The Role of Extracellular Polymeric Substances in Micropollutant Removal
- 1CEB—Centre of Biological Engineering, Universidade do Minho, Braga, Portugal
- 2LABBELS—Associate Laboratory, Braga/Guimarães, Portugal
In biological wastewater treatment (WWT), microorganisms live and grow held together by a slime matrix comprised of extracellular polymeric substances (EPS), forming a three-dimensional microbial structure of aggregates (flocs or granules) and by chemical binding forces. Furthermore, microscopic observations showed that microbial cells within the flocs were cross linked with EPS, forming a network of polymers with pores and channels. The EPS are typically composed of organic substances such as polysaccharides (PS), proteins (PNs), humic acid substances (HAS), nucleic acids, and lipids. It has been established that EPS play an essential role in aggregate flocculation, settling, and dewatering. Moreover, in the presence of toxic substances, such as pharmaceutical compounds and pesticides, EPS form a protective layer for the aggregated biomass against environmental disturbances that might play an important role in the transport and transformation of micropollutants. Some researchers indicated that there is an increase in EPS concentration under toxic conditions, which can induce an increase in the size of microbial aggregates. In this contribution, we critically review the available information on the impact of micropollutants on microbial EPS production and the relationship between EPS and microbial aggregate structure. Also, a general definition, composition, and factors that affect EPS production are presented.
1 Introduction
The microbial aggregates (flocs and granules) in activated sludge (AS) are formed by communities that are embedded in a self-production matrix of extracellular polymeric substances (EPS) (Bitton, 2005). The term aggregate accounts for the fact that most cells in multi-layered biofilms experience cell-to-cell contact (Flemming et al., 2016).
The formation of the EPS matrix is a dynamic process and is influenced by environmental conditions, such as carbon source (Li and Yang, 2007; Geyik et al., 2016), nutrient availability (Liu et al., 2010), temperature, shear stress (Henriques and Love, 2007), and stressors compounds (Wang et al., 2015; Zhang et al., 2019), among others. EPS are mainly composed of proteins (PNs), polysaccharides (PS), humic acid substances (HAS), nucleic acids, lipids, and extracellular DNA (Frølund et al., 1995; Liu and Fang, 2002; Bitton, 2005; Flemming et al., 2016).
EPS represent the main component of AS flocs, microbial granules, and biofilms (Comte et al., 2007). By definition, EPS are present both within the microbial aggregates and outside the cells, being produced from cellular lysis and hydrolysis, absorb organic matter from the wastewater, and form a protective layer for the cells against the adverse external environment. In addition, they serve as a source of carbon and energy during the absence of substrate (Liu and Fang, 2003; Bitton, 2005; Sheng et al., 2006).
EPS properties make them suitable for many applications in AS systems such as sludge flocculation, settling, dewatering, metal binding, and removal of toxic organic compounds (Liu and Fang, 2003; Li and Yang, 2007; Song C. et al., 2014; More et al., 2014; Geyik et al., 2016; Nouha et al., 2016).
Secretion of EPS by bacteria under the presence of toxic compounds (e.g., pharmaceutical compounds and pesticides.) can protect or diminish the possibility of these toxicants reaching microbes (Pan et al., 2010; Lay et al., 2012; Li C. et al., 2015; Tian et al., 2019) due to the presence of functional groups (e.g., hydroxyl, carboxylic, sulfhydryl, and phosphate groups.) in EPS (Sheng et al., 2010).
Changes in microbial aggregates’ structure have been associated with the variation in EPS composition and production (Melo et al., 2021). Furthermore, a positive correlation between EPS components (PS, PN, and HAS) and large aggregation formation has been found, suggesting that high levels of biopolymers produced by biomass influence the size of the aggregates (Melo et al., 2021).
This review intends to fill the knowledge gap in topics related to the impact of micropollutants in the production of microbial EPS, as well as their relationship with the structure of microbial aggregates. Other relevant information, such as a general definition, composition, and factors that affect its production, will also be covered. The information of some EPS characteristics, such as biosynthesis, hydrophobicity/hydrophilicity, surface charge, biodegradability, and others, have been comprehensively described elsewhere (Flemming et al., 2007, 2016; Sheng et al., 2010; More et al., 2014; Nouha et al., 2016) and are beyond the scope of this article.
2 Extracellular Polymeric Substances
2.1 Definition and Composition
EPS are defined as “organic polymers (biopolymers) of microbial origin which in microbial aggregate systems are frequently responsible for binding cells and other particulate materials together (cohesion) and to the substratum (adhesion)” (Wingender et al., 1999a).
EPS comprise soluble EPS (i.e., slime) and bound EPS (Yu et al., 2009, 2008). The soluble EPS can move freely between sludge flocs and the surrounding liquor (Tu et al., 2012), and the bound EPS exhibit a dynamic double-layer-like structure, with a distinct margin outside the cell wall. Bound EPS can be, in turn, classified as loosely bound EPS (LB-EPS) and tightly bound EPS (TB-EPS) (Li and Yang, 2007). However, research focusing on individual EPS component properties and functions in WWT has just started (Lin et al., 2018; Sheng et al., 2013a; Wang et al., 2015).
The bound EPS are intimately adhered to the cells due to the functional groups (carboxyl, phosphoric, sulfhydryl, phenolic, and hydroxyl groups) and nonpolar groups (e.g., aromatic, aliphatic in proteins, and hydrophobic regions in carbohydrates and proteins). On the other hand, soluble EPS are poorly adhered to the cells or dissolved within the solution, which can be explained by the hydrophilic fractions mainly consisting of carbohydrates (Nouha et al., 2016).
A significant variety of functions has been attributed to EPS, most of them related to the performance of biomass–water separation. The EPS matrix presents a sorption function that influences the exchange of nutrients and other molecules between the environment and bioaggregates. Substances captured from the water phase are maintained trapped into the matrix for possible consumption by cells in the bioaggregates. The sorption by the EPS matrix is not specific, indicating that toxic substances can accumulate surrounding bioaggregates. For that reason, functions of the EPS matrix include the formation of a protective layer for the cells against the harmful external environment, when the biomass is exposed to substances such as pesticides and pharmaceutical compounds and with sudden changes of pH, taking up exogenous nutrients and organic molecules, and aggregating bacterial cells in flocs. Consequently, it has been established that EPS play an essential role in the flocculation, settling, and dewatering of bioaggregates (Sheng et al., 2010).
Researchers have found differences in the EPS composition. The concentrations of the compounds are presented in different ways depending on the applied extraction method, the analyzing method of each component, the type of sludge studied, and the type of effluent, among other factors. EPS production and function have been studied for decades; however, earlier studies of EPS in wastewater demonstrate that the major constituents are PS and PN, both representing 60% of the total content and in smaller quantities are the HAS, uronic acid, lipids, and DNA (Frølund et al., 1995; Frølund et al., 1996; Wang et al., 2013). EPS generally contain small amounts of DNA, which are released after cell lysis. Large amounts of DNA in the EPS may indicate that the cells suffered cell lysis during the rigorous extraction process (Liu and Fang, 2002). Besides, amphiphilic compounds (phospholipids), glycosylated proteins (glycoproteins), and HAS have also been shown to appear in significant amounts or even predominate in EPS preparations from AS, trickling filter biofilms, and anammox granular sludge (Boleij et al., 2018; Oliveira et al., 2020; Liu et al., 2021; Melo et al., 2021). Additionally, HAS are constituted of complex heterogeneous mixtures of organic compounds, such as poly-aliphatic and poly-aromatic compounds, containing carboxylic, phenolic, and hydroxyl, which give a highly negative charge. Thus, contrary to many other natural organic products, they cannot be considered in terms of unique chemical structures (Filella et al., 2005).
2.2 Factors That Influence EPS Production
2.2.1 Substrate
Some research studies have found different amounts of EPS produced when different types of substrates are used. On the one hand, LB-EPS content increased slightly when the system was fed with starch and glucose. On the other hand, LB-EPS content increased greatly when the system was fed with sodium acetate. Nevertheless, the substrate change caused an initial decrease in TB-EPS content from 54 to 45 mg TOC g−1 SS, increasing gradually and remaining relatively stable afterward (Ye et al., 2011a).
It has been observed that the production of EPS is related to the type and quantity of the carbon source. Many types of carbohydrates are used as carbon sources, such as glucose, sodium acetate, peptone, and starch, but research shows that glucose, when used by bacteria, favors the production of EPS (Lee et al., 1999; Czaczyk and Myszka, 2007; Miqueleto et al., 2010). However, some contradictory results can be found in the literature. Wang et al. (2014) compared EPS production using starch and glucose as carbon sources and obtained higher productivity with starch. Geyik et al. (2016) assessed three different substrates (solely glucose; acetate + glucose + peptone; and solely peptone) in three parallel reactors. Albeit the carbon source affected the EPS production, PN was the main component of EPS even when the PN was absent in the feed. Additionally, the variety and size of PN were clearly different in the case of a PN feed. Moreover, in the absence of PN in the medium, as in the experiment with glucose, it was assumed that the biomass used the PN found in different bound EPS fractions.
It was observed that in the absence of an organic substrate, the microorganisms used their EPS as the substrate for cell maintenance. In addition, this condition or ability can make the adaptation of microorganisms easier in environments presenting nutrient limitations. It was also observed that the PS was consumed before the PN, indicating that under food shortage, the microorganisms degrade sugars first (Zhang and Bishop, 2003).
