Euthanasia Through Cervical Dislocation or CO2 Might Affect Keel Bone Fracture Prevalence in 30-Week-Old Laying Hens
- Center for Animal Welfare, Department of Animal Science, University of California, Davis, Davis, CA, United States
Laying hens are susceptible to keel bone fractures due to continuous endogenous calcium resorption for eggshell formation. Although it is assumed that external trauma to the keel bone, e.g., due to collisions, is the main cause for fractures, accumulated forces or asymmetric load on a weakened bone might contribute to the high keel bone fracture prevalence found in commercial laying hens. The objective of this study was to investigate whether forces applied to the keel due to involuntary convulsions and uncontrolled wing flapping during euthanasia have the potential to cause keel bone fractures. Two hundred and seventy Dekalb White laying hens were euthanized at 30 weeks of age using cervical dislocation (n = 60) or CO2 (n = 210). All hens were radiographed immediately before and after euthanasia. Radiographs were compared side by side to detect new fractures. Four out of the 270 hens (1.5%) obtained a fracture during euthanasia. Specifically, 0.95% of hens euthanized with CO2 (2 out of 210) and 3.3% of hens euthanized through cervical dislocation (2 out of 60) obtained a euthanasia-induced fracture. All four hens with a euthanasia-induced fracture had signs of damage to the keel before euthanasia, indicating that pre-existing fractures could affect fracture susceptibility. Based on our results, we cannot rule out that convulsions during euthanasia can cause keel bone fractures in laying hens. In studies investigating keel bone integrity in birds euthanized with CO2 or cervical dislocation, fracture prevalence might be overestimated. Future research is needed to assess whether euthanasia might be more likely to cause keel bone fractures in older birds and to quantify the frequency and strength of convulsions as a potential cause of fractures.
Introduction
Laying hens are susceptible to bone fractures due to continuous endogenous calcium resorption for eggshell formation (Whitehead, 2004; Johnson, 2015). Fractures of the keel bone are highly prevalent throughout the laying cycle (reviewed by Rufener and Makagon, 2020), whereas other bones, such as the furculum, scapula, ischium, pubis, or wing bones, may be subject to fractures during handling and transport of end-of-lay hens (Gregory and Wilkins, 1989; Gregory et al., 1990; Budgell and Silversides, 2004; Clark et al., 2008). Irrespective of the bone affected, external trauma to the bone is suspected to be the main direct cause for bone fractures (Rufener and Toscano, 2020). For instance, falls and collisions in the housing system are assumed to result in keel bone fractures (Harlander-Matauschek et al., 2015; Stratmann et al., 2015), and removing birds from transport crates and shackling have been identified as the major source of fractures during depopulation of end-of-lay hens (Gregory and Wilkins, 1989). Despite evidence that external trauma can cause fractures, recent research characterizing keel bone fractures on a histo-pathological level showed that high energy collisions cannot be the only cause for bone damage in laying hens (Thøfner et al., 2020). Alternative pathogeneses such as accumulated forces or asymmetric load on a weak bone might contribute to the high fracture prevalence in commercial laying hens (Harlander-Matauschek et al., 2015).
Involuntary convulsions and severe winging flapping from common euthanasia methods for poultry, such as cervical dislocation or CO2 stunning, may be a source of accumulated forces or asymmetric load (Gerritzen et al., 2000; Sparrey et al., 2014). McKeegan et al. (2007b) speculated that convulsions during euthanasia could result in trauma and injury, but only few studies investigated fractures as a result of the stunning and euthanasia process specifically. In broilers, wing fracture prevalence increased by 2.9% between shackling prior to electric water bath stunning and after stunning (Kittelsen et al., 2015). In addition, the gas composition in biphasic CO2 stunning affects wing flapping frequency and wing fracture prevalence in broilers (Abeyesinghe et al., 2007; McKeegan et al., 2007a), indicating that the stunning process itself, in addition to transport, handling, or slaughter, is linked to the occurrence of fractures. During euthanasia in laying hens, strong or asymmetric load from severe wing flapping might cause keel bone fractures as the wing muscles are attached to the keel bone (Sisson et al., 1975). As a result, researchers interested in bone integrity often use euthanasia methods that might lower the risk for convulsions and thus, fractures. However, these methods, such as the administration of anesthesia (e.g., pentobarbital), have several on-farm limitations. The objective of this study was to investigate whether commonly used euthanasia methods, such as CO2 and cervical dislocation, have the potential to cause keel bone fractures. We hypothesized that CO2 euthanasia and cervical dislocation result in fractures at the keel as indicated by radiographs directly before and after euthanasia. In addition, previous studies suggested that greenstick fractures obtained before full ossification of the keel bone (Casey-Trott et al., 2017; Toscano et al., 2020) or other lesions to the keel (Baur et al., 2020) increase the risk for fractures later in life. Hence, the keel bone status before euthanasia—i.e., whether a fracture is present or absent before euthanasia—might affect the likelihood for euthanasia-induced fractures.
