Septins as membrane influencers: direct play or in association with other cytoskeleton partners
- 1INSERM UMR-S 1193, UFR de Pharmacie, University Paris-Saclay, Orsay, France
- 2Laboratoire de Biochimie-Hormonologie, Hôpital Antoine Béclère, AP-HP, Hôpitaux Universitaires Paris-Saclay, Clamart, France
The cytoskeleton comprises three polymerizing structures that have been studied for a long time, actin microfilaments, microtubules and intermediate filaments, plus more recently investigated dynamic assemblies like septins or the endocytic-sorting complex required for transport (ESCRT) complex. These filament-forming proteins control several cell functions through crosstalks with each other and with membranes. In this review, we report recent works that address how septins bind to membranes, and influence their shaping, organization, properties and functions, either by binding to them directly or indirectly through other cytoskeleton elements.
Introduction
Septins constitute a family of GTPases considered as the fourth element of the cytoskeleton (Mostowy and Cossart, 2012). They are conserved from fungi to animals but are absent from plants (Shuman and Momany, 2022). According to their structure homology, septins are clustered into four classes, among which they are interchangeable (Kinoshita, 2003). Septins assemble into palindromic hetero-oligomers (Sirajuddin et al., 2007) with a subunit order that has recently been revised within human septin hexamers or octamers (McMurray and Thorner, 2019; Mendonça et al., 2019; Soroor et al., 2021) (Figure 1A). These complexes organize into filaments by annealing (Bridges et al., 2014) to form bundles, gauzes or rings depending on the cell context (Cavini et al., 2021; Woods and Gladfelter, 2021). These filaments are non-polar and do not behave as tracks for dedicated motors. However, they are dynamic since they can exchange internal segments and reorganize (Schmidt and Nichols, 2004). Septin filaments often coalign with membranes, actin fibers or in some circumstances with microtubules, and mostly act as diffusion barriers or scaffolds to perform their functions (Spiliotis and McMurray, 2020; Spiliotis and Kesisova, 2021; Spiliotis and Nakos, 2021). As recently suggested for the control of neuronal trafficking, a “septin code” including genetic and post-translational modification diversity might explain the spatiotemporal adaptation to cell types or to local needs (Spiliotis and Nakos, 2021).
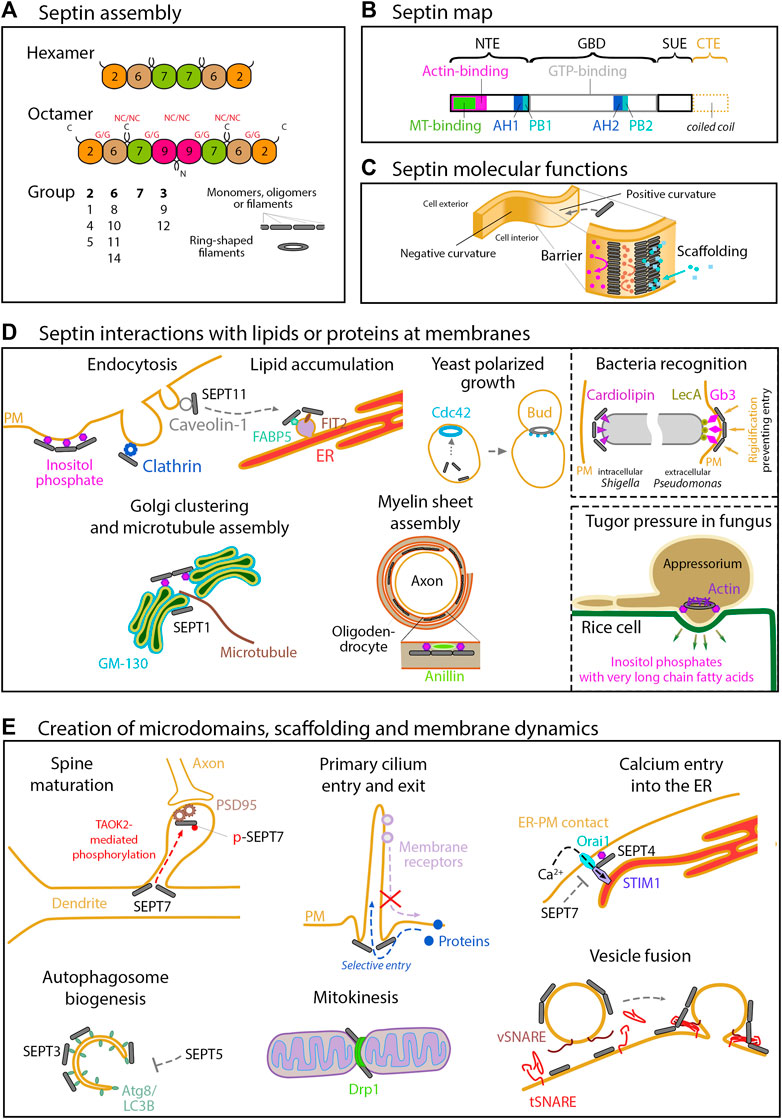
FIGURE 1. Septin structure, assembly and functions related to membranes. (A) Assembly of the septin cytoskeleton. In humans, the septin family comprises 13 members organized into 4 groups (2, 6, 7 and 3) according to their sequence homology. The organization of the four septin group into hexamers and octamers is depicted. Septin monomers either interact through their N- and C-terminal domains (NC/NC interfaces), or through their GTP-binding domain (G/G interfaces). These oligomers can then assemble into filaments or into rings. (B) Structural domains encountered in septin proteins. All the septins exhibit a GTP-binding domain (GBD), a septin unique element (SUE), and N- and C-terminal extensions (NTE, CTE) of variable length. Note that the CTE, which contains one or more coiled-coil regions is absent in group 3 septins. Membrane binding is mediated by two AH (amphipathic helix) and PB (polybasic domain) motifs. SEPT9 exhibits physical binding to F-actin through the first half of its NTE, and to microtubules through its N-terminal K/R-R/x-x-D/E repeat motifs. (C) Septin molecular functions. At the molecular level, septins can form diffusion barriers or act as scaffolds, as illustrated here in the context of binding to a positively curved membrane. As a barrier, septins can either restrict entry or concentrate molecules within a given domain. (D) Septins interact with lipids or proteins at membranes. Septins are enriched at positively curved membranes by binding to phosphoinositides. Through binding to other specific lipids (cardiolipin, Gb3) in bacteria, and through binding to very long chain fatty acid-bearing phosphoinositides in M. orizae fungus, septins ultimately regulate pathogen invasion. In yeast, the membrane recruitment of septins follows high concentrations of active Cdc42, directing further outgrowth of the bud. Through binding to specific proteins (Clathrin, Caveolin, FABP5, FSIT2, Anillin or GM130), septins are involved in a large array of cell processes in higher eukaryotes, including endocytosis, lipid storage, myelin sheet assembly, Golgi integrity and function in nucleating microtubules. (E) Septins can act as barriers or scaffolds at membranes. SEPT7 phosphorylation mediates PSD95 stability and restricts its mobility to favor dendritic maturation. At the base of primary cilia, they control protein entry and retain signaling receptors. Septins also organize endoplasmic reticulum-plasma membrane junctions for STIM1-Orai1 calcium signaling. Septins are regulators of autophagy, especially in yeast and neurons, where an interaction with Atg8 has been observed. SEPT2 can recruit Drp1 to mitochondrial constriction sites to favor Drp1-mediated fission. Septins also contribute to control vesicular trafficking machinery at several steps, including the SNARE-mediated membrane fusion.
Here, we emphasize recent data that report a septin impact on membranes, either by direct interactions, or by their association with other cytoskeleton elements (Figures 1, 2). As the loss/manipulation of a distinct septin can profoundly impair filament/oligomer integrity per se, note that in some studies it remains open if the observed effects can be attributed to the septin under study or rather to septin oligomers/filaments.
