Germline Specific Expression of a vasa Homologue Gene in the Viviparous Fish Black Rockfish (Sebastes schlegelii) and Functional Analysis of the vasa 3′ Untranslated Region
- 1The Key Laboratory of Experimental Marine Biology, Center for Ocean Mega-Science, Institute of Oceanology, Chinese Academy of Sciences, Qingdao, China
- 2Laboratory for Marine Biology and Biotechnology, Qingdao National Laboratory for Marine Science and Technology, Qingdao, China
- 3University of Chinese Academy of Sciences, Beijing, China
- 4Weihai Shenghang Aquatic Product Science and Technology Co., Ltd., Weihai, China
Germ cells play a key role in gonad development. As precursors, primordial germ cells (PGCs) are particularly important for germline formation. However, the origination and migration patterns of PGCs are poorly studied in marine fish, especially for viviparous economic species. The vasa gene has been widely used as a germ cell marker to identify a germline because vasa RNA is a component of germ plasm. In this study, we described the expression pattern of black rockfish (Sebastes schlegelii) vasa (Ssvas) in gonadal formation and development by in situ hybridization. The results showed that Ssvas failed in localization at the cleavage furrows until the late gastrula stage, when PGCs appeared and migrated to the genital ridge and formed elongated gonadal primordia at 10 days after birth. This study firstly revealed the PGCs origination and migration characteristics in viviparous marine fish. Furthermore, we microinjected chimeric mRNA containing EGFP and the 3′untranslated region (3′UTR) of Ssvas into zebrafish (Danio rerio) and marine medaka (Oryzias melastigma) fertilized eggs for tracing PGCs. We found that, although Sebastes schlegelii lacked early localization, similar to red seabream (Pagrus major) and marine medaka, only the 3′UTR of Ssvas vasa 3′UTR of black rockfish was able to label both zebrafish and marine medaka PGCs. In comparison with other three Euteleostei species, besides some basal motifs, black rockfish had three specific motifs of M10, M12, and M19 just presented in zebrafish, which might play an important role in labeling zebrafish PGCs. These results will promote germ cell manipulation technology development and facilitate artificial reproduction regulation in aquaculture.
Introduction
As highly specialized cells, germ cells play a key role in germline development (Xu et al., 2010). In fish, the germ cells undergo a series of basic biological processes, including formation, migration, proliferation, and differentiation, and eventually develop into mature gametes (Braat et al., 1999; Nishimura and Tanaka, 2014). Healthy gonad development and gametogenesis in fish are important for aquaculture. Therefore, investigating the whole development process of germ cells facilitates the reproduction regulation in aquaculture, such as sex control, gonad maturation induction, and artificial insemination (Piferrer, 2001). At the same time, it promotes germ cell manipulations, in which the time of gonad formation can provide a reference for germ cell transplantation (Takeuchi et al., 2004; Okutsu et al., 2006).
Primordial germ cells (PGCs), the precursors of germ cells, are set aside from somatic cells early in embryogenesis (Goto-Kazeto et al., 2010). PGCs arrive in the genital ridge through a long-range cell migration, and they are covered by somatic cells to form elongated gonadal primordia. In recent years, many maternal-effect genes such as vasa, dnd, dazl, and nanos localized in germplasm have been identified and extensively used as germ cell markers to study origination and migration of PGCs in fish (Xu et al., 2007; Lindeman and Pelegri, 2010; Yoshizaki et al., 2010; Hong et al., 2016). This reveals that the PGC migration routes and gonadal formation times vary from fish species. So far, there are two classical origination and migration patterns of PGCs. One representative is the zebrafish (Danio rerio), in which germ plasm granules in fertilized eggs are concentrated to the distal parts of the cleavage furrows, resulting in four aggregates during the first two cleavage divisions, and these cluster cells will differentiate into PGCs at the late blastula (Yoon et al., 1997). The other representative is the medaka (Oryzias latipes), whose granules are distributed uniformly throughout each blastomere until the onset of epiboly, and the PGCs start to appear at late gastrulation, lacking early localization (Shinomiya et al., 2000). Once PGCs form, they migrate and concentrate on the dorsal peritoneum, under the notochord at the hatching stage. The PGCs undergo a post-hatching migration in most oviparous fish. According to histological studies, PGCs migrate from the upper part of the body cavity to the rear and settle on the dorsal side of the gut, a location that future gonad forms. The settlement time of PGCs is species specific. In ukigori (Gymnogobius urotaenia), PGCs reached the genital ridge at 6–8 days post-hatching (dph) (Saito et al., 2004), while in turbot (Schophthalmus maximus), Japanese flounder (Paralichthys olivaceus), and yellowtail kingfish (Seriola lalandi), PGCs arrived in the gonad formation location at 15–20 dph (Fernandez et al., 2015; Zhao et al., 2017; Yang et al., 2018). However, the time of primordial gonadal formation of viviparous fish has not been reported so far. After migration, the germ cells begin mitosis and meiosis, and the formed gonads differentiate into presumptive ovary or testis (Gao et al., 2009). Under the control of the endocrine regulation and the external environment, gonads gradually develop to maturity and produce sexual gametes.
The vasa is the first germ gene identified in fish, which encodes an ATP-dependent RNA helicase of the DEAD (Asp–Glu–Ala–Asp)-box family (Knaut et al., 2000). It has been proved to be able to fulfill potential roles in germ cell origination and migration and maintain throughout the development of germ cells in fish (Kobayashi et al., 2000; Lin et al., 2012b; Ye et al., 2016). Moreover, the function of vasa varied considerably in diverse species. In medaka, vasa knockdown led to defects in PGCs migration but not proliferation, motility, identity, and survival (Li et al., 2009), while in zebrafish, disruption of vasa resulted in sterility in males (Hartung et al., 2014). As a universal germ cell marker, the vasa gene was used to identify the fish germline in economic species such as rainbow trout (Oncorhynchus mykiss) (Yoshizaki et al., 2010), Dabry’s sturgeon (Acipenser dabryanus) (Ye et al., 2016), and Atlantic cod (Gadus morhua) (Presslauer et al., 2012). The vasa mRNA has been shown to degrade rapidly in somatic cells but remain stable in PGCs, which are all mediated by the 3′untranslated region (3′UTR) (Knaut et al., 2002; Wolke et al., 2002; Mishima et al., 2006). For the inherent nature of vasa 3′UTR, the chimeric mRNA fused fluorescent protein-coding region to 3′UTR could be microinjected into fertilized eggs to trace PGCs (Kataoka et al., 2006; Ye et al., 2016). This is a highly efficient, fast, and specific non-transgenic method for labeling PGCs in vivo and has been used in a variety of fish species (Yoshizaki et al., 2005; Lin et al., 2012a; Zhou et al., 2019). Moreover, the chimeric mRNA from one species not only labels itself but also can successfully trace other species, even the genetic highly divergent species. For example, the chimeric mRNA (GFP-Pmvas 3′UTR) that contained GFP and the 3′UTR of red seabream (Pagrus major) could be used to trace migration of PGCs in medaka (Lin et al., 2012a). However, chimeric mRNA from one species cannot label PGCs from all species, just as the red seabream vasa 3′UTR failed to trace turbot PGCs, although they could label the medaka (Lin et al., 2012a; Zhou et al., 2019). This indicated that the conserved localization mechanism of vasa 3′UTR may relate to specific functional elements (Knaut et al., 2002; Kataoka et al., 2006; Zhou et al., 2019). For labeling ability of vasa 3′UTR, there may be rules to follow. However, the relevant researches in other species are limited, so it is still unclear.
Black rockfish (Sebastes schlegelii) is one of the most important economic rockfishes in the western North Pacific and inhabits the coastal waters of China, Japan, and Korea (Kai and Soes, 2009). As a viviparous fish, the embryos develop in female cavity for 1–2 months until birth (Liu et al., 2019). In previous research, Sebastes schlegelii vasa (Ssvas) was only detected and highly expressed in gonad during the reproductive cycle (Mu et al., 2013). There is still a lack of detailed research on germ cells during gonadal formation and development in this viviparous fish species. In this study, we aimed to describe the origination and migration pattern of Sebastes schlegelii PGCs in gonad formation process by vasa and explored the relationship between vasa RNA early localization and species evolution. Furthermore, we also identified the germ cells development and maturation process with vasa, verified the labeling ability of Ssvas 3′UTR in zebrafish and marine medaka (Oryzias melastigma), and analyzed the conservative motifs of vasa 3′UTR in comparison with several other species.
Materials and Methods
Fish and Samples
Matured black rockfish used in this study were obtained from Penglai, China. Ten sexually mature individuals in November were anesthetized with a 0.05% solution of 3-aminobenzoic acid ethyl ester methanesulfonate-222 (MS-222) (Sigma–Aldrich, United States). The tissues of ovary and testis were rapidly removed. Some samples were immediately immersed in liquid nitrogen for cDNA synthesis, while others were fixed overnight with 4% paraformaldehyde in phosphate-buffered saline (PBS) and then preserved in 70% ethanol for section in situ hybridization (SISH). Twenty sexually mature females in April to May were also anesthetized with MS-222. Moreover, the embryos of different development stages in the ovary were removed and fixed by 4% paraformaldehyde in PBS overnight and stored at −20°C in PBS with 50% formamide for whole mount in situ hybridization (WISH).
One-year-old and hatching fry of black rockfish were reared at Shenghang Sci-Tech Co., Ltd. (Shandong Province, China). The testes and ovaries of six 1-year-old individuals were removed after anesthesia and fixed in 4% paraformaldehyde as above. Starting from hatching, about 20 hatched fry were fixed with 4% paraformaldehyde every day for 1 month. After 1 day of fixation, the gonadal tissue samples and fry were stored at 70% ethanol for SISH.
All experiments were performed in accordance with the relevant national and international guidelines and approved by the Institutional Animal Care and Use Committee, Institute of Oceanology, Chinese Academy of Sciences.
