Replacement of fish oil with a high-DHA algal oil in a fishmeal-free diet fed to Florida pompano (Trachinotus carolinus)
- 1Harbor Branch Oceanographic Institute, Florida Atlantic University, Fort Pierce, FL, United States
- 2Aquatic Feed Technologies, Islamorada, FL, United States
- 3Anthropocene Institute, Palo Alto, CA, United States
A 12-week growth trial was conducted to evaluate the complete co-replacement of fish meal (FM) and fish oil (FO) in juvenile Florida pompano (Trachinotus carolinus) diets. Five open-formula experimental diets were formulated as iso-nitrogenous (approximately 46% crude protein) and iso-lipidic (approximately 15% crude lipid). It is assumed that Florida pompano lack the mechanisms for synthesizing sufficient LC-PUFA. In the FM/FO-free diets, to meet the necessary dietary intake of LC-PUFA, a commercially available Schizochytrium algal oil was used. Four diets were prepared from a basal mix, with spirulina and poultry by-product meal as the principal protein sources. They differed only in their oil source, containing fish oil (FO), algal oil (AO), soy oil (SO), or a 50/50 blend of algal oil and soy oil (A/S). The fifth experimental diet (control) was a standard open-formula FM diet. In addition to the experimental diets, two commercial closed-formula diets served as references. The seven diets, each with four replicates, were stocked with 20 Florida pompano (approximately 4 g) in each. The experimental open-formula diets were tested against the control using Dunnett’s t-test, and different oil sources were tested against each other using orthogonal contrasts. The four experimental diets were each tested against the two reference diets with equivalency tests. Feed intake and survival were not different between the experimental diets and control. However, all production metrics were lower in the SO diet than the control. No other differences were observed between the FO, AO, and A/S diets and the control, or between the three diets. The feed conversion ratio (FCR) was higher in fish fed the SO diet than in fish fed the control diet, and no other differences were found. The protein efficiency ratio (PER) was higher in fish fed the control diet than in fish fed the experimental diets. Protein productive values (PPV) were similar to PER, except that the PPV in fish fed the AO diet was not different from the control. The contrasts revealed that the FO, AO, and A/S diets resulted in a lower FCR than the SO diet. The PER was higher in fish fed the AO diet, and lower in fish fed the SO diet. The PPV was higher in fish fed the AO diet than in fish fed the A/S and SO diets, but similar to that of fish fed the FO diet. There were striking differences in performance between the two commercial reference feeds, underscoring the importance of using well-defined open-formula diets as a reference or control. To our knowledge this is the first study to demonstrate that co-replacement of FM and FO can be achieved in Florida pompano by supplying an FM- and FO-free diet. The development of successful open-formula diets without FO will help spur innovation and sustainability in aquaculture production.
1 Introduction
Global human dependence on aquaculture products continues to increase (Froehlich et al., 2018; FAO, 2020). Concomitantly, there has been a marked increase in aquafeed utilization to enhance both the quantity and the quality of farm-raised products. Aquaculture feeds for marine fish typically utilize fish meal (FM) and fish oil (FO) as the primary nutritive ingredients. Despite improvements in feed conversion ratios and reductions in dietary inclusion rates, due to the increasing growth of the aquaculture sector, demand has increased (Naylor et al., 2009). However, FM and FO are regarded as expensive and unsustainable at their current and projected rate of usage (Tacon and Metian, 2008). In addition, the nutritional benefits of fish oil may be diminished by contaminants found in wild-caught fish (Hong et al., 2015). Therefore, there is substantial interest in developing and discovering alternatives to their use (Gatlin et al., 2007; Rust et al., 2011; Froehlich et al., 2018).
Fish feed manufacturers are turning to a diverse array of alternative feedstuffs of plant, animal, and microbial origin, each with inherent characteristics and limitations (Bureau, 2015). These alternatives to FM and FO are complex and require further investigation to determine their nutritional value, productivity, and profitability (Gatlin et al., 2007; Glencross et al., 2007).
The transition away from FM and FO requires accurate information on the nutritive value of prospective alternatives (Gatlin et al., 2007; NRC, 2011) and nutritional requirements of the fed species (McLean et al., 2022). Availability, ease of use, relative animal safety, and nutritional value relative to cost are the drivers that affect the extent to which new and underutilized ingredients will be used (NRC, 2011; Glencross et al., 2020). Accurate assessment of the nutritional value of these ingredients is essential for the formulation of cost-effective feeds with reduced levels of FM and FO (Bureau, 2015).
Feeds typically represent the highest variable cost in aquaculture production (Naylor et al., 2009). Economics and sustainability therefore create a fiscal imperative to understand the interactions of nutrients and their utilization by the fish. Alternatives to FM and FO that provide an economic advantage without affecting health, wellness, and performance are generally accepted as viable alternatives (Rust et al., 2011). Improved animal feeding, animal health and welfare, product quality, and food safety are directly correlated with efficiency, productivity, and profitability (NRC, 1995; Pickova and Mørkøre, 2007).
Completely fish-free diets have proven to be a challenge for many carnivorous marine finfish. While a reduction of dietary FM has been demonstrated in a number of species, complete FO replacement continues to present a greater challenge (NRC, 2011). It is well accepted that most carnivorous marine fish require long-chain polyunsaturated fatty acids (LC-PUFAs), such as docosahexaenoic acid (22:6 n-3, DHA) and eicosapentaenoic acid (20:5 n-3, EPA), in their diets (Tocher, 2003). The requirements are generally met through the utilization of FO and the residual oil associated with FM products. Plant seed oils or terrestrial vegetable oils (VOs) such as soybean, canola/rapeseed, palm, linseed (flax), corn, and olive oil, and poultry oil and beef tallow, are generally deficient in the LC-PUFAs required by marine carnivores (Mourente et al., 2005; Trushenski and Lochman, 2009; Bowzer et al., 2016). These LC-PUFAs are desirable in the final end product (Miller et al., 2011).
An alternative for meeting LC-PUFA requirements is microalgae and other single-cell organisms (SCOs). There is increasing interest in utilizing microalgal oils and/or biomass as partial replacements in aquafeeds. Both heterotrophic and autotrophic organisms have received attention, although, due to their nutritional quality heterotrophic microalgae, are thought to be more promising than other autotrophic organisms (Shah et al., 2018). Due to its compositional profile, the heterotrophic microalgae Schizochytrium sp. is of particular interest (Lewis et al., 1999; Miller et al., 2007).
It has been demonstrated that lipid-rich microalgae, including Schizochytrium, can supplement or replace FO in aquafeeds (Qiao et al., 2014; Kousoulaki et al., 2015; Bélanger-Lamonde et al., 2018; Seong et al., 2019). Schizochytrium oil/biomass is a rich source of LC-PUFA, with particularly high levels of DHA (Lippmeier et al., 2009; Ren et al., 2010; Shah et al., 2018). Moreover, the utilization of SCO as an FO substitute reduces the risk of exposure to persistent organic pollutants sometimes associated with FO (Drew et al., 2007; Bélanger-Lamonde et al., 2018). In addition, algal oil from Schizochytrium sp. is one of only seven algae-based products that carries the generally regarded as safe (GRAS) status as an animal feed ingredient (Shah et al., 2018).
It is assumed that Florida pompano, like other carnivorous marine finfish, lack the mechanisms for synthesizing sufficient LC-PUFA to meet their metabolic needs (Sargent et al., 2002; Rombenso et al., 2016). There is evidence to suggest that they have a dietary requirement for LC-PUFA (Jackson et al., 2020), although this has not yet been verified. Nevertheless, there is evidence that LC-PUFA residuals from FM-based products can contribute substantially to these dietary requirements (Miller et al., 2007). In this context, it is important to understand the benefits of microalgal contributions to meeting these requirements in an FM-free diet, and to understand whether or not feeding Florida pompano a diet free of both FM and FO is technically feasible without adversely affecting performance.
The objectives of this study were to:
evaluate FM-free basal diets, varying only in oil sources, relative to a FM/FO open-formula control diet, with the conditions
evaluate a high-DHA microalgal oil relative to fish oil, with the conditions
evaluate the experimental basal diets relative to two commercial closed-formula reference feeds, with the conditions
where θL and θU represent the lower and upper bounds of the equivalence interval of the two CF diets, respectively (Wachs, 2015). Under this scenario, the question is not if the diets are different, but if they are similar enough in their response (Ialongo, 2017) to evaluate their suitability relative to commercial diets that contain both FM and FO. This third objective should be viewed in the framework of empirical discovery for directing future studies (Kerr, 1998).
2 Materials and methods
2.1 Fish and experimental system
Florida pompano juveniles were commercially spawned and weaned on to a commercially prepared larval diet (Proaquatix, Vero Beach, FL). At approximately 4 g, juveniles were then transported to Florida Atlantic University’s Harbor Branch Oceanographic Institute (HBOI), Fort Pierce, FL. Fish handling and experimental procedures followed all guidelines regarding animal care in the Public Health Service (PHS) policy on the Humane Care and Use of Animals and was approved by Florida Atlantic University’s Institutional Animal Care and Use Committee (protocol A-19-07).
An 8,750-L experimental recirculating system (RAS) with associated filtration, temperature control, and ultraviolet (UV) sterilization was used. Filtration included a 6-ft3 bubble-washed bead filter, for both solids and biofiltration, and a 60-gpm foam fractionator for fine particulates. Culture water on the continuous loop was treated with 30,000 µw-cm–2·−1·sec–1 of 256-nm UV exposure. A 5-ton heat pump with a titanium heat exchanger provided temperature control within 1°C.
2.2 Water quality
Dissolved oxygen (DO), salinity, temperature, and pH were measured (YSI, Yellow Springs, OH). Dissolved oxygen and temperature were measured twice per day (YSI Pro ODO). Salinity (YSI Pro30) and pH (YSI Pro 10) were measured once per day. Ammonia, nitrite, and nitrate were measured using a HACH DR2800 spectrophotometer (HACH Company, Loveland, CO). Total ammonia nitrogen (TAN; HACH method 8155) and Nitrite-NO2 (HACH method 8507) were measured three times per week. Nitrate-NO3 (HACH method 8171) was measured weekly. Alkalinity was also measured three times per week using a HACH digital titrator with bromcresol green-methyl red indicator (HACH method 8203).
2.3 Experimental diets
Five open-formula experimental diets were formulated as iso-nitrogenous (46% CP) and iso-lipidic (15% CL), meeting the optimal levels for Florida pompano (Riche, 2009). The control diet was a Feed Innovation Network (https://f3fin.org/) standard open-formula FM diet. It contained FM and FO and was formulated to contain ingredients common in commercial feeds (Table 1).
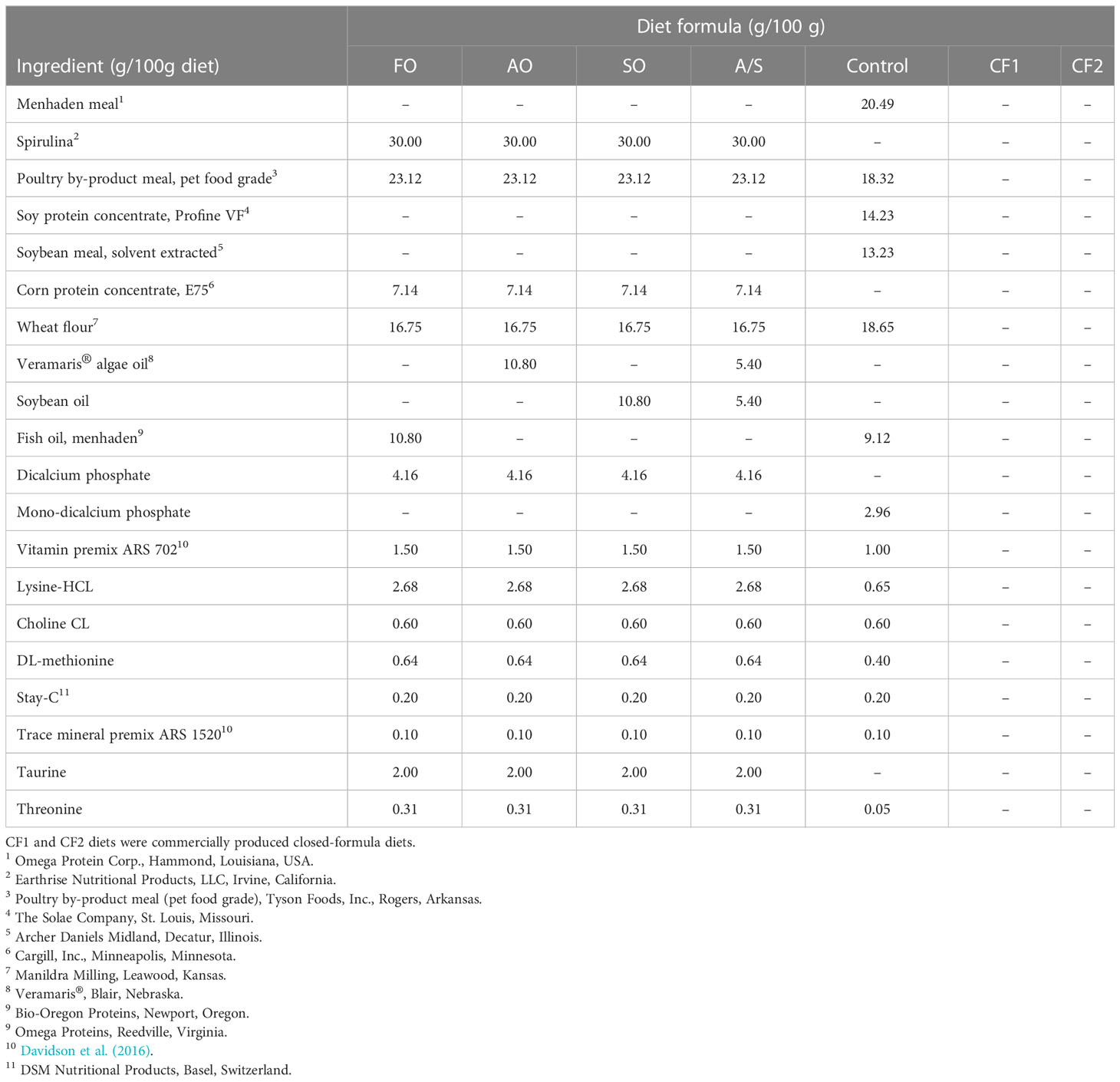
Table 1 Formulations of experimental diets fed to Florida pompano (Trachinotus carolinus) during a 12-week comparative growth trial.
