Marine Bacterial Aromatic Polyketides From Host-Dependent Heterologous Expression and Fungal Mode of Cyclization
- 1CAS Key Laboratory of Tropical Marine Bio-resources and Ecology, Guangdong Key Laboratory of Marine Materia Medica, South China Sea Institute of Oceanology, RNAM Center for Marine Microbiology, Chinese Academy of Sciences, Guangzhou, China
- 2University of Chinese Academy of Sciences, Beijing, China
The structure diversity of type II polyketide synthases-derived bacterial aromatic polyketides is often enhanced by enzyme controlled or spontaneous cyclizations. Here we report the discovery of bacterial aromatic polyketides generated from 5 different cyclization modes and pathway crosstalk between the host and the heterologous fluostatin biosynthetic gene cluster derived from a marine bacterium. The discovery of new compound SEK43F (2) represents an unusual carbon skeleton resulting from a pathway crosstalk, in which a pyrrole-like moiety derived from the host Streptomyces albus J1074 is fused to an aromatic polyketide SEK43 generated from the heterologous fluostatin type II PKSs. The occurrence of a new congener, fluoquinone (3), highlights a bacterial aromatic polyketide that is exceptionally derived from a characteristic fungal F-mode first-ring cyclization. This study expands our knowledge on the power of bacterial type II PKSs in diversifying aromatic polyketides.
Introduction
Aromatic polyketides (APKs) comprise a rich class of natural products with diverse structures and exhibit antimicrobial, antitumor, antiparasitic, antiviral, and other activities (Shen, 2000; Hertweck et al., 2007; Das and Khosla, 2009; Zhou et al., 2010; Zhang Z. et al., 2017). Most bacterial APKs are synthesized by type II polyketide synthases (PKSs). The “minimal” type II PKSs consist of a set of iteratively used enzymes including two ketosynthase units (KSα and KSβ) and an acyl-carrier protein (ACP) (Shen, 2000; Hertweck et al., 2007; Das and Khosla, 2009; Zhou et al., 2010; Zhang Z. et al., 2017). The KSα unit catalyzes iterative decarboxylative condensations of ACP-tethered malonyl to generate a linear poly-β-ketone chain, the length of which is controlled by the KSβ unit (also known as chain-length factor, CLF). The poly-β-ketone chains are converted into diverse APKs by enzymatic tailoring modifications such as cyclization, oxidation, glycosylation, and methylation. In many cases, shunt products are also produced from the reactive poly-β-ketone chains by spontaneous and aberrant cyclization to further enhance the structural diversity of APKs (Shen, 2000; Hertweck et al., 2007; Das and Khosla, 2009; Zhou et al., 2010; Zhang Z. et al., 2017).
Fluostatins (FSTs) are a growing family of atypical angucycline-type APKs and display antibacterial, antitumor and peptidase inhibition activities (Akiyama et al., 1998a,b; Baur et al., 2006; Feng et al., 2010; Zhang et al., 2012; Yang et al., 2015; Jiang et al., 2017; Zhang W. et al., 2017; Huang et al., 2018; Jin et al., 2018). Recently, heterologous expression of the type II PKS gene cluster (fls) of FSTs from South China Sea-derived Micromonospora rosaria SCSIO N160 in Streptomyces coelicolor YF11 (Zhou et al., 2012) enabled the production of new FST derivatives (Yang et al., 2015). Intriguingly, introduction of the fls-gene cluster in Streptomyces albus J1074 led to production of diverse C–C and C–N coupled dimeric FSTs (Huang et al., 2018). Herein we report the discovery of APKs derived from different chain lengths and cyclization patterns in the heterologous host S. albus J1074.