Sequence Analyses
A homology search of the amino acid sequence of Ssvas was carried out using the National Center for Biotechnology Information website1. The amino acid sequences were aligned using the AlignX program in Vector NTI, Suite 8 software package (Life Technologies). Phylogenetic analysis was conducted with Mega4 software using the neighbor-joining method, and the bootstrap replicates were set to 1,000 (Saitou and Nei, 1987). Some potential regulatory motifs in the 3′UTRs of teleost vasa were searched by MEME motif analysis (Bailey and Elkan, 1994).
In situ Hybridization
Total RNA was extracted from the ovaries of Sebastes schlegelii using RNA fast 200 (Fastagen, China). In accordance with the manufacturer’s instructions, the first-strand cDNA was synthesized by the PrimeScriptTM RT reagent kit with gDNA Eraser (Takara, Japan). Then, the cDNA was stored at −20°C as a probe synthesis template.
vasa mRNA distribution was detected in embryo and tissue by in situ hybridization (ISH), and the protocols followed the methods described previously (Lin et al., 2012b), with a few modifications as detailed below. Briefly, the 857 bp Ssvas fragment (nucleotides 1,540–2,396 bp; accession no. JN634874) was inserted into pGEM-T Easy vector (Promega, Madison, WI). The primers are shown in Table 1. Sense and antisense RNA probes were synthesized by in vitro transcription from a vector under the drive of the T7 or SP6 promoter with the DIG RNA Labeling Kit (Roche, Mannheim, Germany). The RNA probes were treated with RNase-free water and purified with SigmaSpinTM Sequencing Reaction Clean-Up (Sigma–Aldrich). ISH was conducted by chemical stain with BCIP/NBT substrates on whole mount and sections samples.
For WISH, embryos were dechorionated, and dehydrated and rehydrated through a graded series of methanol after being taken out from 50% formamide in PBS. The embryos were digested with proteinase K (10 μg/ml) (5–10 min for embryo < 24 h; 15–30 min for embryo > 24 h) and refixed before hybridization. For SISH, samples were dehydrated through a graded series of ethanol, embedded in paraffin wax, and cut into 5 μm-thick sections for testis and 7 μm for ovary. The sections were de-waxed using two washes of xylene and rehydrated through an ethanol gradient. After being washed with PBST, they were refixed using 4% paraformaldehyde in PBS and digested with proteinase K (10 μg/ml) for 10 min. The WISH and SISH were performed with the probes at 65°C for 14 h. Stained samples were observed and photographed through a Nikon ENi microscope, using a Nikon DS-Ri2 imaging system.
Prepare of EGFP-Ssvasa 3′UTR and mCherry-Drvasa 3′UTR mRNAs
Chimeric mRNAs which fused EGFP or mCherry to the 3′UTR of Sebastes schlegelii or Danio rerio vasa gene were synthesized by in vitro transcription, and the protocols followed the methods described previously (Zhou et al., 2019). The main process is as follows: firstly, template plasmids were constructed using Gibson assembly, and the 3′UTRs of EGFP-Pmvasa 3′UTR and mCherry-Omvasa 3′UTR plasmids in our lab were replaced by vasa 3′UTRs of black rockfish and zebrafish, respectively. Then, according to the plasmids, the transcription templates were synthesized by PCR. After purification, capped chimeric mRNAs were synthesized using mMESSAGE mMACHINE SP6/T7 kit (Ambion, United States). The primers for preparing mRNAs are also shown in Table 1. In the previous study, the EGFP-Pmvasa 3′UTR and mCherry-Omvasa 3′UTR mRNAs that contained red seabream and marine medaka vasa 3′UTRs have been synthesized in our lab (Zhou et al., 2019).
Microinjection and Observation
Zebrafish and marine medaka were maintained at 28 ± 0.5°C (16 h light/8 h dark) at the fish facility of the Institute of Oceanology, Chinese Academy of Sciences. The marine medaka is a truly euryhaline species closely related to the medaka. Embryos were collected within 30 min after fertilization. To verify the labeling ability of Ssvas 3′UTR in zebrafish and marine medaka, the EGFP-Ssvasa 3′UTR and mCherry-Drvasa 3′UTR mRNAs were co-injected into zebrafish embryos at the 1–2 cell stage, and the EGFP-Ssvasa 3′UTR and mCherry-Omvasa 3′UTR mRNAs were co-injected into marine medaka embryos in the same manner. Similarly, to compare the ability of vasa 3′UTRs among Sebastes schlegelii and other teleost in marking zebrafish PGCs, the EGFP-Pmvasa 3′UTR and mCherry-Omvasa 3′UTR mRNAs were also microinjected into zebrafish embryos as above. Microinjected eggs were cultured in a biochemical incubator with 28°C for EGFP or mCherry observation and photography under fluorescent microscope (Nikon ENi, Japan) at different stages. The microscope equipped with a FITC filter or a TRITC filter and a DS-Ri2 imaging system.
Results
Structure and Phylogenetic Analysis of Sebastes schlegelii Vasa Protein
The full-length cDNA of Ssvas had been isolated in the previous research. In this study, multiple alignment of different fish Vasa proteins showed that there were eight consensus motifs (I, IA, IB, IC, II, III, IV, V) in the DEAD-box protein family (Figure 1A). This also revealed that the DEAD domain was composed of 180 amino acids and the superfamily Helicase C-terminal (Helicase C) of 77 amino acids, which had high similarity in teleost (Figure 1A). The Sebastes schlegelii presented Vasa typical conserved domains (Figure 1A). Phylogenetic analysis of Vasa protein exhibited that Sebastes schlegelii belonged to Euteleostei species (Figure 1B). In phylogeny, compared to Osteriophysans species like Danio rerio, Sebastes schlegelii was closer to some Euteleostei fish, such as Thunnus orientalis, Scophthalmus maximus, Pagrus major, Oryzias latipes, and Oncorhynchus mykiss (Figure 1B).
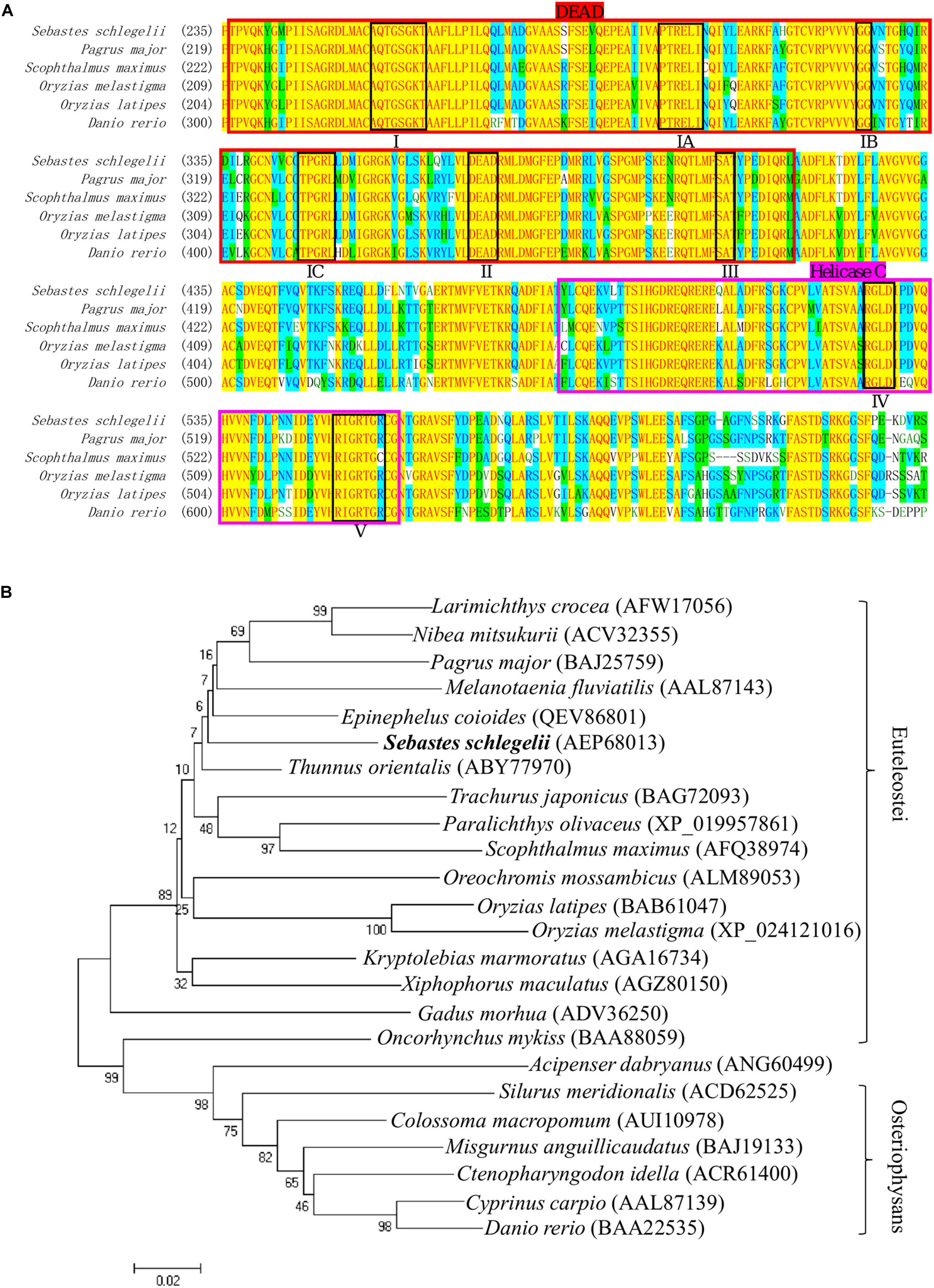
Figure 1. Multiple alignment and phylogenetic analysis of the Vasa between Sebastes schlegelii and other species. (A) Multiple alignment was carried out using the AlignX program. The eight conserved motifs are shown in block, including I domain (AQTGSGKT), IA domain (PTREL), IB domain (GG), IC domain (TPGRL), II domain (DEAD), III domain (SAT), IV domain (ARGLD), and V domain (GRTGR). The DEAD-box sequence was indicated in bright red, while Helicase C was in rose red. (B) The phylogenetic tree of Vasa was established by MEGA4 with neighbor-joining method. The numbers adjacent to nodes indicated bootstrap percentage value for 1000 replicates.