Four diets (basal formulation) were formulated as FM free with spirulina, poultry by-product meal, and corn protein concentrate as the principal protein sources. To maximize the effect of AO substitution for FO, the four experimental diets varied only in lipid source and did not contain FM. The four basal formulations contained either fish oil (FO), algal oil (AO), soy oil (SO), or an algal and soy oil blend (A/S). The FO diet did not contain FM but did contain FO, and this diet has been shown to be effective with California yellowtail (Seriola dorsalis) (Drawbridge et al., unpublished).
The algal oil was a commercially available liquid product extracted from the whole-cell, heterotrophic microalgae Schizochytrium sp. (Veramaris®, Blair, NE). The AO diet contained only algal oil and the SO diet contained only soy oil and served as a negative control. The A/S diet was a 50/50 mix of algal oil and soy oil evaluated to determine if the very high levels of DHA found in the AO are necessary to maintain health and performance (Table 1) and ultimately reduce feed costs. Oil supplements were incorporated at 10.8% of the dry diet.
Experimental diets were manufactured by cooking extrusion at Prairie AquaTech (Brookings, SD) using standard commercial operating temperatures and pressure to produce a sinking feed. A portion of the liquid oil was top-coated on the feed after drying. The feeds were stored in plastic-lined paper bags, shipped to HBOI, and stored in a temperature- and humidity-controlled environment.
In addition, two commercially produced closed-formula diets labeled CF1 and CF2 were included in the trial as reference diets to examine the potential equivalency of the four FM-free diets to existing commercial products. These two reference diets were selected because they are frequently fed to Florida pompano in production systems (Riche, personal communication). The proximate, essential amino acid (EAA), and fatty acid (FA) analyses of the seven diets are given in Tables 2–4, respectively.
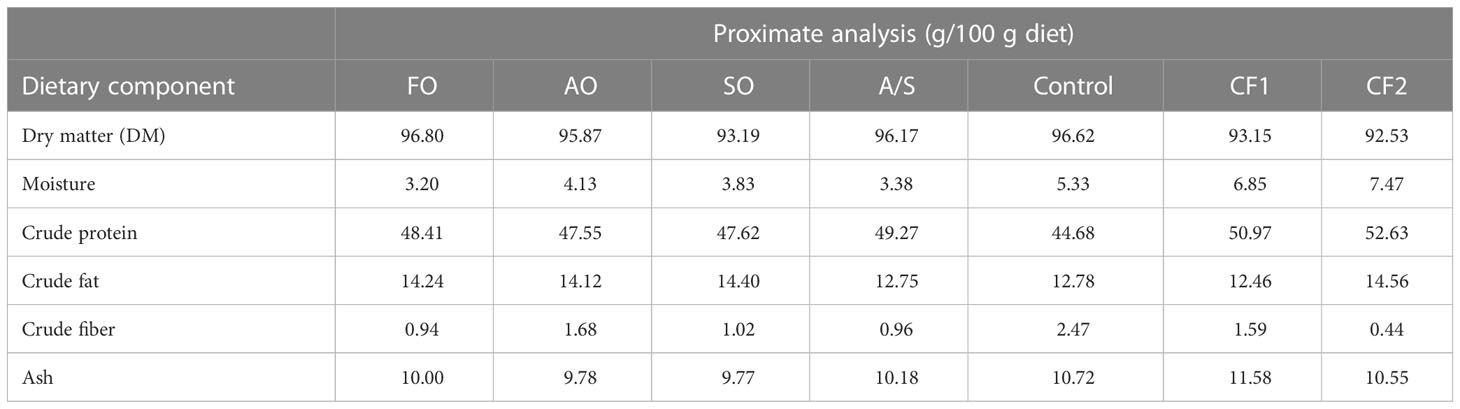
Table 2 Proximate analysis (dry matter basis) of experimental diets fed to Florida pompano (Trachinotus carolinus) during a 12-week comparative growth trial.
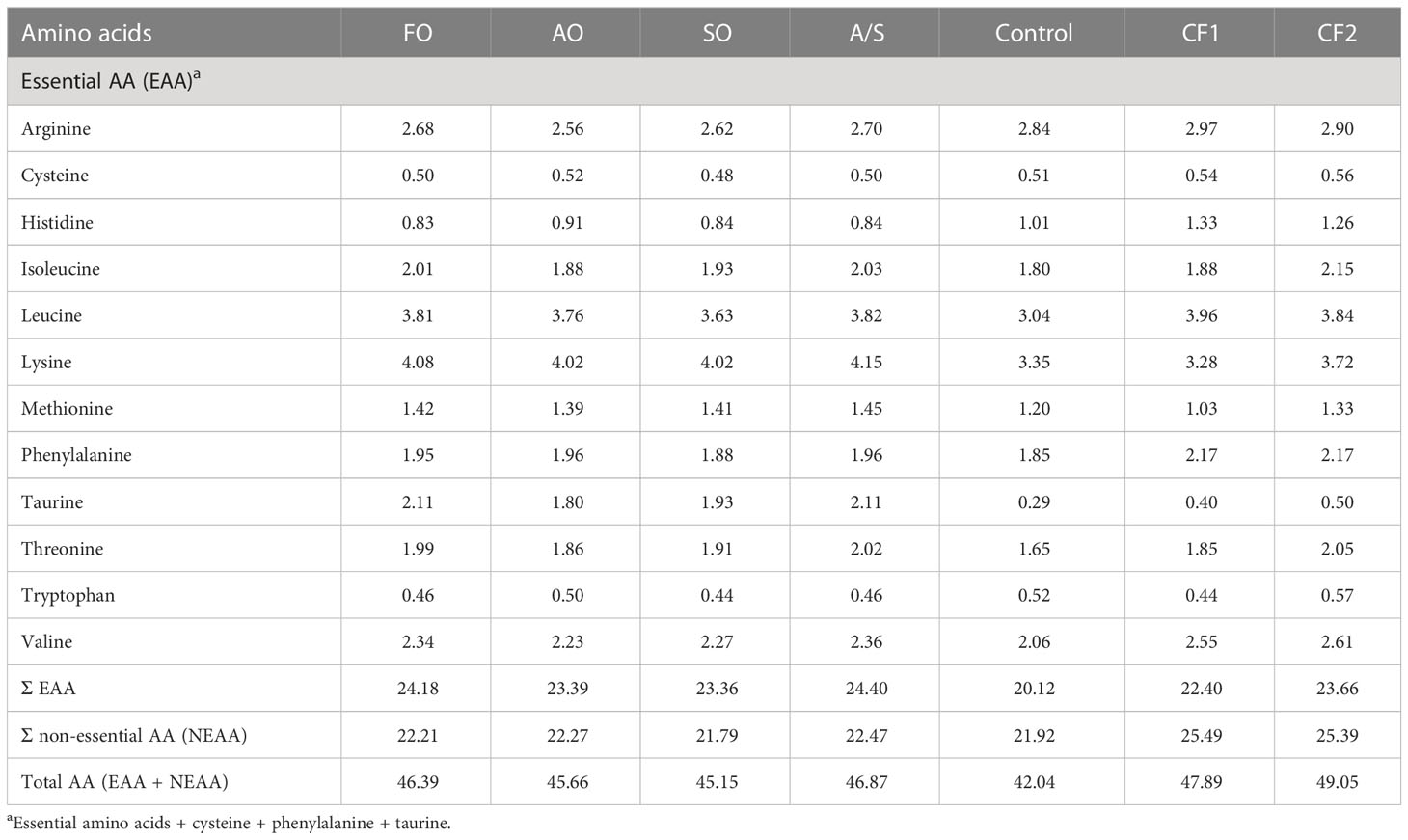
Table 3 Amino acid (AA) composition (% dry diet) of experimental diets fed to Florida pompano (Trachinotus carolinus) during a 12-week comparative growth trial.
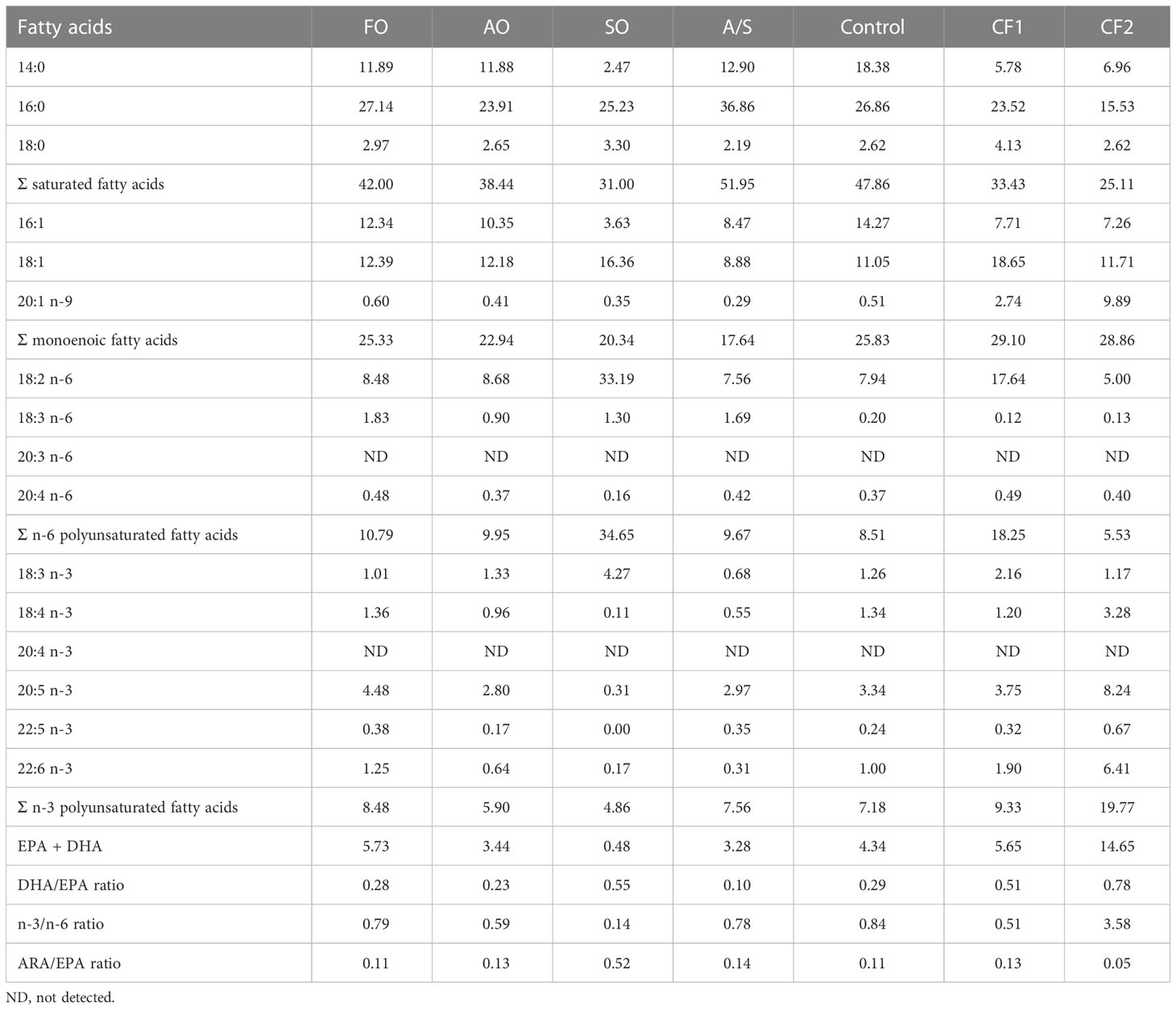
Table 4 Fatty-acid composition (g/100 g diet) of experimental diets fed to Florida pompano (Trachinotus carolinus) during a 12-week comparative growth trial.
2.4 Experimental design and sampling
A completely randomized design was employed with the seven diets (treatments) as the fixed factor effect. Subsequent to stocking, the seven diets were randomly assigned to tanks. All treatments were run with four replicates. For statistical purposes, each tank of fish represented an experimental unit (n = 4).
The fish were stocked into twenty-eight 80-L tanks in the RAS on the day of delivery to HBOI. Each tank was stocked with 20 Florida pompano with a mean weight of 4.1 ± 1.6 g, mean total length (TL) of 6.2 ± 1.1 cm, and mean condition factor (K) of 1.6 ± 0.1.
Fish in each tank were fed, by hand, their respective diet ad libitum to apparent satiation up to a maximum of 5% of their body weight per day (BW/D). Feed was offered four times per day between 8:00 a.m. and 4:00 p.m. Each week, fish in one randomly selected tank from each treatment were bulk weighed to adjust the maximum feed rates (5%). Feed intake (FI) was weighed and recorded daily. There were no uneaten pellets observed or recovered.
The photoperiod (L:D) was set to 12 h light:12 h dark using a system of lights with intensity ramping up in the morning and down in the evening over a 30-min period. The water temperature was maintained at 28 ± 1°C (27.2–28.5°C).
