Tracking nest-rescued green sea turtles in oceanic currents sheds light on eastern Caribbean “lost years”
- 1US Department of the Interior, Bureau of Ocean Energy Management, Washington, DC, United States
- 2School for the Environment, University of Massachusetts, Boston, MA, United States
- 3Oceans Forward, Walnut, CA, United States
- 4Dominica Sea Turtle Conservation Organization, Morne Daniel, Dominica
- 5Blue World Research Institute, Cocoa, FL, United States
Although sea turtles are well-studied charismatic marine species, little is known about the juvenile stage, especially the oceanic phase between hatching on their natal beaches and their adult lives. To collect data on these “lost years,” six juvenile green turtles were equipped with satellite tags, released from southwestern Dominica in the eastern Caribbean, and tracked for up to 3 months. Mapping turtle movement to ocean currents reveals that juvenile green turtles spent most of their travel time within the Caribbean Sea, passively riding the prevailing currents before actively directing themselves toward distant coastal waters. Half of the tagged turtles traveled to coastal foraging grounds off of Colombia and Venezuela, while one traveled north past Puerto Rico. These findings provide novel data for the movements of juvenile green turtles hatched in the Eastern Caribbean region.
1 Introduction
While sea turtles represent some of the most popular charismatic megafauna species, their populations are in rapid decline due to the combination of late age maturity (Goshe et al., 2010; Scott et al., 2011) and exposure to harmful anthropogenic activities. Ashford et al. (2022) identified the greatest threats to sea turtles in their oceanic environment in the Caribbean as light pollution, fishing, and ship traffic. Similarly, Fuentes et al. (2023) reviewed the key threats to sea turtles and listed them as climate change, direct take, disease, pollution, predation, coastal and marine development, and fisheries. Although many nations, especially in the Caribbean, support a legal hunting season for sea turtles, illegal poaching for meat, shells, and eggs continues in most countries outside of the mandated hunting season or legal limit (Senko et al., 2022). Additionally, incidental bycatch of turtles in artisanal and commercial dredge, netting, and longlining fisheries can result in mortalities either immediately or post-release (Finkbeiner et al., 2011). Although these anthropogenic threats can be anticipated, it is difficult to fully assess their impact without sea turtle population size estimates and studies into the spatial overlap of sea turtles with activities of a threatening nature .
Many studies have attempted to quantify sea turtle abundance by extrapolating population size from the clutch size of nesting females (Witherington et al., 2009; Mazaris et al., 2017). However, this may provide an overestimation of sea turtle population size (Casale and Ceriani, 2020), which could be problematic for a category of fauna that has almost all species listed as endangered, critically endangered, or vulnerable and decreasing in number (Abreu-Grobois and Plotkin, 2008; Mortimer and Donnelly, 2008; Wallace et al., 2013; Casale and Tucker, 2017; Wibbels and Bevan, 2019; Seminoff, 2023). Studies focusing on clutch size to estimate sea turtle abundance do not take into account the highly migratory nature of post-hatching oceanic juveniles, knowledge of which can aid in more accurate estimates of population size (Vandeperre et al., 2019). It is also essential to understand migratory behavior and movement patterns of juvenile turtles to recommend best management practices for the oceanic areas sea turtles inhabit (Allen and Singh, 2016).
For several sea turtle species including the green sea turtle Chelonia mydas, the juvenile stage is divided into two distinct ontogenetic stages – the oceanic juvenile phase followed by the neritic juvenile phase (Bolten, 2003). During the oceanic stage, juvenile green turtles display an omnivorous diet relying on available macroalgae, neuston, and plankton within their pelagic environment (Bolten, 2003; Arthur et al., 2008; Witherington et al., 2012). Ontogenetic cues for shifting to the neritic juvenile stage remain largely unknown and vary according to geographic area, but generally transition when turtles reach 20-40 cm in carapace length (Zug and Glor, 1998; Reich et al., 2007; Meylan et al., 2011; Howell et al., 2016; Burgett et al., 2018). Stable isotope studies have confirmed the shift from oceanic to neritic habitats by documenting a change in diet from largely algal and animal matter to predominantly (and in some cases, exclusively) seagrasses (Reich et al., 2007; Arthur et al., 2008; Howell et al., 2016; Burgett et al., 2018). However, some studies indicate that the definitive shift in diet and strict zonal habitat may only apply to certain regions such as eastern Australia and the Greater Caribbean (Cardona et al., 2009, 2010; Carman et al., 2012).
There are lim>Cardonaited studies performed in the Greater Caribbean, defined as the waters south of central South Carolina and north of northern Guyana (33°N to 7°N, respectively) including but not limited to the Gulf of Mexico, Caribbean Sea, and continental shelf waters (Robertson and Cramer, 2014), on oceanic stage juvenile green turtles (Witherington et al., 2012; Putman and Mansfield, 2015; Mansfield et al., 2021). Several studies have outfitted larger neritic juvenile green turtles with satellite tags to determine site fidelity and movement patterns post-release (Godley et al., 2003; Hart and Fujisaki, 2010; Shaver et al., 2013; Chambault et al., 2018; Metz et al., 2020), while only a handful of studies have similarly tracked the smaller oceanic stage juveniles (Putman and Mansfield, 2015; Mansfield et al., 2021). Barbour et al. (2023) tracked captive-reared juvenile green turtles released from the Cayman Islands which represented various age and size classes and demonstrated both neritic and oceanic stage movements. Some of the main reasons why there are so few juvenile turtle tagging studies, in the oceanic stage, include turtles achieving a minimum size to carry the weight of the tag without negatively affecting the animal (Mansfield et al., 2012), locating oceanic juvenile turtles within their natural environment (Wildermann et al., 2018), and collecting individuals uninfluenced by anthropogenic activities which may provide behavioral data contrary to unimpacted individuals.
While migratory data of oceanic juvenile turtles is scarce, there are theories as to their distribution methods and motives. It was once assumed that oceanographic currents are largely responsible for the movement of oceanic juvenile turtles through passive migration (Carr, 1987). However, more recent studies have revealed that oceanic juvenile turtles may play a more active role in their migration by swimming against currents to achieve desired foraging areas (Putman and Mansfield, 2015; Mansfield et al., 2021; Barbour et al., 2023).
It is vital to the protection and survival of sea turtle species to understand the movement patterns of the post-hatchling oceanic juvenile stage and how these may be impacted by anthropogenic activities. This knowledge is necessary to implement best conservation management practices to protect the most vulnerable life stage of sea turtle species. Only about 25% of sea turtle tagging studies have focused on juveniles from both neritic and oceanic stages (Hazen et al., 2012; Jeffers and Godley, 2016), which indicates a major data gap in our understanding of juvenile turtle movement. Additionally, although the Caribbean seems to be a hotspot for sea turtle research (Jeffers and Godley, 2016), no targeted studies on the sea turtle populations native to Dominica have been published to date. Active sea turtle monitoring in Dominica has occurred since 2004. The Dominica Sea Turtle Conservation Organization (DomSeTCO) is responsible for monitoring beaches and flipper tagging nesting females as well as monitoring for nest emergences such as predation by feral dogs or being vulnerable to high surf. Since 2004, fifty uniquely tagged nesting female green turtles have been identified on Dominica beaches, five of which were recaptures. The present study aims to add to the understanding of oceanic juvenile turtle movement by providing satellite tagging data from juvenile green sea turtles (C. mydas). We tagged juveniles that were rescued from Dominica nests post-hatching after failing to transition to their oceanic habitat, reared in captivity, and then released into Dominica offshore waters.
2 Methods
Six C. mydas hatchlings, which did not successfully make the transition from their nests to the open ocean, were collected from two nest sites on Dominica’s east coast and brought to the Dominica Sea Turtle Conservation Organization’s Conservation Research Laboratory for rearing in December 2021. Dorsal and facially lateral photographs were taken of each turtle to identify unique scute patterns (Schofield et al., 2008; Gatto et al., 2018) and uploaded to the global Internet of Turtles database for future recapture analysis (Dunbar et al., 2021).
Two of the hatchlings came from a nest laid on Sept. 28, 2021, and excavated on Dec. 4, 2021. The other four hatchlings came from a nest laid on Oct. 18, 2021, that was relocated due to the natural nest being in an area vulnerable to high surf. The natural emergence of this nest occurred on December 18, 2021. Only six hatchlings were collected because that was the maximum housing capacity of the DomSeTCO Conservation Research Laboratory.
Turtles were housed in seawater tanks equipped with Biosystems System 5000 Marine recirculating filtration system, an Electroheat ECO-V heat pump, and Titan UV sterilizers to ensure optimal living conditions by maintaining stable temperatures and sterilized seawater. Water quality parameters were measured and recorded daily with average water temperatures of 81 ± 1°F, pH of 8.0 ± 0.1, salinity of 32.6 ± 3.8 ppt, nitrite of 0.5 ± 0.5 mg/L, and nitrate of 18.7 ± 18.6 mg/L.
To maintain optimal nutrition, turtles were fed a mixture of Mazuri herbivore sea turtle gel, crabs, shrimp, assorted green leaf lettuces, and fresh plankton three to five times daily (Innis et al., 2017). To track growth, each turtle was weighed and measured weekly by the same researcher. Once turtles reached a curved carapace length (CCL) of at least 14 cm and weighed at least 300 g, typically around 20-22 weeks old, they were considered ready for release because they were large enough to bear the weight of affixed satellite tags with minimal energetic costs (Murray and Fuller, 2000). To track each juvenile turtle’s movements post-release, PTT-100 solar-powered satellite transmitter tags (Microwave Telemetry) weighing approximately 9.5 g were affixed to the turtle’s carapace with a flexible acrylic-silicone adhesive after lightly scouring the vertebral scutes with sandpaper to remove any biofouling (Mansfield et al., 2021). Following the guidelines of Mansfield et al. (2021) and Murray and Fuller (2000), tags weighed an average of 1.7 ± 0.7% (range: 1.0 to 3.0%) of the juvenile turtle’s body weight. Tags were programmed to connect with ARGOS satellites and locations were computed using Kalman filtering which increases the accuracy of reported GPS locations (Lopez and Malarde, 2014).
