Toxicological, biochemical, and histopathological evaluation of rats fed with macrofungal-treated cottonseed cake
- 1Federal University of Grande Dourados, Dourados, Mato Grosso do Sul, Brazil
- 2Federal University of Mato Grosso, Cuiabá, Mato Grosso, Brazil
- 3Federal University of Tocantins, Gurupi, Tocantins, Brazil
- 4University of Brasilia, Brasilia, Brazil
- 5Embrapa Agroenergia, Brasilia, Brazil
Gossypol is a terpene-polyphenolic compound specific to cotton plants. Considered as an anti-nutritional factor, monogastric livestock show greater sensitivity to this metabolite than ruminants. The aim of this investigation was to evaluate the toxicological effects of gossypol-containing and biodetoxified cottonseed-cake on hepatic function in rats. Biodetoxification of cottonseed cake was performed by the macrofungi Fistulina hepatica BRM 047114 and Pleurotus ostreatus BRM 055505 in a solid-state cultivation process. A toxicological study was performed using albino Wistar rat males (Rattus norvergicus). A feed assay was conducted using the macrofungi cultivated on cottonseed cake (5%, 15%, and 25%). Animal growth, feed conversion, hemogram, serum biochemical analyses and histopathology were evaluated. For biodetoxified cottonseed cake treatments with both macrofungi, concentrations of feed up to 15% resulted in animal development similar to that of the untreated group. Histopathological analysis after feeding with biodetoxified cottonseed cake presented an unaltered cordonal arrangement. Nevertheless, the group fed with cottonseed cake biodetoxified using F. hepatica BRM 047114 showed discrete inflammatory infiltrate, vacuolization, and pyknotic nuclei in hepatocytes. P. ostreatus BRM 055505 resulted in efficient reduction in toxic effects of free gossypol, with regular animal growth, maintenance of normal liver activities, and a reduction in circulating cholesterol levels. In conclusion, P. ostreatus and F. hepatica were able to minimize the toxic effects of free gossypol in rat diets, representing an important contribution for feed-formulation development for non-ruminants.
1 Introduction
Gossypium sp. are oilseed plants that generate biomass residue with significant levels of edible oil, crude protein, and diverse biomolecules appropriate for animal nutrition. The application of cottonseed cake, a by-product of cottonseed processing, as a potential nutrient source for animal feed is limited due to the presence of high concentrations of gossypol (Mane et al., 2007). The gossypol molecule (C30H30O8) is a terpenopolyphenol secondary metabolite toxin that naturally plays a role in plant defense responses in cotton to predators (insect herbivores and nematodes) and occurs throughout the plant tissues (seeds, leaves, bark, flower buds, and roots).
Considered an anti-nutritional factor, monogastric livestock shows greater sensitivity to this metabolite than ruminants, with diets containing gossypol leading to systemic adverse effects that include, among others, immunosuppression, weight loss, weakness, male and female reproductive impairment, liver damage, respiratory problems, heart failure and death (Fonseca et al., 2013; Câmara et al., 2015).
Gossypol exists in the cotton plant in both free (FG) and bound (BG) forms. Within cottonseed tissues, concentrations above of 14,000 mg/kg of total gossypol and 7,000 mg/kg of FG have been reported (Alexander et al., 2009). Cottonseed cake is a by-product of the mechanical extraction of cottonseed oil and represents globally an important source of protein in feed for livestock production, surpassed only by soybean (Gadelha et al., 2014). While FG is the main antinutritional factor present in cottonseed cake, thermal methods applied during oil extraction and cottonseed cake production also favor the formation of gossypol complexes with essential amino acids such as lysine, resulting in a decrease in feed digestibility (Paiano et al., 2014).
Despite the scale of the cottonseed industry (4.91 million tons in 2018) and the potential in the nutritional characteristics of cottonseed for feed industries, the employment of cottonseed cake in non-ruminant animal feed applications is currently not recommended or only limited (Martínez-Herrera et al., 2006). Biodetoxification of such crop biomass by-products through solid-state cultivation can, however, not only eliminate toxic metabolites, but also improve nutritional value, increasing available protein content and bioactive molecules, and reducing lignin and fiber fractions. Such an approach has recently been successfully applied to the reduction of toxins in oleaginous plants such as Jatropha curcas (Gomes et al., 2018), with biological treatment of whole cottonseed with macrofungi also reported to eliminate FG (Neto et al., 2021).
The macrofungi genera of Pleurotus and Fistulina, utilized in both food and medicine purposes, stand out among fungi capable of nutraceutically enhancing residual biomass. Depending on the substrate, they exhibit a high daily growth rate with efficient conversion of proteins and β-glucans, making them suitable for animal feed (Conceição et al., 2022).
This study was performed to evaluate the potential toxic effects on Wistar rat hepatic tissues following the intake of formulated feed, contrasting the presence of untreated and macrofungal-treated cottonseed cake.
2 Materials and methods
2.1 Agro-industrial residue raw materials and macrofungi
Agro-industrial biomasses employed in substrate formulations, namely cottonseed cake (Gossypium sp.), elephant grass (Pennisetum purpureum), eucalyptus sawdust (Eucalyptus sp.), oil palm mesocarp fiber (Elaeis guineensis) and sugarcane bagasse (Saccharum sp.), were obtained from the raw material stock collection of lignocellulosic biomasses at Embrapa Agroenergy.
The fungal strains Fistulina hepatica BRM 047114 and Pleurotus ostreatus BRM 055505 were obtained from the Collection of Microorganisms and Microalgae Applied to Agroenergy and Biotechnology (CMMAABio) at Embrapa Agroenergy. These were selected on the basis of previous results highlighting their potential in the biodetoxification of cottonseed (Conceição et al., 2018).
2.2 Animal experiment: laboratory conditions
A total of 110 nine-week-old male Wistar rats (Rattus norvegicus), weighing 163 ± 6.8 g, were maintained at the Federal University of Mato Grosso Sul (UFMS) animal facility in collective cages. Controlled conditions were employed, comprising a temperature of 26°C and a 12h:12h light: dark cycle. All animals were given access to feed and water ad libitum during the 28-day period of tolerance assays. All animal care and experiments complied with the regulations established by the appropriate authorizing committee (CEUA/UFGD-49/2016), in accordance with the National Research Council’s Guide for the Care and Use of Laboratory Animals (National Academic Press, 2010).
2.3 Solid fermentation
For substrate culture preparation, a total of 70 g (dry matter) of cottonseed cake, previously moistened to 60% with distilled water, was autoclaved at 121°C for 15 min (physical treatment). The substrate was then inoculated with five 15 mm diameter mycelial plugs of F. hepatica BRM 047114 and P. ostreatus BRM 055505, previously grown for seven days on potato dextrose agar (PDA). Solid-state cultivation cultures were then incubated for 20 days at 28°C for subsequent FG degradation analysis.
In order to obtain optimal biomass combinations for both fungal growth and productivity (mushroom production) for the selected fungi, 70% cottonseed cake was included in the substrate together with the addition of a 30% portion of one of the following agroindustrial residue biomasses: T1: eucalyptus sawdust; T2: sugarcane bagasse; T3: palm oil fruit pressing fiber; T4: elephant grass. The moisture content of all substrates was adjusted to 70%. Approximately 2.00 ± 0.02 kg of each substrate formulation was distributed into High Density Polyethylene bags (HDPE) containing sterile air exchange filters. Bags containing the substrates were then autoclaved at 121°C for 90 min.
For productivity determination, substrates were inoculated after cooling with 10 g of spawn (mycelial mass) of each fungal strain grown previously on brown rice substrate. After inoculation, bags were maintained at 26°C ± 2 and 70% humidity. After 34 days cultivation and complete mycelial colonization of the substrates, blocks were transferred to a mushroom-fructification room, with humidity maintained between 80% to 90% until fruiting.
2.4 P. ostreatus: mushroom biological efficiency (BE) and productivity (P)
Mushroom fructification was observed only for P. ostreatus BRM 055505. After harvesting, biological efficiency (BE) and productivity (P) were evaluated according to the following equations:
2.5 Preparation of rat diets
The basic diet employed for feeding was a commercial formulation containing ground whole corn, soybean meal, wheat bran, calcium carbonate, dicalcium phosphate, sodium chloride, vitamin premix (Vitamin A 12,000.00 IU; Vitamin D3 1,800.00 IU; Vitamin E 30.00 mg; Vitamin K3 3.00 mg; Vitamin B1 5.00 mg; Vitamin B2 6.00 mg; Vitamin B6 7.00 mg; Vitamin B12 20.00 mcg; Niacin 60.00 mg; Pantothenic Acid 20.00 mg; Folic Acid 1.00 mg, Biotin 0.05 mg; Choline 600.00 mg) minerals (Iron 50.00 mg; Zinc 60.00 mg; Copper 10.00 mg; Iodine 2.00 mg; Manganese 60.00 mg; Selenium 0.05 mg; Cobalt 1.50 mg.) and amino acids (Lysine 100.00 mg; Methionine 300.00 mg) (Labina Purina Ltd).