At stocking, a randomly selected sample of 15 juveniles from the total population used to stock the experiment was euthanized with MS-222 (Western Chemical, Ferndale, WA) at 300 mg/L. Individual euthanized fish were weighed to the nearest 0.1 g, had their TL (mm) measured, and were stored at –80°C for proximate and micronutrient analysis. The experiment lasted 85 days. At termination of the study all remaining fish were counted, weighed, and had their TL measured. A subsample of 8–10 arbitrarily selected animals from each replicate tank were euthanized, pooled by tank, and stored at –80°C for subsequent compositional analysis.
2.5 Whole-body composition and nutritional indices
The pooled fish samples were ground, freeze dried, and pulverized prior to shipping to the University of Missouri (UM). The diets, which had previously been stored at –20°C, were shipped as fed.
All analytical procedures were conducted by the UM Experiment Station Chemical Labs (Columbia, MO) and followed AOAC (2006) methods. Nitrogen (N) was determined using a LECO analyzer (AOAC Method 990.03) and crude protein (CP) was calculated as N × 6.25. Crude lipid (CL) in the fish tissue was determined by AOAC Method 920.39, and CL in the feeds by AOAC Method 954.02. Moisture was determined following drying at 95–100°C under vacuum (AOAC Method 934.01). Ash was determined gravimetrically following ignition at 600°C for 2 h (AOAC 942.05). Crude fiber was determined following the procedures of AOAC Method 978.10. Amino acids were determined following AOAC Method 982.30 E(a,b,c) and complete fatty acid profiles using AOAC Method 996.06 (AOAC, 2006).
Production metrics evaluated comprised the following:
● weight gain (WG) = Wt(final) – Wt(initial) × 100 (%),
● specific growth rate (SGR) = (ln Wt(final) – ln Wt(initial)/t) × 100%,
● biological feed conversion ratio (bFCR) = FI/WG + Wt(mortalities),
● economic feed conversion ratio (eFCR) = FI/WG,
● crude protein intake (CPI) = FI × CP(feed),
● protein efficiency ratio (PER) = WG/CPI,
● protein productive value (PPV) = [(CP(feed) – CP(intake))/CPI)] × 100%,
● protein productive value (PPV) = [(Wt(final) × CP(final)) – (Wt(initial) × CP(initial))]/CPI × 100%, and
● Fulton’s condition factor (K) = Wt (g)/TL3 (cm) × 100 (Ricker, 1975),
where
● Wt(final) = final body weight (g),
● Wt(initial) = initial body weight (g),
● Wt(mortalities) = weight of mortalities (g),
● t = experimental duration (days),
● FI = feed intake (g dry weight),
● CP(feed) = crude protein proportion of feed (g dry weight),
● CPI = crude protein intake (g dry weight),
● CP(initial) = initial crude protein in the fish (g dry weight), and
● CP(final) = final crude protein in the fish (g dry weight).
2.6 Fatty acid analysis in juveniles
Frozen juvenile samples were placed on ice and thawed to room temperature to reduce and avoid lipid degradation by hydrolysis or oxidation of carbon–carbon double bonds. Dichloromethane solvent, an organic lipid solvent, was immediately added (Couturier et al., 2020). Lipids were extracted using the methods described by Folch et al. (1957) and modified by Parrish (1999). The resulting extracts were methylated using methods described by Lepage and Roy (1984) to produce fatty acid methyl esters (FAMEs) for analysis with gas chromatography–mass spectrometry (GC–MS). Samples were then analyzed on a Clarus 680/600 T GC-MS (Perkin-Elmer, Waltham, Mass., USA) using a 30-m Thermo Fisher TR-5 general purpose column with a 250-µm diameter. Samples were individually injected into the column (using an 82-vial autosampler) at a volume of 1.0 µL, heated to 250°C, and held at this temperature for 10 min. Different FAs detected were compared with a 37-component FAME standard (Supelco 37 FAME Mix, Millipore Sigma, Burlington, Mass., USA) with known concentrations for quantification purposes.
2.7 Statistical analysis
All efficiency parameters and production metrics for the four non-fishmeal experimental diets (FO, AO, SO, and A/S) and the control were subjected to a one-way ANOVA using the general linear model procedure of SAS version 9.4 (SAS Institute, Cary, NC). Parametric assumptions were tested using the Shapiro–Wilks test for normality and Levene’s test for homogeneity of variance. A temporary loss of water flow during routine biofilter maintenance resulted in low DO, leading to mortality in one replicate tank each in the FO and control treatments. Therefore, the results for the FO and control diets were based on three replicates for the parameters survival, final weight, and condition factor. Data analysis on these parameters was corrected for unequal sample size. Means for the non-fishmeal diets were tested against the control diet using Dunnett’s t-test. Pairwise orthogonal contrasts were used to evaluate differences between experimental diets with different oil sources. Significance was reported at p ≤ 0.05 unless otherwise reported. Permutational analysis of variance (PERMANOVA with 9,999 permutations), including an a posteriori pairwise comparison, was performed on the fatty acid profiles in juveniles. Each PERMANOVA was tested with one factor: diet (FO, AO, SO, A/S, and control). Assumptions of multivariate homoscedasticity were verified with a PERMDISP test, and data were transformed (arcsine square root) when necessary. Analyses were run using a Bray–Curtis similarity matrix with PRIMER 7 (v. 7.1.12) and PERMANOVA+ (v.1.0.2).
To determine the relative efficacy of the non-FM (basal) diets relative to the two closed-formula commercial diets, the parameters weight gain and FCR were evaluated. Two one-sided t-tests (TOST) of equivalence were performed for each of the four non-fishmeal-based diets against CF1, and again against CF2 (Schuirmann, 1987; Lakens, 2017), where the closed-formula diets served as the reference. It was assumed that the maximum acceptable decrease in performance that could potentially be overcome by cost reduction in feed production was 10%. Therefore, the equivalence limits (θL, θU) for CF1 and CF2 were set to 0.10, defining the equivalence interval around the means. The confidence interval (CI) was set as α = 0.05. Data analysis was performed using the XLSTAT (version 2021.1) add-in for Microsoft Excel.
3 Results
Dissolved oxygen was at 100% saturation or slightly higher, and never fell below 80%. Salinity ranged from 33 to 36 ppt ( = 34.4) and pH from 7.24 to 8.25 ( = 7.75). Temperature ranged from 27.2 to 29.4°C ( = 28.5°C). All were within nominal limits considered acceptable for Florida pompano. Nitrite levels of 0.012–0.167 mg/L and TAN levels of 0–0.20 mg/L were within acceptable limits for Florida pompano (Weirich and Riche, 2006).
The macronutrients (proximate analysis) of the experimental diets and commercial diets are given in Table 2. The data suggest that the commercial diets (CF1 and CF2) were slightly higher in CP and the control diet was slightly lower in CP than the experimental diets. All diets were fairly similar in their essential amino acid (EAA) composition and balance, with the exception of methionine levels in CF1, which were approximately 20%–40% lower than in the other diets (Table 3). Conversely, the level of taurine was higher in the experimental diets than in the control and the commercial diets.
Fatty acid compositions of the experimental diets are given in Table 4. The SO diet had less 14:0 than the other experimental diets, resulting in lower total saturated fatty acids (SFAs). Although 16:1 was also lower in the SO diet, the overall levels of monounsaturated fatty acids (MUFAs) were similar across the experimental diets. Both linoleic acid (18:2 n-6, LA) and linolenic acid (18:3 n-3, LNA) levels were also higher in the SO diet than the other diets. This resulted in a higher total n-6 PUFA concentration in this diet relative to the other diets. Despite the higher concentration of LNA in the soy diet, the total n-3 PUFA concentration was slightly lower than in the other experimental diets. In conjunction with the higher LNA concentration in the SO diet, the concentrations of the essential fatty acids (EFAs) DHA and EPA were considerably lower than the concentration of arachidonic acid (ARA) was less than half of that in the rest of the diets.
Multivariate analysis, i.e., PERMANOVA, showed that fatty acid profiles in juvenile fish varied significantly according to diet (pseudo-F(4, 19) = 9.19, p = 0.001).
Overall, MUFAs and PUFAs made up the largest fraction of the total fatty acid content. Total MUFA ranged from 34% of total FA in the A/S treatment to 38% in the control treatment. PUFA relative percentages were the lowest in the AO treatment and the highest in the FO and A/S treatments (Table 5).
Fatty acid profiles were similar between the 100% FO and A/S diets, as shown in the n-MDS figure (Figure 1), where they had similar FA profiles. Fatty acid profiles from the control, AO, and SO diets were clustered separately in the n-MDS figure, indicating completely different profiles. Juveniles receiving the SO treatment were characterized by higher percentages of 16:0, 18:3 n-3, 18:2 n-6, and 18: 3 n-6, and the lowest percentages of essential fatty acids EPA, ARA, and DHA compared with other treatments. Conversely, the control treatment was characterized by higher percentages of EPA and ARA. The AO treatment samples were mainly rich in SFAs and MUFAs.
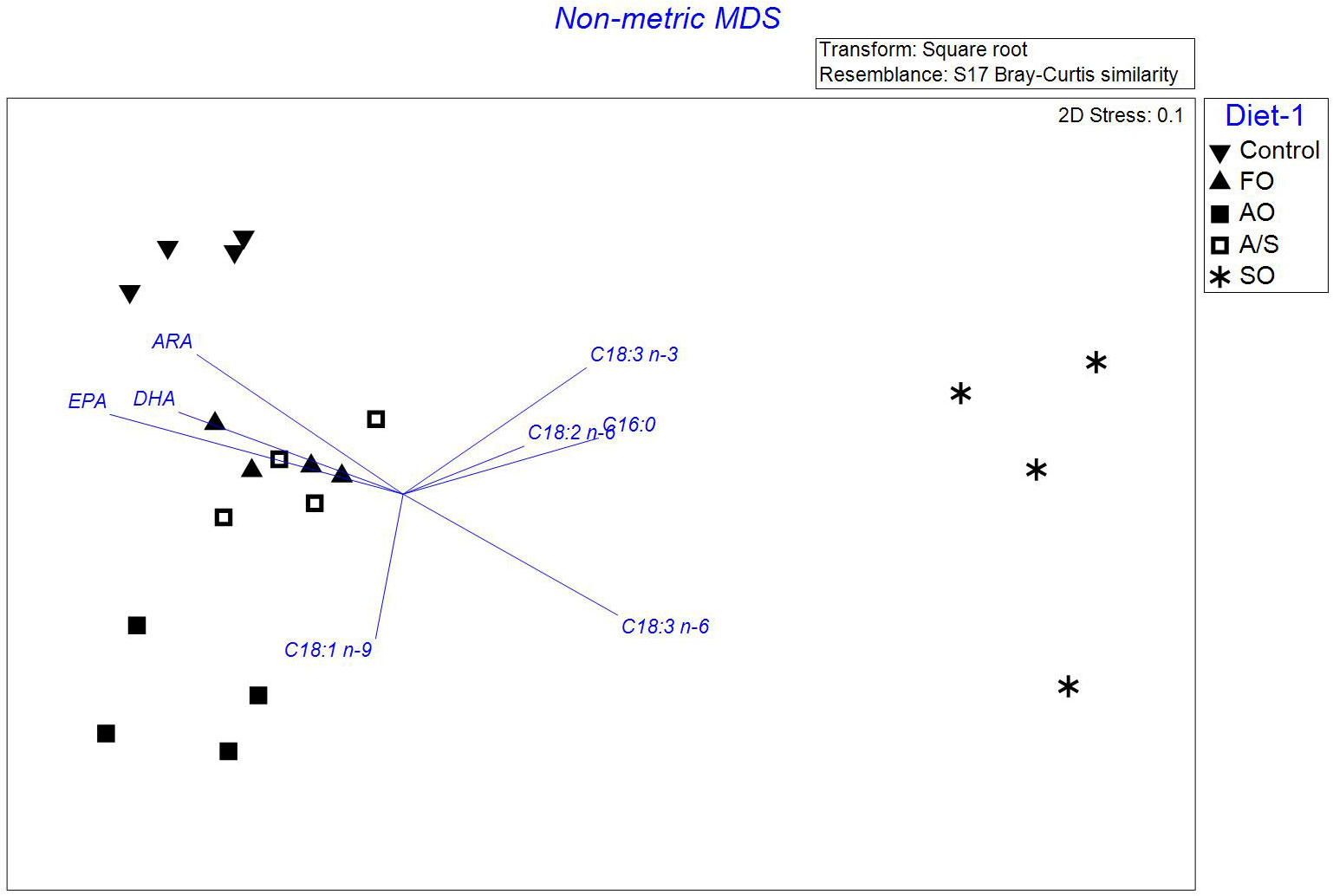
Figure 1 Non-metric multidimensional scaling (n-MDS) of the Bray–Curtis similarity matrix based on the relative abundance of fatty-acid profiles associated with Florida pompano (Trachinotus carolinus). Juveniles were fed a control diet (inversed triangles); a 100% fish oil-based diet (FO; triangles); an algal oil-based diet (AO; filled squares); a 50/50 blend of algal oil and soy oil-based diet (A/S; empty squares); and a soy oil-based diet (SO; stars). The n-MDS shows four main clusters: the first cluster regroups all juvenile samples fed the SO diet; the second and third clusters regroup juveniles fed the control and AO diets (inversed triangles and filled squares, respectively); the fourth cluster regroups juveniles fed the A/S and FO diets. The arrows’ length and direction represent the fatty acids responsible for most of the variation.
