Multifunctional Coatings of Titanium Implants Toward Promoting Osseointegration and Preventing Infection: Recent Developments
- Key Laboratory of Oral Diseases Research of Anhui Province, Stomatologic Hospital and College, Anhui Medical University, Hefei, China
Titanium and its alloys are dominant material for orthopedic/dental implants due to their stable chemical properties and good biocompatibility. However, aseptic loosening and peri-implant infection remain problems that may lead to implant removal eventually. The ideal orthopedic implant should possess both osteogenic and antibacterial properties and do proper assistance to in situ inflammatory cells for anti-microbe and tissue repair. Recent advances in surface modification have provided various strategies to procure the harmonious relationship between implant and its microenvironment. In this review, we provide an overview of the latest strategies to endow titanium implants with bio-function and anti-infection properties. We state the methods they use to preparing these efficient surfaces and offer further insight into the interaction between these devices and the local biological environment. Finally, we discuss the unmet needs and current challenges in the development of ideal materials for bone implantation.
Introduction
With the aging of the population, the incidence of orthopedic diseases has increased, and the use of orthopedic implants has increased rapidly. Titanium and its alloys, exhibiting stable chemical properties and excellent biocompatibility, are often used as materials for orthopedic implants. However, approximately 10% of implants need to be renovated due to their undesirable properties (Kurtz et al., 2005), among which aseptic loosening and infection of the implant are the main reasons for the failure. A study shows that in 337,597 procedures of knee revision, the infectious factors accounted for approximately 20.3% of implant failures, while aseptic loosening accounted for 20.4% (Delanois et al., 2017).
Aseptic loosening is mainly caused by the tiny gaps of prosthesisbone interface (Goodman, 1994; Sundfeldt et al., 2006). In effect of the gravity and pressure, the tiny gap increased and the wear particles will accumulate at the interface and hinder the direct contact between the implant and bone (Schmalzried et al., 1992; Sundfeldt et al., 2006). Accompanied with local inflammation and cascade reactions activated by immune cells, the osteoclast was enriched and bone resorption (Amstutz et al., 1992) occurred, which lead to implant loosening (Valstar et al., 2002). Besides, this process may also cause the transfer of bacteria from oral cavity to implant through the loosened gaps (Zhang, 2014). With the formation of bacteria colonization and biofilm, the undesirable milieu emerged, and even the local immune environment was destroyed, thus making antibiotics invalid to bacteria in the biofilm (Kaestner, 2016). Ultimately, implant failure would happen.
Therefore, aseptic loosening and infection are key risks to orthopedic implants. Long-term stability of orthopedic or orthodontic implants lies on the excellent osseointegration and antibacterial performance, which would do favor to establish a stable microenvironment and in turn restrain biofilm formation. Furthermore, the immune system, which is the first responder to the external microorganism and device, cannot be ignored. Xue et al. reviewed the surface modification of Ti and its alloys with the deep sight on physical and chemical techniques, including plasma spray, chemical vapor deposition, and microarc oxidation (MAO) (Xue et al., 2020). While taking more attention to the service environment of implants, this article mainly focuses on the latest report about how materials interact with the local biological milieu, especially with the microbe, osteocyte, and immune cells.
Prevention of Aseptic Implant Loosening
Osseointegration is a dynamic process during which the cells around implant secrete various cytokines to promote osteoblast recruitment and induce osteogenic differentiation to achieve bone formation (Sims and Martin, 2014). The osseointegration rate determines the bone remodeling and the sealing speed of the interface between bone and implant. A primary strategy is to construct a functional coating with osteoconduction or osteoinduction to promote osseointegration. In this section, three main categories of coatings for promoting osseointegration are introduced (Figure 1; Table 1).
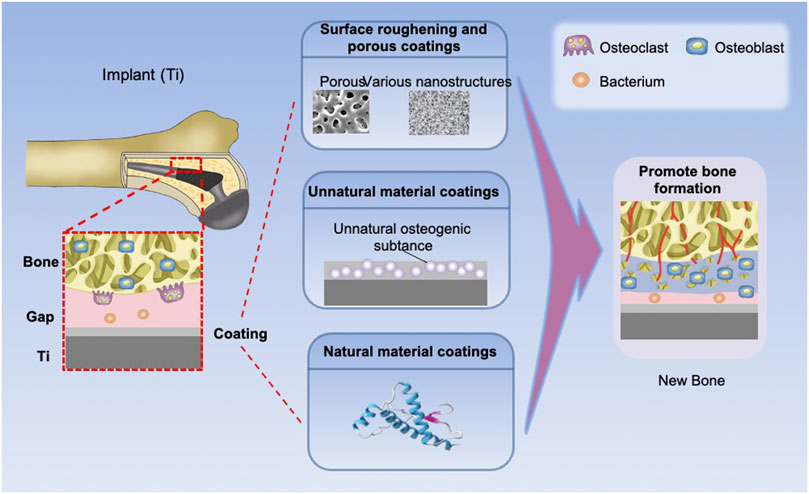
FIGURE 1. Types and effects of osteogenic coatings on titanium implants. The elastic coating can fill the interface gap by expansion. The implants with surface morphology coatings, inactive coatings, and bioactive coatings can promote new bone formation.
Surface Morphology Coatings
Osseointegration rate of orthopedic/dental implants may be attributed to their biochemical property and surface roughness, among which constructing a rough surface on Ti implant that does favor to bone anchoring and provides primary stability is a relatively mature strategy. In clinical trials, the roughened surface could increase the amount of translocated bone particles and provide greater contact area, both leading to outstanding osteogenic responses (Bencharit et al., 2014; Lou et al., 2015). Roughened surfaces were fabricated by various methods. In this section, we classify rough coatings into three categories from the different surface morphologies: porous, various nanostructures, and biomimetic coatings.
Porous Coatings
Porous coatings are one of the most common modifications of titanium surface morphology with a facilitative effect on osseointegration. Novel methods of preparing porous coatings include MAO, dealloying, and 3D printing. MAO can form closely bound porous structures on the titanium surface to promote osteoblast adhesion and proliferation (Zhao et al., 2020a). It was originally used for constructing in-grow oxidation ceramic layers on nonferrous metals (Van et al., 1977). By means of the approved mechanical and anticorrosion properties (Vovna et al., 1998; Gnedenkov et al., 2000), MAO was considered an outstanding method for implant modification to improve their bioactivity (Zhou et al., 2014). However, the sub-microscale pores on the MAO coating limits the in-growth of mature bone tissue (Liu et al., 2011). Some researchers proposed a hybrid treatment of MAO followed with hydrothermal synthesis to form a uniform apatite crystal layer on titanium surface, which promoted the interlocking between implant and bone (Huang et al., 2004). After that, Zhou et al. fabricated double-level porous coatings on Ti plates by three-step MAO (Zhou et al., 2015). Compared with the MAO coating treated with post-heat treatment, this strategy possessed better osseointegration and mineralization ability owing to the OH functional group, which promotes the synostosis between new bone and the implant (Bai et al., 2017). Furthermore, grafting metal ions and compounds is also a common method to improve the osteogenic ability of MAO coating. Some researchers used MAO to prepare manganesetitanium dioxide (Mn-TiO2) microporous coatings on titanium surface, which showed good biocompatibility and osteogenic property (Zhao et al., 2020a). Meanwhile, the Mn2+ release from the coating promotes surface mineralization, which would also favor osteogenesis. Bai et al. prepared a microporous TiO2 coating with MAO and modified with hydroxyapatite (HA) nanoparticles (Bai et al., 2018a). The microarc oxidation coating annealed at 650°C (MAO-650) had excellent physical and chemical properties. The resultant porous coating significantly promoted the proliferation and differentiation of osteoblasts and additionally inhibited the inflammatory reaction, realizing the dual function of immune regulation and bone formation. However, producing the homogeneous and crack-free coatings by MAO is still challenging (Yu et al., 2020b). Dealloying creates porous structures on alloy through a selective corrosion, preferentially dissolving the active alloying elements to form mono or hierarchical porous structures (Song et al., 2018). Wang et al. formed nanoporous structures on Ti-3Zr-2Sn-3Mo-25Nb (TLM) Ti alloy surfaces via dealloying (Wang et al., 2021a). Compared with hierarchical micro/nanoporous surface, mono structure has a higher hydrophilic and protein adsorption capacity, which is more conducive to the early adhesion of osteoblasts.
3D printing is the construction of a three-dimensional object from a digital 3D model, which can accurately control the surface morphology and integrate calcium phosphate, cytokines, and other osteogenic substances on the implant surface. Ma et al. prepared porous titanium alloy scaffolds customized to bone defects by 3D printing (Ma et al., 2018). The HA nanocrystals and collagen fibers induced by the 3D printed porous structure significantly promoted vascularized bone tissue formation in rabbit radius defect models, and the new bone formed a stable combination with the implant. However, in the process of 3D printing, the unmelted or partially melted particles will inevitably attach to the titanium surface to form rigid residues, which can cause chronic inflammation and osteolysis (Zhao et al., 2016). Therefore, removing residual powders on the substrate of 3D printing needs to obtain better biocompatibility. For example, Yu et al. fabricated micro/nanostructures on the surface of 3D-printed Ti-6Al-4V via acid etching and hydrothermal treatment, which played a positive role on promoting the cell proliferation and adhesion (Yu et al., 2020a). In addition, some researchers used a porous titanium scaffold printed by selective laser melting (SLM), which is similar to 3D printing, to modulate the surface topology of the scaffold and then improve the osseointegration (Song et al., 2019).
Coatings of Various Nanostructures
Coatings with different nanostructures, including nanoparticles, nanorods, and nanotubes, were prepared on the titanium surface by techniques such as alkali heat treatment and electrochemical anodization (EA), improving the surface roughness and promoting osseointegration. Some researchers found that the TiO2 nanotubes (TNTs) could increase the adsorption of major proteins involved in osteoblast adhesion, thus enhancing osteogenesis (Grimes and Mor, 2009). Due to the larger surface area, TNTs were used in various osteogenic materials. EA is a surface modification approach that applied an external voltage to the metal plate in the electrolyte to grow a specific structure orderly. Yao et al. fabricated anodic oxide nanotubes and deposited calcium phosphate (CaP) on the surface of Ti and Ti-6Al-4V (Yao et al., 2020). The cell experiment showed that the nanotubes with a higher HA content were suitable for cell adhesion. It should be noted that the fluoride ions and internal stress would cause weak adhesion between TNTs and Ti substrates (Zhang et al., 2015; Cao et al., 2018). Hu et al. performed grain refinement in Ti substrate by high-pressure torsion processing, improving the adhesion between nanotubes and Ti. This strategy also increased the elastic modulus of TNTs and further improved osseointegration (Hu et al., 2020). Moreover, TNTs have also been used as carrier for drug release in recent years because of their simple preparation, controllable size, and high loading capacity (Wang et al., 2017a).
The alkali treatment can produce a homogeneous nanonetwork structure (TNS) on the titanium surface. The study by Zeng et al. showed that this nanostructure enhanced the wettability of Ti to blood and binding to fibrin, which facilitated cell attachment and tissue healing (Zeng et al., 2019). The significant increase in the amount of new bone around the implant also exhibited a greater ability to promote osseointegration. To further improve the adhesion of the surface, Yin et al. coated mussel adhesive protein (MAP) on the TNS, resulting in promotion of new bone growth (Yin et al., 2019). This novel structure has great potential for clinical applications in the dental and orthopedic fields.
Some researchers compared the biological characteristics of different nanostructure coatings. By controlling the duration of the steam-hydrothermal treatment, the researchers prepared HA nanoparticles and nanorods in different sizes on the surface of the MAO micropores of titanium (Bai et al., 2018b). By contrast, the nanoparticles were more conducive to osteogenesis due to the closer resemblance to the structure of natural bone and their larger specific surface area. Huang et al. prepared nanowire-like and nanometal-like topography on the titanium surface using different concentrations of NaOH, both of which showed good biocompatibility compared with untreated titanium, with Ti-5 showing the best osteogenic differentiation ability (Huang et al., 2019b).
Other Morphology Coatings
Recently, some researchers have found that surfaces with well-defined patterns exhibit higher levels of cell adhesion, proliferation, and differentiation (Chang et al., 2016). Zhang et al. fabricated patterned titanium coatings using a template-assisted plasma spraying technique (Zhang et al., 2018d). The surface was more conducive to the polarization of macrophage to anti-inflammatory type M2 and induced osteoblasts to exhibit more mineralization nodules. Inspired by the skin structures of the tree frog toe pads and the adhesion of the corrugated ridges on the scales of Morpho butterfly wings, Li et al. generated micro-hexagons and nano-ripples on titanium surface by microsecond laser (Li et al., 2020a). This structure provided microscale space for cell behaviors, enhancing mechanical interlocking at the boneimplant interface. Similarly, some researchers have designed biomimetic coatings inspired by bone structure. MacBarb et al. compared the response of osteoblasts on the 3D-printed trabecular-like titanium implant surface, the titanium plasma spray-coated surface with and without nanocrystalline HA coating (MacBarb et al., 2017). The results showed that 3D-printed trabecular-like surface could promote the earlier proliferation of osteoblasts and higher calcium production than other surfaces, holding promise for improving the osseointegration of orthopedic implants.