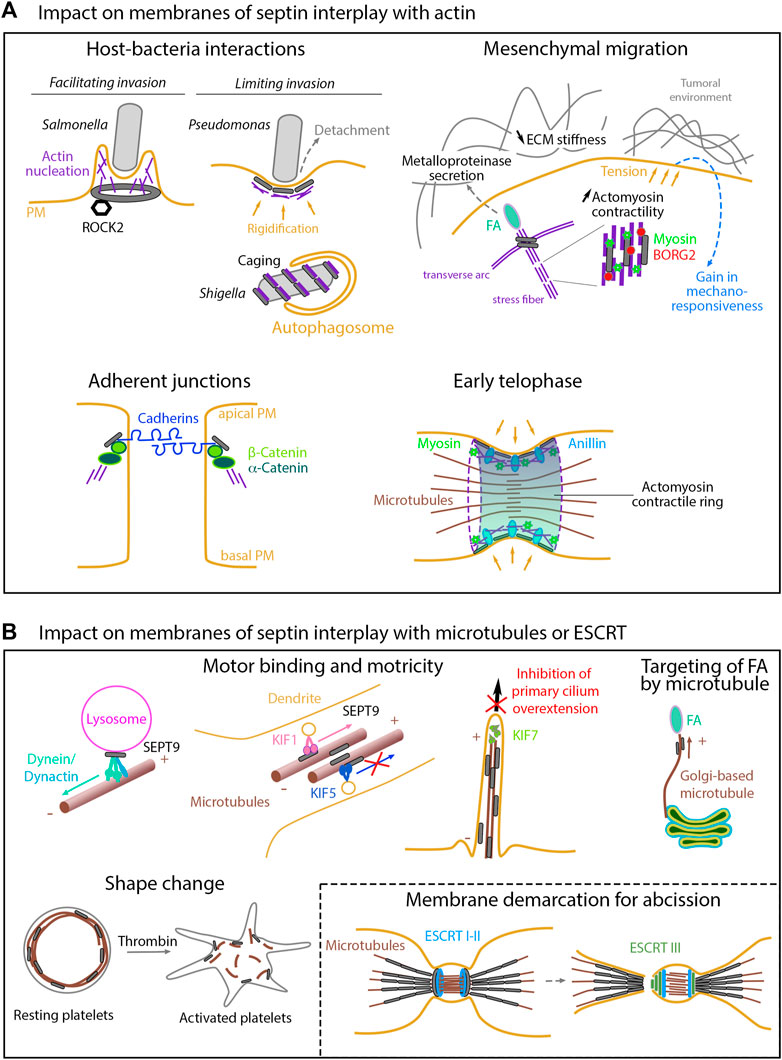
FIGURE 2. Impact on membranes of septin interplay with other cytoskeletons (A) Actin-related impacts. While facilitating cell invasion of specific bacteria, septins can also limit invasion either by enhancing host cell rigidity or by favoring autophagy-mediated degradation once bacteria have been internalized. During mesenchymal migration, septins contribute to the stabilization of nascent focal adhesions and to extracellular matrix degradation through metalloproteinase secretion. Septin-mediated enhancement of actomyosin contractility ultimately increase cell tension forces, thereby impacting the tumoral environment and modulating mechano-responsiveness. By linking the catenin complex to the actin cytoskeleton, septins favor cell-cell junctions required for the apico- basal polarity. During cytokinesis, membrane-associated septins and anillin are required to anchor the actomyosin contractile ring to the cell cortex. (B) Microtubule- and ESCRT-related impacts. Septins control the binding and motricity of molecular motors, thereby regulating the retrograde and anterograde transport on microtubules of proteins or vesicles, as illustrated with the clustering of lysosomes during cell adaptation to stress, the sorting of kinesin motor cargoes during entry into dendrites, or the control of cilia over-elongation. By guiding non-centrosomal Golgi-based microtubules, septins are also involved in focal adhesion disassembly in migrating cells. Delocalization of septins from microtubules impacts cell shape, as illustrated in platelets. During cytokinesis, membrane-bound septin rings demarcate the membrane sites for ESCRT III ring assembly prior to final abscission.
Direct influence of septins on membranes
Septin recruitment at plasma membrane (PM) but also at organelle or pathogen membranes relies on known interactions with lipids and mostly takes place at specific curved regions. Their principal role is to create membrane subdomains of defined composition and function to control membrane protrusion, fusion, fission, polarity, rigidity, traffic and signaling.
Recruitment by curved membranes and lipids
Cell membrane curvatures range from nanometer to micrometer scales. Only a few proteins, including septins, can interact specifically with micrometric curvatures (Bridges et al., 2016), which are found mostly at the cytokinetic furrow and at the base of cell protrusions. In vitro, septin polymers lie flat along the hill axis of positive/convex curvatures while they bend orthogonally in negative/concave curvatures (in valleys), explaining the higher septin density on positive geometries in cells (Beber et al., 2019b). Septin binding to positively curved membranes relies on their amphipathic helix (AH) domains (Cannon et al., 2019; Lobato-Márquez et al., 2021; Woods et al., 2021), and on their interaction with phosphoinositides, especially PI(4,5)P2, which enhances septin arrangement into filaments (Zhang et al., 1999; Bertin et al., 2010; Beber et al., 2019a). Indeed, adjacent to the AH domains, human septins harbor two polybasic domains, which further enrich phosphoinositide-containing membranes with septins (Omrane et al., 2019). In addition, it was shown that phosphoinositides containing very-long-chain fatty acids could organize septins at the membrane of the M. oryzae fungus to mediate appressorium-dependent infection of rice cells (He et al., 2020). Reciprocally, septins may control the content in phosphoinositides, since the Drosophila Peanut/SEPT7 decreases the PIP5 kinase activity and the resulting PI(4,5)P2 resynthesis (Kumari et al., 2022).
Besides phosphoinositides, septins can also bind to other anionic lipids such as cardiolipin (at Shigella poles) (Krokowski et al., 2018), to sterol-rich membrane domains (to contribute to the cell wall integrity response following membrane stress in Aspergillus) (Mela and Momany, 2021) or to LecA-activated Gb3 (globotriaosylceramide)-containing membranes (to rigidify the host membrane and prevent Pseudomonas invasion following its attachment to the host cell PM) (Aigal et al., 2022). Additionally, the protein Cdc42 at PM and its regulators define the recruitment site of septins to control bud emergence in fungi (Chollet et al., 2020; Zheng et al., 2022). Reciprocally, the other septin partners, which are gradually discovered (Benoit et al., 2021), are rather organized via the septin roles of diffusion barrier or scaffold, either at the plasma membrane, or at the membranes of the organelles.
Septin functions at the plasma membrane
Membrane protrusions and invaginations
Once bound to membranes, septins can reshape them by promoting protrusions (Beber et al., 2019b). Indeed, in endothelial cells, the SEPT2 diffusive barrier that assembles at the base of podosomes facilitates their maturation (Collins et al., 2020). In fungi, membrane-bound septins define new regions of hyphal growth, by forming rings at the base of new lateral branches (Li et al., 2012; Feng et al., 2017). The phospholipid-binding Nim1 kinase Gin4, known to be essential for septin ring assembly at the bud neck (Longtine et al., 1998), is also required for hyphal growth of C. albicans (Au Yong et al., 2016). Also, septin filaments lie at the base of primary cilia where they control protein entry (Palander et al., 2017) and retain signaling receptors (Hu et al., 2010). SEPT9 builds a barrier around clathrin-rich patches to stimulate endocytosis and bone resorption by osteoclasts (Møller et al., 2018). Following oleate treatment, caveolin-bound SEPT11 relocates from caveolae of adipocytes to lipid droplets and bind the fatty acid chaperone FABP5 to contribute to insulin signaling and lipid accumulation (Moreno-Castellanos et al., 2017).
Exocytosis
Septins influence exocytic vesicle fusion with the PM (Yang et al., 2010), as evidenced for synaptic vesicles, GLUT4 storage vesicles in podocytes and lytic granules in natural killer cells, through interactions with the SNARE machinery (Beites et al., 1999; Tokhtaeva et al., 2015; Wasik et al., 2017; Phatarpekar et al., 2020). Also, septins of the M. oryzae fungus assemble the exocyst-tethering complex at the pore of the leave-invading structure (the appressorium) to allow local exocytosis and rice infection (Gupta et al., 2015). In this process, the osmo-sensor kinase Sln1 is required for septin ring organization and correct localization of the exocyst component Exo70 (Ryder et al., 2019).
Cell polarity and signaling
In neurons, septin-mediated polarity establishment occurs through membrane compartmentalization processes (Radler and Spiliotis, 2022). For example, septins demarcate the cortical sites of neurite retraction before mitosis to ensure their proper re-emergence location in daughter neurons, thereby maintaining polarity inheritance (Boubakar et al., 2017). Septins stabilize submembranous ankyrins at the axon initial segment for the control of neuronal polarity (Hamdan et al., 2020). Accordingly, in hematopoietic stem cells, the BORG4 protein, belonging to the Cdc42-regulated BORG family known to regulate the septin network (Joberty et al., 2001), compartmentalizes polarity proteins when complexed with SEPT7 (Kandi et al., 2021).
Concerning signaling, the relocalization of SEPT7 following its phosphorylation, from the base of the protrusion to the spine head membranes, restricts PSD95 protein motility to promote dendritic spine maturation (Yadav et al., 2017; Byeon et al., 2022). In astrocytes, septins interact with the glial glutamate transporter GLT-1 to reduce its mobility, and together with Cdc42EP4/BORG4 form patches that influence synaptic activity (Piniella et al., 2018). More broadly, by controlling membrane receptor localization, septins are involved in the regulation of synaptic vesicle trafficking and neurotransmitter release (Ageta-Ishihara et al., 2015; Marttinen et al., 2015). Generally, septins control signaling in diverse cell types by regulating the degradation of PM receptors, such as receptor tyrosine kinases (Vagin and Beenhouwer, 2016).
Septin functions at subcellular organelles
Organelle biogenesis
SEPT7 association with fat storage-inducing transmembrane 2 protein (FIT2) at the endoplasmic reticulum (ER) is essential for lipid droplet biogenesis (Chen et al., 2021). Autophagosome formation in budding yeast involves septin ring-like structure formation at pre-autophagosomal structures and at the membrane of mature autophagosomes via Atg8 and Atg9 (Barve et al., 2018a; 2018b). More precisely, in neurons, Atg8/LC3B binds SEPT3 to promote autophagosome biogenesis, while SEPT5 acts as a negative regulator (Marttinen et al., 2020; Ferreira et al., 2022; Tóth et al., 2022).
Organelle fusion and fission
Septins can localize to mitochondria fission sites, where they bind to Drp1 and promote its recruitment to enhance mitokinesis, both in mammals and in C. elegans (Pagliuso et al., 2016; Sirianni et al., 2016). SEPT9 also regulates lysosome localization before their fusion with lipid droplets (Song et al., 2022). Similarly, septins are required for the fusion of macropinosomes with endosomes/lysosomes (Dolat and Spiliotis, 2016) and of entrapped Shigella with lysosomes (Krokowski et al., 2018).