Materials and Methods
Data was collected in June 2020 at the Hopkins Avian Facility, University of California, Davis, with approval from UC Davis' Institutional Animal Care and Use Committee (Protocol #20307).
Animals and Housing
Day-old Dekalb White chicks were acquired from a commercial hatchery and reared in 15 experimental pens in one barn. Due to the main study conducted simultaneously, three different rearing systems (5 pens per rearing system) were used: FLOOR pens were equipped with 4 round, metal floor perches (length: 122 cm, diameter: 3.8 cm, height: 10 cm). SINGLE pens were furnished with a plastic grid platform (61 × 122 cm) installed at 63 cm above the floor, accessible via a ramp (32 × 97 cm) installed at a 40° angle. In addition, 3 round, metal aerial perches (33, 38, and 65 cm above floor) as well as one floor perch identical to the floor perches in the FLOOR system were provided. The MULTI system contained two adjacent platforms (30.5 × 122 cm), one at 63 cm and one at 124 cm above the floor. The higher platform was accessible via a ramp (32 × 191 cm) installed at a 40° angle. Three aerial perches (at 29, 90, and 126 cm above the floor) and one floor perch were provided. Perch space was identical for all three treatments (16 cm/bird at 16 weeks of age). All pullets had ad libitum access to feed and water through round trough feeders and nipple drinkers. Natural light was provided through windows but could not be regulated. Artificial light was used to provide at least 10 light hours per day.
At 16 weeks of age, all 15 pens were refurnished to a multi-tier housing system with four tiers, housing 30 hens per pen. The lowest tier provided a nest box (Best Nest Box, Hudson, OH; 30.5 × 122 cm nesting area) with a wooden perch in front to facilitate nest access as well as a plastic grid platform (32 × 122 cm), both at 69 cm above the floor. The second tier (height: 137 cm) and third tier (height: 198 cm) consisted of a plastic grid platform (61 × 122 cm). Two additional round metal perches were provided above the third tier (245 cm above floor, length: 122 cm, diameter: 3.8 cm). Additional perches to facilitate movements between tiers were provided at 50, 126, and 181 cm above the floor. Feed and water were provided ad libitum in round trough feeders placed on the floor and nipple drinkers.
Experimental Design
At 30 weeks of age, the effect of euthanasia method (cervical dislocation vs. CO2) and keel status before euthanasia (no/minor fracture vs. severe fracture) on the occurrence of fractures during euthanasia was tested in a 2 × 2 factorial design, using 270 hens in total. Due to the ongoing main study, which required 180 hens with no or minor keel bone fractures to be euthanized using CO2, it was not possible to balance the sample between euthanasia method and fracture status. Furthermore, data collection had to be spread out over 8 days. As a result, 180 hens with no or minor keel bone fracture were euthanized using CO2 on days 1–5 (36 hens/day). For all other treatment combinations (CO2/severe fracture, cervical dislocation/minor fracture, cervical dislocation/severe fracture), hens were euthanized on days 6 and 8 with 30 hens per treatment combination (Table 1). Within treatment combination, the selected hens were balanced for rearing treatment (10 hens per rearing treatment in each treatment combination).
