Acidified diet is not effective in preventing hypocalcemia in dairy cows on French commercial farms
- 1Innoval, Noyal-sur-Vilaine, France
- 2INRAE, Oniris, BIOEPAR, Nantes, France
- 3PEGASE, INRAE, Institut Agro, Saint-Gilles, France
The objective of this study was to assess, in the context of typical dairy farms in western France, the preventive effects of prepartum diet acidification (AcD) and mineral intake during late gestation on the incidence of subclinical hypocalcemia (SHC) and subclinical hypophosphatemia (SHP) after calving. We conducted a longitudinal study that followed a cohort of 371 Holstein cows from 26 French dairy farms; of these, 235 cows (15 farms) were supplied with anionic salts during late gestation, and 136 cows (11 farms) were not. Blood samples were collected from 1 to 22 cows per farm (average of 14.3 cows per farm) between 24 and 48 h after calving. Total calcium and inorganic phosphorus concentrations in plasma were then quantified by inductively coupled plasma - optical emission spectrometry. The effects of AcD on the incidence of SHC and SHP were assessed using mixed linear models that evaluated the cow-level factors parity, milk yield index, and individual health events/treatments, and the farm-level factors diet calcium and magnesium content, dietary phosphorus intake, vitamin D supply, diet crude protein content, and duration of AcD, with farm as a random effect. Approximately 55% of cows were diagnosed with SHC (calcium < 2.0 mmol/l, n = 203) and 37% with SHP (inorganic phosphorus < 1.3 mmol/l, n=136). The first model confirmed earlier findings that the risk of SHC is higher with increased parity (P ≤ 0.0001) and revealed a higher risk associated with high milk yield (P = 0.0005), high phosphorus intake (40–60 g/cow per day, OR = 3.5; ≥ 60 g/cow per day, OR = 7.3; P = 0.0003) and high vitamin D supply (≥ 19950 IU/cow per day, OR = 3, P = 0.007). The second model highlighted a greater risk of SHP with increasing parity (P = 0.03) and showed trends for the preventive effects of AcD (OR = 0.4, P = 0.07) and moderate amounts of phosphorus in the diet (OR = 0.4, P = 0.10). Overall, our results do not support the effectiveness of AcD in preventing SHC or SHP under field conditions examined here, probably resulting from insufficient diet acidification.
Introduction
Hypocalcemia is a major health issue in dairy cattle that occurs around calving. Clinical hypocalcemia, also known as milk fever, occurs in 1.8–7.1% of dairy cows (Saborío-Montero et al., 2017; Venjakob et al., 2017) and is associated with a greater risk of culling in early lactation (Probo et al., 2018). Subclinical hypocalcemia (commonly defined as serum concentration < 2 mmol/l, SHC) is far more frequent, affecting 25–54% of dairy cows, depending on parity (Reinhardt et al., 2011). It can have detrimental effects on immunity by decreasing the percentage of neutrophils involved in phagocytosis and reducing their oxidative burst response (Martinez et al., 2014; Leno et al., 2017). In this way, SHC increases the incidence of disease during the postpartum period (McArt and Neves, 2019), especially metritis (Venjakob et al., 2019). Several programs have been developed to prevent this condition, of which the most common is diet acidification in late gestation (Goff, 2008; Wilkens et al., 2020). This consists of adding anionic salts to the prepartum diet in the last three weeks of gestation in order to obtain a strong imbalance between dietary cations and anions (i.e., negative value for DCAD = ([K+] + [Na+]) – ([Cl-] + [S2-])). This program has been widely and successfully tested in experimental studies over the past three decades, as reported in a recent meta-analysis (Lean et al., 2019). However, no published study has yet attempted to assess the influence of an acidified diet on the incidence of SHC under field conditions, where compliance with theoretical recommendations can vary widely. Consequently, the effectiveness of this strategy in a wide variety of contexts remains uncertain. For example, the effectiveness of an acidified diet in late gestation on SHC incidence can be influenced by the simultaneous intake of phosphorus (P) (Peterson et al., 2005), magnesium (Mg) (Lean et al., 2006), and calcium (Ca) (Goff, 2014). Recent experimental studies have shed much-needed light on these effects, especially for Ca (Lean et al., 2019) and P (Cohrs et al., 2018; Keanthao, 2021; Wächter et al., 2021). Similarly, recent work has questioned the threshold of DCAD for inducing preventive effects (Diehl et al., 2018; Goff and Koszewski, 2018). These new results have led to the suggestion of new recommendations (lowering intake of Ca and P, thus obtaining a slight negative DCAD) (NRC, 2001; National Academies of Sciences, Engineering and M, 2021) but can also lead to confusion in the field, which can bring about the misapplication of prevention programs by farmers and nutritionists, as highlighted by Redfern et al. (2021). In Europe, where the predominant context for dairy production is small farms (< 200 cows) (Institut de l’Elevage, 2019), such misapplications could be more frequent because providing a specific diet during the last three weeks of gestation is labor-intensive: it involves mixing small amounts of feed for a subset of dry cows (those in the “close-up” period) and feeding them separately from the far dry-off cows.
Compared to SHC, less attention has been paid to subclinical hypophosphatemia (SHP), even though its incidence can be high [between 15 and 50% (Staufenbiel, 2002; Macrae, 2012)]. SHP has detrimental effects on immunity (Mullarky et al., 2009; Eisenberg et al., 2014), but its direct impact on cow health has not yet been characterized. Because diet acidification and the intake of P and vitamin D in late gestation can influence its incidence (Grünberg, 2014; Wilkens and Muscher-Banse, 2020), it is important to consider the consequences of feeding recommendations aimed at preventing SHC on SHP risk. Indeed, the intake of P recommended for preventing SHC could actually increase the risk of SHP, as demonstrated by Wächter et al. (Wächter et al., 2021) in an experimental study comparing the effects of 0.15% and 0.30% dietary P on both blood calcium and blood inorganic phosphorus.
The objective of this study was thus to assess, in a context of typical small dairy farms of western France, the preventive effects of prepartum diet acidification and Ca, P, and Mg dietary intake on the incidence of subclinical hypocalcemia (blood calcium < 2.0 mmol/l) and subclinical hypophosphatemia (blood inorganic phosphorus ≤ 1.3 mmol/l) after calving.
Materials and methods
The animal study was reviewed and approved by the ONIRIS ethics committee for clinical and epidemiological veterinary research under decision CERVO-2019-3-V. Written informed consent was obtained from the owners for the participation of their animals in this study.
Study design
We conducted a cohort study between January 2020 and March 2021 in the Brittany region, which is a typical western European plain and the main milk-producing area in France. Our main objective was to compare the incidence of SHC between two groups of cows, those fed with an acidified diet (AcD) and those fed with a standard, i.e., non-acidified, diet (StD). We designed our study by taking into account published data from studies under AcD conditions, in which the incidence of SHC was 40% in the AcD group (McArt and Neves, 2019). Based on this, a sample size of 300 cows in each group (AcD, StD) should reveal a 12% difference in SHC incidence between the two groups with P < 0.05. For practical reasons, we intended to enroll 20 cows from each farm. Thus, we aimed to include 30 farms in total, 15 in each group.
We selected a study population of dairy farms from a database provided by the private animal health company GDS Bretagne (France) which contains all dairy farms in Brittany (n = 9525). Only dairy farms with at least 60 cows were selected (in order to ensure that the dairy herd was the main means of production) and at least 90% of cows had to be Holstein, in order to avoid any breed-induced variability in hypocalcemia risk (N = 4238). Farms were then randomly selected using successive random samplings (without replacement) of 100 farms. To control for variability in hypocalcemia risk linked to milk yield among herds, the random selection was stratified by the median milk yield in the region (7500 kg/cow per year). The selected farms were called to ask for their consent to participate and were included in the study if they met the following criteria: 1) they participated in the official French milk recording scheme, to make it possible for us to obtain values of milk yield index; 2) they did not use grazed pasture to feed dry cows in late gestation, so that it would be easier to estimate feed intake, and 3) for members of the AcD group, they would supply anionic salts to cows during the last three weeks of gestation (the “close-up” period) to prevent hypocalcemia at calving.
Data collection
Each farm included in the study was visited at least once before the experiment began (inclusion visit) by one of the three investigators (the first author and two veterinary students), in the presence of the farmer’s private veterinarian. During the visit, we described in detail the diets to be fed to cows and heifers in the close-up period during the experiment (list of ingredients and corresponding amounts) and collected the composition sheets for all commercial mixes used (i.e., concentrates and minerals) along with samples of forage for further analysis. Forage samples were transported to the laboratory (INRAE, St Gilles, France) at ambient temperature and stored at -20°C until analysis. The farmer was asked to alert the investigator if any ingredients changed during the study period, so that a new forage sample or composition sheet could be collected. The date of the diet change was also recorded. In order to estimate intake, we categorized each farmer’s practices for the quantification of commercial mixes as either “weighing” or “benchmarking” for each concentrate and mineral used. If the farmer used a reference benchmark for mixing the diet instead of weighing, the investigator weighed the benchmark during the inclusion visit. If no benchmark was used, the investigator defined one with the farmer in accordance with the standard practices used on the farm.
Following the inclusion visit, cows and heifers were enrolled in the cohort study upon drying-off. Cows were then followed until they were seen by the farmer’s veterinarian 24 to 48 h after calving. During this visit, the veterinarian estimated each cow’s body condition score (BCS) on a 5-point scale (Bazin, 1984), collected a blood sample from the cow, and recorded 1) any preventive practices or treatments for hypocalcemia administered by the farmer around the time of calving (calcium administration, vitamin D injections, incomplete first milking), 2) the date and time of calving, 3) any health issues that occurred after calving, and 4) the date and time of blood collection. The blood sample was collected from the coccygeal vessels using 25-mm 18 gauge needles and 4 mL lithium heparin vacutainer tubes (Greiner Bio-One, Monroe, NC). Samples were transported to the veterinary clinic at ambient temperature and centrifuged at 1 500 × g for 15 min at ambient temperature; plasma was pipetted into two aliquots and stored at -20°C. At the end of the study period, plasma samples were transported to the laboratory (INARE, St Gilles, France) at -80°C in dry ice (CRYO-Distribution, Pléchâtel, France) and stored at -20°C until analysis.
