Enhancement of Himalayan irregular stone masonry buildings for resilient seismic design
- 1Department of Civil Engineering, Kathmandu University, Dhulikhel, Nepal
- 2Department of Civil Engineering, Merrimack College, North Andover, MA, United States
In the Himalayan region of Nepal, stone masonry has been used for centuries as the primary building material for structures with or without mud mortar. In three distinct remote rural villages, a thorough structural survey of approximately 223 buildings was conducted with an emphasis on their structural irregularities. The thickness of masonry walls frequently varied between floors, which caused mass irregularities. Openings in the front wall of the buildings were not symmetrical in the vertical direction, which caused in-plane discontinuity. There were also out-of-plane offset irregularities due to the cross wall on the ground floor. These buildings were irregular in many aspects and were constructed without seismic considerations. This type of construction is more susceptible to earthquakes as a result of these irregularities. In this study, a thorough examination of a typical building was conducted using construction information obtained following the 2015 Gorkha earthquake. The database for each structural typology was prepared with an emphasis on construction practice to enhance the seismic design. The use of mud/cement mortar was extremely sparse, and the use of timber bands at various heights along the height of the masonry wall and an inappropriate connection between the wall and the roof were also negligible. The three main community-learned improvements following damage were the replacement of the gable wall with a metal sheet, the reduction of individual stone masonry homes to one story, and lighter construction on the upper stories of hotel buildings. Based on regional building techniques, non-linear finite models for typical and enhanced buildings were simulated. Due to the irregular stone units, construction variability, and constrained linear behavior, stone masonry with and without mud mortar presents difficulties in conducting a detailed numerical analysis. The development of these structures using mud/cement mortar and other regional materials, with careful attention to detail, was found to have significant potential as a seismically resilient building form.
1 Introduction
The Himalayan region of Nepal is a highly seismically vulnerable region due to the ongoing neo-tectonic activities, i.e., the subduction of the Indian and Eurasian tectonic plates. A number of large earthquakes have damaged many masonry buildings in this region. A 7.6 magnitude earthquake, as recorded by Nepal’s National Seismological Centre (NSC), struck Barpak in the historic district of Gorkha, Himalayas. This catastrophic earthquake was followed by more than 300 aftershocks greater than a magnitude of 4.0 (as of 7 June 2015). Four aftershocks were greater than a magnitude of 6.0, including one measuring 6.8 that struck 17 days after the first occurrence with an epicenter near Mount Everest in the Himalayan region (National Planning Commission, 2015). Unreinforced masonry (URM) has been the principal construction material for buildings in the Nepalese Himalayas for a long time and is constructed with or without mud mortar depending upon the region of the Himalayas (Khadka, 2013). Due to economic constraints, the replacement of stone masonry is not possible in rural Nepal, and therefore, life safety level seismic measures are immediately needed as 38% of the total building stock sustained a DS-5 damage state during the 2015 Gorkha earthquake (Gautam, 2018). Gautam et al. presented damageability functions of stone masonry buildings in different regions of Nepal using damage statistics from past earthquakes. These functions indicate that stone masonry buildings in Nepal are very fragile to seismic action with stone masonry buildings in central and far-western more vulnerable than eastern and western stone masonry buildings (Gautam et al., 2021). Rawal et al. highlighted that the reconstruction of more than 700,000 houses after the 2015 Nepal earthquake was the largest owner-driven housing reconstruction program globally. It is also clearly evident that there has been a major shift in housing typologies in earthquake-affected districts from stone and mud-based masonry to cement-based construction (Rawal et al., 2021). In a study by B. Khadka, the present status of reconstruction and retrofitting works, mechanical properties of common building materials and mud masonry walls, current construction techniques and changes as seen in local building designs compared to past structures, issues and challenges faced during reconstruction, and lessons learned through these activities in 31 earthquake-affected districts were examined. His study showed that 80% of newly constructed mud-masonry houses were comparatively better than older ones (Khadka, 2020). Gautam et al. indicated that among the vernacular construction technologies in Nepal, Rajbanshi houses in Terai and rounded Gurung houses in the hills are resilient in terms of construction technology and their performance paradigm during past events (Gautam et al., 2016).
Schildkamp et al. (2020) reviewed masonry codes worldwide, including Nepal, and compared the main design requirements, such as the overall length, width, and height dimensions of the buildings, minimum and/or maximum thickness and dimensions of the elements and openings, and specifications of the main horizontal and vertical reinforcements. Bothara et al. (2022) highlighted that current building codes for masonry construction are based on creating box effects in masonry buildings but neglect masonry unit shapes and mortar type. Also, the present buildings do not have stiff floors and roofs. Therefore, it was not possible to create a diaphragm action, as expected by the codes. The upper floor masonry is more vulnerable to earthquakes and so lighter construction of the upper floor is best. Lourenço et al. (2011) suggest that the connection between two orthogonal walls and the flexibility of the horizontal diaphragm and its connection to masonry are the main factors that influence seismic capacity. Vasconcelos and Lourenço (2009) reported in their study that two basic failure modes can occur at the level of the unit-mortar interface as follows: tensile failure associated with stresses acting normal to the joints and leading to the separation of the interface, and shear failure corresponding to a sliding mechanism of the units or shear failure of the mortar joint. Additionally, the materials of the bed joints play a central role in the deformation behavior of stone masonry under compressive loading (Vasconcelos and Lourenço, 2009).
Bothara and Brzev (2011) explained the underlying causes for the poor seismic performance of stone masonry buildings and offered techniques to improve it for both new and existing buildings.
Ali et al. (2013) conducted shake table tests on rubble-stone masonry buildings of the Himalayan belt and compared the damage pattern, capacity curves, damage limit states, and response modification factors for general construction and improved construction. Test data indicated that the seismic performance of rubble-stone masonry structures can be significantly improved by incorporating cost-effective features such as vertical members and relatively thin horizontal bands. Wang et al. (2019) investigated six types of retrofitting techniques using locally affordable materials (wood, gabion wires, and tarpaulin) for dry joint flat-stone masonry walls by conducting cyclic in-plane testing. Their study identified four principles of resilient construction as follows: local materials, structurally effectiveness, cost efficiency, and ease of use. Bothara et al. (2018) found that low-strength masonry buildings are environmentally sustainable in the pre-use, use, and post-use stage as construction material is locally available, requires less operational energy, and their demolition is convenient. An extensive experimental investigation illustrated that it is feasible to make low-strength masonry buildings earthquake resilient. Mukai et al. conducted microtremor measurements of a historic masonry house in Bhaktapur, Nepal, after the 2015 Gorkha earthquake, which elucidated the vibration characteristics of non-engineering buildings (Mukai et al., 2022). Cabboi et al. (2017) conducted preliminary ambient vibration tests on a stone masonry tower and examined the damage location by continuously updating a finite element model based on cleaned modal frequencies.
Smoljanović et al. (2013) proposed a combined finite discrete element method for dry stone masonry where non-linearity of the material, including fracture and fragmentation of discrete elements, as well as cyclic behavior during dynamic load, are considered through contact elements, which are implemented within a finite element mesh. Bothara et al. (2018) performed numerical modeling of Nepal’s proposed school building designs using non-linear static finite element models for unreinforced and reinforced stone masonry buildings using LS-DYNA software. The investigation has shown that buttresses were able to efficiently prevent out-of-plane failure of face-loaded walls and thereby increase the building’s seismic capacity by more than 50% when compared to a building without buttresses. Lemos (2019) suggested the use of a discrete element model analysis of masonry structures under intense earthquake loading. He highlighted the need for modal simplification in representation of the block geometry or in constitutive assumptions for obtaining meaningful results with existing data in practical situations. Adhikari and D'Ayala conducted numerical modeling for stone masonry buildings in mud mortar (SMM) for pre-earthquake and post-earthquake scenarios based on an experimental test by Build Change. The results showed that PRE-SMM has very low seismic capacity in both principal directions compared to POST-SMM. Vertical separation cracks and corner failures that trigger short wall collapse are the main failure modes in PRE-SMM buildings, as confirmed by observed damage and the results of numerical analyses (Adhikari and D’Ayala, 2020). Shrestha et al. (2020) proposed a generalized analysis scheme, and a simplified frame model and results from SFM show strong agreement with the experimental result for base shear and approximately 5% error in the case of ultimate drift. The simplified model can be used in the performance-based design of masonry buildings and for the assessment of existing masonry buildings and for low-rise masonry buildings. Rios et al. (2021) conducted a discrete modeling approach based on a limit analysis, which is capable of reproducing sliding mechanisms, and highlighted that the method is applicable for masonry structures where sliding cannot occur, there is no tensile strength, and infinite compressive strength and failure can occur under small displacement. Rupakhety et al. considered “Bal Mandir,” which is an aggregate masonry complex located in Kathmandu, Nepal, for system identification using ambient vibration records taken after the Gorkha earthquake that significantly damaged the structure. For comparison with ambient vibration, the finite element analysis was conducted and both showed a noticeable change in the dynamic properties of the building (Rupakhety et al., 2022). Khansefid et al. (2022) conducted an eigenvalue and pushover analysis to assess the model properties, crack propagation patterns, and crack widths based on the base shear of the buildings. Acharya et al. (2023) validated a modeling strategy adopted for the generation of a full-scale confined masonry building model with an equivalent prototypical RC building in Nepal through non-linear pushover and fragility analysis.
In the Thame Valley, which is located in the Khumbu area of the Himalayan region, 93% of low-strength stone masonry houses, including tourist structures, were damaged by the earthquake (Sherpa, 2015). Reconstruction started immediately after the earthquake by the community itself. The typical and traditional construction practice was not adopted and more modern housing was constructed in a difficult and more costly way. Seven years after the earthquake, the authors conducted a comprehensive structural survey in the remote villages of Thame, Thameteng, and Hilajung. The state of practice and state of knowledge on the seismic design of such irregular buildings are limited to the community. Additionally, they were unaware of the guidelines for the construction of such buildings.