Data Collection and Euthanasia
The keel bone status of the hens was determined using palpation (Casey-Trott et al., 2015) at 29 weeks of age and later confirmed through radiographs. All selected hens were radiographed before and after euthanasia using a portable X-ray unit (MyVet imaging Citation DR; X-ray generator Poskom with maximal acceleration voltage of 100 kV; Rayence Xmaru 1012 WCC/WGC flat panel detector) at a film-focus distance of 60 cm and voltage of 50 kV/2.5 mAs. Hens were inverted with feet placed into a poultry shackle to induce immobility in live hens (Sirovnic and Toscano, 2017). The X-ray unit was located in the barn to ensure that live hens were radiographed in a familiar environment. Euthanasia took place either outside the barn (cervical dislocation) or in a separate building (CO2). For cervical dislocation, hens were carried outside the barn individually and manually cervically dislocated by one of two trained individuals. The individual performing the euthanasia was recorded. Hens were held on both legs until convulsions stopped. For CO2, all hens were euthanized by the same individual. Hens were carefully placed in a plastic container with a lid (Sterilite, Townsend, MA; 58 × 41 × 31 cm, L × W × H) and opening for the CO2 hose (~1.5 cm in diameter) in groups of three and carried to a separate building ~120 m away. The standard on-site, biphasic procedure was used for CO2 euthanasia, with a concentration of 10 ppm for ~30 s to induce loss of consciousness followed by 30 ppm for at least 90 s. In accordance with the AVMA Guidelines for the Euthanasia of Animals (AVMA, 2020), behavioral indicators (e.g., loss of muscle tension, closed eyes) were used to determine loss of consciousness and thus, the moment to increase CO2 concentration. After euthanasia, hens were brought back to the barn for postmortem radiographs.
Data Processing and Analysis
Radiographs were downloaded as DICOM files, and pre vs. postmortem images were compared side by side in RadiAnt DICOM viewer (version 2020.1.1) by one trained observer (CR). The observer was blind to euthanasia method but not keel bone state before euthanasia. The presence, location and type of euthanasia-induced fractures were recorded according to Baur et al. (2020). Euthanasia-induced fractures were defined as fracture lines, possibly with signs of oedema, dislocation, or angulation which were only present on the postmortem radiographs. As not enough new fractures occurred to justify a statistical analysis, results are presented descriptively.
Results
From the 270 euthanized hens, 4 (1.5 %) obtained a fracture during euthanasia (Table 2). Two of these hens were euthanized using cervical dislocation, while the other two were euthanized using CO2. As a result, 0.95 % of hens euthanized with CO2 (2 out of 210) and 3.3 % of hens euthanized through cervical dislocation (2 out of 60) obtained a euthanasia-induced fracture.

Table 2. Description of the 4 hens with euthanasia-induced fractures, including the euthanasia method (CD, cervical dislocation), keel status before euthanasia, fracture type and location of the euthanasia-induced fracture, rearing system, and whether the fracture was located in a part of the bone with (re-fracture) or without (new fracture) signs of fracture before euthanasia.
Three hens had minor damage before euthanasia, whereas the fourth hen had a severe fracture. In two of the hens, an old fracture re-fractured. In the remaining two hens, the keel bones did not show previous signs of bone damage in the location of the euthanasia-induced fracture. Figure 1 shows radiographs of two hens with a euthanasia-induced fracture.
