Effects of Acipenserid herpesvirus 2 on the outcome of a Streptococcus iniae co-infection in white sturgeon (Acipenser transmontanus)
- 1Department of Medicine and Epidemiology, School of Veterinary Medicine, University of California, Davis, Davis, CA, United States
- 2California Animal Health and Food Safety Laboratory, San Bernardino, CA, United States
- 3Atlantic Veterinary College, University of Prince Edward Island, Charlottetown, PE, Canada
Acipenserid herpesvirus 2 (AciHV-2) is a large double-stranded DNA virus in the family Alloherpesviridae that causes catastrophic outbreaks in young naive white sturgeon (Acipenser transmontanus) populations, with mortalities of up to 80%. Survivors of these infections are suspected to remain latently infected. The gram-positive zoonotic bacterium Streptococcus iniae is another important sturgeon pathogen that causes severe myositis and up to 50% mortality during natural outbreaks. Throughout the last decade, co-infections of AciHV-2 and S. iniae have been reported in cultured white sturgeon in California resulting in severe presentations of piscine streptococcosis. This phenomenon of herpesvirus and streptococcus co-infection appears to span multiple taxa since in humans, it is recognized that a Human herpesvirus 3 infection (VZV) is a negative prognostic indicator for pediatric Invasive Group A Streptococcal infections (IGASI). While a decrease in humoral immunity caused by VZV has been hypothesized as a potentially important factor in IGASI cases, no natural animal model exists to study this process. Moreover, no studies have investigated these reported co-infections in white sturgeon. Therefore, the goal of this study was to investigate the effects of a recent AciHV-2 infection on the outcome of a subsequent S. iniae challenge in white sturgeon fingerlings. When fish were infected with 108 colony forming units (CFU) of S. iniae intramuscularly (IM), a statistically significant decrease in survival of 41% was detected in the co-infection group compared to the S. iniae group (p-value < 0.001). This difference was not observed when fish were infected with 106 CFU of S. iniae IM. At this lower infection dose, however, a statistically significant downregulation of tnfα was observed in the spleen of fish in the co-infection group compared to the S. iniae group (p-value = 0.0098). Analysis of serum from survivors revealed a statistically significant reduction in anti-S. iniae serum IgM and serum serotransferrin in fish from the co-infection group compared to the S. iniae group (p-value = 0.0134 and p-value = 0.0183, respectively). Further studies are indicated to determine what interactions lead to the decreased production of pathogen-specific IgM, serotransferrin, and TNFα in the host.
1 Introduction
The white sturgeon (Acipenser transmontanus) is an anadromous fish native to the Pacific Coast of North America (Kelly, 2019) and a central part of the multi-million-dollar aquaculture industry. This species alone accounts for 95% of caviar and sturgeon meat production (Ethier, 2014), and generates an annual revenue of over 200 million dollars in exports for the western region of the United States (Carocci et al., 2004). These aquaculture practices provide substantial returns to producers, generate local employment opportunities, and allow for conservation of wild populations by offering a sustainable alternative to wild-caught fish. Continued expansion of the industry, however, is limited partially by infectious disease.
Acipenserid herpesvirus 2 (AciHV-2, also known as White sturgeon herpesvirus 2 or WSHV-2) is a large double-stranded DNA virus in the family Alloherpesviridae reported to cause epidermal ulceration, lethargy, inappetence, and erratic swimming (Watson et al., 1995; Lepa and Siwicki, 2012) with up to 50% mortality during natural outbreaks (Hanson et al., 2006; Goodwin, 2012; Mugetti et al., 2020). Streptococcus iniae (S. iniae), a gram-positive, opportunistically zoonotic bacterium that can cause severe myositis and up to 50% mortality during natural outbreaks, is another important pathogen of cultured white sturgeon (Nguyen et al., 2020; Pierezan et al., 2020). In the last decade, co-infections of AciHV-2 and S. iniae have been reported in cultured white sturgeon in California, resulting in severe presentations of piscine streptococcosis (Soto et al., 2017). This emerging presentation is particularly intriguing as S. iniae infections are typically associated with stress events when causing severe disease in white sturgeon (Nguyen et al., 2020; Pierezan et al., 2020), suggesting that immunomodulation is needed for the bacteria to behave with increased pathogenicity.
This herpesvirus-host-bacteria interaction phenomenon has been described in humans, as it pertains to pediatric Invasive Group A Streptococcal infections (IGASI), which have been increasing in prevalence over the last two decades worldwide (Frère et al., 2016; Suárez-Arrabal et al., 2019). Presentations are varied but serious and include necrotizing fasciitis (Wilson et al., 1995; Sturgeon et al., 2015), endocarditis (Laskey et al., 2000), toxic shock syndrome (Strom et al., 2017), and cellulitis (Oyake et al., 2000). These infections may cause long-term morbidity requiring extended hospitalizations and have a mortality rate of approximately 4% (Laupland et al., 2000; Strom et al., 2017). A known risk factor for a worse prognosis and more severe clinical presentation is a preceding varicella zoster infection, caused by Human herpesvirus 3 (also known as Varicella-Zoster Virus - VZV), which has been detected in 15-30% of IGASI cases in children and increases the risk of acquiring IGASI by 58 fold (Laupland et al., 2000; Sturgeon et al., 2015; Frère et al., 2016). Canada, one of the few countries to enact a universal VZV vaccination program, reported a decrease in IGASI severity, but no significant impact on the annual mean rate or overall mortality rate (Frère et al., 2016). Health disparities and disinformation are factors to consider in the success of vaccine programs (Nicoli and Appay, 2017) and there are countries where VZV vaccines are not available at this time.
While a decrease in humoral immunity of the host caused by VZV has been hypothesized as a potential important factor in IGASI (Laupland et al., 2000), the mechanisms underlying the association between VZV infection and IGASI have not been elucidated. Similarly, while AciHV-2 has been detected in various cases throughout the years, little is known about this virus’ pathogenesis and no studies have investigated its potential role in these reported co-infections in white sturgeon.
A particularity about herpesviruses is that they have been described to have a myriad of mechanisms to modulate the host’s immune response in order to establish and maintain latency (Speck and Ganem, 2010; Cohen, 2020). In latency, a reversible state achieved after the active outbreak, the full genome of the virus is maintained in the host cell with limited gene expression and no production of viral particles (Speck and Ganem, 2010). Depending on the viral species, herpesviral DNA is maintained as a circular episome (free in the nucleus or tethered to a chromosome) or integrated into the cellular chromosomes near the telomeric junction (Cohen, 2020). Even though the switch to latency severely decreases gene expression, part of the strategy of long-term persistence in the host is to focus efforts on protection from the immune system by immunomodulation, which can be initiated during the active lytic cycle of the virus or throughout the transition to latency. Latency has been extensively studied in mammalian herpesviruses, particularly in those affecting humans (Jarvis and Nelson, 2007; Speck and Ganem, 2010; Cohen, 2020), and continues to be a topic of active research, but little is known about the Alloherpesviridae family members including AciHV-2 (Stingley et al., 2003; Eide et al., 2011; Reed et al., 2014). As a member of the Herpesvirales order, and based on research done thus far involving other important alloherpesviruses such as Cyprinid Herpesvirus 3 (CyHV-3) (Eide et al., 2011; Reed et al., 2014), it is suspected that AciHV-2 has the potential for latency establishment. While this process may not cause direct mortality or clinical signs, it can have detrimental effects when it comes to the host’s ability to clear other pathogens of interest if immunomodulation is indeed occurring. This may be the case in both pediatric IGASI and the reported white sturgeon AciHV-2/S. iniae co-infections, where the transcriptional profiles of the herpesviruses may be affecting humoral immunity establishment against the bacteria as well as other unrelated pathways that play a role in protection against streptococcosis.
These natural co-infections in the white sturgeon represent an outstanding opportunity to develop an animal model to study the complex interactions that may be taking place between the herpesvirus, the immune system of the host, and the bacteria (Miller and Neely, 2005). This model would be particularly relevant because previous studies have proposed S. iniae as a good candidate for use in animal models in translational research regarding S. pyogenes, S. agalactiae, and S. pneumoniae due to causing similar clinical presentations and having several homologous virulence factors (Miller and Neely, 2005; Baiano and Barnes, 2009). Finally, the study of this phenomenon using the white sturgeon model simultaneously supports a vital aquaculture industry in the United States.
Therefore, this study aimed to assess the hypothesis that a recent, potentially latent, AciHV-2 infection in white sturgeon increases mortality caused by S. iniae and affects the host immune response when compared to single pathogen infections.
2 Materials and methods
2.1 Viral inoculum preparation
White Sturgeon Skin (WSSK-1) cells (Hedrick et al., 1991) were seeded in T75 flasks at approximately 90% confluency in Minimum Essential Media (MEM; Corning Inc, Corning, NY) supplemented with 7.5% Fetal Bovine Serum (FBS; Genesee, El Cajón, CA), L-glutamine (Gibco, Grand Island, NY), and Penicillin/Streptomycin (Gibco, Grand Island, NY) at 20°C. After 24 hours, these cells were used to propagate AciHV-2 isolate UCD3-30 (Watson et al., 1995) passage number 8 from stock. Ten days post-inoculation, the infected cell cultures were collected and centrifuged at 1900 g for 10 min. A 1 mL aliquot of the supernatant was used to determine the median tissue culture infectious dose of each viral inoculum using the Reed-Muench method (Lei et al., 2021). The remaining supernatant was used in the challenges below.
2.2 Bacterial inoculum preparation
Streptococcus iniae WS-10A (Pierezan et al., 2020) was revived from frozen stock on trypticase soy agar supplemented with 5% sheep’s blood (SBA; University of California, Biological Media Services) grown at 30°C for 48 hours. A 0.5 McFarland standard was generated in sterile phosphate-buffered saline (PBS) corresponding to an optical density measurement of 0.15 at 600 nm, read on a UV/Vis photometer (BioPhotometerPlus, Eppendorf AG). The McFarland was diluted 1:10 in sterile PBS.
2.3 Fish screening and acclimation
White sturgeon fry (n = 1000; average weight ~ 1 g) were obtained from a commercial producer and grown in a 259-gallon circular tank for four months with a flow-through system supplied with well water (18 – 20°C) and supplemental aeration maintaining 8 - 9 mg/L of dissolved oxygen. Fish were fed 4% of their body weight daily of a combination of ground and 1 mm salmon sink pellet feed (Skretting: a Nutreco company, Stavanger, Norway) via an automatic feeder. Water temperature was monitored daily and dissolved oxygen was measured weekly.
An arbitrary sample of 12 white sturgeon fry was selected for general health assessment and infectious agent screening. Briefly, fish were euthanized with 1000 mg/L (ppm) buffered tricaine methanesulfonate (MS-222, 1:1 sodium bicarbonate; Syndel USA, Ferndale, WA) and assessed grossly for any external lesions. Skin scrapes and gill clips were evaluated microscopically for evidence of external parasites. Coelomic swabs were plated on tryptic soy agar supplemented with 5% sheep blood (Biological Media Service, University of California-Davis, CA) and incubated at 20°C for 7 days for bacterial assessment. Whole bodies were 1) pooled, 2) diluted 1:5 in MEM supplemented with 2% FBS, L-glutamine, and Penicillin/Streptomycin, 3) processed using a Stomacher 80 Laboratory Blender (Tekmar Company, Cincinnati, OH), 4) brought to a 1:50 final dilution in an antibiotic mixture (FBS, Gentamycin (10 mg/mL; Gemini Bio Products, West Sacramento, CA), Fungizone (amphotericin B 10 mg/mL; Sigma, St. Louis, MO) and HEPES (Mediatech Inc, Manassas, VA), 5) incubated overnight at 10°C, 6) and plated in duplicate onto WSSK-1 and White Sturgeon Spleen (WSS-2) (Hedrick et al., 1991) cells seeded 24 hours in advance at 90% confluency in 12-well plates with MEM supplemented with 2% FBS, L-glutamine, HEPES, and Penicillin/Streptomycin. In addition, the tissue pellet from the virology process was used to screen for AciHV-2 using a recently developed quantitative PCR (Quijano Carde et al., 2024). No external parasites, pathogenic bacteria, or viruses were identified during this screening process. All protocols and procedures using these fish were ethically reviewed and approved by the University of California Institutional Animal Care and Use Committee.
2.4 Laboratory controlled challenges
For the first challenge, henceforth referred to as the “pilot challenge”, white sturgeon fingerlings (n = 144; average weight = 10 g) were used. A subgroup of 96 fish was exposed to a 5.1 x 102 Median Tissue Culture Infectious Dose (TCID50)/mL bath (Lei et al., 2021) of AciHV-2 for 1 hour. The remaining 48 fish were exposed to the same volume of sterile MEM supplemented with 2% FBS, L-glutamine, and Penicillin/Streptomycin for 1 hour and served as negative controls. For purposes of reproducibility, the fish were exposed in 5-gallon tanks. Each tank had 3 L of water, 3 mL of the virus stock or sterile cell culture media, and 12 fish. The virus aliquot used had 5.1x105 TCID50/mL. Fish were maintained at 18-20°C in 5-gallon circular tanks, fed 1% of their body weight of 1 mm-salmon sink pellet feed once daily, and monitored closely. Mortality was recorded for 80 days and a pectoral fin clip was collected from every mortality (n = 37) to quantify AciHV-2 viral load via qPCR (Quijano Carde et al., 2024). Survivors of each group were then arbitrarily sorted into duplicate 35-gallon rectangular flow-through tanks (23 fish/group) to generate four treatment groups (Negative control, AciHV-2 infected, S. iniae infected, and AciHV-2/S. iniae co-infected). Fish were allowed to acclimate to their new environment for four days while the temperature was raised 1°C per day until reaching 22-24°C via commercial submersible water heaters (Aqueon, Franklin, WI). Fish in the S. iniae infected, and AciHV-2/S. iniae co-infected groups were subjected to an S. iniae challenge following published protocols using a 109 CFU of S. iniae per mL suspension (0.1 mL per fish in the epaxial musculature for a final dose of 108 CFU per fish) under anesthesia (100 ppm buffered MS-222) after a netting stress event (two 30 sec periods out of the water within a net with 30 sec in the water within the net in between) (Nguyen et al., 2020). Fish in the Negative control, and AciHV-2 infected groups were treated similarly but were injected with 0.1 mL of PBS intramuscularly in the epaxial musculature. During the anesthetic event, a pectoral fin clip sample was collected from each fish for assessment of AciHV-2 pre-bacterial challenge via qPCR (Quijano Carde et al., 2024). Mortality was monitored for 21 days and the cumulative percent mortality (CPM) per treatment was calculated. Pectoral fin clips and spleen samples were collected from each mortality and stored at -80°C for molecular testing via AciHV-2 (Quijano Carde et al., 2024) and S. iniae (López-Porras et al., 2019) qPCR, respectively. All survivors were euthanized with 1000 ppm of buffered MS-222. Pectoral fin clips were collected from all survivors for testing of AciHV-2 via qPCR (Quijano Carde et al., 2024).