This article begins with a reflection on the Sherpa community who live in the region and the culture regarding local building construction practices. The Sherpa traditional construction technology and traditional building layout are then discussed. The earthquake damage and building failure patterns due to Mw = 7.6 and Mw = 6.8 in 2015 in the Thame Valley are discussed. In August 2022, the authors conducted a rapid visual screening (RVS) in the Thame Valley of reconstructed buildings for potential seismic damage. Improvements in current practices in construction technology practices and their resiliency were evaluated. Based on the survey results, the typical structural layout of a Sherpa house is prepared and associated building irregularities are presented. Based on functional and Sherpa cultural practices, the enhancement of a typical Sherpa house was conducted according to the current code of practice for Nepal. Finally, a non-linear numerical model for current irregular stone masonry building (C_ISMB) and enhanced irregular stone masonry (E_ISMB) was prepared in DIANA. A comparison between buildings was evaluated through lateral load capacity, crack development, and failure patterns.
2 Traditional construction technology and seismic damage in the Thame Valley
2.1 Study area
The Khumbu or Everest region lies between 86˚31′-86˚58′ East Longitude and 27 ˚47′-28° 71′ North Latitude within the district of Solukhumbu in northeastern Nepal. The elevation in Khumbu varies from 3,300 m to 8,848 m, which is the summit of Mount Everest and the highest place on the Earth. Khumbu is a place of world importance since Sagarmatha National Park, a world heritage site, lies here. The region is diverse in its ecosystems and holds a rich history and culture that needs to be protected and preserved (Bruce, 2020). This study was conducted in the Thame Valley (pronounced “Ta-may”) in the Khumbu region of the highlands of the Himalayas, as shown in Figure 1. The Thame Valley lies 20 miles west of Mount Everest in the upper Khumbu region of Nepal. It is the heart of Sherpa country, which encompasses a string of high-altitude villages stretching from the market town of Namche to the border with Tibet (Rebuild Thame, 2015). Specifically, three different remote Himalayan villages, Thame, Thameteng, and Hilajung, were chosen for this survey. Traditionally, these villages were part of the salt trading route that existed between Tibet, Nepal, and India.
2.2 Sherpa community and culture
The Sherpas in Khumbu have a unique way of life with a special social and economic structure that is necessary to understand from a sustainability context. Their distinctive way of life is well adapted to their environmental conditions and the high altitude (Bruce, 2020). Although the population of Khumbu represents only a fraction of the total number of Sherpas, it is a group of vital importance for understanding the characteristic features of the Sherpa way of life (Sestini and Enzo, 1978). Sherpas’ distinctive cultural and religious practices exhibit their creativity in local land use practices and resource management abilities, which are primarily motivated by locally established knowledge systems and the peculiarities of the local environment (Ortner, 1999). Anyone traveling through the high valleys of the area will immediately notice the Sherpas’ attitudes toward their religion, which is embodied in their profound Lamaist beliefs and is another distinguishing aspect of the Sherpa way of life in Khumbu. The chorten, mani-walls, and prayer flags flying on Sherpa homes are clear indications that religion is an important part of their daily lives. To better understand the architecture of Khumbu, one must look to the Sherpas that inhabit the region and consider their great inner strength sustained by profound religious beliefs. Only then is it clear how they have succeeded in adjusting themselves to the rugged countryside of Khumbu (Sestini and Enzo, 1978).
2.3 Traditional construction technology
In the Sherpa villages, most buildings were traditionally built in small groups with all windows and doors facing the same direction, i.e., south, to gain heat from the Sun. The typical Sherpa house is an elongated shape and usually situated parallel to the slope of the hill. The ground floor can be built on the slope of the land behind. The back of the house usually has little to no windows or doors. This is because it is typically built into the slope and because there is no Sun exposure. The buildings consist of an outer protective wall that is constructed of locally available stone and can be up to 1 m thick. This is usually unshaped stone - rubble, plastered with yak dung and a mud mixture. An internal timber frame sits inside the stone and a wooden ceiling is supported by timber beams. Horizontal timber beams can also be built into the outer stone walls to increase the resistance to earthquakes. Although this is not commonly seen in older and more simple rubble-built houses, the traditional technique is apparent in many of the buildings of dressed stone, and these houses have a pitched roof to shed snow and rain in the summer. Additionally, they would historically be roofed with slate or fir shakes. The foundations and floors are built on locally available stone, and houses could be either be single or two story. In the case of a two-story house, the ground floor would often house livestock and be used for storage (such as grain and fuel wood), while the living area would be located above and be accessed by a wooden staircase. The choice and arrangement of furnishings in the living room are such as to afford the greatest possible comfort for the family. There is a living room with a fire pit, drawers and shelves for utensils, bedding, and goods, as well as a prayer room with Buddhist idols. In addition to the living room, there is a private place of worship (Sestini and Enzo, 1978; Sherpa, 2008; “Peoples of Nepal: Sherpa, 2007).
The Khumbu region is rich in mud and stone, and stones and wood are used to build traditional structures (all types of buildings use dry stone that is hand-tooled). The stonework is either held by a soft, earth-like clay mixture or is dry, but these walls lack strength. Additionally, stone is frequently used for paving and roofing. Timber is a crucial but limited resource. Above the tree line, high-alpine land makes up the majority of Khumbu, while the region’s southern, lower-altitude border is where the few remaining forests are located. The main tree species that grow in the Khumbu are silver fir and birch. The limited timber in the region means it is generally flown in or transported from further down the valley where the land is more fertile. Traditional Sherpa houses require timber for beams, floors, rafters, window frames, doors, and furniture; it is not easy to find, and it is expensive to build with timber (Bruce, 2020). The design of newly built Sherpa houses in Khumbu has drastically changed over time, particularly since the rise of Everest tourism after the 1970s (Nepal, 2002). For instance, the number of large houses with more rooms and modern facilities, such as an attached toilet/bathroom and heating systems, has increased (Spoon, 2008; Aubriot et al., 2019; Nepal, 2015). Although this is mostly the case of houses in the villages along the Everest trail, many houses toward off-route locations from this main trail have also been vibrantly renovated. Fisher (1990), Sherpa (2014), and Bhattarai (2021), in their studies, asked about the type of house the participants lived in and the building materials used. None of the participants lived in traditional thatched roof houses with wooden, stone, or mud walls. During the course of our study, we only found one such house in Hilajung, which is in the most remote part of our study area. This building’s nature is purely traditional and replicates traditional Sherpa culture and lifestyle. The heavy slate roofing and supporting inner timber frames had completely separated from the outer thick random rubble stone masonry wall, which is the same as that observed by Sestini and Enzo (1978). The length and width of the building were 15.5 m and 5.8 m, respectively (Figure 2). The thickness of the wall at the ground floor and first floor was 0.635 m. The thick timber floors were supported by timber joists and rested on vertical timber posts.
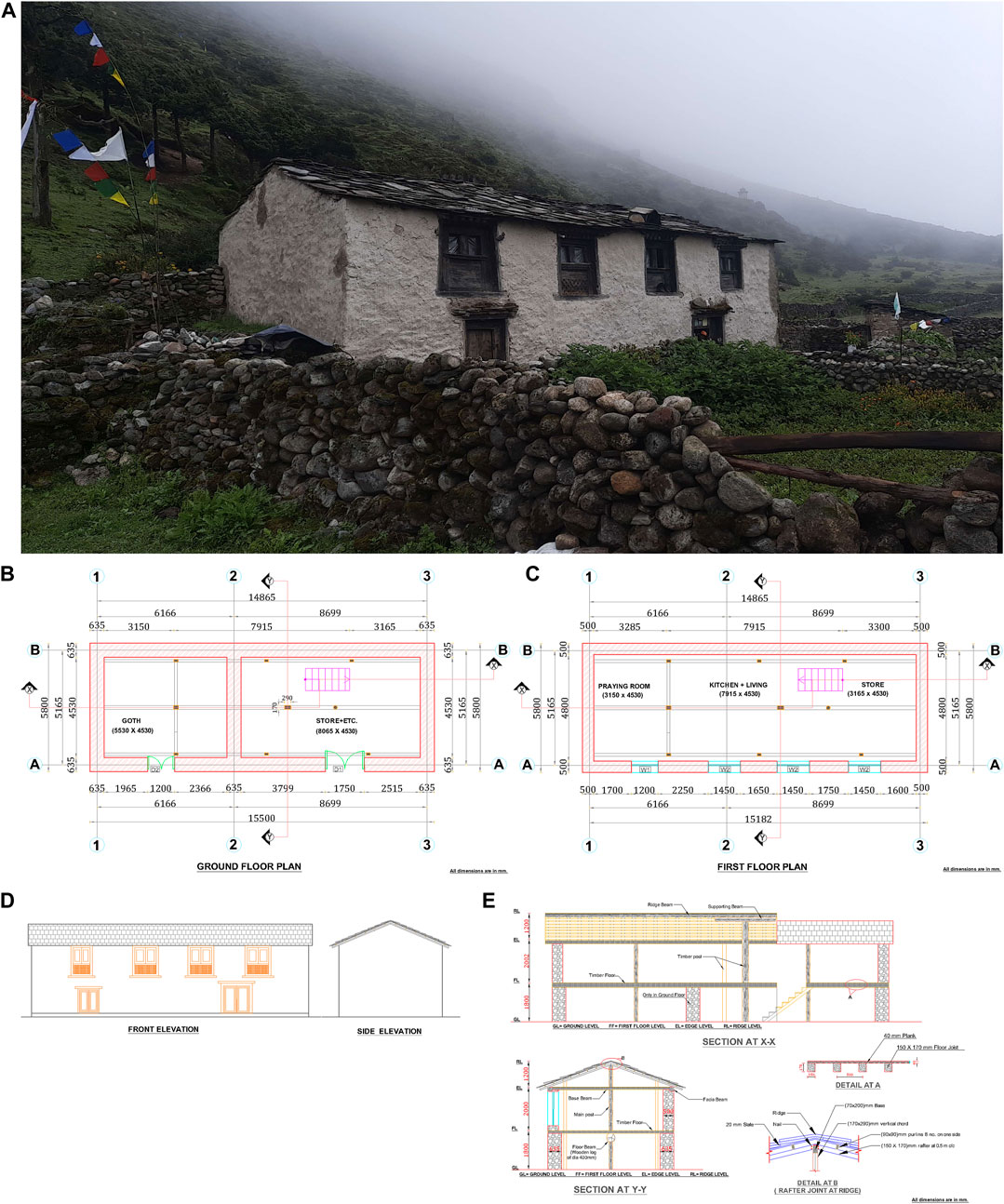
FIGURE 2. (A) Traditional Sherpa home. (B) Ground floor plan. (C) First floor plan. (D) Elevation. (E) Section details (all dimensions are in mm).