Diets employed for cottonseed cake tolerance assays were prepared with different inclusion levels of biodetoxified cottonseed cake (5, 15 or 25%). Control diets were set up using the same levels of untreated cottonseed cake.
Two colonized substrates were selected for tolerance assays based on lowest achievable levels of FG obtained following cultivation and efficiency analysis of fungal cultivation: 1) cottonseed cake biodetoxified by P. ostreatus (from spent mushroom substrate (SMS)) following colonization of cottonseed cake (70%) plus eucalyptus sawdust (30%) over a 34 day period, plus a further 30 days for fruiting and mushroom harvesting; 2) cottonseed cake biodetoxified by F. hepatica following colonization of cottonseed cake (70%) plus sugarcane bagasse (30%) over a 34 day period. Both samples were dried for 48 h at 65°C, powdered with a knife mill (0.5 mm sieve) and maintained at -20°C for further analysis.
All formulations were homogenized, and 30% distilled water added (w/v). Pelletized diets were oven dried at 65°C for 12 h and subsequently stored in plastic containers at 4°C to ensure quality throughout the study.
2.5.1 Cottonseed cake tolerance assay
Forty randomly selected rats were allocated into four equally sized groups. Over a period of 28 days, each group was fed on a different diet, which comprised three different levels of cottonseed cake (5, 15, and 25%) and one control group (commercial diet).
2.5.2 Biodetoxified cottonseed cake tolerance assay
Seventy male Wistar rats were employed in different groups. Diets were prepared using three different levels of biodetoxified cottonseed cake: i) experimental groups with 5, 15, and 25% of spent mushroom substrate (SMS) following growth of P. ostreatus ii) experimental groups with 5, 15, and 25% of colonized substrate following growth of F. hepatica; and one control group (commercial diet).
2.6 Chemical, biochemical and behavior analyses
2.6.1 Bromatological analysis
Bromatological analyses were performed on toxic cottonseed cake and biodetoxified cottonseed cake commercial diets, as well as on formulated diets. Moisture, crude protein, ethereal extract, and ash (mineral matter) content were determined according to the methodology described by the Association of Official Analytical Chemists (AOAC, 2000).
FG content in all samples was analyzed by High Performance Liquid Chromatography (HPLC), according to Conceição et al. (2018). Components were separated using a C18 reverse phase Kinetex column (100 x 2.10 mm, 2.6 μm), with a methanol-0.1% TFA aqueous solution employed as mobile phase. PDA detection was conducted at 254 nm.
2.6.2 Animal behavior
Rats were monitored daily for clinical signs of toxicity (piloerection, tremor, and death) and for behavioral changes (irritability and aggressiveness). To measure feed intake, a predetermined amount of feed was provided to the animals daily ad libitum. The remaining portion was then weighed to calculate the amount ingested each time new feed was provided. Water was also provided ad libitum to the animals without measurement.
2.6.3 Animal growth
Feed intake was monitored throughout the entire experimental period to enable calculation of average daily intake. Animal growth was evaluated on the basis of the parameters of final weight, feed intake, weight gain (WG), protein intake (PI), feed conversion (FC), specific growth rate (SGR), protein efficiency ratio (PER), and survival (S), as follows:
Animal Weight Gain (WG):
Protein Intake (PI):
Feed conversion (FC):
Specific growth rate (SGR):
Protein efficiency ratio (PER):
Animal Survival (S):
After feeding and overnight fasting, animals were anesthetized with ketamine and xylazine (i.p. 20 and 10 mg/kg, respectively). Blood was collected by cardiac puncture and centrifuged (5 min, 3000 g), then plasma was aliquoted and stored at −80°C for analysis. Livers were dissected and weighed for calculation of the hepatosomatic index (HSI = Organ weight/Animal weight).
2.6.4 Hematological analysis
Blood samples from rats were collected and placed in tubes containing anticoagulant (LABOR IMPORT ®). Hematological analysis was performed using a Sysmex KX-21N hematological counter. This analysis was divided into erythrogram, which corresponds to counting and morphological analysis of erythrocytes, and leukogram, which corresponds to counting and morphological analysis of leukocytes.
2.6.5 Biochemical analysis
Analysis of biochemical parameters was performed on plasma. Total cholesterol and albumin levels were determined by colorimetric enzymatic assays. Aspartate aminotransferase (AST) and alanine aminotransferase (ALT) levels were determined by a kinetic test performed with the COBAS MIRA PLUS® (Roche), employing the Gold Analisa Kits Diagnóstica®.
2.6.6 Histopathological analysis
At the end of the experiment, animals were sacrificed by exsanguination. Representative liver fragments were fixed in formaldehyde for 24 h, washed in 70% alcohol, dehydrated, diaphanized, and embedded in paraffin with Histosec plastic polymer (Merck). Slices 2 to 5 μm in thickness were obtained and stained with Hematoxylin & Eosin, with additional slices stained by the histochemical PAS-H method.
Microscopic analyses, histomorphometry, and material documentation were performed on an Olympus BX41 photomicroscope. Two slides per treatment were selected for morphometry analysis, in which a total of seven sections were photographed. The diameter of hepatocytes and their nuclei were subsequently measured using the Image Pro Plus Media Cybernetics software (version 4.5) for calculation of the hepatocyte: hepatocyte nucleus ratio.
2.7 Statistical analysis
The results were submitted to the normality test and to the homogeneity test of the variances. For variables whose distribution was normal, the analysis of variance was applied and when significant, the means were compared by the Tukey test at 5% probability. Toxicity analysis of the diets containing 5, 15, or 25% of the cottonseed cake or biodetoxified substrates followed a completely randomized design, with four treatments and ten replicates. Statistical analyses were performed using RStudio software (version 1.1.423, 2009-2018 RStudio, Inc.).
3 Results
3.1 Cultivation of macrofungi and gossypol biodegradation
As expected, given the growth temperature of 28°C employed for FG degradation analysis, no mushroom production was observed following solid-state cultivation of F. hepatica BRM 047114, with this fungal species typically requiring a lower temperature of approximately 15°C for fruiting (Hattori and Tanaka, 1997). At the temperature employed, however, the substrate formulations favored abundant mycelial growth, with complete colonization of substrate and formation of the typical reddish mycelium of this fungus (Figure 1A). P. ostreatus in contrast, began producing fruiting bodies 3 days after opening solid-state cultivation bags in a high humidity fruiting room (Figure 1B).
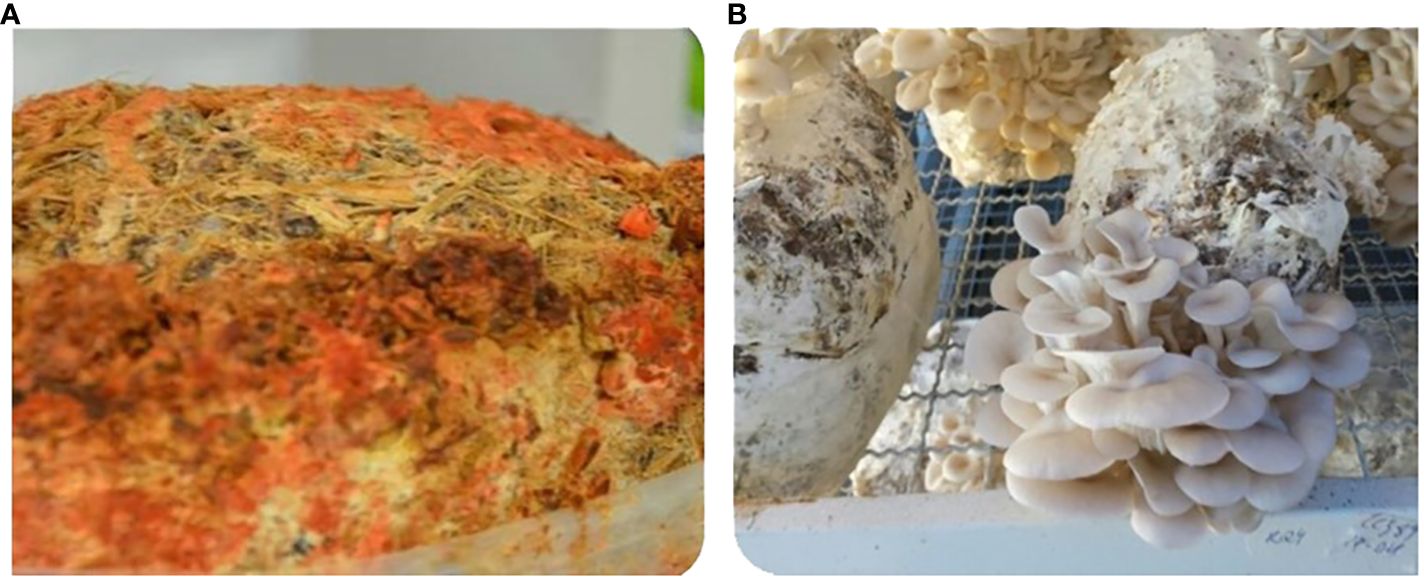
Figure 1 Macrofungal cultivation on substrates of cottonseed cake and agricultural biomass: (A) Solid-state cultivation with Fistulina hepatica (BCC-FH); (B) solid-state cultivation of Pleurotus ostreatus (BCC-PO) and fructification.