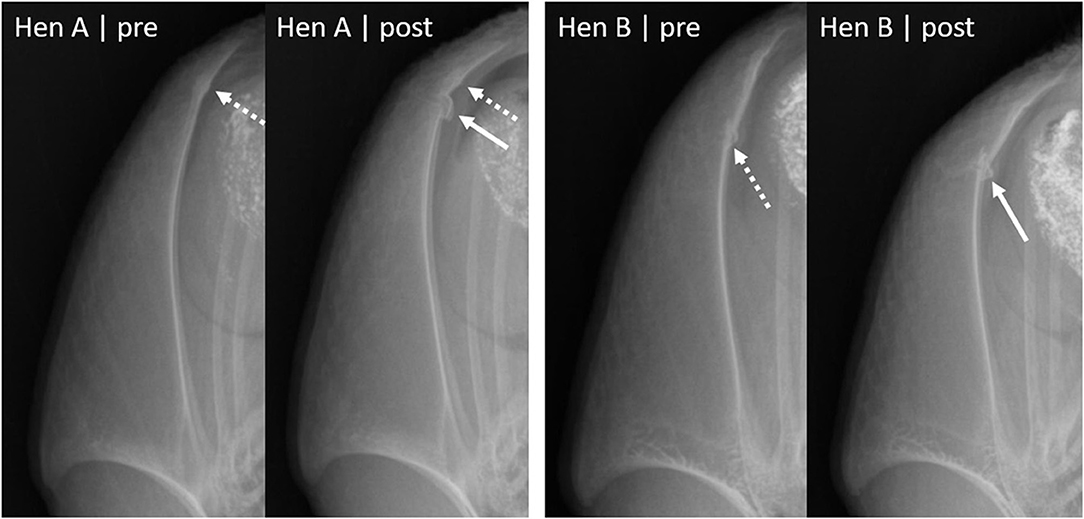
Figure 1. Laterolateral radiographs of two hens before and after euthanasia. Dashed arrows indicate fractures present before euthanasia, solid arrows show euthanasia-induced fractures (new fractures or re-fractures). Hen A had an old minor fracture (indicated by callus formation) before euthanasia and obtained a new fracture in the caudal third of the keel bone. The new, euthanasia-induced fracture is indicated by a transverse fracture line and dislocation of a bone segment on the dorsal side of the keel. Hen B had a minor fracture indicated by bone formation and an interruption of the metasternum on the dorsal side of the keel. The postmortem radiograph shows a re-fracture, with an oblique fracture line, additional fracture segments on the dorsal side of the keel as well as angulation between the caudal third and the rest of the keel bone.
Discussion
The objective of this study was to investigate whether commonly used euthanasia methods, such as CO2 and cervical dislocation, have the potential to cause keel bone fractures. Due to the unbalanced design and a low number of birds obtaining a fracture during euthanasia, the results of our study have to be interpreted with caution. While 1.5% of hens obtaining a euthanasia-induced fracture could be considered a low proportion, we cannot rule out that fractures can occur during the euthanasia process based on our results.
Although the number of hens with euthanasia-induced fractures was too low to explore the role of euthanasia method or keel bone status statistically, it is worth mentioning that the proportion of hens obtaining a fracture during cervical dislocation was more than three times higher than in hens euthanized with CO2 (3.3 vs. 0.95 %). This could be due to differences in the latency to onset, duration, or force of the involuntary convulsions. It is assumed that convulsions are stronger with methods that cause anoxia, i.e., complete depletion of oxygen in the brain (Raj et al., 2006). Due to the immediate separation of the spinal cord from the brain and rupture of the jugular vein and carotid artery, cervical dislocation causes cerebral anoxia whereas biphasic euthanasia with CO2 acts through hypoxia (a steady reduction in oxygen supply).
Accordingly, convulsions start right after cervical dislocation and last up to 150 s in broilers (Jacobs et al., 2019) or 136 s in laying hens (Hernandez et al., 2019). In contrast, the latency to onset of convulsions in birds euthanized using CO2 may take 45 s (Raj et al., 1990) and can be manipulated with concentration of CO2 or gas mixes such as CO2 and Argon (Coenen et al., 2000; Webster and Fletcher, 2001). As it is difficult to quantify convulsion strength, future studies investigating bone fractures in response to euthanasia could consider behavior such as wing flapping as a proxy for uncontrolled forces applied to the keel bone.
A limitation of the present study is that we sampled birds at 30 weeks of age only. The trajectory of keel bone fractures is not linear. Most fractures to the keel occur between 25 and 35 weeks of age (Harlander-Matauschek et al., 2015; Baur et al., 2020), but fracture susceptibility increases until ~49 weeks of age and seems to stabilize thereafter (Toscano et al., 2018). Because of reduced bone strength due to continuous calcium resorption for eggshell formation, older birds might be at a higher risk for keel bone fractures during euthanasia. Given that all four hens obtaining a euthanasia-induced fracture in our study had damage to the keel before euthanasia, pre-existing fractures might further increase fracture susceptibility though more research with a higher number of birds obtaining fractures during euthanasia is needed to further evaluate contributing factors.