Data on parity and the milk yield index of cows included in the study were collected from the official milk records in the French national genetic information system (SIG, Idele, France). We chose to use the milk yield index instead of the 305-day milk yield to characterize each cow’s potential milk yield because we viewed the former as being highly predictive of effective milk yield (Brochard et al., 2013) and more correlated to cow potential than the 305-day milk yield, which can be influenced by different farm management practices.
Chemical analysis
Forages samples were oven-dried in a ventilated oven (48 h, 60°C) and ground with a 0.8-mm grid. Forage nitrogen content (N) was analyzed using the Dumas method (AOAC 7 024, 1831), which is based on sample pyrolysis and direct determination of N2 using an automatic device (LECO Corporation, St. Joseph, MI, USA). Crude protein (CP) was estimated to be N × 6.25. Forage Ca, P, and Mg contents were obtained by ICP-OES (inductively coupled plasma - optical emission spectrometry) according to AFNOR (2017). Plasma Ca and Mg contents were obtained by ICP-OES after dilution with nitric acid (2% v/v) and the addition of Triton X-100. Plasma inorganic phosphorus (Pi) content was determined using a KONE PRO multiparameter analyzer (Thermo Fisher Scientific, Illkirch, France) by the Allen method for P (PIEN J, 1969), without mineralization.
Estimation of element intake
As all farmers participating to the study did not weigh all ingredients from the diet and refusals, we preferred estimating diet fed amounts rather than use amounts reported by farmers. Based on the diets fed to cows and heifers in late gestation, dietary intake of Ca, P, Mg, and CP, along with values of DCAD (DCAD = ([K+] + [Na+]) – ([Cl-] + [S2-]) in mEq/kg DM), was estimated by summing the amounts provided by commercial mixes (i.e., concentrates and mineral supplements) and forage. The supply obtained from commercial mixes was calculated from the farmers’ reports on feeding amounts and the composition provided by the feed manufacturers or from French nutritional tables for raw feedstuffs (Inra, 2018). The reported amounts of forage were compared to the feed intake capacity of a standard cow (or heifer) in late gestation as predicted by INRAtion® software V5 (https://www.inration-ruminal.fr/en/). Feed intake capacity was predicted based on standard cow (or heifer) characteristics and forage composition. In this case, the characteristics of the standard cow were: 2nd parity, 38 weeks pregnant, live body weight of 650 kg, current and expected calving body condition score of 2.5, calf weight at birth of 42 kg, peak milk yield of 45 kg/day, milk fat of 4%, and milk true protein of 3.4%. The standard heifer was characterized by: 1st parity, 38 weeks pregnant, live body weight of 550 kg, age at calving 26 months, current and expected calving body condition score of 2.5, calf weight at birth of 40 kg, peak milk yield of 35 kg/day, milk fat of 4%, and milk true protein of 3.4%. The composition of feedstuffs used by the software to predict feed intake were standard and based on INRAE feeding tables (Inra, 2018). For instance, references for forage were FE4720 for maize silage, FF0080 for hay, and FP0020 for straw. Using these characteristics, feed intake capacity was predicted based on the farmer’s feeding practices for cows (or heifers) in late gestation. When cows (or heifers) were fed a total mixed ration, the prediction was based on the fixed proportions of all feedstuffs in the diet. When forage was fed ad libitum and the quantities of other feedstuffs in the diet were fixed, the prediction was based on the fixed quantities for all feedstuffs except forage, the intake of which was estimated. A total of 52 reports of feeding amounts were compared to predictions (26 diets for cows and 26 for heifers). Any reported amounts that exceeded feed intake capacity were changed to the predicted values.
The dietary supply of vitamin D from forage was considered to be insignificant compared to that obtained from commercial mixes. Moreover, we wished this study to focus only on data that could be routinely obtained, and feedstuff analysis of vitamin D content is difficult and expensive under field conditions. Thus, only the vitamin D supplied by commercial mixes was considered in this study; this was calculated from the declared amounts fed and the composition data provided by feed manufacturers.
Because weighing practices for the estimation of the amount of commercial mixes could influence mineral intake, farms were divided into two categories: 1) those that weighed less than 50% of commercial mixes, or 2) those that weighed 50% of commercial mixes or more.
Strategy of analysis
The statistical unit was the cow. Subclinical hypocalcemia (SHC) was defined using three different cut-offs—plasma calcium below 2.0, 2.1, or 2.2 mmol/l (Reinhardt et al., 2011; Venjakob et al., 2017)—in order to compare the incidence observed here with previous studies that used different thresholds. Subclinical hypophosphatemia (SHP) was defined as levels of plasma inorganic phosphorus below 1.3 mmol/l because this is the level at which detrimental immune effects become apparent (Eisenberg et al., 2014). The incidence of SHC and SHP was determined for each parity group and diet group (i.e., AcD and StD). All other data were collected and presented for the whole study sample and by diet group (AcD and StD). Quantitative data are reported as mean ± standard deviation (µ ± SD).
The effect of AcD versus StD on SHC incidence (binary outcome) and SHP incidence (binary outcome with 1.3 mmol/l cut-off) was assessed using mixed linear models that included the cow-level factors of parity, milk yield index, preventive treatments, BCS, health events, time interval between calving and blood sample, and the farm-level factors of diet Ca, Mg, and CP content, dietary P intake, supply of vitamin D from commercial mixes, weighing practices, and AcD or StD group, with farm as a random effect. For the first model (SHC), we defined SHC using a plasma Ca cut-off of 2.0 mmol/l based on the reference range of values in the Merck Veterinary Manual. All variables were first tested for linear relationship with the logarithmic odds of being with SHC and SHP respectively. If the assumption of linearity was rejected, variables were then described using thresholds determined either by biological assumptions regarding the amounts that influence postpartum blood Ca or with the goal of balancing the number of cows in different categories. For example, P, Mg, and Ca intake were classified based on biological assumptions. Mg intake was categorized as a binary variable with a cut-off of 3.5 g/kg DM, as higher levels stimulate passive absorption of Mg across the rumen wall (NRC, 2001). P intake was categorized as a tertiary variable with cut-offs of 40 and 60 g/day, because a P intake of 40 g/day provides sufficient phosphorus even when dry matter intake is low around calving (NRC, 2001) and a P intake higher than 60 g/day affects blood calcium at calving (Peterson et al., 2005). Ca intake was classified using a cut-off of 6 g/kg DM, which corresponds to the requirements for a cow in late gestation (Inra, 2018). As authors did not have such biological assumption for vitamin D or CP, these variables were categorized as binary variables with a cut-off corresponding to their median value. Then, each variable of interest was included in the model if univariate analysis with a random effect was significant at P = 0.20. Exceptions were made in the SHC model for intake of dietary Ca, P, and Mg and vitamin D supply, which were considered important variables a priori and were included in the SHC model regardless of the outcome of the univariate analyses. Similarly, the SHP model always included dietary P and vitamin D, along with SHC status. We checked for collinearity among variables by ensuring that all variables included in the model had a variance inflation factor (VIF) < 5. The models were fitted using backward stepwise elimination of potential variables that were considered non-significant (P > 0.10 and < 20% effect on the estimates of other variables of interest when removed). The final fitted models were tested for normality and homoscedasticity of residuals, and for normality of random effect. All statistical analyses were conducted in R (https://www.r-project.org/).
Results
Descriptive results from study sample
Our original study design included 32 farms, but six farmers (three from AcD group and three from StD group) withdrew from the study midway because of COVID restriction measures. Of the remaining 26 farms, 15 were in the AcD group and 11 in the StD group. In total, 371 cows were followed, with an average of 14.3 ± 7 cows per farm (range: 1 to 22 cows per farm). Among the 371 cows, 235 received the AcD treatment, and 136 were fed the StD control. Subclinical hypocalcemia (< 2 mmol/l) was identified in 35%, 45%, 67%, and 75% of cows of parity 1, 2, 3, and ≥ 4, respectively, and in 58% and 49% of cows in the AcD and StD groups, respectively (Table 1). Milk fever was diagnosed in 15 cows (4%), all multiparous. Subclinical hypophosphatemia was detected in 30%, 31%, 46%, and 43% of cows of parity 1, 2, 3, and ≥ 4, respectively, and in 33% and 43% of cows in the AcD and StD groups, respectively.