The treatment T2 (cottonseed cake: sugarcane bagasse) resulted in the greatest total mycelial colonization by F. hepatica (BCC-FH), with T1 (cottonseed cake: eucalyptus sawdust) most appropriate for P. ostreatus (BCC-PO). Biological efficiencies were observed in the region of 70% for T1 and 50% for T2, with percentage productivity not significantly different across the substrates (Figure 2). For treatments T3 and T4, a high contamination rate was observed (> 40%), likely as a result of the slow growth of the two fungi on these substrates permitting colonization by other undesirable microorganisms over time.
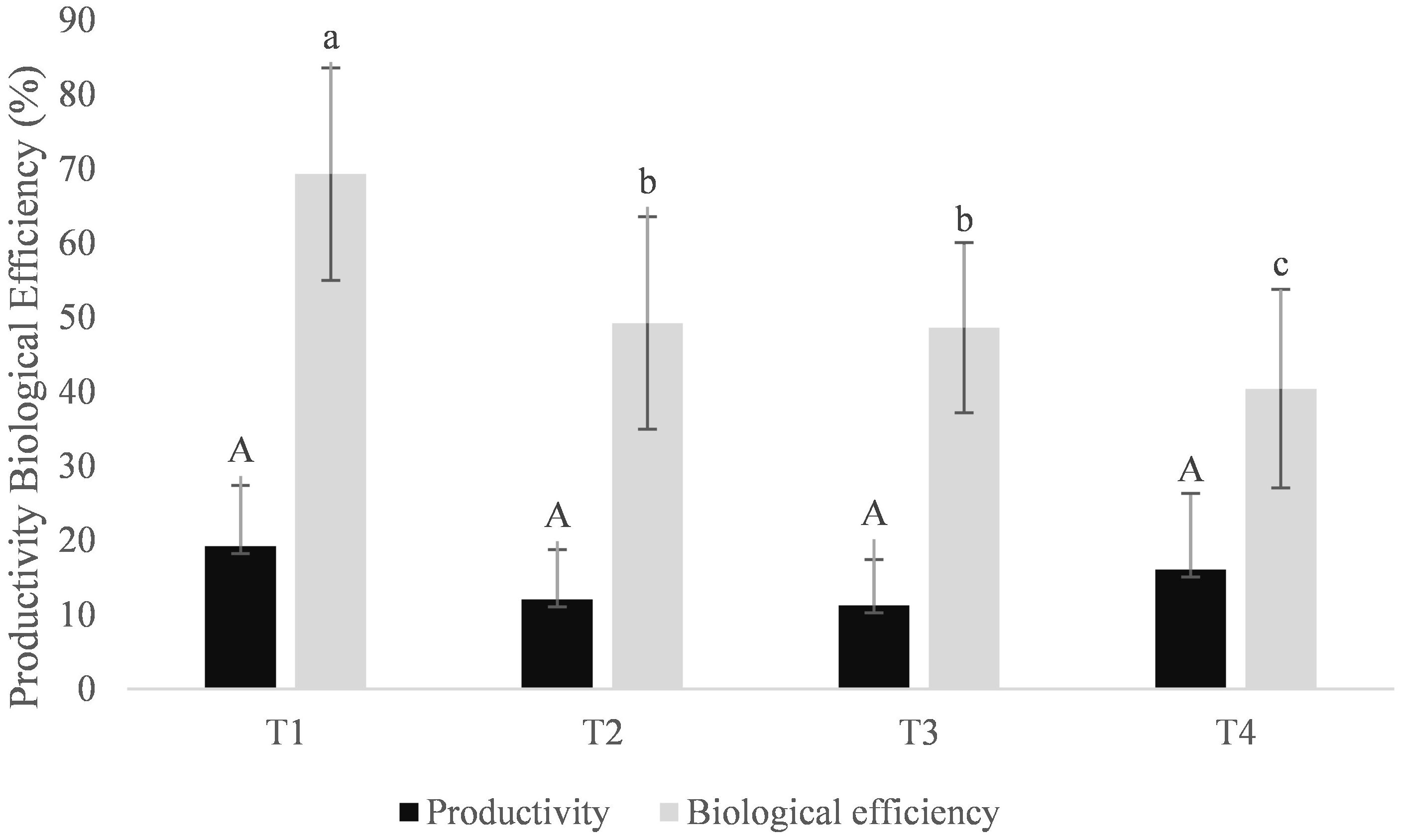
Figure 2 Biological efficiency and productivity during axenic cultivation of Pleurotus ostreatus on substrates containing cottonseed cake (70%) and other lignocellulosic sources (30%). T1: Eucalyptus sawdust; T2: Sugarcane bagasse; T3: Compressed oil palm fruit fiber; T4: Elephant grass. Based on the Tukey test (P<0.05), mean values that are followed by the same lower-case letter do not differ significantly in terms of biological efficiency. Similarly, mean values followed by the same upper-case letter do not differ significantly regarding productivity across treatments (P<0,05).
For both fungal strains, a significant reduction in FG content was observed following T1 and T2 biological assay treatments (Figure 3).
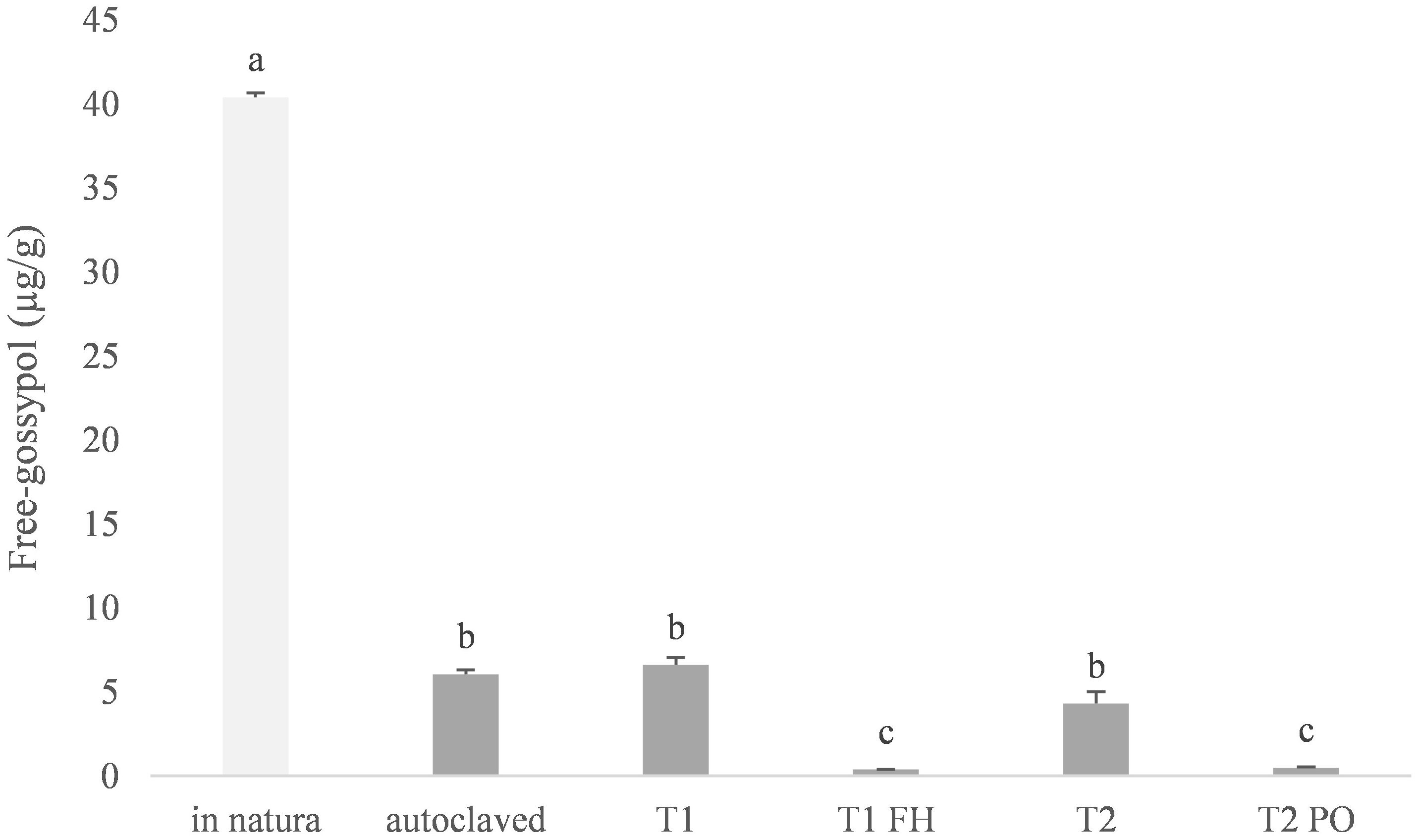
Figure 3 Free-Gossypol levels on cottonseed cake substrate and colonized substrate (Fistulina hepatica) or SMS (Pleurotus ostreatus). “In natura”: cottonseed cake without any treatment; Autoclaved: Cottonseed cake autoclaved for 30 min prior to inoculation; T1) autoclaved substrate of 70% cottonseed cake + 30% eucalyptus sawdust; T1-FH – F. hepatica colonized substrate from T1; T2) autoclaved substrate containing 70% cottonseed cake + 30% sugarcane bagasse; T2-PO – P. ostreatus spent mushroom substrate from T2. Means followed by the same lower-case letter do not differ by the Tukey test (P<0.05).
