Lucerne meal in the diet of indigenous chickens: a review
- College of Graduate Studies, School of Interdisciplinary Research and Graduate Studies, University of South Africa, Pretoria, South Africa
Indigenous chicken production consists of an array of activities important to smallholder poultry farmers in Africa. One of the many factors influencing their production and threating the local food security is in the area of nutrition, particularly, that related to protein supplementation. The available feed resources to farmers are not enough to sustain the productivity of the chickens. Hence, the chickens’ diets often require nutritional supplementation. There is therefore an urgent need for the validation of locally grown feed ingredients to improve the sustainability of poultry production in sub-Saharan Africa. A dietary ingredient that may be used in the diets of chickens is lucerne (Medicago sativa), which is also known as alfalfa. In South Africa, lucerne is the most cultivated forage legume and approximately 1.3 million metric tonnes of lucerne are produced per year. Lucerne has high nutritional value, as it is a source of protein, amino acids, vitamins, and fatty acids. The potential of lucerne as a feed resource for indigenous chickens should, therefore, be investigated so that strategies to improve the nutrition of such chickens can be developed. The purpose of this review was to highlight lucerne as a potential dietary ingredient for indigenous chickens and discuss its effects on the productivity of broilers, egg-layers, and dual-purpose chickens.
1 Introduction
Food production must increase to meet global nutritional needs, particularly in the sub-Saharan countries, where economic and health challenges negatively contribute to food insecurity (Bjornlund et al., 2022). In this area of the globe, sustainable agriculture proves to be critical, as population growth rates continue to increase while arable land availability has been declining due to industrialization (Caillouet et al., 2019). Poultry producers are faced with a plethora of challenges related to the health, nutrition, and predation of their flocks. This has been exacerbated by the increase in ambient temperatures in communities where indigenous chickens are kept (Mapiye et al., 2008; Nyoni et al., 2022). Indigenous chickens have been exposed to unfavourable environmental conditions for centuries, which has resulted in their developing traits to cope with these harsh conditions, which include food scarcity (Köhler-Rollefson, 2004). Such birds can convert a wide range of agri-food by-products into edible products for humans (Mottet and Tempio, 2017). Therefore, indigenous chicken rearing could be an alternative means of maintaining and increasing sustainable food production in Africa. Meat and egg production are crucial for the nutrition of families in Africa (Alabi et al., 2012; Motsepe et al., 2016) and they are marketed as enhancing incomes (Nondzutha et al., 2020). Furthermore, indigenous chickens are used in communities for traditional events (Herd-Hoare and Shackleton, 2020).
In a country such as South Africa, the production of indigenous chickens predominantly takes place under subsistence farming of the extensive type, which is characterised by low-input systems (Mtileni et al., 2016; Idowu et al., 2021). Several researchers have found that poultry farmers in rural communities in South Africa rear from 5 to 30 chickens per cycle (Guèye, 1998; Idowu et al., 2019; Nxumalo et al., 2020). The chickens are often left to search for food that meets their nutritional requirements on their own (Raphulu et al., 2015; Yusuf et al., 2017), especially when supplementary grains are out of season. Chickens reared under subsistence farming in South Africa have been found to suffer greatly because of poor nutrition (Alabi et al., 2013). The rising cost of feed constrains production and is, therefore, a key motivation to seek alternative forms of feed resources for poultry farmers and producers. The main source of protein in poultry diets for the commercial sector, soyabean meal, remains expensive for smallholder farmers. The range of locally available feed resources is wide in terms of dietary ingredients; however, it does not yield enough of the nutrients required by chickens for sustaining production (Rashid et al., 2003; Ncobela and Chimonyo, 2015; Hayat et al., 2016). Protein, which is a limiting factor for growth and productivity, is often inadequate in the diet of chickens (Ncobela and Chimonyo, 2015). Due to the scarcity of some grains, the potential of locally grown alternative feed resources could and should, therefore, be thoroughly explored in African communities to develop nutritional strategies to optimize the nutrition of indigenous birds and consequently ensure local food security.
Plant species such as Carica papaya (Banjoko et al., 2020), Moringa oleifera (Sebola et al., 2015), Morus alba (Mwai, 2021), Ipomoea batatas (Bekele and Sintayehu, 2021), Amaranthus cruentus (Manyelo et al., 2022), and Azadirachta indica (Ubua et al., 2019) are useful in chicken diets as sources of protein (Sugiharto et al., 2019). However, the usage of some of these plants by subsistence farmers of indigenous chickens is limited, not solely because of antinutritional factors, but also due to their limited availability and their high cost of acquisition. The cost of agricultural inputs and of establishing some of these recommended plants in yields may also not be practical. Therefore, the need to further explore adapted and hardy forage crops for indigenous chickens is pertinent.
Lucerne (Medicago sativa), also known as alfalfa, is commonly used as a forage for ruminants as hay or silage, and, to a lesser extent, as grazing material (Suwignyo et al., 2021). However, when harvested at the appropriate growth stage, literature shows that it may be of great importance for poultry feeding (Tkáčová et al., 2011; Jiang et al., 2018; Paredes and Risso, 2020; He et al., 2021; Vlaicu et al., 2023). For a long period lucerne has been used in poultry diets as a source of fibre for egg-laying chickens, and it was used to induce moulting (Donalson et al., 2005; Glatz and Tilbrook, 2021). However, research has indicated that it possesses not only favourable amino acid (Homolka et al., 2008) and fatty acid contents (Bashir et al., 2023), but also pharmacological benefits (Bora and Sharma, 2011). The potential of lucerne as a feed resource, therefore, needs to be investigated to map strategies for improving the nutrition of indigenous chickens, especially in developing countries such as South Africa. The purpose of this review was to assess the available literature on the utilization of lucerne as a dietary ingredient for indigenous chickens and its effects on chickens (broilers, egg-layers, and dual-purpose breeds).
2 Description of lucerne
Lucerne is known as the “queen of forages” because of its ability to sustain growth and adapt to a wide range of climatic conditions (Russelle, 2001). It is a forage that is grown in many parts of the world (Mielmann et al., 2017). It is cultivated in more than 80 countries and in all continents, over areas which exceed 35 million ha (Radović et al., 2009). It is grown in the frozen regions of southern Canada and eastern China, and from the mild climates of Chile to the searing deserts of Mexico and Africa.
Lucerne belongs to the class Magnoliopsida, in the order Fabales, in the family Fabaceae, which are also known as legumes and belong to the genus Medicago, species sativa (Burezq, 2021). The plant has a “crown” that can generate much foliage through bud formation, having many stems at the base of the plant, which grow to the height of about 1 m, and by having a deep tap root system. The leaves of lucerne are trifoliate with smooth, slightly tooth-edged, and oval-shaped leaflets. The flower is mostly purple in colour, and approximately 0.8 cm wide, and has a cluster up to 4 cm long at the top of its branches. Lucerne develops pods in its reproductive stage. Each plant may have four or five coiled spiral pods with a hard outer surface, which are typically hairy or leathery. Each pod may have between three and seven seeds.
Lucerne is a perennial legume crop that may be harvested three to five times per year depending on the harvesting interval and cultivar (Palmonari et al., 2014; Xu et al., 2021; Eckberg et al., 2022). It can survive defoliation through its ability to quickly regenerate shoots. It can respond quickly to significant summer rainfall (>10 mm) but requires 20 mm–25 mm of rainfall to produce substantial growth.
In South Africa, lucerne is the most cultivated forage legume and the country produces approximately 1.3 million tonnes of lucerne per year, which is planted in all provinces (STATSSA, 2017). The western to central regions of the country (i.e., the Northern, Western, and Eastern Capes, the Free State, and the North West provinces) produce a greater amount of lucerne (129,986 to 487, 181 tonnes per year) under dry and irrigated farms. In contrast, the eastern to central regions (i.e., the Mpumalanga, Limpopo, KwaZulu-Natal, and Gauteng provinces) produce smaller amounts of lucerne (1,019 to 4,767 tonnes) per year under dry and irrigated farms. These areas contain a wide range of soil characteristics (texture, organic matter, nitrogen and phosphorus content, and pH) and farm management practices that can influence yields.
3 Nutritional value
The nutritive value of lucerne is affected by its nutritional components, which tend to vary as the plant grows. This is illustrated by the nutrient composition harvested at the different physiological stages (Fan et al., 2018) (Table 1). As the plant matures, the crude protein (CP) content decreases, while the fibre content increases. Thus, the stage of harvesting is important for lucerne. Other climatic factors and edaphic factors (i.e., soil conditions, storage, diseases and insects, weeds, cultivar, water supply, and fertilisation) affect the nutritional quality of lucerne (Scholtz et al., 2009). Xu et al. (2021) have illustrated that the harvesting interval (28 day or 35 days) in a given year also affects the plant’s nutrient composition.
