The relationship between lateral femoral condyle ratio measured by MRI and anterior cruciate ligament injury
- 1Department of Sports Medicine, The First People’s Hospital of Lianyungang, Lianyungang, China
- 2Clinical Research Center, The First People’s Hospital of Lianyungang, Lianyungang, China
Background: Previous studies have shown that the lateral femoral condyle ratio (LFCR) measured by X-ray has a significant relationship with the anterior cruciate ligament (ACL) injury. However, few relevant studies have been performed on LFCR measured by magnetic resonance imaging (MRI).
Purpose: (1) To evaluate the relationship between LFCR measured by MRI and ACL injury or rerupture. (2) To compare the LFCR measured by MRI with existing bony morphological risk factors and screen out the most predictive risk factors for primary ACL injury or rerupture.
Study Design: Cohort study; Level of evidence, 3.
Methods: Totally 147 patients who underwent knee arthroscopic surgery from 2015 to 2019 with minimum follow-up of 48 months were retrospectively evaluated. Patients were placed into three groups: 1) the control group of patients with simple meniscus tears without ligament injury; 2) the primary noncontact ACL injury group; 3) ACL rerupture group (ACL reconstruction failure). The LFCR measured by MRI and other previous known risk factors associated with MRI (notch width index, medial tibial slope, lateral tibial slope, medial tibial depth, lateral tibial height) were performed to evaluate their predictive value for ACL injury and rerupture. All the risk factors with p < 0.01 according to univariate analysis were included in the logistic regression models. Receiver operating characteristic (ROC) curves were analyzed for sensitivity, specificity, cut-off, and area under the curve (AUC). Z tests were used to compare the AUC values.
Results: The LFCR measured by MRI was obviously higher in primary ACL injury group (0.628 ± 0.020) and in ACL rerupture group (0.625 ± 0.021) than that in the control group (0.593 ± 0.030). The best risk factor was the LFCR with a cut-off of 0.602 (AUC, 0.818; 95% CI, 0.748–0.878; sensitivity, 90%; specificity, 66%). When combined with lateral tibial slope (cutoff, 7°) and lateral tibial height (cutoff, 3.6 mm), the diagnostic performance was improved significantly (AUC, 0.896; 95% CI, 0.890–0.950; sensitivity, 87%; specificity, 80%).
Conclusion: The increased LFCR measured by MRI was associated with a significantly higher risk for ACL injury or rerupture. The combination of LFCR, lateral tibial slope and lateral tibial height were the most predictive risk factors. This may help clinicians identify susceptible individuals and allow precision approaches for better prevention, treatment and management of this disease.
Introduction
The incidence of anterior cruciate ligament (ACL) injury among athletes and adolescents is increasing year by year (Beck et al., 2017). The profound effects of ACL injury on quality of life have drawn increased attention. Anterior cruciate ligament reconstruction is the gold standard of treatment for ACL injury (Attia et al., 2014), but the high re-rupture rate has plagued clinicians throughout the world (Lim et al., 2019). Among the leading research topics in sports and orthopedics is ACL injury risk factors (Carcia et al., 2019). There are a number of factors that may play a role in the risk of ACL injury, including gender (Herzog et al., 2017; Webster and Feller, 2016), body mass index (BMI) (DiSilvestro et al., 2019; Kaarre et al., 2023), neuromuscular factors (Smith et al., 2012), hormone (Smith et al., 2012), bony morphological factors (Bayer et al., 2020). Within this scope, we focused on the influence of bony morphological factors on ACL injury.
Multiple bony morphological factors have been reported to be related to the risk of ACL injury, such as the tibial slope (Hashemi et al., 2010; Hohmann et al., 2021), the medial tibial depth (MTD) (Hashemi et al., 2010), the lateral tibial height (LTH) (Kujala et al., 1992), the narrow intercondylar notch (NWI) (Hao et al., 2023). The relationship between the morphological characteristics of the distal femoral condyle and the incidence of noncontact ACL injury has become the hotspot of current research. In particular, the sphericity of lateral femoral condyle is considered to have intimate associations with the rotation and Pivot-shift of knee. Based on the lateral femoral condyle index (LFCI), Hodel et al. (2019) demonstrated that the decreased LFCI was associated with primary ACL injury. Nevertheless, the reproducibility and reliability of this measurement have been questioned by recent studies (Li et al., 2020; Nowak et al., 2022).
Comparably, in order to establish a specific standardized computational method to quantify the morphological characteristics of lateral femoral condyle, Pfeiffer et al. (2018) consider that the lateral femoral condyle ratio (LFCR) measured by X-ray is associated with a higher risk of noncontact ACL injury. It is important to note, however, that X-ray examination has some limitations such as relatively poor imaging quality, the deviation caused by knee rotation and the condylar overlap. As opposed to this, MRI is more standardized and can consistently and accurately visualize the morphological characteristics of femoral lateral condyle. Currently, there are few studies describing the MRI-based measurement of LFCR (He and Li, 2022; Gao et al., 2023).
The primary aim of the current study was to investigate the correlation between the LFCR measured by MRI and the incidence of ACL injury. The secondary aim was to compare the LFCR measured by MRI with existing bony morphological risk factors and to assess the most predictive risk factors for an ACL rupture or rerupture.