Turtles were released offshore in Soufriere Bay off the southwest coast of Dominica on May 5, 2022, in the same approximate location per the example of previously tagged juvenile turtle releases in various other studies (Mansfield et al., 2012, 2021; Christiansen et al., 2016). The release location was chosen due to its accessibility by boat and lower wave energy, being in a sheltered area. The latitude and longitude coordinates for each release point were recorded. Transmitter data were filtered based on the accuracy of transmission using ARGOS location codes (LC) 3-0, A and B (CLS America, 2007).
Since ARGOS estimated error radii of projected coordinates are less robust than desired (Costa et al., 2009; Hazel, 2009), more conservative error buffers derived from the average 68th percentile error radii found in Costa et al.’s (2009) and Hazel’s (2009) studies were applied to coordinates that manifested on land. Centroid coordinates of the portion of the error radius that was in water were used as the adjusted track locations for these points. Turtle locations were also tested to ensure that speeds between any two points fell within reason for the species and age group. Following Godley et al.’s (2003) methods, any locations that demonstrated the turtle’s speed exceeded 5 km/h were excised. Points that occurred within fifteen minutes of each other that also exhibited erroneous speeds were averaged within their given error radii to present the most accurate possible locations.
Current vector data was collected from Earth and Space Research’s Ocean Surface Current Analyses Real-time (OSCAR) project. Watson’s tests were performed on turtle and corresponding current vectors using six-hour interval positions (first transmission point following 0:00, 6:00, 12:00, and 18:00) to determine if the turtles’ orientations were significantly different from the prevailing currents (Zar, 1999). Results that demonstrated non-significance (p>0.05) indicated that the turtles were passively transported by the prevailing current.
Turtle tracks were also analyzed against composite floating algal density maps made available by the University of Southern Florida College of Marine Science Optical Oceanography Lab and derived from the methods of Wang and Hu (2016).
3 Results
Initial turtle tip-to-tail lengths ranged from 9.0 to 9.6 cm (average = 9.5 ± 0.2 cm) and weights ranged from 24.0 to 28.0 g (average = 25.8 ± 1.5 g). Growth rates of the juvenile turtles averaged 29.6 ± 9.7 g/wk (range: -5.0 to 123.0 g/wk) and 0.8 ± 0.1 cm/wk (range: 0.0 to 2.4 cm/wk) with both weight and length displaying strong growth (Figure 1). Final weights and tip-to-tail upon release at 20 and 22 weeks old ranged from 315.0 to 938.0 g (average = 611.2 ± 205.8 g) and 19.7 to 27.5 cm (average = 24.4 ± 2.6 cm), respectively (Table 1). Curved carapace lengths upon release ranged from 14.1 to 21.3 cm (average = 18.0 ± 2.6 cm) (Table 1).
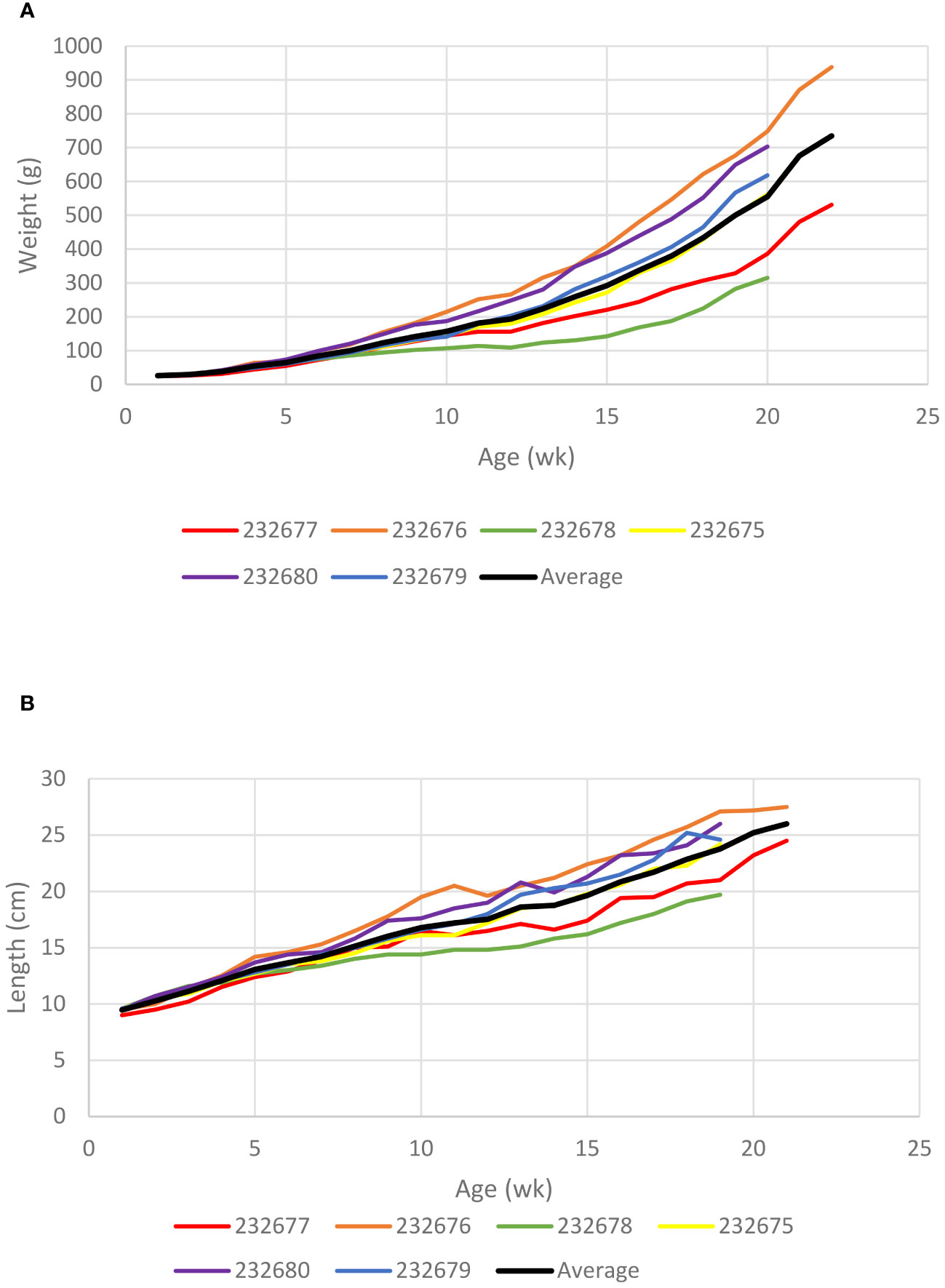
Figure 1 Growth rates of six Chelonia mydas juveniles raised in captivity post-hatching. (A) Weight gain in grams of each turtle plus the average gain of all turtles. (B) Growth rate in total (tip-to-tail) length of each turtle plus the average growth of all turtles.
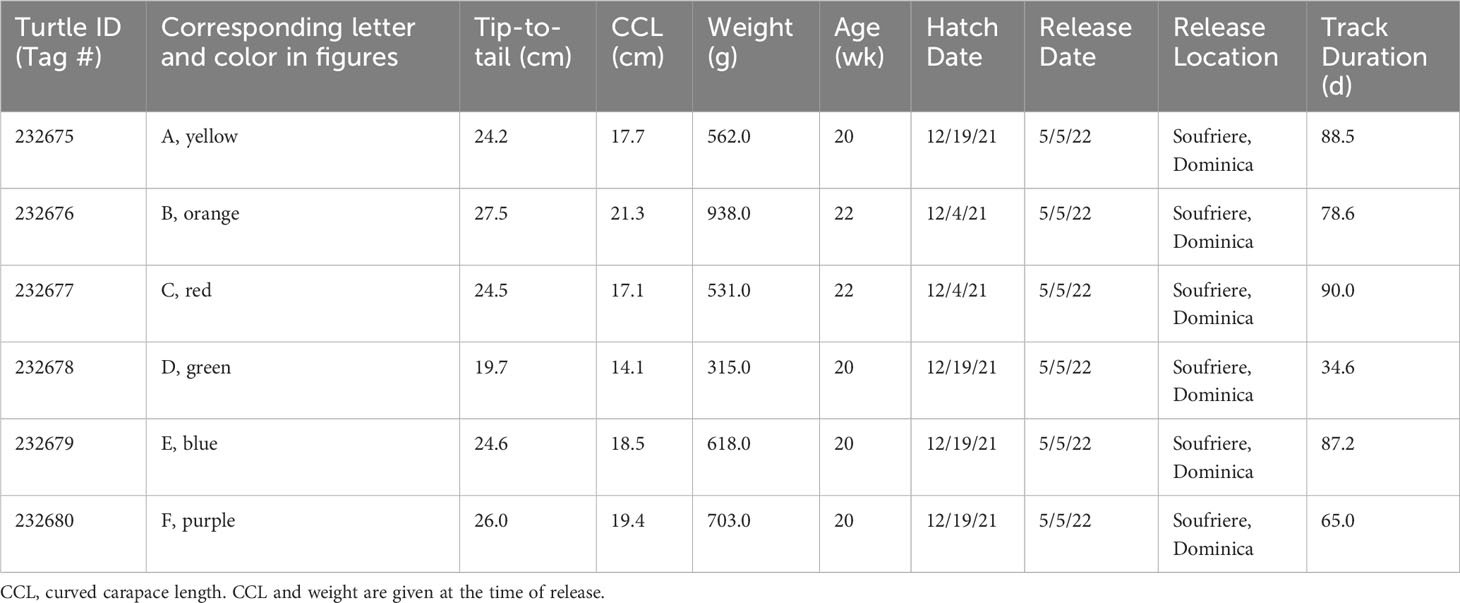
Table 1 Physical measurements of satellite tracked juvenile Chelonia mydas upon release and their subsequent tracking durations.
Turtles were tracked between 34.6 and 90.0 days (average = 74.0 ± 21.4 days). During the tracking window, turtles traveled between 1202.0 and 2749.0 km (average = 2128.3 ± 540.5 km) (Table 2). The farthest west any one turtle traveled was approximately 120 km off the coast of Barranquilla, Colombia; the farthest north was 160 km north of the British Virgin Isles; and the farthest south was in the Gulf of Venezuela off the coast of Maracaibo (Figure 2). No turtles traveled east of Dominica.
