Digital Workflow for the Design, Manufacture, and Application of Custom-Made Short Implants With Wing Retention Device
- 1Department of Oral and Maxillofacial Surgery, The Affiliated Hospital of Qingdao University, Qingdao, China
- 2School of Stomatology of Qingdao University, Qingdao, China
- 3Dental Digital Medicine and 3D Printing Engineering Laboratory of Qingdao, Qingdao, China
- 4Department of Prosthodontics, Peking University School and Hospital of Stomatology, Beijing, China
- 5National Clinical Research Center for Oral Diseases, Beijing, China
- 6National Engineering Laboratory for Digital and Material Technology of Stomatology, Beijing, China
- 7Beijing Key Laboratory of Digital Stomatology, Beijing, China
The severe deficiency of vertical bone height in the posterior maxillary region poses a challenge to implant restoration. In response to this issue, this article introduces a custom-made short implant with a wing retention structure and describes a precise and minimally invasive dental implant restoration scheme with digital technology.
Introduction
Dental implant restoration performed in the loss of posterior maxillary teeth can be a challenge owing to the insufficient vertical bone volume resulting from the resorption of an alveolar bone and the pneumatization of a maxillary sinus (Mu et al., 2020). It has been found that the residual bone height (RBH) of some patients is only 1–2 mm, posing difficulties in stabilizing the implant initially (Pignaton et al., 2019). When RBH is 4 mm or less, the lateral sinus floor elevation with delayed implant placement is suggested in clinical use. However, it usually requires bone grafting, and prolongs the recovery time of patients with missing teeth. Furthermore, lateral antrostomy surgery has a high risk and may damage the branches of the posterior alveolar artery. In addition, the surgery process may cause tearing and perforation of the medial wall mucosa, which in turn affects osteogenesis (Gonzalez et al., 2014; Rammelsberg et al., 2020). Considering this fact, Bedrossian et al. reported a zygomatic implant with a length of 30–52.5 mm placed through a sinus cavity and fixed in a zygomatic bone to guarantee the initial stabilization (Bedrossian et al., 2002). However, this technique is complex and invasive, often associated with serious complications like infection, bleeding, and nerve injury (Agliardi et al., 2021).
With the digitization of modern medicine, some scholars are proposing the application of subperiosteal implants for patients with severe bone insufficiency in the posterior maxillary region (Gellrich et al., 2017a; Jehn et al., 2020). Gellrich et al. designed an improved subperiosteal implant (Individual Patient Solution-dental, IPS-d), equipped with dozens of screw holes. The IPS-d was made of type III titanium (Ti6Al4V) as material and printed by selective laser melting technology, and fixed by miniature screws to complete the denture restoration in the missing area. These have promoted the idea and development of an innovative skeletal anchorage system for prosthodontic rehabilitation. However, in order to ensure the stability of the implant, the titanium plate is designed with a large spread area. In addition, the “pile” structure is integrated with the titanium plate, without a platform transfer structure, so it is difficult to obtain a good biological seal of gingival soft tissue. The clinical application of zygomatic implant and improved subperiosteal implant in the restoration of missing teeth with severe bone insufficiency is also limited by the disadvantages of large surgical trauma, many postoperative complications, and unguaranteed long-term restoration effect. Till now, treating patients with the RBH seriously deficient (RBH<3 mm) in the posterior maxillary area remains difficult.
Therefore, a custom-made short implant with a wing retention structure has been developed with digital technology. This new implant is expected to provide long-term stability through the retention of a wing device, with no need for maxillary sinus lateral antrostomy. Moreover, the surgery process is relatively safe, and can reduce the trauma, pain, and complications of patients.
Methods
The following method was used to design, fabricate, and implant a new implant.
1) Make a preoperative cone-beam computed tomography (CBCT, KaVo, Germany) scan of a patient to obtain high-resolution digital imaging and communications in medicine (DICOM) data for diagnosis and treatment planning (Figure 1). The scanning conditions of CBCT are as follows: the tube voltage is 120 kV, the tube current is 5 mA, the exposure time is 27 s, and the scanning layer thickness is 250 μm. Patients with a residual bone height (RBH<3 mm) of less than 3 mm in the posterior maxillary region were selected.