Spatial Localization of Ssvas RNA During Embryogenesis
The expression pattern of vasa during embryogenesis was analyzed by WISH. At the blastula stage, all blastomeres were strongly stained, without the four cell clusters like zebrafish (Figure 2A). In Sebastes schlegelii, the Ssvas transcripts failed in early localization during the cleavage stage. As the embryo developed, the distribution of the signal in the cell became non-uniform, and the areas of embryonic shield seemed to be darker than the rest at the 30% epiboly stage (Figure 2B). Until the 70% epiboly stage, some Ssvas-positive cells firstly appeared at the germ ring of the epiboly bottom (Figure 2C). According to morphology, these positive cells were early PGCs. At the 90% epiboly stage to early neurula stage, the PGCs gathered on the dorsal side of the narrow embryonic body and randomly aligned along the anterior of the body axes (Figure 2D). Then, they moved posterior-ward and lined along the anterior–posterior axis on both sides of the embryonic body at somite stage (Figure 2E). These cells formed two clusters and aligned bilaterally on the ventral side at heart beating stage (Figures 2F,G). Finally, the PGCs localized at the dorsal sides of the gut, ventral side of notochord at the hatching stage (Figures 2H,I).
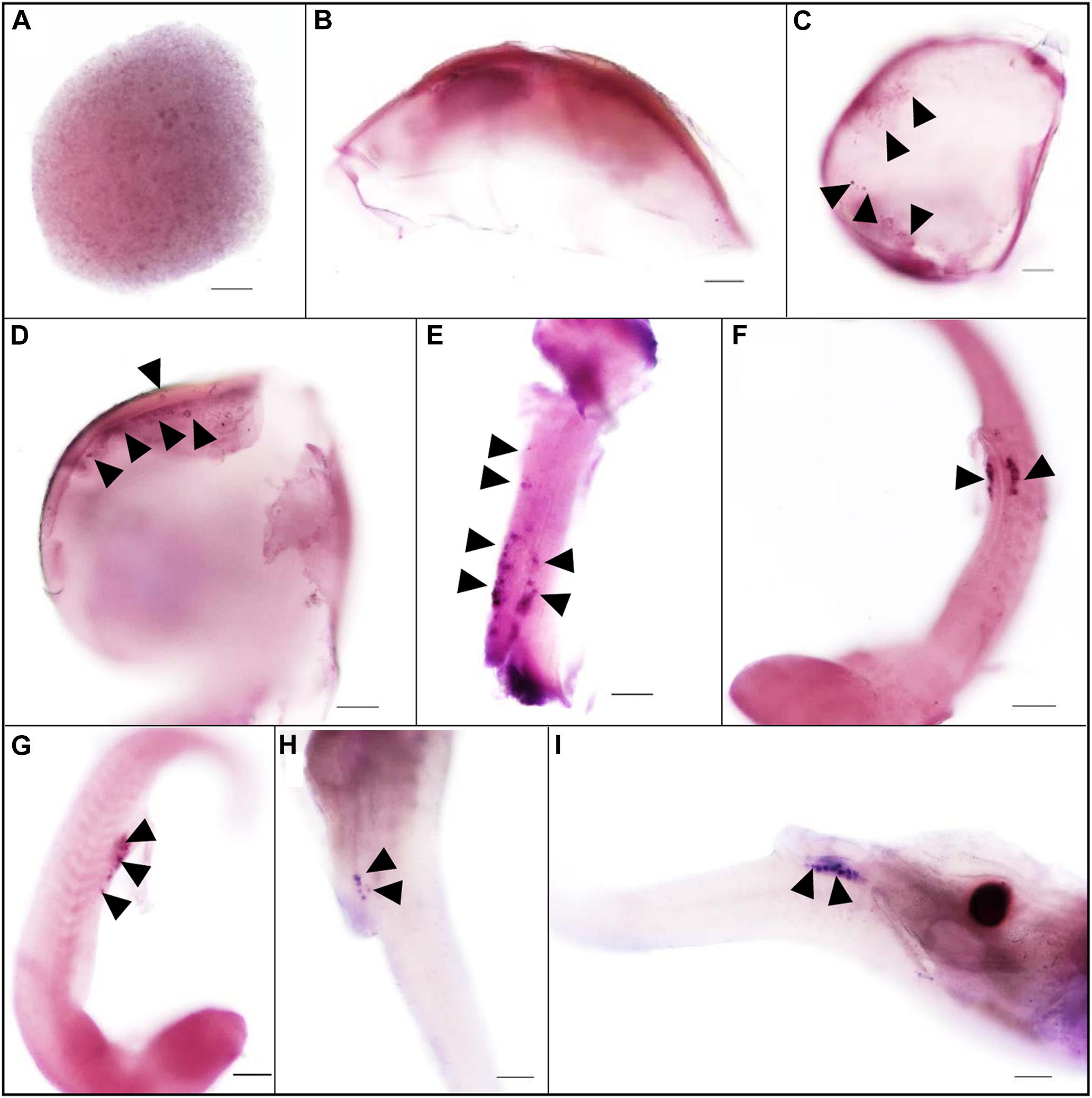
Figure 2. The expression pattern of Ssvas during embryogenesis was analyzed with WISH. All blastomeres were strongly stained at the blastula stage (A). The signal in the cell became non-uniform at the 30%-epiboly stage (B). Ssvas-positive cells appeared at the 70% epiboly stage (C). PGCs randomly aligned along the anterior of the body axes at the 90% epiboly stage–early neurula stage (D) and lined along the anterior–posterior axis on both sides of the embryonic body at the somite stage (E). PGCs formed two clusters and aligned bilaterally on the ventral side at heart beating stage (F,G) and localized at the dorsal sides of the gut, ventral side of notochord at hatching Sage (H,I). Black arrowheads indicated Ssvas-positive cells (PGCs) (stained with dark purple or blue). Scale bar, 100 μm.
The PGC Migration After Birth of Sebastes schlegelii
The PGCs underwent a post-birth migration in Sebastes schlegelii. PGCs are characterized by large cell size and obvious nuclei, which are easily distinguished with somatic cells on tissue sections. At 2 days after birth (dab), PGCs localized in the upper part of the body cavity under the notochord, around the dorsal peritoneum (Figures 3A1,A2). A part of PGCs were covered by a single layer somatic cell at 8 dab (Figures 3B1,B2). PGCs surrounded by single somatic cells were found to migrate to the genital ridge at 9 dab (Figures 3C1,C2). By 10 dab, three PGCs were completely surrounded by somatic cells and arranged in a row to form elongated gonadal primordia, which appeared under the ventral kidney and dorsal sides of the gut (Figures 3D1,D2). Until 15 dab, the distinct primordial gonads were discovered in the posterior part of the body cavity, and the PGCs in gonads were strongly stained with Ssvas antisense probe by SISH (Figures 3E1–E3). Through the identification of Ssvas, the germline formation process of Sebastes schlegelii was summarized, as shown in the schematic illustration (Figure 4).
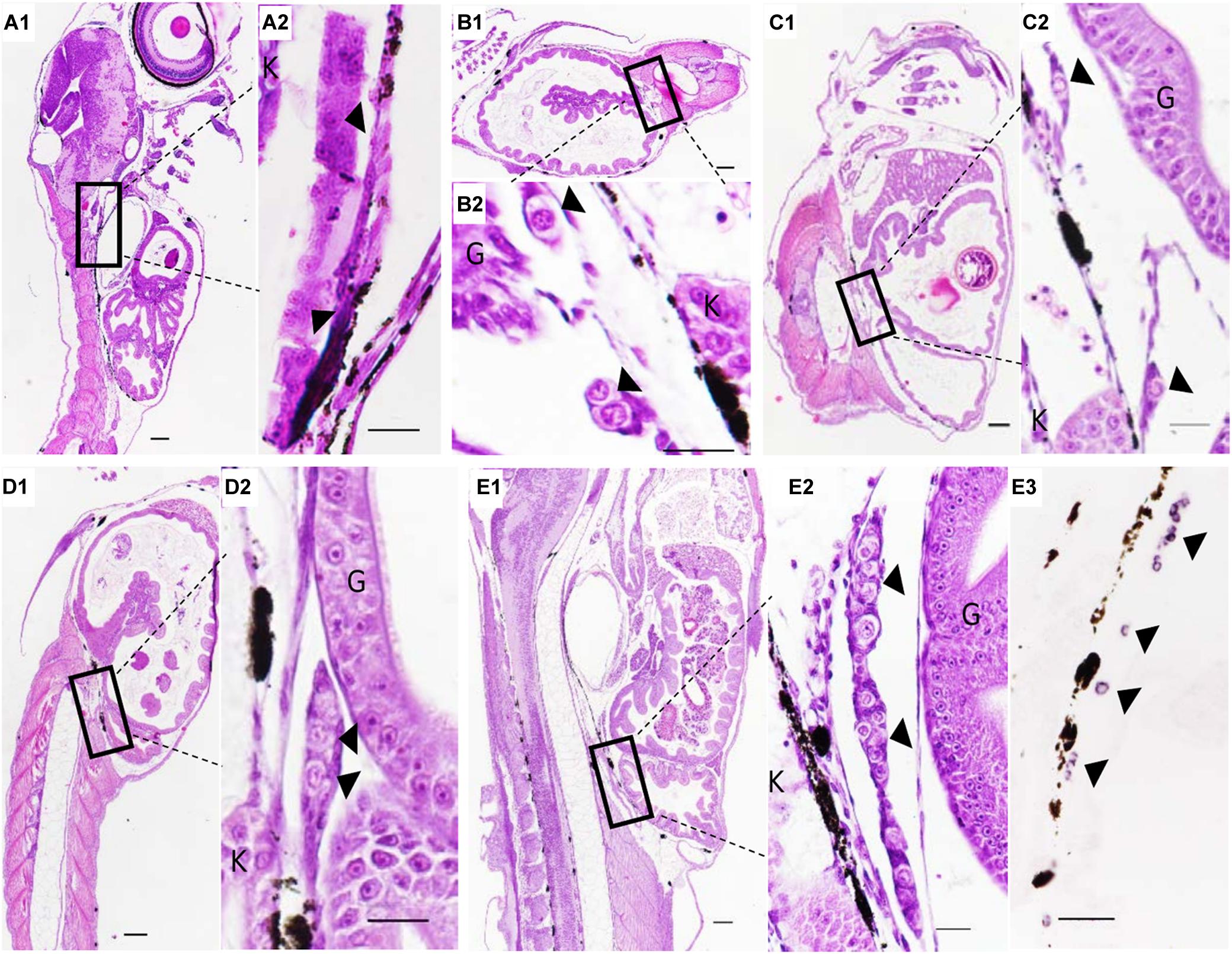
Figure 3. The PGCs migration after birth of Sebastes schlegelii. (A1–E2) were tissue sections with H&E stain. (E3) was SISH corresponding to (E2). PGCs were located in the upper part of the body cavity under the notochord at 2 dab, sagittal sections (A1,A2) and covered by a single-layer somatic cell at 8 dab, coronal sections (B1,B2). PGCs migrated to the genital ridge at 9 dab, sagittal sections (C1,C2) and formed elongated gonadal primordia at 10 dab, sagittal sections (D1,D2). Distinct primordial gonads were discovered in the posterior part of the body cavity (E1,E2), and the PGCs were strongly stained with the Ssvas antisense probe by SISH at 15 dab, sagittal sections (E3). Black arrowheads indicated PGCs; G and K indicated gut and kidney, respectively. Scale bar, 100 μm (A1,B1,C1,D1,E1); 50 μm (A2,B2,C2,D2,E2,E3).
