Screen Wall Fire Resistance Investigation
- 1Department of Integrated Safety in Construction, Moscow State University of Civil Engineering, Moscow, Russia
- 2Department of Supervisory Activity and Preventive Work of the Russian Ministry for Emergency Situations, Moscow, Russia
The assessment of fire resistance for building constructions has been carried out by the solution of the heat engineering problem for heating up building structures under the conditions of non-stationary heat exposure with standard fire conditions. The finite element method is used as the solution method. Numerical simulation of heating of considered building structures under fire conditions was carried out using the Ansys Mechanical computer complex, which allows for a thermal engineering design to be calculated on the basis of its software model. The resulting functional dependence allows for determining the actual fire resistance of Pf depending on the thickness of sheets δ of the outer skin made of the basalt fire-proof material, as well as the geometric parameters of the protective screen (δ) depending on the required fire resistance from the condition of compliance with the requirements of constructive fire safety (Pf > Preq). Experimental studies to determine the fire resistance for membrane wall structures showed that an increase in temperature on its unheated surface vs. structure temperature prior to testing more than 180° С (195°С) occurs not earlier than 31 min. Upon reaching the critical temperatures on the unheated surface of the membrane wall structure independing on its thickness, the fire resistance limits REI 30-REI 120 are reached.
Introduction
In the recent years, large multifunctional and high-rise buildings and complexes have been under construction in many cities. The multifunctional buildings include groups of premises for different functional purposes (residential, administrative, office, cultural and entertainment, health care, parking, etc.). To ensure fire safety and limit the spread of combustion products to other premises outside the fire area, the building is divided into fire compartments separated by Type 1 fire barriers:
(1) for parts of the building of a certain functional fire hazard class (residential, public, car park, etc.);
(2) for groups of premises of one functional fire hazard class, if their area exceeds the maximum allowable floor area between fire barriers of type 1.
Volumetric planning solutions of modern multifunctional buildings are becoming more and more complex, buildings now include multi-level atriums, arcades, premises of large volumes and areas, fully glazed facades.
Among the variety of modern multifunctional facilities, a special place is held by airports–the facilities with unique infrastructure. Fires occurring at airports are in most cases accompanied by heavy death toll, group injury, and significant material damage. The implementation of fire safety requirements for fire resistance and for airport design, as well as for designing atriums (multi-storied) involves the use of innovative solutions, the introduction of new technologies to limit the spread of fire hazards. The implementation of fire safety requirements to fire resistance limits and the construction of airport buildings, as well as the design of atrium spaces involves the use of innovative solutions, the introduction of new technologies to limit the spread of fire hazards.
Materials and Methods
Technical Decisions of the Fireproof Non-load-bearing Walls
The fire barriers also include fireproof non-load-bearing walls. The purpose of non-bearing fire walls is to prevent the spread of fire, as well as to separate the building into fire compartments, so that fire and combustion products do not spread from one part of the building to another. In this case, at normal time (not in fire mode), the load-bearing wall is mounted in a box in the wall partition and does not interfere with the free movement of people, and in case of emergency, the signal is automatically activated, by which the wall is extended, creating an obstacle to the fire (to heat and gas flows).
In the international practice nowadays there are technical decisions concerning the design and application of fire safety barriers, which have been approved for designing and construction of protected facilities of different classes of functional fire hazard. To avoid the spread of fire hazards, the use of screen structures (screen walls) is allowed (Figure 1).
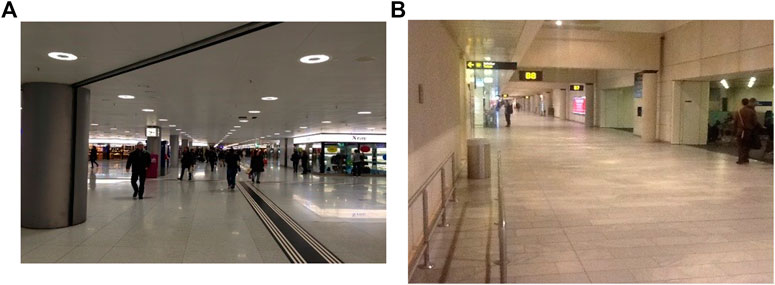
FIGURE 1. The fireproof non-load-bearing walls: (A) The screen structures in the trade center; (B) The screen structures in the airport terminal.