2.4 Earthquake damage in the Thame Valley
The epicenter of the 7.6 earthquake was located approximately 200 km west of the Everest region, and for the 6.8 earthquake, it was 80 km west of the Everest region (Miyamoto, 2015). In the Thame Valley, which is located in the Everest region, 93% of traditional houses and tourist structures were damaged by the earthquake; 66% were completely damaged (unusable without major reconstruction) and 27% were partially damaged (safe to use with repairs) (Sherpa, 2015). In this region, most of the buildings were low-strength stone masonry. Despite the documentation of losses on the ground, governmental reports of earthquake-affected zones on a national level appeared to show Solukhumbu as ‘unaffected’ or ‘less affected’ (Sherpa, 2017).
A rapid visual assessment by Miyamoto (2015) after the 2015 Gorkha earthquake in the Everest region of Nepal for 15 settlements, which excludes our study area in the Thame Valley but the construction practices are nearly the same, concluded that the majority of structures are likely repairable. Uncut stone with mortar and cut rectangular block stone with or without cement mortar were typical building materials. Cement was primarily used in newer construction, in contrast with older construction that frequently used mud as mortar. In earthquakes, newer construction that used cement as mortar typically fared better than older construction that used mud as mortar and uncut stone; these older structures often suffered significant damage and even collapsed. Overall, the majority of the structures are likely repairable. There is a difference between typical residential housing used by village residents and typical accommodation structures despite the fact that many of the buildings have multiple occupancy categories serving as homes, tourist housing, and tea houses. Only locally accessible materials, such as rock and mud that performed poorly as previously mentioned, are typically used to construct residential structures used by village residents. Generally, the main lodging structures are better built and frequently use earthquake-resistant materials like cement, cut rock, or lightweight wood studs (Miyamoto, 2015). The Gorkha earthquake severely damaged the Sherpa buildings in the Thame Valley. The sporadic nature of the structural damage in many Sherpa villages reflects the age and style of construction (dry stacked fieldstone versus the use of mud or cement mortar, the presence of wire mesh or gabion bands/spacers within stone walls, etc.), and the micro-ground conditions beneath the buildings. The Gorkha earthquake frequently caused “X cracking” of external structural walls with cracks often coming from the corners of the window and door jamb. Along with the toppling of stones from the tops of walls and from triangle-shaped spaces directly beneath roofs, large, through-going subvertical cracks were also frequent (Lageson, 2016).
One of the authors of this paper inspected the Thame Valley immediately after the 2015 Gorkha earthquake to assess damaged community buildings. Out-of-plane failure of the long and thick stone masonry wall was the most common damage due to the lack of cross walls and the absence of seismic bands. Figure 3 illustrates the different damage in stone masonry buildings as follows: a) collapse of the roof gable wall, b) collapse and detachment of the outer wall of the buildings with a timber frame, c) masonry walls that separated from the windows and corners, d) bulging of a masonry wall and delamination, e) poor roof and wall connection, f) failure of the longer wall, g) complete collapse of the house, and h) both long and short wall failure. These results indicated that there was a lack of lateral load resistance mechanism and no appropriate roof-to-wall connection during building construction. There were no cross walls supporting the longer wall and seismic bands in the different levels of the walls, and out-of-plane failure was dominant in stone masonry walls. The construction practice of using dry stone stacks in masonry walls and pointing and plastering from outside does not contribute to masonry joint strength. Therefore, in most cases, the failure arises from individual masonry unit failure.
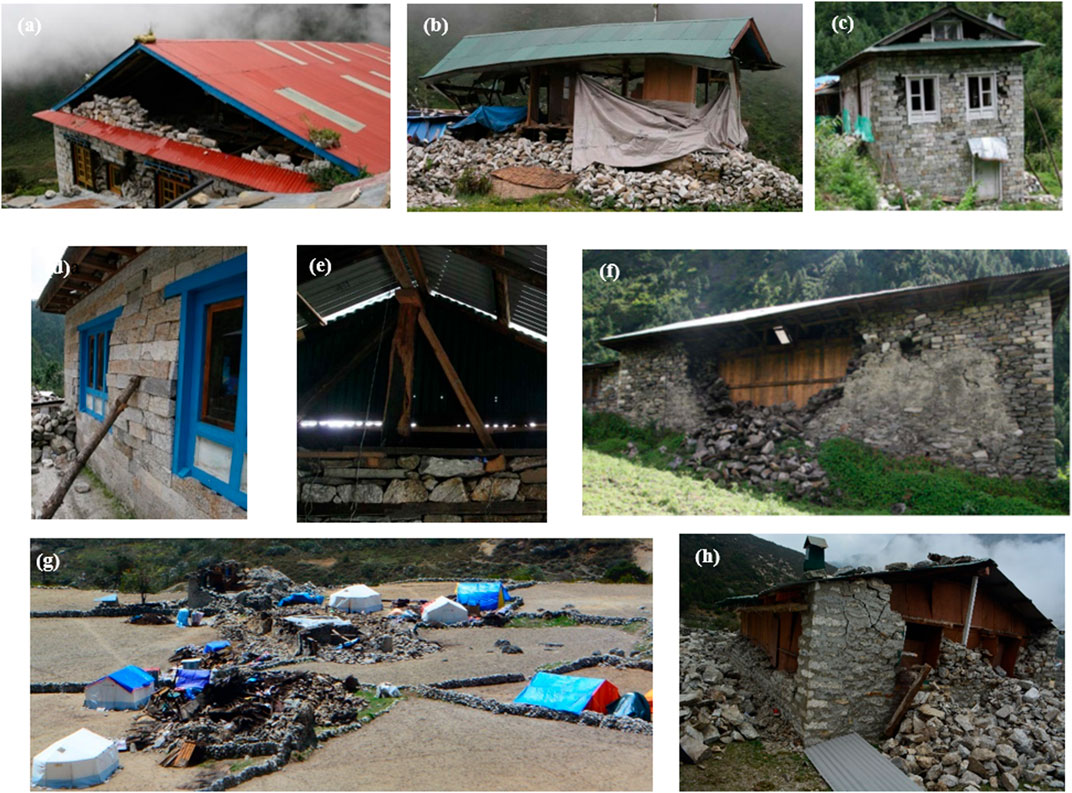
FIGURE 3. Damage in stone masonry buildings: (A) collapsed roof gable wall, (B) collapse and detachment of the outer wall of the buildings with wood frames, (C) masonry walls separating from windows and corners, (D) bulging of the masonry wall and delamination, (E) poor roof and wall connection, (F) failure of the longer wall, (G) complete collapse of the sherpa houses, and (H) failure of both the long and short walls.
3 A post-earthquake reconstruction assessment for possible resilient enhancement
Reconstruction started immediately after the 2015 earthquake by the community with help from local community organizations. Later, the Nepalese government also supported the reconstruction of private buildings, health facilities, and academic institutions. Most of the houses were rebuilt in the same location with or without considering the seismic resistant design. Traditional construction practices were not adopted due to hasty reconstruction processes. Khumbu is changing and more modern housing is being constructed, which does not reflect the Sherpa culture (Nyaupane et al., 2014). The reconstruction assessment survey was conducted in August 2022, and more than 90% of the buildings in each settlement in the Thame Valley were surveyed.
3.1 Assessment methodology
The survey was conducted through the rapid visual screening (RVS) of buildings for potential seismic damage according to “FEMA P-155 (2015) and “FEMA P-154 (2015). Each building dataset was collected using survey forms prepared on the Survey123 Mobile App (Figure 4) and uploaded to the ArcGIS Survey123 web. The survey consisted of major questions that assessed the seismic detailing and the reconstruction of the community such as i) functionality of buildings, ii) types of buildings, iii) the number of stories, iv) seismic detailing, v) floor/roof types, vi) stone masonry types, and vii) use of mortar. The three different settlements of the Thame Valley, including Thame, Hilajung, and Themteng villages, were surveyed, and the red points on the image (Figure 4) show the surveyed buildings. In seismic detail, the survey focused on a traditional approach and current common practices. Seismic bands included timber and reinforced concrete bands, reduced gable weight, wire mesh on exterior walls, improved connections between floor/wall or roof/wall, and cornerstone stitching on exterior walls. Similarly, for the type of building, the survey focused on stone masonry with or without mortar, timber structure, and a hybrid (stone/timber).