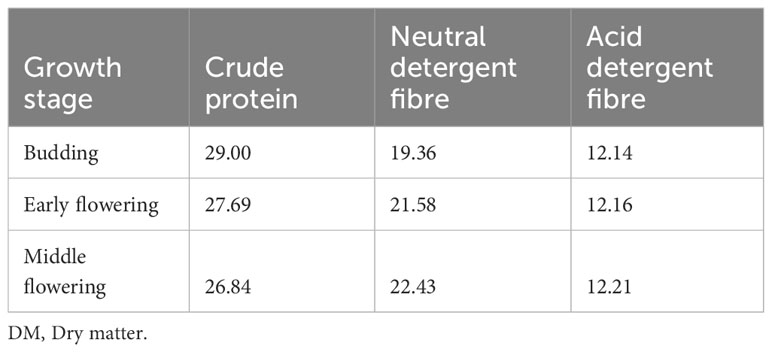
Table 1 Crude protein and fibre contents (% DM) of lucerne harvested at different stages of physiological growth (Fan et al., 2018).
The nutrient composition of lucerne meal is presented in Table 2. Indigenous chickens (growers and adults) require 12% to 16% CP dry matter (DM) in their diets (NRC, 1994; Kingori et al., 2007; Alabi et al., 2013; Kalinda and Tanganyika, 2017). However, Goromela et al. (2006) and Hayat et al. (2016) reported that indigenous chicken diets are provided with only 10% CP DM under free-range systems. This, therefore, indicates that there is a deficit in the diet of chickens in free-range systems which has to be supplemented through various interventions. Lucerne can be utilized as a dietary ingredient providing protein for chickens as it contains 16% to 24% CP (Table 2).
The crude fibre (CF) level of lucerne meal ranges between 20.3% and 30.0% DM (Table 2), while its neutral detergent fibre content ranges between 36.7% and 53.7% according to He et al. (2021) and Hidosa and Biru (2021). When harvested during the budding stage, lucerne will contain a higher moisture content and less fibre [neutral detergent fibre (NDF) and acid detergent fibre (ADF)]. As plants age, they tend to have a higher CF content (Fan et al., 2018). Laudadio et al. (2014) lowered the fibre content in lucerne meal by fractionation and classification process to achieve 15.3% CF in DM. However, such technologies might be inaccessible to, and impractical for, smallholder farmers in Southern Africa.
The ash content of lucerne meal ranges between 9.2% and 12.9% DM (Table 2). The ash content indicates the concentration of minerals in the feed ingredient. Mwalusanya et al. (2002) and Hayat et al. (2016) showed that scavenging chickens do not suffer from the lack of minerals. Raphulu et al. (2015) demonstrated that South African indigenous chickens meet dietary mineral requirements determined by the ash levels of the crop contents for growing and adult indigenous chickens which ranged between 10.5 to 12.3%. Therefore, lucerne may be utilized by subsistence farmers to meet the mineral growth and production requirements of indigenous chickens.
Lucerne meal has a good balance of amino acids (Ensminger, 1992; Sen et al., 1998; Ponte et al., 2004; Markovic et al., 2007). Table 3 shows the concentration of 17 amino acids reported in lucerne meal and other proteinous dietary ingredients (Moringa oleifera, sunflower, and soyabean meals). Lucerne contains high proportions of aspartic acid, glutamic acid, and leucine, but low proportions of methionine and cysteine (Homolka et al., 2008; Pleger et al., 2021; Luo et al., 2022). When compared with the other plant species, lucerne and moringa leaf meal have a similar lysine content (5.4 g/100 g to 5.6 g/100 g CP), which is higher than that of sunflower meal (3.6g/100 g CP). Lucerne and soyabean meal also contain similar methionine levels (1.4 g/100 g CP). However, sunflower seed meal has a slightly higher methionine content (1.7 g/100 g CP) than lucerne meal. The threonine level of lucerne is similar to that of soyabean (3.8 g/100 g CP). However, moringa leaf meal contains a higher (4.5 g/100 g CP) threonine content than lucerne meal.
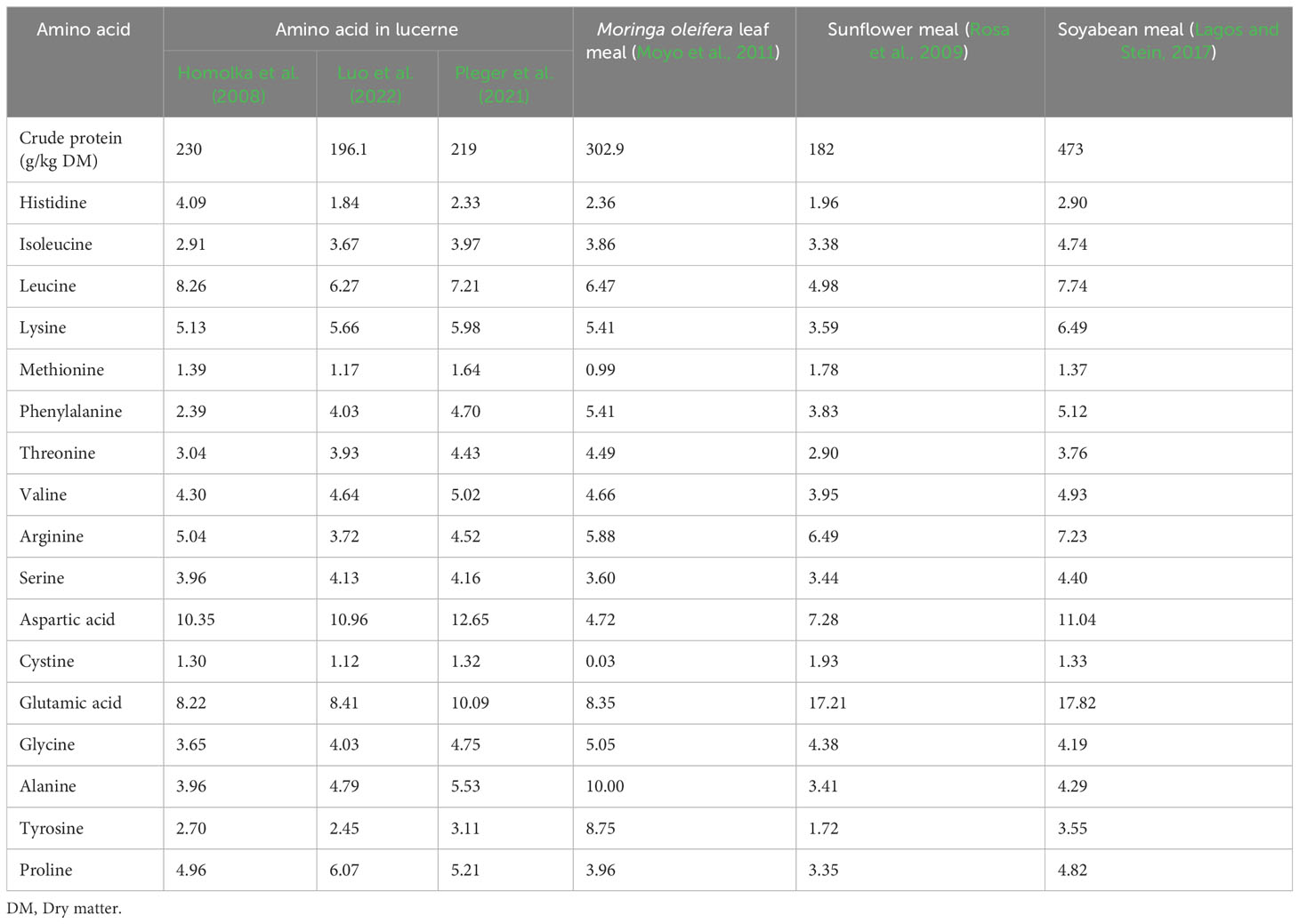
Table 3 Crude protein and amino acid (g/100 g protein) contents of lucerne, sunflower, soyabean, and moringa meals.
3.1 Secondary metabolites in lucerne
Secondary metabolites are a diverse group of compounds with a low molecular weight and are distinct from primary metabolites. They do not play a crucial role in the normal growth and development of plants; they are, however, synthesized for defence purposes and other forms of interspecies protection (Bennett and Wallsgrove, 1994). Secondary metabolites have a myriad of properties including antioxidative, pharmacological, antifungal, anti-inflammatory, and phytoestrogenic properties (Wang et al., 2021). The secondary metabolites of plants can be classified into three broad groups: phenolic compounds, terpenoids, and nitrogen-containing compounds.
