Electrospinning of botanicals for skin wound healing
- 1Department of Biomedical Engineering and Technology, Institute of Basic Theory for Chinese Medicine, China Academy of Chinese Medical Sciences, Beijing, China
- 2Department of Dermatology, Guang’anmen Hospital, China Academy of Chinese Medical Sciences, Beijing, China
Being the first barrier between the human body and external environments, our skin is highly vulnerable to injuries. As one of the conventional therapies, botanicals prepared in different topical formulations have been applied as medical care for centuries. With the current increase of clinical requirements, applications of botanicals are heading towards nanotechnologies, typically fused with electrospinning that forms nanofibrous membranes suitable for skin wound healing. In this review, we first introduced the main process of wound healing, and then presented botanicals integrated into electrospun matrices as either loaded drugs, or carriers, or membrane coatings. In addition, by addressing functional features of individual botanicals in the healing of injured skin, we further discussed the bioactivity of botanical electrospun membranes in relevant to the medical issues solved in the process of wound healing. As achieved by pioneer studies, due to infrequent adverse effects and the diversity in resources of natural plants, the development of electrospun products based on botanicals is gaining greater attention. However, investigations in this field have mainly focused on different methodologies used in the preparation of nanofibrous membranes containing botanicals, their translation into clinical practices remains unaddressed. Accordingly, we propose that potential clinical applications of botanical electrospun membranes require not only the further expansion and understanding of botanicals, but also an establishment of standard criteria for the evaluation of wound healing and evolutions of technologies to support the large-scale manufacturing industry.
1 Introduction
The skin is the first physical barrier of human body that serves a variety of pivotal functions, from preventing external pathogens to conveying somatosensory signals and regulating temperature and hydration. Due to a direct contact with external environments, our skin is extremely labile to injuries, which often lead to bacterial infections and even life-threatening complications. To facilitate the natural healing process, wound dressings that provide favorable environmental conditions for the healing of skin injuries are generally required. Conventionally, cream, gauze, and cotton wool are the major management for wound care; however, their poor air permeability, short residence time, uncontrollable drug dosages, and the resultant infections have put forward the evolution of wound dressings much more than just a topical cover. Currently, wound-care technologies are tremendously expanding to nanotechnology engineering, the application of which has shown excellent outcomes (Kalashnikova et al., 2015). Among different nanomaterials, nanofibers prepared by electrospinning have been extensively implemented as optimal dressing materials owing to their superior physical and mechanical properties. In particular, the high porosity, small diameters and the large surface area have endowed electrospun nanofibers with core competencies, such as excellent breathability and effective control of drug release (Yang and Leong, 2010; Ye and KuangYouMorsiMo, 2019; Hernandez and Woodrow, 2022). Moreover, the relatively low cost and the ease of manufacture have made electrospun nanofibers suitable for a large-scale production.
Botanicals have been developed and utilized as medical care for centuries similar to that of modern pharmaceuticals. Compared to pharmaceutical drugs, botanical ingredients integrated into dermatological preparations are witnessing sharp popularity because of their infrequent adverse effects and diversities in resources and bioactive ingredients (Fuhrmann et al., 2010). Though increasingly attractive, the ancient producing process of topical formulations in phytomedicine has hindered the development and generalization of phytotherapies. In this scenario, a variety of studies have attempted to formulate botanical wound dressings by incorporating electrospinning technologies. In that way, benefits in air permeability, moisture maintenance, exudes absorption, controlled drug release, and the subsequent reduction of infections have been achieved (Sundaramurthi et al., 2014).
Hence, this review aims to present the progress in the fundamental research of electrospun nanofibers with the application of botanicals, focusing on the advanced methodologies employed and medical issues solved during the process of wound healings (Figure 1). Moreover, to put forward the clinical applications of botanical-containing electrospun nanofibers, we propose strategic suggestions to optimize the roadmap of botanical electrospun products.
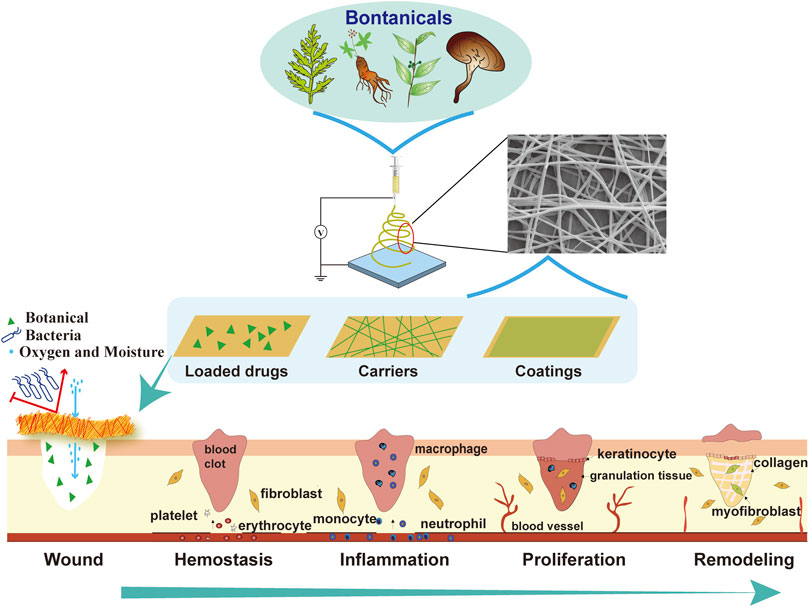
FIGURE 1. Electrospinning of botanicals for skin wound healing. A variety of botanicals can be electrospun as wound dressings in the form of loaded drugs, or carriers, or coatings, to provide a suitable microenvironment for different stages of wound healing, including hemostasis, inflammation, proliferation and remodeling.
2 Wound healing
2.1 The process of wound healing
Wound healing is a dynamic and highly regulated process of cellular, humoral and molecular mechanisms, comprising a series of pathophysiological alterations of four phases, which include hemostasis, inflammation, proliferation, and remodeling (Wilkinson and Hardman, 2020). In general, immediately after injury, blood vessels around the wound undergo reactive contractions, with aggregations of platelets due to collagen exposure, leading to the formation blood clots and extracellular matrix at the injury site. The local necrosis and release of vasoactive substances often cause acute inflammatory responses, as manifested as congestion, plasma exudation, and leukocyte excretion. During this stage, injured cells and pathogens are generally removed from the wound area by immune cells, through mechanisms induced by phagocytosis and reactive oxygen species (ROS). Meanwhile, two opposite activations of macrophages known as the pro-inflammatory M1 and the tissue-remodeling M2 are initiated, with the former gradually converts into the latter to drive wound healings from inflammatory responses to the proliferation phase (Kang et al., 2018; Kim et al., 2019). During the proliferation step, fibroblasts migrate, proliferate, and secrete large amounts of collagen and extracellular matrix, resulting in the formation of granulation tissues (Driskell et al., 2013). When the growing of granulation tissues persists, the skin remodeling is consequently triggered, which is characterized by the development of scar tissues that have disturbed alignment of collagen fibers and excessive dermal fibrosis. Therefore, the complex process of wound healing requires a suitable microenvironment that sequentially functions to repair damaged tissues and facilitates the overall restoration of skin function and texture (Guo and Dipietro, 2010; Broussard and Powers, 2013).