Unnatural Material Coatings
Since the composition of HA is similar to bone, it is widely used in bone tissue engineering (LeGeros, 2002). However, due to the mismatch in coefficients of thermal expansion between metal and HA (Sun et al., 2001), there are potential concerns about the low strength of the bond between implant and coating. Ke et al. prepared gradient HA coatings on a titanium surface by Laser Engineered Net Shaping (LENS) and plasma spray deposition technology (Ke et al., 2019). The gradient coating solved the problem of weak interfacial bond, prevented the diffusion of metal ions effectively, and improved the surface osteoconductivity. Besides HA, various metal ions (Ca2+, Sr2+, Mg2+) have been demonstrated to possess the property to promote osseointegration (Xu et al., 2020). One example of these coatings is a biomimetic mesoporous coating doped with strontium (MPs-Sr), proposed by Zhang et al. (2018a). The mesoporous structure and the doped Sr significantly upregulated the attachment and spreading of preosteoblasts, indicating their ability to promote osseointegration and new bone formation. Huang et al. doped Mg elements with osteogenic ability into micro/nanoporous coatings by MAO and hydrothermal treatment to form MgO nanorods, which were later heat treated to convert the nanorods into nanoparticles, further improving the biological properties of the coatings (Huang et al., 2018b). Others reported the nanostructured Mg(OH)2 films on Ti surface fabricated by hydrothermal treatment (Yao et al., 2021). The animal experimental results and genome expression analysis indicated that the coating with Mg ion release could activate BMP-4-related signaling pathways, thus promoting bone formation and regeneration. At the same time, the alkaline environment has also been shown to favor the deposition of HA, leading to further osteogenesis, which has been proved by various studies (Chen et al., 2020b; Zhao et al., 2020b). Furthermore, researchers prepared the Cu-containing surface to induce macrophages to polarize to M1 phenotype, which could release appropriate pro-inflammatory cytokines to form an inflammatory microenvironment, thereby promoting osteogenic differentiation of MSCs (Huang et al., 2018a).
Besides, non-metallic substances have also been explored for promoting osseointegration. Graphene is a novel nanomaterial with excellent mechanical properties. It is increasingly used in the biomedical field owning to their excellent electronic, optical, mechanical, and chemical properties. Moreover, graphene derivatives such as reduced graphene oxide (rGO) can enhance protein adsorption and cellcell interactions, and have extraordinary osteoinductive ability (Jin et al., 2015). Kang et al. used meniscus-dragging deposition technology to coat rGO on the titanium surface to fabricate an rGO-Ti substrate (Kang et al., 2021). In vitro experimental results showed that the coating enhanced cell proliferation and significantly promoted matrix mineralization. Inspired by the physiological function of bone sialoprotein during osteogenesis, Long et al. synthesized a polymer from glutamic acid and dopamine methacrylamide via reversible addition-fragmentation chain transfer polymerization and immobilized it on a titanium base by means of catechol pendants on the polymer chain (Long et al., 2020). The coating could induce calcium phosphate (CaP) formation with a Ca/P ratio close to that of natural hydroxyapatite, thus effectively promoting mineral deposition. In addition, the coating could promote the adhesion and proliferation of osteoblasts.
Natural Material Coatings
Cellular active substances such as extracellular matrix proteins, growth factors, and chemokines are hotspots of research for osseointegration, and their application on the surface of orthopedic prostheses can effectively improve the biocompatibility of the surface and promote osseointegration. These bioactive coatings could be classified into two parts: 1) directly loaded with osteogenic factors; 2) loaded with immunomodulatory factors. In this part, we will introduce the new progress of bioactive coating in recent years and discuss their application in detail.
Coatings Loaded with Osteogenic Factors
Stem cell encapsulation and extracellular vesicle loading that endow surfaces with bioactive property for rapid osteogenesis are two main strategies in constructing bioactive surfaces. Mesenchymal stem cells (MSCs) have been widely used in research and clinic because of their self-renewal potential and multilineage differentiation (Mushahary et al., 2018). Bone marrow is the prevailing source of MSCs and bone marrow mesenchymal stem cells (BMSCs) have a potential to differentiate into osteoblasts (Pittenger et al., 1999). Bai et al. encapsulated BMSCs, bone morphogenetic protein-2 (BMP2), and other bioactive substances in hydrogels and formed a three-dimensional inorganicorganic supramolecular bioactive interface on a porous titanium alloy scaffold (Bai et al., 2020). Osteogenic differentiation of BMSCs was induced by the continuous release of BMP2. With the degradation of the hydrogel, bone tissue grew into the pores of the scaffold to achieve good bone integration. However, since the harvesting procedure with general anesthesia limits the supply of BMSCs (Li et al., 2016), adipose tissue stem cells (ADSCs), another candidate for bone engineering that is easy be obtained, are attracting more attention (Kolaparthy et al., 2015). The main way to exert the benefit of MSC is to secrete extracellular vesicles (EVs) to promote tissue repair and regeneration (Vizoso et al., 2017; Mushahary et al., 2018). On one hand, MSC-EVs can carry and transfer various cargo such as regulatory miRNAs, growth factors, and cytokines. On the other hand, the membrane of EVs contains bioactive signaling molecules to obtain protection (Malda et al., 2016; Tsiapalis and O’Driscoll, 2020). Based on physisorption of fibronectin, Chen et al. immobilized adipose-derived stem cell-derived extracellular vesicles (ADSC-EVs) onto the titanium surface, which enhanced osteoblast compatibility and osteoinduction activity (Chen et al., 2020a).
Osteogenesis-related molecular loading is another strategy for constructing bioactive surface. Growth factors and cytokines could promote osteogenesis by upregulating the level of osteogenic differentiation-related genes or activating osteogenic-related signal pathways. Bone morphogenetic proteins (BMPs) are the widely used cytokines to confer osteoinductivity (Carson and Bostrom, 2007). However, a burst release will decrease the osteogenic effect (Haidar et al., 2009a; Haidar et al., 2009b). Teng et al. prepared a porous structure on the titanium surface by 3D printing and MAO to endow the coating with osteogenic property, calcium, phosphate, and BMP-2 that was grafted onto the surface (Teng et al., 2019). The results showed that the continuous BMP-2 release sustained for more than 35 days, and enhanced the osseointegration between the implant and surrounding bones. Compared with BMP-2, BMP-9 has higher osteoinductive differentiation ability (Kang et al., 2004; Souza et al., 2018). Zhu et al. implanted thermosensitive collagen and BMP-9 into porous titanium, which could release BMP-9 via temperature-controlled sustained release (Zhu et al., 2021). The thermosensitive collagen degraded slowly at 37°C and the released BMP-9 significantly promoted osteogenesis around the implant. Besides, sympathetic nerves are also widely distributed in bone tissue and can regulate bone formation. Nerve growth factor (NGF) has been shown to enhance the activity of osteoblasts and promote and mineralization after implantation (Lee et al., 2015). The NGFchondroitin sulfate/hydroxyapatite coating (NGF-CS/HA-coating) prepared by modified biomimetic method was placed in the mandible of beagles (Ye et al., 2021). The results showed that the coating significantly upregulated the level of osteogenesis differentiation-related genes in the mandible, promoting the differentiation of osteoblasts and nerve cells in the early bone binding and the bone healing around the implant.
Coatings Loaded with Immunomodulatory Factors
As a foreign body, the implant inevitably leads to a series of immune responses, which mainly arise from the macrophage activation that would reduce the service life of implants. In addition, as mentioned previously, the wear particles produced in the gap of implant and bones can also aggravate inflammatory reaction, and cause a dynamic imbalance between osteoblasts and osteoclasts, which eventually lead to bone resorption and implant loosening (Zhou et al., 2021). Therefore, tuning immunoreaction to keep an appropriate immune environment is beneficial to improve osseointegration and reduce loosening. There are many ways to regulate immune microenvironment. Previous studies have shown that different nanostructured Ti can induce different macrophage responses, which can affect the osseointegration (Wang et al., 2018b). Alternatively, some coatings loaded with immune factors can also regulate the immune response, such as interleukin 4 (IL-4) (Li et al., 2020b) and complement activating immunoglobulin (Harmankaya et al., 2012). Li et al. sprayed graphene oxide (GO) on Ti and loaded IL-4 to construct a GO/IL-4 coating for regulating macrophage-related inflammation (Li et al., 2020b). In the process of acute inflammation, type 1 macrophages (M1) can produce fibers to protect the host, while type 2 macrophages (M2) can inhibit the development of inflammation and promote the growth of osteogenic factors (Franz et al., 2011). The release of IL-4 from GO/IL-4 coating induced the macrophage polarization to the M2 phenotype, weakened the inflammatory response, and promoted osteogenesis. Another method for immune regulation is to regulate the balance between osteoblasts and osteoclasts. Liu et al. conjugated an osteogenic growth peptide (OGP) with N-acetylcysteine (NAC) to synthesize a multifunctional peptide OGP-NAC and then employed OGP-NAC to titanium (Liu et al., 2019). Such an OGP-NAC coating could inhibit the important transcription factors for osteoclastogenesis, such as MAPK, NF-κB, and NFAT c1, and promote osteoblast proliferation and differentiation.
Prevention of Implant Infection
Infections within 3 months are considered as early postoperative infection, while delayed (or subacute) infection occurs after 3–24 months and late infection more than 24 months later (Montanaro et al., 2011; Zimmerli, 2014). Early infection is usually caused by pathogens such as Staphylococcus aureus at the surgical site (Trampuz and Widmer, 2006). After operation, patients need systemic antibiotic treatment to prevent infections, but the rising antibiotic resistance of bacteria can make the existing antibiotics noneffective (Park et al., 2019). Also, the concentration of antibiotics in the focus site is insufficient, resulting in the rapid proliferation and secretion of extracellular polymers to form a biofilm after some pathogens gather and adhere to the implant surface (Gristina and Costerton, 1985). Exopolysaccharides of the biofilm can hinder and delay the penetration of antibiotics, and the quorum sensing of bacteria in the biofilm regulates the development of the biofilm to resist the host immune defense, thus making the biofilm a barrier to antibiotics (Kaestner, 2016). Therefore, the ideal antibacterial coating is supposed to remove or kill the pathogens once the primary contact occurs, thus preventing the formation of the biofilm. Orthopedic implants would maintain for a long time in the organism, so late infections would happen if no defensive measures were taken (Del Pozo and Patel, 2009). At this stage, the biofilm formed, resulting in poor antibiotic treatment (van de Belt et al., 2001), so it is necessary for the implant surface to provide long-term antibacterial properties.
The key to preventing infection is to inhibit the adhesion of microorganisms, but long-term infection can still be a risk to the implant because of continuous pathogens. Therefore, the anti-infective implants should target early infection and long-lasting antibacterial agents. This section introduces preventive and treatment strategies of infections (Figure 2; Table 2).
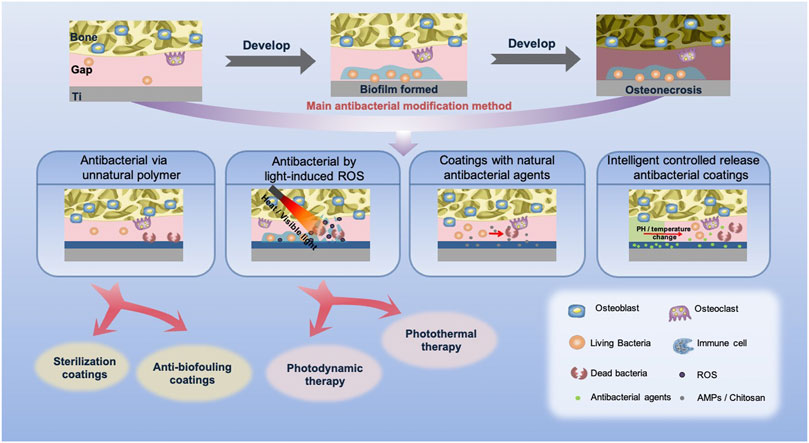
FIGURE 2. Types and effects of antibacterial coatings of titanium implants. After implantation, biofilm may be formed, leading to osteonecrosis in general. At present, to avoid infection, the coatings with inactive polymers, light-induced ROS and bioactive antibacterial agents, and the intelligent controlled release antibacterial coatings are the main antibacterial methods.
Antibacterial via Unnatural Polymers
Some metal ions and polymers are widely used as antimicrobial agents coating the implants. In this section, we divide these unnatural antimicrobial agents, such as metal ions, non-metallic ions, and other synthetic polymers, into sterilization coatings and anti-biofouling coatings to describe them in detail.
Sterilization Coating
Antibacterial Metal Ions
Clinically, antibiotics have been widely used in the treatment of implant infection, but its clinical effect is limited due to the drug resistance of bacteria and the narrow antibacterial spectrum of single antibiotics. Therefore, metal ions with broad-spectrum antimicrobial effects that do not lead to severe resistance are deemed potential antibiotic substitutes for implant surface modification, such as Ag+, Cu2+, and Zn2+ (Sundfeldt et al., 2006). Chen et al. integrated silver nanoparticles on the surface of titanium by in situ reduction of dopamine (Chen et al., 2016). The results showed that the surface had excellent antibacterial activity, but Ag also showed strong cytotoxicity. To reduce the cytotoxicity, Guo et al. prepared a poly-l-lysine (PLL)/sodium alginated (SA)/PLL self-assembled coating on the surface of Ti, then loaded nano-silver and induced mineralization in simulated body fluid (Guo et al., 2020). The antibacterial results showed the coating effectively inhibited the adhesion of bacteria. At the same time, PLL/SA/PLL coating can greatly improve the cytocompatibility and reduce the cytotoxicity. Another potential idea is to decrease the cell cytotoxicity via adjusting the concentration of antimicrobial agents. Wang et al. systematically determined the osteogenesis of dopamine silver-loaded coatings prepared at different pH (4, 7, 10) and different Ag+ concentrations (0.01, 0.1 mg/ml) (Wang et al., 2021b). The results showed that the pH 10/0.1 group had obvious osteogenesis in bacterial environment, which may be due to its strong antibacterial properties to kill the surrounding bacteria and then promote mineralization to achieve good osseointegration. Also, other researches incorporated zinc-doped coating on titanium surface (Ye et al., 2020). The amorphous coating with ZnO bond acts as the Zn2+ donor and generator of reactive oxygen species (ROS), which could trigger the S. aureus killing. In addition, although Zn2+ and ROS can cause osteoblast damage, the surface did not show noteworthy cytotoxicity.
Since the antibacterial and cytotoxicity of Ag+ are both dose dependent, many researches focus on doping adjuvant antibacterial substance. Zhang et al. deposited HA nano-powder mixed with Ag and ZnO on a titanium surface by laser cladding (Zhang et al., 2018c). The combined release of Ag+ and Zn2+ for more than 5 weeks showed a long-term effective antibacterial activity. Moreover, with the assistance of Zn+, the antibacterial efficiency was significantly increased and cytotoxicity of the Ag+ contained coating was reduced. To reduce the negative performance of Ag+, Xie et al. prepared a hybrid coating containing chitosan (CS), dopamine (PDA), HA, and nano-silver. The double chelation of PDA and CS achieved long-term release of silver and a continuous bacteriostatic effect. It is worth noting that this coating exhibits significantly osteogenic potential in both in vitro and in vivo tests (Xie et al., 2019). However, the antibacterial substances released by this kind of coating gradually decrease over time, and with the adhesion of proteins and host cells, the antibacterial property will decrease. Therefore, the long-term and stable antibacterial effect is still a challenge in the development of antimicrobial coatings.