Materials and methods
This retrospective study protocol was approved by the Medical Institutional Ethics Committee of The First People’s Hospital of Lianyungang (KY-20230914002-01). During the period July 2015 through July 2019, 784 patients who underwent knee arthroscopic surgery with the same experienced sports medicine surgeon in The First People’s Hospital of Lianyungang were enrolled in this study. The patients were divided into three groups: 1) the control group, which included patients with simple meniscus tears without ligament injury and no signs of patellofemoral dysplasia; 2) the primary noncontact ACL injury group; 3) ACL rerupture after primary ACL reconstruction (ACLR) group. Patients in different groups were matched by sex and age and then assessed in accordance with the inclusion/exclusion criteria (Figure 1). A patient could not be affiliated with multiple groups. There was a minimum follow-up period of 48 months for all patients. All the patients received MRI examination and underwent radiographic evaluation by the same imaging physician and sports medicine surgeon for evaluation of the knee injury, such as injuries of medial collateral ligament, lateral collateral ligament, posterior cruciate ligament, medial patellofemoral ligament, popliteus tendon, meniscus and cartilage.
Furthermore, it is important to point out that radiographic data of the patients who underwent ACL revision surgical procedures were reviewed to exclude ACLR failure caused by tunnel misplacement (Pinczewski et al., 2008; van Eck et al., 2012). All patients underwent MRI at 3.0 T (Siemens Medical Systems, Germany). Imaging protocols included sagittal, coronal and axial sequences. The slice thickness was 3 mm.
According to the method for X-ray measurement of LFCR as Pfeiffer et al. (2018) described, LFCR was measured by MRI in this research. Through the use of coronal images (T1), the midsagittal plane of the lateral femoral condyle was determined at the level of the popliteal groove (Hodel et al., 2019; Hong et al., 2022). On the corresponding sagittal slide (T1), two circles were drawn at the center of the distal femur. The more distant circle should be as close as possible to the trochlea of the femur. A line that passes through the centers of the two circles was thus used to determine the long axis of the distal femur. Then a line was drawn between the most anterior point and the last point of the lateral condyle to determine the long axis of the lateral condyle of the femur. The distance from the intersection of these two lines to the last point of the lateral condyle was divided by the total length of the lateral condyle. This ratio was defined as the lateral femoral condyle ratio (LFCR) (Figure 2).
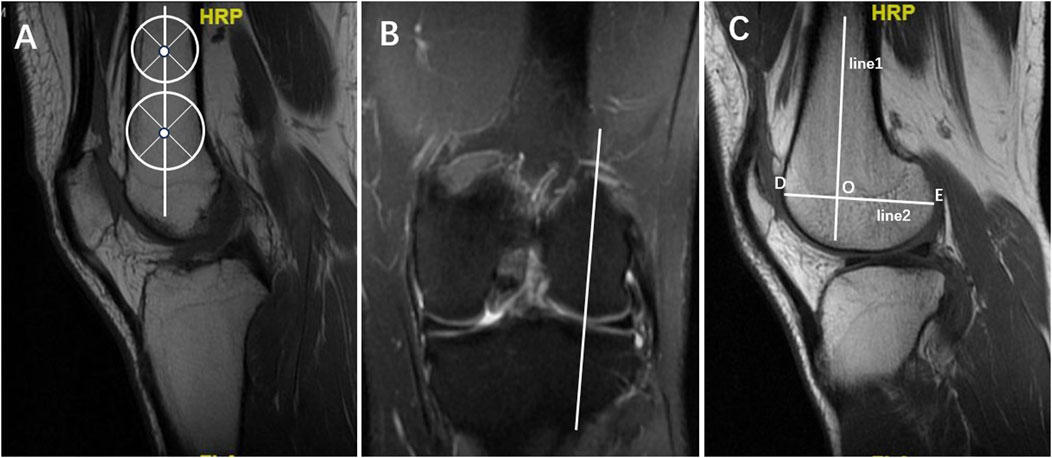
Figure 2. Lateral femoral condyle ratio (LFCI) measurement. (A) In the sagittal T1 centre of the knee MRI, two circles were drawn at the center of the distal femur. The more distant circle should be as close as possible to the trochlea of the femur. A line that passes through the centers of the two circles was thus used to determine the long axis of the distal femur (line1). (B) On T1 coronal sections, the midsagittal plane of the lateral femoral condyle was determined at the level of the popliteal groove. (C) On the corresponding sagittal slide (T1), the long axis of the distal femur (line1) was replicated and another line was drawn between the most anterior point (point D) and the last point of the lateral condyle (point E) to determine the long axis of the lateral condyle of the femur (line2). The distance from the intersection (point O) of these two lines to the last point of the lateral condyle was divided by the total length of the lateral condyle. LFCR = OE/DE.
In order to identify the strongest MRI predictors and construct a credible and convenient risk prediction model for ACL injury, other existing bone morphological risk factors were also performed: medial tibial slope (MTS) (Elmansori et al., 2017; Schneider et al., 2020), lateral tibial slope (LTS) (Kumar et al., 2020), medial tibial depth (MTD) (Misir et al., 2022), lateral tibial height (LTH) (Kujala et al., 1992) and notch width index (NWI) (Basukala et al., 2020; Bouras et al., 2018) (Figure 3). To evaluate the intraobserver reliability, one observer measured LFCRs in 50 patients randomly selected from the entire cohort and performed the same measurements again 2 weeks later. In order to evaluate the interobserver reliability, a second blinded observer made the measurements on the same patients independently.