Materials and Methods
General Experimental Procedures
Optical rotation was determined on a 341 polarimeter (Perkin Elmer, Inc.). UV spectrum was recorded with a U-2900 spectrophotometer (Hitachi). IR spectrum was obtained using a Nicolet*6700 FT-IR spectrometer (Thermo Scientific). 1H, 13C, and 2D NMR spectra were recorded on Bruker 700 spectrometer with tetramethylsilane (TMS) as the internal standard. Low-resolution mass spectrometric data were determined using an amaZon SL ion trap mass spectrometer. High-resolution electrospray ionization mass spectrometric (HRESIMS) data were measured on a MaXis 4G UHR-TOFMS spectrometer (Bruker Daltonics Inc.). Column chromatography (CC) was performed with silica gel (100–200 mesh, Jiangyou Silica Gel Development, Inc., Yantai, P. R. China). Thin layer chromatography (TLC, 0.1–0.2 or 0.3–0.4 mm) was conducted with precoated silica gel GF254 (10–40 nm, Yantai) glass plates. Preparative TLC (pTLC) was conducted with precoated glass plates (silica gel GF254, 10–40 nm). Sephadex LH-20 (40–70 μm; Amersham Pharmacia Biotech AB, Uppsala, Sweden), and YMC*gel ODS-A (12 nm S-50 μm; Japan). MCI gel CHP-20P (75–150 μm, Mitsubishi Chemical Corp., Tokyo, Japan). Medium pressure liquid chromatography (MPLC) was performed on automatic flash chromatography (Cheetahtmmp 200, Bonna-Agela Technologies Co., Ltd.) with the monitoring wavelength at 220 nm and the collecting wavelength at 254 nm. Semi-preparative HPLC was carried out on a Hitachi-L2130 HPLC (equipped with a Hitachi L-2455 diode array detector) using a Phenomenex Luna C18 column (250 × 10 mm, 5 μm). Analytical HPLC was performed on an Agilent 1260 Infinity series instrument (equipped with a quaternary pump, a vacuum degasser, an autosampler, a thermostatic column compartment, and a DAD detector) using an Agilent ZORBAX SB-C18 column (150 × 4.6 mm, 5 μm) under the following program: solvent system (solvent A, 10% acetonitrile in water supplementing with 0.08% formic acid; solvent B, 90% acetonitrile in water); 5% B to 80% B (linear gradient, 0–20 min), 80% B to 100% B (20–21 min),100% B (isocratic elution, 21–24 min), 100% B to 5% B (24–25 min), 5% B (isocratic elution, 25–30 min) with the monitoring wavelength at 400 nm. Small scale production process was performed in a compact sterilizable-in-place fermentation system BioFlo 510 fermentator (Eppendorf AG, German).
Bacterial Material
The recombinant strain S. albus J1074/pCSG5033 has been previously described (Huang et al., 2018).
Fermentation, Extraction, and Isolation
The recombinant strain S. albus J1074/pCSG5033 was cultured in the seed medium (3% tryptic soya broth, pH 7.0) and carried on a rotary shaker (200 rpm) at 28°C for 1–3 days. A 20 L scale fermentation was performed by inoculating 1.5 L of the seed culture into a 40 L fermentator (stirring rate:120–230 rpm; dissolved oxygen: 30%; ventilation volume: 15–20 L min−1; pH: 7.2–7.4; pressure: 3 psi; fermentation temperature: 28°C) containing 20 L of the production medium (0.1% peptone fish, 1% starch soluble, 0.6% corn powder, 0.2% bacterial peptone, 0.5% glycerol, 0.2% CaCO3, 3% sea salt), and cultured at 28°C for 3–5 days. A total of 20 L fermentation cultures were harvested and centrifuged to supernatants and mycelium. The supernatants and mycelium were extracted 3 times with equal volume of butanone and acetone, respectively. And then both were evaporated to dryness under reduced pressure and combined to obtain the crude extract (10.0 g).