2) Use a model scanner (D2000; 3Shape) to scan the maxillary and mandibular plaster casts, and export the model data in a standard tessellation language (STL) file format (Figures 2A,B).
3) Register CBCT data with model scanning data to obtain a virtual 3D bone reconstruction model of the patient. Design the position and shape of the custom-made implant by 3Shape dental system (3Shape, Denmark) according to the model (Figure 3A). Design the body part of the implant in the shape of a conical structure that can be inserted into the implant hole. Make sure that the lower surface of the wing retention structure closely fits the outer surface of the alveolar bone (Figure 3B). Design the upper part of the short implant as an implant platform shaped as a frustum of a cone with a slope of 45° and height of 1 mm (Figure 3C). Use an inverted round table hole with a morse taper, an inner hexagonal hole, and a threaded hole to fix the abutment (Figure 3D). Design screw hole locations to avoid damaging important anatomical structures and healthy adjacent teeth.
4) Transfer the virtual plan to a manufacturer and fabricate the custom-made implant via a seven-axis lathe (480MT; Willemin) (Figure 4A). Design and manufacture a resin surgical template to facilitate implant placement. Test the accuracy and fitness of the custom-made implant on a 3D bone reconstruction resin model (Figure 4B).
5) Carry out periodontal scaling and oral hygiene education for patients before the surgery. Disinfect the oral cavity with iodophor and achieve local infiltration anesthesia with articaine. Make a buccal vertical incision (>5 mm) at the middle of the adjacent tooth, beneath the mucogingival junction. Then make a crestal incision to disclose the alveolar crest and elevate a full-thickness mucoperiosteal flap on the buccal side (Figures 5A,B) (Liu et al., 2021). Prepare an alveolar socket with the implant guide template (Figures 5C,D), and then place the custom-made implant with the healing abutment by hammering the concave osteotome (Dentium, South Korea) (Figures 5E,F). Fix the wings of the retention structure with several self-tapping miniature screws (Shuangyang, China) in the correct position (Figure 5G). Close the flaps with interrupted by using 3-0 Mersilk sutures. (Figure 5H).
6) Remove the healing abutments 6 months after the surgery (Figures 6A,B), and then do the final restoration (Figures 6C,D). Make an impression with polyether silicone rubber impression material and use a zirconia ceramic crown for restoration. Restoration is screw-retained rather than cemented connection of the abutment to the implant body (Liu et al., 2019).
7) Make 6-month and 12-month postoperative CBCT scans to assess the custom-made implant (Figures 7A,B) and conduct a follow-up.
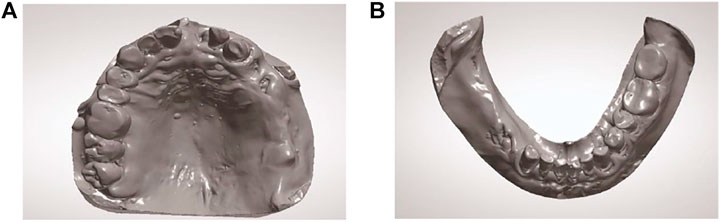
FIGURE 2. Intraoral scans of the patient’s arches. (A) Maxillary STL occlusal view. (B) Mandibular STL occlusal view.
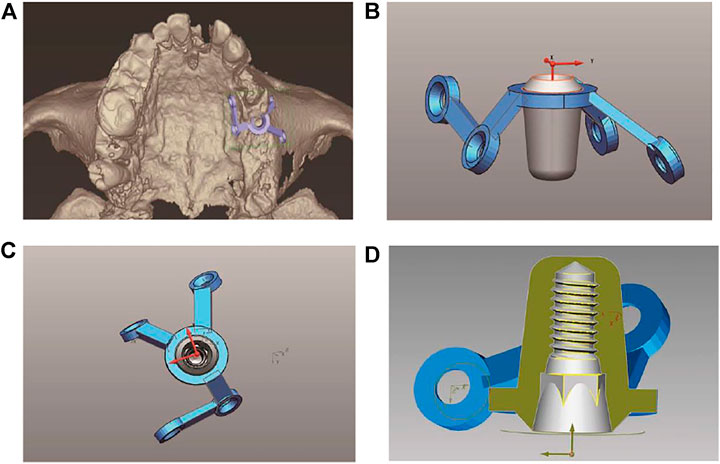
FIGURE 3. Presurgical design. (A) The virtual model of the custom-made implant and its position in the 3D modeling of the alveolar bone. (B) The side view of the virtual model of the custom-made implant. (C) The top view of the virtual model of the custom-made implant. (D) The internal view of the virtual model of the custom-made implant.