2.2 Current wound dressings
With the recognition of the complexity of wound healings, wound dressings have evolved from traditional passive dressings in the available forms of gauze, bandages, and cotton wool, to interactive dressings like films, foams, and hydrogels, then to modern bioactive dressings that are produced by biomaterials (Aderibigbe and Buyana, 2018; Farahani and Shafiee, 2021). Passive dressings, which are non-occlusive, are used to cover wounds and absorb exudates. Since absorptions of wound exudates often result in secondary injuries due to the adhesion of dressings to the damaged area, they practically fulfill few protections (Fraccalvieri et al., 2011). The interactive dressings, which are designed based on interactions between dressings and wounds, are semi-occlusive or occlusive (Mostafalu et al., 2018). Practically, interactive dressings are preferred as a physical barrier against microorganisms in addition to facilitations of healing by enhancing exudates absorption and gas exchange. As for bioactive dressings, their production involves combinations of polymer materials and bioactive substances with high-tech approaches. Compared to the first two forms, bioactive dressings, such as electrospun membranes, have shown their advantages in terms of retaining moisturization and delivering medicinal materials which are conducive to accelerate the healing process (Sood et al., 2014).
3 Electrospinning of botanicals for skin wound healing
3.1 Electrospun nanofibers
Electrospun nanofibers are organized filaments that are fabricated under a strong electric field force. The innate properties of electrospun matrix like the high surface-to-volume ratio and porosity in structure have enabled nanofibrous mats suitable for wound healing (Rieger et al., 2013). Besides, by modulating the arrangement of nanofibers, the controlled release of loaded drugs and the superior mechanical properties which potentially assist to adapt to limb activities can be realized. Regarding materials used for electrospinning, various polymers can be selected according to specific requirements of impaired skin. For example, the poly (lactic-co-glycolic acid), polycaprolactone and silk fibroin as scaffolds provide favorable mechanical properties, and materials like hyaluronic acid, chitosan and polyethylene oxide are polymers with antibacterial effects (Wang et al., 2020a; Ionescu et al., 2021; Luo et al., 2021; Sun et al., 2021; Wu et al., 2022; Zamboni et al., 2023). Besides, the alginate, poly (vinyl alcohol) and gelatin as absorbent polymers are selected to remove wound exudates and keep the wound moist (Yang et al., 2021; Yang et al., 2022). In addition, electrospun nanofibers formed by polymers have favorable biocompatibility and biodegradability, which practically aid in reducing biological heterogeneities (Sheikholeslam et al., 2018; Soares et al., 2018). Overall, the unique advantages of electrospun nanofibers have appeared to be ideal solutions to solve existing problems of topical therapeutics.
3.2 Incorporative strategies of botanicals into electrospun nanofibers
3.2.1 As loaded drugs
The slow onset of therapeutic effects and a higher effective dosage needed due to low bio-availabilities of botanical components in conventional topical formulations constitute the main obstacles preventing the successfully clinical translation of botanicals (Reuter et al., 2010). Though the introduction of electrospinning technology has shown its value in optimizing the unsatisfactory features of botanicals as topical treatments, the poor water solubility and low chemical stability of botanicals have substantially curtailed their loading potentials into nanofibers. To effectively load botanicals into electrospun membranes, both needleless and needle systems have been reported, the latter of which is currently the predominant methodology and can be further categorized as blend, coaxial and multi-jet according to the number of needles installed and parameters set (Table 1).
3.2.1.1 Blending electrospinning
Blending electrospinning involves the mixture of more than two materials into one solution, of which only one material solution is electrospinnable. Typically, in the case when botanicals are not able to be electrospun, electrospinnable polymers are generally added as a companion. For instance, as one of the main components of clove oil, which has wound-healing effects, eugenol is unstable and poorly soluble (Banerjee et al., 2020; Nisar et al., 2021). By designing cyclodextrin inclusion complexes using electrospinning, an enhanced thermal stability and water solubility of eugenol was obtained (Celebioglu et al., 2018). In addition to advances in physical performance, electrospinning-based botanical nanofibers generally exhibit a biphasic mode of drug release. Since the initial burst release caused by drug diffusion from the fiber surface may enable a rapid production of required pharmacological responses, and the subsequent sustained drug release from the interior of nanostructures is beneficial to maintain a similar bioactivity over extended periods, the biphasic mode of drug release provides more flexible controls for botanicals with various therapeutic effects. For example, to take full advantages of antioxidant and anti-inflammatory properties of curcumin, healing potentials of curcumin-loaded silk fibroin nanofibers in combination with hydrophobic polycaprolactone and hydrophilic polyvinyl alcohol (PVA) has been investigated in diabetic wounds. In vitro assessment showed that approximately 20–28% curcumin release occurred within the first 1 h, followed by an increased drug release to 53–75% at 12 h. This biphasic curcumin release was proved essential to restore the normal skin structure of diabetic wounds (Agarwal et al., 2021).
3.2.1.2 Coaxial electrospinning
Considering that some drugs are susceptible to denaturation or inactivation in organic solvents, core-shell nanofibers produced by coaxial electrospinning have been established (Sun et al., 2019). In the double-layer structure of core-shell nanofibers, unstable botanicals can be preserved in the core, while the shell could be utilized to carry stable components according to specific therapeutic demands. The other contribution of coaxial electrospinning is the arrangement of sequential drug release by adjusting either the thickness of shells or the chemical properties of polymers. For example, lidocaine hydrochloride has been constructed with chitosan/polyethylene oxide (PEO) as the shell for the relief of the pain, while anti-inflammatory curcumin was introduced into the core composed of polycaprolactone. In vitro drug release analysis showed that about 21.31% lidocaine release could be detected from the shell at 0.5 h, which was significantly higher compared to 8.89% curcumin released from the core. Then, after 72 h, when lidocaine release (57.43%) tended to be stable, the detected free curcumin raised to 68.24%. The sequential drug release from coaxial nanofibers was shown to provide both immediate analgesic effects and long-term antibacterial activities. Interestingly, the controlled drug release could be additionally achieved by adjusting the pH of solutions, because chitosan is soluble in acidic environments. Furthermore, since sodium bicarbonate (NaHCO3) reacts with hydrogen ions to form CO2, the addition of NaHCO3 to the curcumin/polycaprolactone core potentially promoted formations of fiber pores leading to enhanced curcumin discharge (Guo et al., 2020).
3.2.1.3 Multi-jet electrospinning
In multi-jet electrospinning, different solution systems are proposed to work simultaneously to promote multi-drug delivery and physical properties of fibrous membranes. As illustrated in the treatment of chronic diabetic ulcers, to enhance mechanical and thermal stabilities of nanofibrous scaffolds loaded with antibacterial cerium oxide nanoparticles (nCeO2), a dual spinneret electrospinning technique involving fabrications of PVA/gelatin incorporated with nCeO2 and polyurethane containing cinnamon essential oil was arranged (Hussein et al., 2022). With additions of cinnamon essential oil, the strength of scaffolds was augmented from 5.16 ± 0.41 to 9.12 ± 1.52 MPa, which met the most suitable tensile strength criterion of wound healing (Cui et al., 2009). Besides, the multi-jet electrospinning has been employed to facilitate industrial large-scale productions of nanofibers, though several practical issues have been raised recently (Kumar et al., 2010; Wu et al., 2018; Vass et al., 2020).