3.2 Diets
Formulations and diet compositions for toxic cottonseed cake feed assays and biodetoxified cottonseed cake feed assays are presented in Tables 1, 2, respectively.
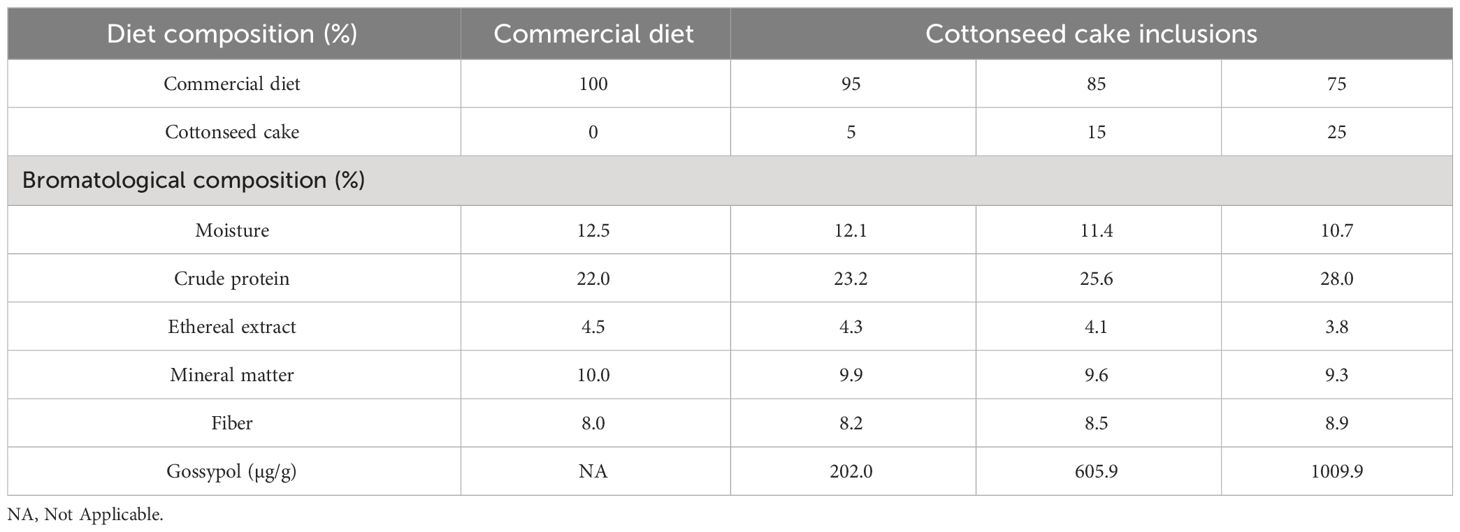
Table 1 Formulation and bromatological composition (%) of the diets containing untreated cottonseed cake.
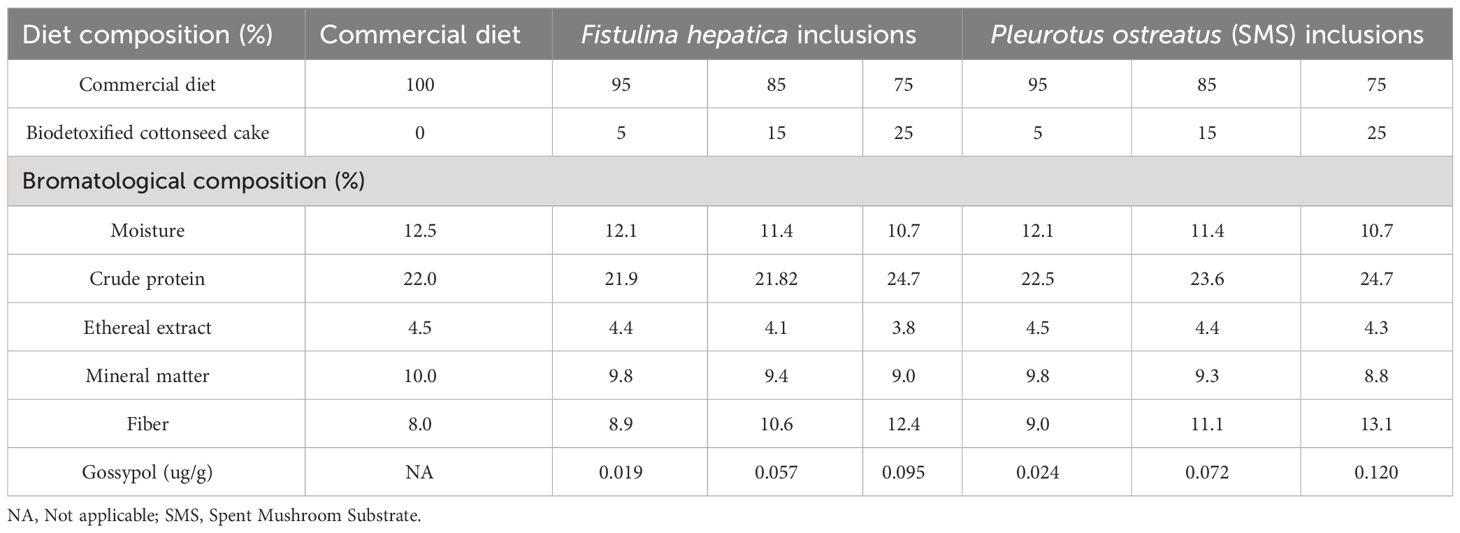
Table 2 Formulation and bromatological composition of the experimental diets containing biodetoxifed cottonseed cake.
3.3 Cottonseed cake toxicity assay
The objective of this assay was to determine the degree of toxicity of cottonseed cake containing gossypol. No deaths were observed in animals during the experimental period in which the feed was employed. However, rats did display observable behavioral changes, with increased aggressiveness and irritation, piloerection, and hair loss. Table 3 summarizes findings for recorded animal characteristics after 34 days of feeding on diets containing different cottonseed cake levels.
Weight gains followed the same pattern of feed intake, consequently reflecting differences in feed conversion (Table 3). The liver, the main organ to metabolize nutrients ingested in the diet, presented a negative response to the inclusion of cottonseed cake, with a loss of liver mass observed, which was reflected in the hepatosomatic index.
Biochemical parameters associated with hepatic functions were also affected, with ALT increasing as cottonseed cake content increased. Conversely, AST levels in rats fed on cottonseed cake were lower than those fed on control diets (Table 4). A significant decrease in serum albumin levels was observed in the group fed on cottonseed cake. Cholesterol levels were not altered by any diets during the toxicity test. Similarly, hemograms were also unaltered by the diets (data not shown).
Microscopical examination of the hepatic parenchyma in both control (Figure 4A) and experimental groups (Figure 4B) revealed normal lobular architecture, with hepatocytes arranged in cordonal arrangement radiating around the center vein and separated by blood sinusoids. Animals fed on cottonseed cake diet (15%) showed intensified cord disarrangement, cholestasis, and dilatation of hepatic sinusoids (Figure 4C). Animals fed on the diet of 25% cottonseed cake displayed hepatocellular necrosis and mild steatosis with inflammatory infiltrate (Figure 4D).