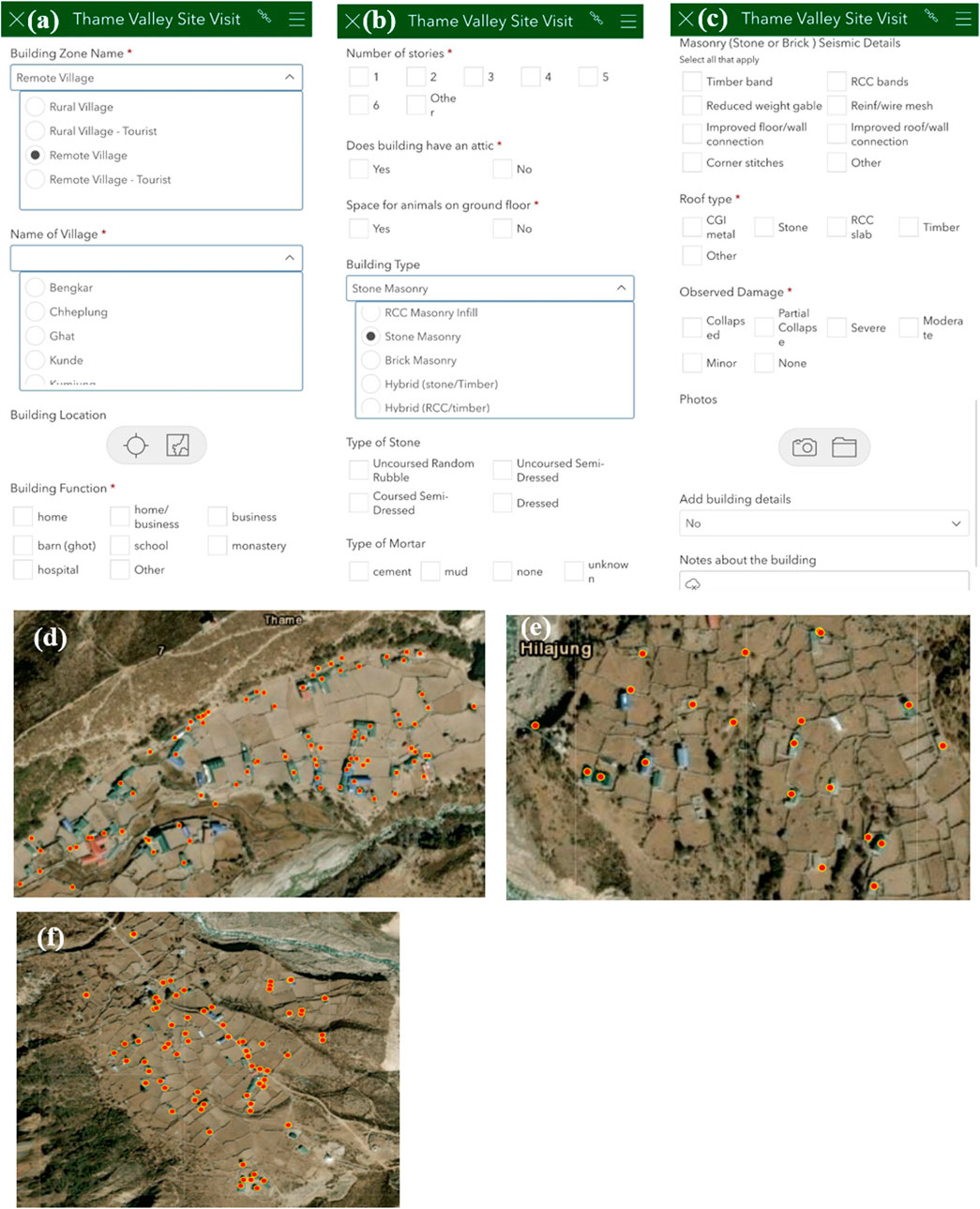
FIGURE 4. (A–C) Building survey using ArcGIS Survey123: (D) Thame settlement, (E) Hilajung settlement, and (F) Thameteng settlement.
The survey focused mainly on masonry, and the type of stone used was considered a good proxy for the quality of the construction. It was further determined that a fully dressed 3D rectangular stone does not exist in this region. Therefore, the dressed stone working definition becomes clear uniform courses with little or no room for pointing between stones or finely hand/machine tooled. Our team spoke with many owners and builders in a variety of communities, and most indicated that mortar of any type was rare in these regions. Some owners/builders commented that cement mortar was present, but after further discussion, it was clear that cement mortar was used sparingly (due to cost) in very thin layers on every other or every third course of stone. From a structural perspective, a thin layer of cement mortar on every alternate or third course is equivalent to no mortar. Furthermore, some owners/builders did not fully understand what we meant by mortar and considered pointing or plastering as mortar. Corner tie stones are relatively long stone blocks laid in the corner along the long and short wall. For the classification of the function of buildings, if there is a hoarding board of any kind of business, then it was considered as business and this permits easy accounting for other types of functional classification. All of the load-bearing structures were stone masonry as there was not a single house of brick masonry and framed structure classified based on the material used in the framing elements. If there was a band present in the lintel of buildings, then this was considered a building with bands. If there are other lighter materials, such as wood and CGI than heavy stone masonry, then this was considered a reduced weight gable. Because there was a negligible stone masonry building with improved floor/roof-to-wall connection, no measures were taken to restrain wall movement; however, for RCC buildings with monolithic slab construction, we considered this as an improved floor-to-wall connection. The number of stories in a building was assessed by the number of layers of doors and windows in the front façade.
3.2 Survey results
During the fieldwork at Thame Valley, 223 buildings were surveyed, in particular, for the enhancement of seismic detailing after the recent earthquake at the three main villages. Specifically, Thame, Thameteng, and Hillajung were surveyed as they are the main villages with high numbers of settlements compared to other villages. Of the 223 buildings, 126 (57%) from Thame, 73 (33%) from Themeteng, and 24 (11%) from Hillajung were surveyed.
From the survey, the main construction material in the region was determined to be stone masonry. A total of 92% of buildings were stone masonry and 5% were timber structures. The stone masonry was classified into uncoursed random rubble (43%), uncoursed semi-dressed (25%), and coursed semi-dressed (32%). Since the 2015 Gorkha earthquake, new types of construction materials have been introduced. Reinforced concrete frame structures (1.5%) and steel frame structures with pre-fabricated infill (1.5%) are used for tourist hotels. After the earthquake, it was determined that the building usage pattern changed. This pattern used separate buildings for animals, and due to migration to nearby cities and abroad, people are not used to having animals. Furthermore, the buildings have been divided into eight categories based on their functions. As shown in Figure 5, 73% of the buildings are used for both residential and small home businesses, and 11% of buildings are for animals as barns outside and nearby the home. The rest of the buildings were schools (1.8%), monasteries (3.6%), primary health centers (<1%), and public utilities (10.31%) including community buildings, electrical stations, and others.
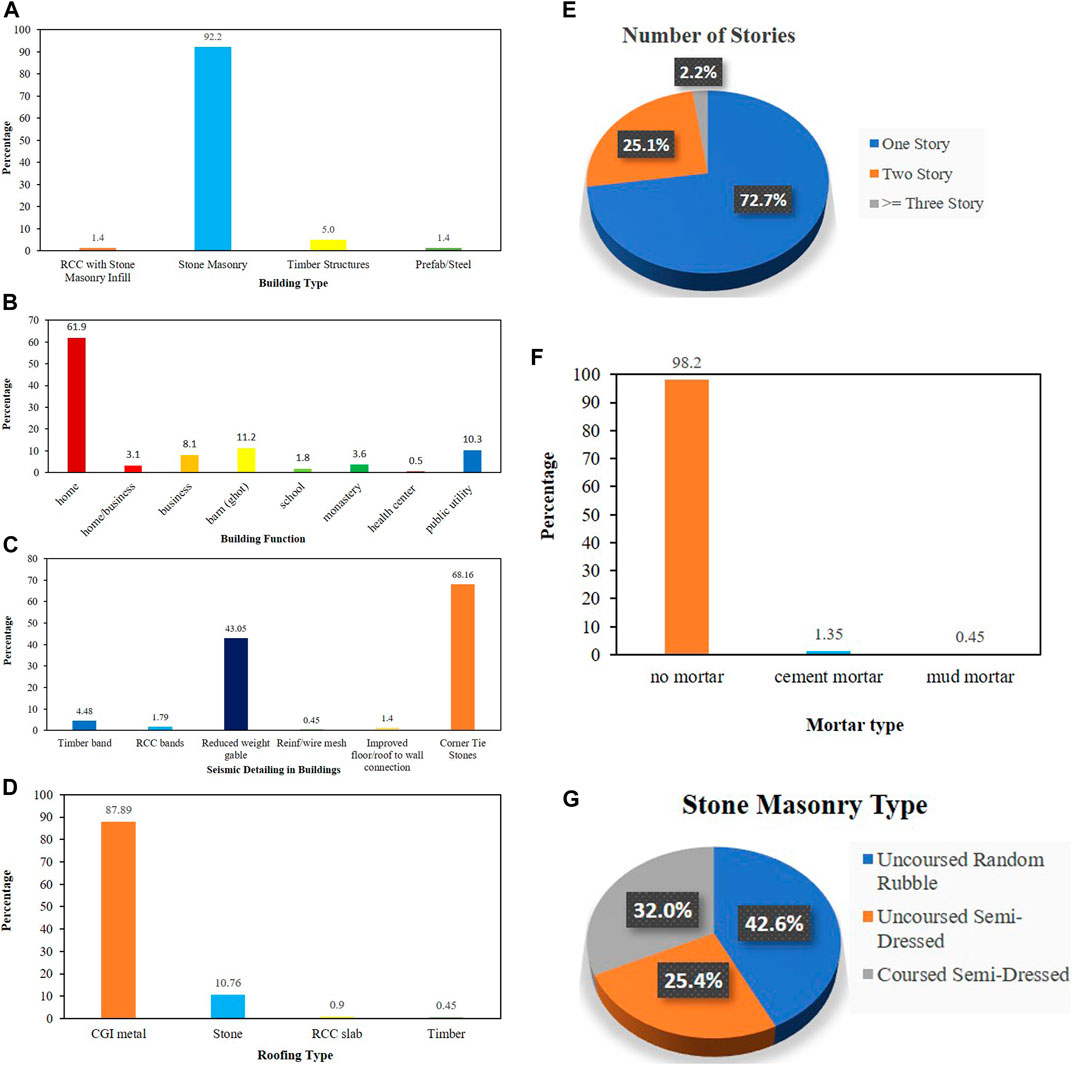
FIGURE 5. Survey results in terms of (A) building types, (B) building function, (C) seismic details, (D) roofing details, (E) number of stories, (F) mortar type, and (G) stone masonry type.