In conclusion, we have to consider that convulsions during euthanasia might cause keel bone fractures in laying hens. If cervical dislocation or CO2 stunning methods are used for studies investigating keel bone integrity, fracture prevalence might be overestimated. An overestimation of fracture prevalence in euthanized birds might further affect the validity of other fracture detection methods. For instance, accuracy of palpation—the most commonly used method for keel bone fracture detection—is often assessed by comparing palpation results in live birds pre-euthanasia with a visual inspection of a dissected bone post-euthanasia.
We recommend considering the euthanasia method as a potential cause for fractures in future keel bone damage research. The administration of anesthetics such as pentobarbital can reduce the duration of convulsions (22 vs. 136 s; Hernandez et al., 2019) and avoid convulsions in 50 % of hens euthanized through cervical dislocation (Bandara et al., 2019). Future research is needed to assess keel bone fractures as a result of euthanasia in older birds and to quantify timing, frequency, and strength of convulsions as a potential cause for fractures.
Data Availability Statement
The raw data supporting the conclusions of this article will be made available by the authors, without undue reservation.
Ethics Statement
The animal study was reviewed and approved by Institutional Animal Care and Use Committee of the University of California, Davis (Protocol #20307).
Author Contributions
CR, AP, and RB contributed to the conceptualization and design of this study. CR and AP collected the data. CR analyzed the radiographs and wrote the first draft of the manuscript. All authors contributed to manuscript revision and approved the submitted version.
Funding
This project was funded in part by USDA Multistate Research Project NC 1029. CR's salary was supported by the Foundation for Food and Agriculture Research under award number–Grant ID 550830.
Disclaimer
The content of this publication is solely the responsibility of the authors and does not necessarily represent the official views of the Foundation for Food and Agriculture Research.
Conflict of Interest
The authors declare that the research was conducted in the absence of any commercial or financial relationships that could be construed as a potential conflict of interest.
Acknowledgments
We thank the staff at the Hopkins Avian Facility, particularly Kristy Portillo and Kevin Bellido, and all student assistants (Chloe Cheuk, Yoonjae Cho, Diana Gonzalez, Elisha Graham, Jessica Hodson, Nhi Hong, Claire Jones, Max Lau, Brenda Lopez, Claudia Lu, Ramit Manhas, Angelica Martinez, Jasmine Orr, Wendon Song, and Elizabeth Zamora) for excellent animal care. We are also grateful to Dr. Maja Makagon for input on experimental design and help with project planning.
References
Abeyesinghe, S. M., McKeegan, D. E. F., Mcleman, M. A., Lowe, J. C., and Demmers, T. G. M. (2007). Controlled atmosphere stunning of broiler chickens. I. Effects on behaviour, physiology and meat quality in a pilot scale system at a processing plant. Br. Poult. Sci. 48, 406–423. doi: 10.1080/00071660701543089
AVMA (2020). AVMA Guidelines for the Euthanasia of Animals: 2020 Edition. Schaumburg, IL: American Veterinary Medical Association.