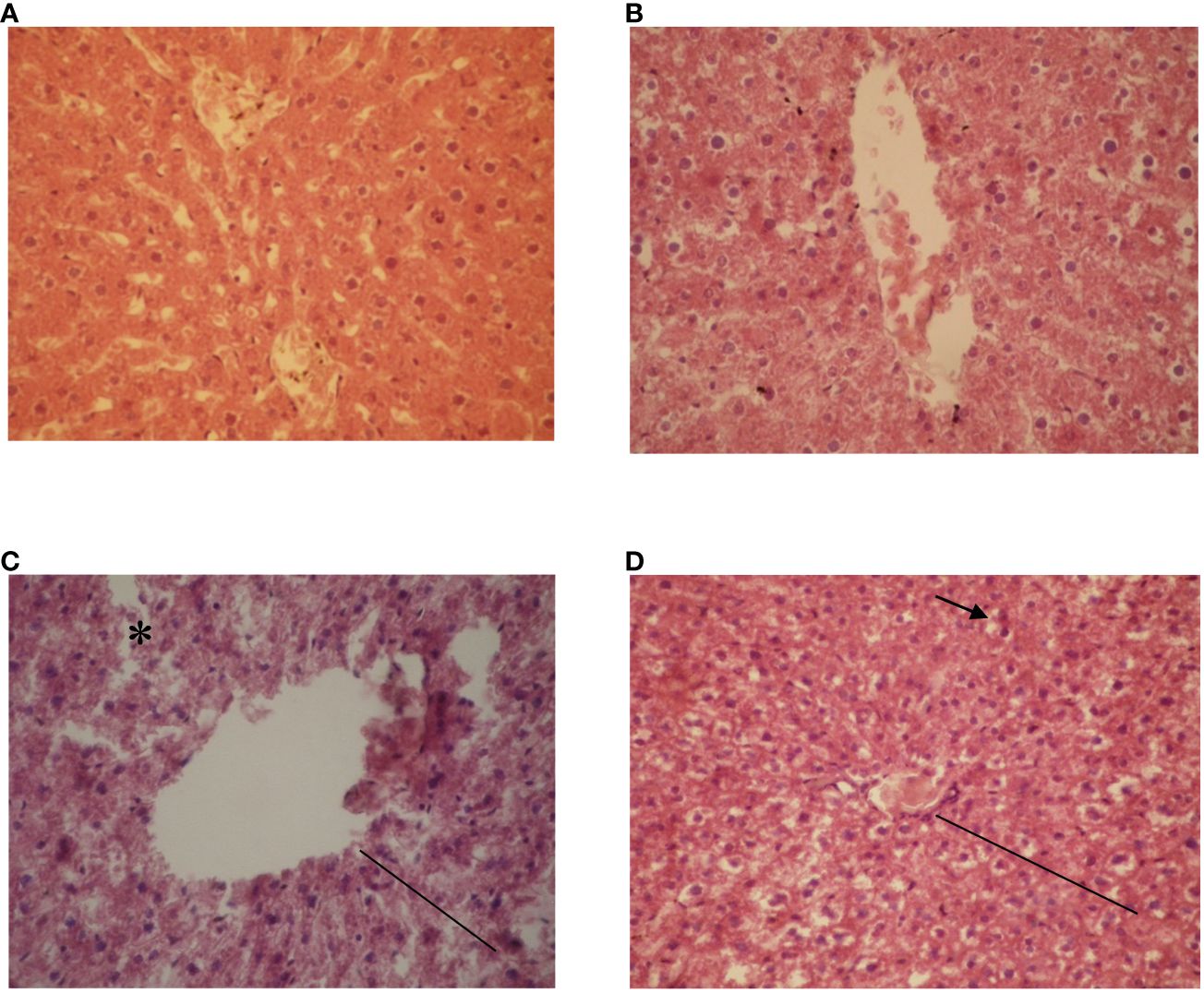
Figure 4 Photomicrography of liver sections from Wistar rats fed on feed diets containing cottonseed cake: (A) control group (commercial diet) displaying no histopathological alterations, with cord arrangement of hepatocytes (fine line) observed; (B) effect of cottonseed cake (5%), with presence of initial necrosis (in), clear cytoplasm (arrowhead); (C) effect of cottonseed cake (15%), occurrence of necrosis (*) and congestion; and, (D) effect of cottonseed cake (25%), occurrence of blood congestion (arrowhead and fine line). HE staining, 40X magnification.
3.4 Biodetoxified cottonseed cake assays
No mortality or changes in behavior were observed during experiments with different quantities of biodetoxified cottonseed cake. Feed intake, however, was not reflected in growth, with FC seen to be impaired as the inclusion of biodetoxified cottonseed cake increased. For both fungi, only increases of up to 15% biodetoxified cottonseed cake in feed resulted in animal development equal to that of control group animals (commercial diet). In contrast, protein efficiency rate and specific growth rate were higher in rats fed on biodetoxified cottonseed cake than for those in the control group. The hepatosomatic index showed a loss in hepatic mass with the ingredient at 25% in animal feed diets for both macrofungi (Table 5).
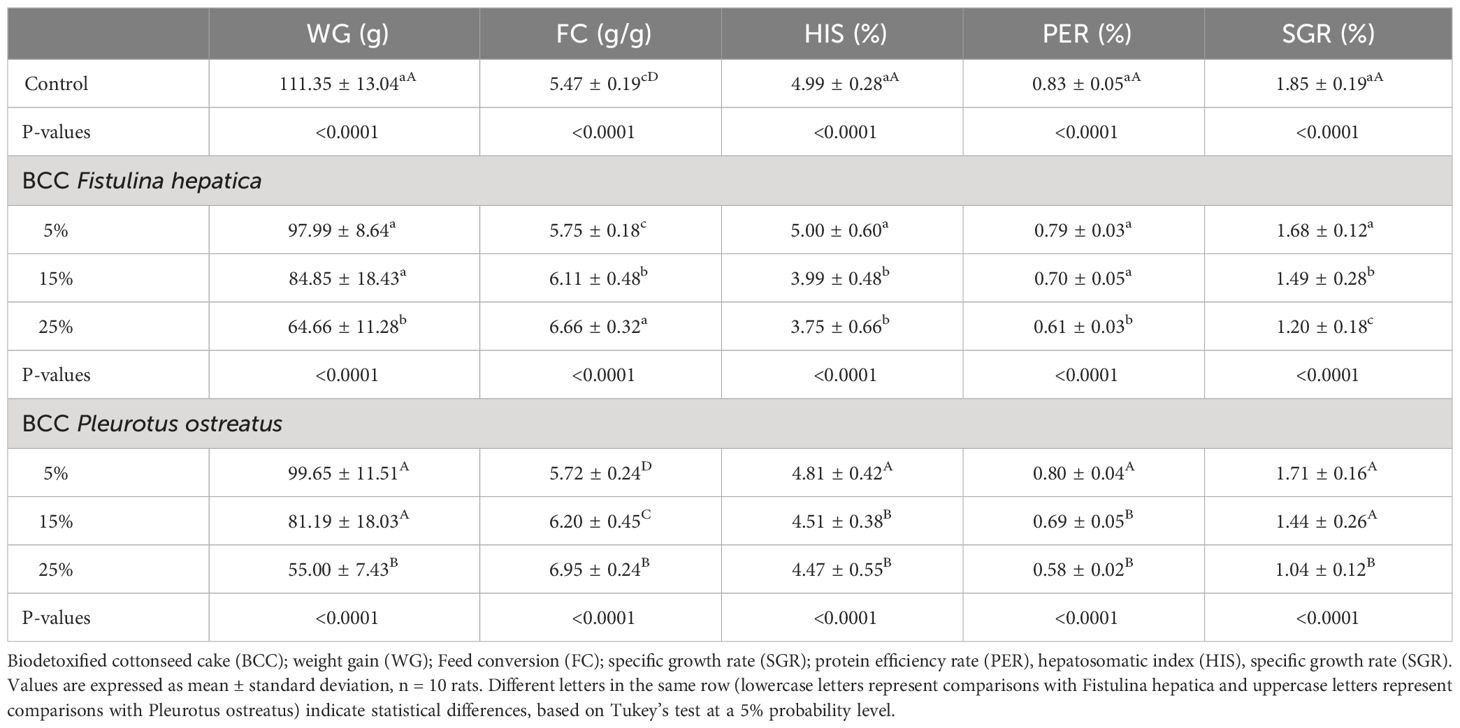
Table 5 Growth parameters of rats fed with different levels of biodetoxified cottonseed cake with macrofungi.
No significant alterations were observed in the hematological parameters analyzed in rats fed on biodetoxified cottonseed cake (data not shown). Diets containing F. hepatica-derived biodetoxified cottonseed cake showed an increase in ALT at all concentrations, in comparison with controls. The activity of AST with feed containing 25% F. hepatica-derived biodetoxified cottonseed cake showed a statistical difference to the lower level treatments (15%), reaching 248.99 U.L-1. The results of the diets with P. ostreatus-derived biodetoxified cottonseed cake showed decreased levels of both ALT and AST in comparison with control diets. No difference was observed in albumin values for either of the biodetoxified cottonseed cake feeds. A decrease in circulating cholesterol levels was observed in rats fed on diet feeds containing above 15% of F. hepatica-derived biodetoxified cottonseed cake and 25% of P. ostreatus-derived biodetoxified cottonseed cake (Table 6).