3.2.2 As carriers
Diversities in compositions and structures of natural plants offer a wealth of opportunities for botanicals as polymers in electrospinning. Natural polymers, such as lignin, guar gum, karaya gum, mucilage and pectin from hibiscus leaves have been used in electrospinning to improve physicochemical properties of wound dressings (Table 2). Gum arabic is a natural polysaccharide from the trunk exudate of Acacia trees. By using gum arabic and the corn protein zein, together with polycaprolactone polymer as a scaffold, studies have shown that the achieved hydrophilicity, elasticity, antibacterial potencies, and the cell penetration strength of nanofibers were mainly attributed to gum arabic, confirming that botanicals hold potentials to function as natural polymers for electrospinning in wound healings (Pedram Rad et al., 2018).
3.2.3 As coatings
In respect that some botanicals with excellent efficacy in promoting wound healing may not be suitable for electrospinning due to the exposure to harsh organic solvents, alternative modifications, such as coating, to directly immobilize botanicals into nanofibrous matrices have been proposed. Compared to methods that incorporate botanicals during electrospinning, the approach of coatings is more flexible and convenient in preparations (Albright et al., 2018). Moreover, physicochemical properties of the fiber surface could be easily remodeled by a cover of botanical envelopes, leading to promoted cell adhesion while reduced water evaporation (Jia et al., 2017; Richbourg et al., 2019). Aloe vera gel has wound-healing potentials due to its antioxidant, antibacterial, anti-inflammatory and anti-itch activities (Kang et al., 2014; Sánchez et al., 2020). In particular, the presence of carboxypeptidase and glucomannan in aloe stimulates fibroblast proliferation and promotes the synthesis and maturation of collagens (Nazeam et al., 2017; Gao et al., 2019; Zhang et al., 2021). The application of aloe gel as coatings for nanofibrous matrices has been attempted in l-polylactic acid (PLLA)-based nanofibers. By evaluating the healing process of mice with full-thickness skin defects, PLLA-coated with aloe had a faster onset of healing and an accelerated rate of wound-repairing compared to that without coating. Particularly, being highly hydrophobic, PLLA scaffold is prone to adhesions of S. aureus, the most important bacteria that cause human diseases, hence the covering by aloe vera gel simultaneously controlled potential bacterial infections (Jouybar et al., 2017).
3.3 Wound-healing effects of botanical electrospun nanofibers
3.3.1 Hemostasis
Effective hemostasis is the prerequisite for wound healing. In addition to intrinsic mechanisms of hemostasis, ideal hemostatic materials that are efficient for blood clotting without causing secondary bleeding when being removed are clinically in need. Take the isoquinoline alkaloid berberine as an example. By embedding berberine in polycaprolactone nanofibrous membranes, a stronger capacity of blood clotting and a higher rate of platelet adhesion were observed. Moreover, the incorporation of berberine retained its antimicrobial potentials and improved the permeability and buffering properties of polycaprolactone matrix (Bao et al., 2013).
3.3.2 Inflammation
During wound healing, adequate inflammatory responses ensure the transition of repair process to the phase of proliferation, whereas a persistent inflammation is detrimental. Shikonin, a naphthoquinone extracted from the root of Lithospermum erythrorhizon, has been loaded into polycaprolactone/polytrimethylene carbonate ultrafine fiber mats and exhibited antibacterial effects against S. aureus and E. coli compared to polymers alone (Han et al., 2009). Moreover, it was reported that an excessive ROS production by immune cells was harmful for wound healings, and applications of antioxidants are alternative ways to alleviate inflammation (Roy et al., 2006). For instance, the antioxidant fenugreek seed, which mainly contains trigonelline, naringenin, nicotinic acid, quercetin and saponins, has been introduced into nanofibers made of silk proteins to facilitate wound healings (Selvaraj and Fathima, 2017). Similarly, chrysin and grape seed extracts, which reduce oxidative stress, have been experimentally applied as wound dressings when loaded in polycaprolactone/polyethylene glycol or silk fibroin/polyethylene glycol, respectively (Lin et al., 2016; Deldar et al., 2018). Additionally, through regulations of pro-inflammatory cytokines, such as IL-1β and TNF-α, the artemisinin originally derived from the sweet wormwood (Artemisia annua), was incorporated into poly (lactic-co-glycolic acid)/silk fibroin and showed a shortened inflammatory cycle with enhanced skin regeneration in a rat model of dorsal full-thickness wounds (Peng et al., 2021). Likewise, by a direct effect on macrophages, ginsenoside Rg1 integrated into a novel asymmetric wettable electrospun membrane displayed a promoted macrophage polarization towards M2 phenotype, therefore facilitated a better infected wound healing compared to commercial Aquacel Ag dressings (Zhou et al., 2022).
3.3.3 Proliferation
The proliferation phase of wound healing is characterized by collagen deposition, angiogenesis, keratinocyte-associated re-epithelialization, and fibroblast-related granulation tissue formation. In particular, the re-epithelialization and granulation tissue formation are the determining factors (Beavers et al., 2015; Martin and Nunan, 2015; Chattopadhyay et al., 2016; Foster et al., 2021). The loading of beet roots extracts Beta vulgaris into nylon 66 polyamide was shown effective in promoting the differentiation of keratinocyte-mesenchyme stem cell to epithelial linage during the healing process, which was associated with increased expression of cytokeratin 10, cytokeratin 14 and loricrin (Hosseinzadeh et al., 2017). When loading Calendula officinalis to polycaprolactone/zein/gum arabic, the polycaprolactone/zein/gum arabic/Calendula officinalis nanocomposite enhanced the proliferation and adhesion of fibroblasts for regenerating skin by mechanisms related to collagen production (Pedram Rad et al., 2019). Consistently, the Calendula officinalis-loaded chitosan/PEO nanofibers revealed excellent wound healing capabilities through enhanced collagen synthesis and deposition (Kharat et al., 2021).
3.3.4 Remodeling
Hypertrophic scar formed by excessive cell proliferation during the healing process constitutes a serious issue that has great impacts on the life quality of patients (Finnerty et al., 2016). Current studies have shown that the formation of hypertrophic scars is closely related to excessive inflammation, overactivation of fibroblasts, and excessive proliferation of blood vessels (Wilgus, 2019; Wang et al., 2020b; Vorstandlechner et al., 2021; Chen et al., 2022). Based on the evidence that 20(R)ginsenoside Rg3 is effective in inhibiting the production of pro-inflammatory cytokines and down-regulating VEGF expressions, Rg3 was incorporated with polylactic acid as wound dressings (Seo et al., 2008; Tang et al., 2018; Ma et al., 2021; Nakhjavani et al., 2021). In vivo evidence showed that Rg3-containing membranes inhibited the early formation and late proliferation of hydrotropic scars, as demonstrated as reduced proliferation of fibroblasts, and minimized thicknesses of dermis and epidermis (Cheng et al., 2013).