Lucerne contains different types of phenolic compounds (Krakowska et al., 2017; Horvat et al., 2022) (Table 4). Phenolics may further be classified as either simple phenols (phenolic acids) or polyphenols (flavonoids and non-flavonoids), as shown in Vardhan and Shukla, 2017. Dietary supplementation with lucerne flavonoids (15 mg/kg diet) improved the growth performance of chickens (Surai, 2014; Ouyang et al., 2016). The factor responsible for the increased growth performance of chickens was explained as the combination of the growth and hepatic growth hormone receptor upregulated by isoflavone (Elkomy and Elghalid, 2014). Additionally, isoflavones promote protein synthesis in the muscles, which in turn leads to increased growth (Kamboh and Zhu, 2013). In egg layers, Iskender et al. (2017) and Prihambodo et al. (2022) showed that the use of dietary supplementation flavonoids (0.5 g/kg of hesperidin, naringin, or quercetin) had no effect on laying performance; however, the egg yolk cholesterol level decreased while the yolk protein percentage increased. Improvements in the growth performance and meat cholesterol have also been reported when 10 mg/kg–15 mg/kg of alfalfa flavonoids was supplemented (Ouyang et al., 2016; Prihambodo et al., 2021).
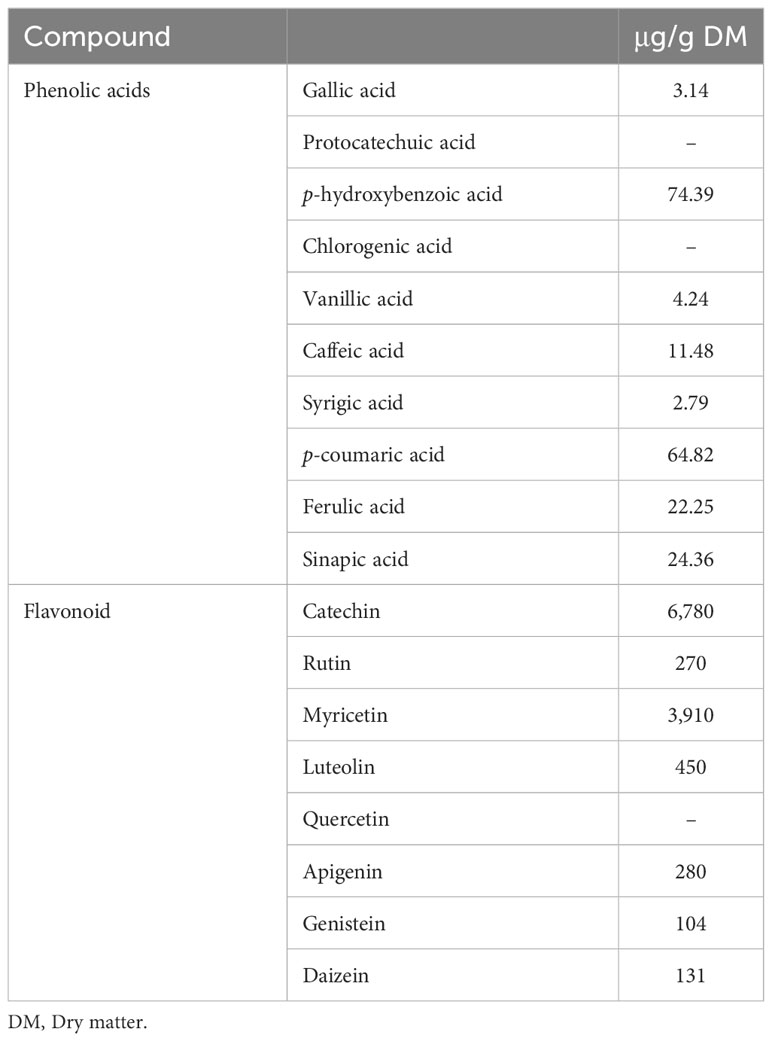
Table 4 Phenolic acids and flavonoids found in lucerne (Srisaikham, 2021).
An important category of non-flavonoids that were also identified in lucerne are tannins. Tannins are prominent metabolites widely distributed in forages such as trees, shrubs, and legumes (including lucerne). These have been implicated in limiting the digestibility of amino acids and energy (Getachew et al., 2008). Tannins exert negative effects on the feed intake, weight gain, and feed conversion ratio of broiler chickens (Hidayat et al., 2021). However, other reports indicate beneficial effects of tannins because they exert antimicrobial, antioxidant, anti-inflammatory, and gut health-promoting effects (Medugu et al., 2012). The literature is inconsistent in terms of the effect of tanniniferous feed ingredients on poultry. There is therefore a need to ascertain the appropriate dosages for lucerne meal supplementation, especially in the diets of indigenous chickens (Wonkyun et al., 2020).
Lucerne possesses antioxidative properties. It has been investigated because of the health benefits it is believed to confer (Bora and Sharma, 2011). One method of measuring the antioxidative capacity of organic material is to determine its 2,2-diphenyl-1-picrylhydrazyl (DHHP) scavenging activity, as outlined by Krakowska et al. (2017). The results are expressed as 6-hydroxy-2,5,7,8-tetramethylchroman-2-carboxylic acid (trolox) equivalence per gram DM of the sample. The same authors, Krakowska et al. (2017), reported that the antioxidative capacity of lucerne ranges between 15.6 mg and 48.3 mg trolox equivalence (TE)/g DM depending on the part of the plant (i.e., leaves, stems, or flowers) studied. Horvat et al. (2022) indicated that lucerne leaf meal had 32.2 mg TE/g DM, while Vlaicu et al. (2023) reported that lucerne meal from ground lucerne cuttings had 17.78 mg TE/g DM. Hence, dietary lucerne supplementation could be useful in enhancing the antioxidant levels in poultry products.
There are phenolic compounds in lucerne which possess powerful phytoestrogenic properties. Phytoestrogens accumulate in the plant during times of environmental stress and disease (Křížová et al., 2019). They contribute to the plant’s defence mechanisms, hence they do not have hormonal functions (Shah and Smith, 2020). These compounds have the capacity to influence growth and reproduction in animals. Phenolic compounds that possess phytoestrogenic properties include non-flavonoids (coumestrol 3-methoxycoumestrol and 4-methoxycoumestrol), flavonoids (luteolin, apigenin, quercetin, genistein, and daidzein) and lignans (Srisaikham, 2021; Wyse et al., 2021) (Table 5). These compounds vary in quantity and oestrogenic activity levels depending on the lucerne cultivar, environmental factors, and stage of growth.
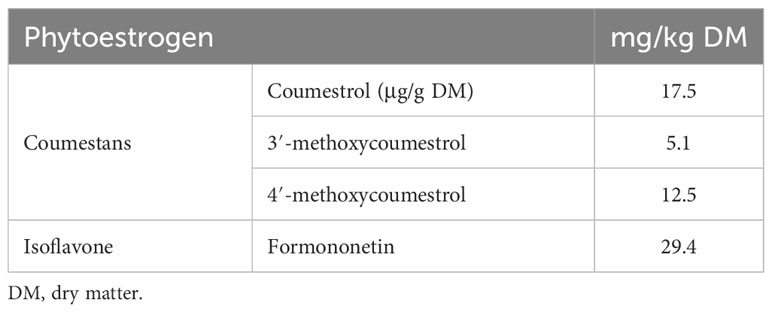
Table 5 Phytoestrogens (coumestans and isoflavone) identified in lucerne (Wyse et al., 2021).
Lucerne contains a particular class of secondary metabolites classified under triterpenes saponins (Bera et al., 2019; Szumacher-Strabel et al., 2019; Nagy and Makleit, 2021). Lucerne leaf meal is composed of different types of saponins (Table 6) (Pleger et al., 2021). Saponins are also capable of forming insoluble complexes with dietary cholesterol in the intestines, which are then excreted (Zheng et al., 2019; Pleger et al., 2021). It has been reported that levels and types of saponins could improve meat quality in poultry by enhancing its lipid oxidative stability (Bera et al., 2019). Dietary saponins, however, have adverse effects on chickens’ performance due to reduced amino acid digestibility (Pleger et al., 2020). Hence, they are considered the main antinutritional component in lucerne. There is a need to determine the type of saponins responsible for poor performance (i.e, reduced digestibility) in poultry.
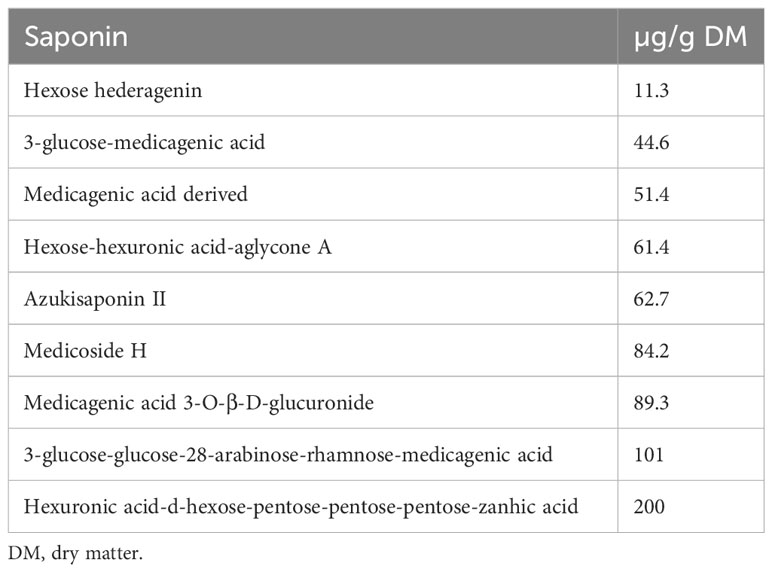
Table 6 Relative contents of putatively identified saponins expressed as equivalent umbelliferone in Medicago sativa (lucerne) leaves (Pleger et al., 2021).