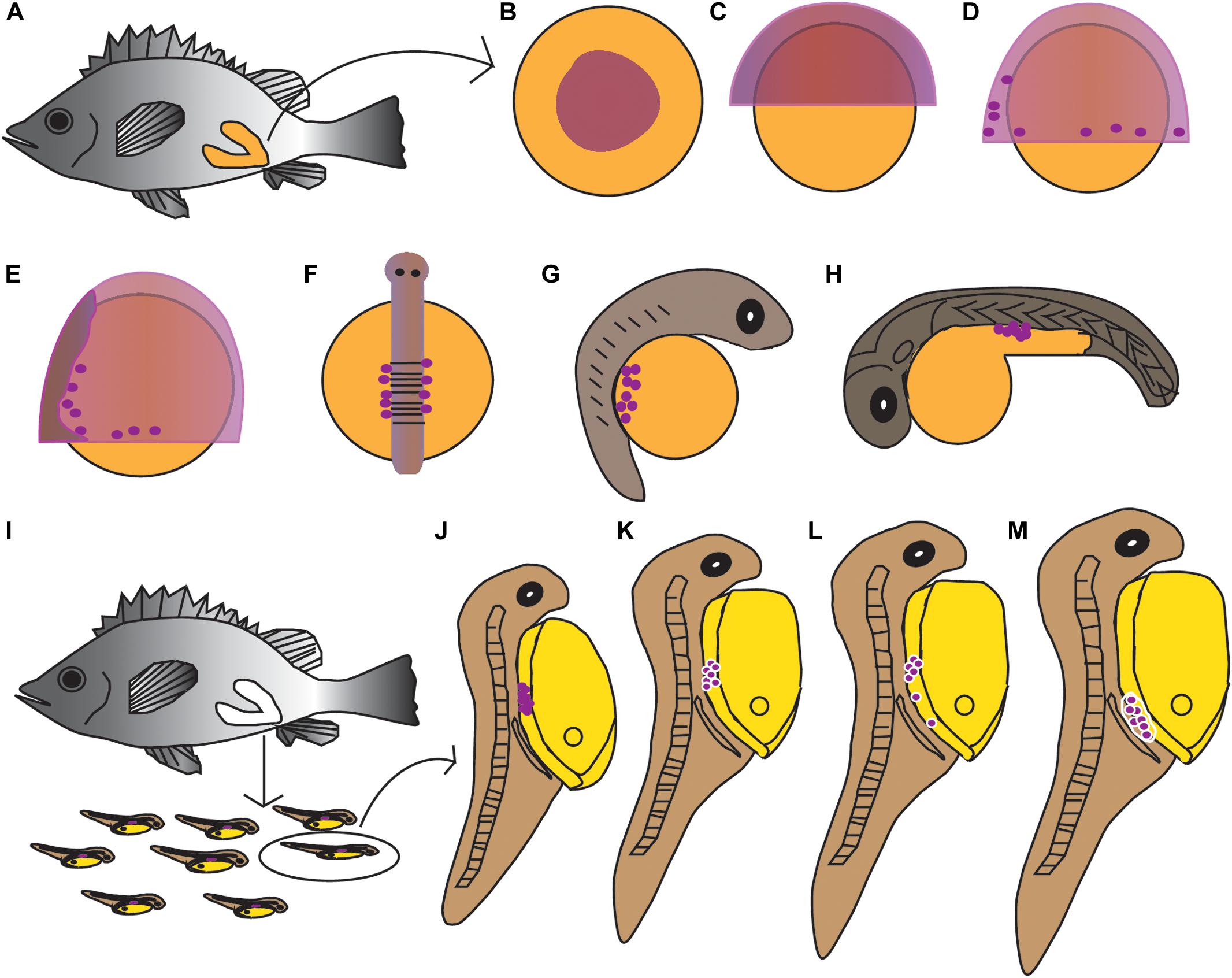
Figure 4. Schematic illustration of the germline formation process in Sebastes schlegelii. Dark purple spots indicated PGCs signals. (A–H) The migration of PGCs from embryos in female fish after fertilization. (A) The overall appearance of the female after fertilization; (B) blastula stage; (C) 30% epiboly stage; (D) 70% epiboly stage; (E) 90% epiboly stage–early neurula stage; (F) somite stage; (G) heart beating stage; (H) hatching stage. (I–M) The migration of PGCs from larvae after birth. (I) The larvae were born from mature females; (J) 2 dab; (K) 8 dab; (L) 9 dab; and (M) 10–15 dab.
Localization of Ssvas RNA in Gonads
The localization of Ssvas RNA was investigated in fish gonads by SISH. In accordance with adjacent histology, the stages of stained cells were identified. For 1-year-old black rockfish, in the ovary, there were mainly oogonia, early previtellogenic phase (I), and late previtellogenic phase (II) (Figure 5A1). Ssvas-specific expression was observed in all germ cells but not somatic cells (Figure 5A2). In oogonia, Ssvas mRNA was distributed evenly in the cytoplasm (Figure 5A2). In I–II oocytes, Ssvas transcripts were concentrated into several patches primarily in the perinuclear region (Figure 5A2). For sexually mature females, Ssvas transcripts were researched throughout oogenesis and showed a dynamic localization pattern in the ovary. A large number of early vitellogenesis phase (III) oocytes were present in the November individuals, but there was no Ssvas expression (Figures 5B1,B2). The tissue section hybridized with the sense probe was not stained, proving that this signal represents the expression of Ssvas (Figure 5B3).