4 Conclusion and future perspectives
Electrospinning has been involved in a broad range of applications, showing great potentials in regenerative medicine, gas filtration, food packaging and environmental purification (Chen et al., 2018; Chen et al., 2020; Das et al., 2020; Min et al., 2022). With continuous endeavors in developing botanicals into electrospun products, the proportion of electrospun wound dressings with medicinal plants in fundamental research is progressively increasing. Compared to synthetic polymers or pharmaceutical drugs/coatings, natural materials have better biocompatibility, bioactivity, and biodegradability, though drawbacks such as compromised flexibility and mechanical strength have been reported (DeFrates et al., 2018). Moreover, owing to their versatility in bioactivities, no or fewer side effects, and environmental friendliness, botanicals may create more possibilities for clinical applications, including but not limited to wound healings. In particular, the conventional bulk electrospinning machines have been upgraded to clinically available hand-held devices, which reduce difficulties in the access to electrospinning, therefore allows an immediate application of dressings according to a specific condition of injured skin (Yan et al., 2019).
At present, investigations in this field mainly focus on different methods in the preparation of botanical nanofibers, whereas their translation into clinical remains unaddressed. To put forward the clinical application of botanical-containing electrospun membranes, several aspects require additional efforts. Firstly, even though the bioactivities of a certain number of botanicals have been reported, their mechanisms of action kept largely uncertain. Therefore, a molecular level exploration of the individual compound in the process of wound healing needs to be emphasized. Secondly, since the wound healing process in clinics involves an orchestration of different cells and molecules, a continuous screening of herbals and their derivatives suitable for wound dressings is of particular importance in the context of complex clinical situations. Thirdly, in addition to effectiveness in wound healing, the improvement in practical issues, such as moisturization and tensile resistance remain to be solved. Fourthly, besides the necessity to formulate criteria for wound dressing, the establishment of standard or comparable experimental models appear to be primarily urgent. Finally, the commercial value of botanical electrospun nanofibers needs to be further reinforced, and the technology for a large-scale production should be developed along with the progress in the basic research.
Author contributions
SG and PW contributed equally to the literature search, data integration, and writing. NL and PS instructed, wrote, and revised the manuscript. All authors contributed to manuscript revision, read, and approved the submitted version.
Funding
The laboratory of the authors benefits from ongoing support from the Scientific and Technological Innovation project, China Academy of Chinese Medical Science (CI 2021A00112, CI 2021B001), the Beijing Traditional Chinese Medicine Technology Development Foundation (JJ-2020-85), the National Natural Science Foundation of China (82074448), the Fundamental Research Funds for the Central Public Welfare Research Institutes (YZ-202110, YZ-202119).
Conflict of interest
The authors declare that the research was conducted in the absence of any commercial or financial relationships that could be construed as a potential conflict of interest.
Publisher’s note
All claims expressed in this article are solely those of the authors and do not necessarily represent those of their affiliated organizations, or those of the publisher, the editors and the reviewers. Any product that may be evaluated in this article, or claim that may be made by its manufacturer, is not guaranteed or endorsed by the publisher.
References
Aderibigbe, B. A., and Buyana, B. (2018). Alginate in wound dressings. Pharmaceutics 10 (2), 42. doi:10.3390/pharmaceutics10020042
Agarwal, Y., Rajinikanth, P., Ranjan, S., Tiwari, U., Balasubramnaiam, J., Pandey, P., et al. (2021). Curcumin loaded polycaprolactone-/polyvinyl alcohol-silk fibroin based electrospun nanofibrous mat for rapid healing of diabetic wound: An in-vitro and in-vivo studies. Int. J. Biol. Macromol. 176 (7), 376–386. doi:10.1016/j.ijbiomac.2021.02.025
Albright, V., Xu, M., Palanisamy, A., Cheng, J., Stack, M., Zhang, B., et al. (2018). Skin wound healing: Micelle-coated, hierarchically structured nanofibers with dual-release capability for accelerated wound healing and infection control (adv. Healthcare mater. 11/2018). Adv. Healthc. Mat. 7 (11), 1870045. doi:10.1002/adhm.201870045
Anand, S., Rajinikanth, P. S., Arya, D. K., Pandey, P., Gupta, R. K., Sankhwar, R., et al. (2022). Multifunctional biomimetic nanofibrous scaffold loaded with asiaticoside for rapid diabetic wound healing. Pharmaceutics 14 (2), 273. doi:10.3390/pharmaceutics14020273
Banerjee, K., Madhyastha, H., Sandur V., R., N.t., M., and Thiagarajan, P. (2020). Anti-inflammatory and wound healing potential of a clove oil emulsion. Colloids Surfaces B Biointerfaces 193, 111102. doi:10.1016/j.colsurfb.2020.111102
Bao, J., Yang, B., Sun, Y., Zu, Y., and Deng, Y. (2013). A berberine-loaded electrospun poly-(epsilon-caprolactone) nanofibrous membrane with hemostatic potential and antimicrobial property for wound dressing. J. Biomed. Nanotechnol. 9 (7), 1173–1180. doi:10.1166/jbn.2013.1629
Beavers, K. R., Nelson, C. E., and Duvall, C. L. (2015). MiRNA inhibition in tissue engineering and regenerative medicine. Adv. Drug Deliv. Rev. 88, 123–137. doi:10.1016/j.addr.2014.12.006
Broussard, K. C., and Powers, J. G. (2013). Wound dressings: Selecting the most appropriate type. Am. J. Clin. Dermatol. 14 (6), 449–459. doi:10.1007/s40257-013-0046-4