3.2 Vitamins and carotenoids
Vitamins are useful in preventing oxidative stress, regulating the immune response, and maintaining normal physiological, biochemical, and homeostatic mechanisms in animals (Alagawany et al., 2021) (Table 7). Lucerne contains vitamins A, D, E, and K (fat soluble) and also vitamin B and vitamin B complex with vitamin C (fat soluble) (González-Calvo et al., 2015; Englmaierová et al., 2019; Jia et al., 2019). Lucerne contains higher levels of vitamin E (205.9 mg/kg) and precursors of vitamin A (carotenoids: zeaxanthin, lutein, and β-carotene). These vitamins have significant roles in egg production, and, therefore, the consumption of lucerne could enhance the egg-laying potential of birds.
3.3 Fatty acids
Lucerne contains a range of fatty acids that may be impactful to poultry (Table 8). The fatty acid profile of lucerne varies with varieties, seasons, and other agronomic factors. Lucerne contains a higher proportion of unsaturated fatty acids than saturated fatty acids. Unsaturated fatty acids are required for the biosynthesis of oxylipids (Raphael and Sordillo, 2013). Some of the polyunsaturated fatty acids contained include linoleic acid and α-linolenic acid, which are essential fatty acids (Nakamura and Nara, 2003). In terms of saturated fatty acids, palmitic acids are the major fatty acids that occur in lucerne. This indicates that lucerne meal supplementation in poultry diets could be useful in manipulating the fatty acid profile in poultry products (i.e., meat and eggs) (Grela et al., 2020; Kop‐bozbay et al., 2021). However, in the case of South African indigenous chickens, the effects of dietary lucerne supplementation on the fatty acid content of meat and eggs have not been investigated.
4 Lucerne in broiler chicken diets
Tkáčová et al. (2011) found that lucerne inclusion, even at a level of 20 g/kg, increased the body weight of Ross 308 broiler chickens. In addition, Shirzadegan and Taheri (2017), showed that the inclusion of lucerne meal at a level of 30 g/kg improved the body weight gain, feed intake, and feed conversion ratio (FCR) in Ross 308 broiler chickens. However, Varzaru et al. (2020) and Sánchez et al. (2022) found that lucerne meal inclusion levels of as high as 50 g/kg in the diet of Hubbard broiler chickens and of 40 g/kg in that of Cobb 500 broiler chickens did not affect either group’s feed intake, daily weight gain, or FCR. The inclusion of dietary lucerne meal at a level ranging from 50 g/kg to 200 g/kg resulted in poor production performance (Tkáčová et al., 2011). This was also supported by the findings of Paredes and Risso (2020), who reported that the body weight of Hubbard broiler chickens decreased sequentially in groups fed 0 g/kg, 50 g/kg, and 100 g/kg lucerne meal, and found no benefits in terms of feed intake and FCR. Pleger et al. (2020) demonstrated that Hubbard broiler chickens consuming dietary lucerne meal at a level between 100 g/kg and 200 g/kg resulted in poor growth rate and feed intake. Therefore, the lower inclusion (<40 g/kg) of dietary lucerne meal may be beneficial to broiler chickens for optimal growth performance.
Dietary lucerne, with its high fibre content, has effects on the development and performance of digestive organs, which ultimately influences productivity (JøRgensen et al., 1996). According to Shirzadegan and Taheri (2017), when lucerne meal content is increased in the diet of chickens, their proventriculus, gizzard, and small intestine increase in weight to adapt to the stimulation of the organs to facilitate thorough mechanical and chemical digestion (Abdollahi et al., 2018). This adaptation is not beneficial to broiler chickens because the nutrients meant for growth and muscle accretion are utilized for this instead.
Varzaru et al. (2020) showed that the inclusion of fibrous ingredients such as lucerne (at a level of 50 g/kg) resulted in the increased composition of beneficial microbial organisms in the intestines of Cobb 500 broiler chickens. This indicates that lucerne meal in the diet of chickens helps to lower the incidence of diseases, thus preventing gut health issues and promoting animal performance. Picoli et al. (2014) supported this benefit by reporting that the histomorphology of the intestines was enhanced by lucerne meal inclusion in the diet of chickens. The intestinal villi height and crypt depth increased with the inclusion of dietary lucerne meal. This response is advantageous because the available surface area for effective nutrient digestion and absorption is increased (Incharoen, 2013; Adibmoradi et al., 2016). Further studies are necessary to elucidate the merits of having increased intestinal villi length and deeper crypt depth when chickens are fed lucerne meals supplemented diets, especially in indigenous chickens.
The carcass traits of broiler chickens were also affected by the dietary inclusion of lucerne meal (Varzaru et al., 2020). Low inclusion levels (30 g/kg lucerne) improved the carcass weight, breast weight, and thigh weight (Shirzadegan and Taheri, 2017) of chickens, while higher levels (of 40 g/kg to 100 g/kg) dietary lucerne meal negatively affected these traits (Shirzadegan and Taheri, 2017; Paredes and Risso, 2020; Varzaru et al., 2020; Sánchez et al., 2022). This might be due to lowered feed intake, with higher dietary lucerne meal content resulting in the lowered body weight of chickens (Paredes and Risso, 2020). The taste of the carcass is also altered by lucerne supplementation. Ponte et al. (2004) reported that lucerne-treated birds had a better taste than their non-lucerne-treated counterparts and indicated that this was due to the antioxidant content of lucerne. Lucerne meal is rich in carotenoids (Englmaierová et al., 2019; Pleger et al., 2020). The carcasses of chickens consuming diets supplemented with lucerne meal were found to have higher yellowness (b*) values (Pleger et al., 2021). Lucerne meal also influenced the cholesterol content of broiler chicken meat, and increasing its supplementation level from 0 g/kg to 75 g/kg in broiler chickens’ diet resulted in their meat having lower cholesterol levels.
5 Lucerne in egg-laying chicken diets
Al-shami et al. (2011) reported that, in White Hisex laying hens, lucerne supplementation at a level of 50 g/kg and 70 g/kg decreased the production performance (i.e., the feed intake and egg production) of layers, while their egg yolk parameters (yolk yellowness, β-carotene content, yolk percentage, and cholesterol levels) improved. The yolk colour and β-carotene content improved due to the presence of carotenoids in lucerne, while the lowered yolk cholesterol content is consistent with the presents of phenolic compounds and saponins in diets supplemented with lucerne. Similar findings were reported by Laudadio et al. (2014), who fed Isa Brown laying hens diets supplemented with as much as 150 g/kg lucerne, and noted no adverse effects on egg production traits but found this to have positive effects on yolk quality. Therefore, improvements on the quality of the eggs occur at the expense of the quantity of eggs produced. The utilization of lucerne in the diets of laying hens to achieve production goals therefore has to be strategic.
6 Lucerne in dual-purpose chicken diets
Dual-purpose chickens are typically indigenous or local/native, and the same can be true of hybrid chickens that are bred selectively because of their possessing good meat and egg production traits. The native chicken breed Zhuanghe Dagu, also called the grass chicken, has the characteristic of herbivorous traits such as strong tolerance to dietary ingredients high in CF (Cui et al., 2022). There is a paucity of research information on the effects of lucerne on the production performance of dual-purpose chickens.
Beijing-You indigenous chickens aged 20 weeks to 28 weeks fed diets supplemented with 50 g/kg to 80 g/kg lucerne did not gain body weight or exhibit improved growth rate and feed intake (Zheng et al., 2019). Instead, their FCR and mortality rates improved when their diets were supplemented with 50 g/kg lucerne. Chinese indigenous chickens (Guangxi-Tiejiaoma broilers) aged 3 weeks to 12 weeks fed diets supplemented with 25 g/kg to 75 g/kg lucerne meal exhibited improved growth performance (in terms of feed intake and body weight) (He et al., 2021). Similarly, Cui et al. (2022) observed that lucerne meal inclusion in the diet of 35-week-old Zhuanghe Dagu chickens at a level of 60 g/kg was beneficial to their production performance. Therefore, the age or stage of growth of these chickens is a critical factor that influences the effects of lucerne on their growth production performance. As chickens aged, their growth performance was less affected by the inclusion of lucerne in their diet. Jiang et al. (2018) reported similar results, namely that the growth productivity of yellow feathered Chinese broiler chickens (aged 6 weeks to 9 weeks) fed diets supplemented with 80g/kg lucerne meal were not affected also indicated that an energy source needs to be provided to complement lucerne.