2.2.2 Cell Growth, pH, and Temperature
The correlation between cell growth and EPS secretion has been reported in many studies. Differences in EPS composition were found at the molecular level observed between exponential and stationary growth phases (Badireddy et al., 2010). The time of biomass cultivation may influence the amount of EPS produced depending on the operational and environmental conditions. Under anaerobic conditions, longer bacteria culture time caused lower EPS production, and consequently, EPS production is commonly related to the bacteria stationary growth phase (Sheng et al., 2006). Under aerobic conditions, in AS and biofilms, the cell retention time caused a significant increase in EPS production (Nielsen et al., 1996; Sesay et al., 2006; Miao et al., 2017). However, in other studies, the cell retention time did not correlate with the EPS production (Liao et al., 2001; Wang et al., 2013).
Many studies, especially at the lab scale, have evaluated the effect of pH on EPS production. In general, the ideal pH value for EPS production is between 5.0 and 7.0 (Shu and Lung, 2004). In addition, extreme pH values (2.0–3.0 and greater than 10.0) in the growth medium may inhibit EPS biosynthesis, affecting bacterial growth, causing morphological changes in aggregates, and influencing the molecular mass of the EPS compounds (Chen et al., 2001; Shu and Lung, 2004; Czaczyk and Myszka, 2007). However, most EPS-producing microorganisms require a constant pH value in the growth medium to achieve maximum EPS production (More et al., 2014).
Temperature is one of the most important parameters that influence EPS production by affecting the microbial population and the enzymatic activity in the biological process (Wingender et al., 1999b; Li W.-W. et al., 2015). Most of the EPS-producing microorganisms have maximum performance when the temperature is in the range of 25–31°C. It was previously found that when a reduction of 10°C of the optimum temperature was observed, inhibition of the EPS biosynthesis process was attained (Sutherland, 2001; Czaczyk and Myszka, 2007; More et al., 2014).
2.3 The Role of EPS in Bioflocculation and Settling Ability
2.3.1 Bioflocculation
Several types of interactions and mechanisms are involved in the bioflocculation process, including electrostatic forces, hydrophobic interactions, and bridges of multivalent cations such as Ca2+, Mg2+, and Fe2+ (Sobeck and Higgins, 2002; Liu and Fang, 2003). In turn, the divalent cation bridge theory states that Ca and Mg are important for the bioflocculation process. In theory, divalent cations negatively carry functional groups within the EPS, and this bridge helps to aggregate and stabilize the matrix of biopolymers and microorganisms, thus promoting bioflocculation.
The biomass flocculation process allows an effective separation of the solid–liquid in sedimentation tanks (continuous flow) or sedimentation phase (sequencing batch reactor (SBR) process), which is decisive for the performance of an AS process and is a crucial factor for low turbidity and high effluent quality (Sheng et al., 2010). According to Bala Subramanian et al. (2010), EPS produced by microorganisms play an essential role in bioflocculation, being the main constituent of microbial flocs (Frølund et al., 1996), and act as a kind of glue that holds the cells together (Li and Yang, 2007). Furthermore, microscopic observations showed that microbial cells within the flocs were cross linked with EPS, forming a network of polymers with pores and channels (Liu and Fang, 2003).
However, high EPS concentrations can adversely affect compaction, bioflocculation, and sedimentation due to strong electrostatic repulsion between the negatively charged components of the flocs as described in DLVO theory (Wilén et al., 2008). Thus, many studies stated that the increase in EPS concentration appeared to have a negative effect on bioflocculation properties (Wilén et al., 2008). However, recent studies suggest a significant role of specific EPS molecules rather than the quantity of EPS, determining the difference in bioflocculation behavior of sludge (Liao et al., 2011). In terms of EPS components, PS are recognized as key elements involved in floc and granular sludge matrix structure, providing structural support for microbial aggregates (Lin et al., 2013). Adav et al. (2008) revealed that hydrolysis of β-PS caused granules to disintegrate, whereas using specific enzymes to remove PN, lipids, and α-PS had minimal impacts on the structural stability of the granules. In these works, the granule structure is viewed as a network with β-PS as the backbone for embedded PN, lipids, α-PS, and cells supported the structural integrity of granules. Furthermore, the decrease in the PS content hindered the attachment of the bacteria to the filamentous skeleton and suppressed the formation of stable sludge flocs during filamentous bulking (Shen et al., 2020). PN in the EPS contains many amphiphilic amino acids, such as alanine, and, thus, is regarded as the hydrophobic component in the EPS (Shen et al., 2020). According to Higgins and Novak (1997), the removal of surface PN was more significant than PS on microbial aggregates deflocculating, affecting the flocculating ability of the AS (Wilén et al., 2003).
Large amounts of EPS, mainly in the form of LB-EPS, caused a deterioration in cell adhesion and weakened the aggregate structure, resulting in the erosion of the slime flocs cells, for instance, by the medium’s turbulence (Li and Yang, 2007). On the other hand, the decrease in the EPS content led to a decrease in the microbial adhesion ability during sludge bulking, which was not conducive to the aggregation of bacterial cells (Shen et al., 2020). The results found in the literature indicate that the role of individual EPS components in the flocculation of microbial aggregates is still complex, and the most straightforward theory about the connection between EPS quantity and bioflocculation cannot be taken into consideration.
2.3.2 Settling Ability
So far, there is a nonconsensus regarding the effect of EPS on the settling ability of AS. However, many studies have shown that the variations on the sludge volume index (SVI) are related to the variation of EPS concentration due to changes in the AS flocs surface properties. Table 1 summarizes the main studies presenting a relationship between the SVI and the EPS components. It could be assumed that, as EPS are negatively charged, a high EPS concentration increases the surface charge of the microorganisms, increasing the repulsive forces between the cells and, thus, contributing to the decrease in the strength of the microbial aggregates (Sheng et al., 2010).
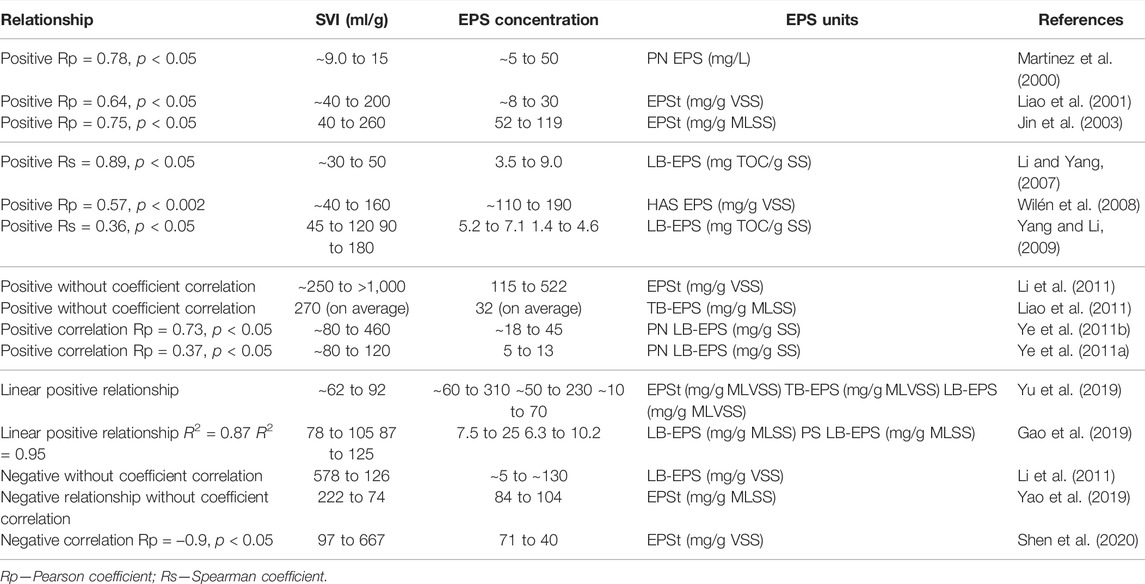
TABLE 1. Effect of EPS components on the settling ability of microbial aggregates: the relationship between EPS and SVI.
In this sense, some researchers have demonstrated a positive correlation between the SVI and EPS concentration or EPS component concentration (Table 1). According to Liao et al. (2001), a higher SVI was associated with a higher amount of total EPS (EPSt). Li and Yang (2007) concluded that the presence of LB-EPS provided a negative effect on bioflocculation, settling ability, and dewatering. In accordance, Yang and Li (2009) found that excessive EPS in the form of LB-EPS deteriorated the structure of the flocs, resulting in increased cell erosion and low settling ability. The authors also found a positive correlation between soluble EPS and deterioration of settling ability. Li et al. (2011) observed that the SVI increased linearly with EPS content in the sludge.
On the other hand, many studies demonstrated that the SVI decreased as LB-EPS, TB-EPS, or EPSt increased. For instance, Shen et al. (2020) found that EPSt production decreased with a bulking phenomenon. Furthermore, the research work of Melo et al. (2021) revealed that, despite the poor floc structure, the rising in EPS content, especially TB-EPS, was the critical point to reaching an AS with well-settling ability properties.