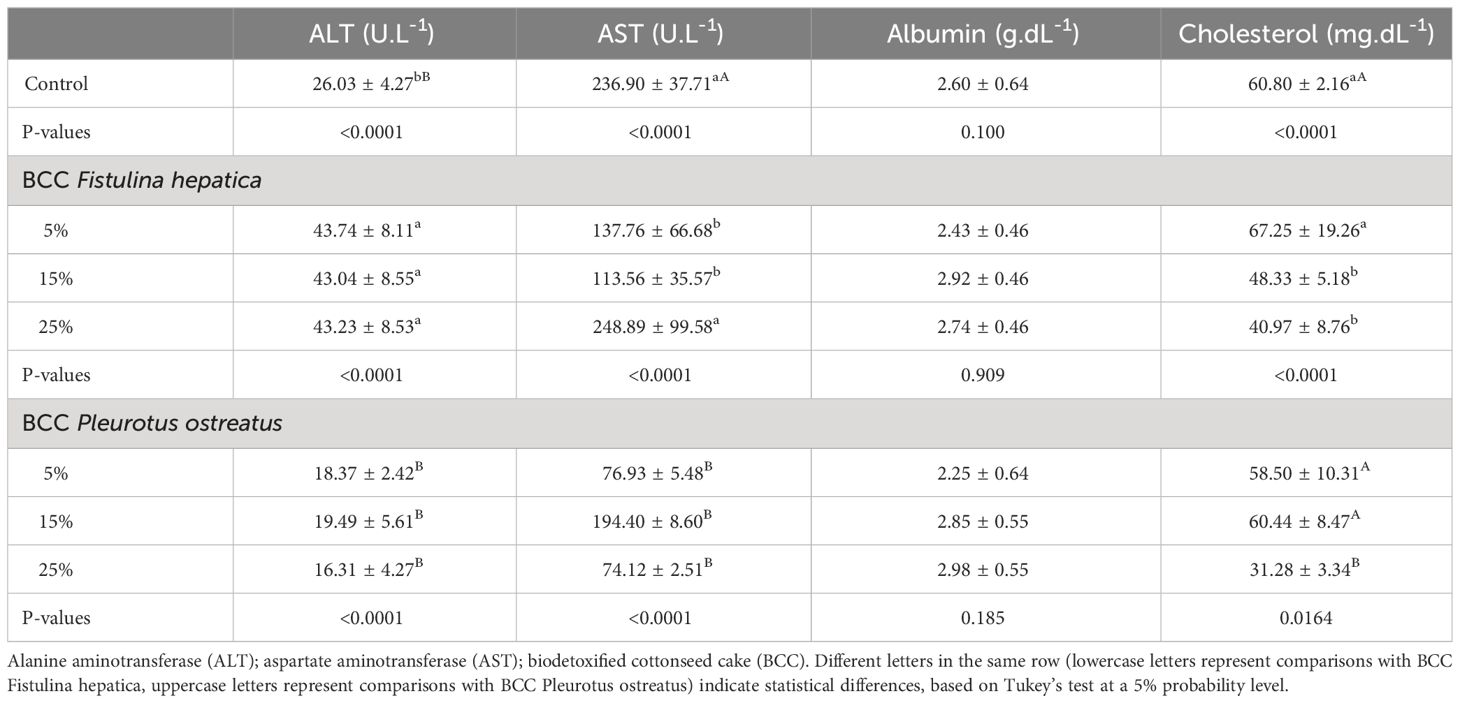
Table 6 Biochemical parameters of the serum of rats fed with different levels of biodetoxified cottonseed cake by macrofungi.
The hepatic parenchyma in the control group and BCC groups exhibited normal lobular architecture, with hepatocytes arranged in cordonal arrangement radiating around the central vein and separated by blood sinusoids (Figures 5A, B). The sinusoidal endothelium is formed by endothelial lining and contains phagocytic Kupffer cells. Cells displayed a polyhedral shape with round nuclei (Figures 5C, D). Alterations, however, were observed in animals fed on F. hepatica-derived biodetoxified cottonseed cake feed diets, with changes in the cordonal arrangement of hepatic cells, leukocyte infiltration (Figure 5B), blood venous congestion, and cytoplasmic and interlobular vacuolation.
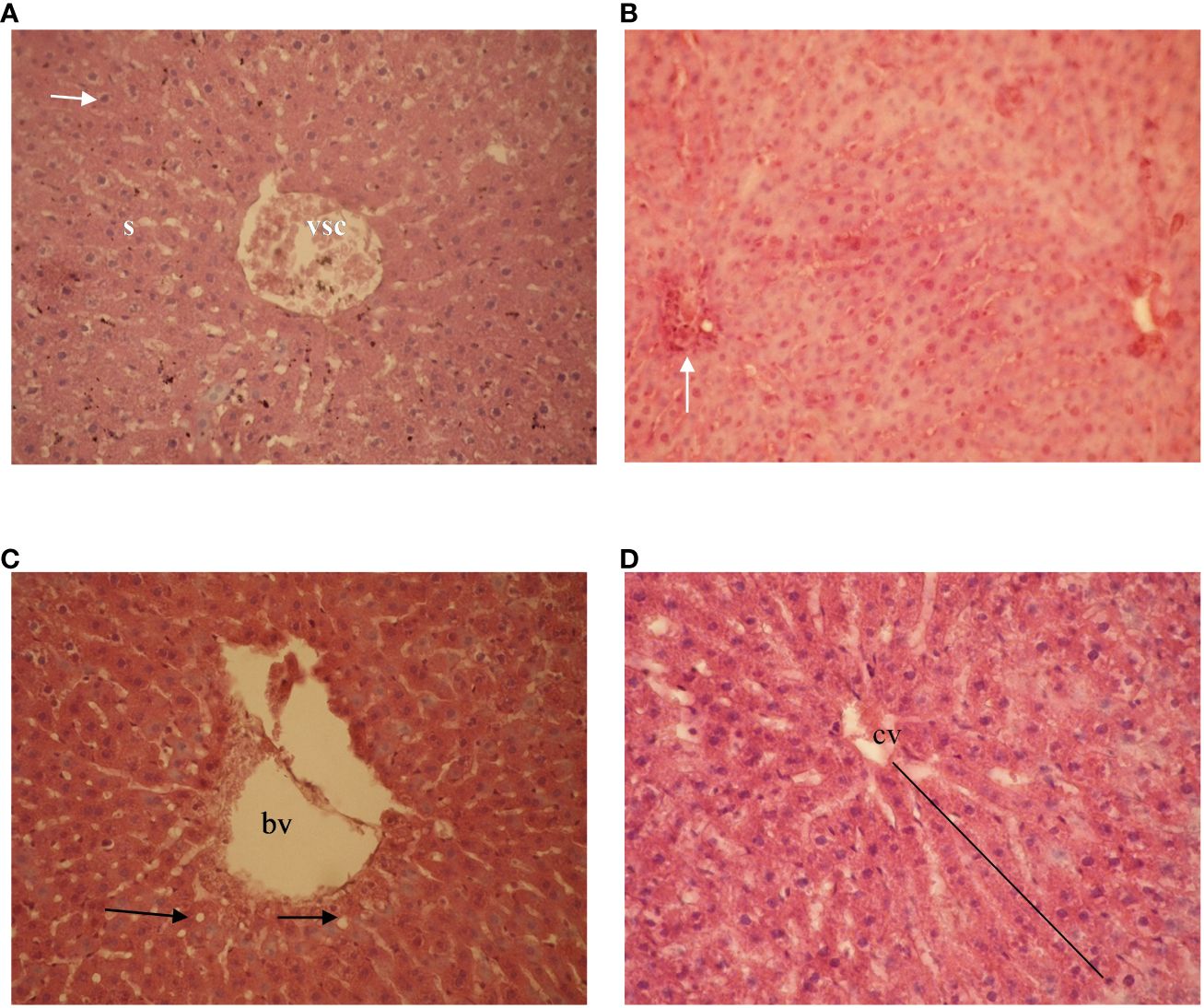
Figure 5 Photomicrography of Wistar rat livers after feeding on diets containing different levels of biodetoxified cottonseed cake. (A) diet containing 5% Fistulina hepatica-derived biodetoxified cottonseed cake showing classic hepatic architecture with congested blood vessel (VSC), hexagonal hepatocyte with round central nucleus (arrow), and sinusoid (S); (B) leukocyte infiltration (white arrow); (C) diet containing 10% Pleurotus ostreatus-derived biodetoxified cottonseed cake - showing blood vessel (bv) and normal hepatocytes (black arrows); and (D) radiation around the central vein (cv). HE staining, 40X magnification.
4 Discussion
The employment of fungi in the bioconversion of lignocellulosic biomass for animal feed has been widely reported, given their ability to not only improve the nutritive value of biomass (Ahmed, 2018; Nayan et al., 2020; Saldarriaga-Hernández et al., 2020), but also produce metabolites that confer different bioactivities, such as antioxidants, antibiotics and anti-inflammatory compounds (Dore et al., 2014; Elsayed et al., 2014). The bioconversion of low-quality biomass into food and feed rich in protein has also been shown to be efficient for the cultivation of certain macrofungi (Mane et al., 2007).