Celebioglu, A., Yildiz, Z. I., and Uyar, T. (2018). Fabrication of electrospun eugenol/cyclodextrin inclusion complex nanofibrous webs for enhanced antioxidant property, water solubility, and high temperature stability. J. Agric. Food Chem. 66 (2), 457–466. doi:10.1021/acs.jafc.7b04312
Chan, W. P., Huang, K. C., and Bai, M. Y. (2017). Silk fibroin protein-based nonwoven mats incorporating baicalein Chinese herbal extract: Preparation, characterizations, and in vivo evaluation. J. Biomed. Mat. Res. 105 (2), 420–430. doi:10.1002/jbm.b.33560
Chattopadhyay, S., Guthrie, K. M., Teixeira, L., Murphy, C. J., Dubielzig, R. R., McAnulty, J. F., et al. (2016). Anchoring a cytoactive factor in a wound bed promotes healing. J. Tissue Eng. Regen. Med. 10 (12), 1012–1020. doi:10.1002/term.1886
Chen, H., Huang, M., Liu, Y., Meng, L., and Ma, M. (2020). Functionalized electrospun nanofiber membranes for water treatment: A review. Sci. Total Environ. 739, 139944. doi:10.1016/j.scitotenv.2020.139944
Chen, K., Pan, H., Ji, D., Li, Y., Duan, H., and Pan, W. (2021). Curcumin-loaded sandwich-like nanofibrous membrane prepared by electrospinning technology as wound dressing for accelerate wound healing. Mater. Sci. Eng. C 127 (6), 112245. doi:10.1016/j.msec.2021.112245
Chen, L., Su, Y., Yin, B., Li, S., Cheng, X., He, Y., et al. (2022). LARP6 regulates keloid fibroblast proliferation, invasion, and ability to synthesize collagen. J. Invest. Dermatol. 142, 2395–2405.e7. doi:10.1016/j.jid.2022.01.028
Chen, S., Li, R., Li, X., and Xie, J. (2018). Electrospinning: An enabling nanotechnology platform for drug delivery and regenerative medicine. Adv. Drug Deliv. Rev. 132, 188–213. doi:10.1016/j.addr.2018.05.001
Cheng, L., Sun, X., Hu, C., Jin, R., Sun, B., Shi, Y., et al. (2013). In vivo inhibition of hypertrophic scars by implantable ginsenoside-Rg3-loaded electrospun fibrous membranes. Acta Biomater. 9 (12), 9461–9473. doi:10.1016/j.actbio.2013.07.040
Croitoru, A. M., Karacelebi, Y., Saatcioglu, E., Altan, E., Ulag, S., Aydogan, H. K., et al. (2021). Electrically triggered drug delivery from novel electrospun poly(lactic acid)/graphene oxide/quercetin fibrous scaffolds for wound dressing applications. Pharmaceutics 13 (7), 957. doi:10.3390/pharmaceutics13070957
Cui, S., Yao, B., Sun, X., Hu, J., Zhou, Y., and Liu, Y. (2016). Reducing the content of carrier polymer in pectin nanofibers by electrospinning at low loading followed with selective washing. Mater. Sci. Eng. C 59, 885–893. doi:10.1016/j.msec.2015.10.086
Cui, W., Zhu, X., Yang, Y., Li, X., and Jin, Y. (2009). Evaluation of electrospun fibrous scaffolds of poly(DL-lactide) and poly(ethylene glycol) for skin tissue engineering. Mater. Sci. Eng. C 29 (6), 1869–1876. doi:10.1016/j.msec.2009.02.013
Das, O., Neisiany, R. E., Capezza, A. J., Hedenqvist, M. S., Forsth, M., Xu, Q., et al. (2020). The need for fully bio-based facemasks to counter coronavirus outbreaks: A perspective. Sci. Total Environ. 736, 139611. doi:10.1016/j.scitotenv.2020.139611
DeFrates, K. G., Moore, R., Borgesi, J., Lin, G., Mulderig, T., Beachley, V., et al. (2018). Protein-based fiber materials in medicine: A review. Nanomater. (Basel) 8 (7), 457. doi:10.3390/nano8070457
Deldar, Y., Pilehvar-Soltanahmadi, Y., Dadashpour, M., Montazer Saheb, S., Rahmati-Yamchi, M., and Zarghami, N. (2018). An in vitro examination of the antioxidant, cytoprotective and anti-inflammatory properties of chrysin-loaded nanofibrous mats for potential wound healing applications. Artif. Cells Nanomed. Biotechnol. 46 (4), 706–716. doi:10.1080/21691401.2017.1337022
Dong, W. H., Liu, J. X., Mou, X. J., Liu, G. S., Huang, X. W., Yan, X., et al. (2020). Performance of polyvinyl pyrrolidone-isatis root antibacterial wound dressings produced in situ by handheld electrospinner. Colloids Surfaces B Biointerfaces 188, 110766. doi:10.1016/j.colsurfb.2019.110766
Driskell, R. R., Lichtenberger, B. M., Hoste, E., Kretzschmar, K., Simons, B. D., Charalambous, M., et al. (2013). Distinct fibroblast lineages determine dermal architecture in skin development and repair. Nature 504 (7479), 277–281. doi:10.1038/nature12783
Farahani, M., and Shafiee, A. (2021). Wound healing: From passive to smart dressings. Adv. Healthc. Mat. 10 (16), e2100477. doi:10.1002/adhm.202100477
Finnerty, C. C., Jeschke, M. G., Branski, L. K., Barret, J. P., Dziewulski, P., and Herndon, D. N. (2016). Hypertrophic scarring: The greatest unmet challenge after burn injury. Lancet 388 (10052), 1427–1436. doi:10.1016/s0140-6736(16)31406-4
Fl, A. (2020). Sesamol incorporated cellulose acetate-zein composite nanofiber membrane: An efficient strategy to accelerate diabetic wound healing - ScienceDirect. Int. J. Biol. Macromol. 149, 627–638.
Foster, D. S., Januszyk, M., Yost, K. E., Chinta, M. S., Gulati, G. S., Nguyen, A. T., et al. (2021). Integrated spatial multiomics reveals fibroblast fate during tissue repair. Proc. Natl. Acad. Sci. U. S. A. 118 (41), e2110025118. doi:10.1073/pnas.2110025118
Fraccalvieri, M., Ruka, E., Bocchiotti, M. A., Zingarelli, E., and Bruschi, S. (2011). Patient's pain feedback using negative pressure wound therapy with foam and gauze. Int. Wound J. 8 (5), 492–499. doi:10.1111/j.1742-481x.2011.00821.x
Fuhrmann, T., Smith, N., and Tausk, F. (2010). Use of complementary and alternative medicine among adults with skin disease: Updated results from a national survey. J. Am. Acad. Dermatol. 63 (6), 1000–1005. doi:10.1016/j.jaad.2009.12.009
Gao, Y., Kuok, K. I., Jin, Y., and Wang, R. (2019). Biomedical applications of Aloe vera. Crit. Rev. Food Sci. Nutr. 59 (1), S244–s256. doi:10.1080/10408398.2018.1496320
Guo, H., Tan, S., Gao, J., and Wang, L. (2020). Sequential release of drugs form a dual-delivery system based on pH-responsive nanofibrous mats towards wound care. J. Mat. Chem. B 8 (8), 1759–1770. doi:10.1039/c9tb02522g
Guo, S., and Dipietro, L. A. (2010). Factors affecting wound healing. J. Dent. Res. 89 (3), 219–229. doi:10.1177/0022034509359125