Bandara, R. M. A. S., Torrey, S., Turner, P. V., Zur Linden, A., Bolinder, A., Schwean-Lardner, K., et al. (2019). Efficacy of a novel mechanical cervical dislocation device in comparison to manual cervical dislocation in layer chickens. Animals 9:407. doi: 10.3390/ani9070407
Baur, S., Rufener, C., Toscano, M. J., and Geissbühler, U. (2020). Radiographic evaluation of keel bone damage in laying hens – morphologic and temporal observations in a longitudinal study. Front. Vet. Sci. 7:129. doi: 10.3389/fvets.2020.00129
Budgell, K. L., and Silversides, F. G. (2004). Bone breakage in three strains of end-of-lay hens. Can. J. Anim. Sci. 84, 745–747. doi: 10.4141/A04-040
Casey-Trott, T., Heerkens, J. L. T., Petrik, M., Regmi, P., Schrader, L., Toscano, M. J., et al. (2015). Methods for assessment of keel bone damage in poultry. Poult. Sci. 94, 2339–2350. doi: 10.3382/ps/pev223
Casey-Trott, T. M., Guerin, M. T., Sandilands, V., Torrey, S., and Widowski, T. M. (2017). Rearing system affects prevalence of keel-bone damage in laying hens: a longitudinal study of four consecutive flocks. Poult. Sci. 96, 2029–2039. doi: 10.3382/ps/pex026
Clark, W. D., Cox, W. R., and Silversides, F. G. (2008). Bone fracture incidence in end-of-lay high-producing, noncommercial laying hens identified using radiographs. Poult. Sci. 87, 1964–1970. doi: 10.3382/ps.2008-00115
Coenen, A., Smit, A., Zhonghua, L., and Van Luijtelaar, G. (2000). Gas mixtures for anaesthesia and euthanasia in broiler chickens. Worlds. Poult. Sci. J. 56, 225–234. doi: 10.1079/WPS20000017
Gerritzen, M. A., Lambooij, E., Hillebrand, S. J. W., Lankhaar, J. A. C., and Pieterse, C. (2000). Behavioral responses of broilers to different gaseous atmospheres. Poult. Sci. 79, 928–933. doi: 10.1093/ps/79.6.928
Gregory, N. G., and Wilkins, L. J. (1989). Broken bones in domestic fowl: handling and processing damage in end-of-lay battery hens. Br. Poult. Sci. 30, 555–562. doi: 10.1080/00071668908417179
Gregory, N. G., Wilkins, L. J., Eleperuma, S. D., Ballantyne, A. J., and Overfield, N. D. (1990). Broken bones in domestic fowls: effect of husbandry system and stunning method in end-of-lay hens. Br. Poult. Sci. 31, 59–69. doi: 10.1080/00071669008417231
Harlander-Matauschek, A., Rodenburg, T. B., Sandilands, V., Tobalske, B. W., and Toscano, M. J. (2015). Causes of keel bone damage and their solutions in laying hens. Worlds. Poult. Sci. J. 71, 461–472. doi: 10.1017/S0043933915002135
Hernandez, E., James, F., Torrey, S., Widowski, T., Schwean-Lardner, K., Monteith, G., et al. (2019). Evaluation of brain death in laying hens during on-farm killing by cervical dislocation methods or pentobarbital sodium injection. Front. Vet. Sci. 6:297. doi: 10.3389/fvets.2019.00297
Jacobs, L., Bourassa, D. V., Harris, C. E., and Buhr, R. J. (2019). Euthanasia: manual versus mechanical cervical dislocation for broilers. Animals 9:47. doi: 10.3390/ani9020047
Johnson, A. L. (2015). “Reproduction in the female,” in Sturkie's Avian Physiology, ed C. G. Scanes (London: Elsevier Academic Press), 635–665. doi: 10.1016/B978-0-12-407160-5.00028-2
Kittelsen, K. E., Granquist, E. G., Vasdal, G., Tolo, E., and Moe, R. O. (2015). Effects of catching and transportation versus pre-slaughter handling at the abattoir on the prevalence of wing fractures in broilers. Anim. Welf. 24, 387–389. doi: 10.7120/09627286.24.4.387
McKeegan, D. E. F., Abeyesinghe, S. M., Mcleman, M. A., Lowe, J. C., and Demmers, T. G. M. (2007a). Controlled atmosphere stunning of broiler chickens II. Effects on behaviour, physiology and meat quality in a commercial processing plant. Br. Poult. Sci. 48, 430–442. doi: 10.1080/00071660701543097
McKeegan, D. E. F., McIntryre, J. A., Demmers, T. G. M., Lowe, J. C., Wathes, C. M., van den Broek, P. L. C., et al. (2007b). Physiological and behavioural responses of broilers to controlled atmosphere stunning: Implications for welfare. Anim. Welf. 16, 409–426.