Zheng et al. (2019) showed that the carcass, breast, and thigh percentages; the albumen protein, breast muscle content of inosine monophosphate; abdominal fat yield; yolk protein; and total amino acid, and cholesterol levels were improved in chickens fed diets supplemented with lucerne compared with those fed no lucerne meal. However, the chemical compositions of breast and thigh meat were not influenced by lucerne supplementation. Jiang et al. (2018) reported improvements in the taste of yellow-feathered broiler chickens aged 6 weeks to 7 weeks when lucerne meal at a level of 40 g/kg was included in their diet. Therefore, the taste of the meat improved with the dietary inclusion of lucerne meal in chickens.
Lucerne had positive effects on the egg production rate, FCR, egg mass, yolk colour, albumen height, and haugh unit (Cui et al., 2022). Previous studies (Al-shami et al., 2011) have shown that lucerne supplementation is beneficial in terms of the internal contents of the egg, i.e., the egg yolk. Zheng et al. (2019) reported that albumen protein was improved by lucerne supplementation, and also that the yolk colour, yolk protein, and cholesterol levels also improved. This indicates the high potential of dual-purpose local chickens when offered lucerne.
7 Use of lucerne in indigenous chicken production
In South Africa, the need for alternative sources of protein is critical for production, especially by smallholder farmers. The lucerne produced for ruminants may also be useful in indigenous chicken diets. Plant-based ingredients, also referred to as “greens”, including lucerne, are being consumed by indigenous chickens (Raphulu et al., 2015; Admasu et al., 2019). There is, however, a knowledge gap concerning the utilization of lucerne for the productivity of indigenous chickens. The impact of lucerne on the production performance, nutrient digestibility, haematology, health status, and meat and egg quality of indigenous chickens is not sufficiently known or understood. Smallholder farmers have not been properly informed about the benefits of using lucerne in the feed of indigenous chickens since it is well established as feed for ruminants, and much benefit could be conferred from its utilization in the diets of indigenous chickens.
8 Conclusion
South African smallholder farmers involved in indigenous poultry production are faced with the challenge of finding local and sustainable feed resources that will improve the production of indigenous chickens. Lucerne, grown in most parts of the world, including South Africa has to be explored as an option for indigenous poultry. Lucerne contains crude protein at sufficient-enough levels to contribute towards meeting the requirements of slow-growing poultry such as indigenous chicken. The high fibre content of lucerne, however, limits dietary intake because it acts as a nutrient diluent. This may be mitigated by harvesting when the forage is still young (in the budding stage) or implementing other mechanical means to varying degrees thereby enabling access to the amino acid contents of this ingredient. Phenolics and saponins are secondary metabolites present in lucerne as antinutritional factors, and there needs to be conclusive evidence about the extent to which these might have beneficial effects in indigenous chickens. These secondary metabolites together with vitamins in lucerne meal may be useful in enhancing the antioxidant content of chicken products. Furthermore, lucerne may be useful in improving the fatty acid profile of poultry products because it has a higher proportion of unsaturated fatty acids (omega-3 and -6 fatty acids). When included at levels up to 50 g/kg, lucerne has shown to be beneficial for broilers, layers, and Asian indigenous chickens. Such findings indicate that lucerne has significant potential as a feed ingredient and should be explored in South African chicken nutrition.
Author contributions
MG: Conceptualization, Investigation, Writing – original draft, Writing – review & editing.
Funding
The author(s) declares that financial support was received for the research, authorship, and/or publication of this article. The author wishes to acknowledge the School of Interdisciplinary Research and Graduate Studies in the College of Graduate Studies at the University of South Africa for the support of the publication of this research manuscript.
Acknowledgments
The author would like to acknowledge the School of Interdisciplinary Research and Graduate Studies, in particular Professor KR Mbatha for the tremendous support in this manuscript.
Conflict of interest
The author declares that the research was conducted in the absence of any commercial or financial relationships that could be construed as a potential conflict of interest.
Publisher’s note
All claims expressed in this article are solely those of the authors and do not necessarily represent those of their affiliated organizations, or those of the publisher, the editors and the reviewers. Any product that may be evaluated in this article, or claim that may be made by its manufacturer, is not guaranteed or endorsed by the publisher.
References
Abdollahi M. R., Zaefarian F., Ravindran V. (2018). Feed intake response of broilers: Impact of feed processing. Anim. Feed Sci. Technol. 237, 154–165. doi: 10.1016/j.anifeedsci.2018.01.013
Adibmoradi M., Navidshad B., Jahromi M. F. (2016). The effect of moderate levels of finely ground insoluble fibre on small intestine morphology, nutrient digestibility and performance of broiler chickens. Ital. J. Anim. Sci. 15, 310–317. doi: 10.1080/1828051X.2016.1147335
Admasu S., Solomon D., Meseret M. (2019). Poultry feed resources and chemical composition of crop content of scavenging indigenous chicken. Online J. Anim. Feed Res. 9 (6), 247–255. doi: 10.36380/scil.2019.ojafr34
Aganga A. A., Tshwenyane S. O. (2003). Lucerne, lablab and Leucaena leucocephala forages: Production and utilization for livestock production. Pakistan J. Nutr. 2, 46–53. doi: 10.3923/pjn.2003.46.53
Alabi O. J., Ng’ambi J. W., Norris D. (2012). Effect of egg weight on physical egg parameters and hatchability of indigenous Venda chickens. Asian J. Anim. Veterinary Adv. 7, 166–172. doi: 10.3923/ajava.2012.166.172
Alabi O. J., Ng’ambi J. W., Norris D. (2013). Dietary energy level for optimum productivity and carcass characteristics of indigenous venda chickens raised in closed confinement. South Afr. J. Anim. Sci. 43 (1), 75–80. doi: 10.4314/SAJAS.V43I5.14
Alagawany M., Elnesr S. S., Farag M. R., Tiwari R., Yatoo M. I., Karthik K., et al. (2021). Nutritional significance of amino acids, vitamins and minerals as nutraceuticals in poultry production and health – a comprehensive review. Veterinary Q. 41, 1. doi: 10.1080/01652176.2020.1857887
Al-shami M. A., Salih M., Abbas T. (2011). Effects of dietary inclusion of alfalfa (Medicago sativa L.) leaf meal and Xylam enzyme on laying hens’ performance and egg quality. Res. Vet. Sci. 11, 754–759. doi: 10.1080/01652176.2020.1857887
Banjoko O., Adebayo I., Osho I., Olumide M., Ajayi O. A., Akinboye O. (2020). Evaluation of varying levels of Carica papaya leaf meal on growth, carcass, haematological parameters and its use as anticoccidial for broiler chicken. Nigerian J. Anim. Sci. 22 (3), 229–241.
Bashir N., Şekeroğlu A., Tainika B., Özer C. O. (2023). Effect of different pasture species on growth performance, carcass traits, internal organ weights, and meat quality of slower growing broilers in free-range production system. Trop. Anim. Health Production 55, 1–14. doi: 10.1007/S11250-023-03581-9/TABLES/9
Bekele B., Sintayehu S. (2021). Effect of various levels of sweat potato (hawassa-83) leaf meal on the laying performance and egg quality of koekoek dual purpose chicken. Poultry Fisheries Wildlife Sci. 9, 1–4.