Non-Metallic Antibacterial Substances
In addition to antimicrobial metal ions, some non-metallic compounds and biomolecules are also prominent candidates for fabricating antibacterial coatings, among which iodine is found to have a wide antibacterial spectrum and do not develop drug resistance (Tsuchiya et al., 2012). Shirai et al. described the novel use of povidone-iodine as the electrolyte to form the iodine coating on the Ti surface (Shirai et al., 2011). After implanting into the femora of rabbits, the implants significantly inhibited the formation of biofilm. Meanwhile, since iodine is an important component of the thyroid hormone, the coating has important biological safety. Since then, Kabata et al. treated 28 patients with iodine-supported implants (Kabata et al., 2015). None of the patients showed infection after 1-year follow-up, indicating that the coating could effectively prevent postoperative infections. Others used the same method to prepare iodine coatings and investigated temporal changes in iodine for implants using animal models for 1 year (Kato and Shirai, 2016). The results showed that the implants retained 30% of the iodine content after 1 year, which proved to have sufficient antibacterial properties. Similarly, chlorhexidine is a commonly used clinical antibacterial agent (Kim et al., 2019), which can be adsorbed on the surface of bacteria to destroy membrane permeability, thus sterilizing. Wang et al. covalently grafted chlorhexidine onto porous titanium through the coupling ability of aminosilane and glutaraldehyde (Wang et al., 2019). The results showed that chlorhexidine could inhibit the adhesion and proliferation of bacteria. What is more, in the cell-bacterial competition environment, this surface is of great help to osteoblast adhesion.
Anti-Biofouling Coatings
The anti-biofouling coatings can change the hydrophilicity of the surface, thus repelling the adhesion of bacteria and proteins. Polyethylene glycol (PEG) is the most common anti-biofouling material that is highly hydrophilic, which can constitute a wide exclusion volume that repels bacterial contamination (Tanaka et al., 2010). Guo et al. deposited both polyphenol tannic acid and PEG on the titanium surface, providing good inhibition of non-specific adsorption of proteins, adhesion of bacteria and platelets, and prevented biofilm formation (Guo et al., 2021). However, since its rejection is non-specific, the coating repels contamination while also inhibiting cell adhesion (Tanaka et al., 2010). The researchers introduced RGD sequence and antimicrobial peptides onto the PEG layer to obtain both cell adhesion and antimicrobial properties (Hoyos-Nogués et al., 2018).
It should be noted that PEG is easily oxidized in biological environment due to its poor stability (Guo et al., 2021). Zwitterionic copolymer is a hydration layer that can form a close bond on the surface of the material, and it is an ideal substitute for PEG (Xie et al., 2012). Huang et al. prepared a phosphonatezwitterionic block copolymer from sulfobetaine methacrylate and phosphonate/phosphonic methyl methacrylate, and immobilized it on titanium sheets (Huang et al., 2017). The results showed that this copolymer effectively inhibited protein adsorption, platelet adhesion, and bacterial adhesion, significantly improving the anti-biofouling ability of the titanium base. In addition, Salvagni et al. applied elastin-like recombinamer to modify the titanium base (Salvagni et al., 2014). The coating was capable of reducing serum-protein adsorption and was biologically active compared with the untreated titanium base, laying the groundwork for future medical applications. However, non-specific anti-biofouling still needs to be addressed.
Antibacterial by Light-Induced ROS
Reactive oxygen species (ROS) can destroy the polysaccharides, which is the main component of the external surface of biofilm, thus representing bactericidal effect to drug-resistant bacteria (Konopka and Goslinski, 2007). Photosensitizers such as TiO2 and ICG can produce ROS when exposed to light. This section introduces the new progress in antimicrobial activity through light-induced ROS.
Photodynamic Therapy
Photodynamic therapy can destroy the biofilm by creating free radicals and then disrupting the biofilm (Konopka and Goslinski, 2007). Sharab et al. combined this therapy with physical methods and applied it to the combating with biofilm of Streptococcus mutans, showing that photodynamic therapy can weaken biofilms of different maturity levels (Sharab et al., 2020). Titanium dioxide (TiO2) is a stable photocatalyst, which can produce ROS to kill bacteria under ultraviolet (UV) irradiation (Carp, 2004), but UV is harmful to the human body. To solve this problem, Nagay et al. prepared nitrogen and bismuth doped TiO2 coatings by plasma electrolytic oxidation of titanium (PEO), which produces ROS to kill bacteria under visible light (Nagay et al., 2019). Others used PEO to prepare TiO2 nano-ceramic coating on titanium surface to kill bacteria and decompose organic residues under visible light irradiation, and effectively prevent the occurrence of peri-implant inflammation (Wu et al., 2019). However, since this strategy is light dependent, they are only fit for oral implants.
Photothermal Therapy
In recent years, photothermal therapy (PTT) based on near infrared radiation has become a hot spot in the field of nanomedicine because it is less traumatic than UV light, allows deep tissue penetration, and has high selectivity (Cheng et al., 2014). PTT can destroy bacterial integrity or biofilm structure through local warming (Hu et al., 2017; Wang et al., 2018a; Li et al., 2018; Qiao et al., 2019), but its unselective heating may adversely affect the surrounding tissue (Lei et al., 2016; Tan et al., 2018). Therefore, PTT has a higher application potential in combination with other antibacterial strategies. Zhang et al. combined indocyanine green (ICG) with mesoporous polydopamine (mPDA) to form a multi-functional coating on titanium surface, using mPDA to convert light energy into heat for microorganism killing, and the ICG can also produce ROS under light radiation to destroy the bacterial cell wall (Yuan et al., 2019). This strategy can be remotely controlled to eradicate the biofilm formed on the surface of implants in vivo, avoiding debridement surgery or invasive treatment, and does not cause side effect to surrounding tissue. Song et al. used dopamine and ferrocene (PDA-Fc) to modify TiO2 nanorods as an antibacterial coating on titanium surface (Song et al., 2020). Local high temperature was attained by photothermal transformation of PDA, and ROS were produced by PDA-Fc redox reactions, which achieve a synergistic and more efficient bactericidal performance.
Photodynamic TiO2 is not only a typical photosensitizer but also sound sensitizers, so ROS can be produced by ultrasound-triggered electron hole separation (Harada et al., 2011). Su et al. proposed a photoacoustic therapy, which produced oxygen defects on titanium implants by sulfur doping (Ti-S-TiO2-x), to endow the implants with good sonodynamic and photothermal properties (Su et al., 2020). The killing rate of S. aureus was as high as 99.995% after 15 min of exposure to near-infrared light and ultrasound. In addition, the implant showed good stability, and the structure and properties did not change after soaking in water for 6 months.
Antibacterial via Natural Antibacterial Agents
Antimicrobial peptides (AMPs) are the most widely studied antibacterial agents in recent years, which are natural anti-infective agents. AMPs are general constituted by two parts: one part is composed of positively charged residues like arginine and lysine, which will do favor to the initial contact; another are hydrophobic residues that may penetrate into the cell to bind intracellular molecules, thus killing the microorganism (Hancock and Sahl, 2006). Shi et al. loaded AMP, Tet213, on titanium via layer-by-layer technique, which effectively inhibited the early S. aureus biofilm formation (Shi et al., 2015). Furthermore, this multilayer coating could release AMP continuously for up to a month. Since the function of the AMP is closely related to their conformation, the surface grafting based on covalent bond would inevitably reduce their antibacterial efficiency (Steckbeck et al., 2014). Therefore, mild methods were developed to prepare AMP-loaded coatings without reducing their activity. The antimicrobial peptide GL13K extracted from parotid secretory protein also showed potent antimicrobial activity. To improve the stability, the researchers replaced the lysine residues in GL13K with d-amino acids, generating an all-d-amino acid version of GL13K (D-GL13K), which reduced the solubility of the peptide (Hirt and Gorr, 2013). Based on this, Acosta et al. developed an extracellular matrix system based on elastin-like recombinant (ELR), an extracellular matrixinspired polymer, to attach the D-GL13K to a titanium surface (Acosta et al., 2020). On the one hand, ELR coating could reduce the adsorption of non-specific proteins (Salvagni et al., 2014), thereby giving the surface antifouling performance. On the other hand, D-GL3K has good bactericidal activity and can reduce the adhesion of bacteria and the formation of biofilm.
Similarly, chitosan, a natural polymer, is widely used in tissue engineering due to its good biocompatibility (Ordikhani and Simchi, 2014). Chitosan is a cationic macromolecule that can bind negatively to cell membrane of bacteria that exhibit antibacterial property (Raafat and Sahl, 2009), but the antibacterial activity is too weak that limits its use on implants. To construct the appropriate antibacterial coatings, other antibacterial agents like AMPs are used to develop an antibacterial composite (Raghavendra et al., 2017; Tabesh et al., 2019). López et al. use chitosan (CH) and hyaluronic acid (HA) polyelectrolyte multilayers (PEM) loaded with β-peptide (a kind of antimicrobial peptide), prepared by layer-by-layer electrostatic assembly technology (Rodríguez López et al., 2019). By the strong chemical cross-link of CH/hyaluronic acid thin films, a prolonged β-peptide retention about 8 weeks was obtained and significant inhibition of S. aureus biofilm was achieved. In addition, the multilayer film retained its antibacterial activity after five attacks by five separate bacterial over 18 days. Such a strategy that can maintain a long-term antibacterial property is critical for the viability of the Ti implants.
Intelligent Controlled Release Antibacterial Coatings
As mentioned previously, despite the potent antibacterial activities, certain cytoxicities of antimicrobial agents remain a concern. Since the cytoxicity is dose dependent, the control release that can tune local concentration of the antimicrobial agents and prolong the effect time is a significant direction. At present, the control release behavior mainly relies on pH and temperature to realize the intelligent release of antibacterial agents (Li et al., 2021a; Sang et al., 2021). As mentioned earlier, antibiotics not only cause drug resistance of bacteria but their rapid release can also produce cytotoxicity. In this regard, some researchers prepared controlled release antibiotic coatings to selectively release drugs when the microenvironment was changed. Silk fibroin that can respond to slightly acidic environment is regarded as a new candidate for drug loading and release (Hassani Besheli et al., 2017). Sang et al. coated a layer of silk protein on the surface of Ti, and then loaded gentamicin (Sang et al., 2021) on it. Bacteriostatic ring tests in vitro and in vivo showed that the coating exhibits a stable release behavior of gentamicin, and the release rate of gentamicin in acidic environment was faster than that in alkaline environment, which achieved the intelligent release. Another mechanism for intelligent controlled release is to use the heating effect caused by infection. Li et al. layered a thermosensitive chitosan-glycerol-hydroxypropyl methyl hydrogel (CGHH) on TiO2 nanotubes (Li et al., 2021b). In vitro antibacterial test showed that CGHH had almost no antibacterial activity against E. coli and S. aureus. However, the results of subcutaneous infection animal models showed that under the high temperature caused by an infection in vivo, CGHH could release a large amount of glycerin, thus suggesting excellent antibacterial properties.
Strategies of Both Preventing Aseptic Loosening and Infection
Promoting osseointegration along with the prevention of infections is the key factor of the orthopedic implants, but most of the current implant coatings exhibit only one of the properties. Since many antibacterial surfaces not only inhibit bacterial colonization but also do damage to mammalian cells, strategies to prepare host-friendly devices was the main direction of the orthopedic implants. In this review, several approaches that address the two properties at one surface were displayed, and we classify the coatings into four categories based on their characteristics (Table 3): 1) rough surface coating loaded with antibacterial substance; 2) osteogenic antibacterial polymer coatings; 3) photoantibacterial and osteogenic coatings; 4) multifunctional coatings with single material.
Roughening/Porous Surface Loaded with Antibacterial Substance
The roughening surface facilitates the attachment of osteoblasts; therefore, the combination of antibacterial agents with porous surface is another general strategy to construct a dual-functional coating. As mentioned previously, we introduced the controlled release antibacterial coating. Some researchers doped osteogenic material into the controlled release antibacterial coating to achieve the multifunction surface. Shen et al. deposited magnesium/zinc on the surface of porous titanium and coordinated with 2,5-dihydroxyterephthalic acid (DHTA) to form a hybrid magnesium/zinc-metal organic framework (Mg/Zn-MOF74) coating (Shen et al., 2019). The dissolution of MOF74 coating was accelerated in an acidic environment. The gradual release of DHTA and Zn2+ formed an alkaline microenvironment around the implant to kill bacteria and promote osteoblast proliferation. Mg2+ improves osteoblast activity and anti-inflammatory gene expression. In vivo results showed that the coating had high antibacterial and anti-inflammatory properties at the initial stage of implantation, and greatly improved the new bone formation around the implant. Gao et al. fabricated a titanium dioxide nanospike coating on the titanium surface and immobilized cationic polypeptides on the surface by coordination (Gao et al., 2020). The results showed that this coating was able to rapidly kill pathogenic bacteria, inhibit biofilm formation for up to 2 weeks, and promote the formation of HA.
3D printing was introduced previously as a method to construct a given morphology on implant that promotes osseointegration. Embedding antibacterial ions into 3D-printed coatings is one of the methods to construct multi-functional coatings. Hengel et al. closely embedded silver and zinc nanoparticles into a 3D-printed porous titanium oxide layer. The porous structure could promote the adhesion and the released Zn2+ and Ag+, which would do favor to promote osteogenesis and achieve an antibacterial effect, respectively (van Hengel et al., 2020a). In addition, this synergistic mechanism apparently reduced the toxicity of Ag to host cells.