In turn, PN and DNA content in EPS seems to have more significant effects on the settling ability of microbial aggregates, presenting a positive relationship with SVI, and it was not possible to establish a relationship between PS and SVI (Sheng et al., 2010). The EPS production or EPS variation in biological WWT can be affected by many factors, such as operational conditions (i.e., sludge retention time—SRT) (Liao et al., 2001), type of substrate or organic loading (Geyik et al., 2016), reactor configuration (anoxic and aerobic) (Martinez et al., 2000), temperature (Liao et al., 2011), toxic substances (Zhang et al., 2019), and proteins to carbohydrates ratio. The change in the environment of the microbial aggregates can induce production of some kind of EPS component, disturbing somehow the forces that govern the interactions between particles and microorganisms, driving the system to a non-steady-state condition.
For example, Liao et al. (2001) assessed the effect of sludge retention time (SRT) on EPS and observed that the most pronounced effect of SRT on EPS was a change in the proportion of components but not the total EPS content. As a result, the change in the EPS influenced the SVI results. Zhang et al. (2019) observed during 200 days the AS and sulfate-reducing bacteria (SRB) systems operated under ciprofloxacin (CIP) exposure. CIP shaped the microbial communities in AS and SRB sludge and significantly inhibited the family Nitrosomonadaceae (ammonia-oxidizing bacteria) and genus Nitrospira (nitrite-oxidizing bacteria/complete ammonia oxidizer (comammox)) and nitrogen removal in AS system. The increase of genera Zoogloea, Acinetobacter and Flavobacterium in AS and Zoogloea and Acinetobacter in SRB sludge systems under CIP exposure promoted EPS production and CIP adsorption for self-protection of biomass against CIP toxicity. Moreover, the increase of genus Zoogloea-like organisms favored the production of viscous EPS, increasing the PS EPS content and, hence, reducing significantly the PN/PS ratio. This overproduction of viscous EPS leads to the formation of the non-filamentous bulking of AS, with SVI values of 313 ± 12 ml/g for an influent CIP = 5,000 μg/L. Thus, the effect of EPS components on the settling ability of microbial aggregates is still not well understood, and further research is needed.
2.4 Relationship Between EPS and Sludge Morphology
Quantitative differences of EPS composition in flocs with different sizes have been observed. The relation between flocs structure and EPS quantity or type of EPS needs to be more explored. The increase in EPS content, changes in the EPS components (PS, PN, and HAS), and variation on PN/PS ratio have been reported in the literature (Basuvaraj et al., 2015; Liu et al., 2021; Xu et al., 2021). The increase in the EPS content and EPS components during the granulation process indicated that EPS have a vital role in this process (Xu et al., 2021), while insufficient EPS and unbalanced PN/PS compromised the stability of aerobic granules (He et al., 2019).
A sharp increase in EPS occurred during the transition from flocs to granules, where EPS remained at high levels during this period. Then, EPS drove on a decreasing trend during the granules maturation, where EPS established in a lower level (Xu et al., 2021). This behavior that EPS raised remarkably during the granulation process, dropping slightly when granules maturated, was corroborated by McSwain et al. (2005) and Adav and Lee (2008).
Liu et al. (2021) observed that when the aggregate size increased, the EPS content also increased. A comparison between a full-scale system and a lab scale system has been conducted by Basuvaraj et al. (2015) with different aggregate sizes. The authors revealed that in full-scale with predominantly small flocs (diameter <50 µm), higher LB-EPS concentration was obtained. In contrast, higher TB-EPS concentration was reached in lab scale with predominantly large flocs (diameter >340 µm). Ye et al. (2011a, 2011b) obtained a negative relationship between the LB-EPS and floc size. However, only a weak negative correlation between PN content in LB-EPS and floc size was found.
Beyond the quantities, the distribution of EPS components on flocs is an important factor in promoting aggregates’ stability. PN EPS have been identified in the core of granules contributing to stability due to increased hydrophobicity and the negative surface charge, promoting cell-to-cell interaction, whereas PS EPS seem to be present in the outer layer of aggregates holding the other EPS components and cells (McSwain et al., 2005; Basuvaraj et al., 2015). Additionally, the granular structures seem to contain a higher portion of PN (PN/PS ratio of 1.4–1.6) in its total EPS fraction, whereas higher quantities of PS (PN/PS ratio of 0.5) are present in flocs (Basuvaraj et al., 2015). During the granulation process, the abundance of β-sheet PS secondary structure was extremely coincident with the changes in aggregates size, showing that β-sheets decrease to 20% simultaneously when aggregates’ size decreased (Shi and Liu, 2021). PN EPS content in anammox sludge and granular sludge (large granules) was prominently higher than that of granular sludge (small granules) and floc sludge, indicating that the concentration of PN EPS increased with the increase in the aggregates’ size (Liu et al., 2021).
These results suggest a trend regarding the aggregate size and EPS quantity, following the same order of aggregate sizes (large granules > small granules > flocs).
2.5 Role of Extracellular Polymeric Substances in Removal of Micropollutants
EPS might play an important role in the transport and transformation of micropollutants due to abundant functional groups (e.g., hydroxyl, carboxyl, sulfhydryl, and phosphate amine groups); hence, the interactions between EPS and micropollutants also affect their removal efficiencies (Ahmed et al., 2017; Torresi et al., 2017; Wang et al., 2018). In this sense, the functions of EPS in biological WWT during the removal of micropollutants have gained the particular attention of researchers during the last two decades since EPS might play an essential role in the adsorption process, which is a relevant mechanism for the elimination of micropollutants from wastewater (Yang et al., 2012; Wang et al., 2018). In addition, many studies have demonstrated that in the presence of toxic substances, such as pharmaceutical compounds and herbicides, EPS form a protective layer for the cells against the adverse external environment (Bitton, 2005; Song Y. et al., 2014; More et al., 2014). Thus, complexes might be formed between EPS and pollutants through hydrophobic interaction, hydrogen bond, or electrostatic interaction, which significantly affect the removal and migration of these pollutants in biological WWT (Xu et al., 2013; Song C. et al., 2014). However, there is still a knowledge gap about the structural interactions between EPS and these compounds in biological WWT systems.
Some researchers indicated that under toxic conditions (an increase of toxic substances’ concentration), the PN EPS far exceed those systems in normal conditions (Table 2) (More et al., 2014; Li C. et al., 2015) and is the main EPS component that interacts with antibiotics (sulfamethizole), antiepileptic drugs (carbamazepine), and antiinflammatory compounds (ibuprofen, naproxen, and diclofenac) (Li W.-W. et al., 2015; Wang et al., 2018). Barret et al. (2010) determined through partial least squares (PLS) regression a correlation between micropollutants and sludge chemical predictors (PN EPS). It was shown by equilibrium constants Kparticles and Kdissolved matter results that PN had a strong influence on micropollutant sorption in sludge. A correlation between Korganic matter and EPS was also found by Khunjar and Love (2011).
Regarding the variation in EPS concentration, it was pointed out that it increased after the dosage as a mix of four pharmaceutical compounds (carbamazepine, diclofenac, ibuprofen, and naproxen) Lay et al. (2012). In addition, an increase in PN content was also observed that could be a natural microbial response to the environmental change. Another hypothesis presented by the authors was the occurrence of cell lysis and the release of intracellular polymers under pharmaceutical stress. Pasquini et al. (2013) also investigated the behavior of biomass towards the presence of eight household micropollutants with different concentrations (erythromycin, ofloxacin, triclosan, 4-nonylphenol, perfluoroalkyl acids—PFAAs, and ibuprofen). The authors concluded that the impact on bound EPS in AS flocs was dependent on the micropollutant. Only ibuprofen at 0.1, 1, and 5 mg/L caused a decrease of bound EPS that was lower than the number of EPS from the control sludge, while the other compounds influenced an increase in EPS production (Table 2).
It should be mentioned that none of these works could reveal in detail the particular functional groups of micropollutants involved in interaction with EPS (and other studies with the same approach) because the characterization is based chiefly on fractionated EPS components such as PN, PS, and HAS, through colorimetric methods, without considering their high compositional and structural heterogeneity. However, to solve the lack regarding their fundamental composition, new instruments and techniques for the characterization of EPS have been used in the last few years, which has generated a large amount of information about the structural and functional properties of EPS (Yu, 2020).
In such wise, spectroscopy, including Fourier-transform infrared spectroscopy (FTIR) (Sheng et al., 2013b; Yin et al., 2016), three-dimensional excitation-emission matrix fluorescence spectroscopy (3D-EEM) (Pan et al., 2010; Sheng et al., 2010; Wei et al., 2015), and X-ray photoelectron spectroscopy (XPS) (Feng et al., 2018), cross-polarization magic angle spinning (CPMAS) with nuclear magnetic resonance spectroscopy (Meng et al., 2012), and quartz crystal microbalance with dissipation (QCM-D) (Lyu et al., 2021) have been used to give a deep insight of structural binding mechanisms between EPS and micropollutants.
In this framework, the influence of different antibiotics on the biological properties of sludge was studied by Avella et al. (2010a) and Avella et al. (2010b) through FTIR spectroscopy. An increase of bound EPS in flocs was observed for erythromycin and roxithromycin, while amoxicillin, tetracycline, and sulfamethoxazole had no noticeable impact on the increase of bound or soluble EPS. They also found by FTIR analysis that spectra exhibit the same IR regions in the presence or absence of antibiotics, showing only a global variation in absorbance, which was related to the change in the amount of PN and PS, but not designating what types of interactions occurred between EPS and these compounds (Avella et al., 2010b). Likewise, cyclophosphamide and its mean metabolites influenced the biomass EPS production, inducing an increase in soluble EPS in bulk solution and a lower concentration in bound EPS on the sludge; equally, through FTIR analysis, cyclophosphamide presence did not seem to induce any chemical changes in soluble species (Avella et al., 2010a).