For the second challenge, henceforth referred to as the “large-scale challenge”, white sturgeon fingerlings (n = 720; average weight = 12 g) were used. A subgroup of 360 fish was exposed to a 5.96 x 102 TCID50/mL bath (Lei et al., 2021) of AciHV-2 for 1 hour. The remaining 360 fish were exposed to the same volume of sterile MEM supplemented with 2% FBS, L-glutamine, and Penicillin/Streptomycin for 1 hour and served as negative controls. For purposes of reproducibility, the fish were exposed in 35-gallon tanks. Each tank had 26 L of water, 77.5 mL of the virus stock or sterile cell culture media, and 180 fish. The virus aliquot used had 2x105 TCID50/mL. Fish were then relocated and maintained at 18-20°C in 259-gallon circular tanks, fed 1% of their body weight of 1 mm-salmon sink pellet feed once daily, and monitored closely. Mortality was recorded for 44 days and a pectoral fin clip was collected from every mortality (n = 180) to quantify AciHV-2 viral load via qPCR (Quijano Carde et al., 2024). Survivors of each group were then arbitrarily sorted into triplicate 35-gallon rectangular flow-through tanks (20 fish/tank) to generate four treatment groups (Negative control, AciHV-2 infected, S. iniae infected, and AciHV-2/S. iniae co-infected; 60 fish/group). Fish were allowed to acclimate to their new environment for four days while the temperature was raised 1°C per day until reaching 22-24°C via commercial submersible water heaters (Aqueon, Franklin, WI). Fish in the S. iniae infected, and AciHV-2/S. iniae co-infected groups were subjected to an S. iniae challenge following published protocols using a 107 CFU of S. iniae per mL suspension (0.1 mL per fish in the epaxial musculature for a final dose of 106 CFU per fish) under anesthesia (100 ppm buffered MS-222) after a netting stress event (two 30 sec periods out of the water within a net with 30 sec in the water within the net in between) (Nguyen et al., 2020). This bacterial concentration was lower than the one used in the pilot challenge discussed above in order to reduce mortalities and thus have enough fish for immune response assessment sampling. Fish in the Negative control, and AciHV-2 infected groups were treated similarly, but were injected with 0.1 mL of PBS intramuscularly in the epaxial musculature. Mortality was monitored for 28 days and the cumulative percent mortality (CPM) per treatment was calculated. Pectoral fin clips and spleen samples were collected from each mortality and stored at -80°C for molecular testing via AciHV-2 (Quijano Carde et al., 2024) and S. iniae (López-Porras et al., 2019) qPCR, respectively. Two fish per tank were euthanized with 1000 ppm buffered MS-222 on day 15 post-injection. The spleen of each euthanized fish was collected aseptically and incubated individually in 0.5 mL of RNAlater (Thermofisher Scientific, Waltham, MA) overnight at 4°C before being transferred to -80°C for storage until reverse-transcription qPCR (RT-qPCR) analysis. In addition, a pectoral fin clip and spleen sample were collected from each euthanized fish for molecular testing via AciHV-2 (Quijano Carde et al., 2024) and S. iniae (López-Porras et al., 2019) qPCR, respectively. At least five moribund individuals per group were euthanized throughout the study and submitted for histological analysis in 10% neutral-buffered formalin (pH 7.2) after fixing for a minimum of 24 hours. Representative tissues (skin, skeletal muscle, gastrointestinal tract, oral cavity, brain, eyes, heart, gills, liver, kidney, spleen, and reproductive tract) were processed routinely, embedded in paraffin, sectioned at 4 μm, and stained with hematoxylin and eosin (H&E) or Gram stain. Slides were scored based on four categories: “degree of erosion/ulceration”, “distribution of erosion/ulceration”, “percentage of section affected”, and “muscle necrosis”. At 28 days post-injection, all survivors were euthanized with 1000 ppm of buffered MS-222. Ten euthanized individuals per group of this time point were used for the collection of blood, fin clip samples, spleen samples, and swabs as described in the remainder of this paragraph. At least 0.8 mL of blood was collected individually in microcentrifuge tubes (with no anticoagulant) from the caudal vein. Clotted blood was then centrifuged at 10,000 g for 5 min and the serum was stored individually at -20°C until enzyme-linked immunosorbent assay (ELISA) and Western Blot analysis. Re-isolation of bacteria from the posterior kidney and brain swabs was performed in SBA. Pectoral fin clips and spleen samples were collected for molecular testing via AciHV-2 (Quijano Carde et al., 2024) and S. iniae (López-Porras et al., 2019) qPCR, respectively. A spleen sample was also collected aseptically and immersed in 0.5 mL of RNAlater (Thermofisher Scientific, Waltham, MA) overnight at 4°C before being transferred to -80°C for storage until reverse-transcription qPCR (RT-qPCR) analysis. A graphical representation of the timeline of the large-scale challenge can be found in Supplementary Figure 1.
2.5 Pathogen quantification and gene expression assay
Genomic DNA from fin and spleen samples was isolated using the DNeasy Blood & Tissue kit (QIAGEN, Hilden, Germany) with the following modifications: tissues were incubated with ATL buffer and proteinase K at 56°C for 1 hour and samples were incubated with AL buffer at 56°C for 10 minutes. Isolated DNA was stored at -20°C until analysis using the recently developed AciHV-2 qPCR (Quijano Carde et al., 2024) and the previously published S. iniae qPCR (López-Porras et al., 2019). Positive controls were included in each assay, consisting of purified genomic DNA extracted from the original inoculums described above in sections 2.1 and 2.2 (107 copies/reaction). The DNA concentration and quality of each sample and control were assessed spectrophotometrically (Nanodrop; Thermofisher Scientific, Waltham, MA) and all samples and controls were diluted in sterile water to 50 ng/μL before being used in the qPCR reactions, for a total of 250 ng of DNA per 12 μL reaction. All samples and controls were run in triplicate while using the TaqMan Environmental Master Mix on the QuantStudio3 Real-Time PCR System (Thermofisher Scientific, Waltham, MA).
Total RNA from spleen samples, as well as genomic DNA, were isolated using the AllPrep DNA and RNA Mini Kit (QIAGEN, Hilden, Germany). The DNA and RNA concentration and quality of each sample were assessed spectrophotometrically (Nanodrop). The DNA samples were used for qPCR as described above. The RNA samples were reversed transcribed to cDNA using the High-capacity RNA-to-cDNA kit (Thermofisher Scientific, Waltham, MA). The cDNA samples were diluted 1:2 in sterile water and used to quantify transcript abundance of cytokines, acute-phase proteins, and other molecules involved in various immune pathways from the innate and adaptive responses (Serum Amyloid A - saa, Major Histocompatibility Complex Class II - mhcII, Interleukin 17 - il17, Interferon Regulatory Factor 8 - irf8, and Tumor Necrosis Factor alpha - tnfα) via previously published reverse-transcription qPCR (RT-qPCR) (Soto et al., 2021; Soto et al., 2022) protocols. The expression of the Elongation Factor and Beta Actin genes was used to normalize the gene expression data and all samples were run in duplicate. The primer sequences used in this study can be found in Supplementary Table 1.
2.6 Detection of serum anti-S. iniae IgM in challenge survivors
An indirect enzyme-linked immunosorbent assay (ELISA) was performed following previously published protocols (Heckman et al., 2022) with modifications. Briefly, S. iniae (isolate WS-10A) was grown from stock on SBA at 28°C for 48 hours. The bacteria was resuspended in coating buffer (1% poly-L-lysine in carbonate-bicarbonate) to an optical density (OD) of 0.245 at 600 nm. Immulon 2HB Flat Bottom Microtiter 96-well plates (Thermofisher Scientific, Waltham, MA) were coated with 100 μL of bacterial suspension per well and incubated overnight at 4°C.
The plates were washed three times with low-salt wash buffer (LSWB; 0.02 M Trizma base, 0.38 M NaCl, 0.05% Tween-20, pH 7.3) and a 250 μL suspension of blocking buffer (5% skim milk powder in double-distilled water) added to each well before incubation at room temperature for 3 hours. Following incubation, the plates were washed again three times with LSWB. The white sturgeon serum samples collected at the end of the challenge were diluted 1:200 in LSWB containing 1% bovine serum albumin (BSA; Sigma) and 100 μL of the diluted serum or sterile PBS was added to respective plate wells in duplicate. Plates were then incubated overnight at 4°C.
The plates were washed five times with high-salt wash buffer (HSWB; 0.02 M Trizma base, 0.5 M NaCl, 0.01% Tween-20, pH 7.7), including a 5 min soak on the last wash. Mouse anti-sturgeon IgM monoclonal antibodies (Aquatic Diagnostics Ltd, UK) were diluted 1:75 in PBS and 100 μL added to each well. The plates were incubated at room temperature for 1 hour before washing five times with HSWB, including a 5 min soak. Goat anti-mouse IgG with conjugated horseradish peroxidase (Sigma-Aldrich, St. Louis, MO) was diluted 1:3000 in LSWB with 1% BSA and 100 μL was added to each well. The plates were incubated at room temperature for 1 hour and then washed with HSWB five times, including a 5 min soak on the last wash. Each well received 100 μL of substrate solution (ABTS 1-component microwell peroxidase substrate kit; SeraCare Life Sciences, Milford, MA) and the plates were incubated at room temperature for 30 min. The reaction was terminated by adding 100 μL of stop solution (0.01% Sodium Dodecyl Sulfate in distilled water) to each well. Absorbance at 410 nm was measured using a Cytation 5 Cell Imaging Multimode Reader (BioTek, Winooski, VT) and standardized against the PBS controls.
2.7 Detection of serum serotransferrin in challenge survivors
Serum serotransferrin (STF-2) levels were determined following previously published protocols (Soto et al., 2022). Briefly, the protein concentration in each serum sample was determined using the Pierce BCA Protein Assay Kit (Thermofisher Scientific, Waltham, MA). An aliquot of 25 μg of total protein per sample was denatured in Laemmli buffer (Bio-Rad Laboratories, Hercules, CA) for 5 min at 95°C. Samples were loaded into Mini-PROTEAN® TGX Stain-Free™ 4–15% gels (Bio-Rad Laboratories, Hercules, CA) and separated using the Mini-PROTEAN® Tetra Cell gel apparatus (Bio-Rad Laboratories, Hercules, CA) for 50 min at 150 v. Total protein per sample was imaged using the stain-free application on the ChemiDoc MP imager (Bio-Rad Laboratories, Hercules, CA). Gels were then activated and transferred onto 0.22 μm nitrocellulose (STF) membranes (Azure Biosystems, Dublin, CA) using the Trans-Blot® Turbo™ Mini-size Transfer Stacks and the Trans-Blot® Turbo™ Transfer System (Bio-Rad Laboratories, Hercules, CA) for 3 min. Total protein per sample was imaged again as described above. Membranes were immediately transferred to the blocking buffer of 5% non-fat dry milk in PBS with Tween (PBSTW; 1.37 M NaCl, 27 mM KCl, 100 mM Na2HPO4, 18 mM KH2PO4, pH 7.4, 0.1% (w/v) Tween 20) and incubated with gentle agitation for 2 h at room temperature. Each blot was then incubated with the following primary/secondary antibody combination: 1) chicken anti-sturgeon serotransferrin (1:500, Somru BioScience Inc., Charlottetown, PE, CA) and 2) donkey anti-chicken IgY++(IgG) (H + L) (1:10,000 Jackson ImmunoResearch Laboratories, Inc.). The primary antibody was diluted in its blocking buffer (5% non-fat dry milk in PBS with Tween) and incubated for 18 hours at 4°C with gentle agitation, while the secondary antibody was diluted in its blocking buffer (5% non-fat dry milk in PBS with Tween) and incubated for 1 h at room temperature with gentle agitation. All protein bands were visualized using Clarity™ Western ECL Substrate Chemiluminescent Detection Reagent (Bio-Rad Laboratories, Hercules, CA) prior to image acquisition and visualized using a ChemiDoc MP Imaging System (Bio-Rad Laboratories, Hercules, CA). Image analysis was performed using Image Lab™ Software (Bio-Rad Laboratories, Hercules, CA). The data is presented as the ratio of protein of interest expressed as a “fold difference” to the loading control, normalized to total protein levels.
2.8 Statistical analysis
The CPM was assessed using Kaplan-Meier survival analysis with a Mantel-Cox test. All data, except survival data, was assessed for normality with a D’Agostino-Pearson omnibus K2 test. Differences between groups for the viral and bacterial loads were determined using unpaired t-tests and Mann-Whitney tests as appropriate for the desired comparison. The significance of differences in relative gene expression levels between treatments was calculated using a one-way ANOVA or a Kruskal-Wallis test as appropriate followed by a Holm-Sídák’s multiple comparison test (with a single pooled variance) or a Dunn’s multiple comparison test, respectively. The IgM quantification between groups was assessed with a one-way ANOVA and a Sídák’s multiple comparison test (with a single pooled variance). The STF-2 quantification between groups was assessed with a Kruskal-Wallis test and a Dunn’s multiple comparison test. The histopathologist was not blinded as to treatment group identity and the histopathological scores were compared via one-way ANOVA and a Sídak’s multiple comparison test (with a single pooled variance). All results were considered significant at p-values ≤ 0.05.
3 Results
3.1 Challenge
During the AciHV-2 portion of both challenges, the sturgeon in the AciHV-2 infected group began showing clinical signs seven days post-immersion. Clinical signs included erythema (Figure 1A), lethargy, splenomegaly (Figure 1B), decreased feed intake, buoyancy abnormalities, epidermal ulcerations (Figure 1C), and acute mortality. In addition, it was common to find moderate to severe colonization of the mouth and opercular cavities by oomycetes (Figure 1D) in fish displaying severe clinical signs. The experimental group experienced a decrease in survival of 40% (pilot) and 50% (large-scale) compared to the negative control, with mortalities starting 18 days post-infection (pilot challenge, Figure 2A) and eight days post-infection (large-scale challenge, Figure 3A). Survivors had no overt clinical signs 52 days (pilot challenge) and 40 days (large-scale challenge) post-immersion, with only sporadic mortalities observed in the following days before the bacterial co-infection (Figures 2A, 3A, respectively). When assessing viral load in the mortalities, 89% in the pilot challenge (Figure 2C) and 94% in the large-scale challenge (Figure 3C) tested positive via qPCR with a mean viral load of 106 copies in the pilot challenge (Figure 2B) and 105.4 copies (large-scale challenge, Figure 3B) per μg of total DNA. Two fish in the negative control group died during the large-scale challenge and they both tested negative for AciHV-2 via qPCR (Figure 3C). Finally, a screening of AciHV-2 via qPCR was performed in the pilot challenge prior to the bacterial infection and only 0.06% of fish tested positive for AciHV-2 (Figure 2D).