Structurally, a fire protection wall consists of a supporting frame made of a profile tube to which steel sheets are welded. The steel sheets are pre-bent to give additional rigidity. The inner side of the sheets is covered with a fire protection compound. Basalt slabs are placed between the sheets. To the lower part of the frame, slides are welded. The wall is moved by a linear actuator automatically on command from sensors (Figures 2, 3).
In accordance with the Program for the Development of National Standards of Russia and the Program of International Standardization, an international GOST standard has been developed “Extended application of the results of fire resistance testing. Non-bearing walls. Screen walls”, part 6. The provisions of this standard define the procedure for establishing the exact parameters and factors governing the design of non-load-bearing enclosing structures (screen walls).
Innovative aspect of this method is the introduction of fire resistance tests of extended application - the expected behavior of a nonbearing enclosure and its components under the influence of flames, which may be based on interpolation and extrapolation of data obtained in the relevant tests.
The nonbearing enclosing structure under test functions as an integral system in which each individual element (frame and fixing system, frame filling panels, perimeter seals and vertical clearance seals) is selected in such a way as to effectively ensure the required fire resistance values. In addition to the rules for the structure as a whole, this document also defines the rules for the individual components of the nonbearing enclosing structures: the fixing system, the frame filling panels, the methods of their fastening, the perimeter gaskets and the vertical clearance gaskets as well as the supporting structures (walls, floor). Not only can this technical solution solve many problems associated with the zoning of multifunctional transport infrastructure facilities, including passenger terminals, but it can also provide technological links and prevent the spread of fire.
The limit state is the state of a structure at which it ceases to meet the operational requirements, i.e. it either loses the ability to resist external influences or suffers unacceptable deformation or local damage. Further operation of such a structure is inadmissible or unreasonable.
The fire resistance limit is an indicator that defines the protection of a building or structure from direct exposure to fire. In fact, it is a time factor during which a building retains its functional and load-bearing characteristics, i.e. it preserves its original construction without being destroyed or deformed (walls, slabs and roofs are not destroyed). The actual fire resistance limit is the value of fire resistance, which is revealed at the stage of fire testing of a structural element. As a rule, the actual fire resistance limit is calculated, but for typical constructions experimental methods of determination of the actual fire resistance limit can also be applied. A structure with an actual fire resistance limit retains its bearing function for the period of time required to ensure safety:
where Rf–the actual value of the fire resistance limit of the structure, Rreq–required value of fire resistance limit of the structure.
As a research method for determining fire and technical characteristics of the screen wall material, the possibility of applying a proven method for determining the thermal radiation of the incident heat flow has been considered. This method is developed on the basis of analysis of the causes of fire propagation among the protected facilities and takes into account the radiant heat exchange. The convective component of the heat flow is neglected, as in fires it is always directed upwards and does not affect the degree of heating of the irradiated object.
The method is based on the classical theory of radiant heat exchange. The problem is reduced to comparison of real (incident) density of heat flux for the irradiated object qinc with the maximum permissible qperm.
The safety condition is fulfilled, if qinc ≤ qperm.
In this case, the permissible radiation intensity is an experimental value. In order to determine this value, appropriate experimental studies have been conducted.
According to the theory of combustion processes, the incident heat flux on the sample is partly reflected, partly absorbed and partly passed (Figure 4).
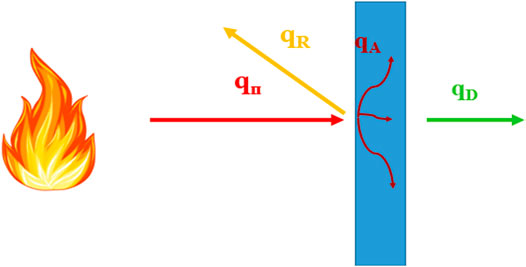
FIGURE 4. The scheme of interaction between incident heat flow and sample: qi–incident heat flow, W/m2; qr–reflected heat flow, W/m2; qA–absorbed heat flow, W/m2; qD–heat flow passed through the body, W/m2.