To achieve better bone-promoting properties, researchers combined other bone-promoting substances with antibacterial agents on the modified surface. Antibacterial agents that can be directly combined with these bone-promoting surfaces can be utilized to achieve multifunctional coatings. He et al. coated polydopamine, cationic antimicrobial peptide LL-37, and phospholipids on a MAO modified titanium surface, which showed good cytocompatibility to mesenchymal stem cells and osteoblasts (He et al., 2018). LL-37 killed bacteria by blocking the expression of bacterial related genes and enhancing immune response under the controlled release of phospholipids. Others hydrothermally grew ZnO nanorod arrays on titanium and modified them by autopolymerization of dopamine and covalent immobilization of RGDC peptides (Li et al., 2017). The results showed that ZnO nanorod arrays could kill bacteria by producing ROS to destroy the cell membrane, and Zn2+ that penetrate the cell membrane could inhibit their metabolism. What is more, Zn2+ bound with dopamine could promote cytocompatibility and minimize possible cytotoxicity via an antioxidant effect to scavenge ROS.
Osteogenic Antibacterial Polymer Coatings
A direct approach to fabricate dual-functional orthopedic implants is to co-immobilize antibacterial agents and bone-promoting substances on Ti implants. The most common strategy is to load osseointegration coating and then graft antibacterial agents on the modified surface. For instance, dimethylaminododecyl methacrylate (DMADDM) is a new antibacterial agent whose amino group and long alkyl chain can destroy the bacterial membrane, thus exerting a strong inhibitory effect to bacteria and fungi, including drug-resistant strains (Wang et al., 2017b; Cheng et al., 2017; Rego et al., 2017). To endow a HA modified surface with antibacterial property, DMADDM was introduced with the aid of polydopamine (PDA) (Zhou et al., 2020). DMADDM was gradually released in the first 4 weeks after implantation, exhibiting strong inhibition to the adhesion and proliferation of pathogenic bacteria. After 4 weeks, the samples induced osteogenic differentiation attributed to the bone-like HA. With the aid of an alternative approach, antimicrobial agents and osteogenic materials can be combined and loaded on Ti. Sung et al. functionalized titanium implants with poly(glycidyl methacrylate) (PGED) polymer brushes, and covalently coupled quaternized polyethyleneimine QPEI, an efficient cationic antibacterial agent rich in amino groups, and alendronate, which has a high affinity for bone minerals, to the polymer brushes (Sun et al., 2019). The coating did inhibit bacterial infection in the early stage and enhanced osseointegration in the later stage. Zhang et al. prepared Ca- and Si-based ceramic (CS) nanorod coatings on Ti using MAO technique, and loaded AMP onto CS coatings with the aid of fluorous-cured collagen scaffolds (Zhang et al., 2021). The release of Ca2+ and Si2+ enhanced osseointegration and the collagen scaffold loaded with AMP had a good antimicrobial effect while promoting cell adhesion.
It is worth noting that some researchers have achieved antibacterial and osseointegration by modulating the microenvironment around the implant. Tao et al. prepared a multifunctional hybrid coating through depositing the zeolitic imidazolate frameworks-67 (ZIF-67) and an osteogenic growth peptide (OGP) on nanotubes (Tao et al., 2021). Under the acidic environment, the coating gradually decomposed, releasing cobalt ions and forming an alkaline microenvironment to kill bacteria. At the same time, OGP inhibited the inflammatory response and promoted differentiation of MSCs, facilitating osseointegration during the late implantation phase.
Photoantibacterial and Osteogenic Coatings
Photosterilization includes photodynamic therapy and photothermal therapy mentioned earlier, which can effectively eradicate bacteria in the biofilm. The application of photosensitizer and osteogenic material on the surface of titanium can promote osseointegration after sterilization. Typical types of materials have been explored for multifunction surfaces, including TiO2. To the best of our knowledge, TiO2 has great photocatalytic properties. After surface treatment, TiO2 can not only be used as a photosensitizer but also promote osseointegration. However, TiO2 can generate ROS to eradicate bacteria under ultraviolet light irradiation, but it cannot be triggered by near-infrared light (Zhang et al., 2020). Han et al. chose photosensitizer MoS2 with broader spectral responses to modify the surface of TiO2 and used MAO and hydrothermal treatment to construct the composite collagen/polydopamine/MoS2-TiO2 (CPM-TiO2) coating on the surface of titanium (Han et al., 2021). Under the combined action of photodynamic and photothermal therapy, S. aureus in biofilm could be quickly eradicated both in vivo and in vitro. The collagen in the coating was shown to promote the adhesion and proliferation of osteoblasts. Zhang et al. prepared a titanium dioxide nano-shovel/quercetin/l-arginine coating and doped ytterbium (Yb) and erbium (Er) on the TiO2 nano-shovel array (Zhang et al., 2022). Under near-infrared II light irradiation, Yb and Er promoted the production of ROS, which could kill bacteria. Meanwhile, ROS catalyzed the release of nitric oxide (NO) free radicals from l-arginine, promoting angiogenesis and osseointegration. The nano-shovel structure and quercetin coupled to the surface via organosilanes promoted osteogenic differentiation of BMSCs while NO promoted angiogenesis and osseointegration. In addition, the complex of generated electrons and holes by TiO2 reduces its photocatalytic properties and limits the antibacterial effect (Anandan et al., 2013). Graphdiyne enhances the catalytic effects of metals and possesses osteoinductive potential (Gu et al., 2014). Based on this, the researchers synthesized TiO2/GDY nanofibers by electrostatic force (Wang et al., 2020). The combination of the two increased the production of photocatalytic ROS and prolonged the antibacterial effect, inhibiting the formation of methicillin-resistant S. aureus biofilms, while also promoting bone tissue regeneration. Red phosphorus (RP) can also be used for photoantibacterial coatings, which have efficient photothermal properties and biodegradability, and can exist for a long time in human body with no toxicity (Latiff et al., 2015). Huang et al. used IR780 as photosensitizer preparing a RP/IR780/RGDC coating on titanium surface (Huang et al., 2019a). In the experiment, IR780 produced ROS under irradiation of 808 nm laser and improved the temperature sensitivity of bacterial biofilm; RP produced photothermal effect by near-infrared (808 nm) radiation of 50°C, which cooperated with ROS sterilization without causing tissue damage; the existence of RGDC promoted osteogenesis.
Multifunctional Coatings with Single Material
It is worth noting that, in addition to combining antibacterial agents and osteotropic substances on titanium surface, some researchers have achieved multifunctional effects by using only one substance. Flavonoids from plants have been shown to have the ability to inhibit the formation of biofilm and bacterial toxicity, and to enhance the efficacy of antibiotics by blocking the efflux pump to reverse the antibiotic resistance of bacteria (Górniak et al., 2019). Among them, the flavonoid quercitrin not only had antibacterial properties but also promoted bone and anti-fibrosis (Córdoba et al., 2015; Gomez-Florit et al., 2016). The researchers prepared quercitrin coating on the surface of porous titanium alloy, and it turned out that the quercitrin coating was able to prevent bacterial adhesion, inhibit bacterial activity, and improve the efficiency of surface osteogenesis (Llopis-Grimalt et al., 2020). Another novel method is to culture Lactobacillus casei on the surface of a heat-treated Ti to form an inactivated probiotic modified coating (Tan et al., 2020). This L. casei biofilm showed an excellent 99.98% antibacterial effectiveness against methicillin-resistant S. aureus. Furthermore, the polysaccharides in the biofilm could promote osteogenic differentiation through stimulating macrophages to secrete osteogenic factors.
Synthetic multifunctional peptides can be used as a single material for multifunctional coatings. Antimicrobial sequences are combined with bone-promoting sequences to form multifunctional peptides for immobilization on titanium surfaces to achieve multifunctional coatings (Zhang et al., 2018b; Liu et al., 2018; Wisdom et al., 2020). The RGD peptide is derived from extracellular matrix proteins, promoting mammalian cell adhesion as an integrin ligand (Atefyekta et al., 2019). Based on this, RGD was linked to the antimicrobial sequence from human β-defensin-3 as a multifunctional chimeric peptide (Zhang et al., 2018b). This chimeric peptide effectively prevented the formation of biofilms by inhibiting bacterial gene expression and induced osteoblast differentiation and mineral deposition. Some studies reported that antimicrobial peptides were covalently immobilized onto titanium surfaces, but direct conjugation of these peptides abolishes their antimicrobial activity (Chouirfa et al., 2019). Wisdom et al. developed high-affinity inorganic binding peptides (TiBP) containing a Ti-binding domain to recognize Ti surfaces (Wisdom et al., 2020). Then, they combined RGD and antibacterial peptides with TiBP, and proved that this biofunctional peptide has antibacterial and bone-promoting properties with good stability and durability. Another method of surface immobilization is silanization of the Ti surface, immobilizing organic molecules by covalent stabilization (Kucharíková et al., 2016). Yang et al. immobilized hyperbranched poly-l-lysine (HBPL) polymers in Ti via silane coupling agents (Yang et al., 2021). The poly-l-lysine was able to interfere with the integrity of bacterial membranes and produce ROS to damage DNA of bacterial, exhibiting efficient sterilization properties in vivo and in vitro. Furthermore, the degradation of the coating in vivo created an alkaline microenvironment around the implant, which promotes osseointegration compared with the control group. Chen et al. synthesized a fusion peptide (FP) containing the antimicrobial sequence and the angiogenic sequence by a cycloaddition and introduced an alkyl group on the Ti surface to immobilize FP (Chen et al., 2021a). In vivo tests proved that the fusion peptide was able to kill 99.63% of S. aureus, as well as promote vascularization and osseointegration.
Discussion
Aseptic loosening and infection are still challenges in fabricating coatings for orthopedic and orthodontic implants, so we introduce the current strategies combating the aseptic loosening and infection of titanium-based implants in this review. Antibacterial metal ions combined with porous structure to achieve antibacterial properties and promote osseointegration are most widely used. However, burst release, cytotoxicity, short half-life, and limited stability are ongoing problems of metal ionbased strategies. These problems are addressed by coatings with controlled release properties and synergistic effects between the substances. It is worth mentioning that smart release coatings have become research hotspots recently but most of them do not exhibit sufficient capacity to promote osteogenesis. In the future, dual-function smart release coatings may become the focus of research. On the one hand, the coating can release antibacterial agents to kill bacteria in a slightly acidic environment suitable for bacteria; on the other hand, it can release osteogenic factors in a slightly alkaline environment that is conducive to osteogenesis. In addition, strategies to regulate the microenvironment around the implant also have to be mentioned, including the regulation to tissue and the local inflammatory system. Proper inflammation milieu will do facilitative effect to osseointegration, but excessive inflammation will cause serious tissue damage. Therefore, the search for an appropriate inflammatory microenvironment by modulating the polarization of macrophages is a new method to promote osteogenesis. Multifunctional coatings on titanium surfaces are formed based on biomolecules with good cytocompatibility; coatings designed by these strategies promote bone formation and provide antibacterial effects at the same time. Four main strategies are currently followed: the direct binding of antibacterial and bone-promoting substances to the surface of titanium, integrating antibacterial substances into roughening/porous surface modified coatings, photoantibacterial and osteogenic coatings, and multifunctional coatings with single material. Among them, multifunctional peptides have better bone-forming properties and avoid resistance formation, which are the shortcomings of traditional antibacterial agents. However, they still face challenges in terms of stability and long-term performance. New substances or surface modification methods with antibacterial and bone-promoting properties need to be explored, and will become the focus of research to simplify surgery, reduce costs, and provide more safety for the patient.
Author Contributions
XL, ZW, and KX contributed equally to this work. XL: conceptualization, investigation, writing—original draft. ZW: investigation, writing—review and editing. KX: investigation, writing—review and editing. XW: writing—review and editing. SW: writing—review and editing. HQ: supervision. XLi: supervision, resources, funding acquisition. JC: conceptualization, project administration, funding acquisition.
Funding
This work was supported by the National Natural Science Foundation of China (nos. 31670967 and 32000932), the Key Research and Development Projects in Anhui Province (no. 202104j07020039), the Scientific Research Foundation of the Institute for Translational Medicine of Anhui Province (no. 2017zhyx19), and research improvement program in Stomatologic Hospital and College of Anhui Medical University (no. 2020kqkyT01).
Conflict of Interest
The authors declare that the research was conducted in the absence of any commercial or financial relationships that could be construed as a potential conflict of interest.
Publisher’s Note
All claims expressed in this article are solely those of the authors and do not necessarily represent those of their affiliated organizations, or those of the publisher, the editors, and the reviewers. Any product that may be evaluated in this article, or claim that may be made by its manufacturer, is not guaranteed or endorsed by the publisher.