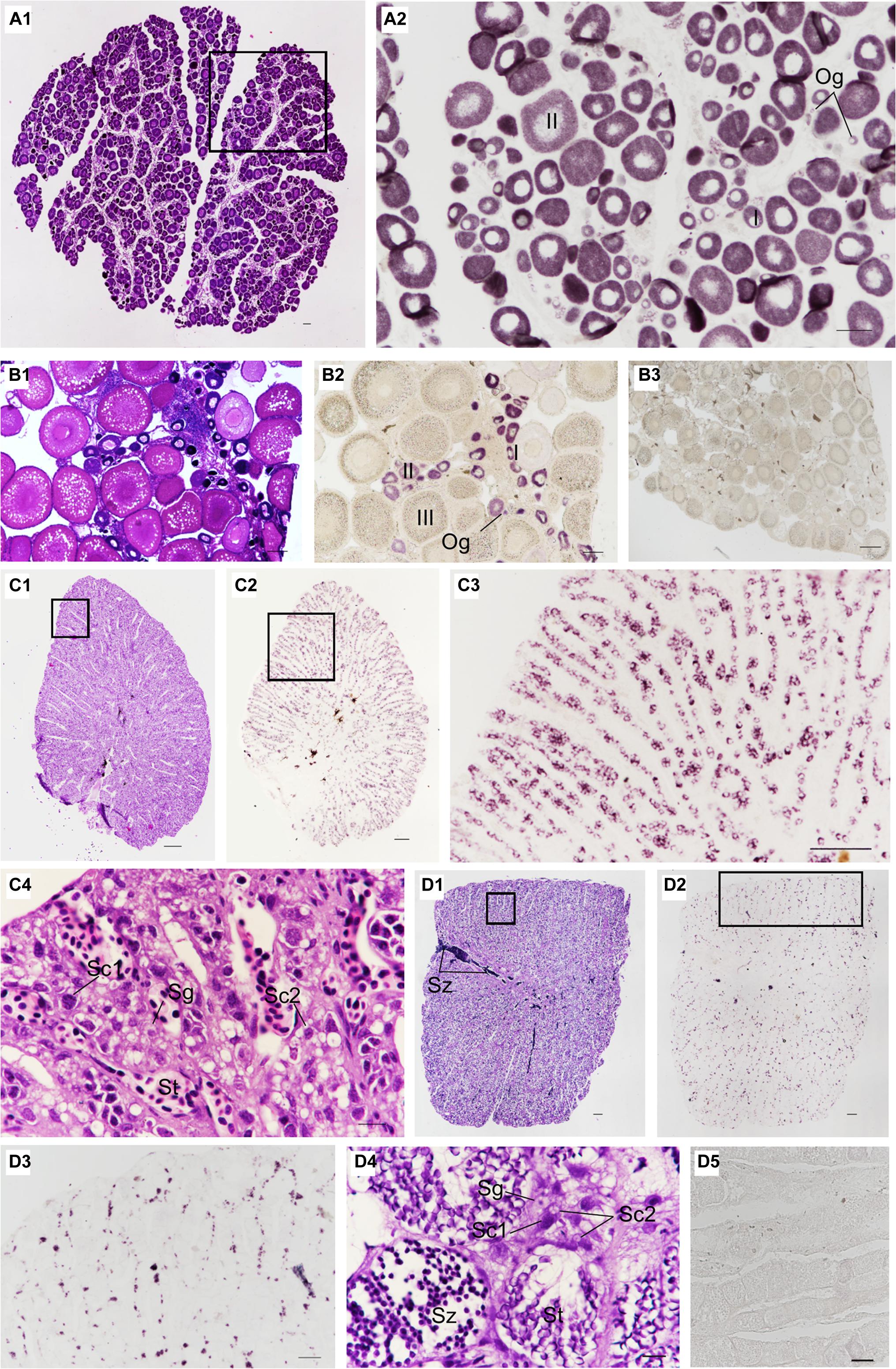
Figure 5. Distribution of Ssvas transcripts in gonad germ cells by SISH. Paraffin section and H&E of ovaries in 1-year-old (A1) and sexually mature black rockfish (B1), respectively. (A2) Adjacent tissue section of the black box in (A1) was hybridized with the Ssvas antisense probe. (B2) Adjacent tissue section of (B1) was hybridized with the Ssvas antisense probe. (B3) The paraffin section of the ovary was hybridized with the Ssvas sense probe. The paraffin section and H&E of testis in 1-year-old (C1) and sexually mature black rockfish (D1), respectively. (C2,D2) Adjacent tissue sections of (C1,D1) were hybridized with the Ssvas antisense probe, respectively. (C3,D3) The high-magnification image of the black boxes in (C2,D2), respectively. (C4–D4) The high-magnification image of the black boxes in (C1,D1), respectively. (D5) The paraffin section of testis was hybridized with the Ssvas sense probe. Og, oogonia; I, early previtellogenic phase; II, late previtellogenic phase; III, early vitellogenic phase; Sg, spermatogonia; Sc1, primary spermatocytes; Sc2, secondary spermatocytes; St, spermatids; Sz, spermatozoon. Scale bar, 100 μm (A1–C4,D3–D5); 200 μm (D1,D2).
Histological sections of the testis demonstrated that Sebastes schlegelii was lobular-type (Figures 5C1, D1). In the testis of 1-year-old black rockfish, there were spermatogonia, primary spermatocytes, secondary spermatocytes, and spermatids, without spermatozoon (Figure 5C4). For sexually mature males more than 3 years old, a lot of spermatozoon were found (Figure 5D4). The testis was composed of many testicular lobules, and the Ssvas signals were only present in spermatogonia and primary spermatocytes, which localized at the edge of the lobules and spread throughout the whole testis except the vas deferens (Figures 5C2,D2). The signals in matured testis were significantly weaker than 1-year-old testis because Ssvas transcripts were predominantly detected in early germ cells, such as spermatogonia and spermatocytes, and no signal was detected in spermatids and spermatozoon (Figures 5C3,D3). As control, the tissue section hybridized with the sense probe was not stained (Figure 5D5).
Visualization the PGCs of Zebrafish and Marine Medaka by Sebastes schlegelii vasa 3′UTR
In order to confirm that the labeling ability of Sebastes schlegelii vasa 3′UTR, EGFP-Ssvasa 3′UTR mRNA and mCherry-Drvasa 3′UTR mRNA were co-microinjected into zebrafish 1–2 cell stage fertilized eggs. After microinjection, mCherry and EGFP firstly expressed in the blastoderm at the 30–50% epiboly stage, and bright fluorescence accumulated in the area where the embryonic shield formed (Figures 6A1–A3). As embryogenesis proceeded, fluorescence concentrated on the embryonic body was more obvious, but PGCs were not distinguished from somatic cells until the early segmentation period. At the somite stage, as the fluorescence of somatic cells reduced gradually, some brighter cells moved and gathered on both sides of embryonic body (Figures 6B1–B3). The rounder and larger fluorescent cells were PGCs. PGCs migrated axial-ward and aggregated under the trunk at the heart beating stage (Figures 6C1–C3). Eventually, PGCs settled on the dorsal part of the gut at one day after hatching (Figures 6D – D3).
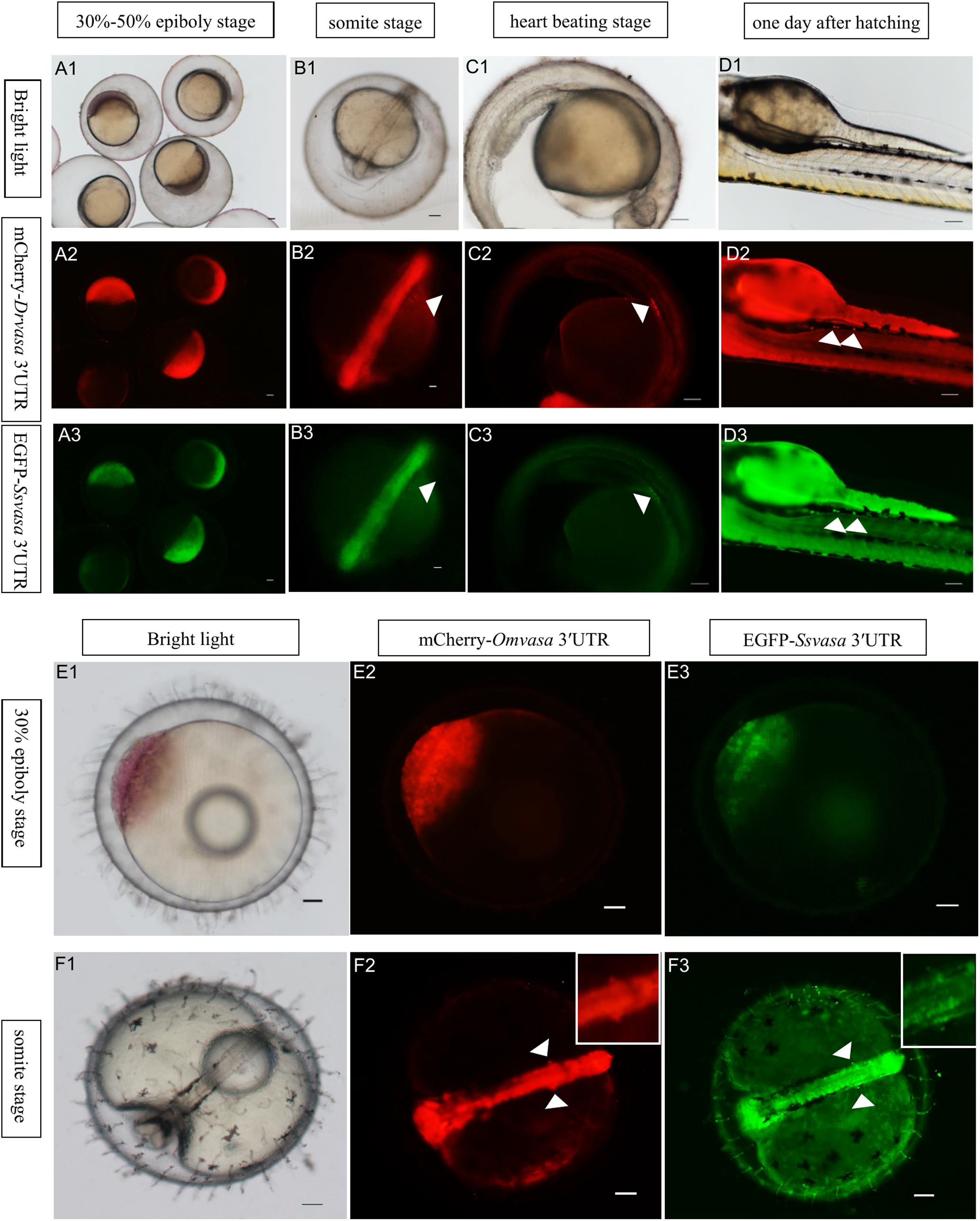
Figure 6. Visualization of the PGCs of zebrafish and marine medaka by Sebastes schlegelii vasa 3′UTR. (A1–D3) Visualization of zebrafish PGCs by co-microinjection EGFP-Ssvas 3′UTR and mCherry-Drvasa 3′UTR mRNAs. Fluorescence first expressed in the blastoderm at the 30–50% epiboly stage (A1–A3); PGCs differentiated from somatic cells and gathered on both sides of the embryonic body at the somite stage (B1–B3); PGCs aggregated under the trunk at the heart beating stage (C1–C3) and settled on the dorsal part of the gut at 1 day after hatching (D1–D3). (E1–F3) Visualization marine medaka PGCs by co-microinjection of EGFP-Ssvas 3′UTR and mCherry-Omvasa 3′UTR mRNAs. Fluorescence appeared at the 30% epiboly stage (E1–E3) and PGCs aligned on both sides of the trunk at the somite stage (F1–F3). The insets in F2 and F3 were magnification of signal areas. PGCs were indicated by white arrows. Scale bars, 100 μm.
In the same way, the EGFP-Ssvasa 3′UTR and mCherry-Omvasa 3′UTR mRNAs were co-microinjected into marine medaka embryos. EGFP and mCherry firstly appeared at the 30% epiboly stage (Figures 6E1–E3). At the somite stage, some cells with strong fluorescence aligned on both sides of the trunk from the first somite to tail bud region (Figures 6F1–F3). These fluorescent cells were PGCs.