Song Y. et al. (2014) also investigated the interaction between tetracycline and EPS, finding additional information regarding the binding mechanisms. PN was identified through FTIR, XPS, NMR, and fluorescence spectroscopy as the dominant active constituent in EPS reaction with tetracycline. They found one binding site during the interaction, and the process is spontaneous in which electrostatic forces play a major role. The authors revealed that the reaction of EPS on tetracycline was a static quenching process, suggesting the formation of a complex. It was also observed that hydroxyl groups were altered during the reaction, reducing the effect of antibiotics and diminishing the inhibitory effect on microorganisms. These results were corroborated by Wang et al. (2018) and confirm that EPS has an essential role in protecting bacteria.
The transport of three antibiotics (sulfamethizole, tetracycline, and norfloxacin) in the biofilm suspension from a moving bed biofilm reactor promoted the adsorption of these three antibiotics onto EPS that accounted for 14.5, 88.2, and 13.1% of total concentration, respectively, at the biodegradation stage. It was also observed that PN dominated interactions between EPS and antibiotics (Wang et al., 2018). The FTIR results showed that the band intensities at 1,340, 1,408, and 1,600 cm−1 decreased as the sulfamethizole concentration increased, suggesting that the major interaction sites for sulfamethizole and EPS were aliphatic C–H, phenolic C–O, and C=O stretching in amides. Norfloxacin had a weaker interaction with EPS, and a slighter spectra variation induced by the addition of norfloxacin was observed. Tetracycline resulted in a significant increase in spectral intensity, associated with O–P–O stretching, P=O stretching, P=O asymmetric stretching, and COO–symmetric stretching. From EEM, the results suggested that the quenching of fluorescence intensities might be ascribed to the formation of EPS–antibiotics complexes (static quenching) rather than dynamic quenching. The quenching constant of three compounds to proteins was higher than that to humic substances, suggesting that proteins, in the form of tryptophan and tyrosine, might be dominant fractions to interact with the biofilm EPS.
Hydrophobic interaction played a major role in the binding reaction and contributed to the stability of the complex formation of sulfamethazine–EPS by a static quenching mechanism. PN instead of HAS dominated the interaction between EPS and sulfamethazine due to the large proportion and the high binding strength. The reason might be that sulfamethazine could enter the internal hydrophobic region of proteins. For the authors, the results imply that tryptophan residues are involved in the binding between EPS and sulfamethazine. After binding, the EPS structure was expanded and became loose, favoring the mass transfer and pollution capture (Xu et al., 2013). The authors revealed that at the initial stage of the reactor operation, sulfamethazine was adsorbed mainly by EPS, and at the later stage, the sulfamethazine adsorption by microorganisms increased, attributed to the biodegradation.
Ciprofloxacin, a fluoroquinolone antibiotic, was used to control filamentous bulking and membrane fouling mitigation in membrane bioreactor (MBR) (Meng et al., 2012). The authors found that the EPS, including PN and PS, experienced an increase and then a decrease during the operational period. Furthermore, the PS seemed to be much more sensitive to the ciprofloxacin exposure than the PN, increasing around 50% in the whole experiment (Meng et al., 2012).
Additionally, to understand the structural makeup of EPS, CPMAS with nuclear magnetic resonance spectroscopy was used to identify the structural difference of EPS between the reactor control and the reactor with ciprofloxacin. Accordingly, six structural groups could be assigned: aliphatic carbon, α-carbon of amino acids, O-alkyl carbon, anomeric carbohydrates, aromatic carbon, and carboxyl carbon. Results showed an increase in PS favored by the addition of ciprofloxacin, containing a much higher carbon content of anomeric carbohydrate (6%) and O-alkyl carbon (20%) than those in the control reactor (Meng et al., 2012). Moreover, the interaction between ciprofloxacin and PN EPS extracted from an anaerobic–anoxic–aerobic process (A2O) are pH dependent, with a strong binding obtained at pH 5 suggesting electrostatic interactions as the dominating binding mechanism. Also, the binding of ciprofloxacin with the extracellular PN followed the pseudo-first-order kinetic equation and the Langmuir model (Lyu et al., 2021). Moreover, EPS from the sulfate-reducing bacteria (SRB) process seemed to have a higher capacity to interact with ciprofloxacin than EPS from the sulfur-oxidizing bacteria (SOB) process (Zhang et al., 2020). Their findings indicated more potential adsorption sites on the SRB sludge for ciprofloxacin adsorption than on SOB sludge since there are higher PN content and more kinds of aromatic amino acid substances in EPS, stronger and more negative zeta potential, and more numbers of functional groups in SRB sludge compared to SOB sludge.
Soluble EPS and bound EPS had a different role in the binding with herbicide dicamba. Regarding the work of Pan et al. (2010), protein-like substances in soluble EPS formed more stable complexes with dicamba than those in bound EPS. Electrostatic forces and hydrophobic interaction forces play a crucial role in the binding of dicamba to EPS, and protein-like substances have a stronger binding capacity for dicamba than humic-like substances governed by a fluorescence quenching static process. Another herbicide, the ametryn, caused a toxicity impact on biomass and affected the EPS production of the MBR operation under different conditions. Regarding EPS components, PN was the dominant component, and it reduced from 77.5 to 74.2%, while PS concentration increased from 22.5 to 28.5% after the addition of ametryn (Navaratna et al., 2012).
3D-EEM fluorescence spectroscopy was used to investigate the interaction of soluble and bound EPS with acetamiprid, a neonicotinoid insecticide. The findings suggest that the fluorescence quenching processes of tryptophan-protein for soluble/bound EPS and fulvic acid-like substances for bound EPS by acetamiprid were mainly controlled by a static quenching process rather than a dynamic quenching process, resulting in this way, in the formation of acetamiprid–EPS complexes. Furthermore, this study demonstrated through binding constants that soluble EPS had a stronger binding capacity for acetamiprid than the bound EPS (Song et al., 2010).
The interactions between micropollutants and EPS are dependent of their hydrophilic and hydrophobic characteristics. Protein-to-carbohydrate ratio (P/C) has been used as an indicator of the relative hydrophobicity of macromolecules and the stickiness of EPS (Santschi et al., 2020). Important findings were reported by these authors suggesting that the P/C ratio is related to parameters that are related to biopolymer aggregation propensity such as relative hydrophobicity, surface activity and surface tension, attachment efficiency, and light-induced chemical cross linking. In this context, the P/C ratio could be predictive for the relative hydrophobicity and “stickiness” of EPS as well as the coagulation efficiency of suspended particles. If such particles have sufficient specific weight, this aggregation process can then lead to sedimentation. The P/C ratio can be used in the future for the prediction of aggregation propensity and sedimentation efficiency. The P/C ratio was also used to evaluate the changes in marine microbial EPS induced by oil and Corexit (Shiu et al., 2020a). These authors cultured four phytoplankton and five marine bacterial species to investigate the changes of the chemical composition of EPS under stress from a water-accommodated fraction of oil or a chemically enhanced water-accommodated fraction. The results obtained by these authors showed a higher linear negative correlation with increasing P/C ratio under chemically enhanced water-accommodated fraction than water-accommodated fraction treatments and also showed that EPS with higher protein ratios were released under higher cellular stress levels induced by a chemically enhanced water-accommodated fraction, suggesting that higher protein ratio EPS, which is more hydrophobic, is secreted to physically or chemically ameliorate the hazardous agents. The evidence obtained by the authors allowed to conclude that microbes can actively modify their EPS release and composition in responses to various adverse stress and support the hypothesis that higher stress not only can trigger more EPS release but also induce changes in its composition (P/C), thereby affecting environmental processes such as marine oil snow formation and the characteristic of marine organic matter. In conclusion, it is important to highlight that EPS secretion is essential for the protection of microbes during unfavorable growth conditions such as exposure to oil and Corexit. In unfavorable growth conditions, such as the presence of toxic substances, EPS usually presented higher PN/PS ratios, which tended to be more hydrophobic due to high amount of proteins, consequently influencing the removal of pollutants (i.e., organic matter, oil, and other organic pollutants, including plastics and microplastics) (Santschi et al., 2021). Recently, it was discovered that nanoparticles, nano- and microplastic (NMP), influenced the production of EPS by marine phytoplankton (Chiu et al., 2017; Shiu et al., 2020b; Santschi et al., 2021). The study conducted by Shiu et al. (2020b) highlights the link between phytoplankton survival and the protective strategies adopted by four phytoplankton species to guard against exposure to NMP. Nine concentrations of NMP were tested, and the results revealed that nano-sized plastics and higher concentrations inhibited growth and induced higher P/C ratios of EPS. The results indicated that nano-sized plastics caused high levels of cellular stress to marine phytoplankton cells and further triggered their EPS secretion with high protein composition. The authors also found that EPS or phytoplankton themselves may act as tackiness agents and play vital roles in the colonization of plastic particles to promote the formation of larger aggregates that can sink to deeper vertical depths and concluded that these findings offer critical information regarding the environmental fate of marine plastic pollution. Chiu et al.(2017) studied the effect of engineered nanoparticles on EPS release from marine phytoplankton. Engineered nanoparticles can potentially impact the marine environment to pose serious threats to marine ecosystems. The authors studied four different diatom species (Odontella mobiliensis, Skeletonema grethae, Phaeodactylum tricornutum, and Thalassiosira pseudonana) and one green algae (Dunaliella tertiolecta) for their EPS release under model engineered nanoparticle treatments, 25 nm titanium dioxide (TiO2), 10–20 nm silicon dioxide (SiO2), and 15–30 nm cerium dioxide (CeO2), and found that SiO2-engineered nanoparticles can significantly stimulate EPS to release from these algae (200–800%), while TiO2-engineered nanoparticle exposure induced the lowest release. An increase in intracellular Ca2+ concentration was also observed suggesting that the EPS release process is mediated through Ca2+ signal pathways, and the authors concluded that with a better understanding of the cellular mechanism-mediated engineered nanoparticle-induced EPS release, potential preventative and safety measures can be developed to mitigate the negative impact on the marine ecosystem.