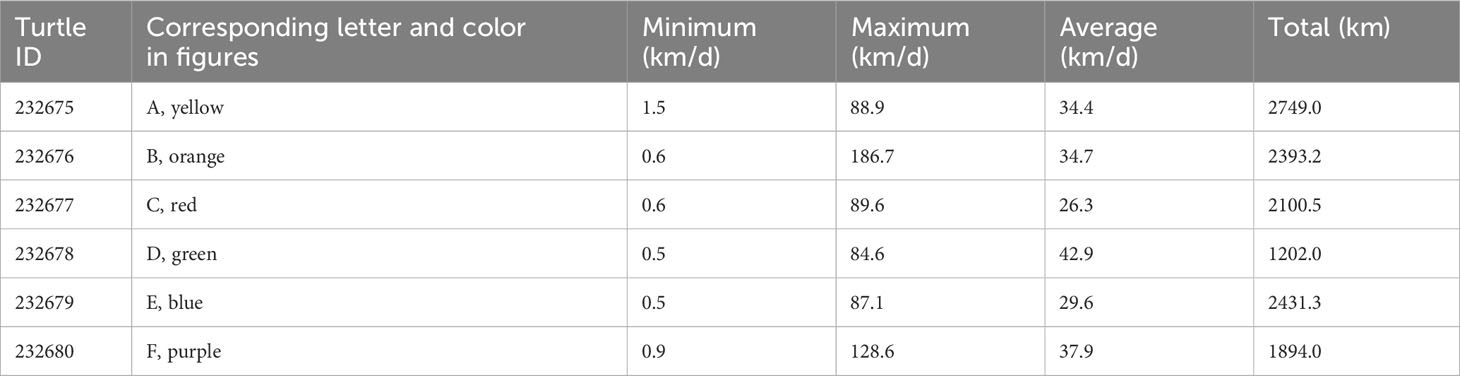
Table 2 Summarization of daily movements of six satellite-tagged juvenile green turtles released from Dominica.
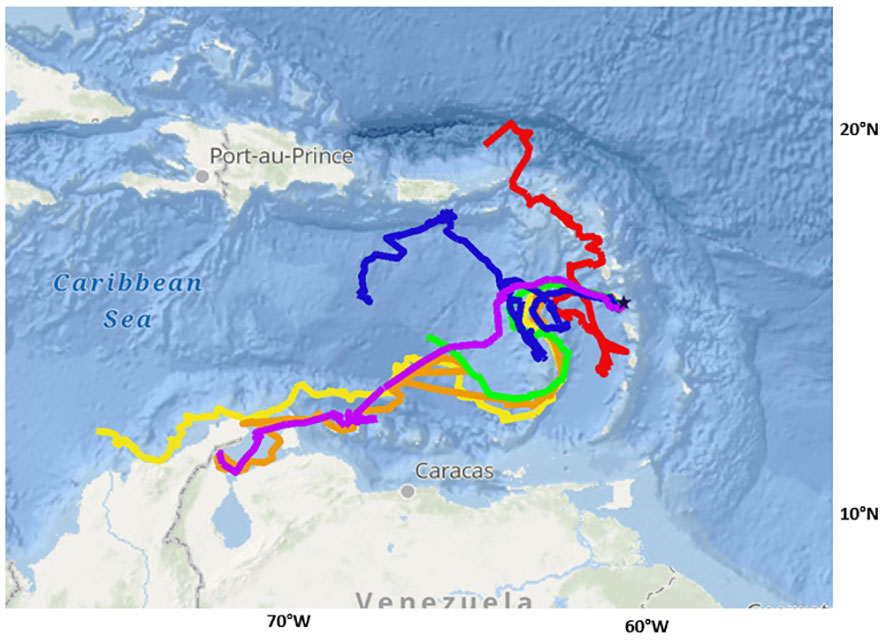
Figure 2 Tracked locations of six satellite-tagged juvenile green turtles released from Dominica (star point). Each track represents a unique turtle with track durations ranging from 39.6 to 90.0 days. Turtle IDs: yellow = 232675, orange =232676, red = 232677, green = 232678, blue = 232679, purple = 232680.
ARGOS satellites reported 3000 total unique location coordinates for the six tagged turtles with 95.8% of points portraying the approximate location of the animals in the water. Of the remaining points, 3.5% were projected on land and were moved within their error radii to an aquatic location, and 0.7% did not project within the water even within their error radii or were grossly inaccurate according to the region in which the turtles were present, and were removed.
OSCAR current vector data could not be provided close to coastal areas. As a result, only certain portions of the turtles’ tracks could be statistically analyzed to determine whether the turtles traveled with the prevailing current (Table 3). All turtles displayed no significant difference in direction from the current at varying points in their tracking for an average among all turtles of 32.2 ± 13.6 days (range: 24.1 to 57.3 days). Overall, the average percentage of time spent following the prevailing current among all turtles for the tracking period in which analysis was possible was 74 ± 24% (range: 34 to 100%).
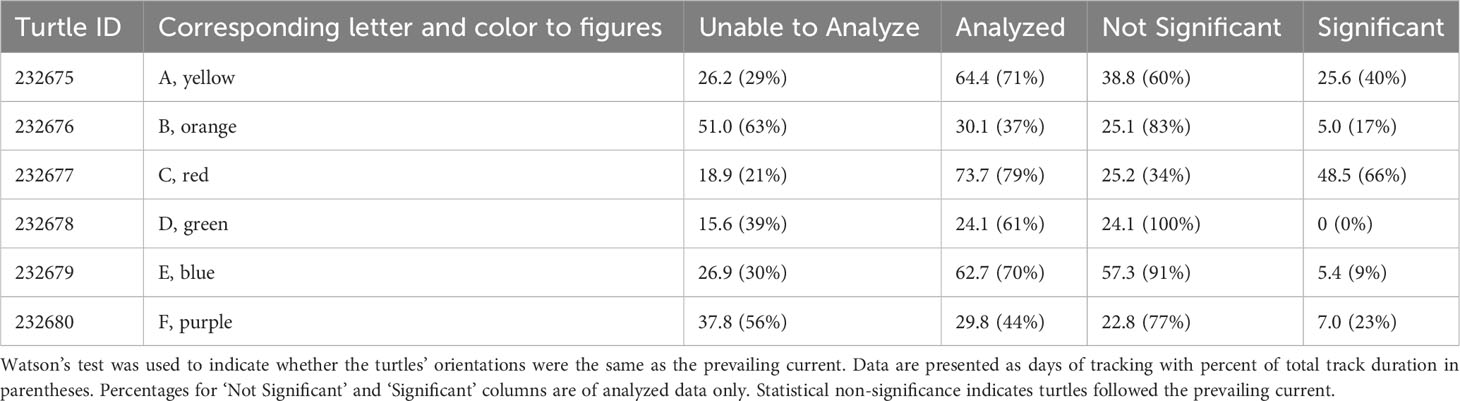
Table 3 Proportion of juvenile green turtle satellite tracking data suitable for statistical analysis.
The tagged turtles spent most of their time in Venezuelan and Dominican waters, although their varying routes took them through different countries’ exclusive economic zones (Figure 3). Twenty-two total national waters were represented in the turtles’ tracks. The turtles did not cross into international waters during the tracking period.
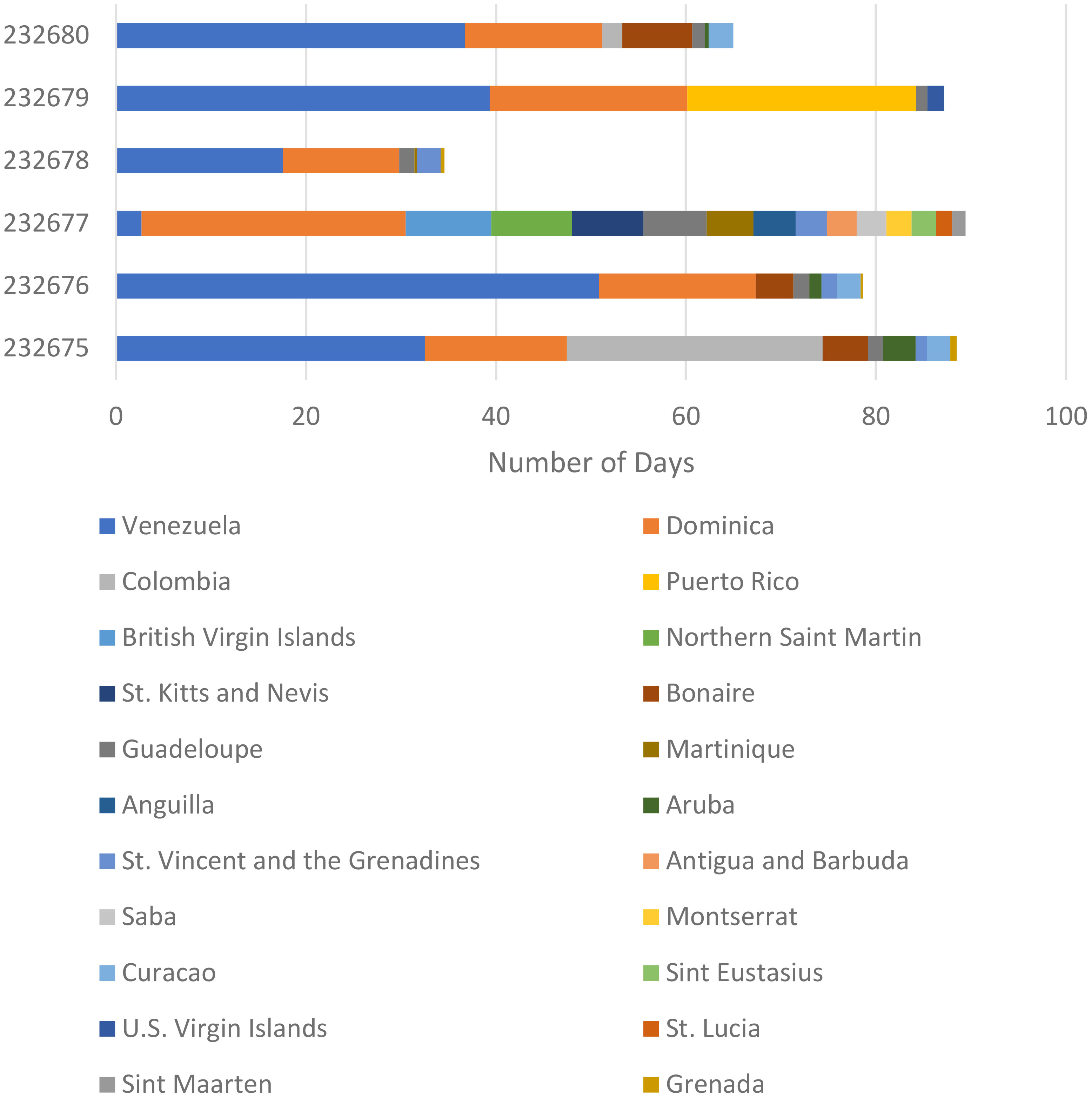
Figure 3 Days six satellite-tagged juvenile green turtles spent in varying Caribbean and South American nations’ exclusive economic zones.