Partitioning at the ER
In budding yeast, septins confine proteotoxic misfolded proteins upon ER stress in the mother compartment during cell division (Clay et al., 2014). Similarly, the septin Shs1 creates an ER barrier at the bud neck to confine the spindle capture protein Num1 to the mother cortical ER (Chao et al., 2014). More generally, as they partition the cortical ER-PM contacts, septins restrict the flow of peripheral proteins between the mother and daughter cells (Sugiyama and Tanaka, 2019). In mammals, SEPT4 regulates the number of ER-PM junctions and locally enhances the interactions between the ER Ca2+ sensor STromal Interaction Molecule 1 (STIM1) and the PM Ca2+ channel Orai1 (Katz et al., 2019; De Souza et al., 2021). By contrast, SEPT7 negatively regulates extracellular Ca2+ entry in neuronal ER at ER-PM contacts, by preventing Orai1/STIM1 colocalization (Deb et al., 2020; Serwach and Gruszczynska-Biegala, 2020). Also, in mouse Purkinje neurons, STIM1 deficiency can be rescued by SEPT7 loss (Dhanya and Hasan, 2021).
Impact of septin/actin crosstalk on membrane organization and functions
Septins often coalign with actin stress fibers with which they interact either directly (Smith et al., 2015) or via actin binding proteins (Spiliotis, 2018). They regulate actin polymerization, stability and bundling, and their depletion or relocalization triggers the thinning of actin stress fibers (Kinoshita et al., 2002; Schmidt and Nichols, 2004; Salameh et al., 2021; Kuzmić et al., 2022), with expected impact on PM remodeling, focal adhesion (FA) turnover, cell migration, and actomyosin contractility (Lam and Calvo, 2019; Spiliotis and Nakos, 2021).
Control of cell shape and cortical rigidity
While septins are excluded from the actin meshwork in PM ruffles, their presence at specific membrane domains stimulates actin bundling and stabilization (Mavrakis et al., 2014; Smith et al., 2015; Iv et al., 2021), increasing cell rigidity (Gilden and Krummel, 2010). During Drosophila wing morphogenesis, septin-mediated actin bundling is required to build up compact microtubule-actin cables into PM projections to reinforce cell-matrix adhesion (Sun et al., 2021). Septin-enhanced PM rigidity also occurs during cell rounding at the transition between interphase and mitosis (Vadnjal et al., 2022). Also, PI(4,5)P2 and the actin-binding protein anillin control septin recruitment to the periaxonal oligodendrocyte membranes to organize myelin sheaths and ensure normal nerve conduction (Patzig et al., 2016; Erwig et al., 2019).
Pathogen invasion
Septin-mediated control of cortical PM rigidity is also used by host cells to prevent infection by microbial agents (Torraca and Mostowy, 2016; Aigal et al., 2022). If internalization occurs, septins cage bacteria or their actin tail to target them to autophagic degradation (Torraca and Mostowy, 2016; Robertin and Mostowy, 2020). During poxvirus infection, septins entrap the virus at the PM, an antiviral function regulated by actin polymerization (Ngo and Mostowy, 2019). In contrast, septin-mediated activation of actin nucleation is required for efficient Salmonella invasion (Boddy et al., 2018). During rice blast disease, the septin ring at the appressorium pore (Eseola et al., 2021) scaffolds actin and provides proper curvature and cortical rigidity necessary for plant cell invasion (Dagdas et al., 2012; Ryder et al., 2022).
Control of migration
Septins regulate both mesenchymal/lamellipodial and amoeboidal/blebbing migration modes. In the lamella, septins localize at the interface of dorsal and transverse actin arcs and stabilize nascent FAs at the PM (Dolat et al., 2014). SEPT9_i1 promotes FA turnover by targeting Rho/ROCK and FAK signaling (Zeng et al., 2019), and long SEPT9_i1-3 isoforms favor metalloprotease secretion at FAs to promote extracellular matrix degradation (Marcus et al., 2019). By contrast, SEPT7 and SEPT9_i2 inhibit cell migration through actin filament disassembly (Hou et al., 2016; Verdier-Pinard et al., 2017). During amoeboid migration, septin-mediated actomyosin contractility controls PM retraction (Gilden et al., 2012), cortical rigidity, directionality (Tooley et al., 2009), and attributable to SEPT9, outside of the filamentous context, invasion and metastasis (Farrugia et al., 2020).
In cancer-associated fibroblasts, the Cdc42EP3/BORG2-dependent dense actomyosin and septin network allows high contractility and increased mechano-responsiveness to changes in extracellular matrix stiffness (Calvo et al., 2015). Regarding mechanotransduction, upregulated SEPT6 drives hepatocellular carcinoma cell proliferation, migration and invasion via the actin/Hippo/YAP-signaling pathway (Fan et al., 2021).
Cytokinesis achievement
During cytokinesis, the rearrangement of septin filaments at the PM from an actomyosin-associated structure into an anillin-stabilized double ring is required for the cortical compartmentalization of cytokinetic factors, maturation of the intercellular bridge (ICB), and actomyosin constriction. Cytokinetic progression is probably the process for which the molecular mechanisms underlying the spatiotemporal septin functions and remodeling into higher-order structures are the best known. (Menon and Gaestel, 2015; Marquardt et al., 2019, 2020, 2021; Carim et al., 2020; Chen et al., 2020; Piatti, 2020; Russo and Krauss, 2021). Septin compaction into two rings is mediated by anillin (Chen et al., 2020; Arbizzani et al., 2022), a known adaptor that recruits septins on F-actin (Kinoshita et al., 2002) and on PI(4,5)P2 (Liu et al., 2012). Also, the ICB assembly relies on the recognition of specific septin units by the CIN85/anillin adaptor complex (Panagiotou et al., 2022). Furthermore, increasing tissue stiffness to mimic a tumoral environment correlates with higher expression of both anillin and SEPT6, leading to abscission failure in cells undergoing epithelial to mesenchymal transition (Simi et al., 2018; Rabie et al., 2021). Besides, the Hof1-septin scaffold directly binds and organizes actin cable spacing to ensure yeast cytokinesis (Garabedian et al., 2020).
To ensure actin contractility during division, mammalian SEPT2 scaffolds myosin II and its kinases (Joo et al., 2007). SEPT9 colocalizes with actomyosin to generate the site of membrane abscission and stabilizes the PM curvature (Wang et al., 2019). Eventually, cytokinesis completion requires SEPT6, SEPT7 and SEPT11 SUMOylation to keep their colocalization with actin and to prevent abnormal septin-bundling in the ICB (Ribet et al., 2017). Moreover, during embryogenesis, actomyosin-anchored septins are required for anaphase chiral cortical rotation in C. elegans zygote (Zaatri et al., 2021) and for generating submembranous curved and packed actomyosin networks during fly blastoderm cellularization, a specialized form of cytokinesis (Mavrakis et al., 2014; Xue and Sokac, 2016).
Cell junction integrity
Septins are also important at cell junctions. During epithelial cyst morphogenesis, they localize to the lateral PM, where they link E-cadherin to beta-catenin, recruit actin filaments and favor adhesion junction and apico-basal polarity (Wang et al., 2021). In endothelial monolayers, localization of SEPT2 at the PM is required for the integrity of adherens and tight junctions to build the vascular barrier (Kim and Cooper, 2018, 2021). SEPT9 also interacts with the atypical cadherin DCHS1 to direct filamentous actin organization and cell-to-extracellular alignment in the cardiac valve (Moore et al., 2022).
Impact of septin/microtubule interplay on membrane organization and functions
In addition to membranes and actin, septins can also bind to microtubules and to a variety of microtubule-associated proteins to control transport, cell shape, growth or adhesion (Bai et al., 2013; Spiliotis, 2018; Spiliotis and Kesisova, 2021; Spiliotis and Nakos, 2021).
Control of cell shape, adhesion and migration
In Xenopus embryos, SEPT7-mediated reorientation of microtubules along the PM is required for cell elongation during wound closure (Shindo et al., 2018). Strikingly, SEPT9 is one of the five proteins that mediate crosstalk between actin, FA and microtubules (Paradžik et al., 2022). By analogy, the C. difficile bacteria, which produce a toxin that locally depolymerizes cellular actin, cause septin-dependent formation of microtubule-based protrusions that enhance bacterial adhesion (Nölke et al., 2016). At the Golgi, PI4P recruits SEPT9 to perform Golgi clustering (Omrane et al., 2019), while GM130/GOLGA2 recruits SEPT1, which, independently of filaments, performs local microtubule nucleation and stimulates anterograde membrane traffic (Song et al., 2019). Additionally, FA-proximal septin filaments promote Golgi-based non-centrosomal microtubule growth into FAs, thereby regulating FA turnover and cell migration (Merenich et al., 2022).
Regulation of molecular motor binding
In RPE1 primary cilium, septins are required to accumulate the microtubule-capping kinesin KIF7 at the tip, which prevents cilia overextension (Kanamaru et al., 2022). Recent data highlight the control that septins exert over the binding of molecular motors to cargoes or to microtubules. They act as scaffolds for the binding of dynein-dynactin to lysosomes in retrograde transport (Kesisova et al., 2021). Septins also control the entry of cargoes into dendrites via regulating their loading on KIF17 (Bai et al., 2016). In line with this, microtubule-bound SEPT9 specifically prevents KIF5-mediated centrifugal transport of membrane proteins, while it boosts the KIF1-mediated one (Karasmanis et al., 2018). SEPT7 binds to the kinesin KIF20A/MKLP2 in the ICB of dividing neuronal progenitors, to maintain the proliferative state of these cells, which is critical for proper brain development (Qiu et al., 2020). Recently, a hypothetical septin code has been proposed to explain how specific septin polymers differentially recruit kinesin and dynein motors to regulate traffic along microtubule tracks (Spiliotis and Kesisova, 2021).