Oryzias melastigma and Pagrus major vasa 3′UTRs Labeling Zebrafish PGCs
To compare the labeling ability of vasa 3′UTRs among Sebastes schlegelii and other teleost in marking zebrafish PGCs, the chimeric mRNAs of EGFP-Pmvasa 3′UTR and mCherry-Omvasa 3′UTR were also microinjected into zebrafish fertilized eggs. The mCherry and EGFP fluorescence firstly appeared in blastoderm at the early gastrula stage after microinjection (Figures 7A1–A3). A high level of fluorescence expression was found in the zebrafish embryonic body at the early somite stage (Figures 7B1–B3), but PGCs were not distinguished from somatic cells during the whole embryonic development (Figures 7C1–D3). In order to avoid misjudgment of results, a large number of embryos at heart beating stage were observed (Figures 7C2′,C3′). Although some fluorescent aggregates were found in the extension of the yolk sac at heart beating stage and 1 day after hatching, PGCs could not be distinguished (Figures 7C1–C3, D1–D3).
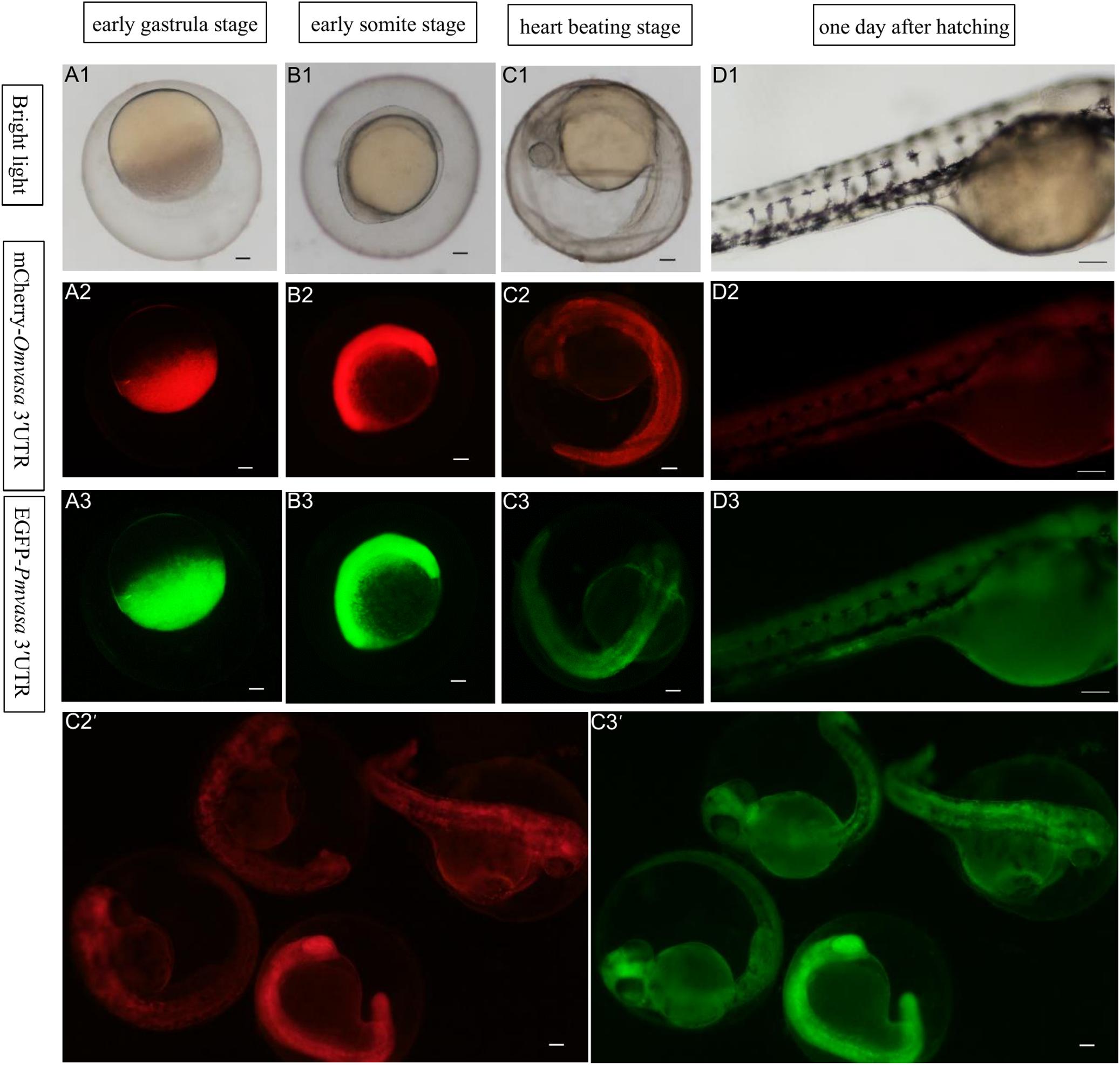
Figure 7. The ability of Oryzias melastigma and Pagrus major vasa 3′UTRs in labeling zebrafish PGCs. The EGFP-Pmvasa 3′UTR and mCherry-Omvasa 3′UTR mRNAs were co-microinjection into zebrafish embryos. Fluorescence appeared at the early gastrula stage (A1–A3) and highly expressed in the embryonic body at the early somite stage (B1–B3); PGCs did not differentiate from somatic cells at the heart beating stage (C1–C3) and 1 day after hatching (D1–D3). View of the embryos of the heart beating stage in a wide range (C2′,C3′). Scale bars, 100 μm.
Phylogenetic Analysis and MEME Motifs Analysis in Teleost vasa 3′UTRs
The teleost of Sebastes schlegelii, Pagrus major, Oryzias latipes, Oryzias melastigma, and Danio rerio were comparative analysis in this study. Phylogenetic analysis showed that Sebastes schlegelii and Pagrus major were clustered in one clade, while Oryzias latipes and Oryzias melastigma were clustered in one clade, both of which belonged to Euteleostei species (Figure 8A). And Danio rerio was grouped into a single branch, belonging to Osteriophysans species (Figure 8A).
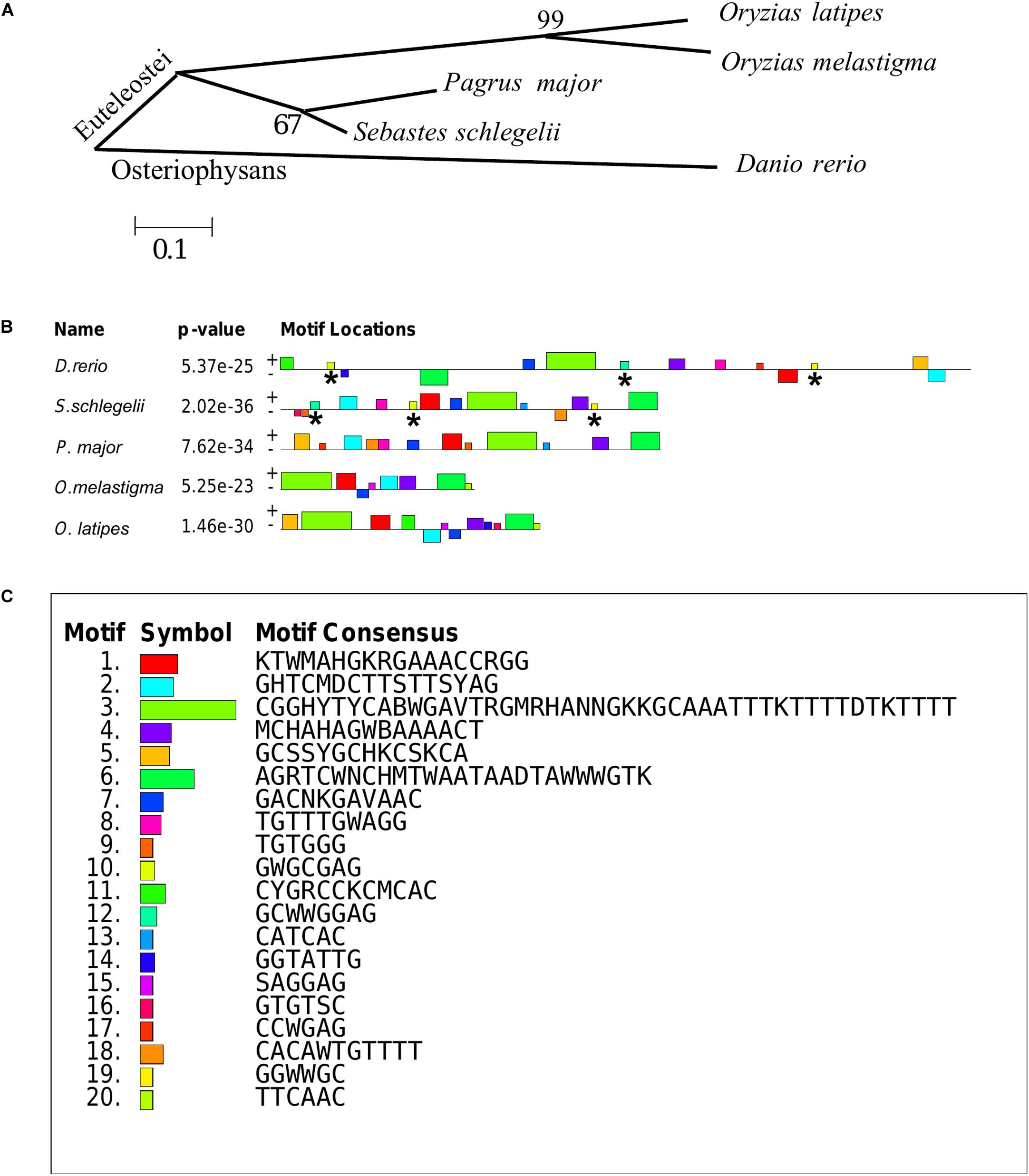
Figure 8. Phylogenetic analysis and MEME motifs analysis in teleost vasa 3′UTRs. (A) Phylogenetic analysis of teleost vasa 3′UTRs by MEGA4. (B,C) Conservative functional motifs analysis in teleost vasa 3′UTRs by MEME. The twenty motifs designated as M1–M20 were indicated by different color codes (B), and every color code represented motif consensus (C). The motifs of M10, M12, and M19 marked with asterisks were only localized in Danio rerio and Sebastes schlegelii rather than the other three species.