The mean production metrics (Table 6) indicated that there were no significant differences in FI or survival between fish receiving the experimental diets and the control diet. However, weight gain, SGR, final weight, and final biomass were significantly lower in the SO-fed fish than in the control-fed fish. Other than a slightly, but significantly, lower SGR in FO-fed fish than in control-fed fish, no other significant differences were detected between the FO-, AO-, and A/S-fed fish and the control-fed fish. The production performance metrics in fish fed the similarly formulated experimental diets varying only in their lipid composition (FO, AO, SO, and A/S) were analyzed in comparison to each other (Table 6).
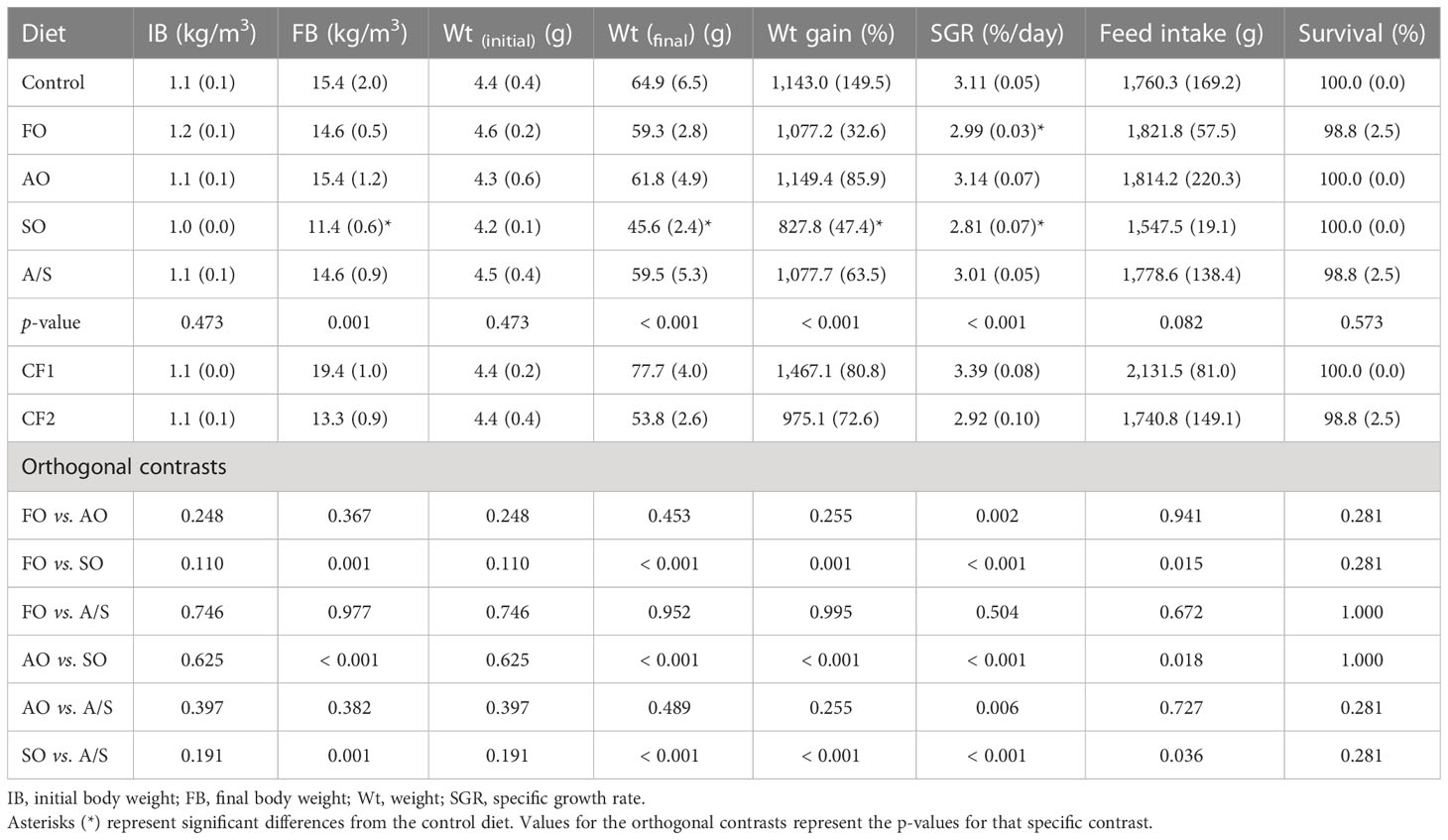
Table 6 Mean (SD) production metrics for Florida pompano (Trachinotus carolinus) fed experimental diets during a 12-week comparative growth trial.
Final weight, weight gain, final biomass, and feed intake were significantly higher in fish fed diets containing FO, AO, or A/S than in fish fed the diet containing SO. No other differences were detected between the other three diets for these parameters. Conversely, SGR was significantly higher in fish fed AO than in fish fed FO and A/S, and no differences were detected between fish fed FO and A/S. As with the other performance parameters, SGR in fish fed SO was lower than in fish fed the other experimental diets.
The mean efficiency parameters are shown in Table 7. Feed conversion ratio was significantly higher in fish fed SO than in fish fed the control diet. No other differences were found in FCR between fish fed the control diet and the other diets. Although there were no differences in final whole-body protein, the PER was significantly higher in the control-fed fish than in fish fed the experimental diets. The results for the PPV were similar to the PER, except that the PPV in the AO-fed fish was not significantly different from the PPV in the control-fed fish. The condition factor (K) was higher in the fish fed the two soy oil-containing diets than in the control-fed fish. No other differences in K were detected.
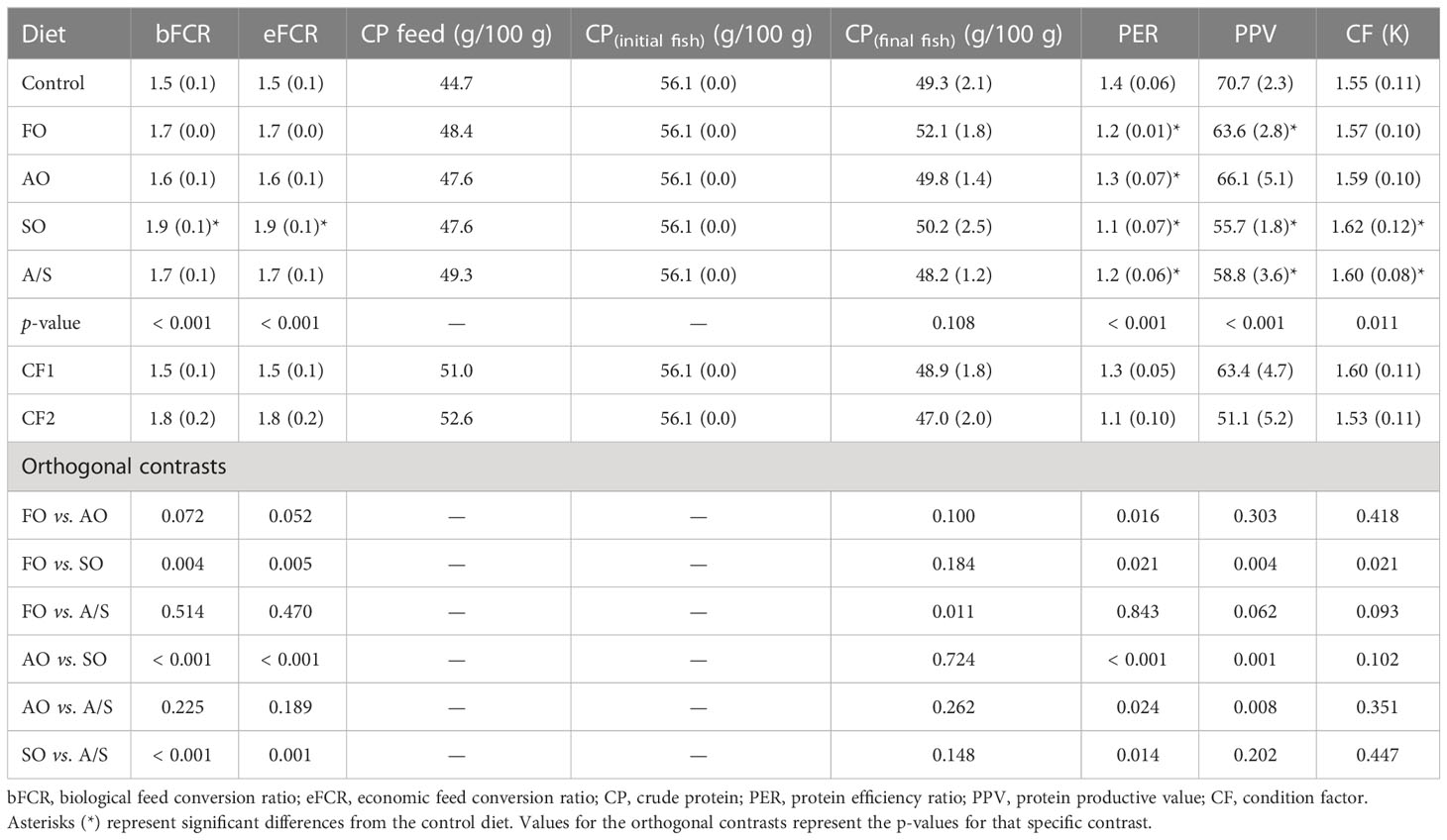
Table 7 Mean (SD) efficiency parameters for Florida pompano (Trachinotus carolinus) fed experimental diets during a 12-week comparative growth trial.
The contrasts between the fish fed the similarly formulated experimental diets varying only in their lipid composition indicated that the FO, AO, and A/S diets resulted in a significantly lower FCR than the SO diet. No differences in FCR were detected among the other diets. The PER was significantly higher in the AO-fed fish than in the other experimental diets. Conversely, PER was significantly lower in the SO-fed fish than in the other experimental diets. The protein productive value was significantly higher in fish fed the AO diet than in fish fed the A/S and SO diets, but similar to that of fish fed the FO diet. The PPV in fish fed FO was significantly higher than the PPV in fish fed the SO diet only.
The mean weight gain and respective equivalence intervals for the fish fed diets CF1 and CF2 were substantially different (Table 6; Figure 2). The TOST equivalency test for weight gain (%) between the fish fed the four experimental diets varying only in lipid composition and the closed commercial feeds CF1 and CF2 suggests that there was not an equivalency with CF1 or CF2, with the upper (θU) and lower (θL) limits of the equivalence interval set at 10% of the CF1 and CF2 means (Figure 2). The mean weight gains and the 95% CIs of fish fed the experimental diets were well below the equivalence interval of fish fed CF1. Conversely, the mean weight gains were higher in the FO-, AO-, and A/S-fed fish, and lower in the SO-fed fish than in fish fed CF2, although the 95% CIs for the experimental diets overlapped the CF2 equivalence interval (Figure 2).
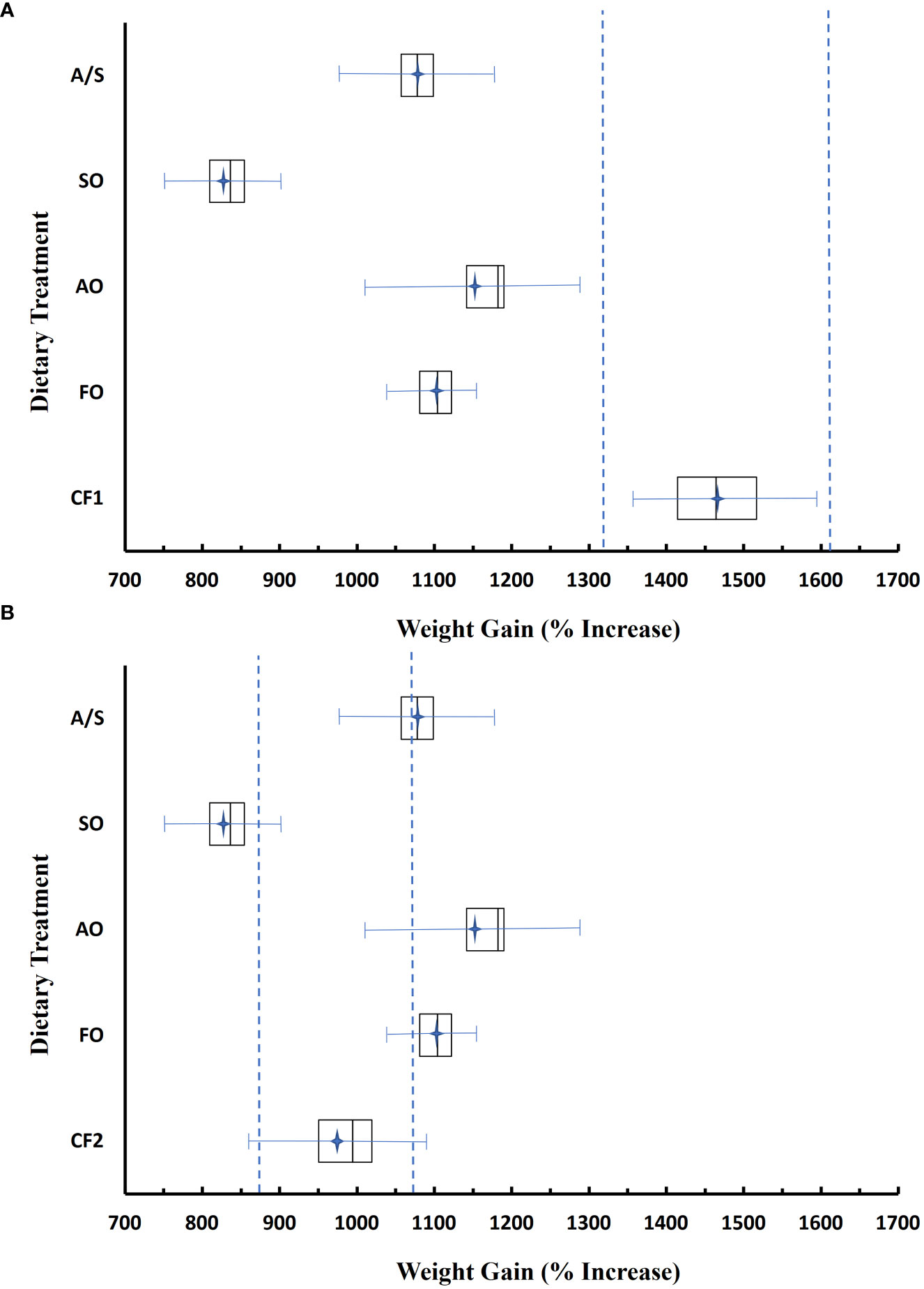
Figure 2 Weight gain of fish fed experimental diets FO (fish oil), AO (algal oil), SO (soy oil), and A/S (algal/soy oil, 50:50 w/w) relative to two closed-formula commercial diets, CF1 (A) and CF2 (B). Data were analyzed using a two one-sided t-tests (TOST) equivalency test. Boxes represent the bounds of quartiles 2 and 3; bars with endcaps represent the 95% CI around the treatment mean (stars), n = 4. Bars within the box represent the median. Dotted lines represent the upper and lower bounds of the equivalency interval, set at ±10% of the mean.