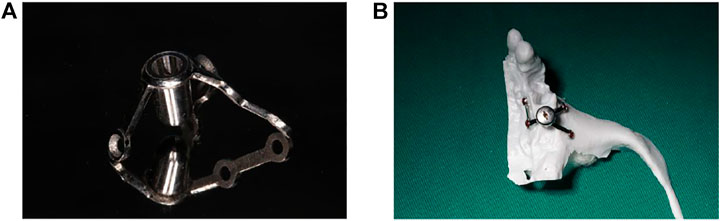
FIGURE 4. The custom-made implant and 3D printed model of the bone. (A) The custom-made implant. (B) The custom-made implant on a 3D bone reconstruction resin model.
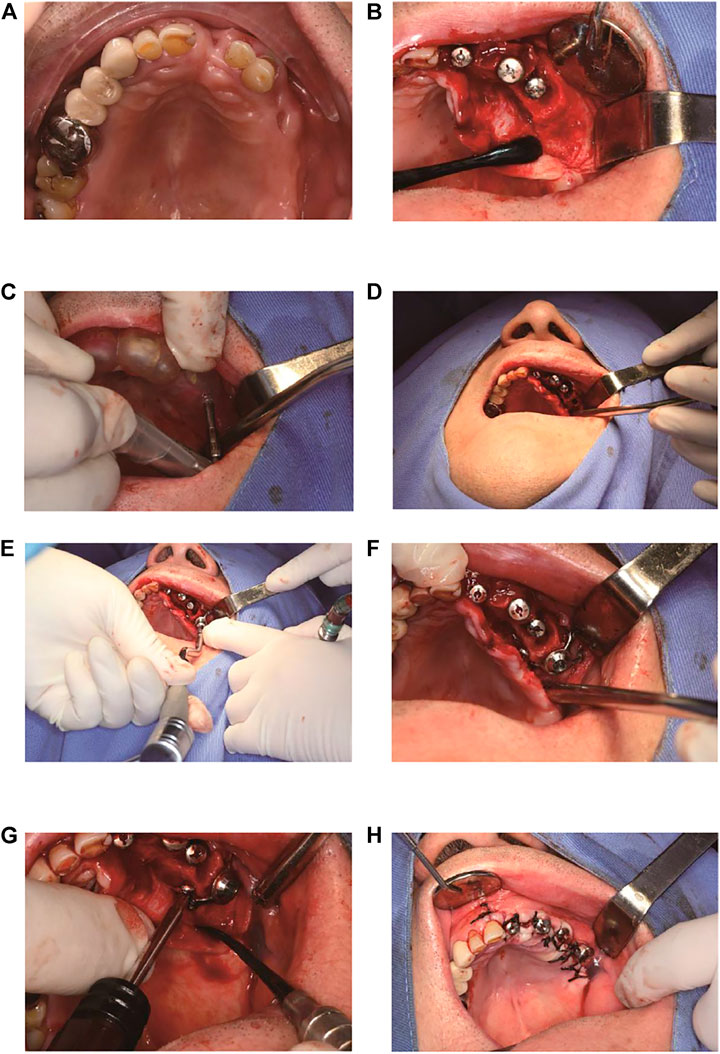
FIGURE 5. The brief surgical procedure. (A) Preoperative intraoral view. (B) Anatomy of the residual bone. (C) Location of the implant by implant guide template. (D) The confirmation of implant socket direction and depth. (E) Placement of the implant. (F) The implant is in position. (G) Fixation with several mini-screws. (H) Sutures.