Tag locations indicate that five out of the six turtles spent the majority of their time in the oceanic zone (>200 m depth) while turtle 232676 split its time almost evenly between the oceanic and neritic (<200 m depth) zones (Figure 4). The average of all turtles’ tracks showed 27.5% time spent in the neritic zone (range: 8.4 to 52.8%) and 72.5% time spent in the oceanic zone (47.2 to 91.6%).
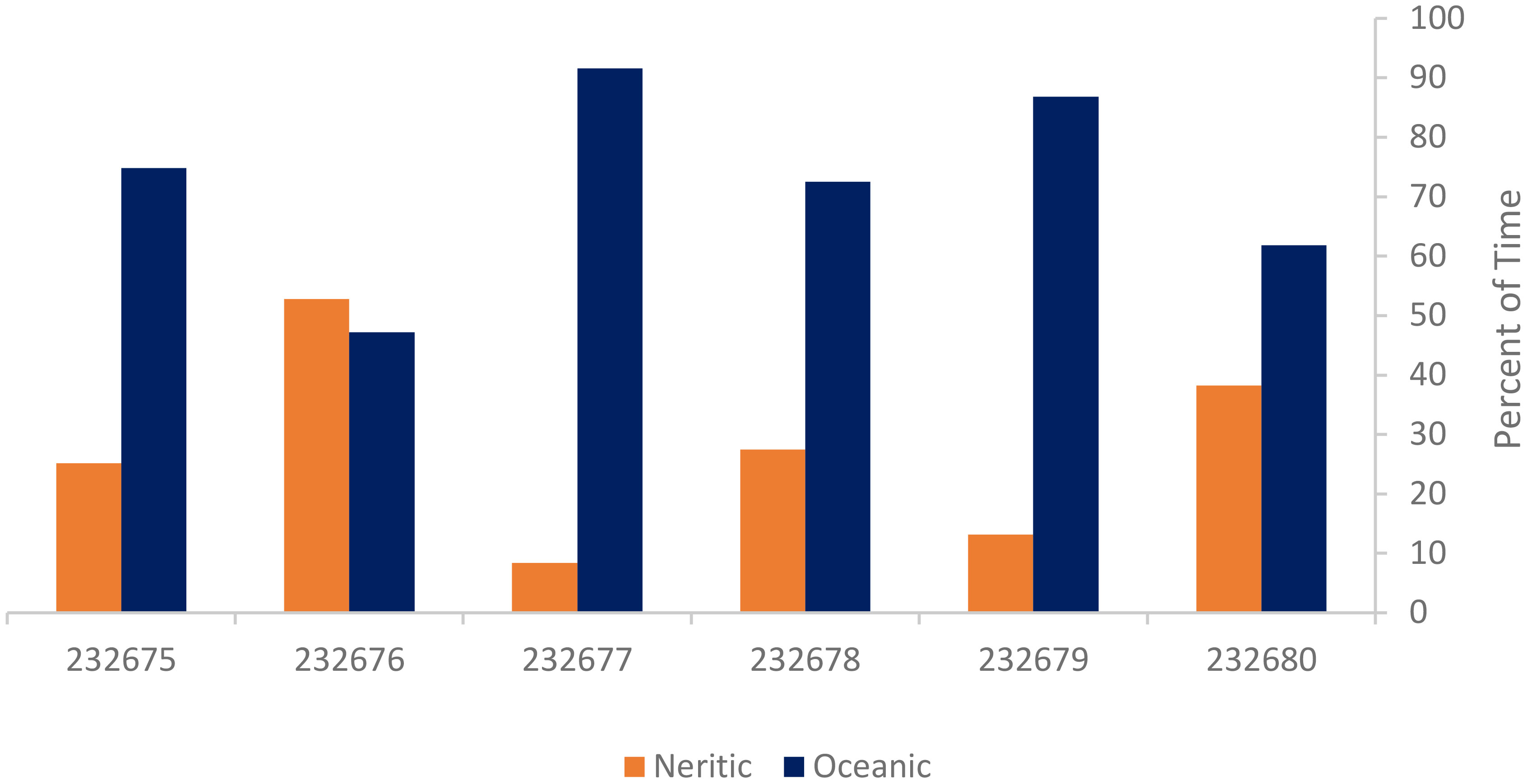
Figure 4 Percent of time six satellite-tagged juvenile green turtles spent in the oceanic and neritic zones during tracking durations.
Turtles 232675, 232676, and 232680 ended their tracks around the northern coasts of Colombia and Venezuela (Figures 5A, B, F). Most of their tracks indicate passive movement with the prevailing current until they near the islands of Bonaire and Curacao where they display a distinct heading away from the current toward the northern coast of South America (Figure 5; Table 4). Turtle 232678 also followed the eddies and Caribbean Current, a similar trajectory to the previously mentioned turtles, however, the track ceased to transmit after forty days (Figure 5D; Table 4).
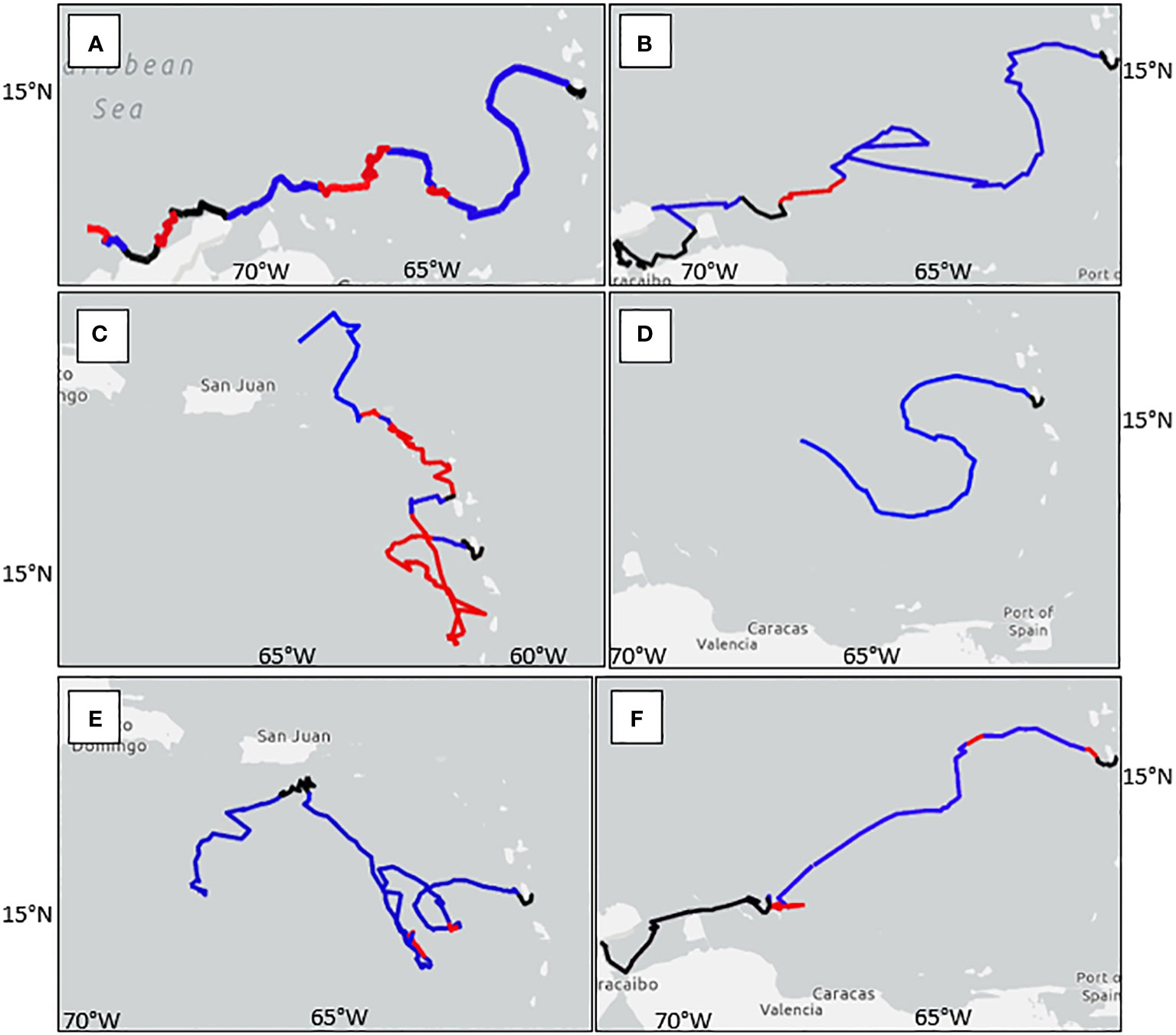
Figure 5 Tracked locations of six satellite-tagged juvenile green sea turtles released from Dominica (star point). Letters correspond with individual turtles: (A) 232675, (B) 232676, (C) 232677, (D) 232678, (E) 232679, (F) 232680. Blue tracks indicate passive movement with the current, red tracks indicate active movement contrary to the current, black tracks indicate insufficient current data.