Similar to weight gain, the respective equivalence intervals for FCR were substantially different for fish fed diets CF1 and CF2 (Table 7; Figure 3). Feed conversion ratios in fish fed the FO and SO diets were considerably higher than in fish fed CF1, and the means and 95% CIs for these diets were outside the equivalence interval. Although the FCRs in fish fed the algal oil -containing diets were determined as not equivalent with CF1, their 95% CIs overlapped with the equivalence interval. Similarly, the 95% CIs for the fish fed the AO, SO, and A/S diets overlapped with the CF2 equivalence interval. Although the mean FCR and 95% CI in fish fed the FO diet graphically appears to meet equivalence to CF2, the large variability in FCR within the CF2 treatment (1.63–2.00) resulted in a wide 95% CI, precluding a declaration of equivalency (Figure 3).
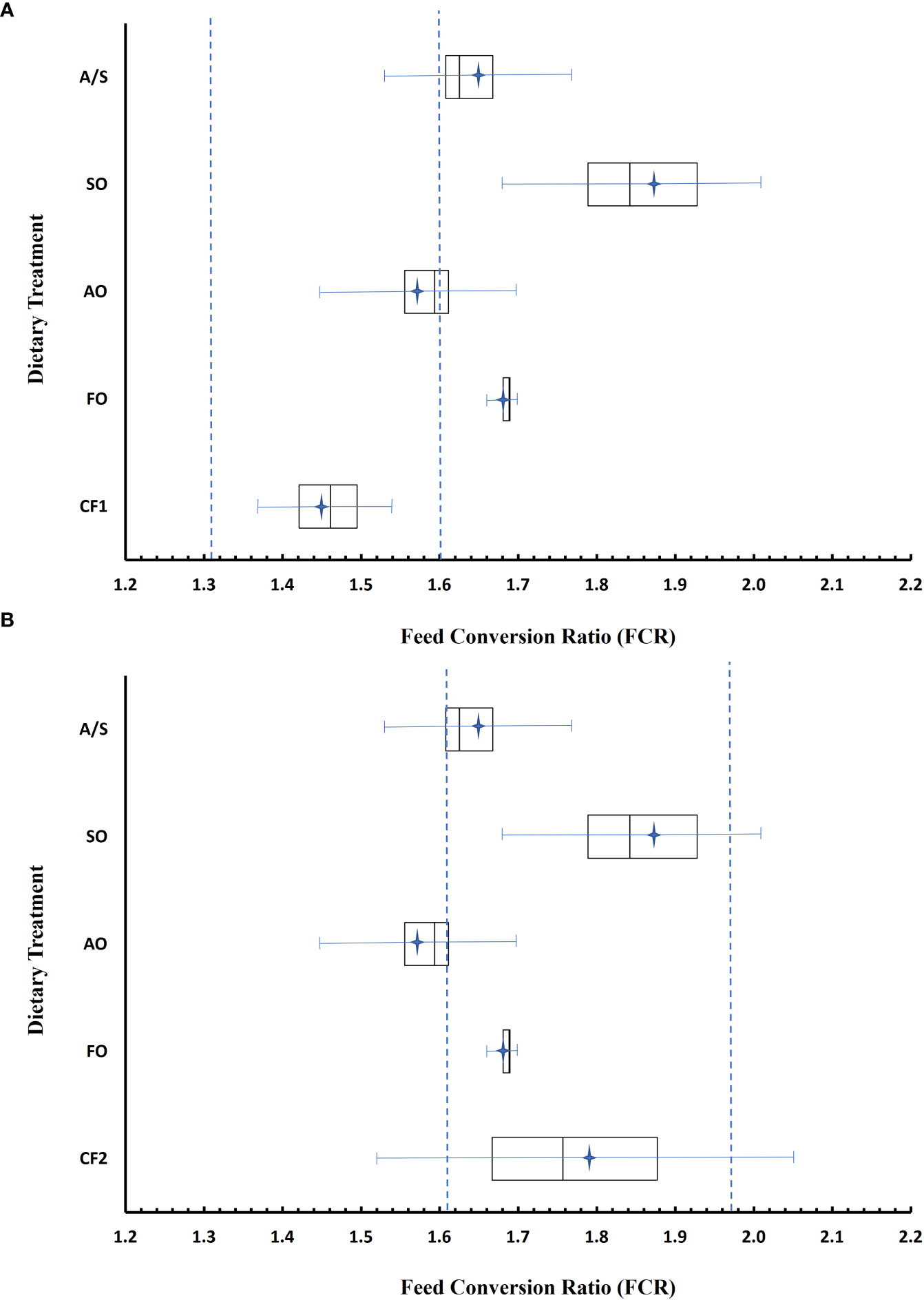
Figure 3 Feed conversion ratio (FCR) of fish fed experimental diets FO (fish oil), AO (algal oil), SO (soy oil), and A/S (algal/soy oil, 50:50 w/w) relative to two closed formula commercial diets, CF1 (A) and CF2 (B). Data were analyzed using a two one-sided t-tests (TOST) equivalency test. Boxes represent the bounds of quartiles 2 and 3; bars with endcaps represent the 95% CI around the treatment mean (stars), n = 4. Bars within the box represent the median. Dotted lines represent the upper and lower bounds of the equivalency interval, set at ±10% of the mean.
4 Discussion
To our knowledge, the use of an FM- and FO-free diet has not previously been demonstrated in Florida pompano. That the growth and performance of fish fed the experimental FM-free diets with AO and A/S was in line with that of fish fed the FM control diet was anticipated. Both the protein (spirulina) and oil (Schizochytrium) components of the diets are high-quality ingredients in aquafeeds (Sarker et al., 2016; Ragaza et al., 2020). Similarly, poultry by-product meal is well utilized by Florida pompano (Riche, 2015), particularly when supplemented with taurine (Rossi and Davis, 2012). The results of this current study are in agreement with those in California yellowtail also fed an FM-free, FO-free, Schizochytrium-based diet (Stuart et al., 2020).
Florida pompano perform well on SCO biomass, within defined limits. Rhodes et al. (2015) demonstrated that dried fermented biomass from bacteria was well utilized in Florida pompano in an FM-free diet supplemented with taurine. However, unlike in the current study, which utilized Schizochytrium-extracted oil, their diet contained FO, and the biomass principally provided dietary protein. The dietary EAA and the EAA : NEAA ratio were similar across all the diets, suggesting that the differences observed were due to differences in individual FA composition. Fish fed the SO diet exhibited a significantly lower PER, the lowest PPV, and the highest condition factor (K), suggesting a metabolic impairment.
In agreement with the results presented here, Seong et al. (2019) successfully reared the red seabream Pagrus major on FM- and FO-free diets with the addition of Schizochytrium dried powder (whole organism). The investigators demonstrated that Schizochytrium substituted for FO at 11% of the diet resulted in equivalent growth to the FO control diet. Rainbow trout (Oncorhynchus mykiss) were able to utilize diets with 75% FO replacement with vegetable oils and Schizochytrium biomass (Bélanger-Lamonde et al., 2018). The fish were able to accumulate equivalent percentages of EPA compared with the FO-fed fish and the Schizochytrium algal biomass contributed to muscle-tissue DHA (Bélanger-Lamonde et al., 2018). Betiku et al. (2016) found that feeding SCO biomass at 6% in an FM-free diet to rainbow trout resulted in equivalent growth and survival to trout fed a control diet incorporating FM and FO. Reports were similar in hybrid striped bass (Morone crhysops ♀ × M. saxatilis ♂) (Perez-Velazquez et al., 2019) and red drum (Sciaenops ocellatus) (Perez-Velazquez et al., 2018); however, both of those trials were short (6 weeks). In the closely related Golden pompano (Trachinotus ovatus), diets incorporating SCO biomass at 5% of the dry diet (approximately 25% FO replacement) resulted in growth and increased deposition of DHA and EPA (He et al., 2018). The benefit of using liquid oil as opposed to Schizochytrium biomass is that it can be used as a direct FO replacement (Tocher et al., 2019), as was done in the current study at 100%.
The SO diet was chosen as a negative control because soy oil is known to be low in DHA, EPA, and ARA (Morais et al., 2005). The FA composition of the SO diet used in the current study supports this assertion (Table 4). The high levels of 18:2 n-6 in soy oil may have interfered with LC-PUFA utilization in fish fed this diet (Glencross, 2009; Rombenso et al., 2016).
Arachidonic acid plays an important role in promoting growth, survival, and resistance to stress (Bell and Sargent, 2003). ARA modulates the stress response in fish through the regulation of cortisol (Wendellar Bonga, 1997; Van Anholt et al., 2004). Gilthead seabream were more susceptible to stress when dietary ARA was low (Van Anholt et al., 2004). Cortisol levels due to chronic stress, including confinement, affect metabolism and present as reduced appetite, lower body weight, reduced FCR, and lower immunocompetence, which all contribute to reduced growth (Wendellar Bonga, 1997; Van Anholt et al., 2004).
In some fish species ARA may be more important than EPA (Barry and Trushenski, 2020). It has been suggested Florida pompano juveniles have an ARA requirement (Mejri et al., 2021), which may not have been met by the SO diet but was met in the AO, A/S, and FO diets. This is consistent with findings in larval Florida pompano (Hauville et al., 2014).
Fatty acid profiles in fish juveniles mimicked FA profiles in the diets (Mejri et al., 2021). Juveniles from SO treatments mirrored the FA profiles of the SO diet, showing higher relative percentages of LA and LNA, and lower percentages of EFAs EPA, ARA, and DHA than fish fed other diet treatments. Our results were comparable to results from Watson et al. (2020). Red drum (Sciaenops ocellatus) juveniles, fed a 100% soy oil-based diet for 9 weeks, exhibited high levels of 18:2 n-6 and 18:3 n-3 FAs with low EFA concentrations under the minimum required by red drum species (Watson et al., 2020). A 100% replacement of FO by SO in largemouth bass (Micropterus salmoides) juveniles led to a decrease in the content of long-chain polyunsaturated fatty acids such as ARA, EPA, and DHA (Chen et al., 2020). In our study, the FA profiles in juveniles fed a blend of 50% AO and 50% SO and those fed 100% FO were similar, highlighting that 50% replacement of fish oil by soy oil could be beneficial, as shown in previous studies (Chen et al., 2020; Watson et al., 2020). Juveniles from the A/S oil treatment had comparable FA profiles to juveniles from the FO treatment. Our results demonstrate that the FA profile of the feed was well reflected in the fillet, suggesting that the 50% algal oil, 50% soy oil replacement of fish oil can be successfully used to produce Florida pompano species, maintaining high levels of EFA with a lower marine footprint than diets formulated with fish oil and a lower cost of feed production.
Growth suppression following FO replacement is not typically encountered as long as essential FAs are provided in adequate levels (Montero et al., 2001; Salini et al., 2015; Weirich et al., 2021). For many species, this condition is met with as much as a 75% replacement of FO in the diet (Sales and Glencross, 2011), and with complete replacement of FO by algal oil in the current 12-week growth trial. It is possible that supplying the diets over the course of an entire production cycle may have resulted differently. It took 27 weeks for a reduction in performance to present itself in rainbow trout fed a 75% reduction in FO (Turchini et al., 2013). Complete replacement of FO with Schizochytrium and olive oil resulted in reduced growth in seabream (Sparus aurata) (Ganuza et al., 2008). Conversely, replacing 60% of FO with various plant oils in the diets of European sea bass (Dicentrarchus labrax) had no effect on growth and survival, although tissue DHA and EPA were significantly reduced (Mourente et al., 2005).
It was suggested that the LC-PUFA requirement of Florida pompano may be lower than for many other marine species (Jackson et al., 2020), and EPA may be less important than DHA in meeting this requirement (Bowzer et al., 2016; Rombenso et al., 2017). The fatty acid profile of Veramaris® algal oil is well characterized. Reported levels are as high as 39.8% and 15.7% of lipid content for DHA and EPA, respectively, with an Omega-3 to Omega-6 ratio of 14 (Santigosa et al., 2020). An evaluation of Veramaris® compared with FO also indicated no difference in performance, digestibility of LC-PUFA, or fillet fatty acid deposition in the diets of rainbow trout (Santigosa et al., 2020).