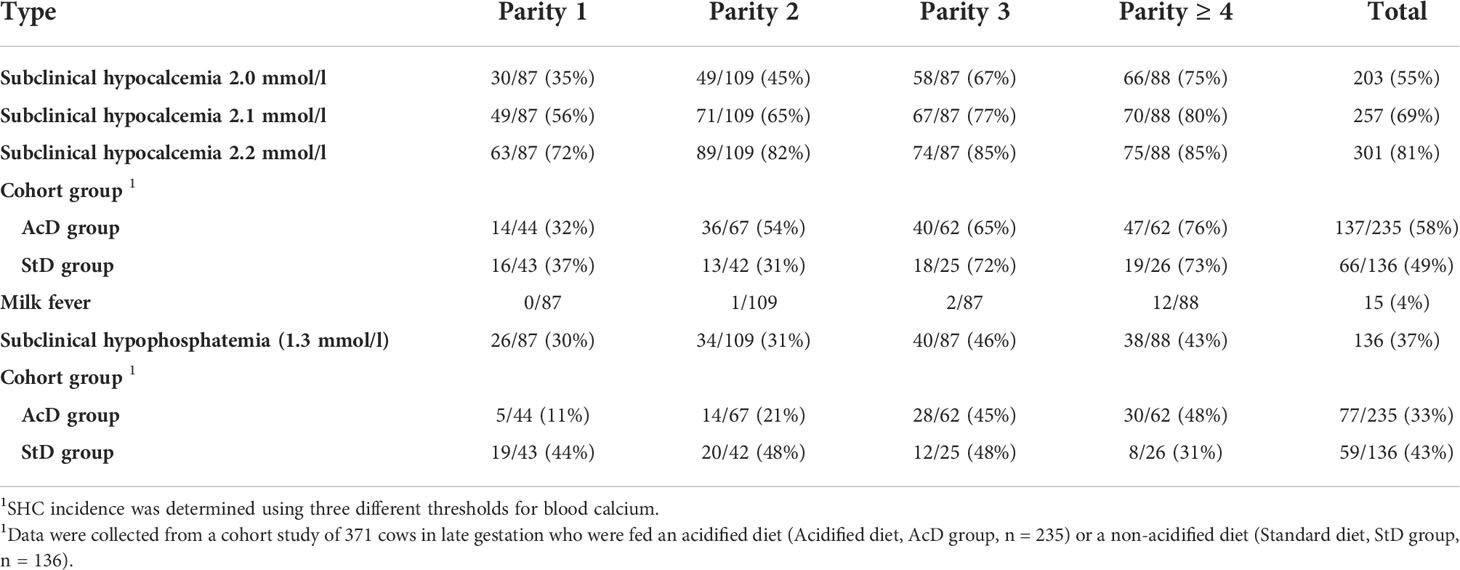
Table 1 Incidence of subclinical hypocalcemia (SHC), milk fever, and subclinical hypophosphatemia (SHP) in cows 24 to 48 h after parturition (no./total; % in parentheses) presented according to parity and the type of diet (acidified or standard) received in late gestation (n = 371 cows).
Quantitatively, diet DCAD values were lower in the AcD group than in the StD group (66 ± 78 and 108 ± 45 mEq/kg DM, respectively, P < 0.001), but levels in the AcD group were high compared to the expected negative values (Table 2). Only 37 cows received a diet for which DCAD was below -50 mEq/kg of DM and only 55 cows received a diet with a DCAD between -50 and 0 mEq/kg DM (Table 3). The intake declared by farmers exceeded the predicted feed intake capacity for 19 of the 52 different diets in the study (11 farms concerned, 11 cow diets and 8 heifer diets). The estimated intake of Ca, P, and Mg from commercial mixes was higher and had a higher variability than that from forage. For instance, Mg intake was estimated as 28.7 ± 13.2 g/day for commercial mixes and 13.1 ± 3.3 g/day for forage (Table 2). Dietary phosphorus intake was higher in the StD group than in the AcD group (49.4 ± 24.9 and 44 ± 19 g/day, respectively, P = 0.02), while dietary magnesium intake was lower in the StD group than in the AcD group (3.1 ± 0.5 and 3.7 ± 1.2 g/kg DM, respectively, P < 0.001). There was no difference in either dietary calcium intake or vitamin D supply between the StD and AcD groups (5.1 ± 1.4 and 5.5 ± 2.3 g/kg DM of Ca, respectively, P = 0.5; 18828 ± 4802 and 21135 ± 10824 IU/day for vitamin D, respectively, P = 0.3).
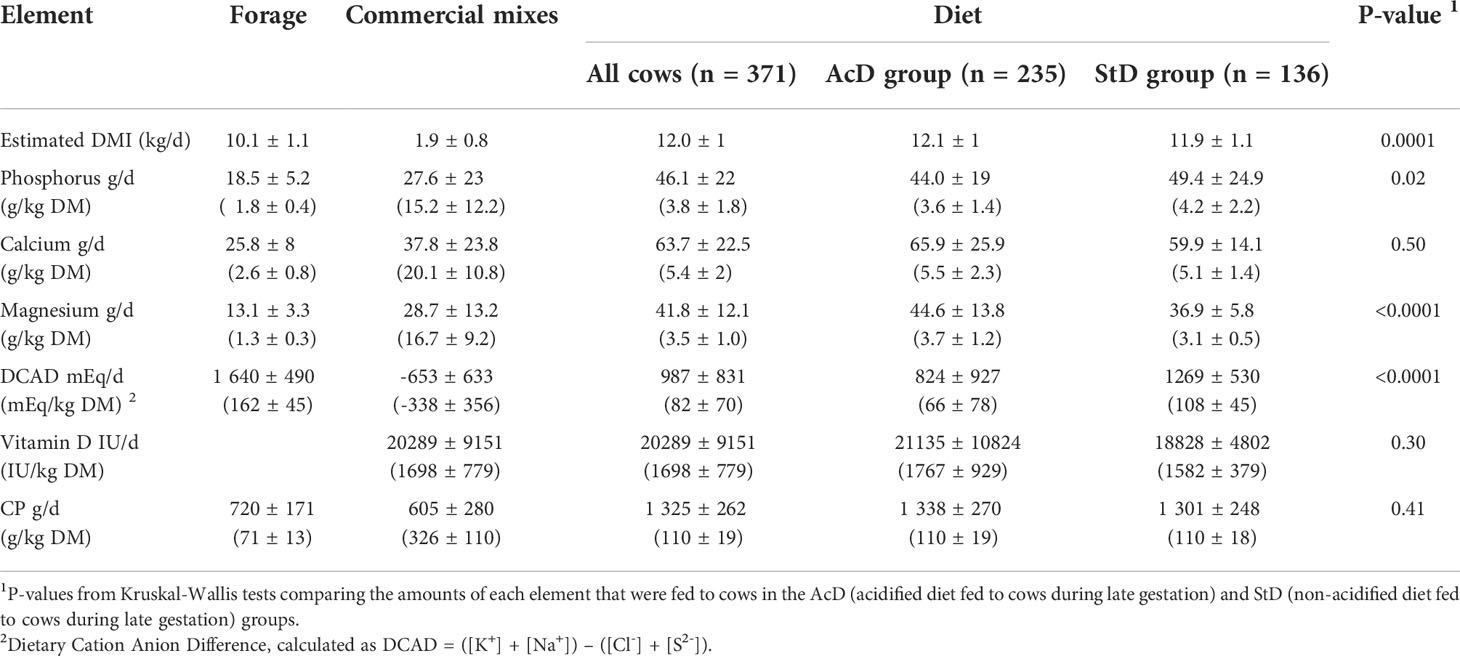
Table 2 Estimates of dry matter intake (DMI), mineral elements, vitamin D, and crude protein (CP) intake from forage, commercial mixes, and diet treatment for the 371 cows included in the cohort study.
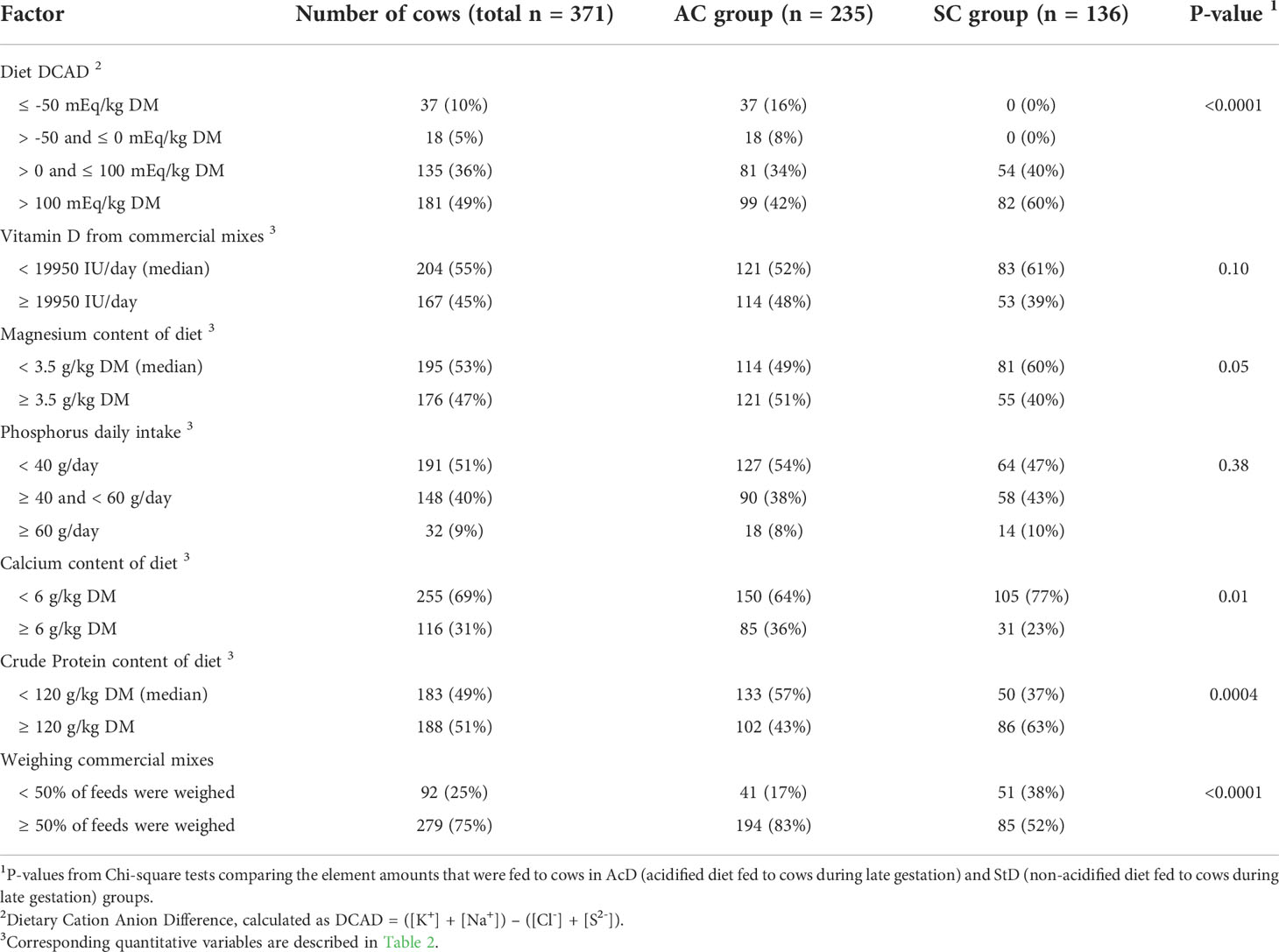
Table 3 Description of cow-level variables related to element amounts and tested for inclusion in the subclinical hypocalcemia (SHC) and subclinical hypophosphatemia (SHP) models. Variables were measured as part of a cohort study of 371 dairy cows.