If the specified heat fluxes are related to the incident heat flux, the energy conservation equation is written by the formula:
where qi–incident heat flux, W/m2; qr–reflected heat flux, W/m2; qA–absorbed heat flux, W/m2; qD–passed through the body heat flux, W/m2.
To determine which fraction of the incident heat flow is reflected, absorbed, or passed through the sample, the formula is presented in a dimensionless form (each term is divided by the incident heat flow):
where R is the reflectivity coefficient of the body; A is the absorption coefficient of the body; D is the body transmittance coefficient.
The investigation of the temperature regime and critical values of the incident heat flow when using screen walls is based on determining the coefficients of reflection, absorption and transmission capacity of the presented sample. To determine these coefficients, a series of experiments are planned, during which the experimental test benches are adapted for determining the specified coefficients. To determine the behavior of the screen wall structure at high temperature (prior to large-scale testing of the structure assembly), it is possible to determine the applicability of the proposed screen wall material.
Evaluation of the fire resistance limit of the filler of the enclosing structure under consideration is carried out by solving the heat engineering problem of heating the building structures in conditions of non-stationary heat influence under the standard fire conditions. When calculating the thermal state of arbitrary structures, the problem of finding a non-stationary temperature field in a predefined area is solved. Several approaches are possible: experimental, analytical and numerical. Available experimental data on testing of structures similar to those under consideration allow us to determine the fire resistance limit without fire tests. In this case, the finite element method (FEM) is used. The method is based on the principle of division of the investigated area into a set of sub-regions with given thermophysical characteristics.
Numerical modeling of the heating of the structures considered under fire conditions was carried out with the help of Ansys Mechanical computing complex, which makes it possible to carry out heat engineering calculation of the structure on the basis of its software model.
Development of the Initial Software Model of the Screen Wall Construction
Numerical The mathematical description of heating of any construction includes the differential equation describing heat distribution in a solid body with known thermophysical properties, as well as initial and boundary conditions.
Unambiguous conditions for solving the differential equation of heat conductivity by numerical methods include:
• Geometric properties of the system (its shape and dimensions).
• Boundary conditions that take into account the interaction with the environment.
• Time (initial) conditions characterizing the state of the system at the initial moment.
• Thermophysical properties containing physical constants of materials in the system under consideration.
It is assumed that the materials that make up the screen wall have isotropic thermophysical properties, and there are no internal heat sources.
Geometric Properties of the System
The screen wall structure is a combination of three layers of isotropic materials in direct contact with each other. The geometrical model of the system, due to the isotropic nature of its materials, is adopted as a fragment having the form of a rectangular parallelepiped with dimensions of 0.1 × 0.1 × 0.03 m and different thicknesses, depending on the type of screen wall construction under study. The geometrical model of the structure is shown on Figure 5.
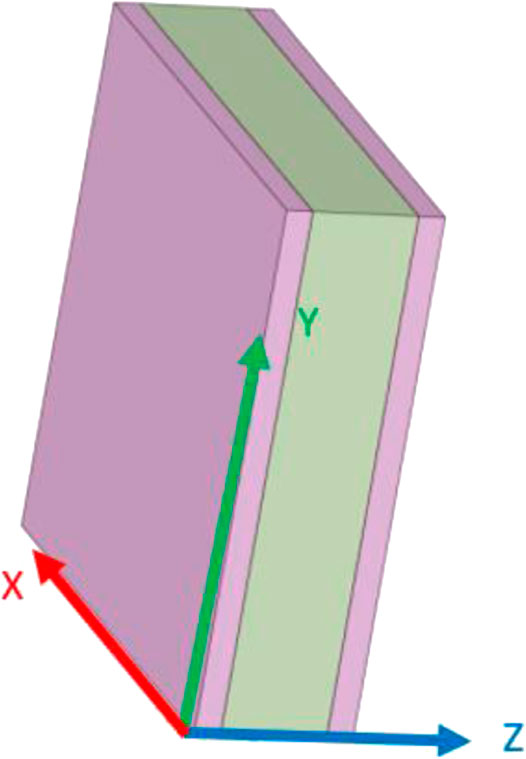
FIGURE 5. The fragment of the geometric model of the investigated screen wall with dimensions: 0,1 × 0,1 × 0,03 m: Outer layer - sandwich panels; inner layer - fireproof composition; fireproof composition consumption: 8.0–8.7 kg/m2.