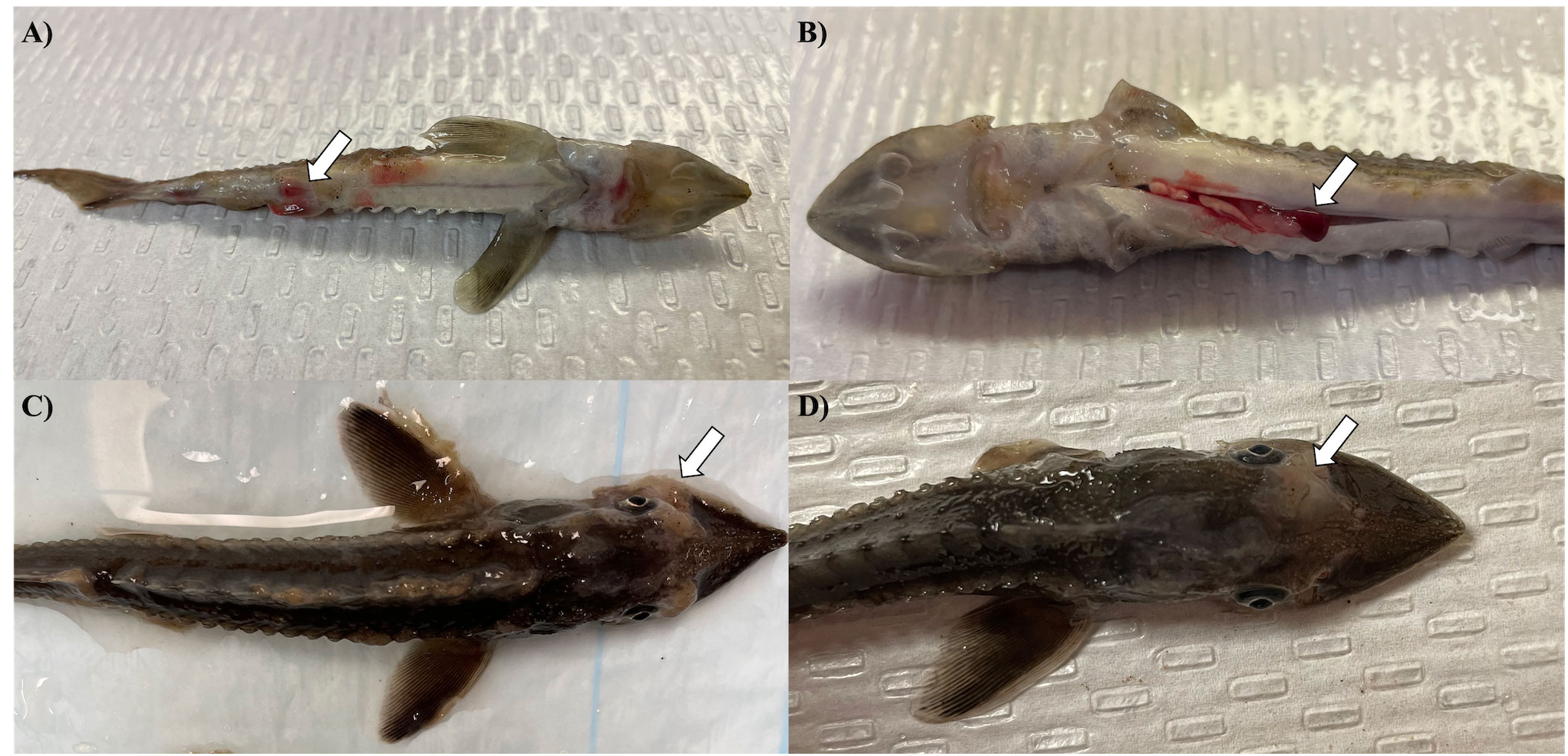
Figure 1 Clinical signs in white sturgeon during AciHV-2 challenge. White sturgeon fingerlings were exposed to a 5.1x102 TCID50/mL (pilot challenge) or a 5.96x102 TCID50/mL (large-scale challenge) bath for 1 hour. Morbidity and mortality were monitored for 44 (large-scale challenge) to 80 (pilot challenge) days after immersion. These are representative images of clinical signs and gross changes observed in both challenges during the AciHV-2 outbreak portion of the experiments. (A) Erythema surrounding pelvic fins in a fresh mortality. (B) Splenomegaly in a fresh mortality. Pectoral fins had been clipped for diagnostic purposes post-mortem. (C) oomycetes colonization in an euthanized fish. (D) Epidermal ulceration medial to the left eye in a fresh mortality. The white arrows point towards the lesions described.
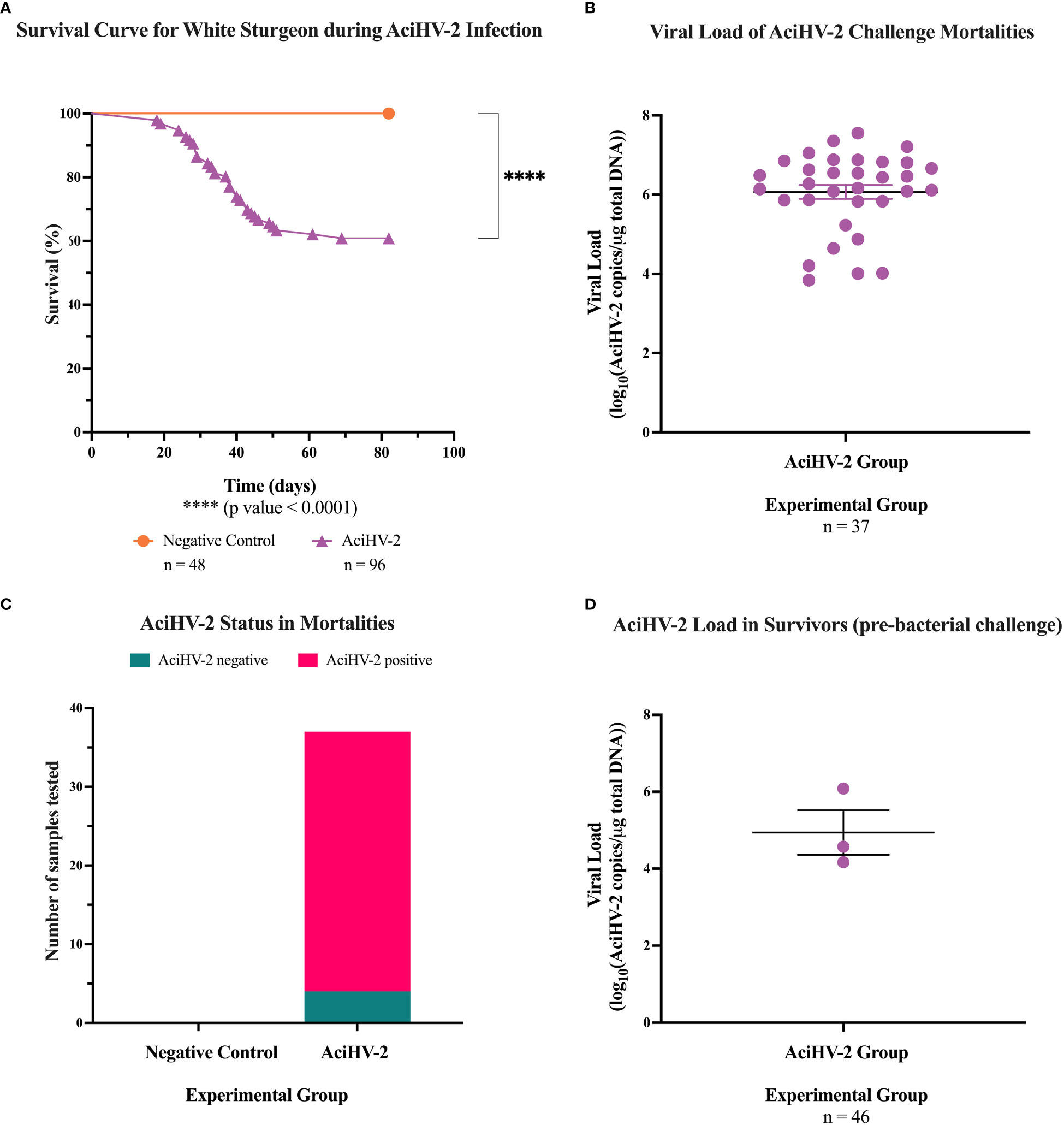
Figure 2 Acipenserid Herpesvirus 2 (AciHV-2) challenge during pilot challenge. A 1- hour static immersion challenge with 5.1x102 TCID50/mL) of Acipenserid Herpesvirus 2 (AciHV-2) was performed (n=96). A negative control group was treated similarly but sterile cell culture media was used instead of virus suspension (n=48). Mortality was monitored for 80 days and mortalities were tested for AciHV-2 via qPCR of pectoral fin DNA. Survivors were tested for AciHV-2 via quantitative polymerase chain reaction (qPCR) of DNA extracted from the pectoral fin of each fish. (A) The solid shapes represent the mean cumulative mortality at each day. The different experimental groups are color-coded. A statistically significant reduction in survival is seen in the AciHV-2 exposed group when compared with the negative control group. (B) The solid dots represent the mean viral load of sampled mortalities, with the standard error of the mean plotted. Four mortalities in the AciHV-2 group had undetermined Ct values and are not displayed in this graph. The mean viral load among mortalities was 106 copies/ug total DNA. (C) The bars represent the number of samples tested for AciHV-2 via qPCR, color-coded by qPCR result. This shows that 89% of the mortalities in the AciHV-2 group tested positive for AciHV-2 via qPCR. (D) The solid dots represent the mean viral load of sampled survivors, with the standard error of the mean plotted. Only three individuals tested positive with a mean viral load of 104.9 copies/ug total DNA.
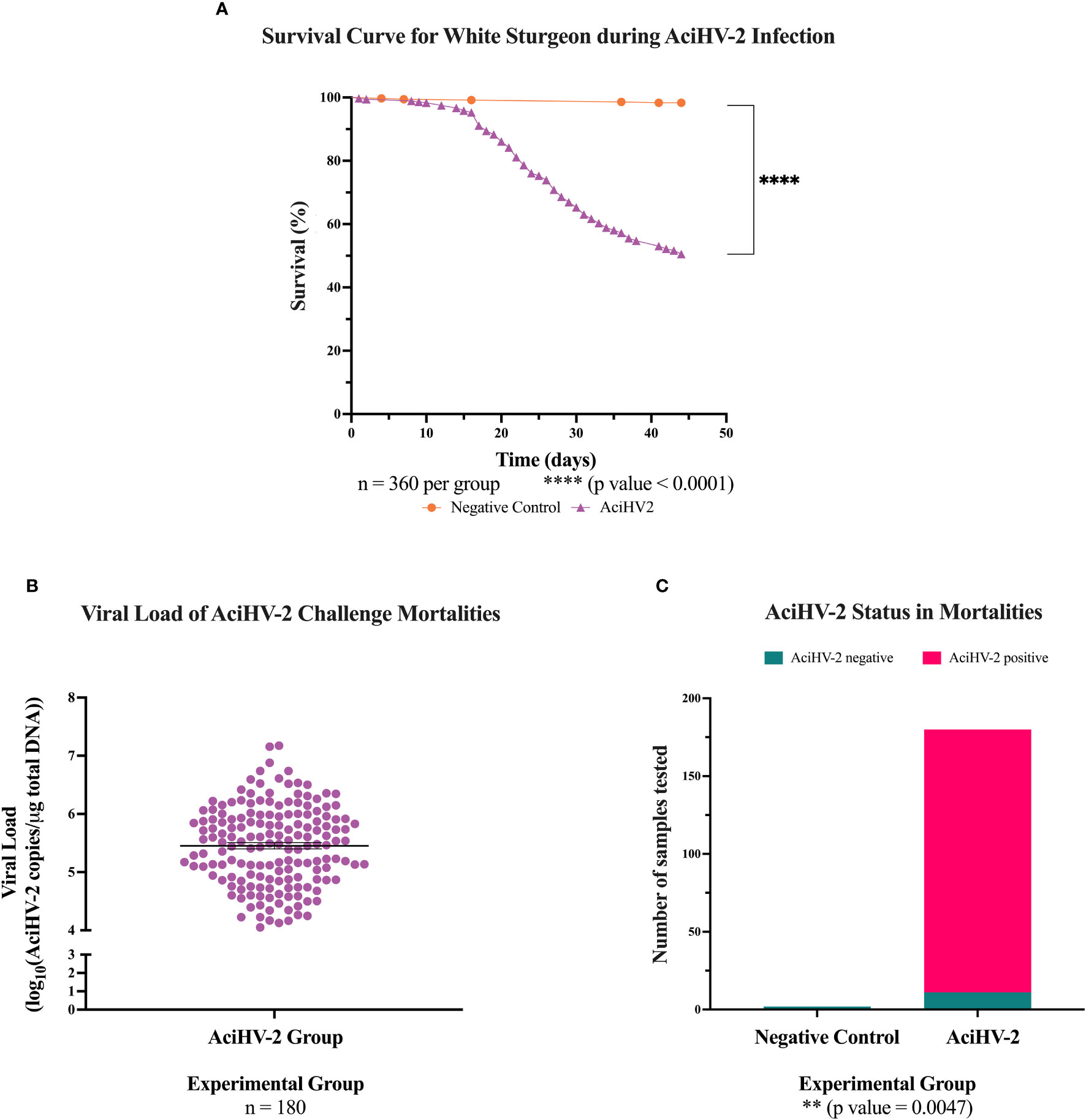
Figure 3 Acipenserid Herpesvirus 2 (AciHV-2) challenge during large-scale challenge. An immersion challenge model was performed by exposing white sturgeon fingerlings to a 5.96x102 TCID50/mL bath or a sterile cell culture media bath for 1 hour. Mortality was monitored for 44 days after immersion and mortalities tested for AciHV-2 via quantitative polymerase chain reaction (qPCR). Survivors were weighed at the end of the challenge. (A) The solid shapes represent the mean cumulative mortality at each day. The different experimental groups are color-coded. A statistically significant reduction in survival is seen in the AciHV-2 exposed group when compared with the negative control group. (B) The solid dots represent the mean viral load of sampled mortalities, with the standard error of the mean plotted. Both mortalities in the negative control group and 11 mortalities in the AciHV-2 group had undetermined Ct values and are not displayed in this graph. The mean viral load among mortalities was 105.4 copies/ug total DNA. (C) The bars represent the number of samples tested for AciHV-2 via qPCR, color-coded by qPCR result. This shows that 94% of the mortalities in the AciHV-2 group tested positive for AciHV-2 via qPCR, while none of the mortalities in the negative control group tested positive for AciHV-2 via qPCR. There is a strong association between an AciHV-2 positive status and the experimental group.
During the co-infection portion, the sturgeon infected with S. iniae began developing clinical signs 24 hours post-injection in both the pilot and the large-scale challenges (Figure 4A). Clinical signs included lethargy, inappetence or anorexia, injection site ulceration (Figures 4B–D), and acute mortality. In the pilot challenge, the co-infection group had a statistically significant decrease in survival of 41% compared to the S. iniae group and 36% compared to AciHV-2 group (Figure 5A). The trends in mortality in the large-scale challenge were consistent with the pilot challenge, with the co-infected group showing a decrease in survival of 7.8% compared to the S. iniae group and 9.1% compared to the AciHV-2 group, but this was only statistically significantly different when compared with the AciHV-2 group and not when compared to the S. iniae group (Figure 6A). In addition, mortalities started in the co-infection group 24 hours earlier than in the S. iniae group during both the pilot and large-scale challenges (Figures 5A, 6A, respectively). In terms of viral and bacterial loads of the challenge mortalities, there was no statistical difference in loads between groups (Figures 5B, C, 6B, C). During the pilot challenge, only four individuals tested positive for AciHV-2 and there was no statistical difference in viral load between groups (p-value = 0.5538, Figure 5D). During the large-scale challenge, all euthanized individuals for gene expression sampling tested negative for AciHV-2 and S. iniae via qPCR (n = 6 per group at 15 days post-bacterial infection and n = 10 per group at 28 days post-bacterial infection).