Han, J., Chen, T. X., Branford-White, C. J., and Zhu, L. M. (2009). Electrospun shikonin-loaded PCL/PTMC composite fiber mats with potential biomedical applications. Int. J. Pharm. 382 (1-2), 215–221. doi:10.1016/j.ijpharm.2009.07.027
Hernandez, J. L., and Woodrow, K. A. (2022). Medical applications of porous biomaterials: Features of porosity and tissue-specific implications for biocompatibility. Adv. Healthc. Mat. 11 (9), e2102087. doi:10.1002/adhm.202102087
Hosseinzadeh, S., Soleimani, M., Vossoughi, M., Ranjbarvan, P., Hamedi, S., Zamanlui, S., et al. (2017). Study of epithelial differentiation and protein expression of keratinocyte-mesenchyme stem cell co-cultivation on electrospun nylon/B. vulgaris extract composite scaffold. Mater. Sci. Eng. C 75, 653–662. doi:10.1016/j.msec.2017.02.101
Hussein, M. A. M., Gunduz, O., Sahin, A., Grinholc, M., El-Sherbiny, I. M., and Megahed, M. (2022). Dual spinneret electrospun polyurethane/PVA-gelatin nanofibrous scaffolds containing cinnamon essential oil and nanoceria for chronic diabetic wound healing: Preparation, physicochemical characterization and in-vitro evaluation. Molecules 27 (7), 2146. doi:10.3390/molecules27072146
Ionescu, O. M., Iacob, A. T., Mignon, A., Van Vlierberghe, S., Baican, M., Danu, M., et al. (2021). Design, preparation and in vitro characterization of biomimetic and bioactive chitosan/polyethylene oxide based nanofibers as wound dressings. Int. J. Biol. Macromol. 193, 996–1008. doi:10.1016/j.ijbiomac.2021.10.166
Jia, B., Fan, D., Li, J., Duan, Z., and Fan, L. (2017). Effect of guar gum with sorbitol coating on the properties and oil absorption of French fries. Int. J. Mol. Sci. 18 (12), 2700. doi:10.3390/ijms18122700
Jouybar, A., Seyedjafari, E., Ardeshirylajimi, A., Zandi-Karimi, A., Feizi, N., Khani, M. M., et al. (2017). Enhanced skin regeneration by herbal extract-coated poly-L-lactic acid nanofibrous scaffold. Artif. Organs 41 (11), E296–e307. doi:10.1111/aor.12926
Kalashnikova, I., Das, S., and Seal, S. (2015). Nanomaterials for wound healing: Scope and advancement. Nanomedicine (Lond) 10 (16), 2593–2612. doi:10.2217/nnm.15.82
Kang, H., Zhang, K., Wong, D. S. H., Han, F., Li, B., and Bian, L. (2018). Near-infrared light-controlled regulation of intracellular calcium to modulate macrophage polarization. Biomaterials 178, 681–696. doi:10.1016/j.biomaterials.2018.03.007
Kang, M. C., Kim, S. Y., Kim, Y. T., Kim, E. A., Lee, S. H., Ko, S. C., et al. (2014). In vitro and in vivo antioxidant activities of polysaccharide purified from aloe vera (Aloe barbadensis) gel. Carbohydr. Polym. 99, 365–371. doi:10.1016/j.carbpol.2013.07.091
Kharat, Z., Amiri Goushki, M., Sarvian, N., Asad, S., Dehghan, M. M., and Kabiri, M. (2021). Chitosan/PEO nanofibers containing Calendula officinalis extract: Preparation, characterization, in vitro and in vivo evaluation for wound healing applications. Int. J. Pharm. X. 609, 121132. doi:10.1016/j.ijpharm.2021.121132
Kim, H., Wang, S. Y., Kwak, G., Yang, Y., Kwon, I. C., and Kim, S. H. (2019). Exosome-guided phenotypic switch of M1 to M2 macrophages for cutaneous wound healing. Adv. Sci. (Weinh). 6 (20), 1900513. doi:10.1002/advs.201900513
Kumar, A., Wei, M., Barry, C., Chen, J., and Mead, J. (2010). Controlling fiber repulsion in multijet electrospinning for higher throughput. Macromol. Mat. Eng. 295 (8), 701–708. doi:10.1002/mame.200900425
Li, A., Li, L., Zhao, B., Li, X., Liang, W., Lang, M., et al. (2022). Antibacterial, antioxidant and anti-inflammatory PLCL/gelatin nanofiber membranes to promote wound healing. Int. J. Biol. Macromol. 194, 914–923. doi:10.1016/j.ijbiomac.2021.11.146
Lin, S., Chen, M., Jiang, H., Fan, L., Sun, B., Yu, F., et al. (2016). Green electrospun grape seed extract-loaded silk fibroin nanofibrous mats with excellent cytocompatibility and antioxidant effect. Colloids Surfaces B Biointerfaces 139, 156–163. doi:10.1016/j.colsurfb.2015.12.001
Lubambo, A. F., Ono, L., Drago, V., Mattoso, N., Varalda, J., Sierakowski, M. R., et al. (2015). Tuning Fe3O4 nanoparticle dispersion through pH in PVA/guar gum/electrospun membranes. Carbohydr. Polym. 134, 775–783. doi:10.1016/j.carbpol.2015.08.013
Luo, H., Yin, X. Q., Tan, P. F., Gu, Z. P., Liu, Z. M., and Tan, L. (2021). Polymeric antibacterial materials: Design, platforms and applications. J. Mat. Chem. B 9 (12), 2802–2815. doi:10.1039/d1tb00109d
Ma, C. H., Chou, W. C., Wu, C. H., Jou, I. M., Tu, Y. K., Hsieh, P. L., et al. (2021). Ginsenoside Rg3 attenuates TNF-α-induced damage in chondrocytes through regulating SIRT1-mediated anti-apoptotic and anti-inflammatory mechanisms. Antioxidants (Basel) 10 (12), 1972. doi:10.3390/antiox10121972
Martin, P., and Nunan, R. (2015). Cellular and molecular mechanisms of repair in acute and chronic wound healing. Br. J. Dermatol. 173 (2), 370–378. doi:10.1111/bjd.13954
Miguel, S. P., Simoes, D., Moreira, A. F., Sequeira, R. S., and Correia, I. J. (2019). Production and characterization of electrospun silk fibroin based asymmetric membranes for wound dressing applications. Int. J. Biol. Macromol. 121, 524–535. doi:10.1016/j.ijbiomac.2018.10.041
Min, T., Zhou, L., Sun, X., Du, H., Zhu, Z., and Wen, Y. (2022). Electrospun functional polymeric nanofibers for active food packaging: A review. Food Chem. x. 391, 133239. doi:10.1016/j.foodchem.2022.133239
Morganti, P., Fusco, A., Paoletti, I., Perfetto, B., Del Ciotto, P., Palombo, M., et al. (2017). Anti-inflammatory, immunomodulatory, and tissue repair activity on human keratinocytes by green innovative nanocomposites. Mater. (Basel) 10 (7), 843. doi:10.3390/ma10070843
Mostafalu, P., Rahimi, R., Ochoa, M., Khalilpour, A., Kiaee, G., and . Yazdi, I. K. (2018). Smart bandage for monitoring and treatment of chronic wounds. Small 14 (33), e1703509. doi:10.1002/smll.201703509