Bennett R. N., Wallsgrove R. M. (1994). Secondary metabolites in plant defence mechanisms. New Phytol. 127, 617–633. doi: 10.1111/j.1469-8137.1994.tb02968.x
Bera I., Tyagi P. K., Mir N. A., Tyagi P. K., Dev K., Sharma D., et al. (2019). Dietary supplementation of saponins to improve the quality and oxidative stability of broiler chicken meat. J. Food Sci. Technol. 56, 2063–2072. doi: 10.1007/s13197-019-03683-z
Bjornlund V., Bjornlund H., van Rooyen A. (2022). Why food insecurity persists in sub-Saharan Africa: A review of existing evidence. Food Secur. 14, 845–864. doi: 10.1007/S12571-022-01256-1/FIGURES/4
Bora K. S., Sharma A. (2011). Phytochemical and pharmacological potential of Medicago sativa: a review. Pharm. Biol. 49 (2), 211–220. doi: 10.3109/13880209.2010.504732
Burezq H. (2021). Reproductive ecology of forage alfalfa (Medicago sativa L.): Plant Reproductive Ecology. Recent Adv. doi: 10.5772/intechopen.100640
Caillouet O. C., Nalley L. L., Farmer A. L. (2019). Increasing economic resilience through mixed farming with maize and poultry in Northern Mozambique. Acta Hortic. 1246, 93–102. doi: 10.17660/ACTAHORTIC.2019.1246.13
Cui Y., Diao Z., Fan W., Wei J., Zhou J., Zhu H., et al. (2022). Effects of dietary inclusion of alfalfa meal on laying performance, egg quality, intestinal morphology, caecal microbiota and metabolites in Zhuanghe Dagu chickens. Ital. J. Anim. Sci. 21 (1), 831–846. doi: 10.1080/1828051X.2022.2067009
Donalson L. M., Kim W. K., Woodward C. L., Herrera P., Kubena L. F., Nisbet D. J., et al. (2005). Utilizing different ratios of alfalfa and layer ration for molt induction and performance in commercial laying hens. Poultry Sci. 84, 362–369. doi: 10.1093/ps/84.3.362
Eckberg J. O., Wells S. S., Jungers J. M., Lamb J. F. S., Sheaffer C. C. (2022). Alfalfa forage yield, milk yield, and nutritive value under intensive cutting. Agrosystems Geosciences Environ. 5, e20246. doi: 10.1002/agg2.20246
Elkomy A. E., Elghalid O. A. (2014). Physiological performance of broiler chicks fed on Medicago sativa seeds as natural source of isoflavones. Asian J. Poultry Sci. 8, 97–105. doi: 10.3923/AJPSAJ.2014.97.105
Englmaierová M., Skřivan M., Vít T. (2019). Alfalfa meal as a source of carotenoids in combination with ascorbic acid in the diet of laying hens. Czech J. Anim. Sci. 64, 17–25. doi: 10.17221/116/2018-CJAS
Fan W., Ge G., Liu Y., Wang W., Liu L., Jia Y. (2018). Proteomics integrated with metabolomics: Analysis of the internal causes of nutrient changes in alfalfa at different growth stages. BMC Plant Biol. 18, 1–15. doi: 10.1186/S12870-018-1291-8/TABLES/5
Getachew G., Pittroff W., DePeters E. J., Putnam D. H., Dandekar A., Goyal S. (2008). Influence of tannic acid application on alfalfa hay: In vitro rumen fermentation, serum metabolites and nitrogen balance in sheep. Animal 2, 381–390. doi: 10.1017/S1751731107001486
Glatz P. C., Tilbrook A. J. (2021). Welfare issues associated with moulting of laying hens. Anim. Production Sci. 61, 1006–1012. doi: 10.1071/AN19700
González-Calvo L., Joy M., Blanco M., Dervishi E., Molino F., Sarto P., et al. (2015). Effect of vitamin E supplementation or alfalfa grazing on fatty acid composition and expression of genes related to lipid metabolism in lambs1. J. Anim. Sci. 93, 3044–3054. doi: 10.2527/jas.2014-8758
Goromela E. H., Kwakkel R. P., Verstegen M. W. A., Katule A. M. (2006). Strategies to optimize the use of scavengeable feed resource base by smallholders in traditional poultry production systems in Africa: a review. Afr. J. Agric. Res. 1, 91–100.
Grela E. R., Knaga S., Winiarska-Mieczan A., Zięba G. (2020). Effects of dietary alfalfa protein concentrate supplementation on performance, egg quality, and fatty acid composition of raw, freeze-dried, and hard-boiled eggs from Polbar laying hens. Poultry Sci. 99, 2256–2265. doi: 10.1016/J.PSJ.2019.11.030
Guèye E. H. F. (1998). Village egg and chicken meat production in Africa. World's Poultry Sci. J. 54, 73–85. doi: 10.1079/wps19980007
Hayat N., Solomon D., Meseret M. (2016). Chemical composition of scavenging feed resource of indigenous chickens. Asian J. Anim. Sci. 10, 182–188. doi: 10.3923/AJAS.2016.182.188
He G., Zhao L., Shishir M. S. R., Yang Y., Li Q., Cheng L., et al. (2021). Influence of alfalfa meal, as a source of dietary fibre, on growth performance, development, pH of gastrointestinal tract, blood biochemical profile, and meat quality of broilers. J. Appl. Anim. Res. 49, 431–439. doi: 10.1080/09712119.2021.2000417
Herd-Hoare S. C., Shackleton C. (2020). Integrating ecosystem services and disservices in valuing smallholder livestock and poultry production in three villages in South Africa. Land 9 (9), 294. doi: 10.3390/LAND9090294
Hidayat C., Irawan A., Jayanegara A., Sholikin M. M., Prihambodo T. R., Yanza Y. R., et al. (2021). Effect of dietary tannins on the performance, lymphoid organ weight, and amino acid ileal digestibility of broiler chickens: A meta-analysis. Veterinary World 14, 1405–1411. doi: 10.14202/vetworld.2021.1405-1411
Hidosa D., Biru S. K. (2021). Dry matter yield and chemical composition of alfalfa (Medicago sativa) varieties as animal feed in the South Omo Zone of South-western Ethiopia. Acta Veterinaria Brno 3, 2582–3183.
Homolka P., Koukolová V., Němec Z., Mudřík Z., Hučko B., Sales J. (2008). Amino acid contents and intestinal digestibility of lucerne in ruminants as influenced by growth stage. Czech J. Anim. Sci. 53, 499–505. doi: 10.17221/367-CJAS
Horvat D., Viljevac Vuletić M., Andrić L., Baličević R., Kovačević Babić M., Tucak M. (2022). Characterization of forage quality, phenolic profiles, and antioxidant activity in alfalfa (Medicago sativa L.). Plants 11, 2735. doi: 10.3390/plants11202735
Incharoen T. (2013). Histological adaptations of the gastrointestinal tract of broilers fed diets containing insoluble fiber from rice hull meal. Am. J. Anim. Vet. Sci. 8(2), 79–88. doi: 10.3844/ajavsp.2013.79.88
Idowu P. A., Mpayipheli M., Muchenje V. (2019). Original research paper a survey study on productive and reproductive performance of indigenous poultry. Am. J. Anim. Veterinary Sci. 14, 33–39. doi: 10.3844/ajavsp.2019.33.39
Idowu P. A., Zishiri O., Nephawe K. A., Mtileni B. (2021). Current status and intervention of South Africa chicken production-A review. World's Poultry Sci. J. 77, 115–133. doi: 10.1080/00439339.2020.1866965
Iskender H., Yenice G., Dokumacioglu E., Kaynar O., Hayirli A., Kaya A. (2017). Comparison of the effects of dietary supplementation of flavonoids on laying hen performance, egg quality and egg nutrient profile. Br. Poultry Sci. 58, 550–556. doi: 10.1080/00071668.2017.1349297
Jia T., Sun Z., Gao R., Yu Z. (2019). Lactic acid bacterial inoculant effects on the vitamin content of alfalfa and Chinese leymus silage. Asian-Australasian J. Anim. Sci. 32, 1873. doi: 10.5713/AJAS.19.0135
Jiang S., Gou Z., Li L., Lin X., Jiang Z. (2018). Growth performance, carcass traits and meat quality of yellow-feathered broilers fed graded levels of alfalfa meal with or without wheat. Anim. Sci. J. 89, 561–569. doi: 10.1111/ASJ.12968
JøRgensen H., Zhao X.-Q., Knudsen K. E. B., Eggum B. O. (1996). The influence of dietary fibre source and level on the development of the gastrointestinal tract, digestibility and energy metabolism in broiler chickens. Br. J. Nutr. 75, 379–395. doi: 10.1079/BJN19960141
Kalinda W., Tanganyika J. (2017). Effects of protein levels on growth and laying performance of the Malawian indigenous chicken. Int. J. Avian Wildlife Biol. 2, 169–171. doi: 10.15406/IJAWB.2017.02.00037
Kamboh A. A., Zhu W. (2013). Individual and combined effects of genistein and hesperidin supplementation on meat quality in meat‐type broiler chickens. J. Sci. Food Agric. 93, 3362–3367. doi: 10.1002/jsfa.6185
Kingori A. M., Tuitoek J. K., Muiruri H. K., Wachira A. M., Birech E. K. (2007). Protein intake of growing indigenous chickens on free-range and their response to supplementation. Int. J. Poultry Sci. 6, 617–621. doi: 10.3923/IJPS.2007.617.621
Koçer B., Bozkurt M., Ege G., Tüzün A. E., Konak R., Olgun O. (2018). Effects of a meal feeding regimen and the availability of fresh alfalfa on growth performance and meat and bone quality of broiler genotypes. Br. poultry Sci. 59.3, 318–329. doi: 10.1080/00071668.2018.1440378
Köhler-Rollefson I. (2004). “Farm animal genetic resources: Safeguarding national assets for food security and trade,” in A summary of workshops on FAnGR held in the Southern African Development Community (SADC). Eschborn, Germany: GTZ; Rome, Italy: FAO and Wageningen; Netherlands: CTA.