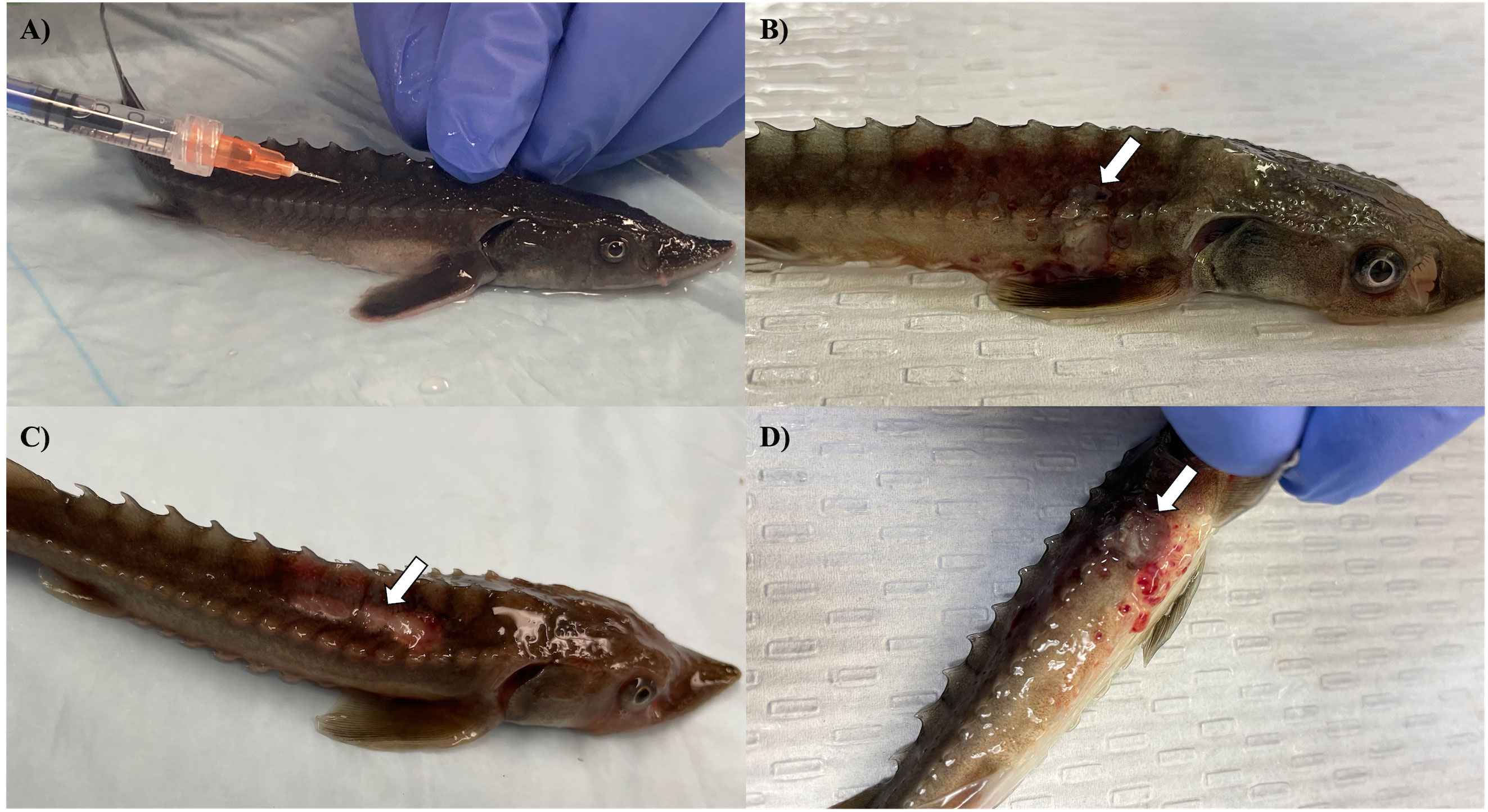
Figure 4 Clinical signs and gross changes in white sturgeon during AciHV-2 and S. iniae co-infection challenge. White sturgeon fingerlings were exposed to a 5.1x102 TCID50/mL (pilot challenge) or a 5.96x102 TCID50/mL (large-scale challenge) bath for 1 hour or an equivalent volume of sterile cell culture media. Survivors were then injected with 106 CFU S. iniae per fish or 0.1 mL of sterile Phosphate Buffered Saline. Clinical signs and mortality were monitored for 28 days. (A) Injection site for the S. iniae intramuscular challenge in an anesthetized white sturgeon fingerling. (B) Mild cutaneous ulceration at the injection site in a fresh mortality. (C) Moderate to severe ulceration at the injection site in a fresh mortality. (D) Moderate to severe ulceration at the injection site with subdermal hemorrhage. The white arrows point towards the lesions described.
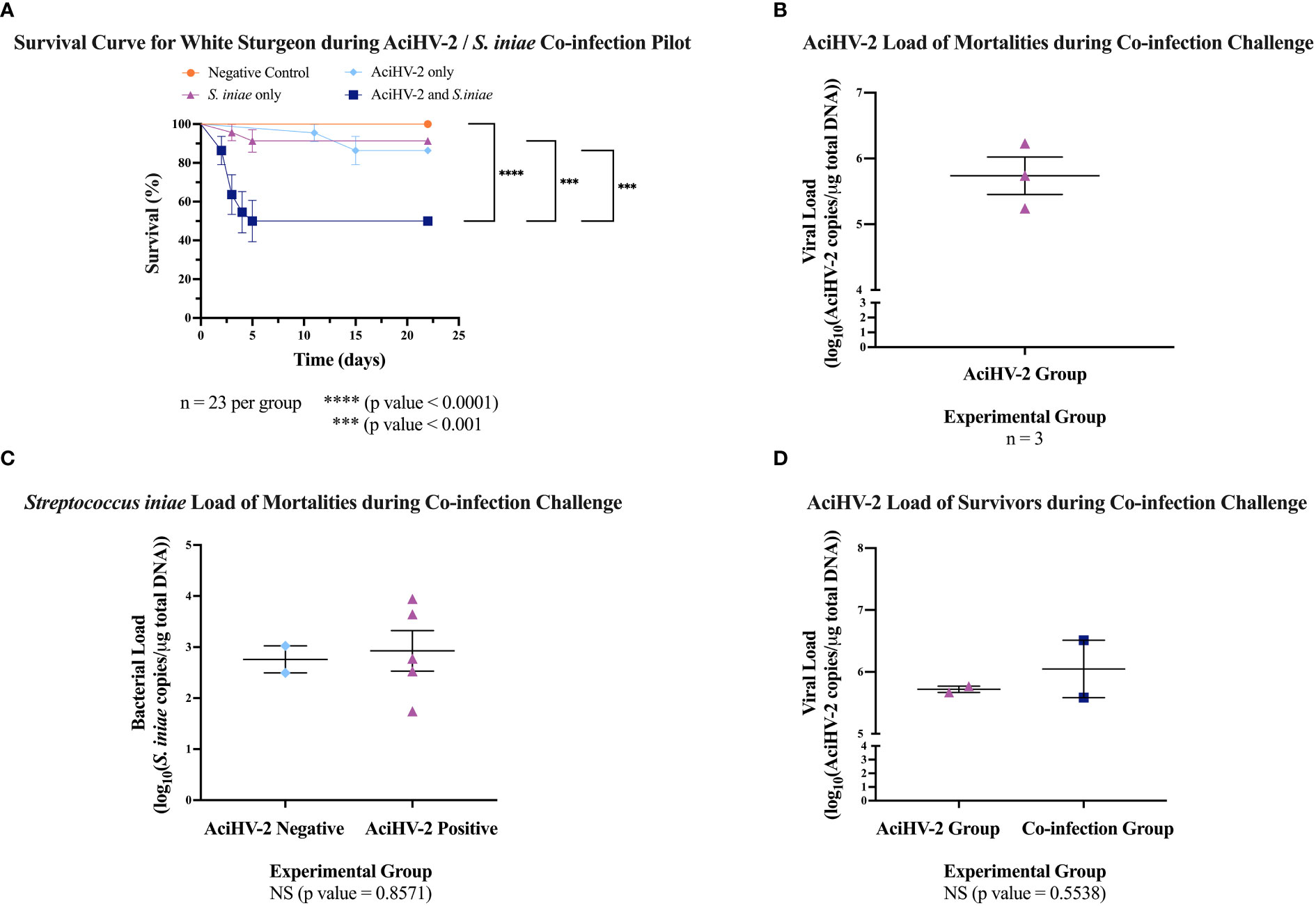
Figure 5 Acipenserid Herpesvirus 2 (AciHV-2) and Streptococcus iniae (S. iniae) co-infection pilot challenge. An immersion challenge model was performed by exposing white sturgeon fingerlings to a 5.1x102 TCID50/mL AciHV-2 bath or a sterile cell culture media bath for 1 hour. Survivors of this challenge at 80 days were re-sorted into groups of 23 fish per treatment. Fish received either 108 CFU of S. iniae or sterile Phosphate Buffered Saline in the epaxial musculature. Mortality was monitored for 21 days after injection and mortalities were tested for AciHV-2 and S. iniae via quantitative polymerase chain reaction (qPCR). (A) The solid shapes represent the mean cumulative mortality at each day, with standard error of the mean plotted. The different experimental groups are color-coded. The black stars indicate statistical significance between the bracketed groups and p values are stated below. Results reveal that the S. iniae group that was previously infected with AciHV-2 had a statistically significant decrease in survival of 41% compared to S. iniae alone and of 36% compared to AciHV-2 alone. (B) The solid dots represent the mean viral load of sampled mortalities, with the standard error of the mean plotted. Only the AciHV-2 group mortalities tested positive for AciHV-2 via qPCR. (C) The solid dots represent the mean bacterial load of sampled mortalities, with the standard error of the mean plotted. There was no statistical difference in bacterial loads between groups. This also shows that 100% and 45% of the mortalities in the S. iniae and in the co-infection groups, respectively, tested positive for S. iniae. There is a strong association between an S. iniae positive status and the experimental group (p value ≤ 0.0001). (D) The solid dots represent the mean viral load of sampled survivors, with the standard error of the mean plotted. Experimental groups are color-coded. There was no statistical difference in viral load between groups.
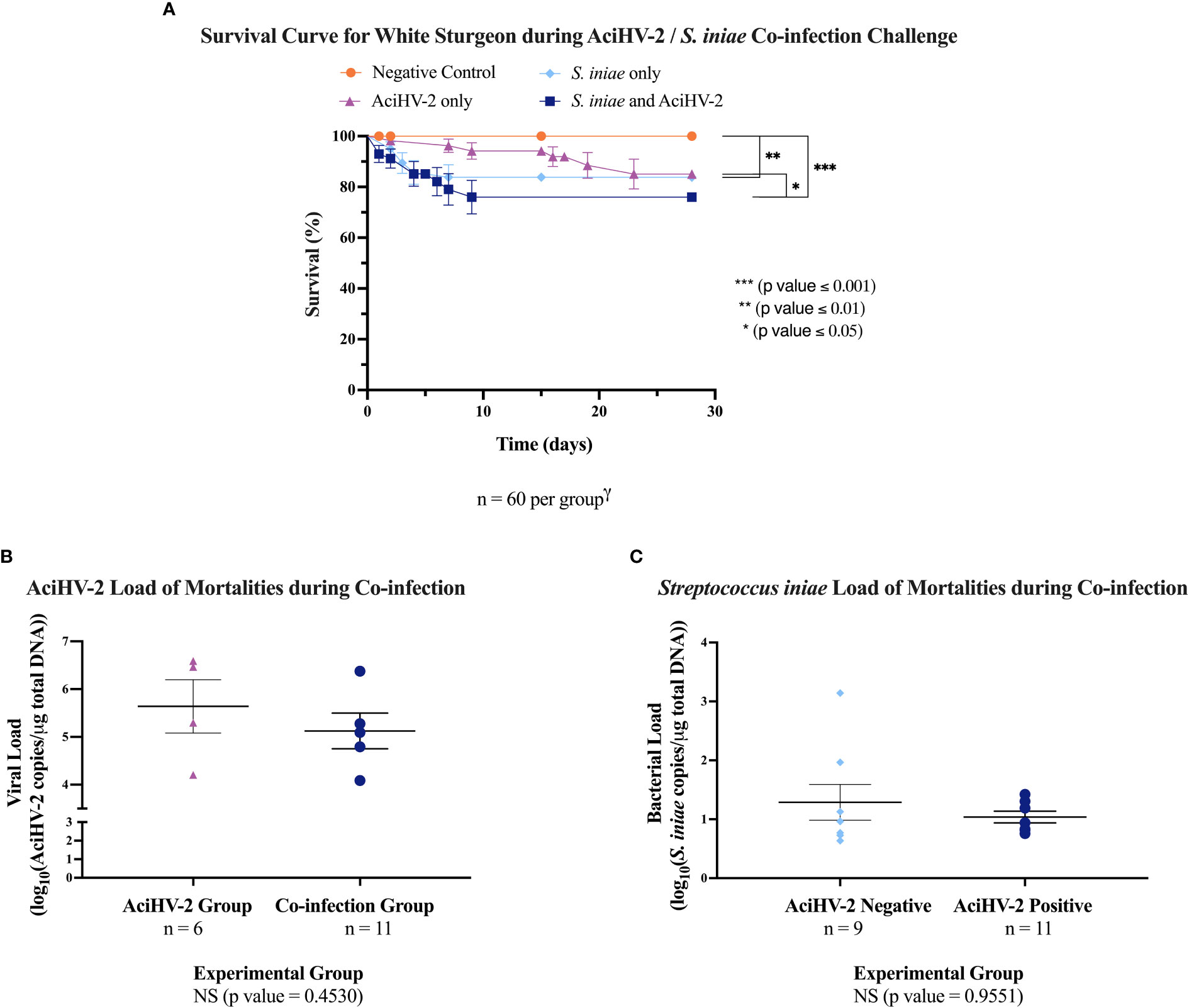
Figure 6 Acipenserid Herpesvirus 2 (AciHV-2) and Streptococcus iniae (S. iniae) co-infection large-scale challenge. An immersion challenge model was performed by exposing white sturgeon fingerlings to a 5.96x102 TCID50/mL AciHV-2 bath or a sterile cell culture media bath for 1 hour. Survivors of this challenge at 44 days were re-sorted into groups of 60 fish (triplicate tanks of 20 fish each) per treatment. Fish received either 106 CFU of S. iniae or sterile Phosphate Buffered Saline in the epaxial musculature. Mortality was monitored for 28 days after injection and mortalities tested for AciHV-2 and S. iniae via quantitative polymerase chain reaction (qPCR). (A) The solid shapes represent the mean cumulative mortality at each day, with standard error of the mean plotted. The different experimental groups are color coded. A statistically significant reduction in survival is seen in all infected groups when compared to the negative control, except for the AciHV-2 group. When comparing infected groups among themselves, the co-infection group has the lowest survival, showing a statistically significant reduction compared to the AciHV-2 group. (B) The solid dots represent the mean viral load of sampled mortalities, with the standard error of the mean plotted. There was no statistical difference in viral loads between groups. This also shows that 67% and 45% of the mortalities in the AciHV-2 and in the co-infection groups, respectively, tested positive for AciHV-2. There is a strong association between an AciHV-2 positive status and the experimental group (p value = 0.0027). (C) The solid dots represent the mean bacterial load of sampled mortalities, with the standard error of the mean plotted. There was no statistical difference in bacterial loads between groups. This also shows that 89% and 45% of the mortalities in the S. iniae and in the co-infection groups, respectively, tested positive for S. iniae. There is a strong association between an S. iniae positive status and the experimental group (p value ≤ 0.0001).
Histopathological analysis of at least five moribund or fresh mortalities per group during the large-scale challenge revealed that erosion and ulceration scores were only statistically significantly increased in the groups infected with AciHV-2 (Figures 7A–C, p-value < 0.0001). In addition, while lesions in fish exposed to S. iniae were most often characterized by muscle necrosis, none of the infected groups had statistically significant muscle necrosis scores present in the sections analyzed when compared to the negative control (Figure 7D). However, it is important to note that the injection site may have been absent in certain slides evaluated, affecting the overall score per group. Representative images of the lesions used for histopathological scoring can be found in Supplementary Figure 2. In fish infected with AciHV-2, lesions were often centered on the epithelium (skin and oral mucosa) and were characterized by areas of erosion and ulceration (Figures 8C, D, G). Secondary colonization of these areas of ulceration by bacteria (Figures 8D, G) and/or oomycetes (Figure 8C) was common. In fish infected with S. iniae, lesions were most pronounced at the injection site and were characterized by degeneration and necrosis of the myocytes surrounding the injection site, as well as large numbers of coccoid bacteria (Figures 8E, H). There also appeared to be large numbers of intravascular coccoid bacteria, consistent with septicemia. Fish infected with both AciHV-2 and S. iniae typically exhibited epithelial lesions and/or injection site lesions.