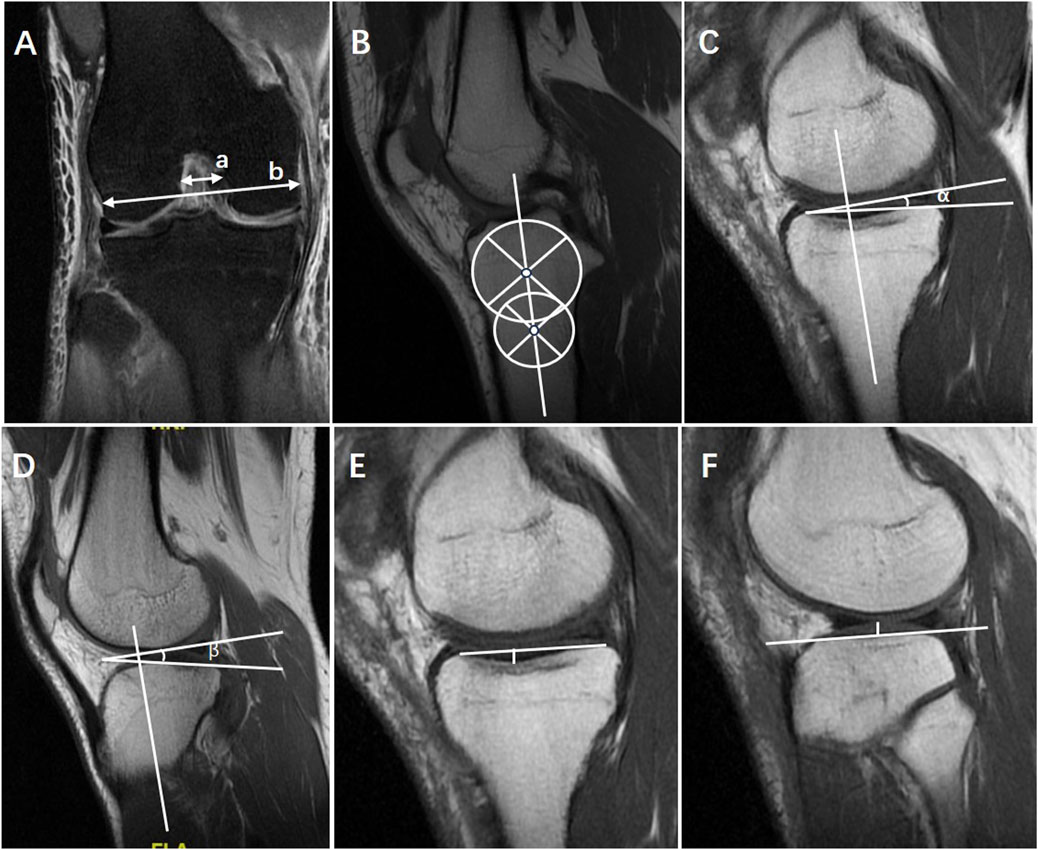
Figure 3. Other existing bone morphological risk factors were also measured by MRI with reference to the previous description, including (A) notch width index (NWI) = a/b, (B) the central axis of proximal tibia, (C) medial tibial slope (MTS) = α, on the center of the medial tibial plateau with the preserved longitudinal axis determined on the central sagittal slice, the tangent to the medial plateau was drawn to the cortex border, (D) lateral tibial slope (LTS) = β, on the center of the lateral tibial plateau with the preserved longitudinal axis determined on the central sagittal slice, the tangent to the lateral plateau was drawn to the cortex border, (E) medial tibial depth (MTD), (F) lateral tibial height (LTH).
Statistical analysis was conducted using the software SPSS 22.0(IBM, New York, USA) and MedCalc 12.7 (MedCalc Software, Ostende, Belgium). Statistical measures of continuous variables were presented as mean, standard deviation. A significant level was set at 0.05 (α = 0.05). The intraobserver and interobserver reliabilities were estimated with intraclass correlation coefficients (ICC). The normal distribution of the variables was assessed using the Kolmogorov-Smirnov test. As the data did not follow a normal distribution, non-parametric tests were used to analyze the bone morphological risk factors. Since there were no significant differences in the data between the ACL rerupture group and the primary ACL injury group, a pooled analysis of these two groups versus the control group was conducted. All the risk factors (LFCR, NWI, MTS, LTS, MTD, LTH) associated with p < 0.01 according to univariate analysis were included in the logistic regression models. Ultimately, LFCR, LTS and LTH were chosen as independent risk factors for noncontact ACL injury. The calculation encompassed not only the predictive performance of each individual risk factor but also the combined utility of LFCR, LTS, and LTH. In this study, we conducted Receiver Operating Characteristic (ROC) analysis and calculated area under the ROC curves (AUC) and 95% confidence intervals (CI) to assess the diagnostic performance. The AUC was compared with Medcalc software, and the Z-test was used to compare the predictive ability of different factors. The cutoff values were determined at maximum Youden index with optimal sensitivity and specificity.
Results
A total of 147 patients were included in the final analysis according to the criteria for inclusion and exclusion (55 in the primary ACL injury group, 22 in the ACL rerupture group and 70 in the control group). The results of a univariable analysis revealed no significant differences between the groups in terms of age, sex, height or BMI. In this study, there was a high level of intra- and interobserver reliability for the LFCR (intraobserver ICC, 0.908; interobserver ICC, 0.945).
The LFCR in the primary ACL injury group (median, 0.628 ± 0.02; range, 0.583–0.685) and in the ACL rerupture group (0.625 ± 0.02; range, 0.599–0.680) was significantly higher compared with that in the control group (0.593 ± 0.03; range, 0.528–0.680) (p < 0.01). There was no statistically significant difference between the primary ACL injury group and the ACL rerupture group (p = 0.680). The LTS, LTH, MTS and MTD were statistically significantly higher in the primary ACL injury group and ACL rerupture group than those in the control group (Table 1). For either of the MRI measurements, there were no statistically significant differences between the primary ACL injury group and the ACL rerupture group. There were no significant differences in LFCRs based on sex among all the study groups, with a mean LFCR of 0.628 for males and 0.628 for females in the primary ACL injury group, 0.625 for males and 0.624 for females in ACL rerupture group, and 0.593 for males and 0.593 for females in the control group.