In our survey, we found that in traditional buildings, inhabitants complete every activity inside the home, including having space for animals, storing wood, and others on the ground floor, while family activities occur on the upper floor. However, after the earthquake, they built separate buildings for animal and storage purposes near the house. For this reason, they built one-story buildings for residential purposes during reconstruction. A total of 73% of buildings are one-story residential and small business homes, 25% are two-story, and the remaining are three-story, especially monasteries. In the highlands of the Himalayas, the traditional use of mud mortar was very rare for residential buildings, and thick dry masonry walls were used. A total of 98% of the buildings were reconstructed without mortar, less than 2% of the buildings used cement mortar (i.e., schools and monasteries), and less than 1% of the buildings used mud mortar.
This survey also focused on the roof status of buildings. Approximately 88% of buildings have CGI metal sheet roofing, while 11% of the buildings still have a slate roof. Less than 1% of roofs are reinforced concrete in monasteries. In seismic detailing, they practiced using corner tie stones in every corner of the building. According to our survey, less than 5% and 2% of buildings have timber and reinforced concrete bands, respectively. In traditional buildings, there is no evidence of a roof-to-floor and floor-to-wall connection. The total roof rested on the timber frame and the floor just rests on the wall. They do not have any practices for roof/floor-to-wall connections. From a visual inspection during the survey, it was found that in modern buildings, they have connected the roof to the wall by simply resting on it and less than 2% of buildings have improved floor/roof-to-wall connections, particularly in school and public buildings. Corner tie stones (68%) are still used at the corner of buildings. In traditional practice, they used stone masonry walls for the roof gable wall, and after the earthquake, most of the gable walls collapsed. A total of 43% of the buildings replaced the stone masonry gable wall with lightweight metal CGI sheets. Some public buildings, like schools and health centers, were found to use a minimum practice of seismic detailing after reconstruction to enhance the seismic performance of buildings.
3.3 Current construction practices and resiliency
Norris et al. (2008) define resilience as “a set of processes linking a set of adaptive capacities to a positive trajectory of functioning and adaptation after a disturbance.” In a study by Sherpa (2017), the community of the Khumbu region was very aware of the devastation and post-earthquake recovery needs after the earthquake. Specifically, in his internal discussions around Khumbu houses, he found that the extent of the devastation was high and there is a wide range of individual needs for resilient reconstruction. Figure 6 provides an overview of the construction methods used in the Thame Valley, following the earthquake. After the authors conducted a survey of the reconstruction, it was found that the lessons learned included replacement of the roof gable wall with a metal sheet and many individual homes downsized their building to one story. For hotels and lodges, they tried to maintain two stories with lighter construction like timber and steel framing with lighter material covering the upper story. Most community buildings were recovered with very limited seismic detail as has been the practice for years. It was found that the state of practice and state of knowledge on the seismic design of such irregular masonry buildings are very limited in the community even though they are highly aware of disasters. Due to a lack of community knowledge and supporting guidelines about proper utilization of local materials, the people are forced to use non-local materials like cement, steel, prefab materials, etc., in these rural areas and because the non-local materials are not affordable. Because the region has a cold climate, the walls are covered with timber planks from inside for insulation, which helps in life safety by creating protection from outward failure of a masonry wall, and at the same time, the timber framing supports the heavy roof. Thus, it prevents the immediate collapse of the building. Because an opening is provided on the front side only with a very small spandrel height and this part is heavily stressed, it is deflected from the dead load above through the protection of heavy timber framing in doors and windows. At the corners, tie stones are provided that replace the corner stitching. The walls of the building have heavier sections than required, which makes the building rigid during an earthquake. The local knowledge of these things was developed by experiencing a damage pattern in the past earthquake rather than by following codes and technical guidance from government authorities. Most of the two-story buildings were damaged during the 2015 Gorkha earthquake (Sherpa, 2015), and so the local community modified their traditional construction style. Now, 70% of the individual homes are one-story and have separate buildings for animals and storage facilities. These newly constructed one-story houses attempt to maintain the layout of traditional ones. The use of modern materials requires difficult and costly transport but has mostly replaced local material; therefore, there is a big question about their resiliency.
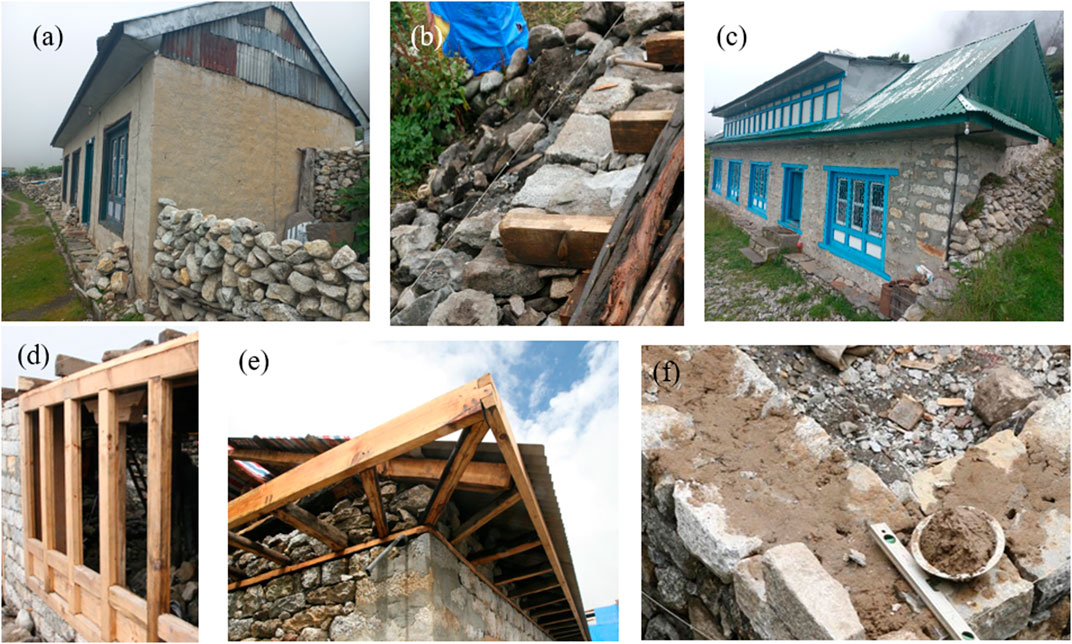
FIGURE 6. Current construction practices in the Thame Valley include (A) a reduced weight gable, (B) laying of the floor joist in the wall, (C) a light upper story, (D) resting of the floor joist on the window frame, (E) a roof-to-wall connection, and (F) a thin layer of mud mortar in the masonry.
3.4 Typical Sherpa house and related irregularities
The seismic safety of the houses after reconstruction in the Thame Valley needs to be checked for further construction recommendations in this area since the houses are constructed to support the daily life and culture of the Sherpa people. After a field survey, we found that individual homes have a similar layout. From the results (Figure 5), it was concluded that individual homes of one story with random rubble dry stone masonry and a reduced weight gable and CGI roof covering are the most commonly practiced construction technology in the Thame Valley. Based on this conclusion, three Sherpa houses were measured and found that they try to maintain the same dimension and aspect ratio as traditional houses with limited cross walls and openings in the front façade only. The layout of a typical Sherpa home of the study area is shown in Figure 7.
Perfect regularity is an idealization that very rarely materializes, and irregularity itself is inherently a nebulous concept. The majority of codes make an effort to define the term “regularity” by considering issues with the distribution of mass, stiffness, and strength in the building both in plan and in elevation. Code specifications for irregular structures, such as (NBC 105 and Nepal National Building Code, 2020), need to be improved because they do not offer precise instructions for how to conduct seismic analysis on these structures. Because of this, the issue of seismic evaluation of irregular structures remains up for debate, and fundamental questions need to be further investigated (Stefano and Mariani, 2014). A building is said to have a horizontal irregularity if the plan of the structure suddenly changes. A building is said to have a vertical irregularity if its shape changes with its height (such as setbacks or overhangs) or if significant load-bearing walls are missing. Being irregular is not good for a building because regular structures fare better in earthquakes (Bothara and Brzev, 2011). Stones of irregular shape, such as small or medium-sized river stones, smooth stone boulders with rounded edges, or stones from a quarry, are used for construction. The walls are made of two wythes, and the space between them is filled with dirt, pebbles, and other debris. Poor transverse response is seen in irregular masonry structures because of the multiple leaf structure (Valluzzi et al., 2001; Binda et al., 2006). Furthermore, it was determined that the Thame Valley does not contain a fully dressed 3D rectangular stone.
To assess the influence of irregularities on non-linear response of walls with openings and variations in their seismic capacity parameters, a series of global and partial irregularity indices were defined. A global irregularity index indicates whether a perforated wall is regular (i = 0) or not (0 < i ⩽ 1) and is calculated using i) and ii), where H is the opening height and L is the opening length (Parisi and Augenti, 2013).