Boundary Conditions, Taking Into Account the Interaction With the Environment
We use boundary conditions of the third kind, which are the law of the ambient temperature change and the law of heat exchange between the body surface and the environment. For high-temperature conditions, the standard fire mode is adopted as the law of the ambient temperature change. The change in the heat transfer coefficient of the “fire chamber - screen wall surface” system is set by the dependence:
Time (Initial) Conditions of the System State
Law of temperature distribution inside the body at the initial moment (at τ = 0): t = t0 = const = 20°C.
Investigation of the thermophysical characteristics of the screen wall materials
These studies are conducted using laboratory methods. The general scheme of the research carried out corresponds to the solution of the inverse problem of heat conductivity, at its solution such values of heat conductivity and heat capacity of the material are set, at which the results of the numerical calculation coincide with the results of heating obtained during laboratory tests of samples.
Based on the results of the conducted tests, the temperature dependences of the thermal conductivity coefficient and thermal capacity of local volumes of materials taking into account their moisture content have been obtained.
Verification of the Software Model of a Protective Screen Wall
On the basis of the developed program model of the screen wall construction using the obtained temperature dependencies of the thermophysical parameters of materials it is possible to estimate the fire resistance limit of the screen wall construction using the selected numerical modeling method.
To obtain reliable results of numerical calculations, verification of the developed software model on the basis of data on fire tests of similar construction is carried out. Verification of the initial model is carried out on the basis of reaching coincidence of the numerical calculation results with the results of fire tests of similar construction.
The conditions of the numerical modeling were accepted similar to those of the conducted fire tests. Figure 6 shows the results of the numerical calculation of the heating of the screen wall structure used in the fire tests.
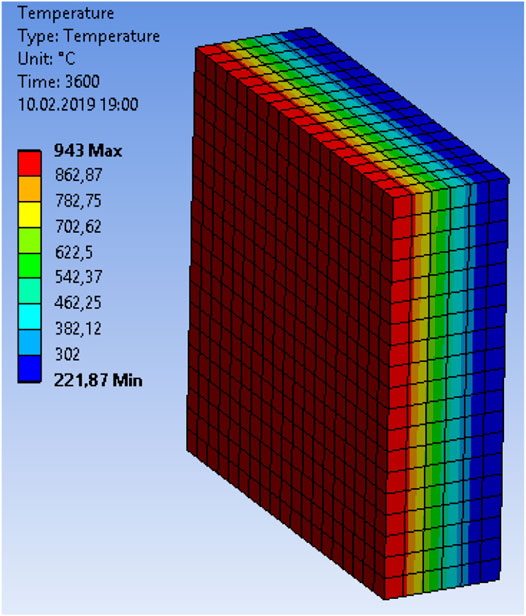
FIGURE 6. The temperature fields in the cross-section of the investigated screen wall after the heating time of 60 min.
The verification results allow us to draw the following conclusions:
• Effective values of heat conductivity and heat capacity coefficients determined in the course of solving the inverse problem provide satisfactory convergence of the results of numerical calculation and results of fire tests, including those for large-scale tests;
• The developed software model can be used for further research into the fire resistance of screen walls with the various geometrical parameters.
• Further research on the fire resistance of screen walls will be carried out for various thickness values of their outer layers (samples 5, 8, 10, 13, 16), in order to build a generalized dependence for the possibility of subsequent analytical calculation of the screen wall fire resistance with any intermediate values of the outer layers thickness of the protective material.
Results
The program complex ANSYS Mechanical was used for the research, based on the calculation-analytical approach to justification of real fire resistance limit for screen wall construction, considered in the work.
Fire Resistance Limits of the Considered “Sandwich” Type Screen Walls
The generalized results of calculation of fire resistance limits of screen walls with various designs are given in Table 1 and shown on Figure 7.