References
Acosta, S., Ibañez-Fonseca, A., Aparicio, C., and Rodríguez-Cabello, J. C. (2020). Antibiofilm Coatings Based on Protein-Engineered Polymers and Antimicrobial Peptides for Preventing Implant-Associated Infections. Biomater. Sci. 8 (10), 2866–2877. doi:10.1039/D0BM00155D
Amstutz, H. C., Campbell, P., Kossovsky, N., and Clarke, I. C. (1992). Mechanism and Clinical Significance of Wear Debris-Induced Osteolysis. Clin. Orthop. Relat. Res. 276, 7–18. doi:10.1097/00003086-199203000-00003
Anandan, S., Narasinga Rao, T., Sathish, M., Rangappa, D., Honma, I., and Miyauchi, M. (2013). Superhydrophilic Graphene-Loaded TiO2 Thin Film for Self-Cleaning Applications. ACS Appl. Mater. Inter. 5 (1), 207–212. doi:10.1021/am302557z
Atefyekta, S., Pihl, M., Lindsay, C., Heilshorn, S. C., and Andersson, M. (2019). Antibiofilm Elastin-like Polypeptide Coatings: Functionality, Stability, and Selectivity. Acta Biomater. 83, 245–256. doi:10.1016/j.actbio.2018.10.039
Bai, Y., Zhou, R., Cao, J., Wei, D., Du, Q., Li, B., et al. (2017). Microarc Oxidation Coating Covered Ti Implants with Micro-scale Gouges Formed by a Multi-step Treatment for Improving Osseointegration. Mater. Sci. Eng. C. 76, 908–917. doi:10.1016/j.msec.2017.03.071
Bai, L., Du, Z., Du, J., Yao, W., Zhang, J., Weng, Z., et al. (2018a). A Multifaceted Coating on Titanium Dictates Osteoimmunomodulation and Osteo/angio-Genesis towards Ameliorative Osseointegration. Biomaterials 162, 154–169. doi:10.1016/j.biomaterials.2018.02.010
Bai, L., Liu, Y., Du, Z., Weng, Z., Yao, W., Zhang, X., et al. (2018b). Differential Effect of Hydroxyapatite Nano-Particle versus Nano-Rod Decorated Titanium Micro-surface on Osseointegration. Acta Biomater. 76, 344–358. doi:10.1016/j.actbio.2018.06.023
Bai, H., Zhao, Y., Wang, C., Wang, Z., Wang, J., Liu, H., et al. (2020). Enhanced Osseointegration of Three-Dimensional Supramolecular Bioactive Interface through Osteoporotic Microenvironment Regulation. Theranostics 10 (11), 4779–4794. doi:10.7150/thno.43736
Bencharit, S., Byrd, W. C., Altarawneh, S., Hosseini, B., Leong, A., Reside, G., et al. (2014). Development and Applications of Porous Tantalum Trabecular Metal-Enhanced Titanium Dental Implants. Clin. Implant Dent. Relat. Res. 16 (6), 817–826. doi:10.1111/cid.12059
Cao, S., Huang, W., Wu, L., Tian, M., and Song, Y. (2018). On the Interfacial Adhesion between TiO2 Nanotube Array Layer and Ti Substrate. Langmuir 34 (46), 13888–13896. doi:10.1021/acs.langmuir.8b03408
Carp, O. (2004). Photoinduced Reactivity of Titanium Dioxide. Prog. Solid State. Chem. 32 (1-2), 33–177. doi:10.1016/j.progsolidstchem.2004.08.001
Carson, J. S., and Bostrom, M. P. G. (2007). Synthetic Bone Scaffolds and Fracture Repair. Injury 38 (1), S33–S37. doi:10.1016/j.injury.2007.02.008
Chang, B., Song, W., Han, T., Yan, J., Li, F., Zhao, L., et al. (2016). Influence of Pore Size of Porous Titanium Fabricated by Vacuum Diffusion Bonding of Titanium Meshes on Cell Penetration and Bone Ingrowth. Acta Biomater. 33, 311–321. doi:10.1016/j.actbio.2016.01.022
Chen, J., Mei, M. L., Li, Q.-L., and Chu, C.-H. (2016). Mussel-inspired Silver-Nanoparticle Coating on Porous Titanium Surfaces to Promote Mineralization. RSC Adv. 6 (106), 104025–104035. doi:10.1039/c6ra20673e
Chen, L., Mou, S., Hou, J., Fang, H., Zeng, Y., Sun, J., et al. (2020a). Simple Application of Adipose-Derived Stem Cell-Derived Extracellular Vesicles Coating Enhances Cytocompatibility and Osteoinductivity of Titanium Implant. Regenerative Biomater. 8 (1), rbaa038. doi:10.1093/rb/rbaa038
Chen, X., Zhu, R.-f., Gao, H., Xu, W.-l., Xiao, G.-y., Chen, C.-z., et al. (2020b). A High Bioactive Alkali-Treated Titanium Surface Induced by Induction Heat Treatment. Surf. Coat. Technol. 385, 125362. doi:10.1016/j.surfcoat.2020.125362
Chen, J., Hu, G., Li, T., Chen, Y., Gao, M., Li, Q., et al. (2021a). Fusion Peptide Engineered “Statically-Versatile” Titanium Implant Simultaneously Enhancing Anti-infection, Vascularization and Osseointegration. Biomaterials 264, 120446. doi:10.1016/j.biomaterials.2020.120446
Chen, Y., Zhou, C., Xie, Y., Xu, A., Guan, Y., Lu, W., et al. (2021b). Zinc‐ and Strontium‐ Co‐incorporated Nanorods on Titanium Surfaces with Favorable Material Property, Osteogenesis, and Enhanced Antibacterial Activity. J. Biomed. Mater. Res. 109 (11), 1754–1767. doi:10.1002/jbm.b.34834
Cheng, L., Wang, C., Feng, L., Yang, K., and Liu, Z. (2014). Functional Nanomaterials for Phototherapies of Cancer. Chem. Rev. 114 (21), 10869–10939. doi:10.1021/cr400532z
Cheng, L., Zhang, K., Zhang, N., Melo, M. A. S., Weir, M. D., Zhou, X. D., et al. (2017). Developing a New Generation of Antimicrobial and Bioactive Dental Resins. J. Dent Res. 96 (8), 855–863. doi:10.1177/0022034517709739
Chouirfa, H., Bouloussa, H., Migonney, V., and Falentin-Daudré, C. (2019). Review of Titanium Surface Modification Techniques and Coatings for Antibacterial Applications. Acta Biomater. 83, 37–54. doi:10.1016/j.actbio.2018.10.036
Córdoba, A., Satué, M., Gómez-Florit, M., Hierro-Oliva, M., Petzold, C., Lyngstadaas, S. P., et al. (2015). Flavonoid-Modified Surfaces: Multifunctional Bioactive Biomaterials with Osteopromotive, Anti-inflammatory, and Anti-fibrotic Potential. Adv. Healthc. Mater. 4 (4), 540–549. doi:10.1002/adhm.201400587
Del Pozo, J. L., and Patel, R. (2009). Clinical Practice. Infection Associated with Prosthetic Joints. N. Engl. J. Med. 361 (8), 787–794. doi:10.1056/NEJMcp0905029
Delanois, R. E., Mistry, J. B., Gwam, C. U., Mohamed, N. S., Choksi, U. S., and Mont, M. A. (2017). Current Epidemiology of Revision Total Knee Arthroplasty in the United States. The J. Arthroplasty 32 (9), 2663–2668. doi:10.1016/j.arth.2017.03.066
Franz, S., Rammelt, S., Scharnweber, D., and Simon, J. C. (2011). Immune Responses to Implants - a Review of the Implications for the Design of Immunomodulatory Biomaterials. Biomaterials 32 (28), 6692–6709. doi:10.1016/j.biomaterials.2011.05.078
Gao, Q., Feng, T., Huang, D., Liu, P., Lin, P., Wu, Y., et al. (2020). Antibacterial and Hydroxyapatite-Forming Coating for Biomedical Implants Based on Polypeptide-Functionalized Titania Nanospikes. Biomater. Sci. 8 (1), 278–289. doi:10.1039/C9BM01396B
Gnedenkov, S. V., Gordienko, P. S., Sinebryukhov, S. L., Kovryanov, A. N., and Khromushkin, K. D. (2000). Antiscuff Coatings Obtained by Microarc Oxidation of Titanium Alloys. Russ. J. Appl. Chem. 73 (1), 6–9. doi:10.1023/A:1008117010991
Górniak, I., Bartoszewski, R., and Króliczewski, J. (2019). Comprehensive Review of Antimicrobial Activities of Plant Flavonoids. Phytochem. Rev. 18 (1), 241–272. doi:10.1007/s11101-018-9591-z
Gomez-Florit, M., Pacha-Olivenza, M. A., Fernández-Calderón, M. C., Córdoba, A., González-Martín, M. L., Monjo, M., et al. (2016). Quercitrin-nanocoated Titanium Surfaces Favour Gingival Cells against Oral Bacteria. Sci. Rep. 6 (1), 22444. doi:10.1038/srep22444
Goodman, S. B. (1994). The Effects of Micromotion and Particulate Materials on Tissue Differentiation. Bone Chamber Studies in Rabbits. Acta Orthop. Scand. Suppl. 258, 1–43. doi:10.3109/17453679409155227
Grimes, C. A., and Mor, G. K. (2009). Tio2 Nanotube Arrays: Synthesis, Properties, and Applications. New York, NY: Springer Science and Business Media.
Gristina, A. G., and Costerton, J. W. (1985). Bacterial Adherence to Biomaterials and Tissue. The Significance of its Role in Clinical Sepsis. J. Bone Jt. Surg. 67 (2), 264–273. doi:10.2106/00004623-198567020-00014
Gu, M., Liu, Y., Chen, T., Du, F., Zhao, X., Xiong, C., et al. (2014). Is Graphene a Promising Nano-Material for Promoting Surface Modification of Implants or Scaffold Materials in Bone Tissue Engineering? Tissue Eng. B: Rev. 20 (5), 477–491. doi:10.1089/ten.teb.2013.0638
Guo, C., Cui, W., Wang, X., Lu, X., Zhang, L., Li, X., et al. (2020). Poly-l-lysine/Sodium Alginate Coating Loading Nanosilver for Improving the Antibacterial Effect and Inducing Mineralization of Dental Implants. ACS Omega 5 (18), 10562–10571. doi:10.1021/acsomega.0c00986
Guo, L. L., Cheng, Y. F., Ren, X., Gopinath, K., Lu, Z. S., Li, C. M., et al. (2021). Simultaneous Deposition of Tannic Acid and Poly(ethylene Glycol) to Construct the Antifouling Polymeric Coating on Titanium Surface. Colloids Surf. B: Biointerf. 200, 111592. doi:10.1016/j.colsurfb.2021.111592
Haidar, Z. S., Hamdy, R. C., and Tabrizian, M. (2009a). Delivery of Recombinant Bone Morphogenetic Proteins for Bone Regeneration and Repair. Part A: Current Challenges in BMP Delivery. Biotechnol. Lett. 31 (12), 1817–1824. doi:10.1007/s10529-009-0099-x
Haidar, Z. S., Hamdy, R. C., and Tabrizian, M. (2009b). Delivery of Recombinant Bone Morphogenetic Proteins for Bone Regeneration and Repair. Part B: Delivery Systems for BMPs in Orthopaedic and Craniofacial Tissue Engineering. Biotechnol. Lett. 31 (12), 1825–1835. doi:10.1007/s10529-009-0100-8
Han, X., Zhang, G., Chai, M., and Zhang, X. (2021). Light-assisted Therapy for Biofilm Infected Micro-arc Oxidation TiO2 Coating on Bone Implants. Biomed. Mater. 16 (2), 025018. doi:10.1088/1748-605x/abdb72
Hancock, R. E. W., and Sahl, H.-G. (2006). Antimicrobial and Host-Defense Peptides as New Anti-Infective Therapeutic Strategies. Nat. Biotechnol. 24 (12), 1551–1557. doi:10.1038/nbt1267
Harada, Y., Ogawa, K., Irie, Y., Endo, H., Feril, L. B., Uemura, T., et al. (2011). Ultrasound Activation of TiO2 in Melanoma Tumors. J. Controlled Release 149 (2), 190–195. doi:10.1016/j.jconrel.2010.10.012
Harmankaya, N., Igawa, K., Stenlund, P., Palmquist, A., and Tengvall, P. (2012). Healing of Complement Activating Ti Implants Compared with Non-activating Ti in Rat Tibia. Acta Biomater. 8 (9), 3532–3540. doi:10.1016/j.actbio.2012.05.017
Hassani Besheli, N., Mottaghitalab, F., Eslami, M., Gholami, M., Kundu, S. C., Kaplan, D. L., et al. (2017). Sustainable Release of Vancomycin from Silk Fibroin Nanoparticles for Treating Severe Bone Infection in Rat Tibia Osteomyelitis Model. ACS Appl. Mater. Inter. 9 (6), 5128–5138. doi:10.1021/acsami.6b14912
He, Y., Zhang, Y., Shen, X., Tao, B., Liu, J., Yuan, Z., et al. (2018). The Fabrication and In Vitro Properties of Antibacterial Polydopamine-LL-37-POPC Coatings on Micro-arc Oxidized Titanium. Colloids Surf. B: Biointerf. 170, 54–63. doi:10.1016/j.colsurfb.2018.05.070
Hirt, H., and Gorr, S.-U. (2013). Antimicrobial Peptide GL13K Is Effective in Reducing Biofilms of Pseudomonas aeruginosa. Antimicrob. Agents Chemother. 57 (10), 4903–4910. doi:10.1128/AAC.00311-13
Hoyos-Nogués, M., Buxadera-Palomero, J., Ginebra, M.-P., Manero, J. M., Gil, F. J., and Mas-Moruno, C. (2018). All-in-one Trifunctional Strategy: A Cell Adhesive, Bacteriostatic and Bactericidal Coating for Titanium Implants. Colloids Surf. B: Biointerf. 169, 30–40. doi:10.1016/j.colsurfb.2018.04.050
Hu, D., Li, H., Wang, B., Ye, Z., Lei, W., Jia, F., et al. (2017). Surface-Adaptive Gold Nanoparticles with Effective Adherence and Enhanced Photothermal Ablation of Methicillin-Resistant Staphylococcus aureus Biofilm. ACS Nano 11 (9), 9330–9339. doi:10.1021/acsnano.7b04731
Hu, N., Wu, Y., Xie, L., Yusuf, S. M., Gao, N., Starink, M. J., et al. (2020). Enhanced Interfacial Adhesion and Osseointegration of Anodic TiO2 Nanotube Arrays on ultra-fine-grained Titanium and Underlying Mechanisms. Acta Biomater. 106, 360–375. doi:10.1016/j.actbio.2020.02.009
Huang, P., Zhang, Y., Xu, K., and Han, Y. (2004). Surface Modification of Titanium Implant by Microarc Oxidation and Hydrothermal Treatment. J. Biomed. Mater. Res. 70B (2), 187–190. doi:10.1002/jbm.b.30009
Huang, T., Liu, H., Liu, P., Liu, P., Li, L., and Shen, J. (2017). Zwitterionic Copolymers Bearing Phosphonate or Phosphonic Motifs as Novel Metal-Anchorable Anti-fouling Coatings. J. Mater. Chem. B 5 (27), 5380–5389. doi:10.1039/C7TB01017F
Huang, Q., Li, X., Elkhooly, T. A., Liu, X., Zhang, R., Wu, H., et al. (2018a). The Cu-Containing TiO2 Coatings with Modulatory Effects on Macrophage Polarization and Bactericidal Capacity Prepared by Micro-arc Oxidation on Titanium Substrates. Colloids Surf. B: Biointerf. 170, 242–250. doi:10.1016/j.colsurfb.2018.06.020
Huang, Q., Li, X., Liu, T., Wu, H., Liu, X., Feng, Q., et al. (2018b). Enhanced SaOS-2 Cell Adhesion, Proliferation and Differentiation on Mg-Incorporated Micro/nano-Topographical TiO2 Coatings. Appl. Surf. Sci. 447, 767–776. doi:10.1016/j.apsusc.2018.04.095
Huang, B., Tan, L., Liu, X., Li, J., and Wu, S. (2019a). A Facile Fabrication of Novel Stuff with Antibacterial Property and Osteogenic Promotion Utilizing Red Phosphorus and Near-Infrared Light. Bioactive Mater. 4 (1), 17–21. doi:10.1016/j.bioactmat.2018.11.002
Huang, Y.-Z., He, S.-K., Guo, Z.-J., Pi, J.-K., Deng, L., Dong, L., et al. (2019b). Nanostructured Titanium Surfaces Fabricated by Hydrothermal Method: Influence of Alkali Conditions on the Osteogenic Performance of Implants. Mater. Sci. Eng. C. 94, 1–10. doi:10.1016/j.msec.2018.08.069
Jin, L., Lee, J. H., Jin, O. S., Shin, Y. C., Kim, M. J., Hong, S. W., et al. (2015). Stimulated Osteogenic Differentiation of Human Mesenchymal Stem Cells by Reduced Graphene Oxide. J. Nanosci. Nanotechnol. 15 (10), 7966–7970. doi:10.1166/jnn.2015.11223
Kabata, T., Maeda, T., Kajino, Y., Hasegawa, K., Inoue, D., Yamamoto, T., et al. (2015). Iodine-Supported Hip Implants: Short Term Clinical Results. Biomed. Res. Int. 2015, 1–6. doi:10.1155/2015/368124
Kaestner, K. (2016). Microbial Biofilm Inhibits Wound Healing. Available at https://microbewiki.kenyon.edu/index.php/Microbial_biofilm_inhibits_wound_healing” (Accessed October 4, 2017).