Impact of subcellular relocalization
Actin-to-microtubule relocalization of SEPT9 following forchlorfenuron/UR214-9 treatment enhances kinesin-microtubule interactions and microtubule bundling into a subcortical giant ring, resulting in a discoid cell architecture in breast cancer cells (Zhovmer et al., 2021). Such a septin-associated microtubule ring is naturally present in resting platelets but dissociates upon their activation (Kim et al., 2022). Accordingly, the redirection of SEPT4 and 8 to the cortex following activation suggests a functional role of at least both septins in platelet granular secretion (Bläser et al., 2004). Moreover, patients bearing a SEPT9 congenital variant suffer from granule secretion defect (Neubauer and Zieger, 2021). Otherwise, octamers that include SEPT9_i1 can relocalize from F-actin to microtubules, which results in a relaxation of peripheral actin stress fibers, affecting actomyosin organization and tension (Kuzmić et al., 2022). Such septin relocalization induces acquired resistance to the microtubule-stabilizing chemotherapy drug paclitaxel (Targa et al., 2019; Salameh et al., 2021).
Impact of septin interplay with ESCRT or intermediate filaments on membranes
During cytokinesis, septins rearrange into two membrane-bound septin rings, which demarcate the membrane sites for ESCRT-III ring assembly into functional cones for final abscission. Indeed, the ESCRT-III recruitment occurs concomitantly with the disassembly of the septin double ring (Addi et al., 2018; Karasmanis et al., 2019; Russo and Krauss, 2021). In non-adherent cells, where ESCRT rings do not assemble, cytokinesis fails because of ICB regression. However, in case of re-adhesion, SEPT7 stabilizes the ingressed PM, thereby restoring tension-mediated rupture in an ESCRT-independent way (Gupta et al., 2018).
At last, intermediate filaments (IFs) have also been identified as binding partners of oncogenic SEPT9 isoforms by proteomic profiling (Devlin et al., 2021). During human spermiogenesis, SEPT12 is recovered in a complex with the IF nucleo-cytoskeleton laminB1 and the nuclear membrane cytoskeleton-linker protein SUN4/SPAG4 during the formation of nuclear envelopes of round spermatids (Yeh et al., 2015). Also, SEPT12 overexpression acts independently of filaments to disrupt laminA/C distribution and sperm morphogenesis (Yeh et al., 2019).
Conclusion
Even though the underlying mechanisms have most often not been elucidated, the direct and indirect roles of septins in membrane organization and functions keep growing, with clear indications that cytoskeleton interplays are key in these processes. Given the pivotal roles of septin filaments in fine-tuning local membrane properties, it seems obvious that their deregulations will be increasingly highlighted in various pathologies, including cancers, neuropathies, infections, ciliopathies, obesity or male sterility.
Author contributions
BB, CP, and AB contributed equally to the writing and conception of figures.
Funding
This work was supported by the Ministère de l’Enseignement Supérieur et de la Recherche and by the Institut National de la Santé et de la Recherche Médicale.
Conflict of interest
The authors declare that the research was conducted in the absence of any commercial or financial relationships that could be construed as a potential conflict of interest.
Publisher’s note
All claims expressed in this article are solely those of the authors and do not necessarily represent those of their affiliated organizations, or those of the publisher, the editors and the reviewers. Any product that may be evaluated in this article, or claim that may be made by its manufacturer, is not guaranteed or endorsed by the publisher.
References
Addi, C., Bai, J., and Echard, A. (2018). Actin, microtubule, septin and ESCRT filament remodeling during late steps of cytokinesis. Curr. Opin. Cell Biol. 50, 27–34. doi:10.1016/j.ceb.2018.01.007
Ageta-Ishihara, N., Yamazaki, M., Konno, K., Nakayama, H., Abe, M., Hashimoto, K., et al. (2015). A CDC42EP4/septin-based perisynaptic glial scaffold facilitates glutamate clearance. Nat. Commun. 6, 10090. doi:10.1038/ncomms10090
Aigal, S., Omidvar, R., Stober, K., Ziegelbauer, J., Eierhoff, T., Schampera, J. N., et al. (2022). Septin barriers protect mammalian host cells against Pseudomonas aeruginosa invasion. Cell Rep. 41, 111510. doi:10.1016/j.celrep.2022.111510
Arbizzani, F., Mavrakis, M., Hoya, M., Ribas, J. C., Brasselet, S., Paoletti, A., et al. (2022). Septin filament compaction into rings requires the anillin Mid2 and contractile ring constriction. Cell Rep. 39, 110722. doi:10.1016/j.celrep.2022.110722
Au Yong, J. Y. A., Wang, Y.-M., and Wang, Y. (2016). The Nim1 kinase Gin4 has distinct domains crucial for septin assembly, phospholipid binding and mitotic exit. J. Cell Sci. 129, 2744–2756. doi:10.1242/jcs.183160
Bai, X., Bowen, J. R., Knox, T. K., Zhou, K., Pendziwiat, M., Kuhlenbäumer, G., et al. (2013). Novel septin 9 repeat motifs altered in neuralgic amyotrophy bind and bundle microtubules. J. Cell Biol. 203, 895–905. doi:10.1083/jcb.201308068
Bai, X., Karasmanis, E. P., and Spiliotis, E. T. (2016). Septin 9 interacts with kinesin KIF17 and interferes with the mechanism of NMDA receptor cargo binding and transport. Mol. Biol. Cell 27, 897–906. doi:10.1091/mbc.e15-07-0493
Barve, G., Sanyal, P., and Manjithaya, R. (2018a). Septin localization and function during autophagy. Curr. Genet. 64, 1037–1041. doi:10.1007/s00294-018-0834-8
Barve, G., Sridhar, S., Aher, A., Sahani, M. H., Chinchwadkar, S., Singh, S., et al. (2018b). Septins are involved at the early stages of macroautophagy in S. cerevisiae. J. Cell Sci. 131, jcs209098. doi:10.1242/jcs.209098
Beber, A., Alqabandi, M., Prévost, C., Viars, F., Lévy, D., Bassereau, P., et al. (2019a). Septin-based readout of PI(4,5)P2 incorporation into membranes of giant unilamellar vesicles. Cytoskeleton 76, 92–103. doi:10.1002/cm.21480
Beber, A., Taveneau, C., Nania, M., Tsai, F.-C., Cicco, A. D., Bassereau, P., et al. (2019b). Membrane reshaping by micrometric curvature sensitive septin filaments. Nat. Commun. 10, 420. doi:10.1038/s41467-019-08344-5
Beites, C. L., Xie, H., Bowser, R., and Trimble, W. S. (1999). The septin CDCrel-1 binds syntaxin and inhibits exocytosis. Nat. Neurosci. 2, 434–439. doi:10.1038/8100
Benoit, B., Baillet, A., and Poüs, C. (2021). Cytoskeleton and associated proteins: Pleiotropic JNK substrates and regulators. Int. J. Mol. Sci. 22, 8375. doi:10.3390/ijms22168375
Bertin, A., McMurray, M. A., Thai, L., Garcia, G., Votin, V., Grob, P., et al. (2010). Phosphatidylinositol-4,5- bisphosphate promotes budding yeast septin filament assembly and organization. J. Mol. Biol. 404, 711–731. doi:10.1016/j.jmb.2010.10.002
Bläser, , Horn, J., Würmell, P., Bauer, H., Strümpell, S., Nurden, P., et al. (2004). The novel human platelet septin SEPT8 is an interaction partner of SEPT4. Thrombosis Haemostasis 5, 959–966. doi:10.1160/th03-09-0578
Boddy, K. C., Gao, A. D., Truong, D., Kim, M. S., Froese, C. D., Trimble, W. S., et al. (2018). Septin-regulated actin dynamics promote Salmonella invasion of host cells. Cell Microbiol. 20, e12866. doi:10.1111/cmi.12866
Boubakar, L., Falk, J., Ducuing, H., Thoinet, K., Reynaud, F., Derrington, E., et al. (2017). Molecular memory of morphologies by septins during neuron generation allows early polarity inheritance. Neuron 95, 834–851.e5. doi:10.1016/j.neuron.2017.07.027
Bridges, A. A., Jentzsch, M. S., Oakes, P. W., Occhipinti, P., and Gladfelter, A. S. (2016). Micron-scale plasma membrane curvature is recognized by the septin cytoskeleton. J. Cell Biol. 213, 23–32. doi:10.1083/jcb.201512029