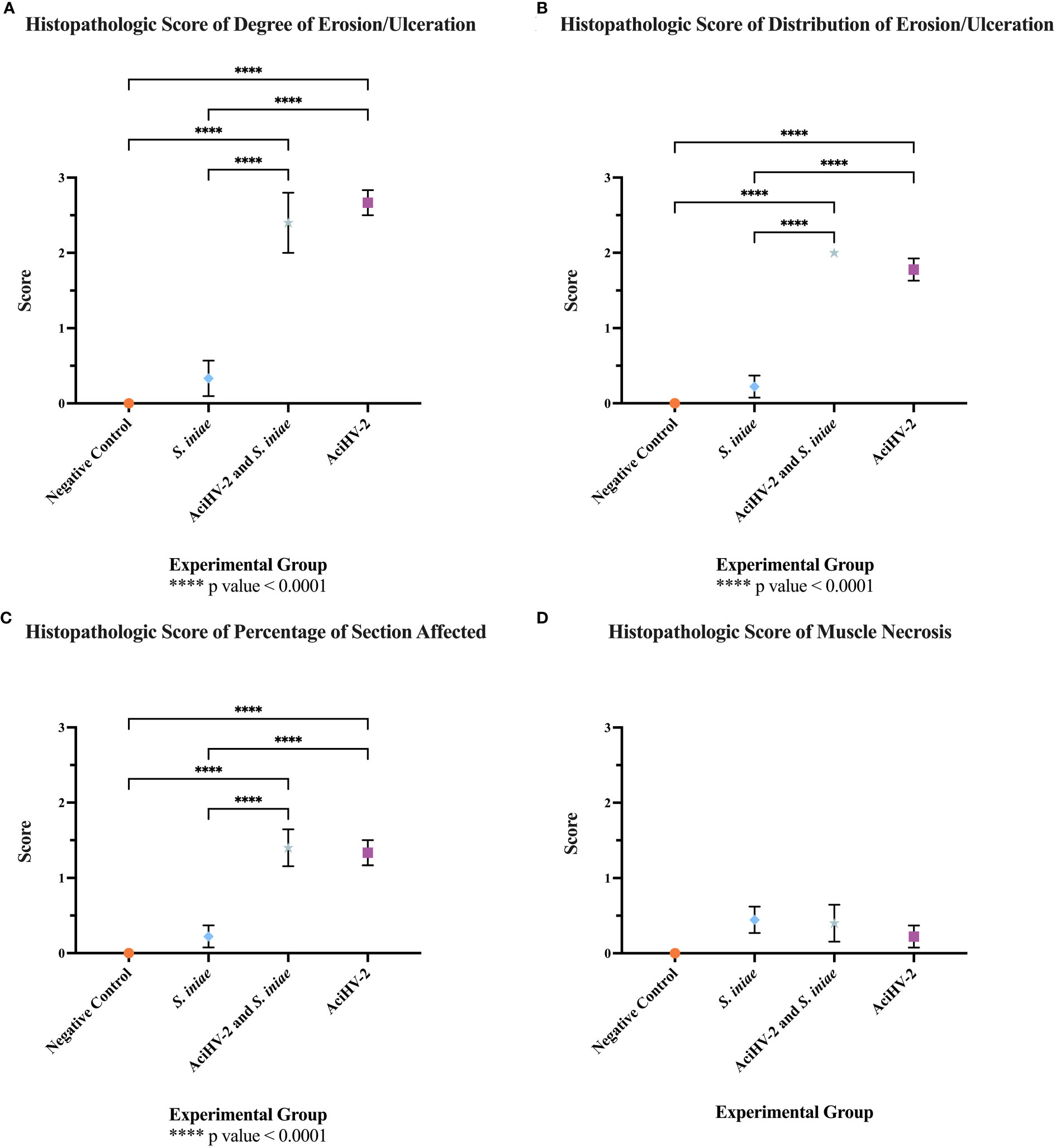
Figure 7 Histopathologic evaluation of tissues from mortalities of the co-infection challenge. An immersion challenge model was performed by exposing white sturgeon fingerlings to a 5.96x102 TCID50/mL Acipenserid herpesvirus 2 (AciHV-2) bath or a sterile cell culture media bath for 1 hour. Survivors of this challenge at 44 days were re-sorted into groups of 60 fish (triplicates of 20 fish) per treatment. Fish received either 106 CFU of S. iniae or sterile Phosphate Buffered Saline in the epaxial musculature. Mortality was monitored for 28 days after injection and at least 5 mortalities from each challenged group (S. iniae group and AciHV-2 group: n = 9 each; co-infection group: n = 5) were submitted for histopathologic analysis. The negative control representatives (n = 10) were submitted as euthanized survivors at the end of the study. (A) The “degree of erosion/ulceration” category was scored from 0-3 per slide where 0 represented no erosion or ulceration, 1 represented loss of superficial epithelial cells (erosion), 2 represented loss of epidermis/mucosal epithelium (ulceration), and 3 represented loss of dermis/underlying connective tissue. (B) The “distribution of erosion/ulceration” category was scored from 1-2 per slide where 0 represented no erosion or ulceration, 1 represented focal erosion or ulceration, and 2 represented multifocal erosion or ulceration. (C) The “percentage of section affected” category was scored from 0-4 per slide where 0 represented no erosion or ulceration, 1 represented less than 25% of the section affected, 2 represented between 25% and 50% of the section affected, 3 represented between 51% and 75% of the section affected, and 4 represented more than 75% of the section affected. (D) The “muscle necrosis” category was scored from 0-1 per slide where 0 represented no muscle necrosis present, and 1 represented muscle necrosis present. Results reveal that the degree of, distribution of, and percentage of section affected with erosion/ulceration appears to be driven by the AciHV-2 infection. In addition, none of the infected groups had significant muscle necrosis present in the sections analyzed. Values represent mean with standard error of the mean plotted.
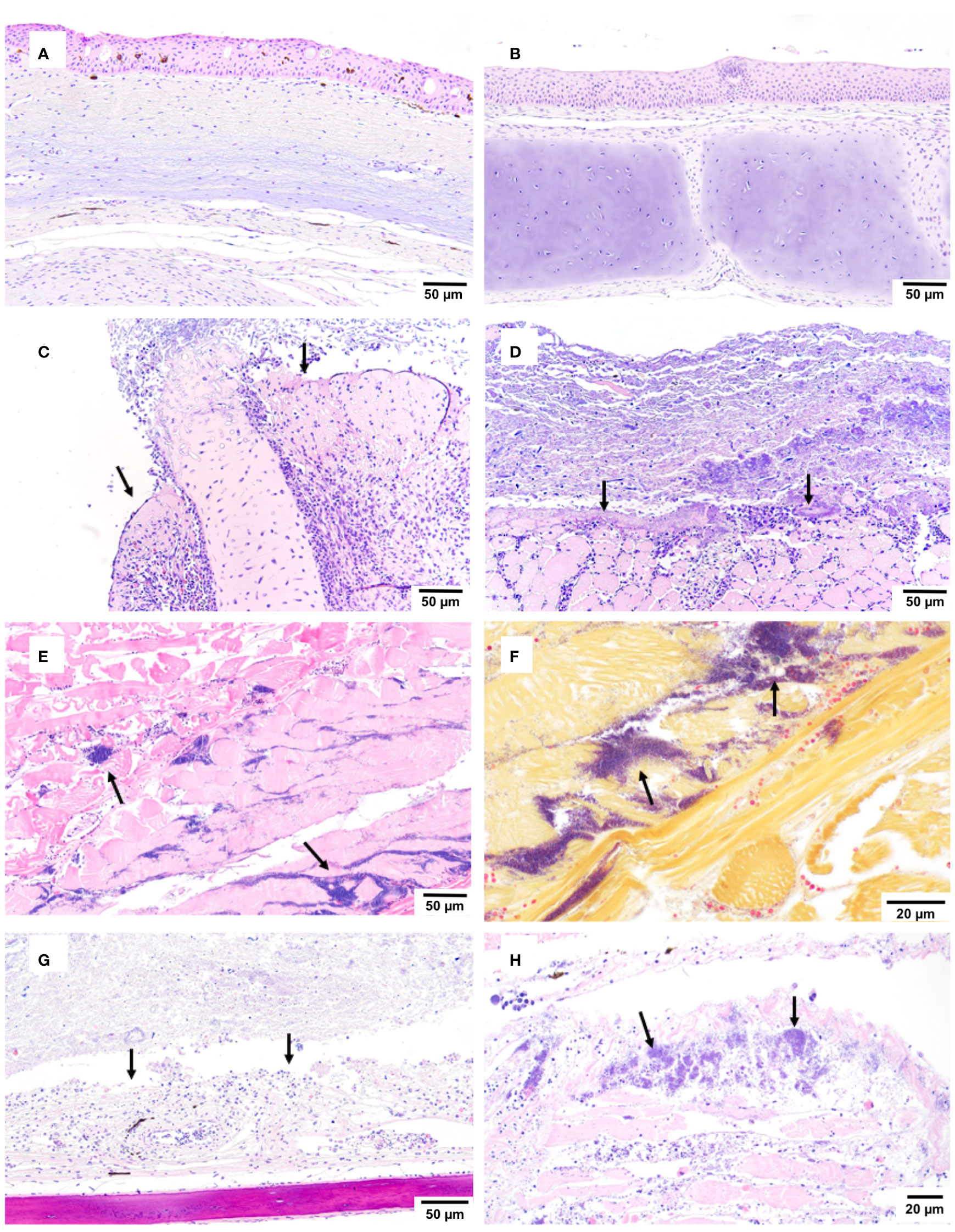
Figure 8 Histopathological findings during co-infection challenge. An immersion challenge model was performed by exposing white sturgeon fingerlings to a 5.96x102 TCID50/mL Acipenserid herpesvirus 2 (AciHV-2) bath or a sterile cell culture media bath for 1 hour. Survivors of this challenge at 44 days were re-sorted into groups of 60 fish (triplicates of 20 fish) per treatment. Fish received either 106 CFU of S. iniae or sterile Phosphate Buffered Saline in the epaxial musculature. Mortality was monitored for 28 days after injection and at least 5 mortalities from each challenged group (S. iniae group and AciHV-2 group: n = 9 each; co-infection group: n = 5) were submitted for histopathologic analysis. The negative control representatives (n = 10) were submitted as euthanized survivors at the end of the study. (A, B) the negative control showed no overt pathology (H&E). (C, D) the AciHV-2 group showed ulceration - loss of epithelium, and exposure of underlying connective tissue/cartilage (C) or skeletal muscle (D) with secondary oomycete (C) or bacterial (D) colonization (H&E). (E) the S. iniae group showed regionally extensive rhabdomyonecrosis with large numbers of intralesional coccoid bacteria (H&E). (F) the S. iniae group showed regionally extensive rhabdomyonecrosis with large numbers of intralesional Gram-positive bacteria (Gram stain). (G) the co-infection group showed ulceration - loss of epidermis, and exposure of underlying connective tissue/cartilage with secondary colonization by bacteria (H&E). (H) the co-infection group showed regionally extensive rhabdomyonecrosis with large numbers of intralesional coccoid bacteria (H&E). The black arrows point towards the lesions described.
3.2 Immune-related gene expression assessment
At 15 days post-bacterial infection during the large-scale challenge, there was a statistically significant downregulation of tnf-α transcripts in the spleen of fish in the co-infection group compared to both single pathogen groups (Figure 9A, p-value = 0.0098). In addition, there was a statistically significant downregulation of irf8 transcripts in the co-infection group compared to the negative control group (Figure 9B, p-value = 0.0374). Finally, there was a statistically significant downregulation of saa transcripts in all infected groups compared to the negative control group (Figure 9D, p-value 0.0472). These changes were not present at 28 days, but there was a statistically significant downregulation of saa transcripts in the groups infected with S. iniae compared to the AciHV-2 group (Figure 10D, p-value 0.0323).
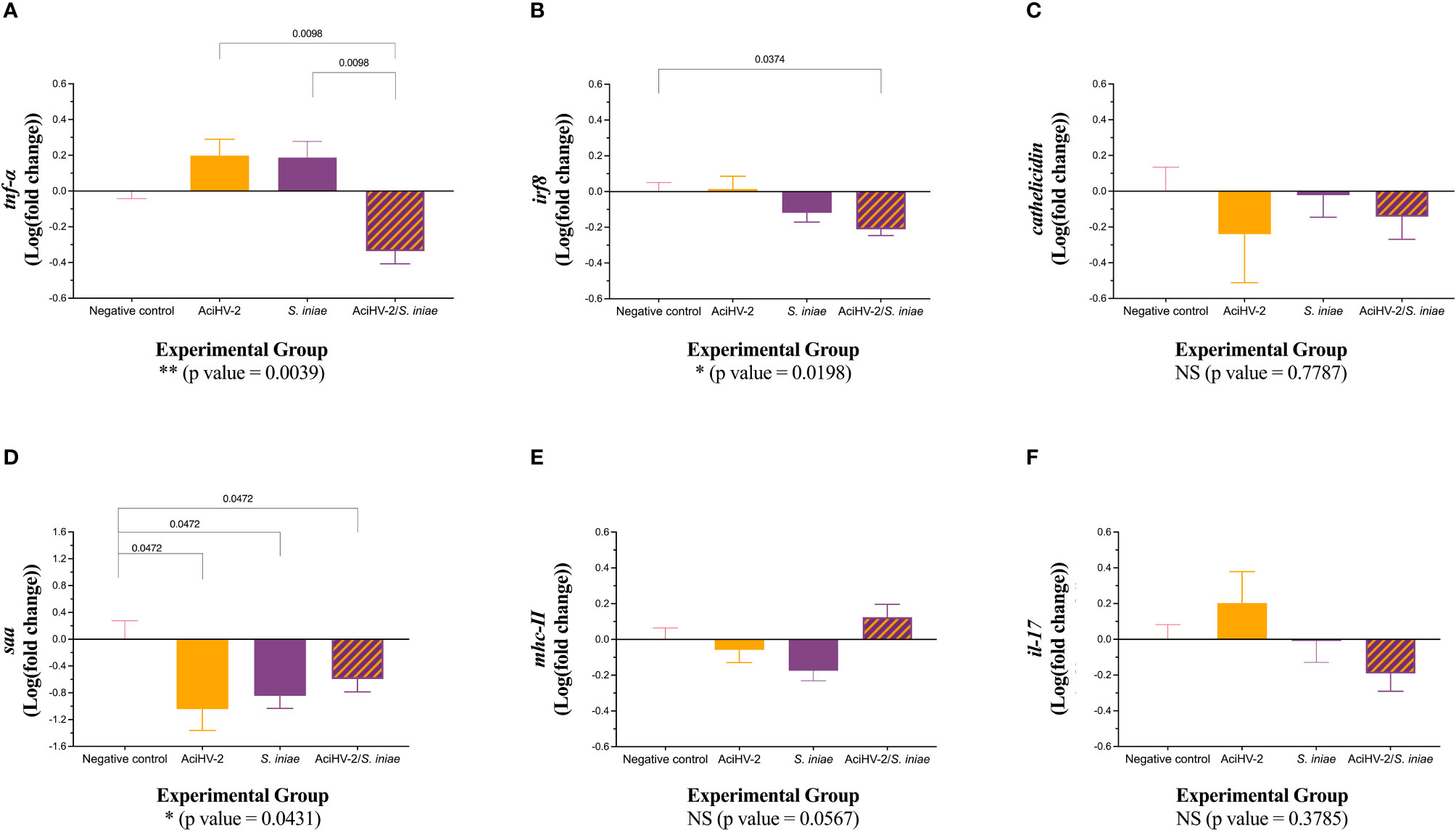
Figure 9 Gene expression at 15 days post co-infection challenge. An immersion challenge model was performed by exposing white sturgeon fingerlings to a 5.96x102 TCID50/mL bath or a sterile cell culture media bath for 1 hour. Survivors of this challenge at 44 days were re-sorted into groups of 60 fish (triplicates of 20 fish) per treatment. Fish received either 106 CFU of S. iniae or sterile Phosphate Buffered Saline in the epaxial musculature. Mortality was monitored for 28 days after injection. Two fish per tank (six fish per group) were euthanized at 15 days post-injection and spleens were collected for reverse transcription quantitative polymerase chain reaction (RT-qPCR) of important players in the immune response, using elongation factor and beta-actin as housekeeping genes. Results are plotted as the mean and standard error of the mean. Results reveal a statistically significant transcriptional downregulation of tumor necrosis factor alpha (tnfα - A) in the coinfection group compared to the single pathogen groups, as well as some differential transcription of interferon regulatory factor 8 (irf8 - B) and serum amyloid a (saa - D) when compared to the negative control. A is for tumor necrosis factor alpha, B is for interferon regulatory factor 8, C is for cathelicidin, D is for serum amyloid A, E is for major histocompatibility complex II, and F is for interleukin 17.