The main studies found in the literature addressing the effect of micropollutants on EPS are presented in Table 2, and the proposed mechanisms of interaction between micropollutants and EPS are presented in Figure 1.
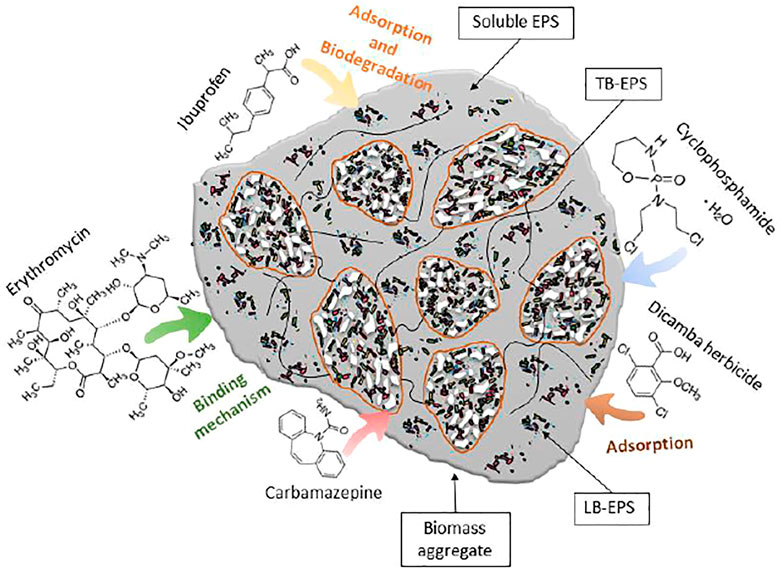
FIGURE 1. Proposed interaction mechanisms of the microbial EPS in bioaggregates interacting with micropollutants. Complexes formed between EPS and pollutants through hydrophobic interaction, hydrogen bond, or electrostatic interaction.
3 Conclusion and Future Perspectives
As evident from this review, EPS remain an unexplored field and several aspects should be clarified to optimize their use for the removal of micropollutants. EPS are composed of mainly carbohydrates and proteins, and they play a very significant role in determining their functionality. The presence of nucleic acid and HAS, as a result of cellular lysis, can further contribute to enhancing the functional properties of EPS. Aspects such as carbon substrate, cell growth, pH, temperature, and other growth conditions play a vital role in determining EPS composition.
The use of modern methods, such as fluorescence spectroscopy, Fourier-transform infrared spectroscopy, X-ray photoelectron spectroscopy, cross-polarization magic angle spinning with nuclear magnetic resonance spectroscopy, scanning electronic microscopy with the classic microbiology techniques, and the integration of different fields are considered fundamental for increasing the knowledge on EPS composition, function, structure, and applications in different areas.
EPS are involved in many applications in biological WWT systems, such as sludge flocculation, settling, dewatering, hydrophobicity/hydrophilicity, biosorption, metal binding, and removal of toxic organic compounds. In the last two decades, the academic community has increased its interest in understanding the role of EPS on the removal of micropollutants, such as pharmaceutical compounds and pesticides. This review is expected to provide information for future research concerning the interaction between EPS and micropollutants.
Despite all the efforts made to better understand EPS and how they can be used to remove micropollutants, there are some gaps that future work can fill. Aspects such as a complete characterization of EPS components and internal relationships, molecular structure, and functions of sludge EPS are still lacking. Multidisciplinary approaches should be considered to overcome the characterization bottlenecks, for promoting EPS functional identification and synthetic pathway elucidation. The development and optimization of new methods to produce, extract, recover, and purify EPS are also imperative. A literature survey reveals that the study of the use of EPS is in the initial phase of research under controlled laboratory conditions, and thus, pilot and full-scale studies are required to overcome the shortcomings of nonuniform EPS characteristics in different operational conditions.
Author Contributions
AM, EF, DM, and CQ contributed to conception and design of the review study. AM selected and organized the papers database. AM wrote the first draft of the manuscript. AM, CQ, EF, and DM wrote sections of the manuscript. All authors contributed to manuscript revision and read and approved the submitted version.
Conflict of Interest
The authors declare that the research was conducted in the absence of any commercial or financial relationships that could be construed as a potential conflict of interest.
Publisher’s Note
All claims expressed in this article are solely those of the authors and do not necessarily represent those of their affiliated organizations, or those of the publisher, the editors, and the reviewers. Any product that may be evaluated in this article, or claim that may be made by its manufacturer, is not guaranteed or endorsed by the publisher.
Acknowledgments
The authors thank the Portuguese Foundation for Science and Technology (FCT) under the scope of the strategic funding of UIDB/04469/2020 unit. The authors also acknowledge the financial support to AM through the Grant Number 240–20170220 provided by Instituto Federal de Educação, Ciência e Tecnologia de Pernambuco (IFPE). DM and CQ thank FCT for funding through program DL 57/2016—Norma transitória.
References
Adav, S. S., and Lee, D.-J. (2008). Extraction of Extracellular Polymeric Substances from Aerobic Granule with Compact interior Structure. J. Hazard. Mater. 154, 1120–1126. doi:10.1016/j.jhazmat.2007.11.058
Adav, S. S., Lee, D.-J., and Tay, J.-H. (2008). Extracellular Polymeric Substances and Structural Stability of Aerobic Granule. Water Res. 42, 1644–1650. doi:10.1016/j.watres.2007.10.013
Ahmed, M. B., Zhou, J. L., Ngo, H. H., Guo, W., Thomaidis, N. S., and Xu, J. (2017). Progress in the Biological and Chemical Treatment Technologies for Emerging Contaminant Removal from Wastewater: A Critical Review. J. Hazard. Mater. 323, 274–298. doi:10.1016/j.jhazmat.2016.04.045
Avella, A. C., Delgado, L. F., Görner, T., Albasi, C., Galmiche, M., and de Donato, P. (2010a). Effect of Cytostatic Drug Presence on Extracellular Polymeric Substances Formation in Municipal Wastewater Treated by Membrane Bioreactor. Bioresour. Tech. 101, 518–526. doi:10.1016/j.biortech.2009.08.057
Avella, A. C., Essendoubi, M., Louvet, J. N., Görner, T., Sockalingum, G. D., Pons, M. N., et al. (2010b). Activated Sludge Behaviour in a Batch Reactor in the Presence of Antibiotics: Study of Extracellular Polymeric Substances. Water Sci. Technol. 61, 3147–3155. doi:10.2166/wst.2010.924
Badireddy, A. R., Chellam, S., Gassman, P. L., Engelhard, M. H., Lea, A. S., and Rosso, K. M. (2010). Role of Extracellular Polymeric Substances in Bioflocculation of Activated Sludge Microorganisms under Glucose-Controlled Conditions. Water Res. 44, 4505–4516. doi:10.1016/j.watres.2010.06.024
Bala Subramanian, S., Yan, S., Tyagi, R. D., and Surampalli, R. Y. (2010). Extracellular Polymeric Substances (EPS) Producing Bacterial Strains of Municipal Wastewater Sludge: Isolation, Molecular Identification, EPS Characterization and Performance for Sludge Settling and Dewatering. Water Res. 44, 2253–2266. doi:10.1016/j.watres.2009.12.046
Barret, M., Carrère, H., Latrille, E., Wisniewski, C., and Patureau, D. (2010). Micropollutant and Sludge Characterization for Modeling Sorption Equilibria. Environ. Sci. Technol. 44, 1100–1106. doi:10.1021/es902575d
Basuvaraj, M., Fein, J., and Liss, S. N. (2015). Protein and Polysaccharide Content of Tightly and Loosely Bound Extracellular Polymeric Substances and the Development of a Granular Activated Sludge Floc. Water Res. 82, 104–117. doi:10.1016/j.watres.2015.05.014
Boleij, M., Pabst, M., Neu, T. R., Van Loosdrecht, M. C. M., and Lin, Y. (2018). Identification of Glycoproteins Isolated from Extracellular Polymeric Substances of Full-Scale Anammox Granular Sludge. Environ. Sci. Technol. 52, 13127–13135. doi:10.1021/acs.est.8b03180
Chen, Y., Yang, H., and Gu, G. (2001). Effect of Acid and Surfactant Treatment on Activated Sludge Dewatering and Settling. Water Res. 35, 2615–2620. doi:10.1016/S0043-1354(00)00565-0
Chiu, M.-H., Khan, Z. A., Garcia, S. G., Le, A. D., Kagiri, A., Ramos, J., et al. (2017). Effect of Engineered Nanoparticles on Exopolymeric Substances Release from Marine Phytoplankton. Nanoscale Res. Lett. 12, 620. doi:10.1186/s11671-017-2397-x
Comte, S., Guibaud, G., and Baudu, M. (2007). Effect of Extraction Method on EPS from Activated Sludge: An HPSEC Investigation. J. Hazard. Mater. 140, 129–137. doi:10.1016/j.jhazmat.2006.06.058
Czaczyk, K., and Myszka, K. (2007). Biosynthesis of Extracellular Polymeric Substances (EPS) and its Role in Microbial Biofilm Formation. Polish J. Environ. Stud. 16, 799–806.