M1–M20 motifs of vasa 3′UTRs were compared among the five teleost species. Sebastes schlegelii and Pagrus major had more similar conservative elements, while Oryzias latipes and Oryzias melastigma had more similar motifs (Figures 8B,C). Moreover, Danio rerio showed obvious different motifs with above species (Figures 8B,C). The results of MEME analysis were totally consistent with those of the phylogenetic analysis. However, in comparison with other three species, the motifs of M10, M12, and M19 specifically existed in Sebastes schlegelii and Danio rerio (Figures 8B,C).
Discussion
The present study reported that the vasa identified the germline in Sebastes schlegelii. The Sebastes schlegelii Vasa protein possessed typical conserved domains of the DEAD-box protein family. By WISH, the Ssvas mRNA distributed uniformly throughout each blastomere at the blastula stage, failing in early localization during the cleavage stage. It was not until the late gastrula stage that PGCs gradually formed and randomly aligned along the anterior of the body axes. Then, PGCs moved posterior-ward and lined both sides of the embryonic body at the somite stage, and localized at the dorsal sides of the gut and ventral side of notochord at hatching stage. After birth, PGCs localized in the upper part of the body cavity. They were covered by single-layer somatic cells, migrated to the genital ridge, and formed elongated gonadal primordia in the posterior part of the body cavity at 10 days. The migration routes and final location of PGCs during embryogenesis were similar to medaka (Shinomiya et al., 2000). Moreover, the time of primordial gonad formation of the viviparous economic fish after birth was earlier than most of oviparous fish such as turbot, Japanese flounder, and rainbow trout after hatching, which might be related to the long embryonic development time of viviparous fish in vivo (Fernandez et al., 2015; Zhao et al., 2017; Yang et al., 2018). This study firstly described the origination and migration pattern of PGCs in viviparous marine economic fish.
Medaka, rainbow trout, and rainbowfish (Melanotaenia fluviatilis) belonged to Euteleostei species, in which the vasa transcripts failed to localize at the cleavage furrows (Knaut et al., 2002). However, in the other Euteleostei, including turbot (Lin et al., 2012b), ukigori (Saito et al., 2004) and Atlantic cod (Presslauer et al., 2012), vasa mRNA is located at the edge of the first two cleavage furrows, which was similar to that reported in Osteriophysans species, such as zebrafish and carp (Cyprinus carpio) (Knaut et al., 2002). We found that the black rockfish was another new viviparous species that lacked the early localization ability in Euteleostei. Although black rockfish, medaka, Atlantic cod, and turbot were closely related in phylogeny, the vasa RNA early localization modes were totally different. This indicated that early germ plasm distribution and PGCs origination in different species had no direct relationship with evolution.
It has been proven that the 3′UTR of vasa plays a critical role for the stabilization of chimeric RNA in PGCs but not in somatic cells (Knaut et al., 2002; Wolke et al., 2002; Kataoka et al., 2006; Mishima et al., 2006). Visualization fish PGCs in vivo could be achieved by chimeric RNA fused fluorescent protein coding sequence to vasa 3′UTR (Knaut et al., 2002; Lin et al., 2012a; Ye et al., 2016). This labeling function of vasa 3′UTR was relatively conservative, and the PGCs of one species could be labeled by 3′UTRs from highly diverged taxonomic groups (Yoshizaki et al., 2005; Saito et al., 2006; Zhou et al., 2019). For example, PGCs of rainbow trout could be visualized by vasa 3′UTRs from both Nibe croaker (Nibea mitsukurii) and zebrafish (Yoshizaki et al., 2005). Also, the turbot PGCs could be traced by vasa 3′UTR from zebrafish (Zhou et al., 2019). However, the chimeric mRNA of the medaka (GFP-olvasa 3′UTR mRNA) failed to label the PGCs in zebrafish or loach (Misgurnus anguillicaudatus) embryos (Saito et al., 2006). The previous study proved that zebrafish had a 180-nucleotide element that encompassed the second conserved region of the vasa 3′UTR, which was sufficient for vasa mRNA localization to the germ plasm, while medaka lacked this region (Knaut et al., 2002). In this study, we firstly discovered that the vasa 3′UTR of black rockfish could be used to mark both zebrafish with early localization and medaka without early localization, while the vasa 3′UTR of red seabream and marine medaka that lacked early localization (article in publishing) failed to trace the PGCs of zebrafish. These results indicated that the vasa 3′UTRs of teleost showed a difference in labeling zebrafish PGCs, which may depend on the specific functional motifs in 3′UTR.
Therefore, we compared the twenty most important motifs of vasa 3′UTRs between black rockfish and other four typical fish species. We discovered that, although the black rockfish had the further genetic relationship with zebrafish than other four species in phylogeny, besides some basal motifs similar to the other four specie, there were three motifs of M10, M12, and M19 specifically presented in zebrafish which only existed in black rockfish rather than the other three species. These three specific motifs might partially explain why only the vasa 3′UTR of black rockfish was able to successfully trace PGCs in zebrafish. All of the above results also indicated that the vasa 3′UTR must possess the essential motifs that play an important role in tracing the PGCs instead of phylogenetic relationships.
In conclusion, we described the expression pattern of Ssvas during germ cell origination, development, and maturation process. We firstly reported a viviparous marine economic fish species which failed in early localization until the late gastrula stage. Interestingly, the vasa 3′UTR of black rockfish could be used to label PGCs of zebrafish and marine medaka, representing two different typical origination and migration patterns. Compared to other three Euteleostei species, we found that besides some basal motifs, black rockfish had three motifs just presented in zebrafish. Therefore, we speculated that these motifs might play an important role in labeling zebrafish PGCs. The results of this study will improve our understanding of the localization of the germplasm component and the origination of PGCs in teleost and facilitate germ cell manipulation.
Data Availability Statement
All datasets presented in this study are included in the article/supplementary material.
Ethics Statement
The animal study was reviewed and approved by the Institutional Animal Care and Use Committee, Institute of Oceanology, Chinese Academy of Sciences.
Author Contributions
LZ, QL, and JL conceived and designed the study. LZ and SD contributed to the experimental work. LZ and QL contributed to the manuscript writing and revision, respectively. XW, HZ, TD, JY, LW, and ZS contributed with the experimental materials. All authors read and approved the final manuscript.
Funding
This research was funded by the National Key Research and Development Program (2018YFD0901205 and 2018YFD0901204), Shandong Province Key R&D Projects (2019GHY112013), Shandong Province Major Science and Technology Innovation Project (2018SDKJ0302-4), and China Agriculture Research System (CARS-47).
Conflict of Interest
ZS was employed by the company Weihai Shenghang Aquatic Product Science and Technology Co., Ltd.
The remaining authors declare that the research was conducted in the absence of any commercial or financial relationships that could be construed as a potential conflict of interest.
Footnotes
References
Bailey, T. L., and Elkan, C. (1994). Fitting a mixture model by expectation maximization to discover motifs in biopolymers. Proc. Int. Conf. Intell. Syst. Mol. Biol. 2, 28–36.