Bridges, A. A., Zhang, H., Mehta, S. B., Occhipinti, P., Tani, T., and Gladfelter, A. S. (2014). Septin assemblies form by diffusion-driven annealing on membranes. Proc. Natl. Acad. Sci. 111, 2146–2151. doi:10.1073/pnas.1314138111
Byeon, S., Werner, B., Falter, R., Davidsen, K., Snyder, C., Ong, S.-E., et al. (2022). Proteomic identification of phosphorylation-dependent septin 7 interactors that drive dendritic spine formation. Front. Cell Dev. Biol. 10, 836746. doi:10.3389/fcell.2022.836746
Calvo, F., Ranftl, R., Hooper, S., Farrugia, A. J., Moeendarbary, E., Bruckbauer, A., et al. (2015). Cdc42EP3/BORG2 and septin network enables mechano-transduction and the emergence of cancer-associated fibroblasts. Cell Rep. 13, 2699–2714. doi:10.1016/j.celrep.2015.11.052
Cannon, K. S., Woods, B. L., Crutchley, J. M., and Gladfelter, A. S. (2019). An amphipathic helix enables septins to sense micrometer-scale membrane curvature. J. Cell Biol. 218, 1128–1137. doi:10.1083/jcb.201807211
Carim, S. C., Kechad, A., and Hickson, G. R. X. (2020). Animal cell cytokinesis: The rho-dependent actomyosin-anilloseptin contractile ring as a membrane microdomain gathering, compressing, and sorting machine. Front. Cell Dev. Biol. 8, 575226. doi:10.3389/fcell.2020.575226
Cavini, I. A., Leonardo, D. A., Rosa, H. V. D., Castro, D. K. S. V., Pereira, H. D., Valadares, N. F., et al. (2021). The structural biology of septins and their filaments: An update. Front. Cell Dev. Biol. 9, 765085. doi:10.3389/fcell.2021.765085
Chao, J. T., Wong, A. K. O., Tavassoli, S., Young, B. P., Chruscicki, A., Fang, N. N., et al. (2014). Polarization of the endoplasmic reticulum by ER-septin tethering. Cell 158, 620–632. doi:10.1016/j.cell.2014.06.033
Chen, F., Yan, B., Ren, J., Lyu, R., Wu, Y., Guo, Y., et al. (2021). FIT2 organizes lipid droplet biogenesis with ER tubule-forming proteins and septins. J. Cell Biol. 220, e201907183. doi:10.1083/jcb.201907183
Chen, X., Wang, K., Svitkina, T., and Bi, E. (2020). Critical roles of a RhoGEF-anillin module in septin architectural remodeling during cytokinesis. Curr. Biol. 30, 1477–1490.e3. doi:10.1016/j.cub.2020.02.023
Chollet, J., Dünkler, A., Bäuerle, A., Vivero-Pol, L., Mulaw, M. A., Gronemeyer, T., et al. (2020). Cdc24 interacts with septins to create a positive feedback loop during bud site assembly in yeast. J. Cell Sci. 133, jcs240283. doi:10.1242/jcs.240283
Clay, L., Caudron, F., Denoth-Lippuner, A., Boettcher, B., Frei, S. B., Snapp, E. L., et al. (2014). A sphingolipid-dependent diffusion barrier confines ER stress to the yeast mother cell. Elife 3, e01883. doi:10.7554/elife.01883
Collins, K. B., Kang, H., Matsche, J., Klomp, J. E., Rehman, J., Malik, A. B., et al. (2020). Septin2 mediates podosome maturation and endothelial cell invasion associated with angiogenesis. J. Cell Biol. 219, e201903023. doi:10.1083/jcb.201903023
Dagdas, Y. F., Yoshino, K., Dagdas, G., Ryder, L. S., Bielska, E., Steinberg, G., et al. (2012). Septin-mediated plant cell invasion by the rice blast fungus, magnaporthe oryzae. Science 336, 1590–1595. doi:10.1126/science.1222934
De Souza, L. B. de, Ong, H. L., Liu, X., and Ambudkar, I. S. (2021). PIP2 and septin control STIM1/Orai1 assembly by regulating cytoskeletal remodeling via a CDC42-WASP/WAVE-ARP2/3 protein complex. Cell Calcium 99, 102475. doi:10.1016/j.ceca.2021.102475
Deb, B. K., Chakraborty, P., Gopurappilly, R., and Hasan, G. (2020). SEPT7 regulates Ca2+ entry through Orai channels in human neural progenitor cells and neurons. Cell Calcium 90, 102252. doi:10.1016/j.ceca.2020.102252
Devlin, L., Okletey, J., Perkins, G., Bowen, J. R., Nakos, K., Montagna, C., et al. (2021). Proteomic profiling of the oncogenic septin 9 reveals isoform-specific interactions in breast cancer cells. Proteomics 21, 2100155. doi:10.1002/pmic.202100155
Dhanya, S. K., and Hasan, G. (2021). Deficits associated with loss of STIM1 in Purkinje neurons including motor coordination can Be rescued by loss of septin 7. Front. Cell Dev. Biol. 9, 794807. doi:10.3389/fcell.2021.794807
Dolat, L., Hunyara, J. L., Bowen, J. R., Karasmanis, E. P., Elgawly, M., Galkin, V. E., et al. (2014). Septins promote stress fiber–mediated maturation of focal adhesions and renal epithelial motility. J. Cell Biol. 207, 225–235. doi:10.1083/jcb.201405050
Dolat, L., and Spiliotis, E. T. (2016). Septins promote macropinosome maturation and traffic to the lysosome by facilitating membrane fusion. J. Cell Biol. 214, 517–527. doi:10.1083/jcb.201603030
Erwig, M. S., Patzig, J., Steyer, A. M., Dibaj, P., Heilmann, M., Heilmann, I., et al. (2019). Anillin facilitates septin assembly to prevent pathological outfoldings of central nervous system myelin. Elife 8, e43888. doi:10.7554/elife.43888
Eseola, A. B., Ryder, L. S., Osés-Ruiz, M., Findlay, K., Yan, X., Cruz-Mireles, N., et al. (2021). Investigating the cell and developmental biology of plant infection by the rice blast fungus Magnaporthe oryzae. Fungal Genet. Biol. 154, 103562. doi:10.1016/j.fgb.2021.103562
Fan, Y., Du, Z., Ding, Q., Zhang, J., Winkel, M. O. D., Gerbes, A. L., et al. (2021). SEPT6 drives hepatocellular carcinoma cell proliferation, migration and invasion via the Hippo/YAP signaling pathway. Int. J. Oncol. 58, 25. doi:10.3892/ijo.2021.5205
Farrugia, A. J., Rodríguez, J., Orgaz, J. L., Lucas, M., Sanz-Moreno, V., and Calvo, F. (2020). CDC42EP5/BORG3 modulates SEPT9 to promote actomyosin function, migration, and invasion. J. Cell Biol. 219, e201912159. doi:10.1083/jcb.201912159
Feng, H., Li, G., Du, S., Yang, S., Li, X., Figueiredo, P. de, et al. (2017). The septin protein Sep4 facilitates host infection by plant fungal pathogens via mediating initiation of infection structure formation. Environ. Microbiol. 19, 1730–1749. doi:10.1111/1462-2920.13613
Ferreira, C. B., Marttinen, M., Coelho, J. E., Paldanius, K. M. A., Takalo, M., Mäkinen, P., et al. (2022). S327 phosphorylation of the presynaptic protein SEPTIN5 increases in the early stages of neurofibrillary pathology and alters the functionality of SEPTIN5. Neurobiol. Dis. 163, 105603. doi:10.1016/j.nbd.2021.105603
Garabedian, M. V., Wirshing, A., Vakhrusheva, A., Turegun, B., Sokolova, O. S., and Goode, B. L. (2020). A septin-Hof1 scaffold at the yeast bud neck binds and organizes actin cables. Mol. Biol. Cell 31, 1988–2001. doi:10.1091/mbc.e19-12-0693
Gilden, J. K., Peck, S., Chen, Y.-C. M., and Krummel, M. F. (2012). The septin cytoskeleton facilitates membrane retraction during motility and blebbing. J. Cell Biol. 196, 103–114. doi:10.1083/jcb.201105127
Gilden, J., and Krummel, M. F. (2010). Control of cortical rigidity by the cytoskeleton: Emerging roles for septins. Cytoskeleton 67, 477–486. doi:10.1002/cm.20461
Gupta, D. K., Du, J., Kamranvar, S. A., and Johansson, S. (2018). Tension-induced cytokinetic abscission in human fibroblasts. Oncotarget 9, 8999–9009. doi:10.18632/oncotarget.24016
Gupta, Y. K., Dagdas, Y. F., Martinez-Rocha, A.-L., Kershaw, M. J., Littlejohn, G. R., Ryder, L. S., et al. (2015). Septin-dependent assembly of the exocyst is essential for plant infection by magnaporthe oryzae. Plant Cell 27, 3277–3289. doi:10.1105/tpc.15.00552
Hamdan, H., Lim, B. C., Torii, T., Joshi, A., Konning, M., Smith, C., et al. (2020). Mapping axon initial segment structure and function by multiplexed proximity biotinylation. Nat. Commun. 11, 100. doi:10.1038/s41467-019-13658-5
He, M., Su, J., Xu, Y., Chen, J., Chern, M., Lei, M., et al. (2020). Discovery of broad-spectrum fungicides that block septin-dependent infection processes of pathogenic fungi. Nat. Microbiol. 5, 1565–1575. doi:10.1038/s41564-020-00790-y
Hou, M., Liu, X., Cao, J., and Chen, B. (2016). SEPT7 overexpression inhibits glioma cell migration by targeting the actin cytoskeleton pathway. Oncol. Rep. 35, 2003–2010. doi:10.3892/or.2016.4609