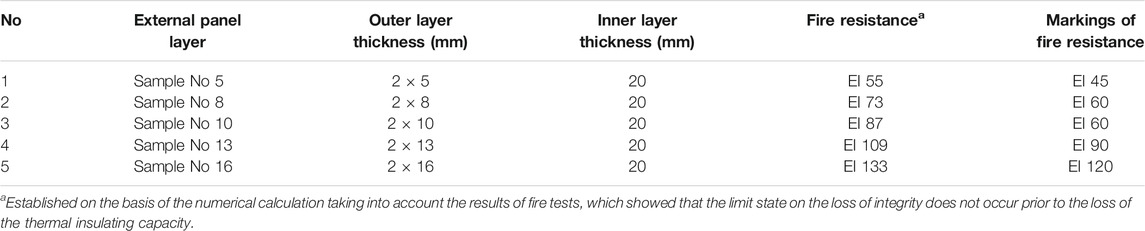
TABLE 1. Results of calculation of fire resistance limits of screen walls with various designs (samples No 5, 8, 10, 13, 16).
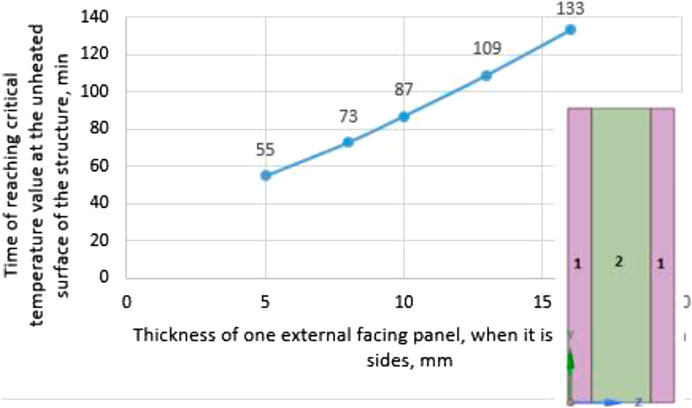
FIGURE 7. The time to reach the critical temperature on the unheated surface of the structure depending on the thickness of one panel of external facing (1) when it is placed on both sides and the middle layer is made of flame retardant composition (2).
The obtained results of fire resistance calculation of the protective screen walls of the various designs allow to reveal the functional dependence between the thickness of the outer paneling made of fireproof basalt material and the fire resistance limit of the protective screen wall (Sharovarnikov and Korolchenko, 2013; Korolchenko and Voevoda, 2016a; Korolchenko and Voevoda, 2016b; Korolchenko and Kholshchevnikov, 2017; Volkov et al., 2018). The research of properties of the components of the protective screen wall taking into account its fire-protective filling is based on experimental and theoretical studies (Markeev et al., 2006; Korolchenko et al., 2014; Korolchenko et al., 2015a; Korolchenko et al., 2015b; Degaev et al., 2015; Korolchenko D. et al., 2015; Bezrodnyy et al., 2016; Polandov et al., 2016; Degaev and Korolchenko, 2017; Konstantinova et al., 2019).
Determination of the functional dependence is carried out by the method of regression analysis of the data obtained from the numerical calculations (Ruban and Zaikov, 2001; Eremina, 2003; Wang and Chow, 2005; Zybina et al., 2009; Khalturinsky and Krupkin, 2011; Eremina et al., 2012; Lyapin et al., 2016; Zybina and Babkin, 2018). The regression equation can be presented as follows:
where X = X(X1, X2, …, Xm) – vector of independent (explanatory) variables; β–vector of parameters (to be defined); ε–random error (deviation); Y–dependent (explanatory) variable.
Evaluation of the Regression Equation
Let us define the vector of regression coefficient estimates. According to the least squares method, vector s is determined from the expression:
The vector of regression equation coefficients estimations has the following form:
The regression equation will be the following:
As a result of calculations the regression equation was obtained:
where Pf–the actual fire resistance limit, min;δ–thickness of external panels, mm.
Conclusion
Taking into account peculiarities of origin and development of fires at multifunctional, technically complicated facilities with large number of people, calculation and analytical approaches to substantiation of actual fire resistance limits of screen wall construction are essential. Based on the series of experiments to determine fire resistance for the screen wall structure it was found that the increase in temperature on its unheated surface in comparison with the temperature of the structure before the test by more than 180°C (195°C) occurs not earlier than in 31 min. New functional dependence in the form of multiple regression equation has been established, which makes it possible to determine the actual fire resistance limits of screen walls by the thickness of the inner heat-and-burn resistant material, ensuring the prevention of fire propagation, as well as their geometrical parameters without large-scale fire tests. Defined new patterns of behavior of structural elements screen walls under high-temperature exposure, which made it possible to install the actual area of application of screen walls, as well as fire-technical characteristics of structures and filling materials.