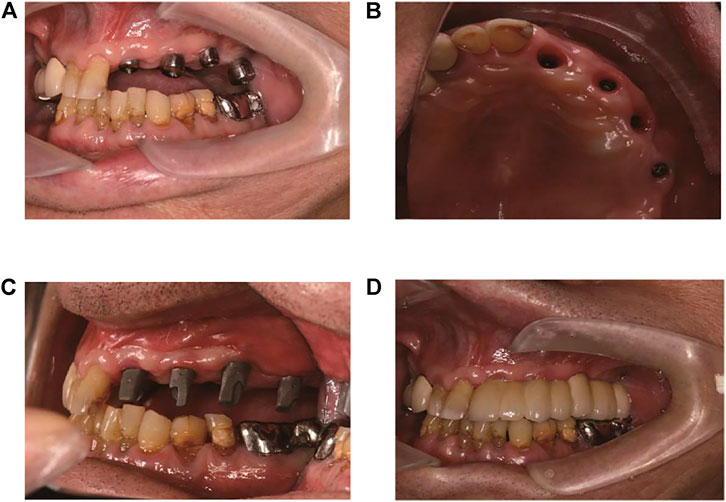
FIGURE 6. The prosthodontics. (A) 6-month postoperative view. (B) Soft tissue cuffs appearance. (C) Custom-made abutments in place. (D) Application of the final prosthetic denture.
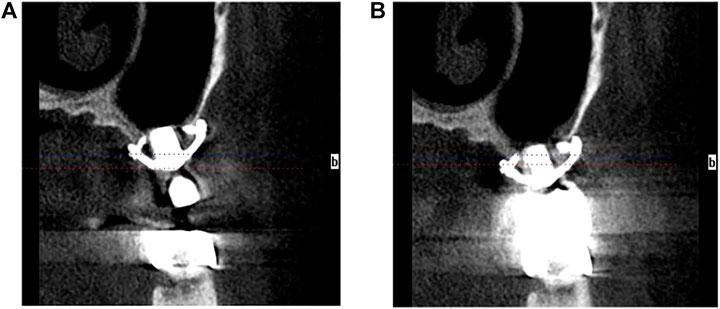
FIGURE 7. The CBCT images. (A) 6-month postoperative CBCT image. (B) 12-month postoperative CBCT image.
Results and Discussion
The patients with severe bone deficiency in the posterior maxillary region successfully completed the placement of custom-made short implants according to this method. In this study, a new custom-made implant is proposed, which consists of two parts: a wing retention structure and a short implant. The wing retention is similar to the subperiosteal retention and is fixed by tiny screws, which is the main fixation of the custom-made implant. However, it is different from a conventional subperiosteal implant regarding the design process. A conventional subperiosteal implant is fixed with titanium plates. In order to ensure stability, the titanium plates need to cover a large area of the bone surface, and a large flap area is required during surgery, which easily affects the blood supply to soft tissue and causes ischemic necrosis in the mucosal area, leading to the failure of implantation. At the same time, the “post” on the upper part of the titanium plates is used to support the denture for restoration. As there is no platform transfer structure, the post structure makes it impossible to attach the gingival soft tissue to the implant to form a biological seal (Mangano et al., 2020). The defective design makes the prepared subperiosteal implant less resistant to bacterial infection, thus affecting the long-term therapeutic effect. On the contrary, this custom-made implant can be implanted at a single tooth missing position. The coverage area of the wing retention is controllable and only needs to be flipped routinely. The wing retention design follows the principles of the triangular retainer and multi-direction placement to ensure mutual antagonism of mechanics. In addition,, the wings are retained by miniature screws to ensure the initial and long-term stability of the implant. At the same time, the short implant structure is preserved, and its length is about 5 mm. The short implant is submerged downward and screw-retained at the bone level. The platform transfer structure is designed to ensure that good soft tissue closure can be formed around the implant after implantation, which is conducive to the long-term stability of the implant. The custom-made implant is neither a subperiosteal implant nor a traditional short one. It retains the short implant structure and adopts an ultra-new concept of wing plate retention. It is a good implant, especially for patients with severe bone insufficiency.
With the development of computer-aided design and computer-aided manufacturing (CAD/CAM), new treatments have been introduced into dentistry (Taha et al., 2021). By analyzing and exporting CBCT data, a 3D resin model of bone reconstruction and a piece of implant guide plate that could accurately locate the implantation position were printed. After that, with the help of CAD/CAM techniques, this study digitally cut a custom-made implant with high mechanical strength with an advanced seven-axis lathe. In this way, the custom-made implant has good biomechanics due to its integrated structure. Previous FEM-stress-test studies can confirm this view (Gellrich et al., 2017b). Moreover, the virtual design of the implant could be precisely transferred into the mouth, ensuring that the lower surface of the wing retention could be closely attached to the outer surface of the alveolar bone. This workflow realizes the digitalization of the implant scheme from preoperative design to precise intraoperative guidance, and finally to postoperative repair.