Hu, Q., Milenkovic, L., Jin, H., Scott, M. P., Nachury, M. V., Spiliotis, E. T., et al. (2010). A septin diffusion barrier at the base of the primary cilium maintains ciliary membrane protein distribution. Science 329, 436–439. doi:10.1126/science.1191054
Iv, F., Martins, C. S., Castro-Linares, G., Taveneau, C., Barbier, P., Verdier-Pinard, P., et al. (2021). Insights into animal septins using recombinant human septin octamers with distinct SEPT9 isoforms. J. Cell Sci. 134, jcs258484. doi:10.1242/jcs.258484
Joberty, G., Perlungher, R. R., Sheffield, P. J., Kinoshita, M., Noda, M., Haystead, T., et al. (2001). Borg proteins control septin organization and are negatively regulated by Cdc42. Nat. Cell Biol. 3, 861–866. doi:10.1038/ncb1001-861
Joo, E., Surka, M. C., and Trimble, W. S. (2007). Mammalian SEPT2 is required for scaffolding nonmuscle myosin II and its kinases. Dev. Cell 13, 677–690. doi:10.1016/j.devcel.2007.09.001
Kanamaru, T., Neuner, A., Kurtulmus, B., and Pereira, G. (2022). Balancing the length of the distal tip by septins is key for stability and signalling function of primary cilia. Embo J. 41, e108843. doi:10.15252/embj.2021108843
Kandi, R., Senger, K., Grigoryan, A., Soller, K., Sakk, V., Schuster, T., et al. (2021). Cdc42-Borg4-Septin7 axis regulates HSC polarity and function. Embo Rep. 22, e52931. doi:10.15252/embr.202152931
Karasmanis, E. P., Hwang, D., Nakos, K., Bowen, J. R., Angelis, D., and Spiliotis, E. T. (2019). A septin double ring controls the spatiotemporal organization of the ESCRT machinery in cytokinetic abscission. Curr. Biol. 29, 2174–2182.e7. doi:10.1016/j.cub.2019.05.050
Karasmanis, E. P., Phan, C.-T., Angelis, D., Kesisova, I. A., Hoogenraad, C. C., McKenney, R. J., et al. (2018). Polarity of neuronal membrane traffic requires sorting of kinesin motor cargo during entry into dendrites by a microtubule-associated septin. Dev. Cell 46, 204–218.e7. doi:10.1016/j.devcel.2018.06.013
Katz, Z. B., Zhang, C., Quintana, A., Lillemeier, B. F., and Hogan, P. G. (2019). Septins organize endoplasmic reticulum-plasma membrane junctions for STIM1-ORAI1 calcium signalling. Sci. Rep-uk 9, 10839. doi:10.1038/s41598-019-46862-w
Kesisova, I. A., Robinson, B. P., and Spiliotis, E. T. (2021). A septin GTPase scaffold of dynein–dynactin motors triggers retrograde lysosome transport. J. Cell Biol. 220, e202005219. doi:10.1083/jcb.202005219
Kim, J., and Cooper, J. A. (2021). Junctional localization of septin 2 is required for organization of junctional proteins in static endothelial monolayers. Arterioscler. Thromb. Vasc. Biol. 41, 346–359. doi:10.1161/atvbaha.120.315472
Kim, J., and Cooper, J. A. (2018). Septins regulate junctional integrity of endothelial monolayers. Mol. Biol. Cell 29, 1693–1703. doi:10.1091/mbc.e18-02-0136
Kim, O. V., Litvinov, R. I., Mordakhanova, E. R., Bi, E., Vagin, O., and Weisel, J. W. (2022). Contribution of septins to human platelet structure and function. Iscience 25, 104654. doi:10.1016/j.isci.2022.104654
Kinoshita, M. (2003). Assembly of mammalian septins. J. Biochem. 134, 491–496. doi:10.1093/jb/mvg182
Kinoshita, M., Field, C. M., Coughlin, M. L., Straight, A. F., and Mitchison, T. J. (2002). Self- and actin-templated assembly of mammalian septins. Dev. Cell 3, 791–802. doi:10.1016/s1534-5807(02)00366-0
Krokowski, S., Lobato-Márquez, D., Chastanet, A., Pereira, P. M., Angelis, D., Galea, D., et al. (2018). Septins recognize and entrap dividing bacterial cells for delivery to lysosomes. Cell Host Microbe 24, 866–874.e4. doi:10.1016/j.chom.2018.11.005
Kumari, A., Ghosh, A., Kolay, S., and Raghu, P. (2022). Septins tune lipid kinase activity and PI(4,5)P2 turnover during G-protein–coupled PLC signalling in vivo. Life Sci. Alliance 5, e202101293. doi:10.26508/lsa.202101293
Kuzmić, M., Linares, G. C., Fialová, J. L., Iv, F., Salaün, D., Llewellyn, A., et al. (2022). Septin-microtubule association via a motif unique to isoform 1 of septin 9 tunes stress fibers. J. Cell Sci. 135, jcs258850. doi:10.1242/jcs.258850
Lam, M., and Calvo, F. (2019). Regulation of mechanotransduction: Emerging roles for septins. Cytoskeleton 76, 115–122. doi:10.1002/cm.21485
Li, L., Zhang, C., and Konopka, J. B. (2012). A Candida albicans temperature-sensitive cdc12-6 mutant identifies roles for septins in selection of sites of germ tube formation and hyphal morphogenesis. Eukaryot. Cell 11, 1210–1218. doi:10.1128/ec.00216-12
Liu, J., Fairn, G. D., Ceccarelli, D. F., Sicheri, F., and Wilde, A. (2012). Cleavage furrow organization requires PIP(2)-mediated recruitment of anillin. Curr. Biol. Cb 22, 64–69. doi:10.1016/j.cub.2011.11.040
Lobato-Márquez, D., Xu, J., Güler, G. Ö., Ojiakor, A., Pilhofer, M., and Mostowy, S. (2021). Mechanistic insight into bacterial entrapment by septin cage reconstitution. Nat. Commun. 12, 4511. doi:10.1038/s41467-021-24721-5
Longtine, M. S., Fares, H., and Pringle, J. R. (1998). Role of the yeast Gin4p protein kinase in septin assembly and the relationship between septin assembly and septin function. J. Cell Biol. 143, 719–736. doi:10.1083/jcb.143.3.719
Marcus, J., Bejerano-Sagie, M., Patterson, N., Bagchi, S., Verkhusha, V. V., Connolly, D., et al. (2019). Septin 9 isoforms promote tumorigenesis in mammary epithelial cells by increasing migration and ECM degradation through metalloproteinase secretion at focal adhesions. Oncogene 38, 5839–5859. doi:10.1038/s41388-019-0844-0
Marquardt, J., Chen, X., and Bi, E. (2019). Architecture, remodeling, and functions of the septin cytoskeleton. Cytoskeleton 76, 7–14. doi:10.1002/cm.21475
Marquardt, J., Chen, X., and Bi, E. (2021). Septin assembly and remodeling at the cell division site during the cell cycle. Front. Cell Dev. Biol. 9, 793920. doi:10.3389/fcell.2021.793920
Marquardt, J., Yao, L.-L., Okada, H., Svitkina, T., and Bi, E. (2020). The LKB1-like kinase Elm1 controls septin hourglass assembly and stability by regulating filament pairing. Curr. Biol. 30, 2386–2394.e4. doi:10.1016/j.cub.2020.04.035
Marttinen, M., Ferreira, C. B., Paldanius, K. M. A., Takalo, M., Natunen, T., Mäkinen, P., et al. (2020). Presynaptic vesicle protein SEPTIN5 regulates the degradation of APP C-terminal fragments and the levels of aβ. Cells 9, 2482. doi:10.3390/cells9112482
Marttinen, M., Kurkinen, K. M., Soininen, H., Haapasalo, A., and Hiltunen, M. (2015). Synaptic dysfunction and septin protein family members in neurodegenerative diseases. Mol. Neurodegener. 10, 16. doi:10.1186/s13024-015-0013-z
Mavrakis, M., Azou-Gros, Y., Tsai, F.-C., Alvarado, J., Bertin, A., Iv, F., et al. (2014). Septins promote F-actin ring formation by crosslinking actin filaments into curved bundles. Nat. Cell Biol. 16, 322–334. doi:10.1038/ncb2921
McMurray, M. A., and Thorner, J. (2019). Turning it inside out: The organization of human septin hetero-oligomers. Cytoskeleton 76, 449–456. doi:10.1002/cm.21571
Mela, A., and Momany, M. (2021). Septins coordinate cell wall integrity and lipid metabolism in a sphingolipid-dependent process. J. Cell Sci. 135, jcs258336. doi:10.1242/jcs.258336
Mendonça, D. C., Macedo, J. N., Guimarães, S. L., Silva, F. L. B. da, Cassago, A., Garratt, R. C., et al. (2019). A revised order of subunits in mammalian septin complexes. Cytoskeleton 76, 457–466. doi:10.1002/cm.21569
Menon, M. B., and Gaestel, M. (2015). Sep(t)arate or not – how some cells take septin-independent routes through cytokinesis. J. Cell Sci. 128, 1877–1886. doi:10.1242/jcs.164830
Merenich, D., Nakos, K., Pompan, T., Donovan, S. J., Gill, A., Patel, P., et al. (2022). Septins guide noncentrosomal microtubules to promote focal adhesion disassembly in migrating cells. Mol. Biol. Cell 33, ar40. doi:10.1091/mbc.e21-06-0334