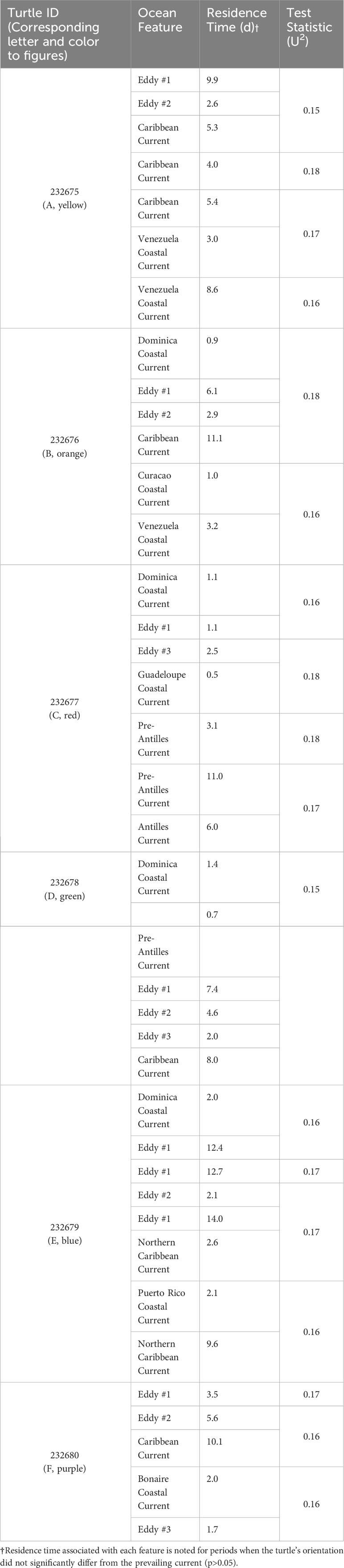
Table 4 Associated oceanic features within which juvenile green turtles resided during their tracking durations.
Turtle 232679 took a different route, following the eddies for the most part with brief breaks from the prevailing current to cease an eastward track and head north to join up with the current again (Figure 5E). This turtle headed north towards the southern coast of Puerto Rico before making its journey southward again in the direction of the northern coast of South America (Table 4).
Turtle 232677 had an altogether unique course compared to the other tagged turtles. It proceeded south toward St. Lucia before turning north, leeward of the Lesser Antilles, within 2-88 km of the coast of the islands (Figure 5C). It periodically followed the current but mostly determined its own orientation until it was due east of the U. S. Virgin Islands and passively moved with the Antilles Current on an apparent heading for the western coast of British Virgin Isles or eastern coast of Puerto Rico (Table 4).
For comparison, if the turtles had engaged in a completely passive transport from the time of release until the tags ceased transmitting, the prevailing currents most likely would have carried them westward across the Caribbean Sea only to travel northward into the Gulf of Mexico (Figure 6).
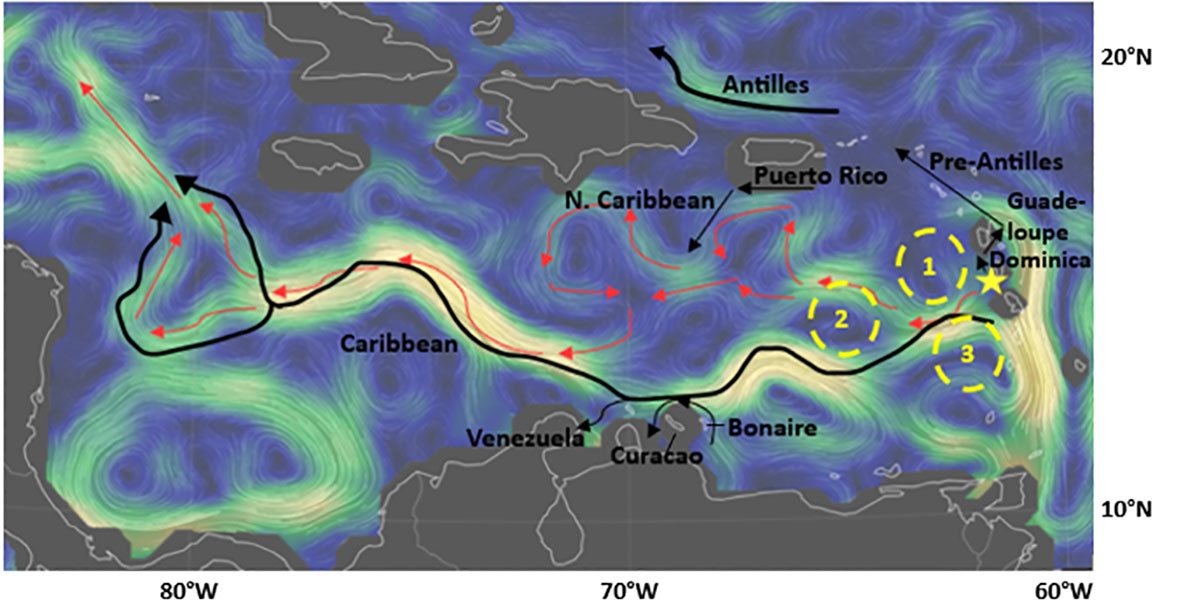
Figure 6 Prevailing oceanic currents from May 2022 when the satellite tagged juvenile green sea turtles were released into the wild. The star indicates the release location. The red arrows follow the most likely trajectory of the turtles’ migration under exclusively passive transport. The black arrows indicate currents, the yellow dashed circles indicate eddies. Data provided by OSCAR; map credit: C. Beccario, earth.nullschool.net.
Four of the turtles’ (232675, 232676, 232678, 232680) tracks revealed minimal association with dense algal patches, mostly traveling in areas of 0.15% floating algal cover (FAC) or less (Figures 7A, B, D, F). Average overall FAC for these turtles ranged from 0.02-0.06%. Turtle 232677 encountered multiple patches of FAC ranging from 0.2-0.35% with its overall FAC average at 0.07% (Figure 7). Similarly, turtle 232679 encountered several patches of 0.2% FAC and spent 6 days in a 0.4% FAC patch with it overall FAC average at 0.08% (Figure 7).
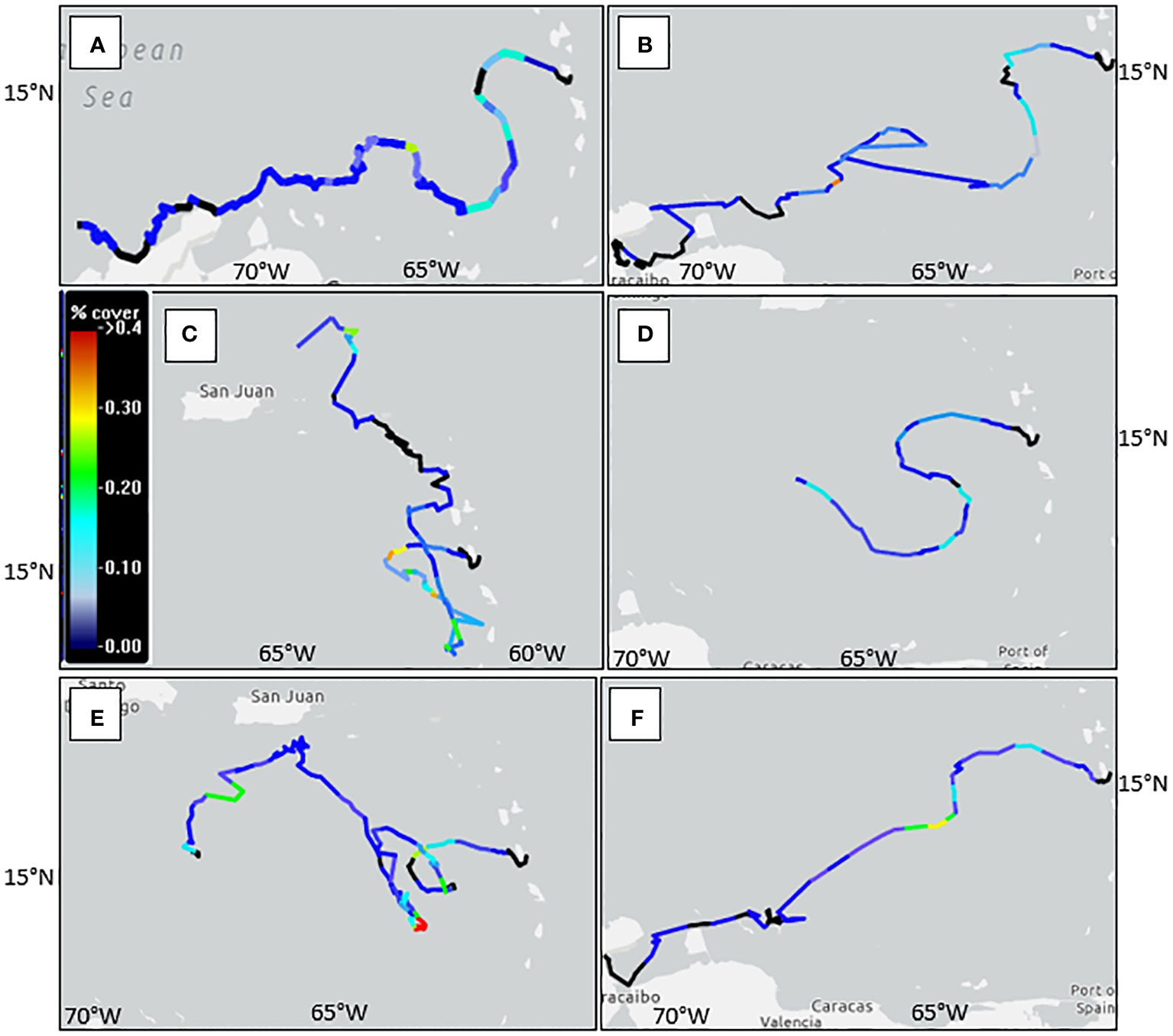
Figure 7 Tracked locations of six satellite-tagged juvenile green sea turtles released from Dominica. Letters correspond with individual turtles: (A) 232675, (B) 232676, (C) 232677, (D) 232678, (E) 232679, (F) 232680. Tracks are color coded according to the percent floating algal cover (FAC) encountered. FAC data and map legend obtained from University of Southern Florida College of Marine Science Optical Oceanography Lab and derived from the methods of Wang and Hu (2016).