Plants that contain toxic agents are a potential problem for downstream industries that require the use of certain co-products (Gomes et al., 2018). However, a number of studies have evaluated the efficiency of different approaches for detoxification (Fonseca et al., 2013), either using techniques based on physical or chemical exposure to toxic compounds or through solid-state cultivation biotreatment techniques (Belewu et al., 2010; Joshi et al., 2011; Xiao et al., 2011). The inclusion of microorganisms that are capable of degrading toxic substances is certainly a promising strategy, as this method can potentially both eliminate toxic molecules present in biomass and also increase nutritional parameters such as proteins and fiber (Xiao et al., 2011; Gomes et al., 2018).
Globally, the cotton industry supplies both textile and biodiesel industries, with large amounts of residual biomass generated (Desrochers and Szurmak, 2017). Cottonseed cake is the main residue obtained after cottonseed oil extraction, offering high nutritional value and potential as a substitute for expensive feeds such as soybean meal (Kandylis et al., 1992; Assis et al., 2019). For supplication in non-ruminant feeds, however, pre-treatment is necessary to eliminate antinutritional factors, principally gossypol. Several studies have shown that biological treatment with certain fungi is one of the most efficient approaches for pre-treatment (Mageshwaran et al., 2018; Grewal et al., 2020).
Cumulative effects of free gossypol have been reported in the male reproductive system, affecting testicular tissue and sperm production (Santana et al., 2015). Other organs, such as the lung, heart, liver, pancreas, and kidney can also be deleteriously affected. In the liver, free gossypol may cause ascites and hepatocyte degeneration, increased plasma concentrations of the liver enzymes gamma glutamyltransferase and alkaline ribonuclease, vacuolation of mitochondria, dilation of endoplasmic reticulum and increases in the perinuclear space (Ali and El-Sewedy, 1984; Gadelha et al., 2014).
In this study, the toxic effects of gossypol in rats were clearly demonstrated in toxicity assays, with poor development rates observed. Reduction in weight as a process of intoxication by gossypol was likely a consequence of liver damage, as reported previously (De Carvalho et al., 2013).
The histopathology associated with hepatocyte degeneration observed in the livers of rats fed on cottonseed cake was evidenced by an increase in aminotransferase enzyme (ALT) and a decrease in liver function. Liver damage promotes negative effects on metabolism and mainly reduces glucose uptake by cells due to the reactivity of gossypol (Li et al., 2016). This alters the functioning of mitochondria, as they start to waste metabolic energy (Wallace and Starkov, 2000). This likely explains the deleterious effect observed in the form of weight gain reduction in the intoxicated rats. Gossypol acts as a competitive glucose transporter inhibitor (GLUT-1), and thus, the low metabolic energy is justified by the reduction in weight gain in rats (Pérez et al., 2009).
It is noteworthy the evidence of hepatitis and hepatocyte degeneration as a consequence of metabolic damage caused by gossypol-containing diets (De Carvalho et al., 2013). The histopathological changes observed here in the cottonseed cake toxicity assay indicate likely impaired liver structures and functioning (Ahmed, 2018).
Biodetoxification processes with certain macrofungi offer potential for decreasing or eliminating toxic compounds (Gomes et al., 2018). In this study, biodetoxification through the cultivation of P. ostreatus was efficient in reducing the toxic effects of free gossypol present in cottonseed cake substrate, demonstrated through maintenance of weight gain (with up to 15% biodetoxified cottonseed cake) as well as normal liver activities. Although the higher proportion of F. hepatica- and P. ostreatus-derived biodetoxified cottonseed cake (25%) was shown to impair all parameters related to safe growth, these diets were more efficient in reducing plasma cholesterol in rats. Biodetoxification of biomass through solid-state culture using certain macrofungi can contribute to promoting increases in bioactive compounds such as β-glucans, which can lead to increased antioxidant properties (Mageshwaran et al., 2018) and hypocholesterolemic action (Liu et al., 2018). Such effects are corroborated in the present study, where cholesterol reduction in rats was apparent after feeding with biodetoxified cottonseed cake produced with both P. ostreatus and F. hepatica.
Hepatoprotective action with albumin maintenance and benefits toward a decrease in ALT and AST enzyme activities have been demonstrated with the use of functional foods (Ahmed, 2018). The hepatoprotective action of bioactive substances has also been demonstrated in liver histopathological studies (Ahmed, 2018). Our study suggests that the use of cottonseed cake bio-detoxified through the cultivation of P. ostreatus resulted in the maintenance of liver tissues and cordonal arrangement, with cells displaying normal nuclei and cytoplasm without vacuoles or fat deposits. Such histopathological data supports the likely bioactive effect of the treatments with the macrofungi and the potential for downstream employment of the macrofungi treated cottonseed cake in feed applications.
5 Conclusions
Biodetoxification of cottonseed cake through solid-state cultivation with the macrofungi P. ostreatus and F. hepatica minimized the toxic effects of free gossypol in feed-formulation for rats. The inclusion of up to 15% P. ostreatus-derived biodetoxified cottonseed cake in feed-formulation diets did not result in toxic effects regarding the health and growth of Wistar rats. Further studies are warranted into the potential for reduction of fiber content of biodetoxified cottonseed cake using macrofungi, as well as mass spectrometry-based investigations into beneficial secondary metabolite accumulation in fermented products that may add further gains for monogastric animal feed.
Data availability statement
The raw data supporting the conclusions of this article will be made available by the authors, without undue reservation.
Ethics statement
The animal study was approved by Commission on Ethics in the Use of Animals - CEUA (CEUA/UFGD-49/2016). The study was conducted in accordance with the local legislation and institutional requirements.
Author contributions
SD: Data curation, Formal analysis, Writing – original draft. CN: Conceptualization, Methodology, Validation, Writing – original draft. JM: Data curation, Investigation, Writing – review & editing. AC: Data curation, Investigation, Writing – original draft. AA: Investigation, Writing – review & editing. CN: Investigation, Writing – review & editing. RM: Conceptualization, Data curation, Writing – review & editing. FD: Conceptualization, Methodology, Resources, Supervision, Writing – review & editing. SM: Conceptualization, Methodology, Project administration, Resources, Supervision, Validation, Writing – review & editing. CH: Conceptualization, Project administration, Validation, Writing – review & editing.
Funding
The author(s) declare that financial support was received for the research, authorship, and/or publication of this article. This work was financially supported by CNPq/Embrapa (Project Number 404786/2013-8). RM was supported by fellowships from CNPq (Grant Numbers 305418/2017-3 and 308165/2021-7).
Acknowledgments
A special thanks goes to all those who made this research possible and to the institutions Embrapa Agroenergy and UFGD.
Conflict of interest
The authors declare the research was conducted in the absence of any commercial or financial relationships that could be construed as a potential conflict of interest.
Publisher’s note
All claims expressed in this article are solely those of the authors and do not necessarily represent those of their affiliated organizations, or those of the publisher, the editors and the reviewers. Any product that may be evaluated in this article, or claim that may be made by its manufacturer, is not guaranteed or endorsed by the publisher.
References
Ahmed R. A. (2018). Hepatoprotective and antiapoptotic role of aged black garlic against hepatotoxicity induced by cyclophosphamide. J. Basic Appl. Zool. 79, 1–8. doi: 10.1186/s41936-018-0017-7
Alexander J., Benford D., Cockburn A., Cravedi J., Dogliotti E., Domenico A. D., et al. (2009). Gossypol as undesirable substance in animal feed - Scientific Opinion of the Panel on Contaminants in the Food Chain. EFSA J. 7, 1–55. doi: 10.2903/j.efsa.2009.908
Ali S. F., El-Sewedy S. M. (1984). Effect of gossypol on liver metabolic enzymes in male rats. Toxicol. Lett. 23, 299–306. doi: 10.1016/0378-4274(84)90025-0
AOAC. (2000). Official methods of analysis. Association of Official Analytical Chemists (Washington, DC: AOAC international).
Assis D. Y. C. de, Carvalho G. G. P. de, Santos E. M., Oliveira F. A. de, Araújo M. L. G. M., Pina D. d. S., et al. (2019). Cottonseed cake as a substitute of soybean meal for goat kids. Ital J. Anim. Sci. 18, 124–133. doi: 10.1080/1828051X.2018.1490633
Belewu M. A., Belewu K. Y., Ogunsola F. O. (2010). Nutritive value of dietary fungi treated Jatropha curcas kernel cake: Voluntary intake, growth and digestibility coefficient of goat. Agric. Biol. J. Of North America 1, 135–138.