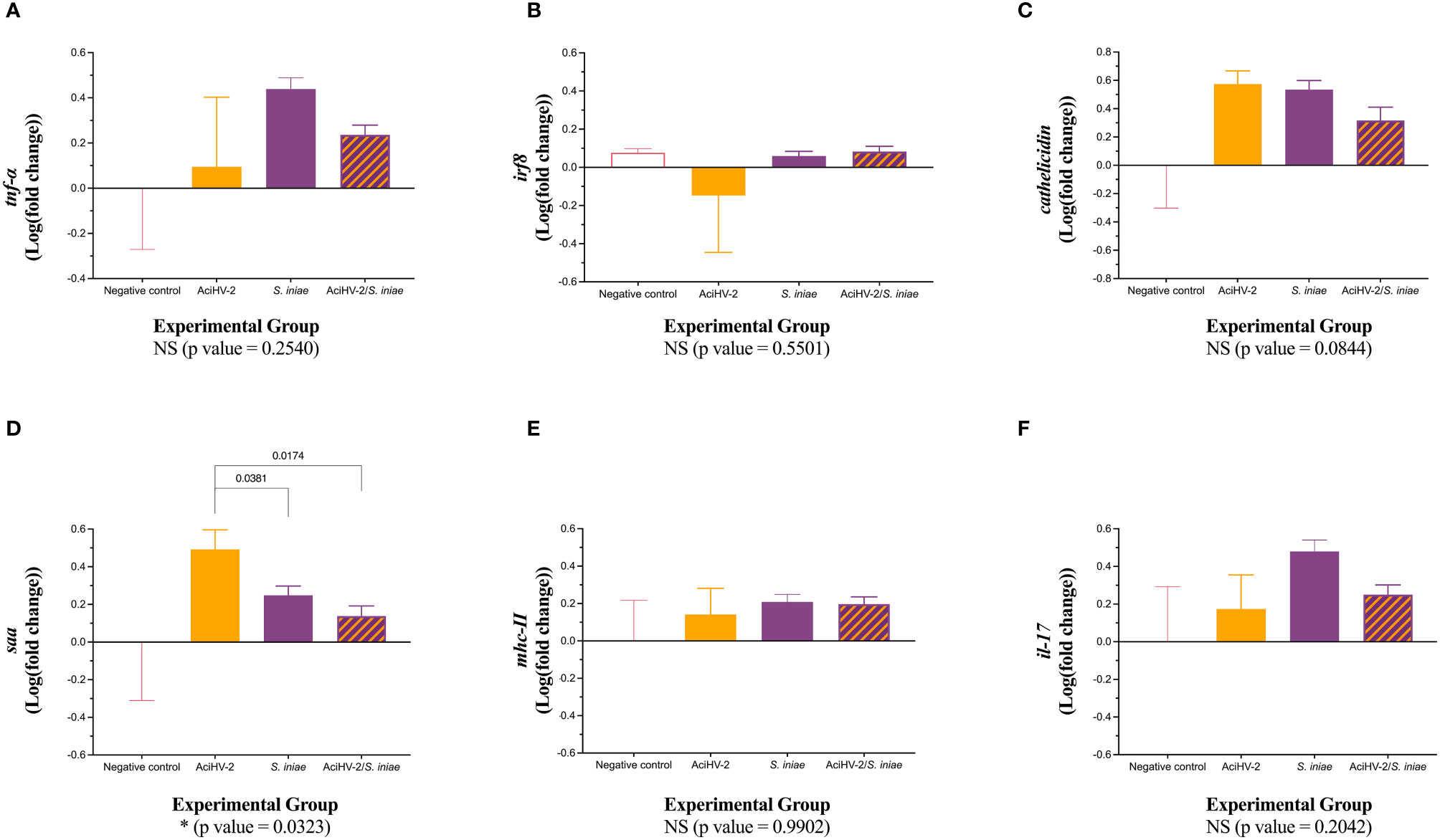
Figure 10 Gene expression at 28 days post co-infection challenge. An immersion challenge model was performed by exposing white sturgeon fingerlings to a 5.96x102 TCID50/mL bath or a sterile cell culture media bath for 1 hour. Survivors of this challenge at 44 days were re-sorted into groups of 60 fish (triplicates of 20 fish) per treatment. Fish received either 106 CFU of S. iniae or sterile Phosphate Buffered Saline in the epaxial musculature. Mortality was monitored for 28 days after injection. Ten fish per group were euthanized at 28 days post-injection and spleens were collected for reverse transcription quantitative polymerase chain reaction (RT-qPCR) of important players in the immune response, using elongation factor and beta actin as housekeeping genes. Results are plotted as the mean and standard error of the mean. Results reveal a statistically significant transcriptional downregulation of serum amyloid a (saa - D) in the groups infected with bacteria when compared to the AciHV-2 group. A is for tumor necrosis factor alpha, B is for interferon regulatory factor 8, C is for cathelicidin, D is for serum amyloid A, E is for major histocompatibility complex II, and F is for interleukin 17.
3.3 Humoral immunity assessment
Assessment of anti-S. iniae IgM present in the serum of challenged fish revealed a statistically significant reduction in anti-S.iniae serum IgM in fish from the coinfection group compared to fish in the S. iniae group (Figure 11, p-value = 0.0134).
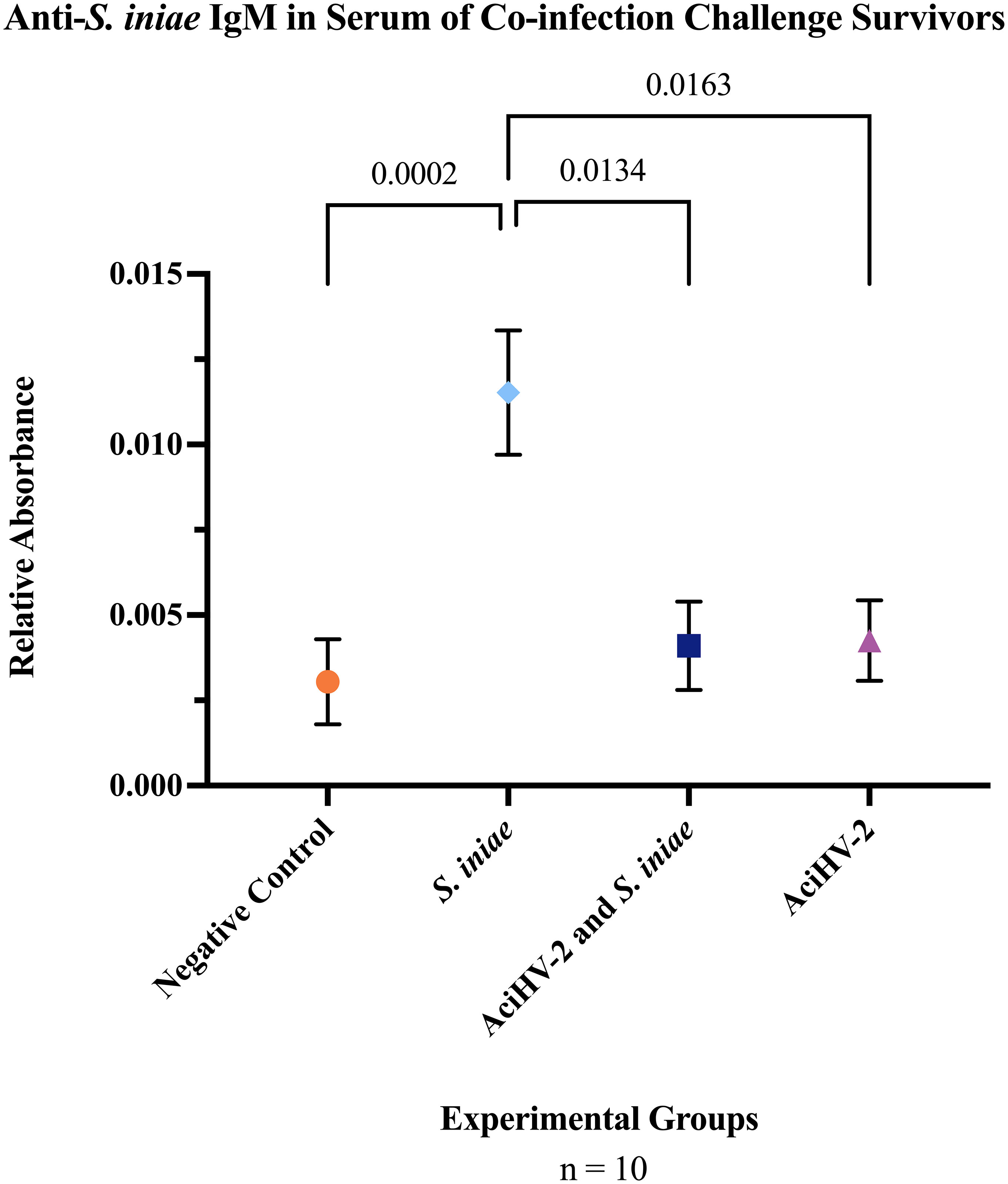
Figure 11 Detection of Serum Anti-S. iniae IgM in Survivors of Co-infection Challenge. An immersion challenge model was performed by exposing white sturgeon fingerlings to a 5.96x102 TCID50/mL bath or a sterile cell culture media bath for 1 hour. Survivors of this challenge at 44 days were re-sorted into groups of 60 fish (triplicates of 20 fish) per treatment. Fish received either 106 CFU of S. iniae or sterile Phosphate Buffered Saline in the epaxial musculature. Mortality was monitored for 28 days after injection and whole blood was collected from the caudal vein of 10 survivors per group. Serum was obtained from the whole blood samples and an indirect enzyme-linked immunosorbent assay (ELISA) was performed to detect anti-S. iniae IgM. The solid shapes represent the mean relative absorbance using an OD405-410, with standard error of the mean. The p values calculated between groups is stated in each comparison bracket. Results reveal that the S. iniae group that was previously infected with AciHV-2 had a statistically significant decrease in serum anti-S. iniae IgM levels compared to the group that received S. iniae alone.
3.4 Acute-phase protein analysis
Assessment of acute phase proteins in the serum of survivors of the co-infection challenge revealed a statistically significant decrease in serum serotransferrin 2 in the groups previously exposed to AciHV-2 compared to the negative control and S. iniae groups (Figure 12).
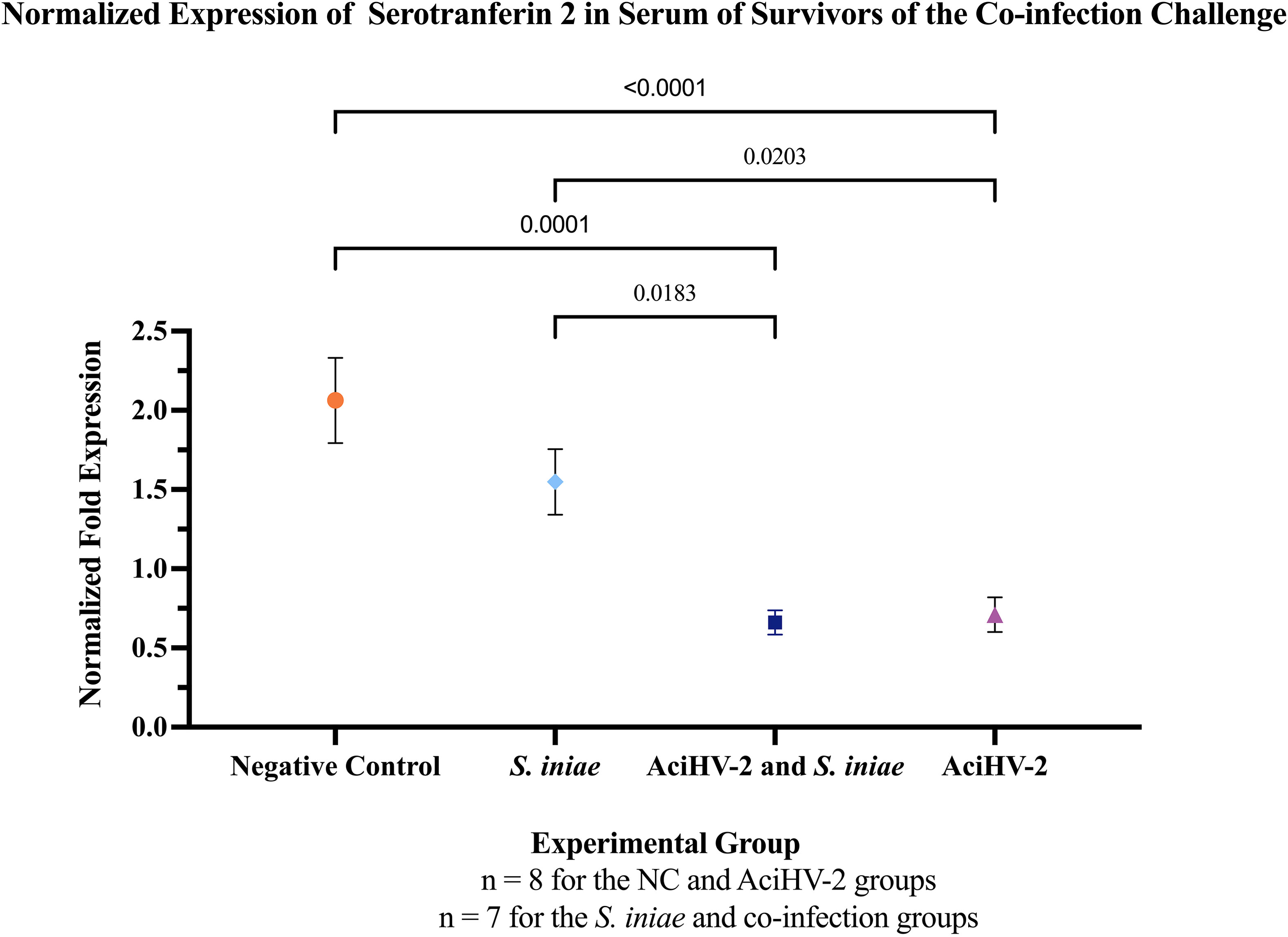
Figure 12 Detection of Serum Serotransferrin in Survivors of Co-infection Challenge. An immersion challenge model was performed by exposing white sturgeon fingerlings to a 5.96x102 TCID50/mL bath or a sterile cell culture media bath for 1 hour. Survivors of this challenge at 44 days were re-sorted into groups of 60 fish (triplicates of 20 fish) per treatment. Fish received either 106 CFU of S. iniae or sterile Phosphate Buffered Saline in the epaxial musculature. Mortality was monitored for 28 days after injection and whole blood was collected from the caudal vein of 10 survivors per group. Serum was obtained from the whole blood samples and Western Blotting was performed to identify Serotransferrin 2 (STF-2) in the samples. The solid shapes represent the mean normalized fold expression, with standard error the mean plotted. The p values calculated between groups are stated in each comparison bracket. Results reveal that the co-infection and AciHV-2 groups had a statistically significant decrease in serum STF-2 levels compared to the group that received S. iniae alone and the negative control.