4 Discussion
The current study reveals novel information regarding the movements of oceanic juvenile C. mydas hatched on the beaches of Dominica and reared in brief captivity. While some studies such as Polovina et al. (2006) have demonstrated that captive-reared sea turtles upon release present behavior expected of wild individuals, other studies highlight the fact that captive-reared sea turtles may not mirror wild behaviors due to size at age (Christiansen et al., 2016; Barbour et al., 2023). Captive-reared turtles tend to have accelerated growth rates compared to their wild counterparts of the same age and, therefore may reflect a different stage of juvenile development based on size (Christiansen et al., 2016; Barbour et al., 2023). However, the turtles hatched and raised in captivity for the studies mentioned above spent at least one year in human care before being released to the wild, whereas the juveniles from the current study were hatched in a natural setting, rescued, and raised in captivity for only 20-22 weeks. Due to this shorter duration under human care, the turtles used in the current study (14-21 cm CL) may have been only slightly larger than wild turtles (<15 cm CL) are expected to be at their age (Goshe et al., 2010) and approaching the minimum size (20 cm CL) at which juveniles have been known shift from the oceanic stage to the neritic stage (Meylan et al., 2011; Howell et al., 2016). However, due to their age (<1 year) and size class, which is at the cusp of the ontogenetic shift to the neritic stage, we expected the juveniles from the current study to display behavior characteristic of the oceanic juvenile stage.
The results of this study revealed that most of the tagged turtles spent the majority of their time in the oceanic zone, with turtle 232676 spending about half of its time in each of the oceanic and neritic zones. Turtle 232676 traveled closer to the coastline once it reached Bonaire compared to turtle 232675 which followed a similar track. Additionally, turtle 232676 spent the greatest residence time (16.5 d) in Dominica waters among all the turtles and the longest residence time (32.1 d) in Venezuela waters among the three turtles whose tracks concluded in the Gulf of Venezuela and off the coast of Colombia. Turtle 232676 was also the largest of the juvenile turtles upon release (CCL=21.3 cm) and may have been starting to exhibit the shift from oceanic to neritic habitats given it was on the lower end of the recorded shift size (Meylan et al., 2011; Howell et al., 2016). Additionally, the juveniles from the current study may demonstrate that the neritic and oceanic stages are not as fixed as other studies have shown (Reich et al., 2007; Arthur et al., 2008; Howell et al., 2016; Burgett et al., 2018). Carman et al. (2012) found that some neritic stage juveniles along the eastern Brazilian coast overwintered in oceanic (>200 m) waters. Given the low sample size from the current study, however, more juveniles should be tagged from the Caribbean to confirm any ontogenetic shift patterns. While the satellite tags used were not programmed to record depth of the animal, future juvenile sea turtle tagging studies should consider this option to better understand the use of their habitat and provide more data to pinpoint when juveniles make the shift from the oceanic to the neritic stage.
Three of the turtles ended their tracking duration within known neritic green turtle foraging grounds in the Gulf of Venezuela and off the coast of Colombia (Barrios-Garrido et al., 2020; Vasquez-Carrillo et al., 2020). Vasquez-Carrillo et al. (2020) genetically analyzed sea turtles surveyed within Colombian seagrass beds and found them to be from a mixed population stock, including populations originating from other Lesser Antilles islands such as Barbados and Trinidad. These widely used sea turtle foraging grounds may be the optimal destination for Dominican oceanic juvenile green turtles in transition to the neritic stage. While it’s impossible to confirm whether these turtles ultimately stayed in the area, two of the three were the largest juveniles at the time of release and may have been starting the shift to the neritic juvenile phase. For most of the tracking durations, these three turtles passively followed the prevailing currents. Once they neared the islands of Curacao and Bonaire, they demonstrated active swimming in opposition to the current to reach the northern coast of Venezuela and follow it westward, ending up in prominent seagrass foraging areas off the northern coast of Colombia and within the Gulf of Venezuela.
Turtle 232678’s track matches the first half of the three South American-bound turtles. However, data transmission ceased after forty days. This may have been due to a faulty satellite tag, potential mortality event, or shedding of shell scutes to accommodate growth. Mansfield et al. (2012) demonstrated that tagged turtles can grow an average of 3.5 cm before shedding tags during ecdysis. This turtle was the smallest, tagged at 14.1 cm CCL, and may have been closer to an ecdysis event or still within an exponential growth period at the time of release compared to its counterparts. It also displayed the lowest weight of the turtles upon release at 315 g, which just barely qualifies it for bearing a tag according to the guidelines of Mansfield et al. (2021). Since the tag comprised 3% of turtle 232678 (compared to the other turtles 1-2%), it may have affected energetics and hydrodynamic movement (Jones et al., 2013) more negatively, resulting in a more vulnerable position to predation (Hall and James, 2021).
All turtles started off lingering near the coast of Dominica for approximately 12-16 days before going their separate ways. Although each of the juvenile turtles took a different route, their motivations may have been the same – locating the most suitable area for food. Juvenile turtles have been found in a variety of foraging habitats including reefs, algal patches, and seagrass beds (Kubis et al., 2009). Patterns of oceanic juveniles targeting food-rich Sargassum patches, even if it requires swimming against the current, have been recorded in other studies determining juvenile green turtle movements (Mansfield et al., 2021). Similarly, Polovina et al. (2006) suggested that oceanic juvenile loggerhead turtles (Caretta caretta) used a region of the North Pacific that experiences seasonal bursts of primary productivity as foraging areas. Given the low residence time of the juvenile turtles from the current study in the oceanic areas of the Caribbean, a targeted oceanic foraging area seems unlikely. However, since 2011, Sargassum patches have been carried from the central Atlantic westward into the Caribbean Sea, causing notable changes in the floating algal density of the region (Johns et al., 2020). These transient patches may be providing habitat and food for oceanic juvenile turtles in the Caribbean (Mansfield et al., 2021). Although four of the turtles from the current study did not seem to direct their courses towards any floating algal patches, the courses of the two turtles (232677 and 232679) that varied from the rest may have been influenced by dense floating algal patches. The first portion of 232677’s track that ran contrary to the current weaved in and out of patches of 0.2-0.35% FAC. Similarly, turtle 232679’s course was contrary to the current just before entering a patch of 0.2% FAC as well as before spending 6 days in a patch of 0.4% FAC. The courses of these two turtles may have been influenced by seeking out the best foraging patches within the oceanic realm rather than closer to shore.
Barbour et al. (2023) tagged juvenile C. mydas ranging in age and size from 1 to 4 years and 35 to 62 cm CL, respectively, and released them from the Cayman Islands. Although larger than the individuals from the current study and hatched and raised completely in captivity, Barbour et al. (2023) found that the initial trajectory of the juveniles upon release followed the prevailing current before swimming in opposition to reach various coastal habitats. Even though the juveniles from the current study were young and small enough to be considered in the oceanic stage, there may have been some attraction to coastal areas due to food availability for the four turtles that displayed similar tracks.
The current study is not the first to show juvenile sea turtles abandoning passive transport in favor of preferred habitats. However, it should be noted that due to the limitations of OSCAR current data in nearshore environments, parts of the turtles’ tracks could not be analyzed for movement with/against the current. Christiansen et al. (2016) found that juvenile loggerhead turtles were more abundant in areas of higher productivity than would be expected if the turtles solely relied on passive movement with the current. Similarly, Polovina et al. (2006) also concluded that juvenile loggerheads will swim directly opposite to the current to reach foraging grounds. Mansfield et al. (2021) tracked juvenile green turtles hatched in Florida that passively rode the Gulf Stream until they departed from the prevailing current to reside in the nursery habitat of the Sargasso Sea. Some studies (Christiansen et al., 2016; Mansfield et al., 2021) demonstrate that movements against the prevailing current may be twofold – toward foraging grounds and away from suboptimal temperatures. The tracks of the green turtles hatched on Dominica do not suggest that unfavorable temperatures were a driving factor in their movements. Ambient sea surface temperatures averaged 27.7 ± 0.4°C for all turtle tracks with minimum sea surface temperatures recorded as 25.8°C, well above the tolerance threshold of 15°C for juvenile green turtles (Southwood et al., 2003).
The average distance traveled by all turtles is less than migration distance expected of post-hatching juveniles on their first oceanic journey (Hays and Scott, 2013). The mean distance which juvenile green turtles travel on their first migration as reported by Hays and Scott (2013) is 4557 ( ± 2525) km. The juveniles from the current study travelled 1202-2749 km. However, Hays and Scott (2013) were calculating the distance between natal beaches and established foraging coastal grounds which indicates the juveniles they studied were in the neritic stage. Since the turtles from the current study still appear to be in the oceanic stage, or at the most, starting to transition to the neritic stage, the final migration distance these turtles make may be greater than recorded.
Given the importance of key foraging grounds of juvenile turtles originating not only from areas adjacent to the foraging grounds but also from smaller islands thousands of kilometers away like Dominica, it is vital to ensure the appropriate level of conservation for key coastal habitats for the growth and survival of these endangered marine megafauna. Genetic analyses on juvenile C. mydas gathered at seagrass foraging grounds off Culebra, Puerto Rico (Patricio et al., 2017), the British Virgin Isles (McGowan et al., 2007), and Colombia (Vasquez-Carrillo et al., 2020) – all locations where juveniles from the current study were tracked within or near – determined a mixed stock. Since Dominica’s turtle population has not been genetically analyzed, it is within reason to conclude that the mixed juvenile green turtle populations within the wider Caribbean region include Dominica turtles as demonstrated by the juveniles from the current study migrating to areas on opposite ends of the Caribbean, specifically Colombia, the Gulf of Venezuela, the British Virgin Isles, and Puerto Rico. Conservation measures will also need to address artisanal fisheries, which also have a major impact on the decline of sea turtle species within the coastal areas where foraging grounds are located. For example, Barrios-Garrido et al. (2020) reported approximately 2200 juvenile C. mydas were taken annually from their study area in the Gulf of Venezuela.
In addition to the conservation of juvenile foraging areas, the protection of turtles within known migratory waters should be considered to ensure they reach their developmental destinations. The current study demonstrates that juvenile green turtles pass through the national waters of dozens of Caribbean island countries as well as a few major South American countries. Most of these nations have protection policies in place for sea turtles, while others have established turtle fisheries with limitations or traditional use exemptions (Eckert and Eckert, 2019). The current study’s findings provide unique additional data on the migration habits of juvenile green turtles. However, more studies aimed at determining the movement patterns of oceanic juvenile sea turtles within the wider Caribbean region, using various techniques such as acoustic and satellite tagging, stable isotopes, and mark-and-recapture methods, are needed to better understand migratory habits and better inform management decisions to help protect sea turtles at various life stages.