I. Horizontal irregularity (iH = 0.167):
II. Vertical irregularity (iv = 0.094):
A Sherpa house has significant irregularity due to the culture and the way of living in the high Himalayas. In the front façade, a typically unsupported long wall has a horizontal irregularity index up to 0.2 and a vertical irregularity index up to 0.1. The irregularity in the stone unit makes the building behavior more non-linear and it is very difficult to understand this by numerical simulations.
3.5 Enhancement of a Sherpa house
A current construction practice comparison was made with (NBC 203 and Nepal National Building Code, 2015) and the International Association for Earthquake Engineering (IAEE), Japan (IAEE, 2004). A major recommendation for such buildings is highlighted in Table 1 and drawings were prepared after, as in Figure 8. The E_ISMB building is provided with timber bands at the plinth, sill, lintel, and eave levels, which help to stabilize walls under face-loading and enhance the shear capacity of walls when subjected to in-plane loading. Buttresses are also provided in walls to enhance the performance of load in the out-of-plane. The use of containment mesh to both faces of the walls tied together by cross ties, stitches in various levels, connection of roof to bands through vertical wires are also recommended.
4 Numerical modeling
To understand the seismic performance of current stone masonry buildings and the effectiveness of the proposed interventions, 3D finite element-based numerical models were prepared (DIANA FEA V10.3, 2019).
In this study, two different numerical models were prepared for i) the current-irregular stone masonry building (C-ISMB) (Figure 7) and ii) the proposed Sherpa enhanced-irregular stone masonry building (E-ISMB) (Figure 8). This was conducted for the purpose of comparison between unreinforced masonry to the enhanced one with buttresses, bands, and a roof-to-wall connection. The other proposed enhancement techniques, such as containment mesh with cross ties, stitches in various levels, the connection of the roof to bands through vertical wires, corner tie stones, and through stones, are not included in the numerical modeling. The snaps of the numerical model prepared for drawings of Figure 7 and Figure 8 are shown in Figure 9. However, it should be considered that the focus of numerical modeling was to feel the improvement in performance of the Sherpa house after interventions, rather than to use it as a verification method. It should also be noted that considering the limitations to the numerical modeling for this type of stone masonry, which has large variability in material properties, boundary conditions, the effect of openings, a lack of continuum and cracking, that modeling alone cannot be fully relied upon. The local failure mode such as the cracking of individual stones, displacement of mortar joints, loosening of stones, and bulging of walls greatly depends on good workmanship and is very difficult to characterize through numerical analysis. Therefore, numerical analysis only attempts to focus on a global failure mode of masonry.
The C-ISMB is a one-story Sherpa house that only has an opening on the front side and a solid cross wall on the first floor to separate a storage room. The floor beam is supported in the vertical post, and the floor joist rests directly in the dry masonry wall. For support of the ridge beam, no vertical post is extended from the ground floor, and the rafter rests directly on the masonry wall. Both heavy masonry gable walls and the roof are replaced with CGI sheets to reduce the weight of the building.
In the E-ISMB, the two cross walls with door openings are provided to separate the praying room and storeroom from the open kitchen/living area in the middle of the building. The two buttresses are of the same thickness as the wall provided on either side of the long wall, and one on each short wall and cross wall is used to maintain an unsupported wall length of 3 m (according to Table 1). The other features of E_ISMB are kept the same as C_ISMB. These buildings were investigated for their performance in terms of lateral load capacity, induced stress, and induced failure patterns.
Due to a lack of measured data and physical tests for such buildings, numerical analysis is the best option to assess such irregular buildings. In such cases, the numerical computation can be categorized as macro and micro modeling. For the macro-model, the wall is discretized into finite elements and the masonry is discretized as a homogenous isotropic continuous medium with no particular attention given to the position of the mortar layers. Thus, the properties of the masonry assemblage as a whole, i.e., the properties of the masonry prism, are used instead of the different properties of each individual material component (Laurenco et al., 1995; Wang et al., 1997; Hamp et al., 2022). This requires less computational effort and is commonly used for the design and analysis of complex and large-scale masonry structures. The global response of masonry can be well predicted even without the inclusion of a local interaction between the masonry components (Laurenco et al., 1995; Hamp et al., 2022).
In this study, a three-dimensional finite element based on a macro-modeling approach was developed in commercial software (“DIANA FEA V10.3” 2019) to analyze the seismic response of the Sherpa house. The timber elements were modeled with linear frame elements, while the masonry walls were modeled with non-linear behavior. The non-linear behavior of such materials is able to reproduce the main failure mechanism of masonry walls. This numerical approach has been extensively used to simulate historic/traditional URM structures (Dais et al., 2021) The non-linear material behavior of the masonry walls was used through Quadratic shell elements (CQ40 S) (Bothara et al., 2018). The location, shape, element class, and geometry of building elements used in DIANA are shown in Table 2.
To model regular plane stress (membrane) and curved shell elements (masonry), the engineering masonry model with smeared failure is considered, and this model is a total-strain-based continuum model. Compared with the total strain crack model, the engineering masonry model describes the unloading behavior more realistically assuming a linear unloading for compressive stresses with initial elastic stiffness in the engineering masonry, and modal tensile cracking is assessed either in the directions normal to the bed-joints or to the head-joints. In addition, a secant non-linear unloading and reloading behavior is assumed. Also, compressive crushing is assessed in the directions normal to the bed-joint and the head-joint; and a non-linear non-secant unloading and reloading behavior is considered. The cracking, crushing, and shearing behavior of the engineering masonry model is explained in Figure 10. For shear stress, a standard Coulomb friction failure criterion is used based on the normal stress to the horizontal joint (DIANA FEA and TU, 2017) The fracture energy in compression (Gc), tension (Gt), and shear (Gs) is determined by the ductility index through equation iii), iv), and v). The ductility index dc = 1.6 mm, dt = 0.029 mm, and ds = 0.093 mm were selected for the fracture energy in compression, tension, and shear, respectively. The cracking type of the masonry is a head joint failure with an angle between a stepped diagonal crack, and the diagonal stepped cracks in wide walls can be properly described with this criterion and bed-joint diagonal staircase cracks as 42° with a crack bandwidth specification, as defined by Rots (Angelillo, Lourenço, and Milani, 2014).
Timber roof rafters and purlins were modeled by adopting a linear elastic truss and beam elements (L13BE and L2TRU). The connection between the roof truss ends and masonry was obtained using linear-elastic-point-interference elements (N6IF) with a stiffness of k = 5000 N/mm. The corrugated roof panels were reproduced using linear elastic shell elements with a non-linear shape function (CT30 S). A free interface was established between the adjacent corrugated roof mesh sets (each spanning from one roof truss to the next) to avoid excessive in-plane stiffening (Bothara et al., 2018).
Due to the very limited experimental studies of the stone masonry buildings, a literature review was performed on a similar structure to define the material properties for numerical analysis. In this study, the basic mechanical properties of stone masonry were referred from the Earthquake Emergency Assistance Project, which is conducted by the University of Engineering and Technology (UET) (Peshawar, 2019). They tested the stone masonry for remote Nepali schools where the material properties of stone masonry are similar to the SH. The material properties of the stone masonry were derived from the experimental testing of the school design for Nepal. UET Peshawar successfully performed the laboratory test for the design and for verification of the school design under the Nepal Earthquake Rehabilitation and Reconstruction Program. The material properties used for the development of the model were taken from (Angelillo et al., 2014; Bothara et al., 2018; Peshawar, 2019) and are shown in Table 3.
Prior to the non-linear analysis, a model analysis was performed. The fundamental time period of the structure was found to be 0.216 s for C_ISMB and 0.155 s for E_ISMB, which confirms the high rigidity of the E_ISMB. The fundamental mode shapes in the transverse and longitudinal direction are shown in Figure 11.
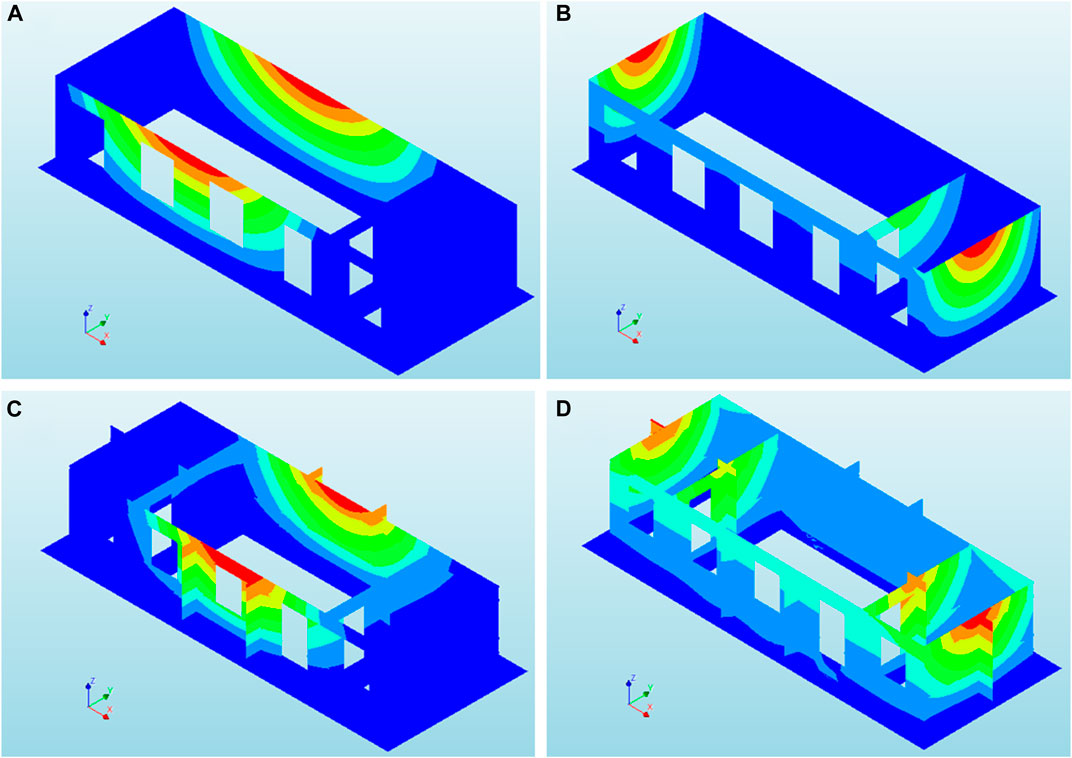
FIGURE 11. Fundamental mode shapes associated with bending (A) mode one transverse C_ISMB, (B) mode 3 longitudinal C_ISMB, (C) mode 1 transverse E_ISMB, and (D) mode 3 longitudinal E_ISMB.