Braat, A. K., Speksnijder, J. E., and Zivkovic, D. (1999). Germ line development in fishes. Int. J. Dev. Biol. 43, 745–760. doi: 10.1007/s11626-999-0044-2
Fernandez, J. A., Bubner, E. J., Takeuchi, Y., Yoshizaki, G., Wang, T., Cummins, S. F., et al. (2015). Primordial germ cell migration in the yellowtail kingfish (Seriola lalandi) and identification of stromal cell-derived factor 1. Gen. Comp. Endocrinol. 213, 16–23. doi: 10.1016/j.ygcen.2015.02.007
Gao, Z., Wang, H. P., Rapp, D., O’Bryant, P., Wallat, G., Wang, W., et al. (2009). Gonadal sex differentiation in the bluegill sunfish Lepomis crochirus and its relation to fish size and age. Aquaculture 294, 138–146. doi: 10.1016/j.aquaculture.2009.05.024
Goto-Kazeto, R., Saito, T., Takagi, M., Arai, K., and Yamaha, E. (2010). Isolation of teleost primordial germ cells using flow cytometry. Int. J. Dev. Biol. 54, 1485–1490. doi: 10.1387/ijdb.092914rg
Hartung, O., Forbes, M. M., and Marlow, F. L. (2014). Zebrafish vasa is required for germ-cell differentiation and maintenance. Mol. Reprod. Dev. 81, 946–961. doi: 10.1002/mrd.22414
Hong, N., Li, M. Y., Yuan, Y. M., Wang, T. S., Yi, M. S., Xu, H., et al. (2016). Dnd is a critical specifier of primordial germ cells in the medaka fish. Stem Cell Rep. 6, 411–421. doi: 10.1016/j.stemcr.2016.01.002
Kai, Y., and Soes, D. M. (2009). A record of Sebastes schlegelii Hilgendorf, 1880 from Dutch coastal waters. Aquat. Invas. 4, 417–419. doi: 10.3391/ai.2009.4.2.23
Kataoka, K., Yamaguchi, T., Orii, H., Tazaki, A., Watanabe, K., and Mochii, M. (2006). Visualization of the Xenopus primordial germ cells using a green fluorescent protein controlled by cis elements of the 3′ untranslated region of the DEADSouth gene. Mech. Dev. 123, 746–760. doi: 10.1016/j.mod.2006.07.006
Knaut, H., Pelegri, F., Bohmann, K., Schwarz, H., and Nüsslein-Volhard, C. (2000). Zebrafish vasa RNA but not its protein is a component of the germ plasm and segregates asymmetrically before germline specification. J. Cell Biol. 149, 875–888. doi: 10.1083/jcb.149.4.875
Knaut, H., Steinbeisser, H., Schwarz, H., and Nüsslein-Volhard, C. (2002). An evolutionary conserved region in the vasa 3′ UTR targets RNA translation to the germ cells in the zebrafish. Curr. Biol. 12, 454–466. doi: 10.1016/S0960-9822(02)00723-6
Kobayashi, T., Kajiura-Kobayashi, H., and Nagahama, Y. (2000). Differential expression of vasa homologue gene in the germ cells during oogenesis and spermatogenesis in a teleost fish, tilapia. Oreochromis niloticus. Mech. Dev. 99, 139–142. doi: 10.1016/S0925-4773(00)00464-0
Li, M. Y., Hong, N., Xu, H. Y., Yi, M. S., Li, C. M., Gui, J. F., et al. (2009). Medaka vasa is required for migration but not survival of primordial germ cells. Mech. Dev. 126, 366–381. doi: 10.1016/j.mod.2009.02.004
Lin, F., Xu, S. H., Ma, D. Y., Xiao, Z. Z., Zhao, C. Y., Xiao, Y. S., et al. (2012b). Germ line specific expression of a vasa homologue gene in turbot (Scophthalmus maximus): evidence for vasa localization at cleavage furrows in euteleostei. Mol. Reprod. Dev. 79, 803–813. doi: 10.1002/mrd.22120
Lin, F., Liu, Q. H., Li, M. Y., Li, Z. D., Hong, N., Li, J., et al. (2012a). Transient and stable GFP expression in germ cells by the vasa regulatory sequences from the red seabream (Pagrus major). Int. J. Biol. Sci. 8, 882–890. doi: 10.7150/ijbs.4421
Lindeman, R. E., and Pelegri, F. (2010). Vertebrate maternal-effect genes: insights into fertilization, early cleavage divisions, and germ cell determinant localization from studies in the zebrafish. Mol. Reprod. Dev. 77, 299–313. doi: 10.1002/mrd.21128
Liu, Q. H., Wang, X. Y., Xiao, Y. S., Zhao, H. X., Xu, S. H., Wang, Y. F., et al. (2019). Sequencing of the black rockfish chromosomal genome provides insight into sperm storage in the female ovary. DNA Res. 26, 453–464. doi: 10.1093/dnares/dsz023
Mishima, Y., Giraldez, A. J., Takeda, Y., Fujiwara, T., Sakamoto, H., Schier, A. F., et al. (2006). Differential regulation of germline mRNAs in soma and germ cells by zebrafish miR-430. Curr. Biol. 16, 2135–2142. doi: 10.1016/j.cub.2006.08.086
Mu, W. J., Wen, H. S., He, F., Li, J. F., Liu, M., Ma, R. Q., et al. (2013). Cloning and expression analysis of vasa during the reproductive cycle of Korean rockfish, Sebastes schlegeli. J. Ocean U. China 12, 115–124. doi: 10.1007/s11802-013-1941-2
Nishimura, T., and Tanaka, M. (2014). Gonadal development in fish. Sex. Dev. 8, 252–261. doi: 10.1159/000364924
Okutsu, T., Yano, A., Nagasawa, K., Shikina, S., Kobayashi, T., Takeuchi, Y., et al. (2006). Manipulation of fish germ cell: visualization, cryopreservation and transplantation. J. Reprod. Dev. 52, 685–693. doi: 10.1262/jrd.18096
Piferrer, F. (2001). Endocrine sex control strategies for the feminization of teleost fish. Aquaculture 197, 229–281. doi: 10.1016/B978-0-444-50913-0.50014-X
Presslauer, C., Nagasawa, K., Fernandes, J. M. O., and Babiak, I. (2012). Expression of vasa and nanos3 during primordial germ cell formation and migration in Atlantic cod (Gadus morhua L.). Theriogenology 78, 1262–1277. doi: 10.1016/j.theriogenology.2012.05.022
Saito, T., Fujimoto, T., Maegawa, S., Inoue, K., Tanaka, M., Arai, K., et al. (2006). Visualization of primordial germ cells in vivo using GFP-nos1 3′UTR mRNA. Int. J. Dev. Biol. 50, 691–699. doi: 10.1387/ijdb.062143ts
Saito, T., Otani, S., Fujimoto, T., Suzuki, T., Takako Nakatsuji, T., Arai, K., et al. (2004). The germ line lineage in ukigori, Gymnogobius species (Teleostei: Gobiidae) during embryonic development. Int. J. Dev. Biol. 48, 1079–1085. doi: 10.1387/ijdb.041912ts
Saitou, N., and Nei, M. (1987). The neighbor-joining method: a new method for reconstructing phylogenetic trees. Mol. Biol. Evol. 4, 406–425. doi: 10.1266/jjg.61.559
Shinomiya, A., Tanaka, M., Kobayashi, T., Nagahama, Y., and Hamaguchi, S. (2000). The vasa-like gene, olvas, identifies the migration path of primordial germ cells during embryonic body formation stage in the medaka, Oryzias latipes. Dev. Growth Differ. 42, 317–326. doi: 10.1046/j.1440-169x.2000.00521.x
Takeuchi, Y., Yoshizaki, G., and Takeuchi, T. (2004). Biotechnology: surrogate broodstock produces salmonids. Nature 430, 629–630. doi: 10.1038/430629a
Wolke, U., Weidinger, G., Köprunner, M., and Raz, E. (2002). Multiple levels of posttranscriptional control lead to germ line-specific gene expression in the zebrafish. Curr. Biol. 12, 289–294. doi: 10.1016/s0960-9822(02)00679-6
Xu, H. Y., Li, M. Y., Gui, J. F., and Hong, Y. H. (2007). Cloning and expression of medaka dazl during embryogenesis and gametogenesis. Gene Exp. Patterns 7, 332–338. doi: 10.1016/j.modgep.2006.08.001
Xu, H. Y., Li, M. Y., Gui, J. F., and Hong, Y. H. (2010). Fish germ cells. Sci. China Life Sci. 53, 435–446. doi: 10.1007/s11427-010-0058-8
Yang, Y., Liu, Q. H., Xiao, Y. S., Wang, X. Y., An, H., Song, Z. C., et al. (2018). Germ cell migration, proliferation and differentiation during gonadal morphogenesis in all-female japanese flounder (paralichthys olivaceus). Anat. Rec. 301, 727–741. doi: 10.1002/ar.23698
Ye, H., Yue, H. M., Yang, X. G., Li, C. J., and Wei, Q. W. (2016). Identification and sexually dimorphic expression of vasa isoforms in Dabry’ s sturgeon (Acipenser dabryanus), and functional analysis of vasa 3′-untranslated region. Cell Tissue Res. 366, 203–218. doi: 10.1007/s00441-016-2418-6
Yoon, C., Kawakami, K., and Hopkins, N. (1997). Zebrafish vasa homologue RNA is localized to the cleavage planes of 2- and 4-cell-stage embryos and is expressed in the primordial germ cells. Development 124, 3157–3165. doi: 10.1007/s004290050089
Yoshizaki, G., Sakatani, S., Tominaga, H., and Takeuchi, T. (2010). Cloning and characterization of a vasa-like gene in rainbow trout and its expression in the germ cell lineage. Mol. Reprod. Dev. 55, 364–371. doi: 10.1002/(SICI)1098-2795(200004)55:43.0.CO
Yoshizaki, G., Tago, Y., Takeuchi, Y., Sawatari, E., Kobayashi, T., and Takeuchi, T. (2005). Green fluorescent protein labeling of primordial germ cells using a nontransgenic method and its application for germ cell transplantation in salmonidae. Biol. Reprod. 73, 88–93. doi: 10.1095/biolreprod.104.034249
Zhao, C. Y., Xu, S. H., Liu, Y. F., Wang, Y. F., Liu, Q. H., and Li, J. (2017). Gonadogenesis analysis and sex differentiation in cultured turbot (Scophthalmus maximus). Fish Physiol. Biochem. 43, 265–278. doi: 10.1007/s10695-016-0284-5
Zhou, L., Wang, X. Y., Liu, Q. H., Xu, S. H., Zhao, H. X., Han, M. M., et al. (2019). Visualization of turbot (Scophthalmus maximus) primordial germ cells in vivo using fluorescent protein mediated by the 3′ untranslated region of nanos3 or vasa gene. Mar. Biotechnol. 21, 671–682. doi: 10.1007/s10126-019-09911-z
Keywords: vasa, Sebastes schlegelii, gonad, germ cell, development, 3cpsdummy′UTR, localization
Citation: Zhou L, Wang X, Du S, Wang Y, Zhao H, Du T, Yu J, Wu L, Song Z, Liu Q and Li J (2020) Germline Specific Expression of a vasa Homologue Gene in the Viviparous Fish Black Rockfish (Sebastes schlegelii) and Functional Analysis of the vasa 3′ Untranslated Region. Front. Cell Dev. Biol. 8:575788. doi: 10.3389/fcell.2020.575788
Received: 24 June 2020; Accepted: 18 September 2020;
Published: 28 October 2020.
Edited by:
Miguel Angel Brieño-Enriquez, Magee-Womens Research Institute, United StatesReviewed by:
Mingyou Li, Shanghai Ocean University, ChinaJelena Lujic, Cornell University, United States
Copyright © 2020 Zhou, Wang, Du, Wang, Zhao, Du, Yu, Wu, Song, Liu and Li. This is an open-access article distributed under the terms of the Creative Commons Attribution License (CC BY). The use, distribution or reproduction in other forums is permitted, provided the original author(s) and the copyright owner(s) are credited and that the original publication in this journal is cited, in accordance with accepted academic practice. No use, distribution or reproduction is permitted which does not comply with these terms.
*Correspondence: Qinghua Liu, qinghualiu@qdio.ac.cn; Jun Li, junli@qdio.ac.cn