This is the first study we are aware of that evaluated the use of an FM-free diet and an SCO as a sole source of oil in Florida pompano diets. When reducing both FM and FO in the diets of marine fish there is the risk of essential fatty acid (EFA) deficiencies (Benedito-Palos et al., 2010). However, the current study suggests that the LC-PUFA requirements were met by the AO diet as there was no residual LC-PUFA, which is often associated with dietary FM, in the FM-free diets. Diluting the AO contribution with 50% soy oil appears to have little effect on growth and performance (AO vs. A/S) for most of the parameters evaluated. The exception was protein utilization measured as PER and PPV (Table 6). This was likely less related to the oil sources and more related to the slightly higher dietary CP and slightly lower lipid content in the A/S diet, as feed intake was the same between the two diets (p = 0.727).
It is likely the LC-PUFA (i.e., DHA and EPA) requirements for Florida pompano were met even at the 5.4% AO inclusion rate. However, this remains inconclusive as tissue FA profiles were not conducted at termination of the trial. Moreover, a quantitative requirement for DHA and EPA has yet to be determined for Florida pompano and this area requires much-needed investigation. Nevertheless, it was estimated that EPA and DHA, each at 0.4% of the diet, may be adequate to meet the metabolic needs of Florida pompano when they are fed diets rich in saturated fatty acid and MUFA (Rombenso et al., 2017), although Mejri et al. (2021) suggest that the required amounts may be slightly higher. These estimates are in line with the dietary requirements of hybrid striped bass, red drum, and many other marine species (Turchini et al., 2009; NRC, 2011).
Diets rich in SFA and MUFA result in a “sparing effect” on LC-PUFA in Florida pompano (Rombenso et al., 2017), hybrid striped bass (Bowzer et al., 2016), and white seabass (Atractoscion nobilis) (Rombenso et al., 2015; Salini et al., 2017). Therefore, in addition to determining the EFA requirements of Florida pompano, a better understanding of the required ratio of LC-PUFA and SFA/MUFA is also needed in Florida pompano (Mejri et al., 2021) and other species with low LC-PUFA requirements (Menoyo et al., 2003; Navarro-Guillén et al., 2014).
It is well documented that dietary FA muscle composition reflects dietary FA profiles in Florida pompano (Rombenso et al., 2016; Rombenso et al., 2017; Jackson et al., 2020), Golden pompano (Sun et al., 2018), and many other species (Bell et al., 2002; Trushenski and Lewis, 2008; Turchini et al., 2009; Trushenski et al., 2011). Golden pompano fed a diet containing FO and supplemented with dried Schizochytrium exhibited significantly better growth performance and feed conversion than fish fed FO alone (Xie et al., 2019). The addition of Schizochytrium also increased intestinal integrity and feed intake in Golden pompano (Xie et al., 2019), but this was not observed in the current trial.
Although histology and other tissue indicators were not evaluated, the high survival among all the treatments would suggest the biological safety of the algal oil when incorporated at up to 10.8% of the diet. Efficacy and safety were also demonstrated in Atlantic salmon (Salmo salar) (Carter et al., 2003) and longfin yellowtail (Seriola rivoliana) (Kissinger et al., 2016) fed similar thraustochytrid biomass incorporated at 5%–10% of the dry diet. This is in general agreement with other demonstrated safe incorporation rates for algal biomass (Patterson and Gatlin, 2013).
There are varying reports on the suitability of ingredients used in aquafeeds. Much of this is due to a lack of standardization in feed ingredients, experimental design, feed formulation, and methodologies (Glencross et al., 2007; Barrows et al., 2008; Collins et al., 2012). In addition, the approaches and methods used by investigators vary appreciably, including methods of analysis, measured biological responses, and predictive modeling of those responses based on different data analysis and interpretation (Shearer, 2000; Glencross et al., 2007; Sales and Glencross, 2011).
The importance of using well-defined open-formula diets as a control in animal studies is well documented (Barnard et al., 2009; Pellizzon and Ricci, 2020). Using closed-formula diets makes it difficult to make scientific inferences and contributes to varying interpretations of results (Rust et al., 2015). Open-formula control diets with well-defined ingredients, as used in this study, can standardize approaches across species and laboratories and provide a basic platform for comparisons (Rust et al., 2015). Reference and control diets and the ingredients used to make them often vary spatially and temporally, and are often unknown or ill-defined. This is an issue that has not received enough attention (Bureau, 2015; Ricci, 2015).
The addition of the closed-formula diets in this study was designed to determine if the growth and survival rates of fish fed the experimental diets was similar to fish fed commercial diets. If that were to occur, then the experimental diets might be considered for testing under commercial conditions. However, as demonstrated here, the choice of closed-formula reference and control diets used can have a profound effect on the interpretation of the results. This is generally the case with closed-formula reference and control diets (Barnard et al., 2009).
The economic feed conversion ratio (eFCR) is a metric used to determine the cost–benefit ratio of feed utilization; it represents the total feed input divided by the total harvested fish output over the entire production cycle (Robb and Crampton, 2013). It integrates a number of not easily quantifiable factors into a single number and ideally it should be averaged over multiple production cycles (MBA, 2016). It allows for comparing the cost of feed to the net gain in productivity at the farm level. For a farmer to track the best use of resources, eFCR is a very useful tool. In commercial production, eFCR is a principal criterion assessed in sustainability certification (MBA, 2016). The bFCR on the other hand accounts for feed eaten, which is impossible to measure in a practical farming system, but is useful in research settings for direct comparisons under controlled conditions (Robb and Crampton, 2013). Under the conditions of this study, as in most controlled studies, these were the same, so no distinction was made for reporting the results of the FCR equivalency tests.
Standard statistical tests can suggest differences between treatments but do not demonstrate similarity among treatments. Moreover, they do not demonstrate biological importance (Perry, 1986). However, the TOST equivalency tests can be used to determine the probability that two treatments are similar enough to be considered “equivalent” (Dixon et al., 2018). Using this approach, a fish farmer can then determine if the results (efficiency, mortality, fish welfare, FCR, etc.) are similar enough that the difference may result in a lower production cost or higher profit margins. This can provide a valuable tool for producers to make on-farm cost–benefit decisions.
TOST equivalency tests were performed on weight gain and FCR. These parameters were chosen for their impact on the cost of protein production. It was deemed that a reduction in performance greater than 10% was beyond a reasonable and practicable cost–benefit breakpoint. Therefore, the equivalence interval was set at 10% around the mean of both closed-formula diets (i.e., CF1 and CF2). This is all a compelling factor for distinguishing between statistical significance and an economically relevant difference (van der Voet and Paoletti, 2019).
To declare equivalency, the CI must fall entirely within the equivalence interval. All of the experimental diets were non-equivalent to CF1 in weight gain and could be considered inferior (Figure 2). Conversely, the CI for the weight gain data crossed the CF2 equivalence interval. The interpretation of the data for the AO, A/S, and FO diets suggests that they more likely than not performed better than CF2 (EFSA, 2011; van der Voet and Paoletti, 2019).
The FCR data also suggest that the fish fed SO converted the feed into weight less efficiently than fish fed CF1; the interpretation of the data for the other diets is less clear. The FCRs for the fish fed the experimental diets, with the exception of those fed the FO diet, were also not equivalent to the FCRs for the fish fed the CF2 diet, although equivalence was more likely than not for the fish fed the A/S and SO diets. (Lakens, 2017). The FCR of fish fed the FO diet fell within the CF2 equivalence interval; however, the FCR of those fed the CF2 diet did not and therefore there was insufficient evidence to declare the experimental diets equivalent (Castelloe and Watts, 2015). In addition, the data suggest that the fish fed the AO diet more likely than not had a better FCR than those fed CF2. However, all these results should be regarded with caution as both the power and sample size were small.
The inherent large standard error variability in Florida pompano growth makes it difficult to make inferences (Campbell and Gustafson, 2018; Dixon et al., 2018). This approach would benefit from a larger sample size and a power analysis (Lakens, 2017; van der Voet and Paoletti, 2019). A post hoc analysis with the Proc Power statement of SAS, using the variability observed in this study and with a power of 80%, would suggest an appropriate sample size for more conclusive evidence (Goodman and Berlin, 1994)
We understand the limitations of the analysis for this study and consider this as a preliminary screening (Campbell and Gustafson, 2018). However, we believe that this approach demonstrates the power in designing experiments to evaluate new formulations with a high probability of declaring equivalence to an open-formula “gold-standard” or other reference diet.
Nevertheless, what is striking is the distinct differences in the equivalence intervals between the two CF diets (Figures 2, 3). Although both interval boundaries were set at 10% of the CF references, the interpretation of the results are different depending on the reference diet chosen. This underscores the importance of using well-defined and open-formula diets for standardizing diet evaluations.
5 Conclusion
In the current study we have demonstrated for the first time that co-replacement of FM and FO can be achieved in juvenile Florida pompano via an FM- and FO-free diet. It is possible to meet the EFA requirements of Florida pompano in an FM-free diet when substituting 100% of the dietary FO with fermented Schizochytrium algal oil (Veramaris®) without incurring loss of growth or efficiency. Furthermore, when feeding an FM-free diet to Florida pompano, a blend of algal oil and soy oil can replace FO without negative effects on bFCR, eFCR, weight gain, or survival. Pompano fed the FM- and FO-free feeds had growth and FCR comparable to pompano fed one commercial feed but not the other, underscoring the need for well-defined control and reference diets. These FM- and FO-free diets show potential, but there is still room for improvements. The use of microalgal oil or dried biomass in fish of different physiological and developmental stages is also an area in great need of investigation. It is important to note that the results of the equivalence test suggest that the use of closed-formula commercial feeds as control diets can lead to very different results. As stated, this trial should be viewed as a framework for directing future research and further areas of investigation.
Data availability statement
The raw data supporting the conclusions of this article will be made available by the authors, without undue reservation.
Ethics statement
The animal study was reviewed and approved by Experimental procedures that followed all guidelines regarding animal care in the Public Health Service (PHS) policy on the Humane Care and Use of Animals and were approved by Florida Atlantic University’s Institutional Animal Care and Use Committee (protocol A-19-07).
Author contributions
MR—data analysis, methodology, statistical analysis, modeling approach development, writing. FB—conceptualization, methodology, resources, research design, draft editing. ZN—research, sample analysis. SM—data analysis, sample analysis, statistical analysis, writing, draft editing. KC—conceptualization, administrative oversight, draft editing. PW—administrative oversight, data analysis, statistical analysis, draft editing. All authors contributed to the article and approved the submitted version.
Acknowledgments
The authors would like to acknowledge the contributions and technical support provided by Tyler Bianchine, Gabriel Jimenez, and Erik Perna of Florida Atlantic University’s Harbor Branch Oceanographic Institute, Fort Pierce, FL. This study was funded in part by the Anthropocene Institute, Palo Alto, CA, under the Anthropocene Institute Science and Technology Support Fund. This work was also supported in part by the U.S. Department of Agriculture (USDA) Agricultural Research Service by a cooperative agreement, number 59-6034-9-007, with Florida Atlantic University’s Harbor Branch Oceanographic Institute.
Conflict of interest
The authors declare that the research was conducted in the absence of any commercial or financial relationships that could be construed as a potential conflict of interest.
The authors MR and SM declare that they were editorial board members of Frontiers at the time of submission. This had no impact on the peer review process and the final decision.
Publisher’s note
All claims expressed in this article are solely those of the authors and do not necessarily represent those of their affiliated organizations, or those of the publisher, the editors and the reviewers. Any product that may be evaluated in this article, or claim that may be made by its manufacturer, is not guaranteed or endorsed by the publisher.
Author disclaimer
Mention of trade names or commercial products in this publication is solely for the purpose of providing specific information and does not imply recommendation or endorsement by the USDA. USDA is an equal opportunity provider and employer.
References
AOAC (2006). Official methods of analysis. 18th edition (Gaithersburg, MD, USA: Association of Official Analytical Chemists).
Barnard D. E., Lewis S. M., Teter B. B., Thigpen J. E. (2009). Open- and closed-formula laboratory animal diets and their importance to research. J. Am. Assoc. Lab. Anim. 48, 709–713.
Barrows F. T., Bellis D., Krogdahl Å., Silverstein J. T., Herman E. M., Sealy W. M., et al. (2008). Report of the plant products in aquafeed strategic planning workshop: an integrated , interdisciplinary research roadmap for increasing utilization of plant feedstuffs in diets for carnivorous fish. Rev. Fish Sci. 16, 449–455. doi: 10.1080/10641260802046734
Barry K. J., Trushenski J. T. (2020). Reevaluating polyunsaturated fatty acid essentiality in hybrid striped bass. N Am. J. Aquacult. 82, 307–320. doi: 10.1002/naaq.10130
Bélanger-Lamonde A., Sarker P. K., Ayotte P., Bailey J. L., Bureau D. P., Chouinard P. Y., et al. (2018). Algal and vegetable oils as sustainable fish oil substitutes in rainbow trout diets: an approach to reduce contaminant exposure. J. Food Qual. 7949782. doi: 10.1155/2018/7949782
Bell J. G., Henderson R. J., Tocher D. R., McGhee F., Dick J. R., Porter A., et al. (2002). Substituting fish oil with crude palm oil in the diet of Atlantic salmon (Salmo salar) affects muscle fatty acid composition and hepatic fatty acid metabolism. J. Nutr. 132, 222–230. doi: 10.1093/jn/132.2.222
Bell J. G., Sargent J. R. (2003). Arachidonic acid in aquaculture feeds: current status and future opportunities. Aquaculture 218, 491–499. doi: 10.1016/S0044-8486(02)00370-8
Benedito-Palos L., Navarro J. C., Kaushik S., Pérez-Sánchez J. (2010). Tissue specific robustness of fatty acid signatures in cultured gilthead seabream (Sparus aurata l.) fed practical diets with a combined high replacement of fish meal and fish oil. J. Anim. Sci. 88, 1759–1770. doi: 10.2527/jas.2009-2564
Betiku O. C., Barrows F. T., Ross C., Sealy W. M. (2016). The effect of total replacement of fish oil with DHA-gold and plant oils on growth and fillet quality of rainbow trout (Oncorhynchus mykiss) fed a plant-based diet. Aquacult. Nutr. 22, 158–169. doi: 10.1111/anu.12234
Bowzer J., Jackson C., Trushenski J. (2016). Hybrid striped bass feeds based on fish oil, beef tallow, and eicosapentaenoic acid/docosahexaenoic acid supplements: insight regarding fish oil sparing and demand for n-3 long-chain polyunsaturated fatty acids. J. Anim. Sci. 94, 978–988. doi: 10.2527/jas2015-9199
Bureau D. P. (2015). Fish feed optimization still needs some work. Inform: Int. News Fats Oils Related Mater. 26, 262–265.