Delgado, L. F., Faucet-Marquis, V., Schetrite, S., Pfohl-Leszkowicz, A., Paranthoen, S., and Albasi, C. (2010). Effect of Cytostatic Drugs on the Sludge and on the Mixed Liquor Characteristics of a Cross-Flow Membrane Bioreactor: Consequence on the Process. J. Membr. Sci. 347, 165–173. doi:10.1016/j.memsci.2009.10.020
Feng, L.-J., Wang, J.-J., Liu, S.-C., Sun, X.-D., Yuan, X.-Z., and Wang, S.-G. (2018). Role of Extracellular Polymeric Substances in the Acute Inhibition of Activated Sludge by Polystyrene Nanoparticles. Environ. Pollut. 238, 859–865. doi:10.1016/j.envpol.2018.03.101
Filella, M., Buffle, J., and Parthasarathy, N. (2005). “HUMIC AND FULVIC COMPOUNDS,” in Encyclopedia of Analytical Science (Oxford: Elsevier), 288–298. doi:10.1016/B0-12-369397-7/00260-0
Flemming, H.-C., Neu, T. R., and Wozniak, D. J. (2007). The EPS Matrix: The "House of Biofilm Cells". J. Bacteriol. 189, 7945–7947. doi:10.1128/JB.00858-07
Flemming, H.-C., Wingender, J., Szewzyk, U., Steinberg, P., Rice, S. A., and Kjelleberg, S. (2016). Biofilms: An Emergent Form of Bacterial Life. Nat. Rev. Microbiol. 14, 563–575. doi:10.1038/nrmicro.2016.94
Fr/olund, B., Griebe, T., and Nielsen, P. H. (1995). Enzymatic Activity in the Activated-Sludge Floc Matrix. Appl. Microbiol. Biotechnol. 43, 755–761. doi:10.1007/BF00164784
Frølund, B., Palmgren, R., Keiding, K., and Nielsen, P. H. (1996). Extraction of Extracellular Polymers from Activated Sludge Using a Cation Exchange Resin. Water Res. 30, 1749–1758. doi:10.1016/0043-1354(95)00323-1
Gao, M., Liu, R., Li, B., Wei, W., and Zhang, Y. (2019). Characteristics of Extracellular Polymeric Substances and Soluble Microbial Products of Activated Sludge in a Pulse Aerated Reactor. Environ. Tech. 41, 2500–2509. doi:10.1080/09593330.2019.1573849
Geyik, A. G., Kılıç, B., and Çeçen, F. (2016). Extracellular Polymeric Substances (EPS) and Surface Properties of Activated Sludges: Effect of Organic Carbon Sources. Environ. Sci. Pollut. Res. 23, 1653–1663. doi:10.1007/s11356-015-5347-0
He, Q., Zhang, J., Gao, S., Chen, L., Lyu, W., Zhang, W., et al. (2019). A Comprehensive Comparison between Non-bulking and Bulking Aerobic Granular Sludge in Microbial Communities. Bioresour. Tech. 294, 122151. doi:10.1016/j.biortech.2019.122151
Henriques, I. D. S., and Love, N. G. (2007). The Role of Extracellular Polymeric Substances in the Toxicity Response of Activated Sludge Bacteria to Chemical Toxins. Water Res. 41, 4177–4185. doi:10.1016/j.watres.2007.05.001
Higgins, M. J., and Novak, J. T. (1997). Characterization of Exocellular Protein and its Role in Bioflocculation. J. Environ. Eng. 123, 4795–5485. doi:10.1061/(ASCE)0733-937210.1061/(asce)0733-9372(1997)123:5(479)
Jin, B., Wilén, B.-M., and Lant, P. (2003). A Comprehensive Insight into Floc Characteristics and Their Impact on Compressibility and Settleability of Activated Sludge. Chem. Eng. J. 95, 221–234. doi:10.1016/S1385-8947(03)00108-6
Khunjar, W. O., and Love, N. G. (2011). Sorption of Carbamazepine, 17α-Ethinylestradiol, Iopromide and Trimethoprim to Biomass Involves Interactions with Exocellular Polymeric Substances. Chemosphere 82, 917–922. doi:10.1016/j.chemosphere.2010.10.046
Lay, W. C. L., Zhang, Q., Zhang, J., McDougald, D., Tang, C., Wang, R., et al. (2012). Effect of Pharmaceuticals on the Performance of a Novel Osmotic Membrane Bioreactor (OMBR). Sep. Sci. Tech. 47, 543–554. doi:10.1080/01496395.2011.630249
Lee, J. W., Yeomans, W. G., Allen, A. L., Deng, F., Gross, R. A., and Kaplan, D. L. (1999). Biosynthesis of Novel Exopolymers by Aureobasidium Pullulans. Appl. Environ. Microbiol. 65, 5265–5271. doi:10.1128/aem.65.12.5265-5271.1999
Li, C., Cabassud, C., Reboul, B., and Guigui, C. (2015a). Effects of Pharmaceutical Micropollutants on the Membrane Fouling of a Submerged MBR Treating Municipal Wastewater: Case of Continuous Pollution by Carbamazepine. Water Res. 69, 183–194. doi:10.1016/j.watres.2014.11.027
Li, J., Liu, X., Liu, Y., Ramsay, J., Yao, C., and Dai, R. (2011). The Effect of Continuous Exposure of Copper on the Properties and Extracellular Polymeric Substances (EPS) of Bulking Activated Sludge. Environ. Sci. Pollut. Res. 18, 1567–1573. doi:10.1007/s11356-011-0492-6
Li, W.-W., Zhang, H.-L., Sheng, G.-P., and Yu, H.-Q. (2015b). Roles of Extracellular Polymeric Substances in Enhanced Biological Phosphorus Removal Process. Water Res. 86, 85–95. doi:10.1016/j.watres.2015.06.034
Li, X. Y., and Yang, S. F. (2007). Influence of Loosely Bound Extracellular Polymeric Substances (EPS) on the Flocculation, Sedimentation and Dewaterability of Activated Sludge. Water Res. 41, 1022–1030. doi:10.1016/j.watres.2006.06.037
Liao, B. Q., Allen, D. G., Droppo, I. G., Leppard, G. G., and Liss, S. N. (2001). Surface Properties of Sludge and Their Role in Bioflocculation and Settleability. Water Res. 35, 339–350. doi:10.1016/S0043-1354(00)00277-3
Liao, B. Q., Lin, H. J., Langevin, S. P., Gao, W. J., and Leppard, G. G. (2011). Effects of Temperature and Dissolved Oxygen on Sludge Properties and Their Role in Bioflocculation and Settling. Water Res. 45, 509–520. doi:10.1016/j.watres.2010.09.010
Lin, Y. M., Sharma, P. K., and van Loosdrecht, M. C. M. (2013). The Chemical and Mechanical Differences between Alginate-like Exopolysaccharides Isolated from Aerobic Flocculent Sludge and Aerobic Granular Sludge. Water Res. 47, 57–65. doi:10.1016/j.watres.2012.09.017
Lin, Y., Reino, C., Carrera, J., Pérez, J., and van Loosdrecht, M. C. M. (2018). Glycosylated Amyloid‐like Proteins in the Structural Extracellular Polymers of Aerobic Granular Sludge Enriched with Ammonium‐oxidizing Bacteria. Microbiologyopen 7, e00616–13. doi:10.1002/mbo3.616
Liu, H., and Fang, H. H. P. (2002). Extraction of Extracellular Polymeric Substances (EPS) of Sludges. J. Biotechnol. 95, 249–256. doi:10.1016/S0168-1656(02)00025-1
Liu, W., Wang, K., Li, B., Yuan, H., and Yang, J. (2010). Production and Characterization of an Intracellular Bioflocculant by Chryseobacterium Daeguense W6 Cultured in Low Nutrition Medium. Bioresour. Tech. 101, 1044–1048. doi:10.1016/j.biortech.2009.08.108
Liu, X., Liu, J., Deng, D., Li, R., Guo, C., Ma, J., et al. (2021). Investigation of Extracellular Polymeric Substances (EPS) in Four Types of Sludge: Factors Influencing EPS Properties and Sludge Granulation. J. Water Process Eng. 40, 101924. doi:10.1016/j.jwpe.2021.101924
Liu, Y., and Fang, H. H. P. (2003). Influences of Extracellular Polymeric Substances (EPS) on Flocculation, Settling, and Dewatering of Activated Sludge. Crit. Rev. Environ. Sci. Tech. 33, 237–273. doi:10.1080/10643380390814479
Lyu, Y., Yu, J., Guo, M., Wang, K., Yu, Z., Zhang, L., et al. (2021). New Insights into Interaction of Proteins in Extracellular Polymeric Substances of Activated Sludge with Ciprofloxacin Using Quartz crystal Microbalance with Dissipation. Chemosphere 263, 128044. doi:10.1016/j.chemosphere.2020.128044
Martinez, F., Favela-Torres, E., and Gomez, J. (2000). Oscillations of Exopolymeric Composition and Sludge Volume index in Nitrifying Flocs. Abab 87, 177–188. doi:10.1385/ABAB:87:3:177
McSwain, B. S., Irvine, R. L., Hausner, M., and Wilderer, P. A. (2005). Composition and Distribution of Extracellular Polymeric Substances in Aerobic Flocs and Granular Sludge. Appl. Environ. Microbiol. 71, 1051–1057. doi:10.1128/AEM.71.2.1051-1057.2005