Logistic regression analysis was used to screen out the risk factors for ACL injury, including LFCR (p < 0.01), MTS (p = 0.029), LTS (p < 0.01),MTD (p = 0.028), LTH (p < 0.01). LFCR, LTS and LTH were screened as independent risk factors for ACL injury. Further analysis of the ROC curves of all the risk factors showed statistical significance (Figure 4). The highest AUC was reported for LFCR (0.818; 95% CI, 0.748–0.878) to predict ACL injury or rerupture, and the sensitivity and specificity were 89.61% and 65.71%. A cutoff value of 0.602 (Youden index = 0.553) was related to a higher risk of ACL injury or rerupture. Among the remaining factors, the AUC of LTS (0.672; 95% CI, 0.583–0.741) (p < 0.001) and LTH (0.794; 95% CI, 0.720–0.857) (p < 0.001) were significantly larger than that of 0.5. The combination of LFCI, LTS and LTH by logistic regression yielded a higher AUC of 0.896 (95% CI, 0.890–0.950) and a higher specificity of 80% compared to those of LFCI alone (Table 2).
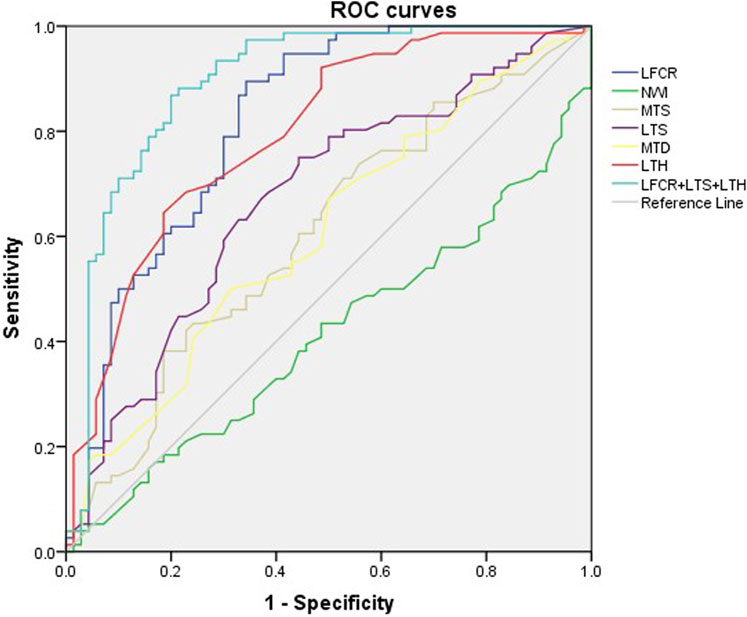
Figure 4. Diagnostic performance of the risk factors (LFCR, NWI, MTS, LTS, MTD, LTH, LFCR+LTS+LTH) was evaluated using receiver operating characteristic curves (AROC) and the reference line was AUC = 0.5. AUC, area under the curve; LFCR, lateral femoral condyle ratio; NWI, notch width index; MTS, medial tibial slope; LTS, lateral tibial slope; MTD, medial tibial depth; LTH, lateral tibial height.
Discussion
This study indicated that an increased lateral femoral condyle ratio (LFCR) measured by MRI was significantly associated with an increased prevalence rate of ACL injury or rerupture. The cutoff value of 0.602 for LFCR, with a sensitivity of ≥90% and a specificity of ≥66% for identifying ACL injury might help clinicians predict the potential risk of ACL injury and rerupture. The combination of the three most predictive factors (LFCR> 0.602, LTS>7° and LTH >3.6 mm) further enhanced the diagnostic performance for ACL injury, with an AUC of 0.896, a sensitivity of 87%, and a specificity of 80%. LFCR measured by MRI was proved to be an accurate, reliable, highly sensitive and reproducible indication for ACL injury.
A significant relationship has been found between the shape of the distal femur and knee joint movement (Bayer et al., 2020; Hodel et al., 2022; Pfeiffer et al., 2019). A larger LFCR means a flatter front of the lateral condyle of the femur and the depth of the posterior condyle is larger. As the limb changed position from safe to provocative to exaggerated provocative, the point of contact moved further anteriorly from the posterior tibial point. During the exaggerated provocative position, the lateral femoral condyle contacted the tibial plateau on its flat anterior surface rather than its rounder posterior surface. This might result in a greater likelihood for sliding instead of rolling, which could strain or tear the ACL with a compressive force (Boden et al., 2009).
There was no statistically significant difference in LFCRs between patients in the primary ACL injury group and in the ACL rerupture group. This might be attributed to three reasons. First, although postoperative CT excluded the possibility of nonanatomic tunnel placement, the risk for an ACL rerupture could be biased by a suboptimal tunnel placement (Hodel et al., 2019; Pinczewski et al., 2008). During ACL reconstruction in this study, as soon as the knee was hyperflexed, the femoral tunnel was drilled through the ACL femoral footprint. The entry of femoral tunnel was either at 2 or 10 o’clock position for left and right knee respectively. In the center of the ACL anatomical tibial footprint, located at the posterior border of the anterior horn of the lateral meniscus, the tibial tunnel was drilled with ACL tibial tunnel guide. Adouni et al. (2023) used a global sensitivity analysis based on variance decomposition to investigate the contribution of the surgical parameters to the uncertainty in response to the joint after ACL reconstruction surgery. Their results indicated that the joint contact center and area were affected mainly by the angle of fixation and the tunnel placements. It was important for surgeons to carefully construct anatomical tunnels with right dimension during ACL reconstruction since the tunnel dimension and the angle can also affect the rate of re-rupture (Vermeijden et al., 2020; Byrne et al., 2022; Snaebjornsson et al., 2017). Second, the insufficient follow-up time may be another disadvantage. We will further expand the sample size and prolong the duration of follow-up in future work in order to obtain more data to explore this correlation between the LFCR and the subsequent risk for ACL rerupture. Third, the effect of collagen diameter on ACL injury and rerupture needs further study. Researchers have determined the collagen fibril diameter distributions in healthy and injured ACL tissue of rat or sheep and created polycaprolactone (PCL) constructs to mimic the distributions of collagen fibrils in healthy and injured tissues successfully (Adeoye et al., 2022; Smatov et al., 2023). Moreover, Pfeiffer et al. (2018) also found that a profoundly increased LFCR could increase the risk for contralateral ACL injury compared with no ACL injury or primary ACL injury. In light of the results here, we anticipate a greater focus on this area of research in the future.