Raj, A. B. M., Gregory, N. G., and Wotton, S. B. (1990). Effect of carbon dioxide stunning on somatosensory evoked potentials in hens. Res. Vet. Sci. 49, 355–359. doi: 10.1016/0034-5288(90)90073-D
Raj, A. B. M., Sandilands, V., and Sparks, N. H. C. (2006). Review of gaseous methods of killing poultry on-farm for disease control purposes. Vet. Rec. 159, 229–235. doi: 10.1136/vr.159.8.229
Rufener, C., and Makagon, M. M. (2020). Keel bone fractures in laying hens: a systematic review of prevalence across age, housing systems, and strains. J. Anim. Sci. 98, 36–51. doi: 10.1093/jas/skaa145
Rufener, C., and Toscano, M. J. (2020). “Bone health and associated problems in layer hens,” in Understanding the Behaviour and Improving the Welfare of Chickens, ed C. J. Nicol Cambridge: Burleigh Dodds Science Publisher. doi: 10.19103/AS.2020.0078.16
Sirovnic, J., and Toscano, M. J. (2017). “Restraining laying hens for radiographic diagnostics of keel bones,” in Proceeding Xth European Symposium on Poultry Welfare (Ploufragan), 162.
Sisson, S., Grossman, J. D., and Getty, R. (1975). Sisson and Grossman's The Anatomy of the Domestic Animals, 5th ed. Philadelphia, PA: Saunders.
Sparrey, J., Sandercock, D. A., Sparks, N. H. C., and Sandilands, V. (2014). Current and novel methods for killing poultry individually on-farm. Worlds. Poult. Sci. J. 70, 737–758. doi: 10.1017/S0043933914000816
Stratmann, A., Fröhlich, E. K. F., Gebhardt-Henrich, S. G., Harlander-Matauschek, A., Würbel, H., and Toscano, M. J. (2015). Modification of aviary design reduces incidence of falls, collisions and keel bone damage in laying hens. Appl. Anim. Behav. Sci. 165, 112–123. doi: 10.1016/j.applanim.2015.01.012
Thøfner, I., Hougen, H. P., Villa, C., Lynnerup, N., and Christensen, J. P. (2020). Pathological characterization of keel bone fractures in laying hens does not support external trauma as the underlying cause. PLoS ONE 15:e0229735. doi: 10.1371/journal.pone.0229735
Toscano, M. J., Booth, F., Richards, G., Brown, S. N., Karcher, D. M., and Tarlton, J. F. (2018). Modeling collisions in laying hens as a tool to identify causative factors for keel bone fractures and means to reduce their occurrence and severity. PLoS ONE 13:e0200025. doi: 10.1371/journal.pone.0200025
Toscano, M. J., Dunn, I. C., Christensen, J. P., Petow, S., Kittelsen, K., and Ulrich, R. (2020). Explanations for keel bone fractures in laying hens: are there explanations in addition to elevated egg production? Poult. Sci. 99, 4183–4194. doi: 10.1016/j.psj.2020.05.035
Webster, A. B., and Fletcher, D. L. (2001). Reactions of laying hens and broilers to different gases used for stunning poultry. Poult. Sci. 80, 1371–1377. doi: 10.1093/ps/80.9.1371
Keywords: laying hen, keel bone fracture, cervical dislocation, CO2, euthanasia
Citation: Rufener C, Pullin AN and Blatchford RA (2021) Euthanasia Through Cervical Dislocation or CO2 Might Affect Keel Bone Fracture Prevalence in 30-Week-Old Laying Hens. Front. Anim. Sci. 2:652115. doi: 10.3389/fanim.2021.652115
Received: 11 January 2021; Accepted: 23 February 2021;
Published: 12 March 2021.
Edited by:
Erin E. Connor, University of Delaware, United StatesReviewed by:
Patricia V. Turner, University of Guelph, CanadaLeonie Jacobs, Virginia Tech, United States
Copyright © 2021 Rufener, Pullin and Blatchford. This is an open-access article distributed under the terms of the Creative Commons Attribution License (CC BY). The use, distribution or reproduction in other forums is permitted, provided the original author(s) and the copyright owner(s) are credited and that the original publication in this journal is cited, in accordance with accepted academic practice. No use, distribution or reproduction is permitted which does not comply with these terms.
*Correspondence: Richard A. Blatchford, rablatchford@ucdavis.edu