic acid lead to contrasting effects on the plant’s metabolome and herbivory. Plant Sci. 303, 110784. doi: 10.1016/j.plantsci.2020.110784
Wise J. C., Vanderpoppen R., Vandervoort C., O’Donnell C., Isaacs R. (2015). Curative activity contributes to control of spotted-wing drosophila (Diptera: Drosophilidae) and blueberry maggot (Diptera: Tephritidae) in highbush blueberry. Can. Entomol 147, 109–117. doi: 10.4039/tce.2014.36
Keywords: Drosophila suzukii, invasive, highbush blueberry, crop sterilant, pest management
Citation: Quadrel A, Urbaneja-Bernat P, Holdcraft R and Rodriguez-Saona C (2024) Elicitors of plant defenses as a standalone tactic failed to provide sufficient protection to fruits against spotted-wing drosophila. Front. Agron. 6:1381342. doi: 10.3389/fagro.2024.1381342
Received: 03 February 2024; Accepted: 17 April 2024;
Published: 30 April 2024.
Edited by:
Rachid Lahlali, Ecole Nationale d’Agriculture de Meknès, MoroccoReviewed by:
Vincenzo Trotta, Università degli Studi della Basilicata, ItalyYasir Iftikhar, University of Sargodha, Pakistan
Copyright © 2024 Quadrel, Urbaneja-Bernat, Holdcraft and Rodriguez-Saona. This is an open-access article distributed under the terms of the Creative Commons Attribution License (CC BY). The use, distribution or reproduction in other forums is permitted, provided the original author(s) and the copyright owner(s) are credited and that the original publication in this journal is cited, in accordance with accepted academic practice. No use, distribution or reproduction is permitted which does not comply with these terms.
*Correspondence: Cesar Rodriguez-Saona, crodriguez@njaes.rutgers.edu