Mouro, C., Dunne, C. P., and Gouveia, I. C. (2020). Designing new antibacterial wound dressings: Development of a dual layer cotton material coated with poly(vinyl Alcohol)_Chitosan nanofibers incorporating agrimonia eupatoria L. Extract. Molecules 26 (1), 83. doi:10.3390/molecules26010083
Nakhjavani, M., Smith, E., Yeo, K., Palethorpe, H. M., Tomita, Y., Price, T. J., et al. (2021). Anti-angiogenic properties of ginsenoside Rg3 epimers: In vitro assessment of single and combination treatments. Cancers (Basel) 13 (9), 2223. doi:10.3390/cancers13092223
Nazeam, J. A., Gad, H. A., El-Hefnawy, H. M., and Singab, A. N. B. (2017). Chromatographic separation and detection methods of aloe arborescens miller constituents: A systematic review. J. Chromatogr. B 1058, 57–67. doi:10.1016/j.jchromb.2017.04.044
Nisar, M. F., Khadim, M., Rafiq, M., Chen, J., Yang, Y., and Wan, C. C. (2021). Pharmacological properties and health benefits of eugenol: A comprehensive review. Oxid. Med. Cell. Longev. 2021, 1–14. doi:10.1155/2021/2497354
Padil, V. V., Senan, C., Wacſawek, S., and Lernik, M. (2016). Electrospun fibers based on Arabic, karaya and kondagogu gums. Int. J. Biol. Macromol. 91, 299–309. doi:10.1016/j.ijbiomac.2016.05.064
Pedram Rad, Z., Mokhtari, J., and Abbasi, M. (2019). Calendula officinalis extract/PCL/Zein/Gum Arabic nanofibrous bio-composite scaffolds via suspension, two-nozzle and multilayer electrospinning for skin tissue engineering. Int. J. Biol. Macromol. 135, 530–543. doi:10.1016/j.ijbiomac.2019.05.204
Pedram Rad, Z., Mokhtari, J., and Abbasi, M. (2018). Fabrication and characterization of PCL/zein/gum Arabic electrospun nanocomposite scaffold for skin tissue engineering. Mater. Sci. Eng. C 93, 356–366. doi:10.1016/j.msec.2018.08.010
Peng, Y., Ma, Y., Bao, Y., Liu, Z., Chen, L., Dai, F., et al. (2021). Electrospun PLGA/SF/artemisinin composite nanofibrous membranes for wound dressing. Int. J. Biol. Macromol. 183, 68–78. doi:10.1016/j.ijbiomac.2021.04.021
Ramalingam, R., Dhand, C., Mayandi, V., Leung, C. M., Ezhilarasu, H., Karuppannan, S. K., et al. (2021). Core-shell structured antimicrobial nanofiber dressings containing herbal extract and antibiotics combination for the prevention of biofilms and promotion of cutaneous wound healing. ACS Appl. Mat. Interfaces 13 (21), 24356–24369. doi:10.1021/acsami.0c20642
Reuter, J., Merfort, I., and Schempp, C. M. (2010). Botanicals in dermatology: An evidence-based review. Am. J. Clin. Dermatol. 11 (4), 247–267. doi:10.2165/11533220-000000000-00000
Richbourg, N. R., Peppas, N. A., and Sikavitsas, V. I. (2019). Tuning the biomimetic behavior of scaffolds for regenerative medicine through surface modifications. J. Tissue Eng. Regen. Med. 13 (8), 1275–1293. doi:10.1002/term.2859
Rieger, K. A., Birch, N. P., and Schiffman, J. D. (2013). Designing electrospun nanofiber mats to promote wound healing - a review. J. Mat. Chem. B 1 (36), 4531–4541. doi:10.1039/c3tb20795a
Roy, S., Khanna, S., Nallu, K., Hunt, T. K., and Sen, C. K. (2006). Dermal wound healing is subject to redox control. Mol. Ther. 13 (1), 211–220. doi:10.1016/j.ymthe.2005.07.684
Sánchez, M., Gonzalez-Burgos, E., Iglesias, I., and Gomez-Serranillos, M. P. (2020). Pharmacological update properties of aloe vera and its major active constituents. Molecules 25 (6), 1324. doi:10.3390/molecules25061324
Selvaraj, S., and Fathima, N. N. (2017). Fenugreek incorporated silk fibroin nanofibers-A potential antioxidant scaffold for enhanced wound healing. ACS Appl. Mat. Interfaces 9 (7), 5916–5926. doi:10.1021/acsami.6b16306
Sen, S., Bal, T., and Rajora, A. D. (2022). Green nanofiber mat from HLM-PVA-Pectin (Hibiscus leaves mucilage-polyvinyl alcohol-pectin) polymeric blend using electrospinning technique as a novel material in wound-healing process. Appl. Nanosci. 12, 237–250. doi:10.1007/s13204-021-02295-4
Seo, Y. J., Kwon, M. S., Choi, H. W., Jang, J. E., Lee, J. K., Sun, Y., et al. (2008). Intracerebroventricular gisenosides are antinociceptive in proinflammatory cytokine-induced pain behaviors of mice. Arch. Pharm. Res. 31 (3), 364–369. doi:10.1007/s12272-001-1165-x
Sheikholeslam, M., Wright, M. E. E., Jeschke, M. G., and Amini-Nik, S. (2018). Biomaterials for skin substitutes. Adv. Healthc. Mat. 7 (5), 1700897. doi:10.1002/adhm.201700897
Shokrollahi, M., Bahrami, S. H., Nazarpak, M. H., and Solouk, A. (2020). Multilayer nanofibrous patch comprising chamomile loaded carboxyethyl chitosan/poly(vinyl alcohol) and polycaprolactone as a potential wound dressing. Int. J. Biol. Macromol. 147, 547–559. doi:10.1016/j.ijbiomac.2020.01.067
Soares, R. M. D., Siqueira, N. M., Prabhakaram, M. P., and Ramakrishna, S. (2018). Electrospinning and electrospray of bio-based and natural polymers for biomaterials development. Mater. Sci. Eng. C 92, 969–982. doi:10.1016/j.msec.2018.08.004
Sood, A., Granick, M. S., and Tomaselli, N. L. (2014). Wound dressings and comparative effectiveness data. Adv. Wound Care (New. Rochelle. 3 (8), 511–529. doi:10.1089/wound.2012.0401
Sun, W., Gregory, D. A., Tomeh, M. A., and Zhao, X. (2021). Silk fibroin as a functional biomaterial for tissue engineering. Int. J. Mol. Sci. 22 (3), 1499. doi:10.3390/ijms22031499
Sun, Y., Cheng, S., Lu, W., Wang, Y., Zhang, P., and Yao, Q. (2019). Electrospun fibers and their application in drug controlled release, biological dressings, tissue repair, and enzyme immobilization. RSC Adv. 9 (44), 25712–25729. doi:10.1039/c9ra05012d
Sundaramurthi, D., Krishnan, U. M., and Sethuraman, S. (2014). Electrospun nanofibers as scaffolds for skin tissue engineering. Polym. Rev. 54 (2), 348–376. doi:10.1080/15583724.2014.881374
Tang, M., Bian, W., Cheng, L., Zhang, L., Jin, R., Wang, W., et al. (2018). Ginsenoside Rg3 inhibits keloid fibroblast proliferation, angiogenesis and collagen synthesis in vitro via the TGF-β/Smad and ERK signaling pathways. Int. J. Mol. Med. 41 (3), 1487–1499. doi:10.3892/ijmm.2018.3362
Vakilian, S., Norouzi, M., Soufi-Zomorrod, M., Shabani, I., Hosseinzadeh, S., and Soleimani, M. (2018). L. inermis-loaded nanofibrous scaffolds for wound dressing applications. Tissue Cell 51, 32–38. doi:10.1016/j.tice.2018.02.004