In addition, the risk factors analysis revealed a no significant difference in LFCR between men and women, which is contradictory to some current research results (Pfeiffer et al., 2018; Pinskerova et al., 2014). Pfeiffer et al. (2018) concluded that the LFCR measured by X-ray was significantly higher in female patients (64.6%) than in male patients (63.1%). This may explain why female athletes were at greater risk of ACL injury than male athletes (Montalvo et al., 2019). However, other studies denied this significance (He and Li, 2022; Hodel et al., 2019). This discrepancy may be due to the limited number of studies and sample size. Therefore, future studies are needed to verify these results.
Previous studies pointed out that as a result of excessive LTS and LTH, the tibia will move forward, the knee joint will become unstable, and the ACL will become tensed, increasing the risk of ACL injury (Chen et al., 2022; Kujala et al., 1992). Tat et al. (2021) mathematically modeled the posterior tibial plateau geometry in patients and found that patients with ACL injuries had a significantly greater posterolateral plateau slope than that of matched controls. The steeper drop off may result in higher anterior translation forces, coupled with internal rotation torques on the knee, which could increase ACL axial strain. Shi et al. (2024) performed a retrospective analysis on 52 patients undergoing primary or revision ACL reconstruction. It was found that compared to patients with intact ACLR, those who sustained nontraumatic ACL reconstruction failure showed significantly increased LTS, MTS and deceased NWI. The related biomechanical mechanism remains a hotspot of current research.
The results of this study suggest a higher risk of the prevalence of ACL injury or rerupture in patients with an increased LFCR, LTS and LTH. This matches the results previously reported and confirms our previous hypothesis that the tibial and femoral bony morphology of the lateral compartment together impact the rotatory stability as well as the anterior-posterior stability of knee. The combination of the three risk factors was most predictive for ACL injury. The prediction model may help clinicians to identify patients at high risk for ACL injury or rerupture who could benefit from a more precise and personalized risk assessment, surgical indication decision, additional extra-capsular reconstruction, adjustment of graft selection, modification of surgical techniques (Choi et al., 2023; Pfeiffer, 2023), prediction of postoperative outcomes and individualized precision rehabilitation therapy (Fu et al., 2021). However, how the three risk factors studied here could influence the biomechanical responses of the knee joint remains worthy of further study. Adouni et al. (2020) presented a novel iterative method for computing the in situ strain at reference configuration. This framework used an in situ strain gradient approach (deformed reference configuration) as well as a detailed finite element (FE) model of the knee joint. Then the effect of the risk factors on the biomechanical responses of the joint could be well investigated during the passive extension and flexion motions, the axial compression and the coupled internal–external rotations.
Numerous studies have proposed various methods for describing the bone morphological characteristics of the lateral compartment and a lot of imaging measurements have been screened out for assessing the risk of ACL injury, such as LFCI, NWI, MTS, LTS, MTD and LTH. Pfeiffer et al. (2018) measured LFCR by X-ray and prove it to be a reliable and independent risk factor for ACL injuries. The advantage of this method is that it is convenient, fast and cheap. However, a sizeable number of patients had to be excluded prior to analysis because of malrotated radiographs in that study. Voleti et al. (2014) believe that as a result of both articular cartilage thickness and the anatomic differences between medial and lateral condyles, Plain radiographs underestimate the posterior condylar depth measurements as well as the asymmetry of the medial and lateral posterior condylar offset compared with MRI. In order to avoid this as much as possible, we proposed a new method of measurement using MRI. MRI exhibits minimal radiation and possesses the capability to assess numerous imaging measurements which are connected with the bone morphological characteristics of the lateral compartment, albeit at a higher cost and with increased time requirements compared to X-ray techniques. In assessing the lateral condyle of the femur, LFCR measured by MRI is independent of rotation and tilt of the femur, which demonstrates high repeatability and reliability.
Conclusion
This research concludes that an increased LFCR measured by MRI is significantly associated with increased incidence of ACL injury or rerupture, and the LFCR, LTS and LTH are the most predictive risk factors for ACL injury. This may ultimately help clinicians identify those individuals at greatest risk of ACL injury, who may greatly benefit from prevention counseling, surgical or nonsurgical intervention and personalized postoperative rehabilitation protocol.
Data availability statement
The original contributions presented in the study are included in the article/supplementary material, further inquiries can be directed to the corresponding author.
Ethics statement
The studies involving humans were approved by the Medical Institutional Ethics Committee of The First People’s Hospital of Lianyungang. The studies were conducted in accordance with the local legislation and institutional requirements. Written informed consent for participation was not required from the participants or the participants’ legal guardians/next of kin in accordance with the national legislation and institutional requirements.