Melo, A., Costa, J., Quintelas, C., Ferreira, E. C., and Mesquita, D. P. (2021). Effect of Ibuprofen on Extracellular Polymeric Substances (EPS) Production and Composition, and Assessment of Microbial Structure by Quantitative Image Analysis. J. Environ. Manage. 293, 112852. doi:10.1016/j.jenvman.2021.112852
Meng, F., Gao, G., Yang, T.-T., Chen, X., Chao, Y., Na, G., et al. (2015). Effects of Fluoroquinolone Antibiotics on Reactor Performance and Microbial Community Structure of a Membrane Bioreactor. Chem. Eng. J. 280, 448–458. doi:10.1016/j.cej.2015.06.025
Meng, F., Wang, Z., and Li, Y. (2012). Cure of Filament-Caused MBR Fouling in the Presence of Antibiotics: Taking Ciprofloxacin Exposure as an Example. Ind. Eng. Chem. Res. 51, 13784–13791. doi:10.1021/ie301401a
Miao, L., Wang, C., Hou, J., Wang, P., Ao, Y., Li, Y., et al. (2017). Response of Wastewater Biofilm to CuO Nanoparticle Exposure in Terms of Extracellular Polymeric Substances and Microbial Community Structure. Sci. Total Environ. 579, 588–597. doi:10.1016/j.scitotenv.2016.11.056
Miqueleto, A. P., Dolosic, C. C., Pozzi, E., Foresti, E., and Zaiat, M. (2010). Influence of Carbon Sources and C/N Ratio on EPS Production in Anaerobic Sequencing Batch Biofilm Reactors for Wastewater Treatment. Bioresour. Tech. 101, 1324–1330. doi:10.1016/j.biortech.2009.09.026
More, T. T., Yadav, J. S. S., Yan, S., Tyagi, R. D., and Surampalli, R. Y. (2014). Extracellular Polymeric Substances of Bacteria and Their Potential Environmental Applications. J. Environ. Manage. 144, 1–25. doi:10.1016/j.jenvman.2014.05.010
Navaratna, D., Elliman, J., Cooper, A., Shu, L., Baskaran, K., and Jegatheesan, V. (2012). Impact of Herbicide Ametryn on Microbial Communities in Mixed Liquor of a Membrane Bioreactor (MBR). Bioresour. Tech. 113, 181–190. doi:10.1016/j.biortech.2011.12.018
Nielsen, P. H., Fr�lund, B., and Keiding, K. (1996). Changes in the Composition of Extracellular Polymeric Substances in Activated Sludge during Anaerobic Storage. Appl. Microbiol. Biotechnol. 44, 823–830. doi:10.1007/bf00178625
Nouha, K., Rd, T., Tyagi, R. D., and Surampalli, R. Y. (2016). EPS Producing Microorganisms from Municipal Wastewater Activated Sludge. J. Pet. Environ. Biotechnol. 07, 1–13. doi:10.4172/2157-7463.1000255
Oliveira, A. S., Amorim, C. L., Ramos, M. A., Mesquita, D. P., Inocêncio, P., Ferreira, E. C., et al. (2020). Variability in the Composition of Extracellular Polymeric Substances from a Full-Scale Aerobic Granular Sludge Reactor Treating Urban Wastewater. J. Environ. Chem. Eng. 8, 104156. doi:10.1016/j.jece.2020.104156
Pan, X., Liu, J., Zhang, D., Chen, X., Song, W., and Wu, F. (2010). Binding of Dicamba to Soluble and Bound Extracellular Polymeric Substances (EPS) from Aerobic Activated Sludge: A Fluorescence Quenching Study. J. Colloid Interf. Sci. 345, 442–447. doi:10.1016/j.jcis.2010.02.011
Pasquini, L., Merlin, C., Hassenboehler, L., Munoz, J.-F., Pons, M.-N., and Görner, T. (2013). Impact of Certain Household Micropollutants on Bacterial Behavior. Toxicity Tests/study of Extracellular Polymeric Substances in Sludge. Sci. Total Environ. 463-464, 355–365. doi:10.1016/j.scitotenv.2013.06.018
Santschi, P. H., Chin, W.-C., Quigg, A., Xu, C., Kamalanathan, M., Lin, P., et al. (2021). Marine Gel Interactions with Hydrophilic and Hydrophobic Pollutants. Gels 7, 83–14. doi:10.3390/gels7030083
Santschi, P. H., Xu, C., Schwehr, K. A., Lin, P., Sun, L., Chin, W.-C., et al. (2020). Can the Protein/carbohydrate (P/C) Ratio of Exopolymeric Substances (EPS) Be Used as a Proxy for Their 'stickiness' and Aggregation Propensity? Mar. Chem. 218, 103734. doi:10.1016/j.marchem.2019.103734
Sesay, M. L., Özcengiz, G., and Dilek Sanin, F. (2006). Enzymatic Extraction of Activated Sludge Extracellular Polymers and Implications on Bioflocculation. Water Res. 40, 1359–1366. doi:10.1016/j.watres.2006.01.045
Shen, Y., Huang, D.-M., Chen, Y.-P., Yan, P., and Gao, X. (2020). New Insight into Filamentous Sludge Bulking during Wastewater Treatment: Surface Characteristics and Thermodynamics. Sci. Total Environ. 712, 135795. doi:10.1016/j.scitotenv.2019.135795
Sheng, G.-P., Xu, J., Li, W.-H., and Yu, H.-Q. (2013a). Quantification of the Interactions between Ca2+, Hg2+ and Extracellular Polymeric Substances (EPS) of Sludge. Chemosphere 93, 1436–1441. doi:10.1016/j.chemosphere.2013.07.076
Sheng, G.-P., Xu, J., Luo, H.-W., Li, W.-W., Li, W.-H., Yu, H.-Q., et al. (2013b). Thermodynamic Analysis on the Binding of Heavy Metals onto Extracellular Polymeric Substances (EPS) of Activated Sludge. Water Res. 47, 607–614. doi:10.1016/j.watres.2012.10.037
Sheng, G.-P., Yu, H.-Q., and Li, X.-Y. (2010). Extracellular Polymeric Substances (EPS) of Microbial Aggregates in Biological Wastewater Treatment Systems: A Review. Biotechnol. Adv. 28, 882–894. doi:10.1016/j.biotechadv.2010.08.001
Sheng, G. P., Yu, H. Q., and Yue, Z. (2006). Factors Influencing the Production of Extracellular Polymeric Substances by Rhodopseudomonas Acidophila. Int. Biodeterioration Biodegradation 58, 89–93. doi:10.1016/j.ibiod.2006.07.005
Shi, Y., and Liu, Y. (2021). Evolution of Extracellular Polymeric Substances (EPS) in Aerobic Sludge Granulation: Composition, Adherence and Viscoelastic Properties. Chemosphere 262, 128033. doi:10.1016/j.chemosphere.2020.128033
Shiu, R.-F., Chiu, M.-H., Vazquez, C. I., Tsai, Y.-Y., Le, A., Kagiri, A., et al. (2020a). Protein to Carbohydrate (P/C) Ratio Changes in Microbial Extracellular Polymeric Substances Induced by Oil and Corexit. Mar. Chem. 223, 103789. doi:10.1016/j.marchem.2020.103789
Shiu, R.-F., Vazquez, C. I., Chiang, C.-Y., Chiu, M.-H., Chen, C.-S., Ni, C.-W., et al. (2020b). Nano- and Microplastics Trigger Secretion of Protein-Rich Extracellular Polymeric Substances from Phytoplankton. Sci. Total Environ. 748, 141469. doi:10.1016/j.scitotenv.2020.141469
Shu, C.-H., and Lung, M.-Y. (2004). Effect of pH on the Production and Molecular Weight Distribution of Exopolysaccharide by Antrodia Camphorata in Batch Cultures. Process Biochem. 39, 931–937. doi:10.1016/S0032-9592(03)00220-6
Sobeck, D. C., and Higgins, M. J. (2002). Examination of Three Theories for Mechanisms of Cation-Induced Bioflocculation. Water Res. 36, 527–538. doi:10.1016/S0043-1354(01)00254-8
Song, C., Sun, X.-F., Xing, S.-F., Xia, P.-F., Shi, Y.-J., and Wang, S.-G. (2014a). Characterization of the Interactions between Tetracycline Antibiotics and Microbial Extracellular Polymeric Substances with Spectroscopic Approaches. Environ. Sci. Pollut. Res. 21, 1786–1795. doi:10.1007/s11356-013-2070-6
Song, W., Mu, G., Zhang, D., and Pan, X. (2010). Interaction of Acetamiprid with Extracellular Polymeric Substances (EPS) from Activated Sludge: A Fluorescence Study. Afr. J. Biotechnol. 9, 7667–7673. doi:10.5897/AJB09.1539
Song, Y., Zheng, G., Huo, M., Zhao, B., and Zhou, L. (2014b). Extracellular Polymeric Substances and Bound Water Drastically Affect Bioleached Sludge Dewaterability at Low Temperature. Environ. Tech. 35, 2538–2545. doi:10.1080/09593330.2014.911755
Sutherland, I. W. (2001). Microbial Polysaccharides from Gram-Negative Bacteria. Int. Dairy J. 11, 663–674. doi:10.1016/S0958-6946(01)00112-1