Campbell H., Gustafson P. (2018). Conditional equivalence testing: an alternative remedy for publication bias. PloS One 13 (4), e0195145. doi: 10.1371/journal.pone.0195145
Carter C. G., Bransden M. P., Lewis T. E., Nichols P. D. (2003). Potential of thraustochytrids to partially replace fish oil in Atlantic salmon feeds. Mar. Biotechnol. 5, 480–492. doi: 10.1007/s10126-002-0096-8
Castelloe J., Watts D. (2015) Equivalence and noninferiority testing using SAS/STAT software, paper SAS1191-2015 (Dallas, TX: SAS Global Forum) (Accessed 11/02/2022).
Chen Y., Sun Z., Liang Z., Xie Y., Tan X., Su J., et al. (2020). Addition of l-carnitine to formulated feed improved growth performance, antioxidant status and lipid metabolism of juvenile largemouth bass, Micropterus salmoides. Aquaculture 518, 734434. doi: 10.1016/j.aquaculture.2019.734434
Collins S. A., Desai A. R., Mansfield G. S., Hill J. E., Van Kessel A. G., Drew M. D. (2012). The effect of increasing inclusion rates of soybean, pea and canola meals and their protein concentrates on the growth of rainbow trout: concepts in diet formulation and experimental design for ingredient evaluation. Aquaculture 344-349, 90–99. doi: 10.1016/j.aquaculture.2012.02.018
Couturier L. I. E., Michel L. N., Amaro T., Budge S. M., Da Costa E., De Troch M., et al. (2020). State of art and best practices for fatty acid analysis in aquatic sciences. ICES J. Mar. Sci. 77 (7-8), 2375–2395. doi: 10.1093/icesjms/fsaa121
Davidson J., Barrows F. T., Kenney P. B., Good C., Schroyer K., Summerfelt S. T. (2016). Effects of feeding fishmeal-free versus a fishmeal-based diet on post-smolt Atlantic salmon Salmo salar performance, water quality, and waste production in recirculation aquaculture systems. Aquacult. Eng. 74, 38–51. doi: 10.1016/j.aquaeng.2016.05.004
Dixon P. M., Saint-Maurice P. F., Kim Y., Bai Y., Welk G. J. (2018). A primer on the use of equivalence testing for evaluating measurement agreement. Med. Sci. Sports Exerc 50, 837–845. doi: 10.1249/MSS.0000000000001481
Drew M. D., Ogunkoya A. E., Janz D. M., Van Kessel A. G. (2007). Dietary influence of replacing fish meal and oil with canola protein concentrate and vegetable oils on growth, performance, fatty acid composition and organochlorine residues in rainbow trout (Oncorhynchus mykiss). Aquaculture 267, 260–268. doi: 10.1016/j.aquaculture.2007.01.002
EFSA (2011). Guidance for the risk assessment of food and feed from genetically modified plants: EFSA panel on genetically modified organisms (GMO). EFSA J. 9, 2150. doi: 10.2903/j.efsa.2011.2150
FAO (2020). The state of world fisheries and aquaculture. sustainability in action (Rome). doi: 10.4060/ca9229en
Folch J., Lees M., Sloane Stanley G. H. (1957). A simple method for the isolation and purification of total lipides from animal tissues. Biol. Chem. 226 (1), 497–509. doi: 10.1016/S0021-9258(18)64849-5
Froehlich H. E., Jacobsen N. S., Essington T. E., Clavelle T., Halpern B. S. (2018). Avoiding the ecological limits of forage fish for fed aquaculture. Nat. Sustain 1, 298–303. doi: 10.1038/s41893-018-0077-1
Ganuza E., Benítez-Santana T., Atalah E., Vega-Orellana O., Ganga R., Izquierdo M. S. (2008). Crypthecodinium cohnii and Schyzochytrium sp. as potential substitutes to fisheries-derived oils from seabream (Sparus aurata) microdiets. Aquaculture 277, 109–116. doi: 10.1016/j.aquaculture.2008.02.005
Gatlin D. M., Barrows F. T., Brown P., Dabrowski K., Gaylord T. G., Hardy R. W., et al. (2007). Expanding the utilization of sustainable plant products in aquafeeds: a review. Aquac. Res. 38, 551–579. doi: 10.1111/j.1365-2109.2007.01704.x
Glencross B. D. (2009). Exploring the nutritional demand for essential fatty acids by aquaculture species. Rev. Aquacult. 1, 7–124. doi: 10.1111/j1753-5131.2009.01006.x
Glencross B. D., Baily J., Berntssen M. H. G., Hardy R., MacKenzie S., Tocher D. R. (2020). Risk assessment of the use of alternative animal and plant raw material resources in aquaculture feeds. Rev. Aquacult. 12, 703–758. doi: 10.1111/raq.12347
Glencross B. D., Booth M., Allan G. L. (2007). A feed is only as good as its ingredients – a review of ingredient evaluation strategies for aquaculture feeds. Aquacult. Nutr. 13, 17–34. doi: 10.1111/j.1365-2095.2007.00450.x
Goodman S. N., Berlin J. A. (1994). The use of predicted confidence intervals when planning experiments and the misuse of power when interpreting results. Ann. Intern. Med. 21, 200–206. doi: 10.7326/0003-4819-121-3-199408010-00008
Hauville M. R., Zambonino-Infante J. L., Bell G., Migaud H., Main K. L. (2014). Impacts of three different microdiets on Florida pompano, Trachinotus carolinus, weaning success, growth, fatty acid incorporation and enzyme activity. Aquaculture 422-423, 268–276. doi: 10.1016/j.aquaculture.2013.12.006
He Y., Lin G., Rao X., Chen L., Jian H., Wang M., et al. (2018). Microalga Isochrysis galbana in feed for Trachinotus ovatus: effect on growth performance and fatty acid composition of fish fillet and liver. Aquacult. Int. 26, 1261–1280. doi: 10.1007/s10499-018-0282-y
Hong M. Y., Lumibao J., Mistry P., Saleh R., Hoh E. (2015). Fish oil contaminated with persistent organic pollutants reduces antioxidant capacity and induces oxidative stress without affecting its capacity to lower lipid concentrations and systemic inflammation in rats. J. Nutr. 145 (5), 939–944. doi: 10.3945/jn.114.206607
Ialongo C. (2017). The logic of equivalence testing and its use in laboratory medicine. Biochem. Med. 27, 5–13. doi: 10.11613/BM.2017.001
Jackson C. J., Trushenski J. T., Schwarz M. H. (2020). Assessing polyunsaturated fatty acid essentiality in Florida pompano. N Am. J. Aquacult. 82, 293–306. doi: 10.1002/naaq.10140
Kerr N. (1998). HARKing: hypothesizing after the results are known. Pers. Soc. Psycol. Rev. 2, 196–217. doi: 10.1207/s15327957pspr0203_4
Kissinger K. R., García-Ortega A., Trushenski J. T. (2016). Partial fish meal replacement by soy protein concentrate, squid and algal meals in low fish-oil diets containing Schizochytrium limacinum for longfin yellowtail Seriola rivoliana. Aquaculture 452, 37–44. doi: 10.1016/j.aquaculture.2015.10.022
Kousoulaki K., Krasnov A., Torgersen J. S., Mørkøre T., Sweetman J. (2015). Metabolism, health and fillet nutritional quality in Atlantic salmon (Salmo salar) fed diets containing n-3-rich microalgae. J. Nutr. Sci. vol 4, e24. doi: 10.1017/jns.2015.14
Lakens D. (2017). Equivalence tests: a practical primer for t-tests, correlations, and meta-analysis. Soc. Psychol. Pers. Sci. 8, 355–362. doi: 10.1177/1948550617697177
Lepage G., Roy C. (1984). Improved recovery of fatty acid through direct transesterification without prior extraction or purification. J. Lipid Res. 25, 1391–1396. doi: 10.1016/S0022-2275(20)34457-6
Lewis T. E., Nichols P. D., McMeekin T. A. (1999). The biotechnological potential of thraustochytrids. Mar. Biotechnol. 1, 580–587. doi: 10.1007/PL00011813
Lippmeier J. C., Crawford K. S., Owen C. B., Rivas A. A., Metz J. G., Apt K. E. (2009). Characterization of both polyunsaturated fatty acid biosynthetic pathways in Schizochytrium sp. Lipids 44, 621–630. doi: 10.1007/s11745-009-3311-9
MBA (2016) Aquaculture standard version A4.0. Available at: https://www.seafoodwatch.org/globalassets/sfw/pdf/standards/aquaculture/seafood-watch-aquaculture-standard-version-a4.pdf.
McLean E., Alfrey K., Gatlin D., Gaylord G., Barrows F. (2022). Muscle amino acid profiles of eleven species of aquacultured animals and their application to ideal protein-based feeds. Aquacult. Fish. doi: 10.1016/j.aaf.2022.04.010
Mejri S. C., Tremblay R., Audet C., Wills P. S., Riche M. (2021). Essential fatty acid requirements in tropical and cold-water marine fish larvae and juveniles. Front. Mar. Sci. doi: 10.3389/fmars.2021.680003
Menoyo D., Lopez-Bote C. J., Bautista J. M., Obach A. (2003). Growth, digestibility and fatty acid utilization in large Atlantic salmon (Salmo salar) fed varying levels of n-3 and saturated fatty acids. Aquaculture 225, 295–230. doi: 10.1016/S0044-8486(03)00297-7
Miller M. R., Nichols P. D., Carter C. G. (2007). Replacement of fish oil with thraustochytrid Schizochytrium sp. l oil in Atlantic salmon parr (Salmo salar l) diets. Comp. Biochem. Phys. A 148, 382–392. doi: 10.1016/j.cbpa.2007.05.018
Miller M. R., Nichols P. D., Carter C. G. (2011). “New alternative n-3 long-chain polyunsaturated fatty acid-rich oil sources,” in Fish oil replacement and alternative lipid sources in aquaculture feeds. Eds. Turchini G. M., Ng W.-K., Tocher D. R. (Boca Raton, FL: CRC Press), 325–349.
Montero D., Robaina L. E., Socorro J., Vergara J. M., Tort L., Izquierdo M. S. (2001). Alteration of liver and muscle fatty acid composition in gilthead seabream (Sparus aurata) juveniles held at high stocking density and fed an essential fatty acid deficient diet. Fish Physiol. Biochem. 24, 63–72. doi: 10.1023/A:1011145426543
Morais S., Koven W., Rønnestad I., Dinis M. T., Conceição L. E. C. (2005). Dietary protein:lipid ratio and lipid nature affects fatty acid absorption and metabolism in a teleost larva. Brit J. Nutr. 93, 813–820. doi: 10.1079/BJN20051378
Mourente G., Good J. E., Bell J. G. (2005). Partial substitution of fish oil with rapeseed, linseed, and olive oils in diets for European sea bass (Dicentrarchus labrax l.): effects on flesh fatty acid composition, plasma prostaglandins E2 and F2α, immune function and effectiveness of a fish oil finishing diet. Aquacult. Nutr. 11, 25–40. doi: 10.1111/j.1365-2095.2004.00320.x
Navarro-Guillén C., Engrola S., Castanheira F., Bandarra N., Hachero-Cruzado I., Tocher D. R., et al. (2014). Effect of varying dietary levels of LC-PUFA and vegetable oil sources on performance and fatty acids of Senegalese sole post larvae: puzzling results suggest complete biosynthesis pathway from C18 PUFA to DHA. Comp. Biochem. Physiol. B 167, 51–58. doi: 10.1016/j.cbpb.2013.10.001
Naylor R. L., Hardy R. W., Bureau D. P., Chiu A., Elliott M., Farrell A. P., et al. (2009). Feeding aquaculture in an era of finite resources. P Natl. Acad. Sci. U.S.A. 106, 15103–15110. doi: 10.1073/pnas.0905235106
NRC (1995). Building a north American feed information system (Washington, D.C: National Academy Press).
Parrish C. C. (1999). “Determination of total lipid, lipid classes, and fatty acids in aquatic samples,” in Lipids in freshwater ecosystems. Eds. Art M. T., Wainman B. C. (New York: Springer Verlag), 4–20.