Farmers in the AcD group were much more likely to weigh commercial mixes (≥ 50% of ingredients) than those in the StD group (83% and 52%, respectively, P < 0.0001; Table 3). Preventive practices around the time of calving were implemented in relatively few cases: incomplete first milking was used for only 10.8% of cows, and calcium supplementation at calving was given to 9.2% (Table 4). No cow was injected with vitamin D before calving. Thirty-nine cows (10.5%) experienced some kind of disease event between calving and blood sample collection, with the most common being milk fever (15.5%), which occurred with equal frequency in both the StD and AcD groups (5% and 3%, respectively, P = 0.27). Blood samples were collected by farm veterinarians at 31 ± 12 hours after calving. Proportions of bloods collected < 24 h and ≥ 48h were smaller in AcD than in StD group (19% and 7% for AcD group, respectively, 30% and 10% for ScD group, respectively, P = 0.01). Compared to the AcD group, members of the StD group were more likely to have a BCS higher than 3.5 at the time of calving (7% and 17%, respectively, P = 0.007). Distributions of milk yield index categories did not differ between groups (P = 0.52).
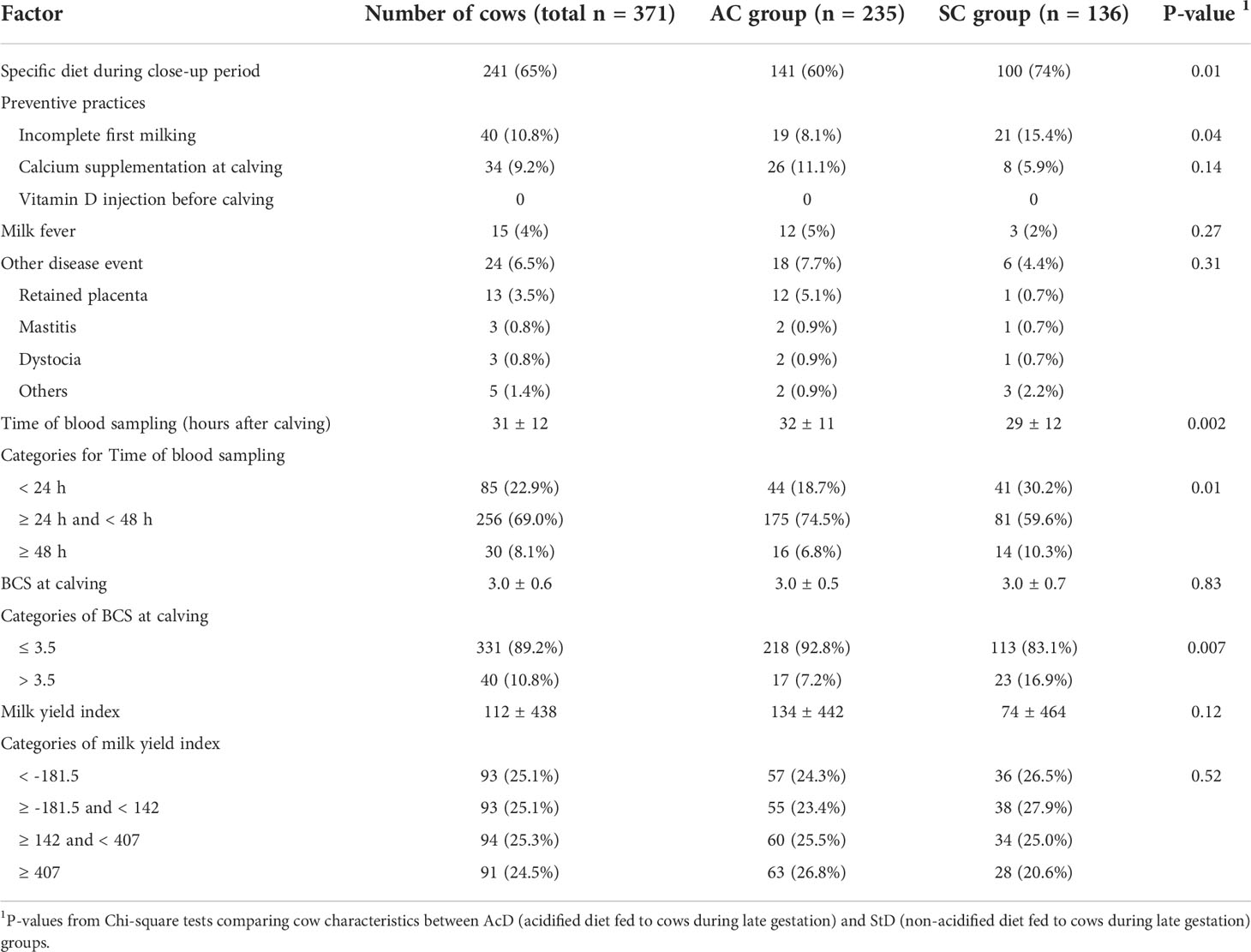
Table 4 Description of cow characteristics tested for inclusion in the subclinical hypocalcemia (SHC) and subclinical hypophosphatemia (SHP) models. Variables were measured as part of a cohort study of 371 dairy cows.
Subclinical hypocalcemia model
The results of the univariate analyses are summarized in Table 5. Disease events, the use of incomplete first milking, and the time interval between calving and blood sample collection were not included in the model because the univariate relationship was not significant at P = 0.2. Moreover, none of the univariate analyses revealed any interactions between the tested variables and SHC.
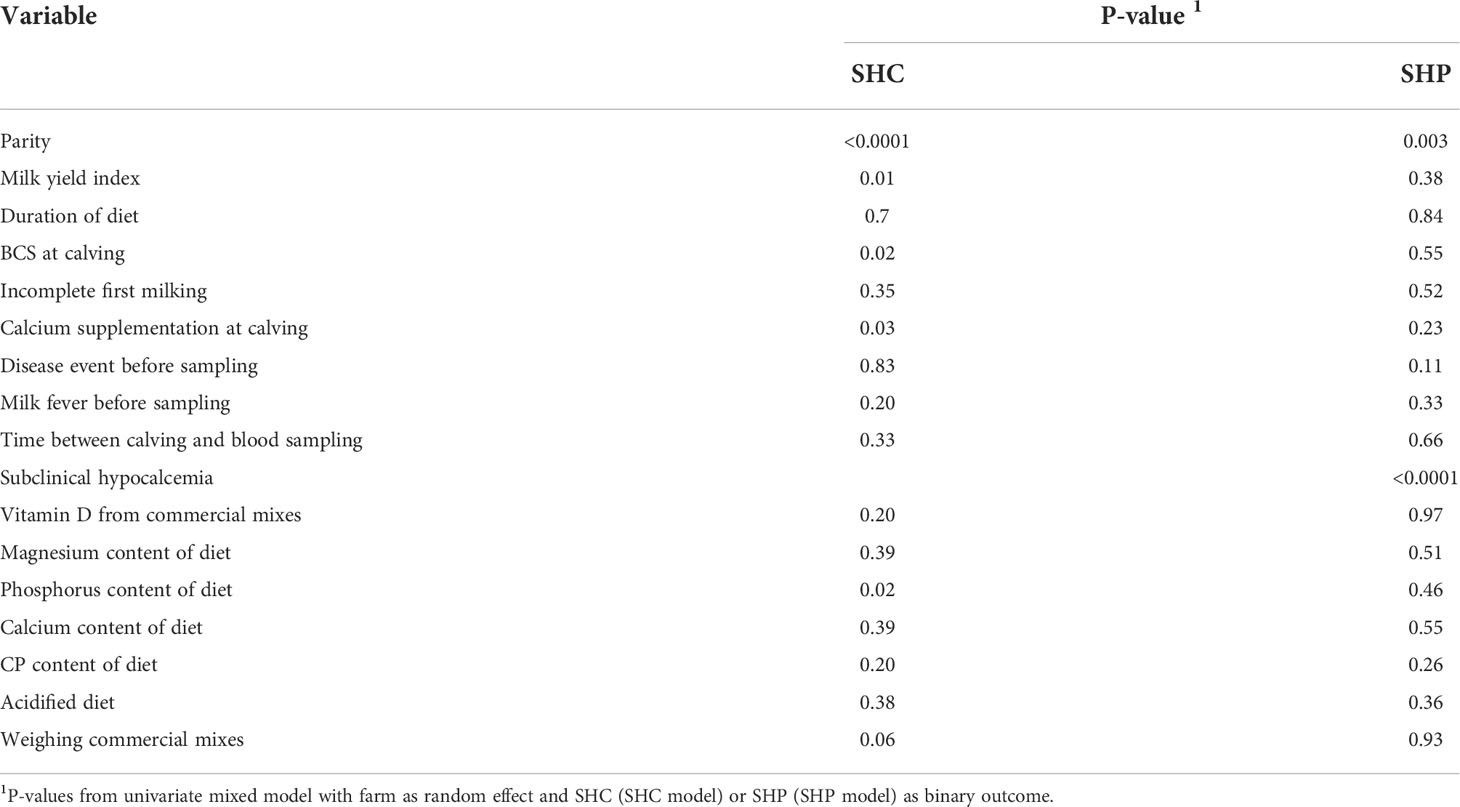
Table 5 Results from univariate tests quantifying the effects of parity, milk yield index and feeding practices during dry off on occurrence of subclinical hypocalcemia (SHC) or subclinical hypophosphatemia (SHP) (n = 371 cows).