Data availability statement
The raw data supporting the conclusions of this article will be made available by the authors, without undue reservation.
Ethics statement
The animal study was approved by Marcella Harris of DomSeTCO. The study was conducted in accordance with the local legislation and institutional requirements.
Author contributions
JL: Conceptualization, Investigation, Methodology, Writing – review & editing. RC: Formal analysis, Writing – review & editing. AW: Data curation, Formal analysis, Writing – original draft, Writing – review & editing. DB: Writing – review & editing, Investigation. JS: Writing – review & editing, Methodology. MH: Writing – review & editing, Validation, Conceptualization, Investigation.
Funding
The author(s) declare financial support was received for the research, authorship, and/or publication of this article. Funding for this research was provided by the DomSeTCO and the US Department of Interior, Bureau of Ocean Energy Management Contract 140M0123D0004 and Cooperative Agreement M20AC10008.
Acknowledgments
We would like to thank Ms. Beverly Deikel, Mr. Patris Oscar, and Mrs. Amy Rosenblatt-Lui of the Beverly Foundation for their emotional and financial support, Dr. Craig A. Harms for veterinary consultations throughout the study, as well as Mrs. Wendy Dow-Piniak of the US National Oceanic and Atmospheric Administration and Doug Nowacek and Charles Muirhead of Duke University. We also wish to thank the tireless support of Simon and Wendy Walsh and Joe Hillman of Nature Island Dive, Mr. Harold Guiste and Stephen Durand of the Dominica Sea Turtle Conservation Organization, Simon George, Kenny George, Judy Joyce, Jovan Jno Baptiste and the entire Rosalie Nature Enhancement Team, and Hon. Julian Defoe, Minister of State, Ministry of Agriculture, Fisheries, Blue and Green Economy. All work was permitted by the government of Dominica, Ministry of the Environment, Rural Modernization, Kalinago Upliftment and Constituency Empowerment; Forestry Wildlife and Parks Division.
Conflict of interest
The authors declare that the research was conducted in the absence of any commercial or financial relationships that could be construed as a potential conflict of interest.
Publisher’s note
All claims expressed in this article are solely those of the authors and do not necessarily represent those of their affiliated organizations, or those of the publisher, the editors and the reviewers. Any product that may be evaluated in this article, or claim that may be made by its manufacturer, is not guaranteed or endorsed by the publisher.
References
Abreu-Grobois A., Plotkin P. (2008). Lepidochelys olivacea. The IUCN red list of threatened species 2008, e.T11534A3292503. doi: 10.2305/IUCN.UK.2008.RLTS.T11534A3292503.en
Allen A. M., Singh N. J. (2016). Linking movement ecology with wildlife management and conservation. Front. Ecol. Evol. 3, 155. doi: 10.3389/fevo.2015.00155
Arthur K. E., Boyle M. C., Limpus C. J. (2008). Ontogenetic changes in diet and habitat use in green sea turtle (Chelonia mydas) life history. Mar. Ecol. Prog. Ser. 362, 303–311. doi: 10.3354/meps07440
Ashford M., Watling J. I., Hart K. (2022). One shell of a problem: cumulative threat analysis of male sea turtles indicates high anthropogenic threat for migratory individuals and Gulf of Mexico residents. Remote Sens. 14 (16), 3887. doi: 10.3390/rs14163887
Barbour N., Bailey H., Fagan W. F., Mustin W., Baboolal V., Casella F., et al. (2023). Satellite tracking of head-started juvenile green turtles (Chelonia mydas) reveals release effects and an ontogenetic shift. Animals 13, 1218. doi: 10.3390/ani13071218
Barrios-Garrido H. A., Montiel-Villalobos M. G., Palmar J., Rodriguez-Clark K. M. (2020). Wayuú capture of green turtles, Chelonia mydas, in the Gulf of Venezuela: a major Caribbean artisanal turtle fishery. Ocean Coast. Manage. 188, 105123. doi: 10.1016/j.ocecoaman.2020.105123
Bolten A. B. (2003). “Variation in sea turtle life history patterns: neritic vs. oceanic developmental stages,” in The biology of sea turtles, vol. II . Eds. Lutz P. L., Musick J. A., Wyneken J. (CRC Press, Boca Raton, FL), 243–257.
Burgett C. M., Burkholder D. A., Coates K. A., Fourqurean V. L., Kenworthy W. J., Manuel S. A., et al. (2018). Ontogenetic diet shifts of green sea turtles (Chelonia mydas) in a mid-ocean developmental habitat. Mar. Biol. 165, 33. doi: 10.1007/s00227-018-3290-6
Cardona L., Aguilar A., Pazos L. (2009). Delayed ontogenic dietary shift and high levels of omnivory in green turtles (Chelonia mydas) from the NW coast of Africa. Mar. Biol. 156, 1487–1495. doi: 10.1007/s00227-009-1188-z
Carman V. G., Falabella V., Maxwell S., Albareda D., Campagna C., Mianzan H. (2012). Revisiting the ontogenetic shift paradigm: the case of juvenile green turtles in the SW Atlantic. J. Exp. Mar. Bio. Ecol. 429, 64–72. doi: 10.1016/j.jembe.2012.06.007
Carr A. (1987). New perspectives on the pelagic stage of sea turtle development. Conserv. Biol. 1 (2), 103–121.
Casale P., Ceriani S. A. (2020). Sea turtle populations are overestimated worldwide from remigration intervals: correction for bias. Endanger. Species Res. 41, 141–151. doi: 10.3354/esr01019
Casale P., Tucker A. D. (2017). Caretta caretta. The IUCN red list of threatened species 2017, e.T3897A119333622. doi: 10.2305/IUCN.UK.2017-2.RLTS.T3897A119333622.en
Chambault P., de Thoisy B., Huguin M., Martin J., Bonola M., Etienne D., et al. (2018). Connecting paths between juvenile and adult habitats in the Atlantic green turtle using genetics and satellite tracking. Ecol. Evol. 8, 12790–12802. doi: 10.1002/ece3.4708
Christiansen F., Putman N. F., Farman R., Parker D. M., Rice M. R., Polovina J. J., et al. (2016). Spatial variation in directional swimming enables juvenile sea turtles to reach and remain in productive waters. Mar. Ecol. Prog. Ser. 557, 247–259. doi: 10.3354/meps11874
CLS America. (2007). ARGOS User’s manual: worldwide tracking and environmental monitoring by satellite.Argos/CLS (Toulouse, France: CLS). Available at: http://www.argos-system.org/manual/index.html#home.htm.
Costa D. P., Robinson P. W., Arnold J. P. Y., Harrison A.-L., Simmons S. E., Hassrick J. L., et al. (2009). Accuracy of ARGOS locations of pinnipeds at-sea estimated using Fastloc GPS. PloS One 5, e8677. doi: 10.1371/journal.pone.0008677
Dunbar S. G., Hudgins J., Jean C. (2021). “Applications of photo identification in sea turtle studies,” in Sea turtle research and conservation: lessons from working in the field. Ed. Nahill B. (Elsevier Academic Press, Cambridge, MA), 45–55.
Eckert K. L., Eckert A. E. (2019). An atlas of sea turtle nesting habitat for the wider Caribbean region. Revised Edition. WIDECAST Technical Report No. 19 (Godfrey, Illinois: WIDECAST), 232. plus electronic appendices.
Finkbeiner E. M., Wallace B. P., Moore J. E., Lewison R. L., Crowder L. B., Read A. J. (2011). Cumulative estimates of sea turtle bycatch and mortality in USA fisheries between 1990 and 2007. Biol. Conserv. 144, 2719–2727. doi: 10.1016/j.biocon.2011.07.033
Fuentes M. M. P. B., McMichael E., Kot C. Y., Silver-Gorges I., Wallace B. P., Godley B. J., et al. (2023). Key issues in assessing threats to sea turtles: knowledge gaps and future directions. Endanger. Species Res. 52, 303–341. doi: 10.3354/esr01278
Gatto C. R., Rotger A., Robinson N. J., Tomillo P. S. (2018). A novel method for photo-identification of sea turtles using scale patterns on the front flippers. J. Exp. Mar. Biol. Ecol. 506, 18–24. doi: 10.1016/j.jembe.2018.05.007
Godley B. J., Lima E. H. S. M., Akesson S., Broderick A. C., Glen F., Godfrey M. H., et al. (2003). Movement patterns of green turtles in Brazilian coastal waters described by satellite tracking and flipper tagging. Mar. Ecol. Prog. Ser. 253, 279–288. doi: 10.3354/meps253279
Goshe L. R., Avens L., Scharf F. S., Southwood A. L. (2010). Estimation of age at maturation and growth of Atlantic green turtles (Chelonia mydas) using skeletochronology. Mar. Biol. 157, 1725–1740. doi: 10.1007/s00227-010-1446-0
Hall K. E., James M. C. (2021). Predation of satellite-tagged juvenile loggerhead turtles Caretta caretta in the Northwest Atlantic Ocean. Endanger. Species Res. 46, 279–291. doi: 10.3354/esr01165
Hart K. M., Fujisaki I. (2010). Satellite tracking reveals habitat use by juvenile green sea turtles Chelonia mydas in the Everglades, Florida, USA. Endang. Species Res. 11, 221–232. doi: 10.3354/esr00284
Hays G. C., Scott R. (2013). Global patterns for upper ceilings on migration distance in sea turtles and comparisons with fish, birds, and mammals. Funct. Ecol. 27, 748–756. doi: 10.1111/1365-2435.12073
Hazel J. (2009). Evaluation of fast-acquisition GPS in stationary tests and fine-scale tracking of green turtles. J. Exper. Mar. Biol. Ecol. 374, 58–68. doi: 10.1016/j.jembe.2009.04.009
Hazen E. L., Maxwell S. M., Bailey H., Bograd S. J., Hamann M., Gaspar P., et al. (2012). Ontogeny in marine tagging tracking science: technologies and data gaps. Mar. Ecol. Prog. Ser. 457, 221–240. doi: 10.3354/meps09857
Howell L. N., Reich K. J., Shaver D. J., Landry A. M. Jr., Gorga C. C. (2016). Ontogenetic shifts in diet and habitat of juvenile green sea turtles in the northwestern Gulf of Mexico. Mar. Ecol. Prog. Ser. 559, 217–229. doi: 10.3354/meps11897
Innis C., Staggs L., Manire C., Norton T., Stacy B., Harms C. (2017). Sea turtle health and rehabilitation (. Jupiter, FL: J. Ross).