Møller, A. M. J., Füchtbauer, E.-M., Brüel, A., Andersen, T. L., Borggaard, X. G., Pavlos, N. J., et al. (2018). Septins are critical regulators of osteoclastic bone resorption. Sci. Rep-uk 8, 13016. doi:10.1038/s41598-018-31159-1
Moore, K. S., Moore, R., Fulmer, D. B., Guo, L., Gensemer, C., Stairley, R., et al. (2022). DCHS1, Lix1L, and the septin cytoskeleton: Molecular and developmental etiology of mitral valve prolapse. J. Cardiovasc Dev. Dis. 9, 62. doi:10.3390/jcdd9020062
Moreno-Castellanos, N., Rodríguez, A., Rabanal-Ruiz, Y., Fernández-Vega, A., López-Miranda, J., Vázquez-Martínez, R., et al. (2017). The cytoskeletal protein septin 11 is associated with human obesity and is involved in adipocyte lipid storage and metabolism. Diabetologia 60, 324–335. doi:10.1007/s00125-016-4155-5
Mostowy, S., and Cossart, P. (2012). Septins: The fourth component of the cytoskeleton. Nat. Rev. Mol. Cell Bio 13, 183–194. doi:10.1038/nrm3284
Neubauer, K., and Zieger, B. (2021). Role of septins in endothelial cells and platelets. Front. Cell Dev. Biol. 9, 768409. doi:10.3389/fcell.2021.768409
Ngo, H. V., and Mostowy, S. (2019). Role of septins in microbial infection. J. Cell Sci. 132, jcs226266. doi:10.1242/jcs.226266
Nölke, T., Schwan, C., Lehmann, F., Østevold, K., Pertz, O., and Aktories, K. (2016). Septins guide microtubule protrusions induced by actin-depolymerizing toxins like Clostridium difficile transferase (CDT). Proc. Natl. Acad. Sci. 113, 7870–7875. doi:10.1073/pnas.1522717113
Omrane, M., Camara, A. S., Taveneau, C., Benzoubir, N., Tubiana, T., Yu, J., et al. (2019). Septin 9 has two polybasic domains critical to septin filament assembly and Golgi integrity. Iscience 13, 138–153. doi:10.1016/j.isci.2019.02.015
Pagliuso, A., Tham, T. N., Stevens, J. K., Lagache, T., Persson, R., Salles, A., et al. (2016). A role for septin 2 in Drp1-mediated mitochondrial fission. Embo Rep. 17, 858–873. doi:10.15252/embr.201541612
Palander, O., El-Zeiry, M., and Trimble, W. S. (2017). Uncovering the roles of septins in cilia. Front. Cell Dev. Biol. 5, 36. doi:10.3389/fcell.2017.00036
Panagiotou, T. C., Chen, A., and Wilde, A. (2022). An anillin-CIN85-SEPT9 complex promotes intercellular bridge maturation required for successful cytokinesis. Cell Rep. 40, 111274. doi:10.1016/j.celrep.2022.111274
Paradžik, T., Podgorski, I. I., Zeljko, T. V., and Paradžik, M. (2022). Ancient origins of cytoskeletal crosstalk: Spectraplakin-like proteins precede the emergence of cortical microtubule stabilization complexes as crosslinkers. Int. J. Mol. Sci. 23, 5594. doi:10.3390/ijms23105594
Patzig, J., Erwig, M. S., Tenzer, S., Kusch, K., Dibaj, P., Möbius, W., et al. (2016). Septin/anillin filaments scaffold central nervous system myelin to accelerate nerve conduction. Elife 5, e17119. doi:10.7554/elife.17119
Phatarpekar, P. V., Overlee, B. L., Leehan, A., Wilton, K. M., Ham, H., and Billadeau, D. D. (2020). The septin cytoskeleton regulates natural killer cell lytic granule release. J. Cell Biol. 219, e202002145. doi:10.1083/jcb.202002145
Piatti, S. (2020). Cytokinesis: An anillin–RhoGEF module sets the stage for septin double ring assembly. Curr. Biol. 30, R347–R349. doi:10.1016/j.cub.2020.02.035
Piniella, D., Martínez-Blanco, E., Ibáñez, I., Bartolomé-Martín, D., Porlan, E., Díez-Guerra, J., et al. (2018). Identification of novel regulatory partners of the glutamate transporter GLT-1. Glia 66, 2737–2755. doi:10.1002/glia.23524
Qiu, R., Runxiang, Q., Geng, A., Liu, J., Xu, C. W., Menon, M. B., et al. (2020). SEPT7 interacts with KIF20A and regulates the proliferative state of neural progenitor cells during cortical development. Cereb. Cortex 30, 3030–3043. doi:10.1093/cercor/bhz292
Rabie, E. M., Zhang, S. X., Dunn, C. E., and Nelson, C. M. (2021). Substratum stiffness signals through integrin-linked kinase and β1-integrin to regulate midbody proteins and abscission during EMT. Mol. Biol. Cell 32, 1664–1676. doi:10.1091/mbc.e21-02-0072
Radler, M. R., and Spiliotis, E. T. (2022). Right place, right time - spatial guidance of neuronal morphogenesis by septin GTPases. Curr. Opin. Neurobiol. 75, 102557. doi:10.1016/j.conb.2022.102557
Ribet, D., Boscaini, S., Cauvin, C., Siguier, M., Mostowy, S., Echard, A., et al. (2017). SUMOylation of human septins is critical for septin filament bundling and cytokinesis. J. Cell Biol. 216, 4041–4052. doi:10.1083/jcb.201703096
Robertin, S., and Mostowy, S. (2020). The history of septin biology and bacterial infection. Cell Microbiol. 22, e13173. doi:10.1111/cmi.13173
Russo, G., and Krauss, M. (2021). Septin remodeling during mammalian cytokinesis. Front. Cell Dev. Biol. 9, 768309. doi:10.3389/fcell.2021.768309
Ryder, L. S., Cruz-Mireles, N., Molinari, C., Eisermann, I., Eseola, A. B., and Talbot, N. J. (2022). The appressorium at a glance. J. Cell Sci. 135, jcs259857. doi:10.1242/jcs.259857
Ryder, L. S., Dagdas, Y. F., Kershaw, M. J., Venkataraman, C., Madzvamuse, A., Yan, X., et al. (2019). A sensor kinase controls turgor-driven plant infection by the rice blast fungus. Nature 574, 423–427. doi:10.1038/s41586-019-1637-x
Salameh, J., Cantaloube, I., Benoit, B., Poüs, C., and Baillet, A. (2021). Cdc42 and its BORG2 and BORG3 effectors control the subcellular localization of septins between actin stress fibers and microtubules. Curr. Biol. 31, 4088–4103.e5. doi:10.1016/j.cub.2021.07.004
Schmidt, K., and Nichols, B. J. (2004). Functional interdependence between septin and actin cytoskeleton. Bmc Cell Biol. 5, 43. doi:10.1186/1471-2121-5-43
Serwach, K., and Gruszczynska-Biegala, J. (2020). Target molecules of STIM proteins in the central nervous system. Front. Mol. Neurosci. 13, 617422. doi:10.3389/fnmol.2020.617422
Shindo, A., Audrey, A., Takagishi, M., Takahashi, M., Wallingford, J. B., and Kinoshita, M. (2018). Septin-dependent remodeling of cortical microtubule drives cell reshaping during epithelial wound healing. J. Cell Sci. 131, jcs212647. doi:10.1242/jcs.212647
Shuman, B., and Momany, M. (2022). Septins from protists to people. Front. Cell Dev. Biol. 9, 824850. doi:10.3389/fcell.2021.824850
Simi, A. K., Anlas, A. A., Stallings-Mann, M., Zhang, S., Hsia, T., Cichon, M. A., et al. (2018). A soft microenvironment protects from failure of midbody abscission and multinucleation downstream of the EMT-promoting transcription factor Snail. Cancer Res. 78, 2277–2289. doi:10.1158/0008-5472.can-17-2899
Sirajuddin, M., Farkasovsky, M., Hauer, F., Kühlmann, D., Macara, I. G., Weyand, M., et al. (2007). Structural insight into filament formation by mammalian septins. Nature 449, 311–315. doi:10.1038/nature06052
Sirianni, A., Krokowski, S., Lobato-Márquez, D., Buranyi, S., Pfanzelter, J., Galea, D., et al. (2016). Mitochondria mediate septin cage assembly to promote autophagy of Shigella. Embo Rep. 17, 1029–1043. doi:10.15252/embr.201541832
Smith, C., Dolat, L., Angelis, D., Forgacs, E., Spiliotis, E. T., and Galkin, V. E. (2015). Septin 9 exhibits polymorphic binding to F-actin and inhibits myosin and cofilin activity. J. Mol. Biol. 427, 3273–3284. doi:10.1016/j.jmb.2015.07.026
Song, K., Gras, C., Capin, G., Gimber, N., Lehmann, M., Mohd, S., et al. (2019). A SEPT1-based scaffold is required for Golgi integrity and function. J. Cell Sci. 132, jcs225557. doi:10.1242/jcs.225557
Song, P. X., Peng, J., Omrane, M., Cai, T., Samuel, D., and Gassama-Diagne, A. (2022). Septin 9 and phosphoinositides regulate lysosome localization and their association with lipid droplets. Iscience 25, 104288. doi:10.1016/j.isci.2022.104288
Soroor, F., Kim, M. S., Palander, O., Balachandran, Y., Collins, R. F., Benlekbir, S., et al. (2021). Revised subunit order of mammalian septin complexes explains their in vitro polymerization properties. Mol Biol Cell 32, 289–300. doi:10.1091/mbc.e20-06-0398