To simulate the response of the structure during an earthquake, a non-linear static analysis or pushover analysis was first performed. The response of the structure was presented by the capacity curve, which shows the value of the base shear or seismic coefficient versus the displacement at a control point (usually taken at the top of the structure). To perform a pushover analysis for the assessment of seismic safety, a load pattern that approximates the distribution of inertia forces is defined according to Chopra & Goel (2002). A pushover analysis was performed on the FE model of the whole building but not on individual walls.
The introduction of timber bands and wall buttresses (i.e., E_ISMB) showed significant building improvement under lateral loading. Figure 12 C_ISMB showed slight failure at the lateral load at approximately 200 kN, whereas E_ISMB showed slight failure at the lateral load at approximately 410 kN. In C_ISMB, the whole wall height was engaged by the collapse mechanism at a lateral load of 370 kN, but in the same case in E_ISMB, cracks started to develop in the corner of buttresses and walls. The presence of the buttress delayed the activation of the out-of-plane mechanism and showed a lateral capacity of approximately 642 kN before failure occurred. The lower time period of E_ISMB signified that the structure was stiff because of the presence of buttresses. The long wall of C_ISMB was more vulnerable as the wall was significantly deflected under a lower lateral load.
5 Summary and conclusion
Stone masonry buildings are very common in the highlands of the Himalayan region of Nepal. These buildings were traditionally constructed with locally available materials such as stones, timber, and mud. In the Thame Valley, these buildings were typically two-story with thick masonry walls and an opening in the front façade and a heavy slate roof supported by a timber frame. Instead of enhancing the seismic capacity of buildings by using local materials, people are using modern materials that are difficult and costlier to transport. Therefore, this study focused on the enhancement of Sherpa houses by using local materials with the consideration of the Sherpa way of life. A detailed field survey was conducted to examine the construction practice and seismic enhancement of such irregular masonry buildings for seismic resilient design. From the survey, we found that during reconstruction and rebuilding, the existing irregularities present in the buildings were not considered. A total of 43% of buildings replaced the heavy gable wall with a lightweight metal sheet, and 95% of the buildings do not have seismic bands. Additionally, 69% of the buildings used corner tie stones at the corners of the buildings with some buildings using cement mortar in the corners only. A total of 72% of the buildings are one-story with dry stone masonry. More than 88% of reconstructed buildings replace the heavy slate roof with a lightweight CGI metal sheet. The reduced gable weight and construction of a one-story building with a CGI light roof were the main seismic enhancement techniques after the devastating earthquake. Based on traditional Sherpa building layout and current construction practices, the drawing of a typical Sherpa building was prepared. Then, the seismic enhancement of a typical Sherpa building with buttresses, bands, and improved roof-to-wall connection according to the current codes and Nepal manuals was performed. A 3D non-linear numerical analysis was performed for the two types of models discussed previously to determine improvement in the performance of the Sherpa house after interventions. The various enhancement techniques, such as a containment mesh with cross-ties, stitches in various levels, the connection of roof to bands through vertical wires, corner tie stones, and through stones, are not included in the numerical modeling. The numerical analysis only focuses on the global failure mode of masonry. To understand the local failure mode, such as cracking of individual stones, displacement of mortar joints, loosening of stones, and bulging of walls, experimental tests are recommended for these types of buildings.
From the numerical analysis, it was determined that the fundamental time period of E_ISMB was reduced by 28.2% compared to C_ISMB, which confirms a high rigidity-enhanced Sherpa house. The static pushover analysis was conducted for the purpose of the capacity assessment, and from the base shear calculation, it was found that the lateral strength of E_ISMB was 73.5% more than C_ISMB. Hence, the cross walls, buttress walls, and timber bands in conjunction with mud mortar, can be a simple technique of seismic enhancement in the region. The use of these bands and buttresses mainly helps reduce out-of-plane deformation in the wall, which delays the collapse of the long wall, and the stresses are more likely uniformly distributed instead of accumulating in the corners of the openings. The proposed strengthening methods were able to reduce the unstable failure mode and out-of-plane toppling of walls. The numerical modeling indicated that despite a minimal upgrading of unreinforced masonry, the performance and seismic capacity can be significantly improved. In addition to those mentioned previously, additional numerical and experimental shake table studies are required to further understand the effect of the local failure mode and the performance of the building for additional enhancement techniques that are recommended in this article.
Data availability statement
The original contributions presented in this study are included in the article/supplementary material, and further inquiries can be directed to the corresponding author.
Author contributions
SK performed the field assessment, field data result interpretation, development of methodology for research, and supervised all work. SA worked on the field assessment, field result preparation, and numerical modeling. AA worked on the field assessment, helped in the results interpretation, and numerical modeling. MV prepared the data acquisition methodology, developed tools for results interpretation and numerical analysis, and supervised all of the work.
Conflict of interest
The authors declare that the research was conducted in the absence of any commercial or financial relationships that could be construed as a potential conflict of interest.
Publisher’s note
All claims expressed in this article are solely those of the authors and do not necessarily represent those of their affiliated organizations, or those of the publisher, the editors, and reviewers. Any product that may be evaluated in this article, or claim that may be made by its manufacturer, is not guaranteed or endorsed by the publisher.
References
Acharya, O., Dahal, A., and Shrestha, K C. (2023). Confined masonry in seismic regions: Application to a prototype building in Nepal. Structures 47, 2281–2299. doi:10.1016/j.istruc.2022.12.045
Adhikari, R K., and D’Ayala, D. (2020). 2015 Nepal earthquake: Seismic performance and post-earthquake reconstruction of stone in mud mortar masonry buildings. Bull. Earthq. Eng. 18 (8), 3863–3896. doi:10.1007/s10518-020-00834-y
Ali, Q., Khan, A N., Ashraf, M., Ahmed, A., Alam, B., Ahmad, N., et al. (2013). “Seismic performance of stone masonry buildings used in the himalayan belt.”29. 4: 1159–1181. doi:10.1193/091711EQS228M
Angelillo, M., Lourenço, P B., and Milani, G. (2014). Masonry behaviour and modelling. Courses Lect. 551, 1–26. doi:10.1007/978-3-7091-1774-3_1/COVER
Aubriot, O., Faulon, M., Sacareau, I., Puschiasis, O., Jacquemet, E., Smadja, J., et al. (2019). Reconfiguration of the water–energy–food nexus in the everest tourist region of Solukhumbu, Nepal. Mt. Res. Dev. 39 (1), R47–R59. doi:10.1659/MRD-JOURNAL-D-17-00080.1
Bhattarai, U. (2021). A study of livelihood management practices of the sherpa people in the Khumbu (everest) region, Nepal.” doctoral thesis. Sydney: Western Sydney University.
Binda, L., Pina-Henriques, J., Anzani, A., Fontana, A., and Lourenço, P. B. (2006). A contribution for the understanding of load-transfer mechanisms in multi-leaf masonry walls: Testing and modelling. Eng. Struct. 28 (8), 1132–1148. doi:10.1016/j.engstruct.2005.12.004
Bothara, J., Ingham, J., and Dizhur, D. (2022). Qualifying the earthquake resilience of vernacular masonry buildings along the himalayan arc. J. Build. Eng. 52, 104339. doi:10.1016/J.JOBE.2022.104339
Bothara, J., and Brzev, S. (2011). Tutorial: Improving the seismic performance of stone masonry buildings. Oakland California 94612-1934.
Bothara, J., Giongo, I., Ingham, J., and Dizhur, D, (2018). “Numerical study on partially-reinforced semidressed stone masonry for build-back-better in Nepal,” in 10th International Masonry Conference (Milan, Italy.
Bothara, J., Ingham, J., and Dizhur, D. (2018). Understanding, experience and research on seismic safety of low-strength loadbearing masonry buildings vulnerability analysis of unreinforced masonry churches view Project.
Bruce, K E. (2020). Future sustainable development of buildings in the high altitude Khumbu region of Nepal. Trondheim, Norway: NTNU: Norwegian University of Science and Technology. Chukhung 4730m. Available at: https://www.ntnu.edu/.
Cabboi, A., Gentile, C., and Saisi, A. (2017). From continuous vibration monitoring to FEM-based damage assessment: Application on a stone-masonry tower. Constr. Build. Mater. 156, 252–265. doi:10.1016/J.CONBUILDMAT.2017.08.160
Chopra, A. K., and Goel, R. K. (2002). A modal pushover analysis procedure for estimating seismic demands for buildings. Earthq. Eng. Struct. Dyn. 31 (3), 561–582. doi:10.1002/EQE.144
Dais, D., Sarhosis, V., Smyrou, E., and Engin Bal, İ. (2021). Seismic intervention options for multi-tiered Nepalese pagodas: The case study of jaisedewal temple. Eng. Fail. Anal. 123, 105262. doi:10.1016/J.ENGFAILANAL.2021.105262
Diana Fea, B. V., and Tu, Delft (2017). DIANA validation report for masonry modelling date validation masonry models under seismic conditions. Delftechpark 19a 2628. Delft Netherlands: DIANA FEA BV and TU Delft.