Kang, Q., Sun, M. H., Cheng, H., Peng, Y., Montag, A. G., Deyrup, A. T., et al. (2004). Characterization of the Distinct Orthotopic Bone-Forming Activity of 14 BMPs Using Recombinant Adenovirus-Mediated Gene Delivery. Gene Ther. 11 (17), 1312–1320. doi:10.1038/sj.gt.3302298
Kang, M. S., Jeong, S. J., Lee, S. H., Kim, B., Hong, S. W., Lee, J. H., et al. (2021). Reduced Graphene Oxide Coating Enhances Osteogenic Differentiation of Human Mesenchymal Stem Cells on Ti Surfaces. Biomater. Res. 25 (1), 4. doi:10.1186/s40824-021-00205-x
Kato, T., and Shirai, T. (2016). Temporal Attenuation of Iodine Content and its Effect on the Antibacterial Activity of Iodine-Supported Titanium Implants. J. Microb. Biochem. Technol. 8 (4), 285–289. doi:10.4172/1948-5948.1000298
Ke, D., Vu, A. A., Bandyopadhyay, A., and Bose, S. (2019). Compositionally Graded Doped Hydroxyapatite Coating on Titanium Using Laser and Plasma spray Deposition for Bone Implants. Acta Biomater. 84, 414–423. doi:10.1016/j.actbio.2018.11.041
Kim, T.-S., Park, S.-H., Park, D., Lee, J.-H., and Kang, S. (2019). Surface Immobilization of Chlorhexidine on a Reverse Osmosis Membrane for In-Situ Biofouling Control. J. Membr. Sci. 576, 17–25. doi:10.1016/j.memsci.2019.01.030
Kolaparthy, L. K., Sanivarapu, S., Moogla, S., and Kutcham, R. S. (2015). Adipose Tissue - Adequate, Accessible Regenerative Material. Ijsc 8 (2), 121–127. doi:10.15283/ijsc.2015.8.2.121
Konopka, K., and Goslinski, T. (2007). Photodynamic Therapy in Dentistry. J. Dent Res. 86 (8), 694–707. doi:10.1177/154405910708600803
Kucharíková, S., Gerits, E., De Brucker, K., Braem, A., Ceh, K., Majdič, G., et al. (2016). Covalent Immobilization of Antimicrobial Agents on Titanium prevents Staphylococcus aureus and Candida Albicans colonization and Biofilm Formation. J. Antimicrob. Chemother. 71 (4), 936–945. doi:10.1093/jac/dkv437
Kurtz, S., Mowat, F., Ong, K., Chan, N., Lau, E., and Halpern, M. (2005). Prevalence of Primary and Revision Total Hip and Knee Arthroplasty in the United States from 1990 through 2002. J. Bone Jt. Surg. Am. Vol. 87 (7), 1487–1497. doi:10.2106/JBJS.D.02441
Lampé, I., Beke, D., Biri, S., Csarnovics, I., Csík, A., Dombrádi, Z., et al. (2019). Investigation of Silver Nanoparticles on Titanium Surface Created by Ion Implantation Technology. Int. J. Nanomed. 14, 4709–4721. doi:10.2147/IJN.S197782
Latiff, N. M., Teo, W. Z., Sofer, Z., Fisher, A. C., and Pumera, M. (2015). The Cytotoxicity of Layered Black Phosphorus. Chem. Eur. J. 21 (40), 13991–13995. doi:10.1002/chem.201502006
Lee, J.-Y., Jahng, J.-W., Kim, S.-M., Kim, M.-J., and Lee, J.-H. (2015). Simultaneous Inferior Alveolar Nerve Regeneration and Osseointegration with a Nerve Growth Factor-Supplying Implant: a Preliminary Study. J. Oral Maxillofac. Surg. 73 (3), 410–423. doi:10.1016/j.joms.2014.07.009
LeGeros, R. Z. (2002). Properties of Osteoconductive Biomaterials: Calcium Phosphates. Clin. Orthop. Relat. Res. 395 (395), 81–98. doi:10.1097/00003086-200202000-00009
Lei, W., Ren, K., Chen, T., Chen, X., Li, B., Chang, H., et al. (2016). Polydopamine Nanocoating for Effective Photothermal Killing of Bacteria and Fungus upon Near-Infrared Irradiation. Adv. Mater. Inter. 3 (22), 1600767. doi:10.1002/admi.201600767
Li, H., Ghazanfari, R., Zacharaki, D., Lim, H. C., and Scheding, S. (2016). Isolation and Characterization of Primary Bone Marrow Mesenchymal Stromal Cells. Ann. N.Y. Acad. Sci. 1370 (1), 109–118. doi:10.1111/nyas.13102
Li, J., Tan, L., Liu, X., Cui, Z., Yang, X., Yeung, K. W. K., et al. (2017). Balancing Bacteria-Osteoblast Competition through Selective Physical Puncture and Biofunctionalization of ZnO/Polydopamine/Arginine-Glycine-Aspartic Acid-Cysteine Nanorods. ACS nano 11 (11), 11250–11263. doi:10.1021/acsnano.7b05620
Li, Y., Liu, X., Tan, L., Cui, Z., Yang, X., Zheng, Y., et al. (2018). Rapid Sterilization and Accelerated Wound Healing Using Zn2+ and Graphene Oxide Modified G-C3 N4 under Dual Light Irradiation. Adv. Funct. Mater. 28 (30), 1800299. doi:10.1002/adfm.201800299
Li, C., Yang, Y., Yang, L., Shi, Z., Yang, P., and Cheng, G. (2020a). In Vitro Bioactivity and Biocompatibility of Bio-Inspired Ti-6Al-4V Alloy Surfaces Modified by Combined Laser Micro/Nano Structuring. Molecules 25 (7), 1494. doi:10.3390/molecules25071494
Li, Q., Liang, B., Wang, F., and Wang, Z. (2020b). Delivery of Interleukin 4 from a Titanium Substrate Coated with Graphene Oxide for Enhanced Osseointegration by Regulating Macrophage Polarization. ACS Biomater. Sci. Eng. 6 (9), 5215–5229. doi:10.1021/acsbiomaterials.0c01011
Li, B., Zhang, L., Wang, D., Peng, F., Zhao, X., Liang, C., et al. (2021a). Thermosensitive -Hydrogel-Coated Titania Nanotubes with Controlled Drug Release and Immunoregulatory Characteristics for Orthopedic Applications. Mater. Sci. Eng. C. 122, 111878. doi:10.1016/j.msec.2021.111878
Li, K., Tian, H., Guo, A., Jin, L., Chen, W., and Tao, B. (2021b). Gallium (Ga)–strontium (Sr) Layered Double Hydroxide Composite Coating on Titanium Substrates for Enhanced Osteogenic and Antibacterial Abilities. J. Biomed. Mater. Res. A. doi:10.1002/jbm.a.37284
Liu, X., Wu, S., Yeung, K. W. K., Chan, Y. L., Hu, T., Xu, Z., et al. (2011). Relationship between Osseointegration and Superelastic Biomechanics in Porous NiTi Scaffolds. Biomaterials 32 (2), 330–338. doi:10.1016/j.biomaterials.2010.08.102
Liu, J., Zhu, L., Xiang, S., Wei, Y., Xie, M., Liu, H., et al. (2018). Growing High-Quality CsPbBr3 by Using Porous CsPb2Br5 as an Intermediate: a Promising Light Absorber in Carbon-Based Perovskite Solar Cells. Sustain. Energ. Fuels 3 (1), 184–194.
Liu, J., Tang, Y., Yang, W., Tao, B., He, Y., Shen, X., et al. (2019). Functionalization of Titanium Substrate with Multifunctional Peptide OGP-NAC for the Regulation of Osteoimmunology. Biomater. Sci. 7 (4), 1463–1476. doi:10.1039/c8bm01611a
Llopis-Grimalt, M. A., Arbós, A., Gil-Mir, M., Mosur, A., Kulkarni, P., Salito, A., et al. (2020). Multifunctional Properties of Quercitrin-Coated Porous Ti-6Al-4V Implants for Orthopaedic Applications Assessed In Vitro. J. Clin.Med. 9 (3), 855. doi:10.3390/jcm9030855
Long, X., Xu, H., Zhang, D., and Li, J. (2020). Bioinspired by Both Mussel Foot Protein and Bone Sialoprotein: Universal Adhesive Coatings for the Promotion of Mineralization and Osteogenic Differentiation. Polym. Chem. 11 (31), 4995–5004. doi:10.1039/D0PY00774A
Lou, W., Dong, Y., Zhang, H., Jin, Y., Hu, X., Ma, J., et al. (2015). Preparation and Characterization of Lanthanum-Incorporated Hydroxyapatite Coatings on Titanium Substrates. Int. J. Mol.Sci. 16 (9), 21070–21086. doi:10.3390/ijms160921070
Ma, L., Wang, X., Zhao, N., Zhu, Y., Qiu, Z., Li, Q., et al. (2018). Integrating 3D Printing and Biomimetic Mineralization for Personalized Enhanced Osteogenesis, Angiogenesis, and Osteointegration. ACS Appl. Mater. Inter. 10 (49), 42146–42154. doi:10.1021/acsami.8b17495
MacBarb, R. F., Lindsey, D. P., Bahney, C. S., Woods, S. A., Wolfe, M. L., and Yerby, S. A. (2017). Fortifying the Bone-Implant Interface Part 1: An In Vitro Evaluation of 3D-Printed and TPS Porous Surfaces. Int. J. Spine Surg. 11 (3), 15. doi:10.14444/4015
Malda, J., Boere, J., van de Lest, C. H. A., van Weeren, P. R., and Wauben, M. H. M. (2016). Extracellular Vesicles - New Tool for Joint Repair and Regeneration. Nat. Rev. Rheumatol. 12 (4), 243–249. doi:10.1038/nrrheum.2015.170
Montanaro, L., Speziale, P., Campoccia, D., Ravaioli, S., Cangini, I., Pietrocola, G., et al. (2011). Scenery of Staphylococcus Implant Infections in Orthopedics. Future Microbiol. 6 (11), 1329–1349. doi:10.2217/fmb.11.117
Mushahary, D., Spittler, A., Kasper, C., Weber, V., and Charwat, V. (2018). Isolation, Cultivation, and Characterization of Human Mesenchymal Stem Cells. Cytometry 93 (1), 19–31. doi:10.1002/cyto.a.23242
Nagay, B. E., Dini, C., Cordeiro, J. M., Ricomini-Filho, A. P., de Avila, E. D., Rangel, E. C., et al. (2019). Visible-Light-Induced Photocatalytic and Antibacterial Activity of TiO2 Codoped with Nitrogen and Bismuth: New Perspectives to Control Implant-Biofilm-Related Diseases. ACS Appl. Mater. Inter. 11 (20), 18186–18202. doi:10.1021/acsami.9b03311
Ordikhani, F., and Simchi, A. (2014). Long-term Antibiotic Delivery by Chitosan-Based Composite Coatings with Bone Regenerative Potential. Appl. Surf. Sci. 317, 56–66. doi:10.1016/j.apsusc.2014.07.197
Park, C., Seong, Y.-J., Kang, I.-G., Song, E.-H., Lee, H., Kim, J., et al. (2019). Enhanced Osseointegration Ability of Poly(lactic Acid) via Tantalum Sputtering-Based Plasma Immersion Ion Implantation. ACS Appl. Mater. Inter. 11 (11), 10492–10504. doi:10.1021/acsami.8b21363
Pittenger, M. F., Mackay, A. M., Beck, S. C., Jaiswal, R. K., Douglas, R, Mosca, J. D., et al. (1999). Multilineage Potential of Adult Human Mesenchymal Stem Cells. Science 284 (5411), 143–147. doi:10.1126/science.284.5411.143
Qiao, Y., Ping, Y., Zhang, H., Zhou, B., Liu, F., Yu, Y., et al. (2019). Laser-Activatable CuS Nanodots to Treat Multidrug-Resistant Bacteria and Release Copper Ion to Accelerate Healing of Infected Chronic Nonhealing Wounds. ACS Appl. Mater. Inter. 11 (4), 3809–3822. doi:10.1021/acsami.8b21766
Raafat, D., and Sahl, H. (2009). Chitosan and its Antimicrobial Potential-Aa Critical Literature Survey. Microb. Biotechnol. 2 (2), 186–201. doi:10.1111/j.1751-7915.2008.00080.x
Raghavendra, G. M., Jung, J., Kim, D., and Seo, J. (2017). Effect of Chitosan Silver Nanoparticle Coating on Functional Properties of Korean Traditional Paper. Prog. Org. Coat. 110, 16–23. doi:10.1016/j.porgcoat.2017.04.040