Patterson D., Gatlin D. M. III (2013). Evaluation of whole and lipid-extracted algae meals in the diets of juvenile red drum (Sciaenops ocellatus). Aquaculture 416-417, 92–98. doi: 10.1016/j.aquaculture.2013.08.033
Pellizzon M. A., Ricci M. R. (2020). Choice of laboratory rodent diet may confound data interpretation and reproducibility. Curr. Dev. Nutr. 4, nzaa031. doi: 10.1093/cdn/nzaa031
Perez-Velazquez M., Gatlin D. M. III, González-Félix M. L., García-Ortega A. (2018). Partial replacement of fishmeal and fish oil by algal meals in diets of red drum Sciaenops ocellatus. Aquaculture 487, 41–50. doi: 10.1016/j.aquaculture.2018.01.001
Perez-Velazquez M., Gatlin D. M. III, González-Félix M. L., García-Ortega A., de Cruz C. R., Juárez-Gómez M. L., et al. (2019). Effect of fish meal and fish oil replacement by algal meals on biological performance and fatty acid profile of hybrid striped bass (Morone crhysops ♀ x m. saxatilis ♂). Aquacult. 506, 83–90. doi: 10.1016/j.aquaculture.2019.04.011
Perry J. N. (1986). Multiple-comparison procedures: a dissenting view. J. Econ. Entomol. 79, 1149–1155. doi: 10.1093/jee/79.5.1149
Pickova J., Mørkøre T. (2007). Alternate oils in fish feeds. Eur. J. Lipid Sci. Tech. 109, 256–263. doi: 10.1002/ejlt.200600222
Qiao H., Wang H., Song Z., Ma J., Li B., Liu X., et al. (2014). Effects of dietary fish oil replacement by microalgae raw materials on growth performance, body composition and fatty acid profile of juvenile olive flounder, Paralichthys olivaceus. Aquacult. Nutr. 20, 646–653. doi: 10.1111/anu.12127
Ragaza J. A., Hossain M. S., Meiler K. A., Velasquez S. F., Kumar V. (2020). A review on spirulina: alternative media for cultivation and nutritive value as an aquafeed. Rev. Aquacult. 12, 2371–2395. doi: 10.1111/raq.12439
Ren L.-J., Ji X.-J., Huang H., Qu L., Feng Y., Tong Q.-Q., et al. (2010). Development of a stepwise aeration control strategy for efficient docosahexaenoic acid production by Schizochytrium sp. Appl. Microbiol. Biot. 87, 1649–1656. doi: 10.1007/s00253-010-2639-7
Rhodes M. A., Zhou Y., Davis D. A. (2015). Use of dried fermented biomass as a fish meal replacement in practical diets of Florida pompano, Trachinotus carolinus. J. Appl. Aquacult. 27, 29–39. doi: 10.1080/10454438.2014.959834
Ricci M. (2015). Laboratory animal control diets: very important, often neglected. Lab. Anim. 44, 240–241. doi: 10.1038/laban.786
Riche M. (2009). Evaluation of digestible energy and protein for growth and nitrogen retention in juvenile Florida pompano Trachinotus carolinus. J. World Aquacult. Soc. 40, 45–57. doi: 10.1111/j.1749-7345.2008.00234.x
Riche M. (2015). Nitrogen utilization from diets with refined and blended poultry by-products as partial fishmeal replacements in diets for low-salinity cultured Florida pompano, Trachinotus carolinus. Aquaculture 435, 458–466. doi: 10.1016/j.aquaculture.2014.10.001
Ricker W. E. (1975). Computation and interpretation of biological statistics of fish populations. B Fish Res. Board Can. 191, 1–382. doi: 10.2307/3800109
Robb D. H. F., Crampton V. O. (2013). “On-farm feeding and feed management: perspectives from the fish feed industry,” in On-farm feeding and farm management in aquaculture, vol. 583 . Eds. Hasan M. R., New M. B. (Rome: FAO), 489–518. Available at: http://www.fao.org/3/i3481e/i3481e.pdf.
Rombenso A. N., Trushenski J. T., Jirsa D., Drawbridge M. (2015). Successful fish oil sparing in white seabass feeds using saturated fatty acid-rich soybean oil and 22:6n-3 (DHA) supplementation. Aquaculture 448, 176–185. doi: 10.1016/j.aquaculture.2015.05.041
Rombenso A. N., Trushenski J. T., Schwarz M. H. (2016). Fish oil replacement in feeds for juvenile Florida pompano: composition of alternative lipid influences degree of tissue fatty acid profile distortion. Aquaculture 458, 177–186. doi: 10.1016/j.aquaculture.2016.03.009
Rombenso A. N., Trushenski J. T., Schwarz M. H. (2017). Beef tallow is suitable as a primary lipid source in juvenile Florida pompano feeds. Aquacult. Nutr. 23, 1274–1286. doi: 10.1111/anu.12502
Rossi W. Jr., Davis D. A. (2012). Replacement of fishmeal with poultry by-product meal in the diet of Florida pompano Trachinotus carolinus l. Aquaculture 338-341, 160–166. doi: 10.1016/j.aquaculture.2012.01.026
Rust M. B., Barrows F. T., Drawbridge M., Hart E. R., Stuart K., Webb K., et al. (2015). Development and characterization of several open formula reference diets for marine fish larvae. Bull. Fish Res. Agen 40, 75–77.
Rust M. B., Barrows F. T., Hardy R. W., Lazur A., Naughten K., Silverstein J. (2011) The future of aquafeeds, NOAA/USDA alternative feeds initiative (U.S. Dept. of Commerce, NOAA Tech). Available at: https://spo.nmfs.noaa.gov/sites/default/files/tm124.pdf (Accessed November 02, 2022).
Sales J., Glencross B. (2011). A meta-analysis of the effects of dietary marine oil replacement with vegetable oils on growth, feed conversion and muscle fatty acid composition of fish species. Aquacult. Nutr. 17, e271–e287. doi: 10.1111/j.1365-2095.2010.00761.x
Salini M., Irvin S., Bourne N., Blyth D., Cheers S., Habilay N., et al. (2015). Marginal efficiencies of long chain-polyunsaturated fatty acid use by barramundi (Lates calcarifer) when fed diets with varying blends of fish oil and poultry fat. Aquaculture 449, 48–57. doi: 10.1016/j.aquaculture.2015.02.027
Salini M. J., Turchini G. M., Glencross B. D. (2017). Effect of dietary saturated and monounsaturated fatty acids in juvenile barramundi Lates calcarifer. Aquacult. Nutr. 23, 264–275. doi: 10.1111/anu.12389
Santigosa E., Constant D., Prudence D., Wahli T., Verlhac-Trichet V. (2020). A novel marine algal oil containing both EPA and DHA is an effective source of omega-3 fatty acids for rainbow trout (Oncorhynchus mykiss). J. World Aquacult. Soc. 51, 649–655. doi: 10.1111/jwas.12699
Sargent J. R., Tocher D. R., Bell J. G. (2002). “The lipids,” in Fish nutrition, 3rd edition. Eds. Halver J. E., Hardy R. W. (San Diego, CA: Elsevier), 181–257.
Sarker P. K., Gamble M. M., Kelson S., Kapuscinski A. R. (2016). Nile Tilapia (Oreochromis niloticus) show high digestibility of lipid and fatty acids from marine Schizochytrium sp. and of protein and essential fatty acids from freshwater Spirulina sp. feed ingredients. Aquacult. Nutr. 22, 109–119. doi: 10.1111/anu.12230
Schuirmann D. J. (1987). A comparison of the two one-sided tests procedure and the power approach for assessing the equivalence of average bioavailability. J. Pharmacokinet. Pharm. 15, 657–680. doi: 10.1007/BF01068419
Seong T., Matsutani H., Hagata Y., Kitagima R., Satoh S. (2019). First step of non-fishmeal, non-fish oil diet development for red seabream, (Pagrus major), with plant protein sources and microalgae Schizochytrium sp. Aquac. Res. 50, 2460–2468. doi: 10.1111/are.14199
Shah M, Lutzu G. A., Alam A., Sarker P., Chowdhury M.A.K., Parsaeimehr A., et al. (2018). Microalgae in aquafeeds for a sustainable aquaculture industry. J. Appl. Phycol 30, 197–213. doi: 10.1007/s10811-017-1234-z
Shearer K. D. (2000). Experimental design, statistical analysis and modelling of dietary nutrient requirement studies for fish: a critical review. Aquacult. Nutr. 6, 91–102. doi: 10.1046/j.1365-2095.2000.00134.x
Stuart K. R., Barrows F. T., Silbernagel C., Alfrey K., Rotstein D., Drawbridge M. A. (2020). Complete replacement of fish oil and fish meal in the diet of juvenile California yellowtail Seriola dorsalis. Aquacult. Res. 52, 655–665. doi: 10.1111/are.14923
Sun X., Guo H., Zhu K., Zhang N., Yu W., Wu N., et al. (2018). Feed type regulates the fatty acid profiles of golden pompano Trachinotus ovatus (Linnaeus 1758). J. Appl. Anim. Res. 46, 60–63. doi: 10.1080/09712119.2016.1259110
Tacon A. G. J., Metian M. (2008). Global overview on the use of fish meal and fish oil in industrially compounded aquafeeds: trends and future prospects. Aquaculture 285, 146–1158. doi: 10.1016/j.aquaculture.2008.08.015
Tocher D. R. (2003). Metabolism and functions of lipids and fatty acids in teleost fish. Rev. Fish Sci. 11, 107–184. doi: 10.1080/713610925
Tocher D. R., Betancor M. B., Sprague M., Olsen R. E., Napier J. A. (2019). Omega-3 long-chain polyunsaturated fatty acids, EPA and DHA: bridging the gap between supply and demand. Nutrients 11, 89. doi: 10.3390/nu11010089
Trushenski J. T., Gause B., Lewis H. A. (2011). Selective fatty acid metabolism, not the sequence of dietary fish oil intake, prevails in fillet fatty acid profile change in sunshine bass. N Am. J. Aquacult. 73, 204–211. doi: 10.1080/15222055.2011.579029
Trushenski J. T., Lewis H. A. (2008). Fatty acid profile of sunshine bass: i. profile change is affected by initial composition and differs among tissues. Lipids 43, 629–641. doi: 10.1007/s11745-008-3188-z
Trushenski J. T., Lochman R. T. (2009). Potential, implications, and solutions regarding the use of rendered animal fats in aquafeeds. Am. J. Anim. Vet. Sci. 4, 108–128. doi: 10.3844/ajavsp.2009.108.128
Turchini G. M., Hermon K., Cleveland B. J., Emery J. A., Rankin T., Francis D. S. (2013). Seven fish oil substitutes over a rainbow trout grow-out cycle: I) effects on performance and fatty acid metabolism. Aquacult. Nutr. 19, 82–94. doi: 10.1111/anu.12046
Turchini G. M., Torstensen B. E., Ng W.-K. (2009). Fish oil replacement in finfish nutrition. Rev. Aquacult. 1, 10–57. doi: 10.1111/j.1753-5131.2008.01001.x
Van Anholt R. D., Spannings F. A. T., Koven W. M., Nixon O., Wendelaar Bonga S. E. (2004). Arachidonic acid reduces the stress response of gilthead seabream Sparus aurata l. J. Exp. Biol. 20, 3419–3430. doi: 10.1242/jeb.01166
van der Voet H., Paoletti C. (2019). Equivalence testing approaches in genetically modified organism risk assessment. J. Agric. Food Chem. 67, 13506–13508. doi: 10.1021/acs.jafc.9b05149
Wachs S. (2015) What is equivalence testing & when should we use it. Available at: https://www.integral-concepts.com/wp-content/media/What-is-Equivalence-Testing-and-When-Should-We-Use-It.pdf (Accessed November 16, 2022).
Watson A. M., Napolitano M. P., Schock T. B., Bowden J. A., Frost J., Yost J., et al. (2020). Evaluation of graded levels of soy oil as a fish oil replacement in high soy protein feeds for juvenile red drum, Sciaenops ocellatus. Aquaculture 529, 735627. doi: 10.1016/j.aquaculture.2020.735627
Weirich C. R., Riche M. (2006). Acute tolerance of juvenile Florida pompano, Trachinotus carolinus l. @ to ammonia and nitrite at various salinities. Aquac. Res. 37, 855–861. doi: 10.1111/j.1365-2109.2006.01502.x
Weirich C. R., Riley K. L., Riche M., Main K. L., Wills P. S., Illán G., et al. (2021). The status of Florida pompano Trachinotus carolinus, as a commercially ready species for U.S. marine aquaculture. J. World Aquacult. Soc. 52, 731–763. doi: 10.1111/jwas.12809
Wendellar Bonga S. E. (1997). The stress response in fish. Physiol. Rev. 77, 591–625. doi: 10.1152/physrev.1997.77.3.591
Xie J., Fang H., Liao S., Guo T., Yin P., Liu Y., et al. (2019). Study on Schizochytrium sp. improving the growth performance and non-specific immunity of golden pompano (Trachinotus ovatus) while not affecting the antioxidant capacity. Fish Shellfish Immun. 95, 617–623. doi: 10.1016/j.fsi.2019.10.028
Keywords: DHA, EPA, Florida pompano, microalgae, open-formula, fish-meal free, Trachinotus carolinus
Citation: Riche M, Barrows FT, Nilles Z, Mejri S, Campbell K and Wills PS (2023) Replacement of fish oil with a high-DHA algal oil in a fishmeal-free diet fed to Florida pompano (Trachinotus carolinus). Front. Aquac. 2:1163542. doi: 10.3389/faquc.2023.1163542
Received: 10 February 2023; Accepted: 01 June 2023;
Published: 06 July 2023.
Edited by:
Iciar Martinez, University of the Basque Country, SpainReviewed by:
Lee Seong Wei, Universiti Malaysia Kelantan, MalaysiaAlice Marie Pedersen, Calanus, Norway
Trygve Sigholt, BioMar, Norway
Copyright © 2023 Riche, Barrows, Nilles, Mejri, Campbell and Wills. This is an open-access article distributed under the terms of the Creative Commons Attribution License (CC BY). The use, distribution or reproduction in other forums is permitted, provided the original author(s) and the copyright owner(s) are credited and that the original publication in this journal is cited, in accordance with accepted academic practice. No use, distribution or reproduction is permitted which does not comply with these terms.
*Correspondence: Marty Riche, richem@fau.edu