The final fitted model included the following variables: diet group (AcD/StD), dietary phosphorus intake (g/day), diet magnesium content (g/kg DM), dietary vitamin D supply (IU/day), diet calcium content (g/kg DM), diet CP content (g/day), the practice of weighing commercial mixes, parity, milk yield index, and BCS. Although some of these variables by themselves did not have a significant effect on SHC in the model, they were not excluded so as to not alter the parameter estimates for significant effects. The acidified diet (AcD) did not affect SHC risk. A higher magnesium intake (≥ 3.5 g/kg DM) reduced SHC risk (odds ratio (OR) = 0.5, P = 0.04), while high phosphorus intake sharply increased SHC risk: cows fed between 40 and 60 g P per day were 3.5 times more likely to have SHC than those who received less than 40 g per day, and cows fed ≥ 60 g/day were 7.3 times more likely to have SHC (Table 6). The risk of subclinical hypocalcemia gradually increased with parity, especially for parities 3 and higher (OR = 3 for 3rd parity and OR = 5.9 for parity ≥ 4, P < 0.0001). Calcium content (< or ≥ 6 g/kg DM) and CP content (< or ≥ 120 g/kg DM) did not affect SHC risk. Higher values for milk yield index (≥ 142) were associated with a gradually increased risk of SHC (OR = 2.3 for index between 142 and 407 and OR = 3.4 for index ≥ 407, P = 0.0005), as were higher body condition scores at calving (BCS ≥ 3.5, OR = 2.6, P = 0.04). Finally, SHC risk was also higher for cows who received higher amounts of vitamin D from commercial mixes (≥ 19950 IU/day, OR = 3, P = 0.007).
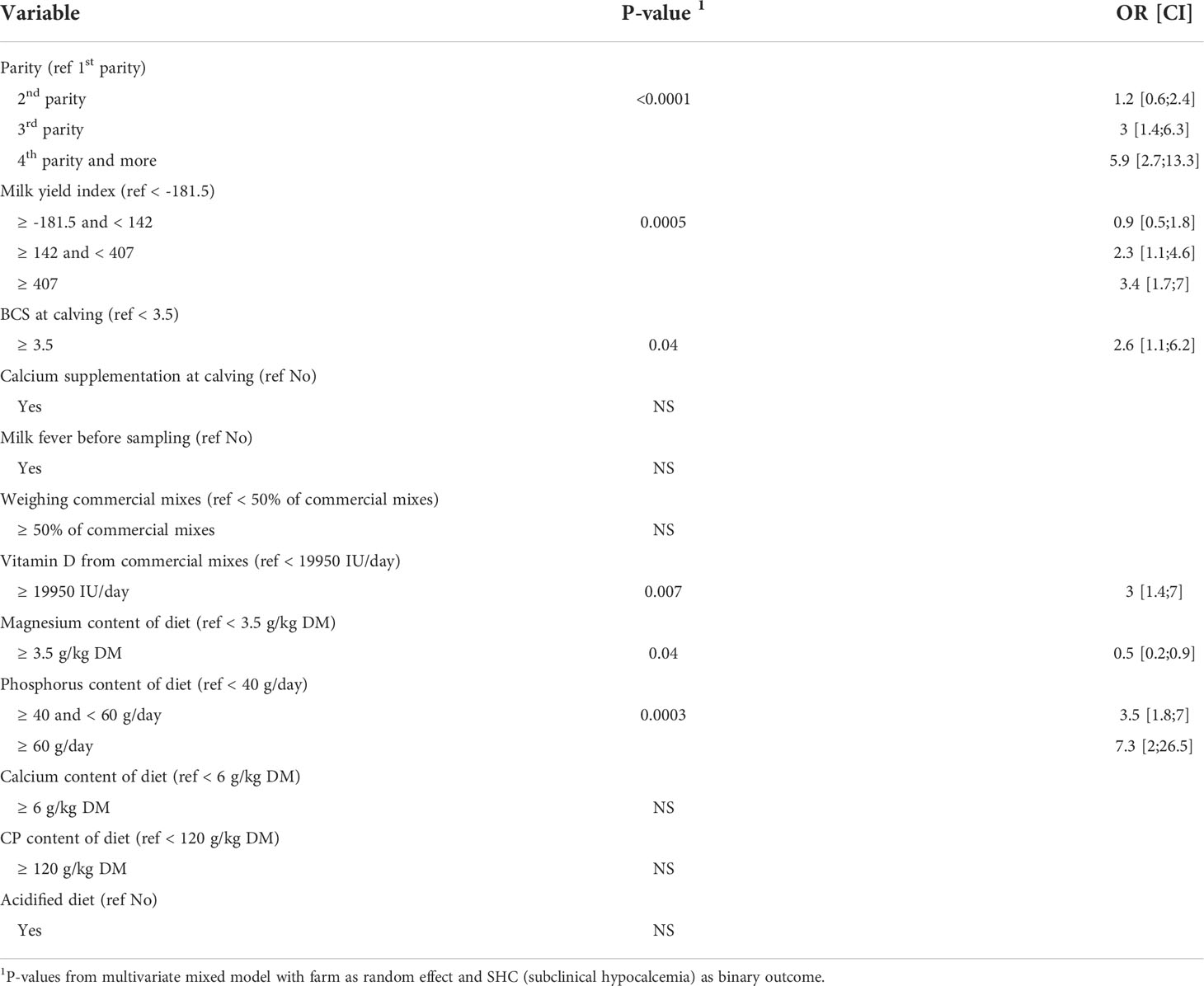
Table 6 Results from multivariate model quantifying the effects of parity, milk yield index and feeding practices during dry off on occurrence of subclinical hypocalcemia (SHC), P-values, odds ratios (OR) and associated confidence intervals (CI) (n = 371 cows).
Subclinical hypophosphatemia model
The results of the univariate analyses are summarized in Table 5. The final model did not include the time interval between calving and blood sample collection or the occurrence of milk fever or incomplete first milking because these univariate models were not significant at P = 0.2. As for SHC, no significant interaction was detected in the univariate analyses between any variable and SHP. The final fitted model included the following variables: diet group (AcD/StD), dietary vitamin D supply (IU/day), phosphorus intake (g/day), the practice of weighing commercial mixes, calcium supplementation at calving, disease events, SHC status, parity, and milk yield index. Not all of these variables had a significant effect on SHP in the model, but they were not excluded so as to not alter parameter estimates for significant effects. The acidified diet (AcD) tended to decrease the risk of SHP (OR = 0.4, P = 0.07), as did intermediate amounts of dietary phosphorus (between 40 and 60 g/d, OR = 0.4, P = 0.10) (Table 7). Subclinical hypocalcemia increased SHP risk (OR = 3.1, P < 0.0001), along with higher parity (OR = 2.9 for 3rd parity and OR = 2.3 for parity ≥ 4, P = 0.03).
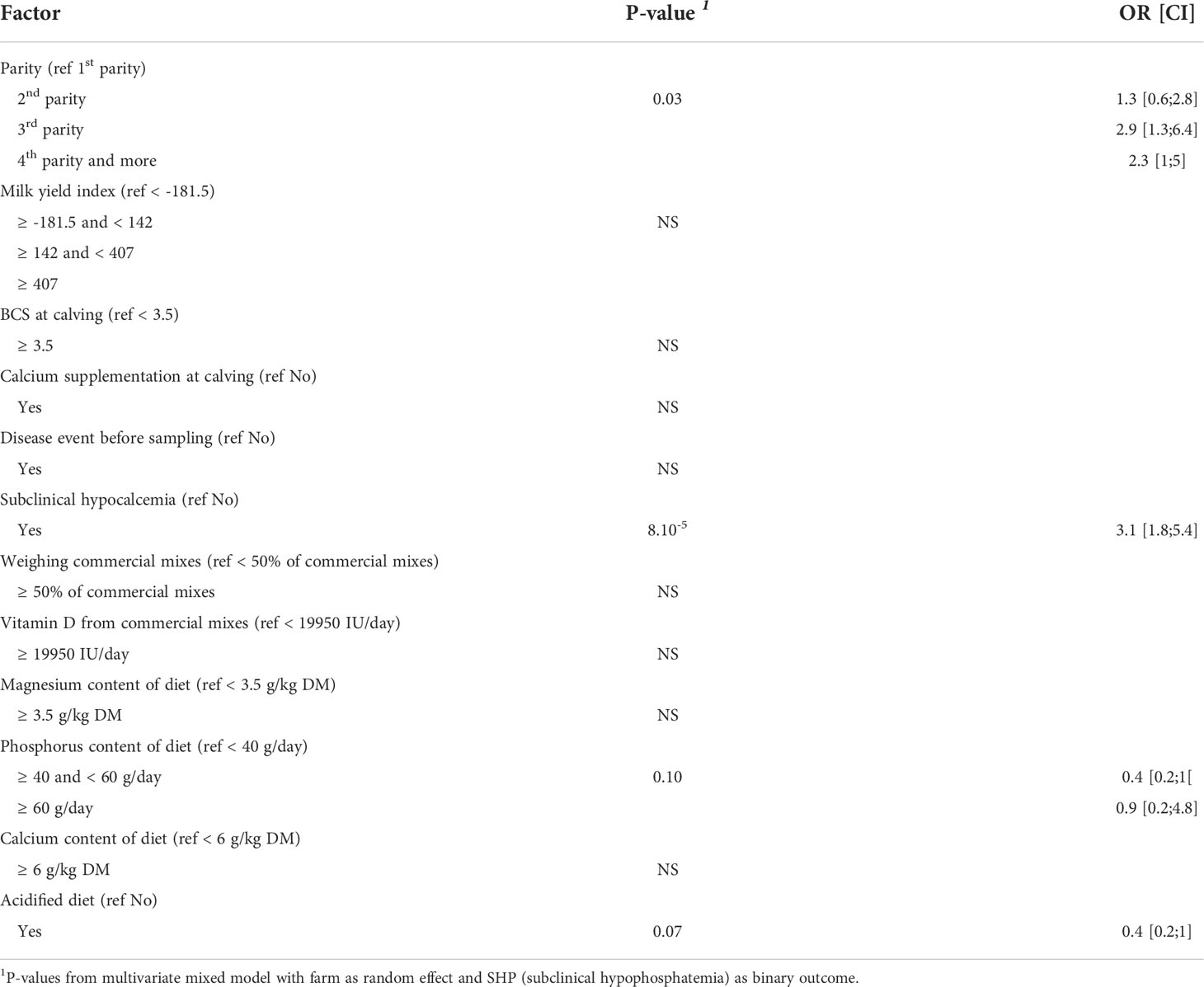
Table 7 Results from multivariate model quantifying the effects of parity, milk yield index and feeding practices during dry off on occurrence of subclinical hypophosphatemia (SHP), P-values, odds ratios (OR) and associated confidence intervals (CI) (n = 371 cows).