Rego, G. F., Vidal, M. L., Viana, G. M., Cabral, L. M., Schneider, L. F. J., Portela, M. B., et al. (2017). Antibiofilm Properties of Model Composites Containing Quaternary Ammonium Methacrylates after Surface Texture Modification. Dental Mater. : Official Publ. Acad. Dental Mater. 33 (10), 1149–1156. doi:10.1016/j.dental.2017.07.010
Ren, L., Zhao, Y., Yang, L., Cao, W., Wang, H., Lian, X., et al. (2021). Preparation and Characterization of the Catechol Functionalized Chitosan-Ag NPs Deposited onto Titanium Surface. Surf. Coat. Technol. 420, 127319. doi:10.1016/j.surfcoat.2021.127319
Rodríguez López, A. d. L., Lee, M.-R., Ortiz, B. J., Gastfriend, B. D., Whitehead, R., Lynn, D. M., et al. (2019). Preventing S. aureus Biofilm Formation on Titanium Surfaces by the Release of Antimicrobial β-Peptides from Polyelectrolyte Multilayers. Acta Biomater. 93, 50–62. doi:10.1016/j.actbio.2019.02.047
Rodríguez-Contreras, A., Torres, D., Rafik, B., Ortiz-Hernandez, M., Ginebra, M. P., Calero, J. A., et al. (2021). Bioactivity and Antibacterial Properties of Calcium- and Silver-Doped Coatings on 3D Printed Titanium Scaffolds. Surf. Coat. Technol. 421, 127476. doi:10.1016/j.surfcoat.2021.127476
Salvagni, E., Berguig, G., Engel, E., Rodriguez-Cabello, J. C., Coullerez, G., Textor, M., et al. (2014). A Bioactive Elastin-like Recombinamer Reduces Unspecific Protein Adsorption and Enhances Cell Response on Titanium Surfaces. Colloids Surf. B: Biointerf. 114, 225–233. doi:10.1016/j.colsurfb.2013.10.008
Sang, S., Guo, G., Yu, J., and Zhang, X. (2021). Antibacterial Application of Gentamicin-Silk Protein Coating with Smart Release Function on Titanium, Polyethylene, and Al2O3 Materials. Mater. Sci. Eng. C, Mater. Biol. Appl. 124, 112069. doi:10.1016/j.msec.2021.112069
Schmalzried, T. P., Jasty, M., and Harris, W. H. (1992). Periprosthetic Bone Loss in Total Hip Arthroplasty. Polyethylene Wear Debris and the Concept of the Effective Joint Space. The J. bone Jt. Surg. Am. vol. 74 (6), 849–863. doi:10.2106/00004623-199274060-00006
Shahmohammadi, P., and Khazaei, B. A. (2021). Characterization of Zn/Mg-Enriched Calcium Phosphate Coating Produced by the Two-step Pulsed Electrodeposition Method on Titanium Substrate. Surf. Inter. 22, 100819. doi:10.1016/j.surfin.2020.100819
Sharab, L., Baier, R., Ciancio, S., and Mang, T. (2020). Influence of Photodynamic Therapy on Bacterial Attachment to Titanium Surface. J. Oral Implantol. 47 (5), 427–435. doi:10.1563/aaid-joi-D-19-00344
Shen, X., Zhang, Y., Ma, P., Sutrisno, L., Luo, Z., Hu, Y., et al. (2019). Fabrication of Magnesium/zinc-Metal Organic Framework on Titanium Implants to Inhibit Bacterial Infection and Promote Bone Regeneration. Biomaterials 212, 1–16. doi:10.1016/j.biomaterials.2019.05.008
Shi, J., Liu, Y., Wang, Y., Zhang, J., Zhao, S., and Yang, G. (2015). Biological and Immunotoxicity Evaluation of Antimicrobial Peptide-Loaded Coatings Using a Layer-By-Layer Process on Titanium. Sci. Rep. 5, 16336. doi:10.1038/srep16336
Shirai, T., Shimizu, T., Ohtani, K., Zen, Y., Takaya, M., and Tsuchiya, H. (2011). Antibacterial Iodine-Supported Titanium Implants. Acta Biomater. 7 (4), 1928–1933. doi:10.1016/j.actbio.2010.11.036
Sims, N. A., and Martin, T. J. (2014). Coupling the Activities of Bone Formation and Resorption: a Multitude of Signals within the Basic Multicellular Unit. BoneKEy Rep. 3, 481. doi:10.1038/bonekey.2013.215
Song, T., Yan, M., and Qian, M. (2018). The Enabling Role of Dealloying in the Creation of Specific Hierarchical Porous Metal Structures—A Review. Corrosion Sci. 134, 78–98. doi:10.1016/j.corsci.2018.02.013
Song, P., Hu, C., Pei, X., Sun, J., Sun, H., Wu, L., et al. (2019). Dual Modulation of Crystallinity and Macro-/microstructures of 3D Printed Porous Titanium Implants to Enhance Stability and Osseointegration. J. Mater. Chem. B 7 (17), 2865–2877. doi:10.1039/c9tb00093c
Song, J., Liu, H., Lei, M., Tan, H., Chen, Z., Antoshin, A., et al. (2020). Redox-Channeling Polydopamine-Ferrocene (PDA-Fc) Coating to Confer Context-dependent and Photothermal Antimicrobial Activities. ACS Appl. Mater. Inter. 12 (7), 8915–8928. doi:10.1021/acsami.9b22339
Souza, A. T. P., Bezerra, B. L. S., Oliveira, F. S., Freitas, G. P., Bighetti Trevisan, R. L., Oliveira, P. T., et al. (2018). Effect of Bone Morphogenetic Protein 9 on Osteoblast Differentiation of Cells Grown on Titanium with Nanotopography. J. Cell Biochem. 119 (10), 8441–8449. doi:10.1002/jcb.27060
Steckbeck, J. D., Deslouches, B., and Montelaro, R. C. (2014). Antimicrobial Peptides: New Drugs for Bad Bugs? Expert Opin. Biol. Ther. 14 (1), 11–14. doi:10.1517/14712598.2013.844227
Su, K., Tan, L., Liu, X., Cui, Z., Zheng, Y., Li, B., et al. (2020). Rapid Photo-Sonotherapy for Clinical Treatment of Bacterial Infected Bone Implants by Creating Oxygen Deficiency Using Sulfur Doping. ACS nano 14 (2), 2077–2089. doi:10.1021/acsnano.9b08686
Sun, L., Berndt, C. C., Gross, K. A., and Kucuk, A. (2001). Material Fundamentals and Clinical Performance of Plasma-Sprayed Hydroxyapatite Coatings: A Review. J. Biomed. Mater. Res. 58 (5), 570–592. doi:10.1002/jbm.1056
Sun, Y., Zhao, Y.-Q., Zeng, Q., Wu, Y.-W., Hu, Y., Duan, S., et al. (2019). Dual-Functional Implants with Antibacterial and Osteointegration-Promoting Performances. ACS Appl. Mater. Inter. 11 (40), 36449–36457. doi:10.1021/acsami.9b14572
Sundfeldt, M., Carlsson, L. V., Johansson, C. B., Thomsen, P., and Gretzer, C. (2006). Aseptic Loosening, Not Only a Question of Wear: a Review of Different Theories. Acta Orthop. 77 (2), 177–197. doi:10.1080/17453670610045902
Tabesh, E., Salimijazi, H. R., Kharaziha, M., Mahmoudi, M., and Hejazi, M. (2019). Development of an In-Situ Chitosan-Copper Nanoparticle Coating by Electrophoretic Deposition. Surf. Coat. Technol. 364, 239–247. doi:10.1016/j.surfcoat.2019.02.040
Tan, L., Li, J., Liu, X., Cui, Z., Yang, X., Zhu, S., et al. (2018). Rapid Biofilm Eradication on Bone Implants Using Red Phosphorus and Near-Infrared Light. Adv. Mater. 30 (31), 1801808. doi:10.1002/adma.201801808
Tan, L., Fu, J., Feng, F., Liu, X., Cui, Z., Li, B., et al. (2020). Engineered Probiotics Biofilm Enhances Osseointegration via Immunoregulation and Anti-infection. Sci. Adv. 6(46), eaba5723. doi:10.1126/sciadv.aba5723
Tanaka, Y., Matsuo, Y., Komiya, T., Tsutsumi, Y., Doi, H., Yoneyama, T., et al. (2010). Characterization of the Spatial Immobilization Manner of Poly(ethylene Glycol) to a Titanium Surface with Immersion and Electrodeposition and its Effects on Platelet Adhesion. J. Biomed. Mater. Res. Part A 92A (1), 350–358. doi:10.1002/jbm.a.32375
Tao, B., Lin, C., He, Y., Yuan, Z., Chen, M., Xu, K., et al. (2021). Osteoimmunomodulation Mediating Improved Osteointegration by OGP-Loaded Cobalt-Metal Organic Framework on Titanium Implants with Antibacterial Property. Chem. Eng. J. 423, 130176. doi:10.1016/j.cej.2021.130176
Teng, F.-Y., Tai, I. C., Ho, M.-L., Wang, J.-W., Weng, L. W., Wang, Y. J., et al. (2019). Controlled Release of BMP-2 from Titanium with Electrodeposition Modification Enhancing Critical Size Bone Formation. Mater. Sci. Eng. C. 105, 109879. doi:10.1016/j.msec.2019.109879
Trampuz, A., and Widmer, A. F. (2006). Infections Associated with Orthopedic Implants. Curr. Opin. Infect. Dis. 19 (4), 349–356. doi:10.1097/01.qco.0000235161.85925.e8
Tsiapalis, D., and O’Driscoll, L. (2020). Mesenchymal Stem Cell Derived Extracellular Vesicles for Tissue Engineering and Regenerative Medicine Applications. Cells 9 (4), 991. doi:10.3390/cells9040991
Tsuchiya, H., Shirai, T., Nishida, H., Murakami, H., Kabata, T., Yamamoto, N., et al. (2012). Innovative Antimicrobial Coating of Titanium Implants with Iodine. J. Orthopaedic Sci. 17 (5), 595–604. doi:10.1007/s00776-012-0247-3
Valstar, E. R., Nelissen, R. G. H. H., Reiber, J. H. C., and Rozing, P. M. (2002). The Use of Roentgen Stereophotogrammetry to Study Micromotion of Orthopaedic Implants. ISPRS J. Photogramm. Remote Sens. 56 (5), 376–389. doi:10.1016/S0924-2716(02)00064-3
van de Belt, H., Neut, D., Schenk, W., van Horn, J. R., van der Mei, H. C., and Busscher, H. J. (2001). Infection of Orthopedic Implants and the Use of Antibiotic-Loaded Bone Cements. A Review. Acta Orthop. Scand. 72 (6), 557–571. doi:10.1080/000164701317268978
Van, T. B., Brown, S. D., and Wirtz, G. P. (1977). Mechanism of Anodic Spark Deposition. Am. Ceram. Soc. Bull. U. S.
van Hengel, I. A. J., Putra, N. E., Tierolf, M. W. A. M., Minneboo, M., Fluit, A. C., Fratila-Apachitei, L. E., et al. (2020a). Biofunctionalization of Selective Laser Melted Porous Titanium Using Silver and Zinc Nanoparticles to Prevent Infections by Antibiotic-Resistant Bacteria. Acta Biomater. 107, 325–337. doi:10.1016/j.actbio.2020.02.044
van Hengel, I. A. J., Tierolf, M. W. A. M., Valerio, V. P. M., Minneboo, M., Fluit, A. C., Fratila-Apachitei, L. E., et al. (2020b). Self-defending Additively Manufactured Bone Implants Bearing Silver and Copper Nanoparticles. J. Mater. Chem. B. 8 (8), 1589–1602. doi:10.1039/C9TB02434D
Vizoso, F., Eiro, N., Cid, S., Schneider, J., and Perez-Fernandez, R. (2017). Mesenchymal Stem Cell Secretome: Toward Cell-free Therapeutic Strategies in Regenerative Medicine. Int. J. Mol. Sci. 18 (9), 1852. doi:10.3390/ijms18091852
Vovna, V. I., Gnedenkov, S. V., Gordienko, P. S., Kuznetsov, M. V., and Khrisanfova, O. A. (1998). Surface Layers Produced on Titanium by Microarc Oxidation: An X-ray Diffractometry Study. Russ. J. Electrochem. 34 (10), 1090–1093.