Vass, P., Szabo, E., Domokos, A., Hirsch, E., Galata, D., Farkas, B., et al. (2020). Scale-up of electrospinning technology: Applications in the pharmaceutical industry. Wiley Interdiscip. Rev. Nanomed. Nanobiotechnol. 12 (4), e1611. doi:10.1002/wnan.1611
Vorstandlechner, V., Laggner, M., Copic, D., Klas, K., Direder, M., Chen, Y., et al. (2021). The serine proteases dipeptidyl-peptidase 4 and urokinase are key molecules in human and mouse scar formation. Nat. Commun. 12 (1), 6242. doi:10.1038/s41467-021-26495-2
Wang, W., Meng, Q., Li, Q., Liu, J., Zhou, M., Jin, Z., et al. (2020). Chitosan derivatives and their application in biomedicine. Int. J. Mol. Sci. 21 (2), 487. doi:10.3390/ijms21020487
Wang, Z. C., Zhao, W. Y., Cao, Y., Liu, Y. Q., Sun, Q., Shi, P., et al. (2020). The roles of inflammation in keloid and hypertrophic scars. Front. Immunol. 11, 603187. doi:10.3389/fimmu.2020.603187
Wilgus, T. A. (2019). Vascular endothelial growth factor and cutaneous scarring. Adv. Wound Care (New. Rochelle. 8 (12), 671–678. doi:10.1089/wound.2018.0796
Wilkinson, H. N., and Hardman, M. J. (2020). Wound healing: Cellular mechanisms and pathological outcomes. Open Biol. 10 (9), 200223. doi:10.1098/rsob.200223
Wu, S., Dong, T., Li, Y., Sun, M., Qi, Y., Liu, J., et al. (2022). State-of-the-art review of advanced electrospun nanofiber yarn-based textiles for biomedical applications. Appl. Mat. Today 27, 101473. doi:10.1016/j.apmt.2022.101473
Wu, Y. K., Wang, L., Fan, J., Shou, W., Zhou, B. M., and Liu, Y. (2018). Multi-jet electrospinning with auxiliary electrode: The influence of solution properties. Polym. (Basel) 10 (6), 572. doi:10.3390/polym10060572
Yan, X., Yu, M., Ramakrishna, S., Russell, S. J., and Long, Y. Z. (2019). Advances in portable electrospinning devices for in situ delivery of personalized wound care. Nanoscale 11 (41), 19166–19178. doi:10.1039/c9nr02802a
Yang, X., Chen, M., Li, P., Ji, Z., Wang, M., Feng, Y., et al. (2021). Fabricating poly(vinyl alcohol)/gelatin composite sponges with high absorbency and water-triggered expansion for noncompressible hemorrhage and wound healing. J. Mat. Chem. B 9 (6), 1568–1582. doi:10.1039/d0tb02480e
Yang, Y., and Leong, K. W. (2010). Nanoscale surfacing for regenerative medicine. WIREs Nanomed. Nanobiotechnol. 2 (5), 478–495. doi:10.1002/wnan.74
Yang, Y., Wang, F., Yin, D., Fang, Z., and Huang, L. (2015). Astragulus polysaccharide-loaded fibrous mats promote the restoration of microcirculation in/around skin wounds to accelerate wound healing in a diabetic rat model. Colloids Surfaces B Biointerfaces 136, 111–118. doi:10.1016/j.colsurfb.2015.09.006
Yang, Y., Xu, L., Wang, J., Meng, Q., Zhong, S., Gao, Y., et al. (2022). Recent advances in polysaccharide-based self-healing hydrogels for biomedical applications. Carbohydr. Polym. 283, 119161. doi:10.1016/j.carbpol.2022.119161
Yao, C. H., Yeh, J. Y., Chen, Y. S., Li, M. H., and Huang, C. H. (2017). Wound-healing effect of electrospun gelatin nanofibres containing Centella asiatica extract in a rat model. J. Tissue Eng. Regen. Med. 11 (3), 905–915. doi:10.1002/term.1992
Ye, K., Morsi, Y., and Mo, (2019). Electrospun nanofibers for tissue engineering with drug loading and release. Pharmaceutics 11 (4), 182. doi:10.3390/pharmaceutics11040182
Ye, P., Wei, S., Luo, C., Wang, Q., Li, A., and Wei, F. (2020). Long-term effect against methicillin-resistant Staphylococcus aureus of emodin released from coaxial electrospinning nanofiber membranes with a biphasic profile. Biomolecules 10 (3), 362. doi:10.3390/biom10030362
Zamboni, F., Wong, C. K., and Collins, M. N. (2023). Hyaluronic acid association with bacterial, fungal and viral infections: Can hyaluronic acid be used as an antimicrobial polymer for biomedical and pharmaceutical applications? Bioact. Mat. 19, 458–473. doi:10.1016/j.bioactmat.2022.04.023
Zhang, D., Zhou, X., Liu, L., Guo, M., Huang, T., Zhou, W., et al. (2021). Glucomannan from aloe vera gel promotes intestinal stem cell-mediated epithelial regeneration via the wnt/β-catenin pathway. J. Agric. Food Chem. 69 (36), 10581–10591. doi:10.1021/acs.jafc.1c03814
Zhang, H., Zhang, M., Wang, X., Zhang, M., Wang, X., Li, Y., et al. (2022). Electrospun multifunctional nanofibrous mats loaded with bioactive anemoside B4 for accelerated wound healing in diabetic mice. Drug Deliv. (Lond). 29 (1), 174–185. doi:10.1080/10717544.2021.2021319
Zhang, J., Chen, K., Ding, C., Sun, S., Zheng, Y., Ding, Q., et al. (2022). Fabrication of chitosan/PVP/dihydroquercetin nanocomposite film for in vitro and in vivo evaluation of wound healing. Int. J. Biol. Macromol. 206, 591–604. doi:10.1016/j.ijbiomac.2022.02.110
Keywords: botanical, electrospinning, wound healing, wound dressing, nanofiber
Citation: Guo S, Wang P, Song P and Li N (2022) Electrospinning of botanicals for skin wound healing. Front. Bioeng. Biotechnol. 10:1006129. doi: 10.3389/fbioe.2022.1006129
Received: 29 July 2022; Accepted: 22 August 2022;
Published: 19 September 2022.
Edited by:
Qun Wang, Iowa State University, United StatesReviewed by:
Ehsan Nazarzadeh Zare, Damghan University, IranChuanxu Yang, Shandong University, China
Zhongyang Zhang, Technical University of Denmark, Denmark
Copyright © 2022 Guo, Wang, Song and Li. This is an open-access article distributed under the terms of the Creative Commons Attribution License (CC BY). The use, distribution or reproduction in other forums is permitted, provided the original author(s) and the copyright owner(s) are credited and that the original publication in this journal is cited, in accordance with accepted academic practice. No use, distribution or reproduction is permitted which does not comply with these terms.
*Correspondence: Ning Li, lili.li.ning@gmail.com; Ping Song, songping_cacms@163.com
†These authors have contributed equally to this work