Spiliotis, E. T., and Kesisova, I. A. (2021). Spatial regulation of microtubule-dependent transport by septin GTPases. Trends Cell Biol. 31, 979–993. doi:10.1016/j.tcb.2021.06.004
Spiliotis, E. T., and McMurray, M. A. (2020). Masters of asymmetry – lessons and perspectives from 50 years of septins. Mol. Biol. Cell 31, 2289–2297. doi:10.1091/mbc.e19-11-0648
Spiliotis, E. T., and Nakos, K. (2021). Cellular functions of actin- and microtubule-associated septins. Curr. Biol. 31, R651–R666. doi:10.1016/j.cub.2021.03.064
Spiliotis, E. T. (2018). Spatial effects − site-specific regulation of actin and microtubule organization by septin GTPases. J. Cell Sci. 131, jcs207555. doi:10.1242/jcs.207555
Sugiyama, S., and Tanaka, M. (2019). Distinct segregation patterns of yeast cell-peripheral proteins uncovered by a method for protein segregatome analysis. Proc. Natl. Acad. Sci. 116, 8909–8918. doi:10.1073/pnas.1819715116
Sun, T., Song, Y., Teng, D., Chen, Y., Dai, J., Ma, M., et al. (2021). Atypical laminin spots and pull-generated microtubule-actin projections mediate Drosophila wing adhesion. Cell Rep. 36, 109667. doi:10.1016/j.celrep.2021.109667
Targa, B., Klipfel, L., Cantaloube, I., Salameh, J., Benoit, B., Poüs, C., et al. (2019). Septin filament coalignment with microtubules depends on SEPT9_i1 and tubulin polyglutamylation, and is an early feature of acquired cell resistance to paclitaxel. Cell Death Dis. 10, 54. doi:10.1038/s41419-019-1318-6
Tokhtaeva, E., Capri, J., Marcus, E. A., Whitelegge, J. P., Khuzakhmetova, V., Bukharaeva, E., et al. (2015). Septin dynamics are essential for exocytosis. J. Biol. Chem. 290, 5280–5297. doi:10.1074/jbc.m114.616201
Tooley, A. J., Gilden, J., Jacobelli, J., Beemiller, P., Trimble, W. S., Kinoshita, M., et al. (2009). Amoeboid T lymphocytes require the septin cytoskeleton for cortical integrity and persistent motility. Nat. Cell Biol. 11, 17–26. doi:10.1038/ncb1808
Torraca, V., and Mostowy, S. (2016). Septins and bacterial infection. Front. Cell Dev. Biol. 4, 127. doi:10.3389/fcell.2016.00127
Tóth, V., Vadászi, H., Ravasz, L., Mittli, D., Mátyás, D., Molnár, T., et al. (2022). Neuronal-specific septin-3 binds Atg8/LC3B, accumulates and localizes to autophagosomes during induced autophagy. Cell Mol. Life Sci. 79, 471. doi:10.1007/s00018-022-04488-8
Vadnjal, N., Nourreddine, S., Lavoie, G., Serres, M., Roux, P. P., and Paluch, E. K. (2022). Proteomic analysis of the actin cortex in interphase and mitosis. J. Cell Sci. 135, jcs259993. doi:10.1242/jcs.259993
Vagin, O., and Beenhouwer, D. O. (2016). Septins: Regulators of protein stability. Front. Cell Dev. Biol. 4, 143. doi:10.3389/fcell.2016.00143
Verdier-Pinard, P., Salaun, D., Bouguenina, H., Shimada, S., Pophillat, M., Audebert, S., et al. (2017). Septin 9_i2 is downregulated in tumors, impairs cancer cell migration and alters subnuclear actin filaments. Sci. Rep-uk 7, 44976. doi:10.1038/srep44976
Wang, K., Wloka, C., and Bi, E. (2019). Non-muscle myosin-II is required for the generation of a constriction site for subsequent abscission. Iscience 13, 69–81. doi:10.1016/j.isci.2019.02.010
Wang, X., Wang, W., Wang, X., Wang, M., Zhu, L., Garba, F., et al. (2021). The septin complex links the catenin complex to the actin cytoskeleton for establishing epithelial cell polarity. J. Mol. Cell Biol. 13, 395–408. doi:10.1093/jmcb/mjab036
Wasik, A. A., Dumont, V., Tienari, J., Nyman, T. A., Fogarty, C. L., Forsblom, C., et al. (2017). Septin 7 reduces nonmuscle myosin IIA activity in the SNAP23 complex and hinders GLUT4 storage vesicle docking and fusion. Exp. Cell Res. 350, 336–348. doi:10.1016/j.yexcr.2016.12.010
Woods, B. L., Cannon, K. S., Vogt, E. J. D., Crutchley, J. M., and Gladfelter, A. S. (2021). Interplay of septin amphipathic helices in sensing membrane-curvature and filament bundling. Mol. Biol. Cell 32, br5. br5. doi:10.1091/mbc.e20-05-0303
Woods, B. L., and Gladfelter, A. S. (2021). The state of the septin cytoskeleton from assembly to function. Curr. Opin. Cell Biol. 68, 105–112. doi:10.1016/j.ceb.2020.10.007
Xue, Z., and Sokac, A. M. (2016). Back-to-back mechanisms drive actomyosin ring closure during Drosophila embryo cleavage. J. Cell Biol. 215, 335–344. doi:10.1083/jcb.201608025
Yadav, S., Oses-Prieto, J. A., Peters, C. J., Zhou, J., Pleasure, S. J., Burlingame, A. L., et al. (2017). TAOK2 kinase mediates PSD95 stability and dendritic spine maturation through Septin7 phosphorylation. Neuron 93, 379–393. doi:10.1016/j.neuron.2016.12.006
Yang, Y.-M., Fedchyshyn, M. J., Grande, G., Aitoubah, J., Tsang, C. W., Xie, H., et al. (2010). Septins regulate developmental switching from microdomain to nanodomain coupling of Ca2+ influx to neurotransmitter release at a central synapse. Neuron 67, 100–115. doi:10.1016/j.neuron.2010.06.003
Yeh, C.-H., Kuo, P.-L., Wang, Y.-Y., Wu, Y.-Y., Chen, M.-F., Lin, D.-Y., et al. (2015). SEPT12/SPAG4/LAMINB1 complexes are required for maintaining the integrity of the nuclear envelope in postmeiotic male germ cells. Plos One 10, e0120722. doi:10.1371/journal.pone.0120722
Yeh, C.-H., Wang, Y.-Y., Wee, S.-K., Chen, M.-F., Chiang, H.-S., Kuo, P.-L., et al. (2019). Testis-specific SEPT12 expression affects SUN protein localization and is involved in mammalian spermiogenesis. Int. J. Mol. Sci. 20, 1163. doi:10.3390/ijms20051163
Zaatri, A., Perry, J. A., and Maddox, A. S. (2021). Septins and a formin have distinct functions in anaphase chiral cortical rotation in the Caenorhabditis elegans zygote. Mol. Biol. Cell 32, 1283–1292. doi:10.1091/mbc.e20-09-0576
Zeng, Y., Cao, Y., Liu, L., Zhao, J., Zhang, T., Xiao, L., et al. (2019). SEPT9_i1 regulates human breast cancer cell motility through cytoskeletal and RhoA/FAK signaling pathway regulation. Cell Death Dis. 10, 720. doi:10.1038/s41419-019-1947-9
Zhang, J., Kong, C., Xie, H., McPherson, P. S., Grinstein, S., and Trimble, W. S. (1999). Phosphatidylinositol polyphosphate binding to the mammalian septin H5 is modulated by GTP. Curr. Biol. 9, 1458–1467. doi:10.1016/s0960-9822(00)80115-3
Zheng, S., Zheng, B., Liu, Z., Ma, X., Liu, X., Yao, X., et al. (2022). The Cdc42 GTPase activating protein Rga6 promotes the cortical localization of Septin. J. Cell Sci. 135, jcs259228. doi:10.1242/jcs.259228
Keywords: septin, membrane, cytoskeleton, actin, microtubule, intermediate filament, ESCRT
Citation: Benoit B, Poüs C and Baillet A (2023) Septins as membrane influencers: direct play or in association with other cytoskeleton partners. Front. Cell Dev. Biol. 11:1112319. doi: 10.3389/fcell.2023.1112319
Received: 30 November 2022; Accepted: 23 January 2023;
Published: 17 February 2023.
Edited by:
Michael Krauß, Leibniz-Institut für Molekulare Pharmakologie (FMP), GermanyReviewed by:
Jian-Qiu Wu, The Ohio State University, United StatesWilliam Trimble, University of Toronto, Canada
Copyright © 2023 Benoit, Poüs and Baillet. This is an open-access article distributed under the terms of the Creative Commons Attribution License (CC BY). The use, distribution or reproduction in other forums is permitted, provided the original author(s) and the copyright owner(s) are credited and that the original publication in this journal is cited, in accordance with accepted academic practice. No use, distribution or reproduction is permitted which does not comply with these terms.
*Correspondence: Béatrice Benoit, beatrice.benoit@universite-paris-saclay.fr; Anita Baillet, anita.baillet@universite-paris-saclay.fr
†ORCID ID: Béatrice Benoit, orcid.org/0000-0003-2622-7791; Christian Poüs, orcid.org/0000-0002-2502-7854; Anita Baillet, orcid.org/0000-0003-1784-8254