Compared with traditional implants, the custom-made implants are implanted without bone grafting, thus improving the implant precision, reducing the intraoperative time, and making the operation easier. Despite the underlying problem that it takes about 1 day to design and manufacture the implant, the patients who cannot undergo regular implants due to severe bone insufficiency have supported this technique and taken the implant restoration treatment up to date. Hence, the custom-made implant is more economical, practical, and safer, which is a different modality of treatment from maxillary sinus floor elevation, zygomatic implants, or subperiosteal implants.
Conclusion
This article introduces a new invention of a short implant with a wing retention structure that can be fixed to both subperiosteum and alveolar bones, and describes a precise, minimally invasive, and effective dental implant restoration scheme custom-designed for patients with severe vertical bone height insufficiency in the posterior maxillary region.
Data Availability Statement
The original contributions presented in the study are included in the article/Supplementary Material, further inquiries can be directed to the corresponding author.
Ethics Statement
The studies involving human participants were reviewed and approved by the ethical committee of the affiliated hospital of Qingdao University. The patients/participants provided their written informed consent to participate in this study.
Author Contributions
ZX contributed to the investigation, data curation, visualization, and writing—original draft. ZY helped with visualization, software, and writing—original draft. JY involved in conceptualization, methodology, and resources.
Funding
This work was supported by the Qingdao Science and Technology Project for People (No. 21-1-4-rkjk-20-nsh), the “Clinical Medicine + X” Scientific Research Project of the Medical Department of Qingdao University (No. 2017M41), and the Qingdao Municipal Medical and Health Key Discipline Construction Project.
Conflict of Interest
The authors declare that the research was conducted in the absence of any commercial or financial relationships that could be construed as a potential conflict of interest.
Publisher’s Note
All claims expressed in this article are solely those of the authors and do not necessarily represent those of their affiliated organizations, or those of the publisher, the editors, and the reviewers. Any product that may be evaluated in this article, or claim that may be made by its manufacturer, is not guaranteed or endorsed by the publisher.
Acknowledgments
This study was prepared in compliance with the World Medical Association Declaration of Helsinki guidelines and was approved by the ethical committee of the affiliated hospital of Qingdao University (No. 95311920). The authors thank Beijing Datsing Technology Company and WEGO Medical Technology Company for the support in fabricating the implants. The authors would like to thank the Qingdao Wilhelm Denture Technology Company.
Supplementary Material
The Supplementary Material for this article can be found online at: https://www.frontiersin.org/articles/10.3389/fbioe.2022.885746/full#supplementary-material
References
Agliardi, E. L., Panigatti, S., Romeo, D., Sacchi, L., and Gherlone, E. (2021). Clinical Outcomes and Biological and Mechanical Complications of Immediate Fixed Prostheses Supported by Zygomatic Implants: A Retrospective Analysis from a Prospective Clinical Study with up to 11 Years of Follow‐up. Clin. Implant Dent. Relat. Res. 23, 612–624. doi:10.1111/cid.13017
Bedrossian, E., Stumpel, L., Beckely, M. L., Indresano, T., and Indersano, T. (2002). The Zygomatic Implant: Preliminary Data on Treatment of Severely Resorbed Maxillae. A Clinical Report. Int. J. Oral Maxillofac. Implants 17, 861–865. doi:10.1007/s00384-002-0417-4