Tian, X., Shen, Z., Han, Z., and Zhou, Y. (2019). The Effect of Extracellular Polymeric Substances on Exogenous Highly Toxic Compounds in Biological Wastewater Treatment: An Overview. Bioresour. Tech. Rep. 5, 28–42. doi:10.1016/j.biteb.2018.11.009
Torresi, E., Polesel, F., Bester, K., Christensson, M., Smets, B. F., Trapp, S., et al. (2017). Diffusion and Sorption of Organic Micropollutants in Biofilms with Varying Thicknesses. Water Res. 123, 388–400. doi:10.1016/j.watres.2017.06.027
Tu, X., Song, Y., Yu, H., Zeng, P., and Liu, R. (2012). Fractionation and Characterization of Dissolved Extracellular and Intracellular Products Derived from Floccular Sludge and Aerobic Granules. Bioresour. Tech. 123, 55–61. doi:10.1016/j.biortech.2012.07.075
Wang, H., Deng, H., Ma, L., and Ge, L. (2013). Influence of Operating Conditions on Extracellular Polymeric Substances and Surface Properties of Sludge Flocs. Carbohydr. Polym. 92, 510–515. doi:10.1016/j.carbpol.2012.09.055
Wang, H., Deng, H., Ma, L., and Ge, L. (2014). The Effect of Carbon Source on Extracellular Polymeric Substances Production and its Influence on Sludge Floc Properties. J. Chem. Technol. Biotechnol. 89, 516–521. doi:10.1002/jctb.4147
Wang, L., Li, Y., Wang, L., Zhu, M., Zhu, X., Qian, C., et al. (2018). Responses of Biofilm Microorganisms from Moving Bed Biofilm Reactor to Antibiotics Exposure: Protective Role of Extracellular Polymeric Substances. Bioresour. Tech. 254, 268–277. doi:10.1016/j.biortech.2018.01.063
Wang, Y., Qin, J., Zhou, S., Lin, X., Ye, L., Song, C., et al. (2015). Identification of the Function of Extracellular Polymeric Substances (EPS) in Denitrifying Phosphorus Removal Sludge in the Presence of Copper Ion. Water Res. 73, 252–264. doi:10.1016/j.watres.2015.01.034
Wei, D., Wang, B., Ngo, H. H., Guo, W., Han, F., Wang, X., et al. (2015). Role of Extracellular Polymeric Substances in Biosorption of Dye Wastewater Using Aerobic Granular Sludge. Bioresour. Tech. 185, 14–20. doi:10.1016/j.biortech.2015.02.084
Wilén, B.-M., Jin, B., and Lant, P. (2003). The Influence of Key Chemical Constituents in Activated Sludge on Surface and Flocculating Properties. Water Res. 37, 2127–2139. doi:10.1016/S0043-1354(02)00629-2
Wilén, B.-M., Lumley, D., Mattsson, A., and Mino, T. (2008). Relationship between Floc Composition and Flocculation and Settling Properties Studied at a Full Scale Activated Sludge Plant. Water Res. 42, 4404–4418. doi:10.1016/j.watres.2008.07.033
Wingender, J., Neu, T. R., and Flemming, H. C. (1999b). Microbial Extracellular Polymeric Substances. Berlin, Heidelberg: Springer. doi:10.1007/978-3-642-60147-7
Wingender, J., Neu, T. R., and Flemming, H.-C. (1999a). “What Are Bacterial Extracellular Polymeric Substances?” in Microbial Extracellular Polymeric Substances (Berlin, Heidelberg: Springer Berlin Heidelberg), 1–19. doi:10.1007/978-3-642-60147-7_1
Xu, D., Liu, J., Ma, T., Gao, Y., Zhang, S., and Li, J. (2021). Rapid Granulation of Aerobic Sludge in a Continuous-Flow Reactor with a Two-Zone Sedimentation Tank by the Addition of Dewatered Sludge. J. Water Process Eng. 41, 101941. doi:10.1016/j.jwpe.2021.101941
Xu, J., Sheng, G.-P., Ma, Y., Wang, L.-F., and Yu, H.-Q. (2013). Roles of Extracellular Polymeric Substances (EPS) in the Migration and Removal of Sulfamethazine in Activated Sludge System. Water Res. 47, 5298–5306. doi:10.1016/j.watres.2013.06.009
Yang, S.-f., and Li, X.-y. (2009). Influences of Extracellular Polymeric Substances (EPS) on the Characteristics of Activated Sludge under Non-steady-state Conditions. Process Biochem. 44, 91–96. doi:10.1016/j.procbio.2008.09.010
Yang, S.-F., Lin, C.-F., Wu, C.-J., Ng, K.-K., Yu-Chen Lin, A., and Andy Hong, P.-K. (2012). Fate of Sulfonamide Antibiotics in Contact with Activated Sludge - Sorption and Biodegradation. Water Res. 46, 1301–1308. doi:10.1016/j.watres.2011.12.035
Yao, J., Liu, J., Zhang, Y., Xu, S., Hong, Y., and Chen, Y. (2019). Adding an Anaerobic Step Can Rapidly Inhibit Sludge Bulking in SBR Reactor. Sci. Rep. 9, 1–10. doi:10.1038/s41598-019-47304-3
Ye, F., Peng, G., and Li, Y. (2011a). Influences of Influent Carbon Source on Extracellular Polymeric Substances (EPS) and Physicochemical Properties of Activated Sludge. Chemosphere 84, 1250–1255. doi:10.1016/j.chemosphere.2011.05.004
Ye, F., Ye, Y., and Li, Y. (2011b). Effect of C/N Ratio on Extracellular Polymeric Substances (EPS) and Physicochemical Properties of Activated Sludge Flocs. J. Hazard. Mater. 188, 37–43. doi:10.1016/j.jhazmat.2011.01.043
Yin, C., Meng, F., Meng, Y., and Chen, G.-H. (2016). Differential Ultraviolet-Visible Absorbance Spectra for Characterizing Metal Ions Binding onto Extracellular Polymeric Substances in Different Mixed Microbial Cultures. Chemosphere 159, 267–274. doi:10.1016/j.chemosphere.2016.05.089
Yu, G.-H., He, P.-J., and Shao, L.-M. (2009). Characteristics of Extracellular Polymeric Substances (EPS) Fractions from Excess Sludges and Their Effects on Bioflocculability. Bioresour. Tech. 100, 3193–3198. doi:10.1016/j.biortech.2009.02.009
Yu, G.-H., He, P.-J., Shao, L.-M., and He, P.-P. (2008). Stratification Structure of Sludge Flocs with Implications to Dewaterability. Environ. Sci. Technol. 42, 7944–7949. doi:10.1021/es8016717
Yu, H.-Q. (2020). Molecular Insights into Extracellular Polymeric Substances in Activated Sludge. Environ. Sci. Technol. 54, 7742–7750. doi:10.1021/acs.est.0c00850
Yu, N., Zhao, C., Ma, B., Li, S., She, Z., Guo, L., et al. (2019). Impact of Ampicillin on the Nitrogen Removal, Microbial Community and Enzymatic Activity of Activated Sludge. Bioresour. Tech. 272, 337–345. doi:10.1016/j.biortech.2018.10.048
Zhang, H., Song, S., Jia, Y., Wu, D., and Lu, H. (2019). Stress-responses of Activated Sludge and Anaerobic Sulfate-Reducing Bacteria Sludge under Long-Term Ciprofloxacin Exposure. Water Res. 164, 114964. doi:10.1016/j.watres.2019.114964
Zhang, H., Song, S., Sun, L., Zhao, Q., and Lu, H. (2020). Comparative Study on Ciprofloxacin Removal in Sulfur-Mediated Biological Systems. Chin. Chem. Lett. 31, 1432–1437. doi:10.1016/j.cclet.2020.04.048
Keywords: aggregates, polysaccharides, proteins, bioflocculation, micropollutants
Citation: Melo A, Quintelas C, Ferreira EC and Mesquita DP (2022) The Role of Extracellular Polymeric Substances in Micropollutant Removal. Front. Chem. Eng. 4:778469. doi: 10.3389/fceng.2022.778469
Received: 16 September 2021; Accepted: 18 March 2022;
Published: 04 May 2022.
Edited by:
Antoni Sánchez, Universitat Autònoma de Barcelona, SpainReviewed by:
Joan Dosta, University of Barcelona, SpainPeter H. Santschi, Texas A&M University, United States
Mildred Fernanda Lemus Pérez, Universidad de los Andes, Colombia
Copyright © 2022 Melo, Quintelas, Ferreira and Mesquita. This is an open-access article distributed under the terms of the Creative Commons Attribution License (CC BY). The use, distribution or reproduction in other forums is permitted, provided the original author(s) and the copyright owner(s) are credited and that the original publication in this journal is cited, in accordance with accepted academic practice. No use, distribution or reproduction is permitted which does not comply with these terms.
*Correspondence: Eugénio C. Ferreira, ecferreira@deb.uminho.pt