Data Availability Statement
The raw data supporting the conclusions of this article will be made available by the authors, without undue reservation.
Author Contributions
TE and DK contributed to the conception and design of the study. DK organized the database, TE wrote the first draft of the manuscript, DK corrected all the sections of the manuscript. All authors contributed to manuscript revision, read, and approved the submitted version of manuscript.
Funding
This work was financially supported by the Ministry of Science and Higher Education of the Russian Federation (Project: Theoretical and experimental design of new composite materials to ensure safety during the operation of buildings and structures under conditions of technogenic and biogenic threats #FSWG-2020-0007).
Conflict of Interest
The authors declare that the research was conducted in the absence of any commercial or financial relationships that could be construed as a potential conflict of interest.
Supplementary Material
The Supplementary Material for this article can be found online at: https://www.frontiersin.org/articles/10.3389/fbuil.2020.607305/full#supplementary-material.
References
Bezrodnyy, I. F., Giletich, A. N., Merkulov, V. A., Molchanov, V. P., and Shvyrkov, A. N. (1996). Tusheniye nefti i nefteproduktov [Extinguishing of oil and oil products]. Moscow, Russia: All-Russian Research Institute for Fire Protection Publ., 216 [in Russian, with English summary]
Degaev, E., and Korolchenko, D. (2017). Improving fire protection of pontoon tanks or floating roof tanks. MATEC Web Conf. 117, 00036. doi:10.1051/matecconf/201711700036
Degaev, E. N., Korolchenko, D. A., and Sharovarnikov, A. F. (2015). Effect of the multiplicity of foams on the main parameters of the hydrocarbon quenching process. In: Problemy tekhnosfernoy bezopasnosti-2015. Materialy 4-y mezhdunarodnoy nauchno-prakticheskoy konferentsii molodykh uchenykh i spetsialistov [problems of technospheric security-2015. Proceedings of 4th international scientific and practical conference of young scientists and specialists]. (Moscow, Russia: State Fire Academy of Emercom of Russia Publ.) 24–28. [in Russian, with English summary].
Korolchenko, D., and Voevoda, S. (2016a). Influence of dispersion degree of water drops on efficiency of extinguishing of flammable liquids. MATEC Web Conf. 86, 04056. doi:10.1051/matecconf/20168604056
Korolchenko, D., and Voevoda, S. (2016b). Influence of spreading structure in an aqueous solution-hydrocarbon system on extinguishing of the flame of oil products. MATEC Web Conf. 86, 04038. doi:10.1051/matecconf/20168604038
Korolchenko, D., and Kholshchevnikov, V. (2017). Conceptual problems of high-rise construction and differentiation of research within the urban environment system. MATEC Web Conf. 106, 01038. doi:10.1051/matecconf/201710601038
Eremina, T. (2003). Modeling and evaluation of fire retardant efficiency of intumescent flame retardants. Fire and Expl. Safety 12 (5), 22.
Eremina, T., Gravit, M., and Dmitrieva, Yu. (2012). Aspects and principles of formulation development for flame retardant intumescent compositions based on epoxy resins. Fire and expl. safety 7, 52–56.
Khalturinsky, N., and Krupkin, V. (2011). On the mechanism of action of fire retardant intumescent coatings. Fire and Expl. Safety 10, 33–41.
Konstantinova, N., Eremina, T., and Kuznetsova, I. (2019). Development of fire-proof textile materials safe for human skin. Chemical Fib. 2, 54–57. doi:10.1007/s10692-019-10052-1
Korolchenko, D. A., Degaev, E. N., and Sharovarnikov, A. F. (2015b). Dependence of fire extinguishing efficacy of low expansion foams solutions homology sodium sulfate on the molecular weight of the surface-active substances. In 2nd international conference on material engineering and application (ICMEA 2015). (Lancaster, United Kingdom: DEStech Publications, Inc.), 23–27.