Câmara A. C. L., Gadelha I. C. N., Borges P. A. C., de Paiva S. A., Melo M. M., Soto-Blanco B. (2015). Toxicity of gossypol from cottonseed cake to sheep ovarian follicles. PloS One 10, 1–11. doi: 10.1371/journal.pone.0143708
Conceição A. A., Mendes T. D., Mendonça S., Quirino B. F., Almeida E.G. de, Siqueira F. (2022). Nutraceutical enrichment of animal feed by filamentous fungi fermentation. Fermentation 8, 1–17. doi: 10.3390/fermentation8080402
Conceição A. A., Neto C. B. S., de Aquino Ribeiro J. A., de Siqueira F. G., Miller R. N. G., Mendonça S. (2018). Development of an RP-UHPLC-PDA method for quantification of free gossypol in cottonseed cake and fungal-treated cottonseed cake. PloS One 13, 1–17. doi: 10.1371/journal.pone.0196164
De Carvalho W. L., Maioli M. A., Mendes L. C. N., Rozza D. B., Mingatto F. E. (2013). Mecanismos da intoxicação do fígado de rato causada pelo gossipol. Pesquisa Veterinaria Bras. 33, 339–344. doi: 10.1590/S0100-736X2013000300011
Desrochers P., Szurmak J. (2017). Long distance trade, locational dynamics and by-product development: Insights from the history of the American cottonseed industry. Sustainability (Switzerland) 9, 579. doi: 10.3390/su9040579
Dore M. G., Alves G. das C., Santos M. L. G., de Souza L. R. A., Baseia I. G., Leite E. L.. (2014). Antioxidant and anti-inflammatory properties of an extract rich in polysaccharides of the mushroom Polyporus dermoporus. Antioxidants 3, 730–744. doi: 10.3390/antiox3040730
Elsayed E. A., El Enshasy H., Wadaan M. A. M., Aziz R. (2014). Mushrooms: A potential natural source of anti-inflammatory compounds for medical applications. Mediators Inflammation 2014, 1–16. doi: 10.1155/2014/805841
Fonseca N. B., da S., Gadelha I. C. N., Oloris S. C. S., Soto-Blanco B. (2013). Effectiveness of albumin-conjugated gossypol as an immunogen to prevent gossypol-associated acute hepatotoxicity in rats. Food Chem. Toxicol. 56, 149–153. doi: 10.1016/j.fct.2013.02.010
Gadelha I. C. N., Fonseca N. B. S., Oloris S. C. S., Melo M. M., Soto-Blanco B. (2014). Gossypol toxicity from cottonseed products. Sci. World J. 2014, 1–11. doi: 10.1155/2014/231635
Gomes T. G., Hadi S. I. I. A., Costa Alves G. S., Mendonça S., de Siqueira F. G., Miller R. N. G. (2018). Current strategies for the detoxification of Jatropha curcas seed cake: A review. J. Agric. Food Chem. 66, 2510–2522. doi: 10.1021/acs.jafc.7b05691
Grewal J., Tiwari R., Khare S. K. (2020). Secretome analysis and bioprospecting of lignocellulolytic fungal consortium for valorization of waste cottonseed cake by hydrolase production and simultaneous gossypol degradation. Waste Biomass Valorization 11, 2533–2548. doi: 10.1007/s12649-019-00620-1
Hattori R., Tanaka H. (1997). Method for growing fruit body of Fistulina hepatica. United States Patent No. 5,590,489. 1–6.
Joshi C., Mathur P., Khare S. K. (2011). Degradation of phorbol esters by Pseudomonas aeruginosa PseA during solid-state fermentation of deoiled Jatropha curcas seed cake. Bioresour. Technol. 102, 4815–4819. doi: 10.1016/j.biortech.2011.01.039
Kandylis K., Nikokyris P., Liamadis D., Deligiannis K. (1992). Evaluation of cotton seed cake as a feed ingredient for fattening sheep. J. Sci. Food Agric. 58, 291–299. doi: 10.1002/jsfa.2740580302
Li C., Ng A., Xie L., Mao H., Qiu C., Srinivasan R., et al. (2016). Engineering low phorbol ester Jatropha curcas seed by intercepting casbene biosynthesis. Plant Cell Rep. 35, 103–114. doi: 10.1007/s00299-015-1871-0
Liu B., Yang T., Luo Y., Zeng L., Shi L., Wei C., et al. (2018). Oat β-glucan inhibits adipogenesis and hepatic steatosis in high fat diet-induced hyperlipidemic mice via AMPK signaling. J. Funct. Foods 41, 72–82. doi: 10.1016/j.jff.2017.12.045
Mageshwaran V., Sharma V., Chinnkar M., Parvez N., Krishnan V. (2018). Biodegradation of gossypol by mixed fungal cultures in minimal medium. Appl. Biochem. Microbiol. 54, 301–308. doi: 10.1134/S0003683818030092
Mane V. P., Patil S. S., Syed A. A., Baig M. M. V. (2007). Bioconversion of low quality lignocellulosic agricultural waste into edible protein by Pleurotus sajor-caju (Fr.) Singer. J. Zhejiang Univ Sci. B 8, 745–751. doi: 10.1631/jzus.2007.B0745
Martínez-Herrera J., Siddhuraju P., Francis G., Dávila-Ortíz G., Becker K. (2006). Chemical composition, toxic/antimetabolic constituents, and effects of different treatments on their levels, in four provenances of Jatropha curcas L. from Mexico. Food Chem. 96, 80–89. doi: 10.1016/j.foodchem.2005.01.059
National Academic P. (2010). Guide for the care and use of laboratory animals. Washington, DC: The National Academies Press.
Nayan N., Sonnenberg A. S. M., Hendriks W. H., Cone J. W. (2020). Prospects and feasibility of fungal pretreatment of agricultural biomass for ruminant feeding. Anim. Feed Sci. Technol. 268, 114577. doi: 10.1016/j.anifeedsci.2020.114577
Neto C. B. S., Conceição A. A., Gomes T. G., de Aquino Ribeiro J. A., Campanha R. B., Barroso P. A. V., et al. (2021). A comparison of physical, chemical, biological and combined treatments for detoxification of free gossypol in crushed whole cottonseed. Waste Biomass Valorization 12, 3965–3975. doi: 10.1007/s12649-020-01290-0
Paiano D., Moreira I., Carvalho P. L., de O., Furlan A. C., Silva M. A. A., et al. (2014). Farelo de algodão na alimentação de suínos (30 - 90 kg). Rev. Bras. Saude e Producao Anim. 15, 790–800. doi: 10.1590/S1519-99402014000300016
Pérez A., Ojeda P., Valenzuela X., Ortega M., Sánchez C., Ojeda L., et al. (2009). Endofacial competitive inhibition of the glucose transporter 1 activity by gossypol. Am. J. Physiol. Cell Physiol. 297, 86–93. doi: 10.1152/ajpcell.00501.2008
Saldarriaga-Hernández S., Velasco-Ayala C., Leal-Isla Flores P., de Jesús Rostro-Alanis M., Parra-Saldivar R., Iqbal H. M. N., et al. (2020). Biotransformation of lignocellulosic biomass into industrially relevant products with the aid of fungi-derived lignocellulolytic enzymes. Int. J. Biol. Macromol. 161, 1099–1116. doi: 10.1016/j.ijbiomac.2020.06.047
Santana A. T., Guelfi M., Medeiros H. C. D., Tavares M. A., Bizerra P. F. V., Mingatto F. E. (2015). Mechanisms involved in reproductive damage caused by gossypol in rats and protective effects of vitamin E. Biol. Res. 48, 1–8. doi: 10.1186/s40659-015-0026-7
Wallace K. B., Starkov A. A. (2000). Mitochondrial targets of drug toxicity. Annu. Rev. Pharmacol. Toxicol. 40, 353–388. doi: 10.1146/annurev.pharmtox.40.1.353
Keywords: cottonseed cake, Free-Gossypol, biodetoxification, edible mushroom, solid fermentation
Citation: Deboleto SGdC, do Nascimento CA, Melo JCS, Conceição AA, Araujo APF, Neto CBS, Miller RNG, de Siqueira FG, Mendonça S and Honorato CA (2024) Toxicological, biochemical, and histopathological evaluation of rats fed with macrofungal-treated cottonseed cake. Front. Anim. Sci. 5:1343680. doi: 10.3389/fanim.2024.1343680
Received: 24 November 2023; Accepted: 28 March 2024;
Published: 18 April 2024.
Edited by:
Demin Cai, Yangzhou University, ChinaReviewed by:
Maghsoud Besharati, University of Tabriz, IranGabriela Miotto Galli, Federal University of Rio Grande, Brazil
Copyright © 2024 Deboleto, do Nascimento, Melo, Conceição, Araujo, Neto, Miller, de Siqueira, Mendonça and Honorato. This is an open-access article distributed under the terms of the Creative Commons Attribution License (CC BY). The use, distribution or reproduction in other forums is permitted, provided the original author(s) and the copyright owner(s) are credited and that the original publication in this journal is cited, in accordance with accepted academic practice. No use, distribution or reproduction is permitted which does not comply with these terms.
*Correspondence: Felix Gonçalves de Siqueira, felix.siqueira@embrapa.br