Discussion
This study reveals that the declared use of an acidified diet in the last three weeks of gestation (the close-up period) had no significant effect on the incidence of SHC under typical field conditions on French dairy farms. However, we did observe effects linked to the dietary content of certain vitamins and minerals. Of the 15 farmers in our study who used an acidified diet, only three (corresponding to 37 cows) managed to achieve diet DCAD values below -50 mEq/kg DM, which is the threshold recommended by Goff and Horst (1997) for inducing metabolic acidosis and thus preventing hypocalcemia. Recent experimental trials have suggested that less-negative values (diet DCAD between -10 to -20 mEq/kg DM) could also have preventive effects (Diehl et al., 2018; Goff and Koszewski, 2018), but the typical diet DCAD values obtained here in the AcD group (66 ± 78 mEq/kg DM) were much too high to expect any preventive effects. This discrepancy between theoretical recommendations and practical implementations is likely the main reason why the AcD program was ineffective in reducing SHC in the study population. Indeed, the incidence of SHC in this study was 55%, which is higher than the 39% reported by Reinhardt et al. (2011), or the 41% found in a prevalence study recently performed by Venjakob et al. in Germany (Venjakob et al., 2017). Even when we broke the numbers down by parity groups, SHC incidence remained higher than previously reported values. However, it is difficult to compare between studies due to differences in experimental design: for example, only 8.7% of farmers from the Venjakob study used acidified diets to prevent hypocalcemia in comparison to 58% (15 of 26 farms) in our study. Factors other than diet acidification could explain the different frequencies obtained in our study and that of Venjakob et al., such as cow breed or Ca, P, Mg, and vitamin D intake. Nevertheless, one of the main findings of this work is that diet acidification is challenging to carry out under real-world context of small dairy farms, and our results highlight the need to provide French farmers with clear and effective guidance for the correct implementation of this preventive strategy.
Because there is no consensus in the literature on the best cut-off for defining SHC (Couto Serrenho et al., 2021), we assessed the effect of the AcD treatment on SHC using a threshold of plasma Ca < 2.0 mmol/l, which was based on the reference range of values in the Merck Veterinary Manual. Ideally, the threshold for defining SHC should be based on measured effects on outcomes of interest (Couto Serrenho et al., 2021), such as disease incidence, reproductive performance, or culling risk. However, this approach can lead to the use of different thresholds for different parameters, depending on the outcome of interest, cow parity, and the time of sampling. As a result, the threshold for defining SHC could range widely, from 1.77 mmol/l to 2.3 mmol/l depending on the parameter of interest (Roberts et al., 2012; McArt and Neves, 2019).
Increasing dietary P during the prepartum period increased SHC risk, but with a non-significant dose effect. This result is consistent with the literature, as feeding a diet high in P has been demonstrated to negatively influence blood calcium in the week after calving (Peterson et al., 2005; Cohrs et al., 2018; Keanthao, 2021; Wächter et al., 2021). However, levels of dietary P in the “at risk” categories in our study (medium and high dietary P, i.e., 40–60 g/day and ≥ 60 g/day) were higher than the high dietary P treatments of the abovementioned experiments. Thus, effect of prepartum dietary P on hypocalcemia incidence needs to be assessed in field conditions using a wide range of dietary P, including values below 0.2% of DM, i.e., 24 g/day for a cow consuming 12 kg DM/day.
Subclinical hypophosphatemia was identified in 37% of the cows of our study, which is not greatly different from the 55% incidence of SHC. Only two published studies have assessed hypophosphatemia incidence, reporting values of 15% (Macrae, 2012) and 55% (Staufenbiel, 2002); the large difference is likely due, at least in part, to the fact that the two studies used different thresholds to define SHP. Tsiamadis et al. (2016) highlighted that SHP was more frequent at day four after calving, which means that our study, which sampled blood at 31 ± 12 h after calving, could have underestimated the true incidence of postpartum hypophosphatemia. Regardless, the frequencies reported in this and previous studies indicate that hypophosphatemia may be a common problem in dairy herds that merits further attention.
Although diet acidification had no effect on SHC incidence in this study, we observed a trend for a preventive effect on SHP incidence. Overall, published data on the effects of an acidified diet on hypophosphatemia are inconsistent. Grünberg et al. (2011) did not observe any effect of a negative-DCAD diet (-90 mEq/kg DM) on postpartum plasma Pi, while other studies have reported significant preventive effects (Weiss et al., 2015; Martinez et al., 2017; Lean et al., 2019). However, in some cases, it was not always possible to discriminate between the effects of DCAD and those of vitamin D (Weiss et al., 2015; Martinez et al., 2017). Here, though, the wide range of diet DCAD and vitamin D intake in our study, especially in the AcD group, prevented the risk of confusion. Theoretically, we might have expected that the acidified diet would prevent hypophosphatemia through the activation of PTH and the consequent effects on the regulation of both Ca and P metabolism. However, the absence of any effects on hypocalcemia incidence in our study means that we must seek other explanations.
Although a P intake between 40 and 60 g/day had negative effects on SHC incidence in the current study, it did have preventive effects on SHP incidence, as expected from the literature (Kichura et al., 1982; Barton, 1987; Peterson et al., 2005; Cohrs et al., 2018; Wächter et al., 2021). Peterson et al. (2005) demonstrated that cows fed a 0.21% P diet had lower serum Pi than cows fed a 0.31% or a 0.44% P diet from 7 days prepartum to the day of calving. Likewise, Wächter et al. (2021) highlighted a decline in plasma Pi in cows on a 0.16% P diet in comparison to a 0.30% P diet between day 4 before parturition to day 1 after parturition. Keanthao (2021) reported more complex relationships between dietary P and plasma Pi after calving, which depended on both prepartum and postpartum dietary P. Overall, the opposing effects of dietary P on hypocalcemia on the one hand and hypophosphatemia on the other make it challenging for nutritionists to provide recommendations to farmers in the field. The consequences of SHC on health and reproduction have been widely studied worldwide, but those of SHP remain uncertain. Its detrimental effects on immunity have been demonstrated (Mullarky et al., 2009; Eisenberg et al., 2014; Eisenberg et al., 2019), but epidemiological studies quantifying the consequences regarding disease incidence and reproductive performance are lacking. Such studies are sorely needed to assess the need to take hypophosphatemia into consideration when implementing hypocalcemia prevention programs.
The preventive effect of high dietary Mg on the incidence of milk fever is well documented (Lean et al., 2006; Kronqvist et al., 2012), but our study is the first to demonstrate its effect on SHC incidence in field conditions. The result we observed is consistent with the literature, as Mg enhances tissue responsiveness to PTH and indirectly stimulates PTH secretion (Goff, 2014), and thus indirectly increases blood Ca. Moreover, the amount of dietary Mg in our study that was associated with lower SHC risk (≥ 3.5 g/kg DM) is close to the 0.4% that was associated with a 62% reduction in milk fever risk in the meta-analysis of Lean et al. (2006). The high degree of variability in Mg supply in this study was largely due to Mg supplementation with the anionic salt magnesium chloride, which has an absorption coefficient [90% (NRC, 2001)] that is higher than that of other mineral sources of Mg. Thus, diet acidification could have enhanced the preventive effect of Mg through the improved absorption of magnesium chloride. However, even if levels of dietary Mg were higher in the AcD group than in the StD group, the group means were not very different and within-group variabilities were high. This indicates that variability in Mg supply was relatively high regardless of anionic salts supplementation.
The current study does not support the hypothesis that increasing dietary Ca intake increases postpartum SHC risk (Lean et al., 2006; Santos et al., 2019), because a higher-Ca diet (more than 6 g/kg DM) was not associated with any increase in hypocalcemia risk. Santos et al. (2019) reported an increase in the risk of milk fever with a dietary calcium content of 1.6–19.8 g/kg DM, and the risk was more pronounced when the diet DCAD was negative. Only a few cows in our study experienced such acidified conditions, which complicates any attempt to compare our results with theirs. In addition, despite the large number of studies included in their meta-analysis, only a few examined diets with low calcium content. In our study, daily calcium intake (63.7 ± 22.5 g/day) was close to standard requirements for cows in late gestation (Inra, 2018) and had relatively low variability, making it difficult to assess its effects on SHC risk. In the same way, although higher-than-recommended Ca intake has been associated with reduced absorption of P in the intestine (NRC, 2001), the range of Ca intake in our study was probably too low to assess this relationship and the subsequent effect of Ca intake on SHP risk. The effect of prepartum dietary Ca on SHP must be further investigated in the field using a larger range of dietary Ca, which illustrates once again the challenge of confirming results from experimental studies in real-world conditions.