Kop‐bozbay C., Akdag A., Bozkurt‐kiraz A., Gore M., Kurt O., Ocak N. (2021). Laying performance, egg quality characteristics, and egg yolk fatty acids profile in layer hens housed with free access to chicory- and/or white clover-vegetated or non-vegetated areas. Animals 11, 1708. doi: 10.3390/ANI11061708
Krakowska A., Rafińska K., Walczak J., Kowalkowski T., Buszewski B. (2017). Comparison of various extraction techniques of Medicago sativa: yield, antioxidant activity, and content of phytochemical constituents. J. AOAC Int. 100, 1681–1693. doi: 10.5740/jaoacint.17-0234
Křížová L., Dadáková K., Kašparovská J., Kašparovský T. (2019). Isoflavones. Molecules 24, 1076. doi: 10.3390/molecules24061076
Lagos L. V., Stein H. H. (2017). Chemical composition and amino acid digestibility of soybean meal produced in the United States, China, Argentina, Brazil, or India1. J. Anim. Sci. 95, 1626–1636. doi: 10.2527/jas.2017.1440
Laudadio V., Ceci E., Lastella N. M. B., Introna M., Tufarelli V. (2014). Low-fiber alfalfa (Medicago sativa L.) meal in the laying hen diet: Effects on productive traits and egg quality. Poultry Sci. 93, 1868–1874. doi: 10.3382/PS.2013-03831
Liu Q. H., Dong Z. H., Shao T. (2018). Effect of additives on fatty acid profile of high moisture alfalfa silage during ensiling and after exposure to air. Anim. Feed Sci. Technol. 236, 29–38. doi: 10.1016/j.anifeedsci.2017.11.022
Luo C., Wang D., Lu N., Li H., Liu G., Cao Z., et al. (2022). Analysis of chemical composition, amino acid content, and rumen degradation characteristics of six organic feeds. Animals 12, 682. doi: 10.3390/ani12060682
Manyelo T. G., Sebola N. A., Mabelebele M. (2022). Effect of amaranth leaf meal on performance, meat, and bone characteristics of Ross 308 broiler chickens. PLoS One 17 (8). doi: 10.1371/JOURNAL.PONE.0271903
Mapiye C., Mwale M., Mupangwa J. F., Chimonyo M., Foti1 R., Mutenje1 M. J. (2008). A research review of village chicken production constraints and opportunities in Zimbabwe. Asian-Australian J. Anima Sci. 21, 1680–1688. doi: 10.5713/ajas.2008.r.07
Markovic J., Radovic J., Lugic Z., Sokolovic D. (2007). The effect of development stage on chemical composition of alfalfa leaf and stem. Biotechnol. Anim. Husbandry 23, 383–388. doi: 10.2298/BAH0702383M
McDonald P., Greenhalgh J. F. D., Morgan C. A., Edwards R., Sinclair L., Wilkinson R. (2011). Animal Nutrition. 7th ed (London: Pearson).
Medugu C. I., Saleh B., Igwebuike J. U., Ndirmbita R. L. (2012). Strategies to improve the utilization of tannin-rich feed materials by poultry. Int. J. Poultry Sci. 11, 417. doi: 10.3923/ijps.2012.417.423
Mielmann A., Bothma C., Steyn F., Hugo C. J., Mielmann A., Bothma C., et al. (2017). Consumers’ knowledge about and attitudes towards lucerne (Medicago sativa L.). J. Consumer Sciences Special Edition Food Nutr. challenges South. Afr. 2, 69–82.
Motsepe R., Mabelebele M., Norris D., Brown D., Ngambi J., Ginindza M. (2016). Carcass and meat quality characteristics of South African indigenous chickens. Indian J. Anim. Res. 50, 580–587. doi: 10.18805/ijar.11159
Mottet A., Tempio G. (2017). Global poultry production: Current state and future outlook and challenges. World's Poultry Sci. J. 73, 245–256. doi: 10.1017/S0043933917000071
Moyo B., Masika P. J., Hugo A., Muchenje V. (2011). Nutritional characterization of Moringa (Moringa oleifera Lam.) leaves. Afr. J. Biotechnol. 10, 12925–12933. doi: 10.5897/AJB10.1599
Mtileni B., Dzama K., Nephawe K., Rhode C. (2016). Estimates of effective population size and inbreeding in South African indigenous chicken populations: implications for the conservation of unique genetic resources. Trop. Anim. Health Production 48, 943–950. doi: 10.1007/s11250-016-1030-9
Mwai L. M. (2021). Mulberry (Morus alba) leaf meal in indigenous chicken layer diets effect on egg production and quality. MSc thesis (Kenya: Egerton University).
Mwalusanya N. A., Katule A. M., Mutayoba S. K., Mtambo M. M. A., Olsen J. E., Minga U. M. (2002). Productivity of local chickens under village management conditions. Trop. Anim. Health Production 34, 405–416. doi: 10.1023/a:1020048327158
Nagy B., Makleit P. (2021). Total saponin content (TSC) of different alfalfa (Medicago sativa l.) cultivars cultivated in field experiment. Rev. Agric. Rural Dev. 10, 176–180. doi: 10.14232/rard.2021.1-2.176-180
Nakamura M. T., Nara T. Y. (2003). Essential fatty acid synthesis and its regulation in mammals. Prostaglandins Leukotrienes Essential Fatty Acids 68, 145–150. doi: 10.1016/S0952-3278(02)00264-8
Ncobela C. N., Chimonyo M. (2015). Potential of using non-conventional animal protein sources for sustainable intensification of scavenging village chickens: A review. Anim. Feed Sci. Technol. 208, 1–11. doi: 10.1016/J.ANIFEEDSCI.2015.07.005
Nondzutha T., Mdoda L., Qange S. (2020). The profitability and contribution of indigenous chicken production towards improving household well-being in Lusikisiki. J. Hum. Ecol. 71, 212–221. doi: 10.31901/24566608.2020/71.1-3.3257
NRC (National Research Council) (1994). Nutrient requirements of poultry. 9th ed (Washington DC: National Academy Press).
Nxumalo N., Ceccobelli S., Cardinali I., Lancioni H., Lasagna E., Kunene N. W. (2020). Genetic diversity, population structure and ancestral origin of KwaZulu-Natal native chicken ecotypes using microsatellite and mitochondrial DNA markers. Ital. J. Anim. Sci. 19, 1277–1290. doi: 10.1080/1828051X.2020.1838350
Nyoni N. M. B., Grab S., Archer E., Hetem R. (2022). Perceived impacts of climate change on rural poultry production: a case study in Limpopo Province, South Africa. Climate Dev. 14, 389–397. doi: 10.1080/17565529.2021.1929803
Ouyang K., Xu M., Jiang Y., Wang W. (2016). Effects of alfalfa flavonoids on broiler performance, meat quality, and gene expression. Can. J. Anim. Sci. 96, 332–341. doi: 10.1139/cjas-2015-0132
Palmonari A., Fustini M., Canestrari G., Grilli E., Formigoni A. (2014). Influence of maturity on alfalfa hay nutritional fractions and indigestible fiber content. J. Dairy Sci. 97, 7729–7734. doi: 10.3168/jds.2014-8123
Paredes M., Risso A. L. (2020). Effects of dietary inclusion of alfalfa meal on productive performance, carcass and weight of digestive and lymphoid organs in organic type broiler chicken. Rev. Investigaciones Veterinarias del Peru 31 (2). doi: 10.15381/rivep.v31i2.17846
Picoli K. P., Murakami A. E., Duarte C. R. A., Eyng C., Ospina-Rojas I. C., Massuda E. M. (2014). Effect of dietary restriction and hay inclusion in the diet of slow-growing broilers. Ital. J. Anim. Sci. 13, 771–775. doi: 10.4081/IJAS.2014.3216
Pleger L., Weindl P. N., Weindl P. A., Carrasco L. S., Leitao C., Zhao M., et al. (2021). Precaecal digestibility of crude protein and amino acids from alfalfa (Medicago sativa) and red clover (Trifolium pratense) leaves and silages in broilers. Anim. Feed Sci. Technol. 275, 114856. doi: 10.1016/J.ANIFEEDSCI.2021.114856
Pleger L., Weindl P. N., Weindl P. A., Carrasco L. S., Leitao C., Zhao M., et al. (2020). Effects of increasing alfalfa (Medicago sativa) leaf levels on the fattening and slaughtering performance of organic broilers. J. Anim. Physiol. Anim. Nutr. 104, 1317–1332. doi: 10.1111/JPN.13353
Ponte P. I. P., Mendes I., Quaresma M., Aguiar M. N. M., Lemos J. P. C., Ferreira L. M. A., et al. (2004). Cholesterol levels and sensory characteristics of meat from broilers consuming moderate to high levels of alfalfa. Poultry Sci. 83, 810–814. doi: 10.1093/PS/83.5.810
Prihambodo T. R., Sholikin M. M., Nahrowi N., Batubara I., Utomo D. B., Jayanegara A. (2022). Flavonoids as dietary additives in laying hens: a meta-analysis of production performance, egg quality, liver, and antioxidant enzyme profile. Poultry Sci. J. 10 (1), 27–34. doi: 10.22069/PSJ.2022.19393.1714
Prihambodo T. R., Sholikin M. M., Qomariyah N., Jayanegara A., Batubara I., Utomo D. B., et al. (2021). Effects of dietary flavonoids on performance, blood constituents, carcass composition and small intestinal morphology of broilers: a meta-analysis. Anim. Bioscience 34, 434–442. doi: 10.5713/ajas.20.0379
Radović J., Sokolović D., Marković J. (2009). Alfalfa-most important perennial forage legume in animal husbandry. Biotechnol. Anim. Husbandry 25, 465–475. doi: 10.2298/BAH0906465R
Raphael W., Sordillo L. M. (2013). Dietary polyunsaturated fatty acids and inflammation: the role of phospholipid biosynthesis. Int. J. Mol. Sci. 14, 21167–21188. doi: 10.3390/IJMS141021167
Raphulu T., Jansen Van Rensburg C., Van Ryssen J. B. J. (2015). Assessing nutrient adequacy from the crop contents of free-ranging indigenous chickens in rural villages of the Venda region of South Africa. South Afr. J. Anim. Sci. 45, 143–152. doi: 10.4314/sajas.v45i2.5
Rashid M., Asaduzzaman, R. B. C. (2003). Chemical Composition of crop contents of local scavenging chickens. Pakistan J. Nutr. 3, 26–28. doi: 10.3923/PJN.2004.26.28
Rosa P. M., Antoniassi R., Freitas S. C., Bizzo H. R., Zanotto D. L., Oliveira M. F., et al. (2009). Chemical composition of Brazilian sunflower varieties. Helia 32, 145–156. doi: 10.2298/hel0950145r
Russelle M. P. (2001). Alfalfa: After an 8,000-year journey, the “Queen of Forages” stands poised to enjoy renewed popularity. Am. Scientist 89, 252–261. doi: 10.1511/2001.3.252
Sánchez Q. ,. A., Rodrigue H. R., Pinza D. V. C., Troya G. A. P., Calco A., Diaz C. A. A. (2022). Effect of the inclusion of Medicago sativa in feed chicken Cobb 500. Revista Científica Facultad de Ciencias Veterinariasa 32, 1–7. doi: 10.52973/RCFCV-E32108
Scholtz G., Van der Merwe H., T. T. (2009). The nutritive value of South African Medicago sativa L. hay. Afr. Journals Online 39 (1), 179–182.