Jeffers V. F., Godley B. J. (2016). Satellite tracking in sea turtles: How do we find our way to the conservation dividends? Biol. Conserv. 199, 172–184. doi: 10.1016/j.biocon.2016.04.032
Johns E. M., Lumpkin R., Putman N. F., Smith R. H., Muller-Karger F. E., Rueda-Roa D. T., et al. (2020). The establishment of a pelagic Sargassum population in the tropical Atlantic: biological consequences of a basin-scale long distance dispersal event. Prog. Oceanogr. 182, 102269. doi: 10.1016/j.pocean.2020.102269
Jones T. T., Van Houtan K. S., Bostrom B. L., Ostafichuk P., Mikkelsen J., Tezcan E., et al. (2013). Calculating the ecological impacts of animal-borne instruments on aquatic organisms. Methods Ecol. Evol. 4, 1178–1186. doi: 10.1111/2041-210X.12109
Kubis S., Chaloupka M., Ehrhart L., Bresette M. (2009). Growth rates of juvenile green turtles Chelonia mydas from three ecologically distinct foraging habitats along the east central coast of Florida, USA. Mar. Ecol. Prog. Ser. 389, 257–269. doi: 10.3354/meps08206
Lopez R., Malarde J.-P. (2014). Improving ARGOS Doppler location using Kalman filtering. CLS Memorandum: CLS-DT-MEMO-11-65. 14.
Mansfield K. L., Wyneken J., Luo J. (2021). First Atlantic satellite tracks of ‘lost years’ green turtles support the importance of the Sargasso Sea as a sea turtle nursery. Proc. R. Soc B: Biol. Sci. 288, 20210057. doi: 10.1098/rspb.2021.0057
Mansfield K. L., Wyneken J., Rittschof D., Walsh M., Lim C. W., Richards P. M. (2012). Satellite tag attachment methods for tracking neonate sea turtles. Mar. Ecol. Prog. Ser. 457, 181–192. doi: 10.3354/meps09485
Mazaris A. D., Schofield G., Gkazinou C., Almpanidou V., Hays G. C. (2017). Global sea turtle conservation successes. Sci. Adv. 3, e1600730. doi: 10.1126/sciadv.1600730
McGowan A., Broderick A. C., Frett G., Gore S., Hastings M., Pickering A., et al. (2007). Down but not out: marine turtles of the British Virgin Islands. Anim. Conserv. 11, 92–103. doi: 10.1111/j.1469-1795.2007.00152.x
Metz T. L., Gordon M., Mokrech M., Guillen G. (2020). Movements of juvenile green turtles (Chelonia mydas) in the nearshore waters of the northwestern Gulf of Mexico. Front. Mar. Sci. 7, 647. doi: 10.3389/fmars.2020.00647
Meylan P. A., Meylan A. B., Gray J. A. (2011). The ecology and migrations of sea turtles 8. Tests Dev. habitat hypothesis. Bull. Am. Mus. Nat. Hist. 357, 1–70. doi: 10.1206/357.1
Mortimer J. A., Donnelly M. (2008). Eretmochelys imbricata. The IUCN red list of threatened species 2008, e.T8005A12881238. doi: 10.2305/IUCN.UK.2008.RLTS.T8005A12881238.en
Murray D. L., Fuller M. R. (2000). “A critical review of the effects of marking on the biology of vertebrates,” in Research techniques in animal ecology. Ed. Boitani F. T. (Columbia University Press, New York, NY), 15–64.
Patricio A. R., Velez-Zuazo X., van Dam R. P., Diez C. E. (2017). Genetic composition and origin of juvenile green turtles foraging at Culebra, Puerto Rico, as revealed by mtDNA. Lat. Am. J. Aquat. Res. 45, 506–520. doi: 10.3856/vol45-issue3-fulltext-2
Polovina J., Uchida I., Balazs G., Howell E. A., Parker D., Dutton P. (2006). The Kuroshio extension bifurcation region: a pelagic hotspot for juvenile loggerhead sea turtles. Deep-Sea Res. Pt II 53, 326–339. doi: 10.1016/j.dsr2.2006.01.006
Putman N. F., Mansfield K. L. (2015). Direct evidence of swimming demonstrates active dispersal in the sea turtle “lost years.” Curr. Biol. 25, 1221–1227. doi: 10.1016/j.cub.2015.03.014
Reich K. J., Bjorndal K. A., Bolten A. B. (2007). The “lost years” of green turtles: using stable isotopes to study cryptic lifestages. Biol. Lett. 3, 712–714. doi: 10.1098/rsbl.2007.0394
Robertson D. R., Cramer K. L. (2014). Defining and dividing the Greater Caribbean: insights from the biogeography of shorefishes. PloS One 9, 7, e102918. doi: 10.1371/journal.pone.0102918
Schofield G., Katselidis K. A., Dimopoulos P., Pantis J. D. (2008). Investigating the viability of photo-identification as an objective tool to study endangered sea turtle populations. J. Exper. Mar. Biol. Ecol. 360, 103–108. doi: 10.1016/j.jembe.2008.04.005
Scott R., Marsh R., Hays G. C. (2011). Life in the really slow lane: loggerhead sea turtles mature late relative to other reptiles (227-235: Funct. Ecol. 26:1).
Seminoff J. A. (2023). Chelonia mydas. The IUCN red list of threatened species 2023, e.T4615A247654386. doi: 10.2305/IUCN.UK.2023-1.RLTS.T4615A247654386.en
Senko J. F., Burgher K. M., del Mar Mancha-Cisneros M., Godley B. J., Kinan-Kelly I., Fox T., et al. (2022). Global patterns of illegal marine turtle exploitation. Glob. Change Biol. 28, 6509–6523. doi: 10.1111/gcb.16378
Shaver D. J., Hart K. M., Fujisaki I., Rubio C., Sartain A. R. (2013). Movement mysteries unveiled: spatial ecology of juvenile green sea turtles,” in Reptiles in Research: Investigators of Ecology, Physiology, and Behavior from Desert to Sea. Ed. Lutterschmidt W. I. (Hauppauge, NY: Nova Science Publishers), 463–484.
Southwood A. L., Darveau C. A., Jones D. R. (2003). Metabolic and cardiovascular adjustments of juvenile green turtles to seasonal changes in temperature and photoperiod. J. Exper. Biol. 206, 4521–4531. doi: 10.1242/jeb.00689
Vandeperre F., Parra H., Pham C. K., Machete M., Santos M., Bjorndal K. A., et al. (2019). Relative abundance of oceanic juvenile loggerhead sea turtles in relation to nest production at source rookeries: implications for recruitment dynamics. Sci. Rep. 9, 13019. doi: 10.1038/s41598-019-49434-0
Vasquez-Carrillo C., Noriega-Hoyos C. L., Hernandez-Rivera L., Jauregui-Romero G. A., Sealey K. S. (2020). Genetic diversity and demographic connectivity of Atlantic green sea turtles at foraging grounds in northeastern Colombia, Caribbean Sea. Front. Mar. Sci. 7, 96. doi: 10.3389/fmars.2020.00096
Wallace B. P., Tiwari M., Girondot M. (2013). Dermochelys coriacea. The IUCn red list of threatened species 2013, e.T649A43526147. doi: 10.2305/IUCN.UK.2013-2.RLTS.T6494A43526147.en
Wang M., Hu C. (2016). Mapping and quantifying Sargassum distribution and coverage in the Central West Atlantic using MODIS observations. Remote Sens. Environ. 183, 356–367. doi: 10.1016/j.rse.2016.04.019
Wibbels T., Bevan E. (2019). Lepidochelys kempii. The IUCN red list of threatened species 2019, e.T11533A155057916. doi: 10.2305/IUCN.UK.2019-2.RLTS.T11533A155057916.en
Wildermann N. E., Gredzens C., Avens L., Barrios-Garrido H. A., Bell I., Blumenthal J., et al. (2018). Informing research priorities for immature sea turtles through expert elicitation. Endanger. Species Res. 37, 55–76. doi: 10.3354/esr00916
Witherington B., Hirama S., Hardy R. (2012). Young sea turtles of the pelagic Sargassum-dominated drift community: habitat use, population density, and threats. Mar. Ecol. Prog. Ser. 463, 1–22. doi: 10.3354/meps09970
Witherington B., Kubilis P., Brost B., Meylan A. (2009). Decreasing annual nest counts in a globally important loggerhead sea turtle population. Ecol. Appl. 19, 30–54. doi: 10.1890/08-0434.1
Keywords: juvenile sea turtles, marine telemetry, sea turtle movement, sea turtle lost years, Caribbean marine species
Citation: Levenson JJ, Cooper R, Weissman A, Bell D, Smith J and Harris M (2024) Tracking nest-rescued green sea turtles in oceanic currents sheds light on eastern Caribbean “lost years”. Front. Amphib. Reptile Sci. 2:1308717. doi: 10.3389/famrs.2024.1308717
Received: 07 October 2023; Accepted: 11 March 2024;
Published: 26 March 2024.
Edited by:
Natalie Elizabeth Wildermann, King Abdullah University of Science and Technology, Saudi ArabiaReviewed by:
Michelle Cazabon-Mannette, Coastal Dynamics Limited, Trinidad and TobagoMarco García, University of Florida, United States
Copyright © 2024 Levenson, Cooper, Weissman, Bell, Smith and Harris. This is an open-access article distributed under the terms of the Creative Commons Attribution License (CC BY). The use, distribution or reproduction in other forums is permitted, provided the original author(s) and the copyright owner(s) are credited and that the original publication in this journal is cited, in accordance with accepted academic practice. No use, distribution or reproduction is permitted which does not comply with these terms.
*Correspondence: J. Jacob Levenson, jacob.levenson@boem.gov