Wang, Q., Huang, J., Li, H., Zhao, A., Wang, Y., Zhang, K., et al. (2017a). Recent Advances on Smart TiO Nanotube Platforms for Sustainable Drug Delivery Applications. Int. J. Nanomed. 12, 151–165. doi:10.2147/ijn.S117498
Wang, S., Wang, H., Ren, B., Li, H., Weir, M. D., Zhou, X., et al. (2017b). Do quaternary Ammonium Monomers Induce Drug Resistance in Cariogenic, Endodontic and Periodontal Bacterial Species? Dental Mater. : official Publ. Acad. Dental Mater. 33 (10), 1127–1138. doi:10.1016/j.dental.2017.07.001
Wang, C., Wang, Y., Zhang, L., Miron, R. J., Liang, J., Shi, M., et al. (2018a). Pretreated Macrophage-Membrane-Coated Gold Nanocages for Precise Drug Delivery for Treatment of Bacterial Infections. Adv. Mater. 30 (46), 1804023. doi:10.1002/adma.201804023
Wang, J., Meng, F., Song, W., Jin, J., Ma, Q., Fei, D., et al. (2018b). Nanostructured Titanium Regulates Osseointegration via Influencing Macrophage Polarization in the Osteogenic Environment. Int. J. Nanomed. 13, 4029–4043. doi:10.2147/ijn.S163956
Wang, S., Yang, Y., Li, W., Wu, Z., Li, J., Xu, K., et al. (2019). Study of the Relationship between Chlorhexidine-Grafted Amount and Biological Performances of Micro/Nanoporous Titanium Surfaces. ACS omega 4 (19), 18370–18380. doi:10.1021/acsomega.9b02614
Wang, R., Shi, M., Xu, F., Qiu, Y., Zhang, P., Shen, K., et al. (2020). Graphdiyne-modified TiO2 Nanofibers with Osteoinductive and Enhanced Photocatalytic Antibacterial Activities to Prevent Implant Infection. Nat. Commun. 11 (1), 4465. doi:10.1038/s41467-020-18267-1
Wang, L., Zhou, W., Yu, Z., Yu, S., Zhou, L., Cao, Y., et al. (2021a). An In Vitro Evaluation of the Hierarchical Micro/Nanoporous Structure of a Ti3Zr2Sn3Mo25Nb Alloy after Surface Dealloying. ACS Appl. Mater. Inter. 13 (13), 15017–15030. doi:10.1021/acsami.1c02140
Wang, X., Xu, K., Cui, W., Yang, X., Maitz, M. F., Li, W., et al. (2021b). Controlled Synthesis of Mussel-Inspired Ag Nanoparticle Coatings with Demonstrated In Vitro and In Vivo Antibacterial Properties. Mater. Des. 208, 109944. doi:10.1016/j.matdes.2021.109944
Wisdom, E., Zhou, Y., Chen, C., Tamerler, C., and Snead, M. (2020). Mitigation of Peri-Implantitis by Rational Design of Bifunctional Peptides with Antimicrobial Properties. ACS Biomater. Sci. Eng. 6 (5), 2682–2695. doi:10.1021/acsbiomaterials.9b01213
Wu, H., Xie, L., He, M., Zhang, R., Tian, Y., Liu, S., et al. (2019). A Wear-Resistant TiO Nanoceramic Coating on Titanium Implants for Visible-Light Photocatalytic Removal of Organic Residues. Acta Biomater. 97, 597–607. doi:10.1016/j.actbio.2019.08.009
Xie, Y., Liu, M., and Zhou, J. (2012). Molecular Dynamics Simulations of Peptide Adsorption on Self-Assembled Monolayers. Appl. Surf. Sci. 258 (20), 8153–8159. doi:10.1016/j.apsusc.2012.05.013
Xie, K., Zhou, Z., Guo, Y., Wang, L., Li, G., Zhao, S., et al. (2019). Long-Term Prevention of Bacterial Infection and Enhanced Osteoinductivity of a Hybrid Coating with Selective Silver Toxicity. Adv. Healthc. Mater. 8 (5), e1801465. doi:10.1002/adhm.201801465
Xu, N., Fu, J., Zhao, L., Chu, P. K., and Huo, K. (2020). Biofunctional Elements Incorporated Nano/Microstructured Coatings on Titanium Implants with Enhanced Osteogenic and Antibacterial Performance. Adv. Healthc. Mater. 9 (23), 2000681. doi:10.1002/adhm.202000681
Xue, T., Attarilar, S., Liu, S., Liu, J., Song, X., Li, L., et al. (2020). Surface Modification Techniques of Titanium and its Alloys to Functionally Optimize Their Biomedical Properties: Thematic Review. Front. Bioeng. Biotechnol. 8 (1261), 603072. doi:10.3389/fbioe.2020.603072
Yang, Z., Xi, Y., Bai, J., Jiang, Z., Wang, S., Zhang, H., et al. (2021). Covalent Grafting of Hyperbranched Poly-L-Lysine on Ti-Based Implants Achieves Dual Functions of Antibacteria and Promoted Osteointegration In Vivo. Biomaterials 269, 120534. doi:10.1016/j.biomaterials.2020.120534
Yao, Q., Jiang, Y., Tan, S., Fu, X., Li, B., and Liu, L. (2020). Composition and Bioactivity of Calcium Phosphate Coatings on Anodic Oxide Nanotubes Formed on Pure Ti and Ti-6Al-4V alloy Substrates. Mater. Sci. Eng. C, Mater. Biol. Appl. 110, 110687. doi:10.1016/j.msec.2020.110687
Yao, M., Cheng, S., Zhong, G., Zhou, J., Shao, H., Ma, L., et al. (2021). Enhanced Osteogenesis of Titanium with nano-Mg(OH) Film and a Mechanism Study via Whole Genome Expression Analysis. Bioactive Mater. 6 (9), 2729–2741. doi:10.1016/j.bioactmat.2021.02.003
Ye, J., Li, B., Li, M., Zheng, Y., Wu, S., and Han, Y. (2020). ROS Induced Bactericidal Activity of Amorphous Zn-Doped Titanium Oxide Coatings and Enhanced Osseointegration in Bacteria-Infected Rat Tibias. Acta Biomater. 107, 313–324. doi:10.1016/j.actbio.2020.02.036
Ye, J., Huang, B., and Gong, P. (2021). Nerve Growth Factor-Chondroitin Sulfate/hydroxyapatite-Coating Composite Implant Induces Early Osseointegration and Nerve Regeneration of Peri-Implant Tissues in Beagle Dogs. J. Orthop. Surg. Res. 16 (1), 51. doi:10.1186/s13018-020-02177-5
Yin, D., Komasa, S., Yoshimine, S., Sekino, T., and Okazaki, J. (2019). Effect of Mussel Adhesive Protein Coating on Osteogenesis In Vitro and Osteointegration In Vivo to Alkali-Treated Titanium with Nanonetwork Structures. Int. J. Nanomed. 14, 3831–3843. doi:10.2147/ijn.S206313
Yu, M., Wan, Y., Ren, B., Wang, H., Zhang, X., Qiu, C., et al. (2020a). 3D Printed Ti-6Al-4V Implant with a Micro/Nanostructured Surface and its Cellular Responses. ACS omega 5 (49), 31738–31743. doi:10.1021/acsomega.0c04373
Yu, S., Guo, D., Han, J., Sun, L., Zhu, H., Yu, Z., et al. (2020b). Enhancing Antibacterial Performance and Biocompatibility of Pure Titanium by a Two-step Electrochemical Surface Coating. ACS Appl. Mater. Inter. 12 (40), 44433–44446. doi:10.1021/acsami.0c10032
Yuan, Z., Tao, B., He, Y., Mu, C., Liu, G., Zhang, J., et al. (2019). Remote Eradication of Biofilm on Titanium Implant via Near-Infrared Light Triggered Photothermal/photodynamic Therapy Strategy. Biomaterials 223, 119479. doi:10.1016/j.biomaterials.2019.119479
Zeng, Y., Yang, Y., Chen, L., Yin, D., Zhang, H., Tashiro, Y., et al. (2019). Optimized Surface Characteristics and Enhanced In Vivo Osseointegration of Alkali-Treated Titanium with Nanonetwork Structures. Int. J. Mol. Sci. 20 (5), 1127. doi:10.3390/ijms20051127
Zhang, Y., Han, Y., and Zhang, L. (2015). Interfacial Structure of the Firmly Adhered TiO2 Nanotube Films to Titanium Fabricated by a Modified Anodization. Thin Solid Films 583, 151–157. doi:10.1016/j.tsf.2015.03.060
Zhang, M., Huang, X., Hang, R., Zhang, X., and Tang, B. (2018a). Effect of a Biomimetic Titania Mesoporous Coating Doped with Sr on the Osteogenic Activity. Mater. Sci. Eng. C Mater. Biol. Appl. 91, 153–162. doi:10.1016/j.msec.2018.05.036
Zhang, X., Geng, H., Gong, L., Zhang, Q., Li, H., Zhang, X., et al. (2018b). Modification of the Surface of Titanium with Multifunctional Chimeric Peptides to Prevent Biofilm Formation via Inhibition of Initial Colonizers. Int. J. Nanomed. 13, 5361–5375. doi:10.2147/ijn.S170819
Zhang, Y., Liu, X., Li, Z., Zhu, S., Yuan, X., Cui, Z., et al. (2018c). Nano Ag/ZnO-Incorporated Hydroxyapatite Composite Coatings: Highly Effective Infection Prevention and Excellent Osteointegration. ACS Appl. Mater. Inter. 10 (1), 1266–1277. doi:10.1021/acsami.7b17351
Zhang, Z., Xie, Y., Pan, H., Huang, L., and Zheng, X. (2018d). Influence of Patterned Titanium Coatings on Polarization of Macrophage and Osteogenic Differentiation of Bone Marrow Stem Cells. J. Biomater. Appl. 32 (7), 977–986. doi:10.1177/0885328217746802
Zhang, G., Zhang, X., Yang, Y., Chi, R., Shi, J., Hang, R., et al. (2020). Dual Light-Induced In Situ Antibacterial Activities of biocompatibleTiO2/MoS2/PDA/RGD Nanorod Arrays on Titanium. Biomater. Sci. 8 (1), 391–404. doi:10.1039/C9BM01507H
Zhang, L., Xue, Y., Gopalakrishnan, S., Li, K., Han, Y., and Rotello, V. M. (2021). Antimicrobial Peptide-Loaded Pectolite Nanorods for Enhancing Wound-Healing and Biocidal Activity of Titanium. ACS Appl. Mater. Inter. 13 (24), 28764–28773. doi:10.1021/acsami.1c04895
Zhang, G., Wu, Z., Yang, Y., Shi, J., Lv, J., Fang, Y., et al. (2022). A Multifunctional Antibacterial Coating on Bone Implants for Osteosarcoma Therapy and Enhanced Osteointegration. Chem. Eng. J. 428, 131155. doi:10.1016/j.cej.2021.131155
Zhang, J. (2014). Choice of Treatment for Dental Implant Restoration in Patients with Severe Periodontitis. Chin. J. Stomatol. 6 (49), 324–327. doi:10.3760/cma.j.issn.1002-0098.2014.06.002
Zhao, Y.-p., Wei, J.-l., Tian, Q.-y., Liu, A. T., Yi, Y.-S., Einhorn, T. A., et al. (2016). Progranulin Suppresses Titanium Particle Induced Inflammatory Osteolysis by Targeting TNFα Signaling. Sci. Rep. 6 (1), 20909. doi:10.1038/srep20909
Zhao, Q.-M., Sun, Y.-Y., Wu, C.-S., Yang, J., Bao, G.-F., and Cui, Z.-M. (2020a). Enhanced Osteogenic Activity and Antibacterial Ability of Manganese-Titanium Dioxide Microporous Coating on Titanium Surfaces. Nanotoxicology 14 (3), 289–309. doi:10.1080/17435390.2019.1690065
Zhao, X., Ren, X., Wang, C., Huang, B., Ma, J., Ge, B., et al. (2020b). Enhancement of Hydroxyapatite Formation on Titanium Surface by Alkali Heat Treatment Combined with Induction Heating and Acid Etching. Surf. Coat. Technol. 399, 126173. doi:10.1016/j.surfcoat.2020.126173
Zhou, R., Wei, D., Feng, W., Cheng, S., Yang, H., Li, B., et al. (2014). Bioactive Coating with Hierarchical Double Porous Structure on Titanium Surface Formed by Two-step Microarc Oxidation Treatment. Surf. Coat. Technol. 252, 148–156. doi:10.1016/j.surfcoat.2014.04.061
Zhou, R., Wei, D., Cao, J., Feng, W., Cheng, S., Du, Q., et al. (2015). Conformal Coating Containing Ca, P, Si and Na with Double-Level Porous Surface Structure on Titanium Formed by a Three-step Microarc Oxidation. RSC Adv. 5 (37), 28908–28920. doi:10.1039/C4RA14685A
Zhou, W., Peng, X., Ma, Y., Hu, Y., Wu, Y., Lan, F., et al. (2020). Two-staged Time-dependent Materials for the Prevention of Implant-Related Infections. Acta Biomater. 101, 128–140. doi:10.1016/j.actbio.2019.10.023
Zhou, Z., Shi, Q., Wang, J., Chen, X., Hao, Y., Zhang, Y., et al. (2021). The Unfavorable Role of Titanium Particles Released from Dental Implants. Nanotheranostics 5 (3), 321–332. doi:10.7150/ntno.56401
Zhu, Z., Li, X., Li, Y., Zhu, L., Zhu, C., Che, Z., et al. (2021). Three-dimensionally Printed Porous Biomimetic Composite for Sustained Release of Recombinant Human Bone Morphogenetic Protein 9 to Promote Osteointegration. Mater. Des. 208, 109882. doi:10.1016/j.matdes.2021.109882
Zhuang, Y., Ren, L., Zhang, S., Wei, X., Yang, K., and Dai, K. (2021). Antibacterial Effect of a Copper-Containing Titanium alloy against Implant-Associated Infection Induced by Methicillin-Resistant Staphylococcus aureus. Acta Biomater. 119, 472–484. doi:10.1016/j.actbio.2020.10.026
Keywords: orthopedic titanium implants, titanium implants, osseointegration, anti-infection, functional coatings
Citation: Lu X, Wu Z, Xu K, Wang X, Wang S, Qiu H, Li X and Chen J (2021) Multifunctional Coatings of Titanium Implants Toward Promoting Osseointegration and Preventing Infection: Recent Developments. Front. Bioeng. Biotechnol. 9:783816. doi: 10.3389/fbioe.2021.783816
Received: 27 September 2021; Accepted: 25 October 2021;
Published: 07 December 2021.
Edited by:
Jingan Li, Zhengzhou University, ChinaReviewed by:
Tao Liu, Guangzhou University of Chinese Medicine, ChinaJin Wang, Southwest Jiaotong University, China
Lumei Liu, Abigail Wexner Research Institute, United States
Copyright © 2021 Lu, Wu, Xu, Wang, Wang, Qiu, Li and Chen. This is an open-access article distributed under the terms of the Creative Commons Attribution License (CC BY). The use, distribution or reproduction in other forums is permitted, provided the original author(s) and the copyright owner(s) are credited and that the original publication in this journal is cited, in accordance with accepted academic practice. No use, distribution or reproduction is permitted which does not comply with these terms.
*Correspondence: Jialong Chen, jialong_dt@126.com; Xiangyang Li, hlxiangyang@163.com
†These authors have contributed equally to this work