Author contributions
YS: Data curation, Methodology, Software, Writing–original draft, Investigation, Project administration. YT: Investigation, Methodology, Project administration, Supervision, Validation, Writing–review and editing.
Funding
The author(s) declare that no financial support was received for the research, authorship, and/or publication of this article.
Conflict of interest
The authors declare that the research was conducted in the absence of any commercial or financial relationships that could be construed as a potential conflict of interest.
Publisher’s note
All claims expressed in this article are solely those of the authors and do not necessarily represent those of their affiliated organizations, or those of the publisher, the editors and the reviewers. Any product that may be evaluated in this article, or claim that may be made by its manufacturer, is not guaranteed or endorsed by the publisher.
References
Adeoye, A. O., Mukasheva, F., Smatov, S., Khumyrzakh, B., Kadyr, S., Shulgau, Z., et al. (2022). A biomimetic synthetic nanofiber-based model for anterior cruciate ligament regeneration. Front. Bioeng. Biotechnol. 10, 969282. doi:10.3389/fbioe.2022.969282
Adouni, M., Faisal, T., and Dhaher, Y. (2023). Effect of surgical Design variations on the knee contact behavior during anterior cruciate ligament reconstruction. J. Knee Surg. 36 (3), 310–321. doi:10.1055/s-0041-1733879
Adouni, M., Faisal, T. R., and Dhaher, Y. Y. (2020). Computational frame of ligament in situ strain in a full knee model. Comput. Biol. Med. 126, 104012. doi:10.1016/j.compbiomed.2020.104012
Attia, E., Bohnert, K., Brown, H., Bhargava, M., and Hannafin, J. A. (2014). Characterization of total and active matrix metalloproteinases-1, -3, and -13 synthesized and secreted by anterior cruciate ligament fibroblasts in three-dimensional collagen gels. Tissue Eng. Part A 20 (1-2), 171–177. doi:10.1089/ten.TEA.2012.0669
Basukala, B., Joshi, A., and Pradhan, I. (2020). The effect of the intercondylar notch shape and notch width index on anterior cruciate ligament injuries. J. Nepal Health Res. Counc. 17 (4), 532–536. doi:10.33314/jnhrc.v17i4.1858
Bayer, S., Meredith, S. J., Wilson, K. W., de Sa, D., Pauyo, T., Byrne, K., et al. (2020). Knee morphological risk factors for anterior cruciate ligament injury: a systematic review. J. Bone Jt. Surg. Am. 102 (8), 703–718. doi:10.2106/JBJS.19.00535
Beck, N. A., Lawrence, J., Nordin, J. D., DeFor, T. A., and Tompkins, M. (2017). ACL tears in school-aged children and adolescents over 20 years. Pediatrics 139 (3), e20161877. doi:10.1542/peds.2016-1877
Boden, B. P., Breit, I., and Sheehan, F. T. (2009). Tibiofemoral alignment: contributing factors to noncontact anterior cruciate ligament injury. J. Bone Jt. Surg. Am. 91 (10), 2381–2389. doi:10.2106/JBJS.H.01721
Bouras, T., Fennema, P., Burke, S., and Bosman, H. (2018). Stenotic intercondylar notch type is correlated with anterior cruciate ligament injury in female patients using magnetic resonance imaging. Knee Surg. Sports Traumatol. Arthrosc. 26 (4), 1252–1257. doi:10.1007/s00167-017-4625-4
Byrne, K. J., Hughes, J. D., Gibbs, C., Vaswani, R., Meredith, S. J., Popchak, A., et al. (2022). Non-anatomic tunnel position increases the risk of revision anterior cruciate ligament reconstruction. Knee Surg. Sports Traumatol. Arthrosc. 30 (4), 1388–1395. doi:10.1007/s00167-021-06607-7
Carcia, C. R., Cacolice, P. A., and McGeary, S. (2019). Defining lower extremity dominance: the relationship between preferred lower extremity and two functional tasks. Int. J. Sports Phys. Ther. 14 (2), 188–191. doi:10.26603/ijspt20190188
Chen, Y., Ding, J., Dai, S., Yang, J., Wang, M., Tian, T., et al. (2022). Radiographic measurement of the posterior tibial slope in normal Chinese adults: a retrospective cohort study. BMC Musculoskelet. Disord. 23 (1), 386. doi:10.1186/s12891-022-05319-4
Choi, N. H., Lee, D. M., Shin, H. J., and Victoroff, B. N. (2023). Combined anterolateral ligament and anterior cruciate ligament injury is associated with increased lateral femoral condyle ratio. Arthroscopy 39 (5), 1235–1241. doi:10.1016/j.arthro.2022.11.019
DiSilvestro, K. J., Jauregui, J. J., Glazier, E., Cherkalin, D., Bennett, C. H., Packer, J. D., et al. (2019). Outcomes of anterior cruciate ligament reconstruction in obese and overweight patients: a systematic review. Clin. J. Sport Med. 29 (4), 257–261. doi:10.1097/JSM.0000000000000521
Elmansori, A., Lording, T., Dumas, R., Elmajri, K., Neyret, P., and Lustig, S. (2017). Proximal tibial bony and meniscal slopes are higher in ACL injured subjects than controls: a comparative MRI study. Knee Surg. Sports Traumatol. Arthrosc. 25 (5), 1598–1605. doi:10.1007/s00167-017-4447-4