Korolchenko, D. A., Degaev, E. N., Sharovarnikov, A. F., and Sharovarnikov, A. F. (2015a). Combustion of heptane in a model tank. Pvb 24 (2), 67–70. [in Russian, with English summary]. doi:10.18322/pvb.2015.24.2.67-70
Korolchenko, D. A., Sharovarnikov, A. F., and Byakov, A. V. (2014). The analysis of oil suppression by aqueous film forming foam through a gas-salt layer of water. Adv. Magn. Reson. 1073–1076, 2353–2357. doi:10.4028/www.scientific.net/amr.1073-1076.2353
Korolchenko D., , Tusnin, A., Trushin, S., and Korolchenko, A. (2015). Physical parameters of high expansion foam used for fire suppression in high-rise buildings. Int. J. Appl. Eng. Res. 10 (21), 42541–42548.
Lyapin, A., Korolchenko, A., and Meshalkin, E. (2016). Analysis of causes of combustible mixture explosions inside production floor areas. MATEC Web Conf. 86, 04030. doi:10.1051/matecconf/20168604030
Markeev, V. A., Voevoda, S. S., and Korolchenko, D. A. (2006). Fire protection of tank farm objects of RosneftNK OAO. Oil Indus. 9, 83–85. [in Russian, with English summary].
Polandov, Y., Korolchenko, A., and Dobrikov, S. (2016). Gas explosion in a room with a window and passage to an adjacent room. MATEC Web Conf. 86, 04031. doi:10.1051/matecconf/20168604031
Ruban, L., and Zaikov, G. (2001). Importance of intumescence in polymers fire retardancy. Int. J. Polym. Mater. 48, 295–310. doi:10.1080/00914030108050787
Sharovarnikov, A. F., and Korolchenko, D. A. (2013). Fighting fires of carbon dioxide in the closed buildings. in Applied mechanics and materials, vol. 475–476, (Freienbach, Switzerland: Trans Tech Publications, Ltd.), 1344–1350. doi:10.4028/www.scientific.net/amm.475-476.1344
Volkov, A. A., Roitman, V. M., Pri Prikruk, D. N., and Fedorov, V. Y. (2018). Influence of building materials humidity on accuracy of calculations of structures heating at estimations of their fire resistance (Electronic resource): collection of materials of the seminar held in the framework of the VI International scientific conference "Integration, partnership and innovation in building science and education. Moscow, Russia: Russian Federation, National Research University. Moscow State University of Civil Engineering and Construction. Available at: http://mgsu.ru/resources/izdatelskaya-deyatelnost/izdaniya/izdaniya-otkr-dostupa/ (Accessed 07 August 2020).
Wang, J. Q., and Chow, W. K. (2005). A brief review on fire retardants for polymeric foams. J. Appl. Polym. Sci. 97, 366–376. doi:10.1002/app.21758
Zybina, O. A., Varlamov, A. V., Chernova, N. S., and Mnatsakanov, S. S. (2009). On the role and transformations of components of intumescent fire-retardant paint-and-varnish formulations in the course of thermolysis. Russ. J. Appl. Chem. 82 (9), 1542–1546. doi:10.1134/s1070427209090079
Keywords: fire barriers, screen wall, fire-proof material, fire tests, fire safety
Citation: Eremina T, Korolchenko D and Fadeev VE (2021) Screen Wall Fire Resistance Investigation. Front. Built Environ. 6:607305. doi: 10.3389/fbuil.2020.607305
Received: 16 September 2020; Accepted: 17 December 2020;
Published: 19 April 2021.
Edited by:
Kambiz Narmashiri, Islamic Azad University, Zahedan, IranReviewed by:
Faiz Ahmad, University of Technology Petronas, MalaysiaMengjie Song, Beijing Institute of Technology, China
Muhammad Yasir, Institute of Space Technology, Pakistan
Copyright © 2021 Eremina, Korolchenko and Fadeev. This is an open-access article distributed under the terms of the Creative Commons Attribution License (CC BY). The use, distribution or reproduction in other forums is permitted, provided the original author(s) and the copyright owner(s) are credited and that the original publication in this journal is cited, in accordance with accepted academic practice. No use, distribution or reproduction is permitted which does not comply with these terms.
*Correspondence: Tatiana Eremina, main@stopfire.ru