FEMA P-154 (2015). Rapid visual screening of buildings for potential seismic hazards. Washington, D.C: FEMA.
FEMA P-155 (2015). Rapid visual screening of buildings for potential seismic hazards: Supporting documentation. Washington, D.C: FEMA.
Fisher, J F. (1990). Sherpas: Reflections on change in himalayan Nepal. University of California Press.
Gautam, D. (2018). Observational fragility functions for residential stone masonry buildings in Nepal. Bull. Earthq. Eng. 16 (10), 4661–4673. doi:10.1007/s10518-018-0372-2
Gautam, D., Prajapati, J., Valencia Paterno, K., Kumar Bhetwal, K., and Neupane, P. (2016). Disaster resilient vernacular housing technology in Nepal. Geoenvironmental Disasters 3 (1), 1–14. doi:10.1186/s40677-016-0036-y
Gautam, D., Rupakhety, R., Adhikari, R., Shrestha, B. C., Baruwal, R., and Bhatt, L. (2021). Seismic vulnerability of himalayan stone masonry: Regional perspectives. Mason. Constr. Act. Seismic Regions, January, 25–60. doi:10.1016/B978-0-12-821087-1.00014-4
Hamp, E., Gerber, R., Pulatsu, B., Santana Quintero, M., and Erochko, J. (2022). Nonlinear seismic assessment of a historic rubble masonry building via simplified and advanced computational approaches. Buildings 12 (8), 1130. doi:10.3390/BUILDINGS12081130
IAEE (2004). Guidelines for Earthquake Resistant Non-Engineered Construction, 108. Tokyo: Shiba Minato-kuJapan.
Khadka, B. 2020. “Mud masonry houses in Nepal: A detailed study based on entire reconstruction scenario in 31 earthquake-affected districts.” Structures 25: 816–838. doi:10.1016/j.istruc.2020.03.042
Khadka, S S. (2013). Seismic performance of traditional unreinforced masonry building in Nepal. Kathmandu Univ. J. Sci. Eng. Technol. 9 (I), 15–28.
Khansefid, A., Yadollahi, S M., Müller, G., Taddei, F., and Kumawat, A. (2022). “Seismic performance assessment of a masonry building under earthquakes induced by geothermal power plants operation.” J. Build. Eng. 48: 103909. doi:10.1016/j.jobe.2021.103909
Lageson, D R. (2016). Damage from the april-may 2015 Gorkha earthquake sequence in the Solukhumbu district (everest region). Nepal,” September.Monique fort, roshan raj Bhattarai, and mary hubbard
Laurenco, P. B., Rots, J. G., and Blaauwendraad, J. (1995). Two approaches for the analysis of masonry structures: Micro and macro-modeling. HERON 40 (4), 1995.
Lemos, J v. (2019). Discrete element modeling of the seismic behavior of masonry construction. doi:10.3390/buildings9020043
Lourenço, P B., Mendes, N., Ramos, L F., and Oliveira, D v. (2011). Analysis of masonry structures without box behavior. Analysis Mason. Struct. Without Box Behav. 5, 369–382. doi:10.1080/15583058.2010.528824
Mukai, Y., Hoshino, H., Yamamoto, N., Masui, M., Miyauchi, A., and Prasad Suwal, R. (2022). Investigation of microtremors observed at historic masonry townhouse buildings after Nepal earthquake. Front. Built Environ. 8, 163. doi:10.3389/fbuil.2022.918960
NBC 203, Nepal National Building Code (2015). Guidelines for earthquake resistant building construction: Low strength masonry.
Nepal, S. (2015). Irish pubs and dream cafes: Tourism, tradition and modernity in Nepal’s Khumbu (everest) region. Tour. Recreat. Res. 40 (2), 248–261. doi:10.1080/02508281.2015.1047625
Nepal, S K. (2002). Linking parks and people: Nepal’s experience in resolving conflicts in parks and protected areas. Int. J. Sustain. Dev. World Ecol. 9 (1), 75–90. doi:10.1080/13504500209470104
Norris, F H., Stevens, S P., Pfefferbaum, B, Wyche, K F., and Pfefferbaum, R L. (2008). Community resilience as a metaphor, theory, set of capacities, and strategy for disaster readiness. Am. J. Community Psychol. 41 (1–2), 127–150. doi:10.1007/S10464-007-9156-6
Nyaupane, G P., Lew, A A., and Kevin, T. (2014). Perceptions of trekking tourism and social and environmental change in Nepal’s Himalayas. Tour. Geogr. 16 (3), 415–437. doi:10.1080/14616688.2014.942233
Parisi, F., and Augenti, N. (2013). Seismic capacity of irregular unreinforced masonry walls with openings. Earthq. Eng. Struct. Dyn. 42 (1), 101–121. doi:10.1002/EQE.2195
Peshawar, U. E. T. (2019). TA-8910 nep: Earthquake emergency assistance Project experimental verification of remote school type designs. Peshawar.
Rawal, V., Bothara, J., Pradhan, P., Narasimhan, R., and Singh, V. (2021). Inclusion of the poor and vulnerable: Learning from post-earthquake housing reconstruction in Nepal. Prog. Disaster Sci. 10, 100162. doi:10.1016/J.PDISAS.2021.100162
Rebuild Thame (2015). An integrated grassroots approach towards post-earthquake construction in A remote himalayan valley. Zürich: Ten Ngo Zuric, Thame Sherpa Heritage Fund.
Rios, A., Jiménez, A., Pingaro, M, Reccia, E., and Trovalusci, P. (2021). Statistical assessment of in-plane masonry panels using limit analysis with sliding mechanism. J. Eng. Mech. 148 (2), 04021158. doi:10.1061/(ASCE)EM.1943-7889.0002061
Rupakhety, R., Gautam, D., Adhikari, R., Jha, P., Bhatt, L., and Baruwal, R. (2022). “System identification and finite element modelling of damaged bal Mandir monument in Kathmandu after the 2015 Gorkha earthquake,”Ioannis Vayas and Federico M Mazzolani. in Protection of historical constructions (Cham: Springer International Publishing).
Sherpa, P. (2017). Community and resilience among sherpas in the post-earthquake everest region. HIMALAYA, J. Assoc. Nepal Himal. Stud. 37 (2).
Schildkamp, M., Silvestri, S., and Araki, Y. (2020). Rubble stone masonry buildings with cement mortar: Design specifications in seismic and masonry codes worldwide. Front. Built Environ. 6, 176. doi:10.3389/fbuil.2020.590520
Sherpa, P. (2014). Climate change, perceptions, and social heterogeneity in pharak, Mount Everest region of Nepal. Hum. Organ. 73 (2), 153–161. doi:10.17730/HUMO.73.2.94Q43152111733T6
Shrestha, J K., Bhandari, S., Pradhan, S., and Gautam, D. (2020). Simplified frame model for capacity assessment of masonry buildings. Soil Dyn. Earthq. Eng. 131, 106056. doi:10.1016/J.SOILDYN.2020.106056
Smoljanović, H., Živaljić, N., and Nikolić, Ž. (2013). A combined finite-discrete element analysis of dry stone masonry structures. Eng. Struct. 52, 89–100. doi:10.1016/J.ENGSTRUCT.2013.02.010
Spoon, J. (2008). “Tourism in a sacred landscape: Political economy and sherpa ecological knowledge,” in Beyul khumbu/sagarmatha national Park, Nepal (Honolulu: Doctoral DissertationUniversity).
Stefano, M d., and Mariani, V. (2014). Pushover analysis for plan irregular building structures. Geotechnical, Geol. Earthq. Eng. 34, 429–448. doi:10.1007/978-3-319-07118-3_13/FIGURES/6
Valluzzi, M., Porto, F., and Modena, C. (2001). Behaviour of multi-leaf stone masonry walls strengthened by different intervention techniques.
Vasconcelos, G., and Lourenço, P. B. (2009). Experimental characterization of stone masonry in shear and compression. Constr. Build. Mater. 23 (11), 3337–3345. doi:10.1016/J.CONBUILDMAT.2009.06.045
Wang, M., Liu, K., Lu, H., Shrestha, H., Guragain, R., Pan, W., et al. (2019). Increasing the lateral capacity of dry joint flat-stone masonry structures using inexpensive retrofitting techniques. Bull. Earthq. Eng. 17 (1), 391–411. doi:10.1007/s10518-018-0454-1
Keywords: stone masonry building, rapid visual screening (RVI), irregular buildings, nonlinear analysis, resilient seismic design
Citation: Khadka SS, Acharya S, Acharya A and Veletzos MJ (2023) Enhancement of Himalayan irregular stone masonry buildings for resilient seismic design. Front. Built Environ. 9:1086008. doi: 10.3389/fbuil.2023.1086008
Received: 31 October 2022; Accepted: 13 February 2023;
Published: 06 March 2023.
Edited by:
Vagelis Plevris, Qatar University, QatarReviewed by:
Antonio Formisano, University of Naples Federico II, ItalyAlejandro Jiménez Rios, Oslo Metropolitan University, Norway
Copyright © 2023 Khadka, Acharya, Acharya and Veletzos. This is an open-access article distributed under the terms of the Creative Commons Attribution License (CC BY). The use, distribution or reproduction in other forums is permitted, provided the original author(s) and the copyright owner(s) are credited and that the original publication in this journal is cited, in accordance with accepted academic practice. No use, distribution or reproduction is permitted which does not comply with these terms.
*Correspondence: Shyam Sundar Khadka, sskhadka@ku.edu.np