Fu, F., Byrne, K. J., and Lucidi, G. A. (2021). Editorial commentary: outcomes after anterior cruciate ligament reconstruction are defined by individual anatomy, including both soft tissue and bone morphology: it's all important. Arthroscopy 37 (8), 2542–2544. doi:10.1016/j.arthro.2021.04.019
Gao, Y. T., Yang, Y. P., Meng, Q. Y., Chen, N. Y., Ma, Y., Liu, P., et al. (2023). Increased lateral femoral condyle ratio measured by magnetic resonance imaging is associated with anterior cruciate ligament rerupture. Arthroscopy. doi:10.1016/j.arthro.2023.09.026
Hao, K., Niu, Y., Kong, L., and Wang, F. (2023). The patient with patellar instability has a stenotic intercondylar notch and a thin anterior cruciate ligament: a retrospective comparative study. J. Orthop. Surg. Res. 18 (1), 144. doi:10.1186/s13018-023-03632-9
Hashemi, J., Chandrashekar, N., Mansouri, H., Gill, B., Slauterbeck, J. R., Schutt, R. J., et al. (2010). Shallow medial tibial plateau and steep medial and lateral tibial slopes: new risk factors for anterior cruciate ligament injuries. Am. J. Sports Med. 38 (1), 54–62. doi:10.1177/0363546509349055
He, M., and Li, J. (2022). Increased lateral femoral condyle ratio measured by MRI is associated with higher risk of noncontact anterior cruciate ligament injury. BMC Musculoskelet. Disord. 23 (1), 190. doi:10.1186/s12891-022-05134-x
Herzog, M. M., Marshall, S. W., Lund, J. L., Pate, V., Mack, C. D., and Spang, J. T. (2017). Incidence of anterior cruciate ligament reconstruction among adolescent females in the United States, 2002 through 2014. JAMA Pediatr. 171 (8), 808–810. doi:10.1001/jamapediatrics.2017.0740
Hodel, S., Kabelitz, M., Tondelli, T., Vlachopoulos, L., Sutter, R., and Fucentese, S. F. (2019). Introducing the lateral femoral condyle index as a risk factor for anterior cruciate ligament injury. Am. J. Sports Med. 47 (10), 2420–2426. doi:10.1177/0363546519858612
Hodel, S., Postolka, B., Flury, A., Schutz, P., Taylor, W. R., Vlachopoulos, L., et al. (2022). Influence of bone morphology on in vivo tibio-femoral kinematics in healthy knees during gait activities. J. Clin. Med. 11 (17), 5082. doi:10.3390/jcm11175082
Hohmann, E., Tetsworth, K., Glatt, V., Ngcelwane, M., and Keough, N. (2021). Medial and lateral posterior tibial slope are independent risk factors for noncontact ACL injury in both men and women. Orthop. J. Sports Med. 9 (8), 232596712110159. doi:10.1177/23259671211015940
Hong, C. K., Lin, Y. J., Cheng, T. A., Chang, C. H., Hsu, K. L., Kuan, F. C., et al. (2022). Adult patients with ACL tears have greater tibial internal rotation in MRI compared to adolescent patients. J. Orthop. Surg. Res. 17 (1), 17. doi:10.1186/s13018-022-02912-0
Kaarre, J., Zsidai, B., Winkler, P. W., Narup, E., Horvath, A., Svantesson, E., et al. (2023). Different patient and activity-related characteristics result in different injury profiles for patients with anterior cruciate ligament and posterior cruciate ligament injuries. Knee Surg. Sports Traumatol. Arthrosc. 31 (1), 308–315. doi:10.1007/s00167-022-07131-y
Kujala, U. M., Nelimarkka, O., and Koskinen, S. K. (1992). Relationship between the pivot shift and the configuration of the lateral tibial plateau. Arch. Orthop. Trauma Surg. 111 (4), 228–229. doi:10.1007/BF00571483
Kumar, P. T., Das, A., Mohanty, T., Samanta, S., and Kumar, M. S. (2020). Study of relationship of posterior tibial slope in anterior cruciate ligament injury. J. Orthop. 21, 487–490. doi:10.1016/j.jor.2020.08.032
Li, R., Liu, Y., Fang, Z., and Zhang, J. (2020). Introducing the lateral femoral condyle index as a risk factor for anterior cruciate ligament injury: letter to the editor. Am. J. Sports Med. 48 (7), P42. doi:10.1177/0363546520920546
Lim, W. L., Liau, L. L., Ng, M. H., Chowdhury, S. R., and Law, J. X. (2019). Current progress in tendon and ligament tissue engineering. Tissue Eng. Regen. Med. 16 (6), 549–571. doi:10.1007/s13770-019-00196-w
Misir, A., Sayer, G., Uzun, E., Guney, B., and Guney, A. (2022). Individual and combined anatomic risk factors for the development of an anterior cruciate ligament rupture in men: a multiple factor analysis Case-Control study. Am. J. Sports Med. 50 (2), 433–440. doi:10.1177/03635465211062594
Montalvo, A. M., Schneider, D. K., Webster, K. E., Yut, L., Galloway, M. T., Heidt, R. J., et al. (2019). Anterior cruciate ligament injury risk in sport: a systematic review and Meta-Analysis of injury incidence by sex and sport classification. J. Athl. Train. 54 (5), 472–482. doi:10.4085/1062-6050-407-16
Nowak, E. K., Beaulieu, M. L., Beynnon, B. D., Ashton-Miller, J. A., Sturnick, D. R., and Wojtys, E. M. (2022). The lateral femoral condyle index is not a risk factor for primary noncontact anterior cruciate ligament injury. Am. J. Sports Med. 50 (1), 85–92. doi:10.1177/03635465211057271
Pfeiffer, T. R. (2023). Editorial commentary: bony morphology determines the extent of concomitant injuries in anterior cruciate ligament injured knees. Arthroscopy 39 (5), 1242–1243. doi:10.1016/j.arthro.2022.12.019