Sebola N. A., Mlambo V., Mokoboki H. K., Muchenje V. (2015). Growth performance and carcass characteristics of three chicken strains in response to incremental levels of dietary Moringa oleifera leaf meal. Livestock Sci. 178, 202–208. doi: 10.1016/j.livsci.2015.04.019
Sen S., Makkar H. P. S., Becker K. (1998). Alfalfa saponins and their implication in animal nutrition. J. Agric. Food Chem. 46, 131–140. doi: 10.1021/JF970389I
Shah A., Smith D. L. (2020). Flavonoids in agriculture: Chemistry and roles in, biotic and abiotic stress responses, and microbial associations. Agronomy 10, 1209. doi: 10.3390/agronomy10081209
Shirzadegan K., Taheri H. R. (2017). Insoluble fibers affected the performance, carcass characteristics and serum lipid of broiler chickens fed wheat-based diet. Iranian J. Appl. Anim. Sci. 7, 109–117.
Srisaikham S. (2021). A comparison of nutritional values, bioactive compounds, amino acids, and antioxidant activities of alfalfa (Medicago sativa) plant and Pellet for use as beneficial material ruminant feed. Walailak J. Sci. Technol. 18, 10312–10316. doi: 10.48048/wjst.2021.10312
Stavarache M., Vintu V., Samuil C., Muntianu I., Albu A., Tarcău D., et al. (2012). Quality of alfalfa (Medicago sativa L.), in the first year of vegetation. Lucrări Ştiinţifice seria Agronomi 1, 55–60.
Sugiharto S., Yudiarti T., Isroli I., Widiastuti E., Wahyuni H. I., Sartono T. A. (2019). Recent advances in the incorporation of leaf meals in broiler diets. Livestock Res. Rural Dev. 31 (7).
Surai P. F. (2014). Polyphenol compounds in the chicken/animal diet: from the past to the future. J. Anim. Physiol. Anim. Nutr. 8, 19–31. doi: 10.1111/jpn.12070
Suwignyo B., Rini E. A., Fadli M. K., Ariyadi B. (2021). Effects of alfalfa (Medicago sativa L.) supplementation in the diet on the growth, small intestinal histomorphology, and digestibility of hybrid ducks. Veterinary World 14, 2719. doi: 10.14202/VETWORLD.2021.2719-2726
Szumacher-Strabel M., Stochmal A., Cieslak A., Kozłowska M., Kuznicki D., Kowalczyk M., et al. (2019). Structural and quantitative changes of saponins in fresh alfalfa compared to alfalfa silage. J. Sci. Food Agric. 99, 2243–2250. doi: 10.1002/JSFA.9419
Tessema Z., Baars R. (2006). Chemical composition, dry matter production and yield dynamics of tropical grasses mixed with perennial forage legumes. Trop. Grasslands 40 (3), 150.
Tkáčová J., Angelovičová M., Mrázová Ľ., Kliment M., Král M. (2011). Effect of different proportion of lucerne meal in broiler chickens. Sci. Papers Anim. Sci. Biotechnologies 44, 141–144. doi: 10.3/JQUERY-UI.JS
Toral P., Hervás G., Missaoui H., Andrés S., Giráldez F., Jellali S., et al. (2016). Effects of a tannin-rich legume (Onobrychis viciifolia) on in vitro ruminal biohydrogenation and fermentation. Spanish J. Agric. Res. 14, e0602. doi: 10.5424/sjar/2016141-8989
Ubua J. A., Ozung P. O., Inagu P. G. (2019). Dietary inclusion of neem (Azadirachta indica) leaf meal can influence growth performance and carcass characteristics of broiler chickens. Asian J. Biol. Sci. 12, 180–186. doi: 10.3923/ajbs.2019.180.186
Vardhan P. V., Shukla L. I. (2017). Gamma irradiation of medicinally important plants and the enhancement of secondary metabolite production. Int. J. Radiat. Biol. 93 (9), 967–979. doi: 10.1080/09553002.2017.1344788
Varzaru I., Panaite T. D., Untea A. E. (2020). Effects of dietary supplementation of alfalfa meal and rice bran on growth performance, carcass characteristics and intestinal microbiota in broilers. Archiva Zootechnica 23, 117–128. doi: 10.2478/AZIBNA-2020-0017
Vlaicu P. A., Untea A. E., Turcu R. P., Saracila M., Varzaru I., Oancea A. G. (2023). Chemical composition of dietary alfalfa and its effectiveness on broiler chicken thigh meat quality. Czech J. Food Sci. 41, 279–286. doi: 10.17221/79/2023-CJFS
Wang E., Wang J., Lv J., Sun X., Kong F., Wang S., et al. (2021). Comparison of ruminal degradability, indigestible neutral detergent fiber, and total-tract digestibility of three main crop straws with alfalfa hay and corn silage. Animals 11, 3218. doi: 10.3390/ani11113218
Wonkyun C., Ryong K., Song L. H., Ro L. J. (2020). A Multiplex PCR method for the detection of genetically modified alfalfa (Medicago sativa L.) and analysis of feral alfalfa in South Korea. Proc. Natl. Institute Ecol. 1, 83–89. doi: 10.22920/PNIE.2020.1.1.83
Wyse J. M., Latif S., Gurusinghe S., Berntsen E. D., Weston L. A., Stephen C. P. (2021). Characterization of phytoestrogens in Medicago sativa L. and grazing beef cattle. Metabolites 11, 550. doi: 10.3390/metabo11080550
Xu X., Min D., McDonald I. (2021). Effects of harvest intervals and seeding rates on dry matter yield and nutritive value of alfalfa cultivars. J. Anim. Sci. Technol. 63 (5), 1098–1113. doi: 10.5187/jast.2021.e97
Yusuf S. F. G., Lategan F. S., Masika P. J. (2017). Characterization of Indigenous poultry production systems in the Nkonkobe Municipality, Eastern Cape Province South Africa. Kamla Raj Enterprises 5, 31–44. doi: 10.1080/09766898.2014.11884710
Keywords: Medicago sativa, poultry, diet, protein source, fibre, saponins
Citation: Ginindza MM (2023) Lucerne meal in the diet of indigenous chickens: a review. Front. Anim. Sci. 4:1274473. doi: 10.3389/fanim.2023.1274473
Received: 08 August 2023; Accepted: 07 November 2023;
Published: 19 December 2023.
Edited by:
Juana Catarina Cariri Chagas, Swedish University of Agricultural Sciences, SwedenReviewed by:
Sakine Yalçin, Ankara University, TürkiyeGabriel Da Silva Viana, Natural Resources Institute Finland (Luke), Finland
Copyright © 2023 Ginindza. This is an open-access article distributed under the terms of the Creative Commons Attribution License (CC BY). The use, distribution or reproduction in other forums is permitted, provided the original author(s) and the copyright owner(s) are credited and that the original publication in this journal is cited, in accordance with accepted academic practice. No use, distribution or reproduction is permitted which does not comply with these terms.
*Correspondence: Muzi M. Ginindza, gininmm@unisa.ac.za