4 Discussion
The Herpesvirales order is characterized by the ability to establish latency, and this process can lead to changes to the immunocompetence of the host (Cohen, 2020). While this has not been explored for AciHV-2, reports of co-infections in white sturgeon (Soto et al., 2017) similar to human pediatric IGASI (Laupland et al., 2000) suggest there is an interaction between the infectious agents and the host that impacts disease outcome. Neither of these presentations across taxa has been investigated in a controlled environment and the study of this co-infection scenario in white sturgeon may inform future studies regarding human pediatric IGASI. Therefore, our initial studies aimed to perform a pilot challenge to assess the hypothesis that a recent, potentially latent, AciHV-2 infection in white sturgeon fingerlings would increase mortalities by a subsequent S. iniae infection. Results revealed that the co-infection group had a statistically significant decrease in survival of 41% compared to the S. iniae group and of 36% compared to AciHV-2 group (Figure 5A). This provided proof of concept that a recent, potentially latent, AciHV-2 infection has a profound impact on the outcome of a subsequent S. iniae infection in white sturgeon. While it was hypothesized that this was going to be the case based on natural cases of piscine streptococcosis in white sturgeon that also had AciHV-2 (Soto et al., 2017), these results are consistent with additional reports on S. iniae-virus co-infections in other fish species. For example, Japanese flounder (Paralichthys olivaceus) experiences statistically increased mortality when infected with S. iniae 1 week after an aquabirnavirus (ABV) infection compared with fish infected with S. iniae alone (Pakingking et al., 2003). To the authors’ knowledge, however, our study is the first to investigate it in the context of a herpesvirus infection in sturgeon during what is suspected to be the latent phase (80 days post-infection, 30 days past the last mortality of the active outbreak, with no AciHV-2 detectable in fin tissue of the majority of survivors – Figures 3A, D).
A large-scale challenge was then performed to investigate aspects of the immune system potentially affected by AciHV-2 and leading to altered interactions between the host and S. iniae. For the large-scale challenge presented here, while following similar trends to the pilot challenge (Figure 5A), there was no significant difference in survival between the co-infection group and the S. iniae group (Figure 6A). The primary differences between the pilot and large-scale challenge experiments were time between viral and bacterial infection (80 vs 44 dpi, respectively) and bacterial dose (108 vs 106 CFU/fish, respectively). While the overall lower mortality rate may preclude statistical significance, this still raises interesting questions into the effect of timing and bacterial infectious dose in co-infections, and these concepts have been investigated before for S. iniae in other co-infections.
In terms of the bacterial infection dose, a study assessing S. iniae infections in tilapia (Oreochromis niloticus) showed that infection dose had an impact on the mortality rate observed only if high-density conditions were present (Shoemaker et al., 2000). High-density environments have been associated with immunosuppression in fish due to eliciting a physiologic stress response (Aidos et al., 2020). Our two studies combined seem to support that both factors (high bacterial infection dose and immunomodulation) must be present in order to impact mortality in a statistically significant manner.
In terms of the timing of the infection, in the ABV-S. iniae study using Japanese flounder mentioned above, the authors observed that if they infected the flounder with S. iniae 3 weeks post ABV infection rather than 1-week, no difference in mortality was observed between co-infection and single pathogen groups (Pakingking et al., 2003). The authors suggest that active replication of ABV, which happens during early infection, may be required to induce immunosuppression and worsen the outcome of the S. iniae infection. In our large-scale challenge, fish were infected with S. iniae 44 days after the AciHV-2 immersion compared to 80 days post-immersion in the pilot challenge. It is possible that the transcriptional profile of AciHV-2 is different this early after the active outbreak and the immunomodulatory effects are thus distinct from later in its life cycle, which has been described for multiple herpesviruses of mammals (Ye et al., 2011; Rozman et al., 2022). Further comparative studies are warranted to tease apart the contribution to mortality of bacterial infectious dose and of bacterial infection timing in relation to viral infection during this co-infection scenario.
It is well described that latency is not a static, but rather a dynamic state where the virus is mostly dormant, yet the transcriptional profile is not fixed. For example, low levels of lytic gene expression have been detected in cells harboring Herpes Simplex Virus 1 (HSV-1) in the absence of any other evidence of reactivation (Bloom, 2016). Furthermore, studies have also shown that within a population of cells latently infected with HSV-1, a sub-population of those cells can go through transient reactivation while the remaining cells still lack detectable infectious virus or viral lytic transcripts (Bloom, 2016). Given this information, the term latency is applicable only at the cellular level rather than the tissue level. This is important to consider when we assess the effects of the viral population on the immune system of the host. In theory, at any time after the initial outbreak, herpesviruses have the capacity to benefit from the immunomodulation obtained through the expression of specific latency-associated transcripts while going through reactivation events that ensure passing progeny to new hosts. In this study, our main hypothesis suggests that the potentially latent AciHV-2 interacts with the immune system of the white sturgeon in a way that changes the fish’s interaction with S. iniae. In the pilot challenge, fin clips were collected from all survivors prior to the bacterial infection (Figure 2D), from all mortalities after the bacterial infection (Figure 5B), and from all survivors after the bacterial infection (Figure 5D) to test AciHV-2 detection via qPCR as a marker of reactivation. When assessing the distribution of positive fish throughout the tanks, it is most notably observed that for the co-infection group there is one fish in Tank 1 that was positive during the pre-bacterial infection timepoint that is not detected positive again (Supplementary Figure 3, Tank 1 of the co-infection group) and two fish in Tank 2 that test negative during the pre-bacterial infection timepoint but positive during the survivor timepoint (Supplementary Figure 3, Tank 2 of the co-infection group). These results are evidence of the population undergoing some level of herpesviral reactivation. Further studies are needed to show that AciHV-2 achieves latency in a cell population and then to evaluate if latency is maintained in a subpopulation of cells while these reactivation events occur.
It is established that mammalian herpesviruses have multiple pathways for regulating host cellular immune competency, resulting in immune system evasion (Alcami, 2003; Alibek et al., 2014; Crow et al., 2016). The potential for this has also been demonstrated to a lesser extent for certain herpesviruses that affect fish (Piazzon et al., 2015; Lu et al., 2021; Zhou et al., 2021). The evasion mechanisms are diverse, including complement activation inhibition, impaired antigen presentation via numerous pathways, apoptosis and natural killer cell inhibition, interferon signaling interference, and others (Chang et al., 2004; Sehrawat et al., 2018). From the host side, results revealed a significant transcriptional downregulation of tnfα at 15 days post-bacterial infection in the co-infection group compared to the S. iniae group (Figure 9A). This transcriptional downregulation is not maintained at 28 days post-bacterial infection (Figure 10A). The cytokine TNFα, a potent inflammatory modulator reported to have a prominent pro-inflammatory role while also participating in regulating the extent and duration of the immune response, is mainly produced by activated macrophages, T lymphocytes, and natural killer cells, but it is also expressed at lower levels by various other cells, including fibroblasts, smooth muscle cells, and even tumor cells. Some studies have specifically looked at the augmenting effects of TNFα on B cell proliferation and immunoglobulin production (Pasparaki et al., 1996), which suggests that the transcriptional downregulation of tnfα in the spleen at 15 days post-bacterial infection in this study could be having an impact on antibody response. This was supported by the significant decrease in serum anti-S. iniae IgM in fish from the co-infection group compared to fish in the single-pathogen group (Figure 11) as had been suggested for pediatric IGASI (Laupland et al., 2000). While a decrease in humoral immunity could be playing a role in this co-infection during the late stages of the disease, and it is most concerning for survivors of the co-infection that were to encounter S. iniae again as older fish in the caviar production pipeline, it is unclear at this time if this would be significant in the acute onset of disease where most mortalities were detected during the challenges in this study (first 10 days post-bacterial infection, Figures 5A, 6A). It is important to note though that teleost B cells have been shown to have phagocytic and intracellular killing capacity (Ye and Li, 2020), which may play a role in early immune response against S. iniae. More studies are warranted to determine which B cell populations are being affected during these co-infection scenarios and to describe the timeline of humoral immunity development in white sturgeon against S. iniae.
Recently, sequencing of the full genome of AciHV-2 demonstrated that one of the open reading frames of AciHV-2, ORF 101, has the TNFRSF14_teleost conserved domain (Quijano Carde et al., 2024). This is present in members of the TNF Receptor superfamily, which are common targets for viral manipulation due to their important roles in the regulation of immune responses as well as viral entry (Tiwari et al., 2005; Rakus et al., 2017). While further characterization of ORF 101 in AciHV-2 is needed to understand its expression patterns and effects on the host, other teleost herpesviruses have been shown to influence tnfα expression. For example, Cyprinid Herpesvirus 3 (CyHV-3) has been shown to encode two TNFR homologs, of which one causes upregulation of tnfα (Zhou et al., 2021). To our knowledge, AciHV-2 alone has not been shown to change expression patterns of tnfα in the spleen, but this changes during the co-infection state. Further studies are indicated to determine what interactions lead to the decreased transcript abundance of tnfα and of pathogen-specific IgM in the host, and to understand the temporal transcriptional patterns of the host and AciHV-2, particularly as it pertains to the expression of tnfα and ORF 101. Assessing translation to determine if these gene expression changes lead to effects at the protein level is also warranted.
The impact of co-infection on the production of the acute-phase protein serotransferrin 2 (STF-2), which has been shown to play a role in the inflammatory response during certain infections in white sturgeon (Soto et al., 2021), was also assessed. Fish exposed to AciHV-2 (both single pathogen and co-infection) had a significant decrease in STF-2 serum levels compared to the Negative control and S. iniae groups (Figure 12) at 28 days post-bacterial infection. This finding provides an alternate or perhaps complementary hypothesis on the mechanism behind the decreased anti-S. iniae IgM levels in the serum of fish in the co-infection group (Figure 11). Serotransferrin 2 is a protein in the transferrin family, which is known for its iron transportation role. Because of its ability to bind iron, serotransferrin is considered part of the immune response where it creates an iron-limited environment that is not conducive to pathogen replication, including S. iniae (Stafford and Belosevic, 2003). This function alone has been identified as a mechanism against herpesvirus as well (Maffettone et al., 2008). Nonetheless, even though this direct mechanism could explain the worsened disease state observed in the co-infection group, serotransferrin has an additional function related to immunity against herpesviruses as it has been described to be a primary activator of fish macrophages, an important antigen-presenting cell to CD4+ T cells in fish (Stafford and Belosevic, 2003). Evidence indicating that CD4+ T cells are vital for herpesvirus control continues to emerge (Walton et al., 2013). This is particularly highlighted in both susceptibility to and reactivation of herpesviruses when human host has a CD4+ T cell deficiency (Komanduri et al., 2001). CD4+ T cells play a role in herpesvirus control not only by using helper functions, but also by using direct effector functions that impact viral replication via secreted interferon (IFN) gamma and TNFα (Casazza et al., 2006). Finally, CD4+ T cells are also involved in a robust and appropriate humoral response, being shown to be necessary for the generation of plasma and memory B cells, as well as germinal center establishment and responses (Elong Ngono et al., 2019). Given the importance of CD4+ T cell response in the control of herpesviral infections, both during the lytic and latent stages, it has been described that herpesviruses have a variety of strategies to manipulate CD4+ T cell responses (Walton et al., 2013). A potential mechanism by which AciHV-2 – white sturgeon – S. iniae interactions lead to an inferior antibody response against S. iniae during a co-infection may involve the potential effects of AciHV-2 on the general CD4+ T cell population via a decrease in serotransferrin. Affected CD4+ T cells have been reported to have decreased production of TNFα as well as inhibited cytotoxic effector functions in human and murine cytomegalovirus infections (Walton et al., 2013). This may indicate that the downregulation of TNFα may be related to the poor activation of both fish macrophages and CD4+ T cells.
The results from this study support an altered immune response in the co-infection group, including the hypothesized impaired humoral immunity against S. iniae and additional impacts to important players of the innate immune response. Further studies are indicated to determine what interactions lead to the decreased production of pathogen-specific IgM in the host. Piscine streptococcosis is a significant emerging disease of white sturgeon with the capacity to cause outstanding losses in white sturgeon aquaculture, particularly considering its effect on subadult populations. Our understanding of the circumstances under which S. iniae causes significant disease and the mechanisms responsible for creating the ideal scenario for this to occur will focus prevention and therapeutic programs in aquaculture farms.
Data availability statement
The raw data supporting the conclusions of this article will be made available by the authors, without undue reservation.
Ethics statement
The animal study was approved by the University of California Institutional Animal Care and Use Committee. The study was conducted in accordance with the local legislation and institutional requirements.
Author contributions
EQC: Conceptualization, Data curation, Formal analysis, Funding acquisition, Investigation, Methodology, Validation, Writing – original draft, Writing – review & editing. KA: Investigation, Writing – review & editing. SY: Formal analysis, Investigation, Methodology, Writing – review & editing. TH: Investigation, Methodology, Writing – review & editing. HJ: Investigation, Writing – review & editing. EH: Formal analysis, Investigation, Methodology, Visualization, Writing – review & editing. SP: Data curation, Formal analysis, Investigation, Methodology, Writing – review & editing. MF: Conceptualization, Formal analysis, Funding acquisition, Investigation, Methodology, Project administration, Resources, Supervision, Validation, Visualization, Writing – review & editing. ES: Conceptualization, Funding acquisition, Investigation, Methodology, Project administration, Resources, Supervision, Validation, Visualization, Writing – review & editing.
Funding
The author(s) declare financial support was received for the research, authorship, and/or publication of this article. This work was supported in part by the Comparative Medical Science Training Program NIH T-32 OD011147 (EMQC) and the USDA National Institute of Food and Agriculture (Animal Health project CACALV-AH-410).
Acknowledgments
The authors wish to thank the team of the Center for Aquatic Biology and Aquaculture at UC Davis for their constant support with the housing and care of the fish used in this project and to Dr. Eric Littman for his assistance with fish care during the pilot study.
Conflict of interest
The authors declare that the research was conducted in the absence of any commercial or financial relationships that could be construed as a potential conflict of interest.
Publisher’s note
All claims expressed in this article are solely those of the authors and do not necessarily represent those of their affiliated organizations, or those of the publisher, the editors and the reviewers. Any product that may be evaluated in this article, or claim that may be made by its manufacturer, is not guaranteed or endorsed by the publisher.
Supplementary material
The Supplementary Material for this article can be found online at: https://www.frontiersin.org/articles/10.3389/faquc.2024.1306518/full#supplementary-material
References
Aidos L., Cafiso A., Serra V., Vasconi M., Bertotto D., Bazzocchi C., et al. (2020). How different stocking densities affect growth and stress status of acipenser baerii early stage larvae. Animals. 10 (8), 1289. doi: 10.3390/ani10081289
Alcami A. (2003). Viral mimicry of cytokines, chemokines and their receptors. Nat. Rev. Immunol. 3 (1), 36–50. doi: 10.1038/nri980
Alibek K., Baiken Y., Kakpenova A., Mussabekova A., Zhussupbekova S., Akan M., et al. (2014). Implication of human herpesviruses in oncogenesis through immune evasion and supression. Infect. Agent Cancer. 9 (1), 3. doi: 10.1186/1750-9378-9-3
Baiano J. C. F., Barnes A. C. (2009). Towards control of streptococcus iniae. Emerg. Infect. Dis. 15 (12), 1891–1896. doi: 10.3201/eid1512.090232
Bloom D. C. (2016). “Chapter two - alphaherpesvirus latency: A dynamic state of transcription and reactivation,” in Advances in virus research (Cambridge, MA; San Diego, CA, United States; Kidlington, Oxford, London: Academic Press), 53–80. Available at: https://linkinghub.elsevier.com/retrieve/pii/S0065352715000901.
Carocci F., Lagrange C., Levavasseur V., Yakimushkin A. (2004). Sturgeons (Acipenseriformes) (Rome, Italy: Food and Agriculture Organization of the United Nations). Available at: https://www.fao.org/3/Y5261E/y5261e06.htm.