Pfeiffer, T. R., Burnham, J. M., Hughes, J. D., Kanakamedala, A. C., Herbst, E., Popchak, A., et al. (2018). An increased lateral femoral condyle ratio is a risk factor for anterior cruciate ligament injury. J. Bone Jt. Surg. Am. 100 (10), 857–864. doi:10.2106/JBJS.17.01011
Pfeiffer, T. R., Burnham, J. M., Kanakamedala, A. C., Hughes, J. D., Zlotnicki, J., Popchak, A., et al. (2019). Distal femur morphology affects rotatory knee instability in patients with anterior cruciate ligament ruptures. Knee Surg. Sports Traumatol. Arthrosc. 27 (5), 1514–1519. doi:10.1007/s00167-018-5269-8
Pinczewski, L. A., Salmon, L. J., Jackson, W. F., von Bormann, R. B., Haslam, P. G., and Tashiro, S. (2008). Radiological landmarks for placement of the tunnels in single-bundle reconstruction of the anterior cruciate ligament. J. Bone Jt. Surg. Br. 90 (2), 172–179. doi:10.1302/0301-620X.90B2.20104
Pinskerova, V., Nemec, K., and Landor, I. (2014). Gender differences in the morphology of the trochlea and the distal femur. Knee Surg. Sports Traumatol. Arthrosc. 22 (10), 2342–2349. doi:10.1007/s00167-014-3186-z
Schneider, A., Arias, C., Bankhead, C., Gaillard, R., Lustig, S., and Servien, E. (2020). Greater medial tibial slope is associated with increased anterior tibial translation in females with an ACL-deficient knee. Knee Surg. Sports Traumatol. Arthrosc. 28 (6), 1901–1908. doi:10.1007/s00167-019-05643-8
Shi, W. L., Gao, Y. T., Zhang, K. Y., Liu, P., Yang, Y. P., Ma, Y., et al. (2024). Femoral tunnel malposition, increased lateral tibial slope, and decreased notch width index are risk factors for Non-Traumatic anterior cruciate ligament reconstruction failure. Arthroscopy 40 (2), 424–434.e3. doi:10.1016/j.arthro.2023.06.049
Smatov, S., Mukasheva, F., and Erisken, C. (2023). Collagen fibril diameter distribution of sheep anterior cruciate ligament. Polym. (Basel) 15 (3), 752. doi:10.3390/polym15030752
Smith, H. C., Vacek, P., Johnson, R. J., Slauterbeck, J. R., Hashemi, J., Shultz, S., et al. (2012). Risk factors for anterior cruciate ligament injury: a review of the literature - part 1: neuromuscular and anatomic risk. Sports Health 4 (1), 69–78. doi:10.1177/1941738111428281
Snaebjornsson, T., Hamrin, S. E., Ayeni, O. R., Alentorn-Geli, E., Krupic, F., Norberg, F., et al. (2017). Graft diameter as a predictor for revision anterior cruciate ligament reconstruction and KOOS and EQ-5D values: a cohort study from the Swedish national knee ligament register based on 2240 patients. Am. J. Sports Med. 45 (9), 2092–2097. doi:10.1177/0363546517704177
Tat, J., Crapser, D., Alaqeel, M., Schupbach, J., Lee-Howes, J., Tamimi, I., et al. (2021). A quantifiable risk factor for ACL injury: applied mathematics to model the posterolateral tibial plateau surface geometry. Orthop. J. Sports Med. 9 (4), 232596712199831. doi:10.1177/2325967121998310
van Eck, C. F., Schkrohowsky, J. G., Working, Z. M., Irrgang, J. J., and Fu, F. H. (2012). Prospective analysis of failure rate and predictors of failure after anatomic anterior cruciate ligament reconstruction with allograft. Am. J. Sports Med. 40 (4), 800–807. doi:10.1177/0363546511432545
Vermeijden, H. D., Yang, X. A., van der List, J. P., DiFelice, G. S., Rademakers, M. V., and Kerkhoffs, G. (2020). Trauma and femoral tunnel position are the most common failure modes of anterior cruciate ligament reconstruction: a systematic review. Knee Surg. Sports Traumatol. Arthrosc. 28 (11), 3666–3675. doi:10.1007/s00167-020-06160-9
Voleti, P. B., Stephenson, J. W., Lotke, P. A., and Lee, G. C. (2014). Plain radiographs underestimate the asymmetry of the posterior condylar offset of the knee compared with MRI. Clin. Orthop. Relat. Res. 472 (1), 155–161. doi:10.1007/s11999-013-2946-2
Keywords: anterior cruciate ligament, ACL, lateral femoral condyle ratio, LFCR, MRI, risk factor
Citation: Sun Y and Tang Y (2024) The relationship between lateral femoral condyle ratio measured by MRI and anterior cruciate ligament injury. Front. Bioeng. Biotechnol. 12:1362110. doi: 10.3389/fbioe.2024.1362110
Received: 27 December 2023; Accepted: 18 March 2024;
Published: 27 March 2024.
Edited by:
Yujiang Fan, Sichuan University, ChinaReviewed by:
Malek Adouni, Abdullah Al Salem University, KuwaitCevat Erisken, Nazarbayev University, Kazakhstan
Copyright © 2024 Sun and Tang. This is an open-access article distributed under the terms of the Creative Commons Attribution License (CC BY). The use, distribution or reproduction in other forums is permitted, provided the original author(s) and the copyright owner(s) are credited and that the original publication in this journal is cited, in accordance with accepted academic practice. No use, distribution or reproduction is permitted which does not comply with these terms.
*Correspondence: Yun Tang, 1021802445@qq.com