Our study revealed a strong detrimental effect on SHC of increasing the prepartum supply of vitamin D above 19 950 IU/day. Some authors have recommended supplementing dry cows with 20 000 to 35 000 IU/day of vitamin D in order to increase intestinal Ca absorption (Goff, 2014; Wilkens and Muscher-Banse, 2020). However, vitamin D supplementation also activates negative feedback processes (Martín-Tereso and Martens, 2014): the increase in blood Ca induced by higher intestinal absorption leads to PTH inhibition. Moreover, vitamin D supplementation can also induce soft tissue calcification. For this reason, Wilkens et al. (2012) emphasized that the preventive effects of vitamin D are determined by the degree of acidification of the prepartum diet, which can maintain PTH activity despite the vitamin D–induced feedback. As diet acidification was insufficient in most of the AcD farms in our study, we could not expect to find any preventive effect of vitamin D on SHC. Furthermore, vitamin D supplementation had no effect on the incidence of SHP in our study sample. This was not altogether surprising given that the ability of vitamin D to regulate intestinal P absorption has been questioned (Wilkens and Muscher-Banse, 2020), and its real implications for hypophosphatemia prevention remain controversial (Grünberg, 2014).
Calcium supplementation at calving had no significant preventive effect on SHC in our study. This could be due to a lack of statistical power, as only 34 cows (9.2%) received such supplementation. However, it could also be the result of the fleeting effect of this prophylactic treatment: calcium supplementation is usually performed immediately after calving and its effect on blood Ca level lasts for only few hours (Blanc et al., 2014; Martinez et al., 2016; Leno et al., 2018). Our blood samples were collected at 31 ± 12 h, which may have been too late to detect any effects. Preventive treatments, such as calcium supplementation or incomplete first milking, were probably focused on cows whose SHC risk seemed higher to farmers, such as cows of higher parity or those who had previously experienced milk fever. As SHC is far more frequent than milk fever and concerns every parity group, it could be helpful for farmers to have tests at their disposal for the identification of cows with SHC who might benefit from prophylactic treatment. This could also be useful in assessing the effectiveness of preventive programs. Because of the presumed strong genetic influence on SHC risk (milk yield effect), such tests could also help to characterize phenotypes and assist with genomic selection for SHC resistance.
This cohort study revealed that nutritional strategies aimed at preventing hypocalcemia were ineffective in the real-world context of small dairy farms in western France, leading to a high incidence of hypocalcemia in this population. Guidelines and recommendations regarding vitamin and mineral intake during late gestation must be improved and strengthened in order to increase their value in the field; to achieve this, better collaboration among researchers, nutritionists, and farmers is required. Additionally, recommendations regarding the administration of minerals to dry cows should take into consideration their effects on hypophosphatemia risk. To this end, further research is required on the prevalence of hypophosphatemia and its consequences for health and reproductive performance.
Data availability statement
The raw data supporting the conclusions of this article will be made available by the authors, without undue reservation.
Ethics statement
The animal study was reviewed and approved by ONIRIS ethics committee for clinical and epidemiological veterinary research under decision CERVO-2019-3-V. Written informed consent was obtained from the owners for the participation of their animals in this study.
Author contributions
TA: Conceptualization, Methodology, Formal analysis, Investigation, Writing - Original Draft, Funding acquisition. AB: Conceptualization, Methodology, Writing - Review & Editing, Funding acquisition. RG: Conceptualization, Methodology, Writing - Review and Editing, Visualization, Supervision, Funding acquisition. All authors contributed to the article and approved the submitted version.
Funding
This study was supported by DELTAVIT, Cargill, TECHNA, VILOFOSS, Innoval and INRAE.
Acknowledgments
The authors thank Maxime Pocher and Alexandre Gallais (ONIRIS) for participating in the study; Alexandre Gallais, Nadine Brisseau (ONIRIS) and Anne Lehébel (INRAE BIOEPAR) for contributing to data analysis; Maryline Lemarchand, Cécile Perrier, and Arnaud Mottin (INRAE PEGASE) for sample analysis and chemical analyses, and Vincent Ballard (CCPA), Alexandre Budan (Cargill), Lionel Raulin (TECHNA), and Benoît Astruc (VILOFOSS) for their input on the study design.
Conflict of interest
The authors declare that the research was conducted in the absence of any commercial or financial relationships that could be construed as a potential conflict of interest.
Publisher’s note
All claims expressed in this article are solely those of the authors and do not necessarily represent those of their affiliated organizations, or those of the publisher, the editors and the reviewers. Any product that may be evaluated in this article, or claim that may be made by its manufacturer, is not guaranteed or endorsed by the publisher.
References
AFNOR (2017). Aliments pour animaux: Méthodes d’échantillonnage et d’analyse - dosage du calcium, du sodium, du phosphore, du magnésium, du potassium, du soufre, du fer, du zinc, du cuivre, du manganèse et du cobalt après digestion sous pression par ICP-AES. norme NF (Saint-Denis, France: AFNOR), 41 p.
Barton B. A. (1987). Impact of prepartum dietary phosphorus intake on calcium homeostasis at parturition 1. J. Dairy Sci. 70, 1186–1191. doi: 10.3168/jds.S0022-0302(87)80130-3
Bazin S. (1984). Grille de notation de l’état d’engraissement des vaches pie noires, (Paris, France: I.T.E.B.) Vol. 31.
Blanc C. D., van der List M., Aly S. S., Rossow H. A., Silva-del-Río N. (2014). Blood calcium dynamics after prophylactic treatment of subclinical hypocalcemia with oral or intravenous calcium. J. Dairy Sci. 97, 6901–6906. doi: 10.3168/jds.2014-7927
Brochard M., Boichard D., Ducrocq V., Fritz S. (2013). La sélection pour des vaches et une production laitière plus durables: Acquis de la génétique et opportunités offertes par la sélection génomique. Prod Anim. 26, 145–156. doi: 10.20870/productions-animales.2013.26.2.3144
Cohrs I., Wilkens M. R., Grünberg W. (2018). Short communication: Effect of dietary phosphorus deprivation in late gestation and early lactation on the calcium homeostasis of periparturient dairy cows. J. Dairy Sci. 101, 9591–9598. doi: 10.3168/jds.2018-14642
Couto Serrenho R., DeVries T. J., Duffield T. F., LeBlanc S. J. (2021). Graduate student literature review: What do we know about the effects of clinical and subclinical hypocalcemia on health and performance of dairy cows? J. Dairy Sci 104(5):6304–6326. doi: 10.3168/jds.2020-19371
Diehl A. L., Bernard J. K., Tao S., Smith T. N., Kirk D. J., McLean D. J., et al. (2018). Effect of varying prepartum dietary cation-anion difference and calcium concentration on postpartum mineral and metabolite status and milk production of multiparous cows. J. Dairy Sci. 101, 9915–9925. doi: 10.3168/JDS.2018-14828
Eisenberg S. W. F., Ravesloot L., Koets A. P., Grünberg W. (2014). Influence of feeding a low-phosphorus diet on leucocyte function in dairy cows. J. Dairy Sci. 97, 5176–5184. doi: 10.3168/jds.2014-8180
Eisenberg S. W. F., Ravesloot L., Koets A. P., Grünberg W. (2019). Effect of dietary phosphorus deprivation on leukocyte function in transition cows. J. Dairy Sci. 102, 1559–1570. doi: 10.3168/jds.2018-15417
Goff J. P. (2008). The monitoring, prevention, and treatment of milk fever and subclinical hypocalcemia in dairy cows. Vet. J. 176, 50–57. doi: 10.1016/J.TVJL.2007.12.020
Goff J. P. (2014). Calcium and magnesium disorders. Vet. Clin. North Am. - Food Anim. Pract. 30, 359–381. doi: 10.1016/j.cvfa.2014.04.003
Goff J. P., Horst R. L. (1997). Effects of the addition of potassium or sodium, but not calcium, to prepartum ratios on milk fever in dairy cows. J. Dairy Sci. 80, 176–186. doi: 10.3168/jds.S0022-0302(97)75925-3
Goff J. P., Koszewski N. J. (2018). Comparison of 0.46% calcium diets with and without added anions with a 0.7% calcium anionic diet as a means to reduce periparturient hypocalcemia. J. Dairy Sci. 101, 5033–5045. doi: 10.3168/jds.2017-13832
Grünberg W. (2014). Treatment of phosphorus balance disorders. Vet. Clin. North Am. - Food Anim. Pract. 30, 383–408. doi: 10.1016/j.cvfa.2014.03.002
Grünberg W., Donkin S. S., Constable P. D. (2011). Periparturient effects of feeding a low dietary cation-anion difference diet on acid-base, calcium, and phosphorus homeostasis and on intravenous glucose tolerance test in high-producing dairy cows. J. Dairy Sci. 94, 727–745. doi: 10.3168/jds.2010-3230
Inra (2018). Alimentation des ruminants. editions quae (Versailles, France: Editions Quae), 728p. doi: 10.3920/978-90-8686-292-4
Institut de l’Elevage (2019). Dossiers economie de l’élevage n° 502: Europe du nord filière laitière (Paris, France: Technipel). Available at: https://idele.fr/?eID=cmis_download&oID=workspace://SpacesStore/a943c2a2-7b22-49c3-a626-70dc7c84bc1c.