Gellrich, N.-C., Rahlf, B., Zimmerer, R., Pott, P.-C., and Rana, M. (2017a). A New Concept for Implant-Borne Dental Rehabilitation; How to Overcome the Biological Weak-Spot of Conventional Dental Implants? Head. Face Med. 13, 1–5. doi:10.1186/s13005-017-0151-3
Gellrich, N.-C., Zimmerer, R. M., Spalthoff, S., Jehn, P., Pott, P.-C., Rana, M., et al. (2017b). A Customised Digitally Engineered Solution for Fixed Dental Rehabilitation in Severe Bone Deficiency: A New Innovative Line Extension in Implant Dentistry. J. Cranio-Maxillofacial Surg. 45, 1632–1638. doi:10.1016/j.jcms.2017.07.022
Gonzalez, S., Tuan, M.-C., Ahn, K. M., and Nowzari, H. (2014). Crestal Approach for Maxillary Sinus Augmentation in Patients with ≤4 Mm of Residual Alveolar Bone. Clin. Implant Dent. Relat. Res. 16, 827–835. doi:10.1111/cid.12067
Jehn, P., Spalthoff, S., Korn, P., Stoetzer, M., Gercken, M., Gellrich, N.-C., et al. (2020). Oral Health-Related Quality of Life in Tumour Patients Treated with Patient-Specific Dental Implants. Int. J. Oral Maxillofac. Surg. 49, 1067–1072. doi:10.1016/j.ijom.2020.01.011
Liu, H., Liu, R., Wang, M., and Yang, J. (2019). Immediate Implant Placement Combined with Maxillary Sinus Floor Elevation Utilizing the Transalveolar Approach and Nonsubmerged Healing for Failing Teeth in the Maxillary Molar Area: A Randomized Controlled Trial Clinical Study with One‐year Follow‐up. Clin. Implant Dent. Relat. Res. 21, 462–472. doi:10.1111/cid.12783
Liu, L., Yuhui, W., Li, X.-t., Zhaojun, H., and Yang, J.-j. (2021). Clinical Application Study of Immediate Implantation without Bone Grafting in Maxillary Molars: a Clinical Study with One-Year Follow up. Br. J. Oral Maxillofac. Surg. 60, 3332 –336. doi:10.1016/j.bjoms.2021.07.023
Mangano, C., Bianchi, A., Mangano, F. G., Dana, J., Colombo, M., Solop, I., et al. (2020). Custom-Made 3D Printed Subperiosteal Titanium Implants for the Prosthetic Restoration of the Atrophic Posterior Mandible of Elderly Patients: a Case Series. 3D Print. Med. 6, 1–14. doi:10.1186/s41205-019-0055-x
Mu, Z., He, Q., Xin, L., Li, Y., Yuan, S., Zou, H., et al. (2020). Effects of Injectable Platelet Rich Fibrin on Bone Remodeling in Combination with DBBM in Maxillary Sinus Elevation: a Randomized Preclinical Study. Am. J. Transl. Res. 12, 7312–7325.
Pignaton, T. B., Wenzel, A., Ferreira, C. E. d. A., Borges Martinelli, C., Oliveira, G. J. P. L., Marcantonio, E., et al. (2019). Influence of Residual Bone Height and Sinus Width on the Outcome of Maxillary Sinus Bone Augmentation Using Anorganic Bovine Bone. Clin. Oral Impl Res. 30, 315–323. doi:10.1111/clr.13417
Rammelsberg, P., Kilian, S., Büsch, C., and Kappel, S. (2020). The Effect of Transcrestal Sinus‐floor Elevation Without Graft on the Long‐Term Prognosis of Maxillary Implants. J. Clin. Periodontol. 47, 640–648. doi:10.1111/jcpe.13278
Keywords: bone height, custom-made, CAD/CAM, dental implant, digital workflow
Citation: Xu Z, Yang Z and Yang J (2022) Digital Workflow for the Design, Manufacture, and Application of Custom-Made Short Implants With Wing Retention Device. Front. Bioeng. Biotechnol. 10:885746. doi: 10.3389/fbioe.2022.885746
Received: 28 February 2022; Accepted: 19 April 2022;
Published: 08 June 2022.
Edited by:
Mika Salmi, Aalto University, FinlandReviewed by:
Mihaela Baciut, Iuliu Hațieganu University of Medicine and Pharmacy, RomaniaHenrique de Amorim Almeida, Polytechnic Institute of Leiria, Portugal
Copyright © 2022 Xu, Yang and Yang. This is an open-access article distributed under the terms of the Creative Commons Attribution License (CC BY). The use, distribution or reproduction in other forums is permitted, provided the original author(s) and the copyright owner(s) are credited and that the original publication in this journal is cited, in accordance with accepted academic practice. No use, distribution or reproduction is permitted which does not comply with these terms.
*Correspondence: Jianjun Yang, yjjqd@qdu.edu.cn
†These authors have contributed equally to this work