Casazza J. P., Betts M. R., Price D. A., Precopio M. L., Ruff L. E., Brenchley J. M., et al. (2006). Acquisition of direct antiviral effector functions by CMV-specific CD4+ T lymphocytes with cellular maturation. J. Exp. Med. 203 (13), 2865–2877. doi: 10.1084/jem.20052246
Chang W. L. W., Baumgarth N., Yu D., Barry P. A. (2004). Human cytomegalovirus-encoded interleukin-10 homolog inhibits maturation of dendritic cells and alters their functionality. J. Virol. 78 (16), 8720–8731. doi: 10.1128/JVI.78.16.8720-8731.2004
Cohen J. I. (2020). Herpesvirus latency. J. Clin. Invest. 130 (7), 3361–3369. doi: 10.1172/JCI136225
Crow M. S., Lum K. K., Sheng X., Song B., Cristea I. M. (2016). Diverse mechanisms evolved by DNA viruses to inhibit early host defenses. Crit. Rev. Biochem. Mol. Biol. 51 (6), 452–481. doi: 10.1080/10409238.2016.1226250
Eide K. E., Miller-Morgan T., Heidel J. R., Kent M. L., Bildfell R. J., LaPatra S., et al. (2011). Investigation of koi herpesvirus latency in koi. J. Virol. 85 (10), 4954–4962. doi: 10.1128/JVI.01384-10
Elong Ngono A., Young M. P., Bunz M., Xu Z., Hattakam S., Vizcarra E., et al. (2019). CD4+ T cells promote humoral immunity and viral control during Zika virus infection. PloS Pathog. 15 (1), e1007474. Fernandez-Sesma A. doi: 10.4049/jimmunol.202.Supp.140.15
Ethier V. (2014). Farmed sturgeon (Monterey, CA, United States: Monterey Bay Aquarium Seafood Watch). Available at: https://seafood.ocean.org/wp-content/uploads/2016/10/Sturgeon-Farmed-US.pdf.
Frère J., Bidet P., Tapiéro B., Rallu F., Minodier P., Bonacorsi S., et al. (2016). Clinical and microbiological characteristics of invasive group A streptococcal infections before and after implementation of a universal varicella vaccine program: table 1. Clin. Infect. Dis. 62 (1), 75–77. doi: 10.1093/cid/civ793
Goodwin A. (2012). Herpesviruses in fish (Stoneville, MS, United States: Southern Regional Aquaculture Center).
Hanson L. A., Rudis M. R., Vasquez-Lee M., Montgomery R. D. (2006). A broadly applicable method to characterize large DNA viruses and adenoviruses based on the DNA polymerase gene. Virol. J. 3 (1), 28. doi: 10.1186/1743-422X-3-28
Heckman T. I., Shahin K., Henderson E. E., Griffin M. J., Soto E. (2022). Development and efficacy of Streptococcus iniae live-attenuated vaccines in Nile tilapia, Oreochromis niloticus. Fish Shellfish Immunol. 121, 152–162. doi: 10.1016/j.fsi.2021.12.043
Hedrick R., McDowell T., Groff J., Yun S., Wingfield W. (1991). Isolation of an epitheliotropic herpesvirus from white sturgeon Acipenser transmontanus. Dis. Aquat Organ. 11, 49–56. doi: 10.3354/dao011049
Hedrick R. P., McDowell T. S., Rosemark R., Aronstein D., Lannan C. N. (1991). Two cell lines from white sturgeon. Trans. Am. Fish Soc 120 (4), 528–534. doi: 10.1577/1548-8659(1991)120<0528:TCLFWS>2.3.CO;2
Jarvis M. A., Nelson J. A. (2007). “Chapter 42: Molecular basis of persistence and latency,” in Human herpesviruses: biology, therapy, and immunoprophylaxis (Cambridge: Cambridge University Press). Available at: https://www.ncbi.nlm.nih.gov/books/NBK47414/?report=reader#_NBK47414_pubdet_.
Kelly J. (2019). White sturgeon (West Sacramento, CA, United States: California Department of Fish and Wildlife). Available at: https://wildlife.ca.gov/Conservation/Fishes/Sturgeon/White-Sturgeon.
Komanduri K. V., Feinberg J., Hutchins R. K., Frame R. D., Schmidt D. K., Viswanathan M. N., et al. (2001). Loss of cytomegalovirus-specific CD4 + T cell responses in human immunodeficiency virus type 1–infected patients with high CD4 + T cell counts and recurrent retinitis. J. Infect. Dis. 183 (8), 1285–1289. doi: 10.1086/319683
Laskey A. L., Johnson T. R., Dagartzikas M. I., Tobias J. D. (2000). Endocarditis attributable to group A hemolytic streptococcus after uncomplicated varicella in a vaccinated child. PEDIATRICS. 106 (3), 4. doi: 10.1542/peds.106.3.e40
Laupland K. B., Davies H. D., Low D. E., Schwartz B., Green K., the Ontario Group A Streptococcal Study Group, et al. (2000). Invasive group A streptococcal disease in children and association with varicella-zoster virus infection. Pediatrics 105 (5). doi: 10.1542/peds.105.5.e60
Lei C., Yang J., Hu J., Sun X. (2021). On the calculation of TCID50 for quantitation of virus infectivity. Virol. Sin. 36 (1), 141–144. doi: 10.1007/s12250-020-00230-5
Lepa A., Siwicki A. K. (2012). Fish herpesvirus diseases: a short review of current knowledge. Acta Vet. Brno. 81 (4), 383–389. doi: 10.2754/avb201281040383
López-Porras A., Elizondo C., Chaves A. J., Camus A. C., Griffin M. J., Kenelty K., et al. (2019). Application of multiplex quantitative polymerase chain reaction methods to detect common bacterial fish pathogens in Nile tilapia, Oreochromis niloticus , hatcheries in Costa Rica. J. World Aquac Soc 50 (3), 645–658. doi: 10.1111/jwas.12576
Lu J. F., Jin T. C., Zhou T., Lu X. J., Chen J. P., Chen J. (2021). Identification and characterization of a tumor necrosis factor receptor like protein encoded by Cyprinid Herpesvirus 2. Dev. Comp. Immunol. 116, 103930. doi: 10.1016/j.dci.2020.103930
Maffettone C., De Martino L., Irace C., Santamaria R., Pagnini U., Iovane G., et al. (2008). Expression of iron-related proteins during infection by bovine herpes virus type-1. J. Cell Biochem. 104 (1), 213–223. doi: 10.1002/jcb.21618
Miller J. D., Neely M. N. (2005). Large-scale screen highlights the importance of capsule for virulence in the zoonotic pathogen streptococcus iniae. Infect. Immun. 73 (2), 921–934. doi: 10.1128/IAI.73.2.921-934.2005
Mugetti D., Pastorino P., Menconi V., Pedron C., Prearo M. (2020). The old and the new on viral diseases in sturgeon. Pathogens. 9 (2), 146. doi: 10.3390/pathogens9020146
Nguyen D. T., Marancik D., Soto E. (2020). Intracoelomic- and intramuscular-injection challenge model of piscine streptococcosis in white sturgeon fingerlings. J. Aquat Anim. Health 32 (3), 133–138. doi: 10.1002/aah.10112
Nicoli F., Appay V. (2017). Immunological considerations regarding parental concerns on pediatric immunizations. Vaccine. 35 (23), 3012–3019. doi: 10.1016/j.vaccine.2017.04.030
Oyake S., Ohi T., Koga M. (2000). A case of varicella complicated by cellulitis and scarlet fever due to streptococcus pyogenes. J. Dermatol. 27 (11), 750–752. doi: 10.1111/j.1346-8138.2000.tb02272.x
Pakingking R., Takano R., Nishizawa T., Mori K., Iida Y., Arimoto M., et al. (2003). Experimental Coinfection with Aquabirnavirus and Viral Hemorrhagic Septicemia Virus (VHSV), Edwardsiella tarda or Streptococcus iniae in Japanese Flounder Paralichthys olivaceus. Fish Pathol. 38 (1), 15–21. doi: 10.3147/jsfp.38.15
Pasparaki M., Alexopoulou L., Episkopou V., Kollias G. (1996). Immune and inflammatory responses in TNF~-deficient mice: A critical requirement for TNFoL in the formation of primary B cell follicles, follicular dendritic cell networks and germinal centers, and in the maturation of the humoral immune response. J. Exp. Med. 184, 1397–1411. doi: 10.1084/jem.184.4.1397
Piazzon M. C., Wentzel A. S., Tijhaar E. J., Rakus K. Ł., Vanderplasschen A., Wiegertjes G. F., et al. (2015). Cyprinid herpesvirus 3 Il10 inhibits inflammatory activities of carp macrophages and promotes proliferation of Igm + B cells and memory T cells in a manner similar to carp Il10. J. Immunol. 195 (8), 3694–3704. doi: 10.4049/jimmunol.1500926
Pierezan F., Shahin K., Heckman T. I., Ang J., Byrne B. A., Soto E. (2020). Outbreaks of severe myositis in cultured white sturgeon ( Acipenser transmontanus L.) associated with Streptococcus iniae. J. Fish Dis. 43 (4), 485–490. doi: 10.1111/jfd.13145
Quijano Carde E. M., Anenson K., Waldbieser G., Brown C. T., Griffin M., Henserson E., et al. (2024). Acipenserid herpesvirus 2 genome and partial validation of a qPCR for its detection in white sturgeon Acipenser transmontanus. Dis. Aquat. Org. 157, 45-59. doi: 10.3354/dao03768
Rakus K., Ronsmans M., Forlenza M., Boutier M., Piazzon M. C., Jazowiecka-Rakus J., et al. (2017). Conserved fever pathways across vertebrates: A herpesvirus expressed decoy TNF-α Receptor delays behavioral fever in fish. Cell Host Microbe 21 (2), 244–253. doi: 10.1016/j.chom.2017.01.010
Reed A. N., Izume S., Dolan B. P., LaPatra S., Kent M., Dong J., et al. (2014). Identification of B cells as a major site for cyprinid herpesvirus 3 latency. J. Virol. 88 (16), 9297–9309. doi: 10.1128/JVI.00990-14
Rozman B., Nachshon A., Levi Samia R., Lavi M., Schwartz M., Stern-Ginossar N. (2022). Temporal dynamics of HCMV gene expression in lytic and latent infections. Cell Rep. 39 (2), 110653. doi: 10.1016/j.celrep.2022.110653
Sehrawat S., Kumar D., Rouse B. T. (2018). Herpesviruses: harmonious pathogens but relevant cofactors in other diseases? Front. Cell Infect. Microbiol. 8, 177. doi: 10.3389/fcimb.2018.00177
Shoemaker C. A., Evans J. J., Klesius P. H. (2000). Density and dose: factors affecting mortality of Streptococcus iniae infected tilapia žOreochromis niloticus/. Aquaculture. 188, 229–235. doi: 10.1016/S0044-8486(00)00346-X
Soto E., Coleman D., Yazdi Z., Purcell S. L., Camus A., Fast M. D. (2021). Analysis of the white sturgeon (Acipenser transmontanus) immune response during immunostimulation and Veronaea botryosa infection. Comp. Biochem. Physiol. Part D Genomics Proteomics. 40, 100879. doi: 10.1016/j.cbd.2021.100879
Soto E., Fast M. D., Purcell S. L., Denver Coleman D., Yazdi Z., Kenelty K., et al. (2022). Expression of immune markers of white sturgeon (Acipenser transmontanus) during Veronaea botryosa infection at different temperatures. Comp. Biochem. Physiol. Part D Genomics Proteomics. 41, 100950. doi: 10.1016/j.cbd.2021.100950
Soto E., Richey C., Stevens B., Yun S., Kenelty K., Reichley S., et al. (2017). Co-infection of Acipenserid herpesvirus 2 (AciHV-2) and Streptococcus iniae in cultured white sturgeon Acipenser transmontanus. Dis. Aquat Organ. 124 (1), 11–20. doi: 10.3354/dao03108
Speck S. H., Ganem D. (2010). Viral latency and its regulation: lessons from the gammaherpesviruses. Cell Host Microbe 8 (1), 100–115. doi: 10.1016/j.chom.2010.06.014
Stafford J. L., Belosevic M. (2003). Transferrin and the innate immune response of fish: identification of a novel mechanism of macrophage activation. Dev. Comp. Immunol. 27 (6–7), 539–554. doi: 10.1016/S0145-305X(02)00138-6
Stingley R. L., Griffin B. R., Gray W. L. (2003). Channel catfish virus gene expression in experimentally infected channel catfish, Ictalurus punctatus (Rafinesque). J. Fish Dis. 26 (8), 487–493. doi: 10.1046/j.1365-2761.2003.00484.x
Strom M. A., Hsu D. Y., Silverberg J. I. (2017). Prevalence, comorbidities and mortality of toxic shock syndrome in children and adults in the USA: Epidemiology of Toxic Shock Syndrome. Microbiol. Immunol. 61 (11), 463–473. doi: 10.1111/1348-0421.12539
Sturgeon J. P., Segal L., Verma A. (2015). Going out on a limb: do not delay diagnosis of necrotizing fasciitis in varicella infection. Pediatr. Emerg. Care 31 (7), 503–507. doi: 10.1097/PEC.0000000000000255
Suárez-Arrabal M. C., Sánchez Cámara L. A., Navarro Gómez M. L., Santos Sebastián M del M., Hernández-Sampelayo T., Cercenado Mansilla E., et al. (2019). Enfermedad invasiva por Streptococcus pyogenes: cambios en la incidencia y factores pronósticos. Pediatría. 91 (5), 286–295. doi: 10.1016/j.anpedi.2018.12.017
Tiwari V., Clement C., Scanlan P. M., Kowlessur D., Yue B. Y. J. T., Shukla D. (2005). A role for herpesvirus entry mediator as the receptor for herpes simplex virus 1 entry into primary human trabecular meshwork cells. J. Virol. 79 (20), 13173–13179. doi: 10.1128/JVI.79.20.13173-13179.2005
Walton S., Mandaric S., Oxenius A. (2013). CD4 T cell responses in latent and chronic viral infections. Front. Immunol. 4. doi: 10.3389/fimmu.2013.00105/abstract
Watson L., Yun S., Groff J., Hedrick R. (1995). Characteristics and pathogenicity of a novel herpesvirus isolated from adult and subadult white sturgeon Acipenser transmontanus. Dis. Aquat Organ. 22, 199–210. doi: 10.3354/dao022199
Wilson G. J., Talkington D. F., Gruber W., Edwards K., Dermody T. S. (1995). Group A streptococcal necrotizing fasciitis following varicella in children: case reports and review. Clin. Infect. Dis. 20 (5), 1333–1338. doi: 10.1093/clinids/20.5.1333
Ye F., Lei X., Gao S. J. (2011). Mechanisms of kaposi’s sarcoma-associated herpesvirus latency and reactivation. Adv. Virol. 2011, 1–19. doi: 10.1155/2011/193860
Wu L., Qin Z., Liu H., Lin L., Ye J., Li J. (2020). Recent advances on phagocytic B cells in teleost fish. Front. Immunol. Dis. Aquat. Org. 157, 45-59. doi: 10.3389/fimmu.2020.00824
Keywords: WSHV-2, invasive group A streptococcal infection, IGASI, varicella, immunomodulation, latency, aquaculture, streptococcosis
Citation: Quijano Cardé EM, Anenson KM, Yun S, Heckman TI, Jungers HT, Henderson EE, Purcell SL, Fast M and Soto E (2024) Effects of Acipenserid herpesvirus 2 on the outcome of a Streptococcus iniae co-infection in white sturgeon (Acipenser transmontanus). Front. Aquac. 3:1306518. doi: 10.3389/faquc.2024.1306518
Received: 03 October 2023; Accepted: 18 January 2024;
Published: 14 March 2024.
Edited by:
Iddya Karunasagar, Nitte University, IndiaReviewed by:
Norma Estrada, Centro de Investigación Biológica del Noroeste (CIBNOR), MexicoMakesh Marappan, Central Institute of Brackishwater Aquaculture (ICAR), India
Copyright © 2024 Quijano Cardé, Anenson, Yun, Heckman, Jungers, Henderson, Purcell, Fast and Soto. This is an open-access article distributed under the terms of the Creative Commons Attribution License (CC BY). The use, distribution or reproduction in other forums is permitted, provided the original author(s) and the copyright owner(s) are credited and that the original publication in this journal is cited, in accordance with accepted academic practice. No use, distribution or reproduction is permitted which does not comply with these terms.
*Correspondence: Esteban Soto, sotomartinez@ucdavis.edu
†Present address: Hali T. Jungers, Department of Animal Care, Newport Aquarium, Newport, KY, United States