Keanthao P., Goselink R. M. A., Dijkstra J., Bannink A., Schonewille J. T. (2021). Effects of dietary phosphorus concentration during the transition period on plasma calcium concentrations, feed intake, and milk production in dairy cows. J Dairy Sci. 104 (11), 11646–11659. doi: 10.3168/jds.2021-20488
Kichura T. S., Horst R. L., Beitz D. C., Littledike E. T. (1982). Relationship between prepartal dietary calcium and phosphorus, vitamin d metabolism, and parturient paresis in dairy cows. J. Nutr. 112, 480–487. doi: 10.1093/jn/112.3.480
Kronqvist C., Emanuelson U., Travén M., Spörndly R., Holtenius K. (2012). Relationship between incidence of milk fever and feeding of minerals during the last 3 weeks of gestation. Animal 6(8) 1316–1321. doi: 10.1017/S175173111200033X
Lean I. J., DeGaris P. J., McNeil D. M., Block E. (2006). Hypocalcemia in dairy cows: Meta-analysis and dietary cation anion difference theory revisited. J. Dairy Sci. 89, 669–684. doi: 10.3168/JDS.S0022-0302(06)72130-0
Lean I. J., Santos J. E. P., Block E., Golder H. M. (2019). Effects of prepartum dietary cation-anion difference intake on production and health of dairy cows: A meta-analysis. J. Dairy Sci. 102, 2103–2133. doi: 10.3168/JDS.2018-14769
Leno B. M., Martens E. M., Felippe M. J. B., Zanzalari K. P., Lawrence J. C., Overton T. R. (2017). Short communication: Relationship between methods for measurement of serum electrolytes and the relationship between ionized and total calcium and neutrophil oxidative burst activity in early postpartum dairy cows. J. Dairy Sci. 100(11):9285–9293. doi: 10.3168/jds.2017-12971
Leno B. M., Neves R. C., Louge I. M., Curler M. D., Thomas M. J., Overton T. R., et al. (2018). Differential effects of a single dose of oral calcium based on postpartum plasma calcium concentration in Holstein cows. J. Dairy Sci. 101, 3285–3302. doi: 10.3168/jds.2017-13164
Macrae A. I. (2012). Assessment of nutrition in dairy herds: Use of metabolic profiles Cattle practice 20, 120–127.
Martinez N., Rodney R. M., Block E., Hernandez L. L., Nelson C. D., Lean I. J., et al. (2017). Effects of prepartum dietary cation-anion difference and source of vitamin d in dairy cows: Health and reproductive responses. J. Dairy Sci. 101, 2563–2578. doi: 10.3168/jds.2017-13740
Martinez N., Sinedino L. D. P., Bisinotto R. S., Daetz R., Lopera C., Risco C. A., et al. (2016). Effects of oral calcium supplementation on mineral and acid-base status, energy metabolites, and health of postpartum dairy cows. J. Dairy Sci. 99, 8397–8416. doi: 10.3168/jds.2015-10527
Martinez N., Sinedino L. D. P., Bisinotto R. S., Ribeiro E. S., Gomes G. C., Lima F. S., et al. (2014). Effect of induced subclinical hypocalcemia on physiological responses and neutrophil function in dairy cows. J. Dairy Sci. 97, 874–887. doi: 10.3168/jds.2013-7408
Martín-Tereso J., Martens H. (2014). Calcium and magnesium physiology and nutrition in relation to the prevention of milk fever and tetany (Dietary management of macrominerals in preventing disease). Vet. Clin. North Am. - Food Anim. Pract. 30, 643–670. doi: 10.1016/j.cvfa.2014.07.007
McArt J. A. A., Neves R. C. (2019). Association of transient, persistent, or delayed subclinical hypocalcemia with early lactation disease, removal, and milk yield in Holstein cows. J. Dairy Sci. 103(1):690–701 doi: 10.3168/JDS.2019-17191
Mullarky I. K., Wark W. A., Dickenson M., Martin S., Petersson-Wolfe C. S., Knowlton K. F. (2009). Short communication: analysis of immune function in lactating dairy cows fed diets varying in phosphorus content. J. Dairy Sci. 92, 365–368. doi: 10.3168/jds.2008-1323
National Academies of Sciences, Engineering and M (2021). Nutrient requirements of dairy cattle: Eighth revised edition (Washington, DC: Washington DTNAP), 502 p. doi: 10.17226/25806
NRC (2001). Nutrient requirements of dairy cattle. 7th ed (Washington, DC: National Academies Press). doi: 10.17226/9825
Peterson A. B., Orth M. W., Goff J. P., Beede D. K. (2005). Periparturient responses of multiparous Holstein cows fed different dietary phosphorus concentrations prepartum. J. Dairy Sci. 88, 3582–3594. doi: 10.3168/jds.S0022-0302(05)73043-5
Probo M., Pascottini O. B., LeBlanc S., Opsomer G., Hostens M. (2018). Association between metabolic diseases and the culling risk of high-yielding dairy cows in a transition management facility using survival and decision tree analysis. J. Dairy Sci. 101, 9419–9429. doi: 10.3168/jds.2018-14422
Redfern E. A., Sinclair L. A., Robinson P. A. (2021). Why isn’t the transition period getting the attention it deserves? farm advisors’ opinions and experiences of managing dairy cow health in the transition period. Prev. Vet. Med. 194, 105424. doi: 10.1016/j.prevetmed.2021.105424
Reinhardt T. A., Lippolis J. D., McCluskey B. J., Goff J. P., Horst R. L. (2011). Prevalence of subclinical hypocalcemia in dairy herds. Vet. J. 188, 122–124. doi: 10.1016/j.tvjl.2010.03.025
Roberts T., Chapinal N., Leblanc S. J., Kelton D. F., Dubuc J., Duffield T. F. (2012). Metabolic parameters in transition cows as indicators for early-lactation culling risk. J. Dairy Sci. 95, 3057–3063. doi: 10.3168/jds.2011-4937
Saborío-Montero A., Vargas-Leitón B., Romero-Zúñiga J. J., Sánchez J. M. (2017). Risk factors associated with milk fever occurrence in grazing dairy cattle. J. Dairy Sci. 100, 1–8. doi: 10.3168/jds.2017-13065
Santos J. E. P., Lean I. J., Golder H., Block E. (2019). Meta-analysis of the effects of prepartum dietary cation-anion difference on performance and health of dairy cows. J. Dairy Sci. 102, 2134–2154. doi: 10.3168/JDS.2018-14628
Staufenbiel R., Dallmeyer M., Horner S., Gelfert C.-C (2002). Gebärparese des Rindes – neue Aspekte zum klinischen Bild und zur Therapie. Proceedings Kongress Bund praktischer Tierärzte, Nürnberg. Vet. Med. Rev. V6 (26), 61–66.
Tsiamadis V., Banos G., Panousis N., Kritsepi-Konstantinou M., Arsenos G., Valergakis G. E. (2016). Genetic parameters of subclinical macromineral disorders and major clinical diseases in postparturient Holstein cows. J. Dairy Sci. 99, 8901–8914. doi: 10.3168/jds.2015-10789
Venjakob P. L., Borchardt S., Heuwieser W. (2017). Hypocalcemia–cow-level prevalence and preventive strategies in German dairy herds. J. Dairy Sci 100:9258–9266. doi: 10.3168/jds.2016-12494
Venjakob P. L., Staufenbiel R., Heuwieser W., Borchardt S. (2019). Serum calcium dynamics within the first 3 days in milk and the associated risk of acute puerperal metritis. J. Dairy Sci. 102, 11428–11438. doi: 10.3168/JDS.2019-16721
Wächter S., Cohrs I., Golbeck L., Wilkens M. R., Grünberg W. (2021). Effects of restricted dietary phosphorus supply to dry cows on periparturient calcium status. J. Dairy Sci 105(1):748–760. doi: 10.3168/jds.2021-20726
Weiss W. P., Azem E., Steinberg W., Reinhardt T. A. (2015). Effect of feeding 25-hydroxyvitamin D3 with a negative cation-anion difference diet on calcium and vitamin d status of periparturient cows and their calves. J. Dairy Sci. 98, 5588–5600. doi: 10.3168/jds.2014-9188
Wilkens M. R., Muscher-Banse A. S. (2020). Review: Regulation of gastrointestinal and renal transport of calcium and phosphorus in ruminants. Animal 14, S29–S43. doi: 10.1017/S1751731119003197
Wilkens M. R., Nelson C. D., Hernandez L. L., McArt J. A. A. (2020). Symposium review: Transition cow calcium homeostasis–health effects of hypocalcemia and strategies for prevention. J. Dairy Sci. 103, 2909–2927. doi: 10.3168/JDS.2019-17268
Keywords: dairy cow, hypocalcemia, hypophosphatemia, calcium, prevention
Citation: Aubineau T, Boudon A and Guatteo R (2022) Acidified diet is not effective in preventing hypocalcemia in dairy cows on French commercial farms. Front. Anim. Sci. 3:1027183. doi: 10.3389/fanim.2022.1027183
Received: 02 September 2022; Accepted: 17 October 2022;
Published: 27 October 2022.
Edited by:
Vishwajit S. Chowdhury, Kyushu University, JapanReviewed by:
James Drackley, University of Illinois at Urbana-Champaign, United StatesTakashi Bungo, Okayama University of Science, Japan
Copyright © 2022 Aubineau, Boudon and Guatteo. This is an open-access article distributed under the terms of the Creative Commons Attribution License (CC BY). The use, distribution or reproduction in other forums is permitted, provided the original author(s) and the copyright owner(s) are credited and that the original publication in this journal is cited, in accordance with accepted academic practice. No use, distribution or reproduction is permitted which does not comply with these terms.
*Correspondence: Thomas Aubineau, thomas.aubineau@innoval.com