The crude extract was subjected to normal phase silica gel (100–200 mesh) column chromatography eluted with a gradient solvent system of chloroform/methanol (from 100:0 to 0:100, v/v) to give four fractions (Fr.1–Fr.4) on the basis of initial assessment by thin-layer chromatography (TLC). Fr.1 was subjected to Sephadex LH-20 column chromatography, eluting with CHCl3/MeOH (1:1) to afford four subfractions (Fr.1.L1–Fr.1.L4). Subfraction Fr.1.L3 was further purified using C18 reversed phase MPLC [40 × 2.5 cm ID, eluting with a linear gradient of H2O/CH3CN (100:0 → 0:100, 15 mL min−1, 200 min)], Sephadex LH-20 column chromatography, and reversed-phase semi-preparative HPLC (H2O/CH3CN) successively, to yield 3 (7.0 mg), 4 (1.4 mg), 5 (9.6 mg), and 6 (7.0 mg). Subfraction Fr.2 was further separated with MCI gel column (CH3CN/H2O, from 0:100 to 100:0) to give five fractions Fr.2.M1-Fr.2.M5. SEK43F (2, 4.0 mg) was obtained from Fr.2.M3 by Sephadex LH-20 column chromatography and pTLC (petroleum ether/acetone 50:50), successively. FST C (1, 56.0 mg) was obtained from subfraction Fr.4.
SEK43F (2)
Yellow-green powder; UV (MeOH) λmax (log ε) 452 (4.21), 296 (3.65), 257 (3.37), 205 (4.07) nm; IR νmax 3,273, 1,593, 1,435, 1,287 cm−1; 1H and 13C NMR spectroscopic data, Table 1; HRESIMS m/z 486.1557 [M - H]− (calcd for C28H24NO7, 486.1558).
Fluoquinone (3)
Yellow powder; [α] + 1.6 (c 0.22, CHCl3); UV (MeOH) λmax (log ε) 414 (2.49), 275 (3.02), 253 (3.00), 227 (3.19), 203 (3.30) nm; IR νmax 2,922, 1,686, 802 cm−1; 1H and 13C NMR spectroscopic data, Table 1; HRESIMS m/z 365.0672 [M - H]− (calcd for C20H13O7, 365.0667).
Biological Assays
Antimicrobial activities were measured against seven indicator strains, Staphylococcus aureus ATCC 29213, Enterococcus faecalis ATCC 29212, Escherichia coli ATCC 25922, Acinetobacter baumannii ATCC 19606, Bacillus subtilis SCSIO BS01, Micrococcus luteus SCSIO ML01, and methicillin resistant S. aureus ATCC 43300, by previously described broth microdilution method (Yang et al., 2015). MCF7 (human breast adenocarcinoma cell line), NCI-H460 (human non-small cell lung cancer cell line), HepG2 (Human hepatocellular liver carcinoma cell line), and SF268 (human glioma cell line) were kindly used for cytotoxic activities assay in vitro according to the previously established protocols (Mosmann, 1983).
Results and Discussion
Isolation and Structure Elucidation
Upon a large scale fermentation of S. albus J1074/pCSG5033 (containing the intact fls gene cluster (Yang et al., 2015; Supplementary Figure 1) in a 40-L fermentator and the subsequent isolation with various chromatographic methods, FST C (1) was obtained as the major product, along with several new dimeric FSTs derived from non-enzymatic reactions (Huang et al., 2018). In addition, two new decaketide derivatives SEK43F (2) and fluoquinone (3), two known non-aketide-derived anthraquinones 2-acetylchrysophanol (4) (Abdelfattah, 2009) and 4-acetylchrysophanol (5) (Shaaban et al., 2007), and a previously synthesized compound 3,3′,4,4′,5,5′-hexamethyl-2,2′-dipyrrolylmethene (6) (Figure 1), were also isolated (Guseva et al., 2008; Lund and Thompson, 2014).
SEK43F (2) was isolated as a yellow-green solid. The molecular formula of 2 was established to be C28H25NO7 (m/z 486.1557 [M - H]−, calcd for 486.1558) by high-resolution electrospray ionization mass spectroscopy (HRESIMS), suggesting 17 degrees of unsaturation. The NMR data of 2 (Table 1, Supplementary Figure 2) revealed the presence of four methyls, one methylene, seven sp2 methines, and 16 sp2 quaternary carbons. HMBC correlations from H3-7′ to C2′/C3′/C4′, H3-8′ to C3′/C4′/C5′, H3-9′ to C4′/C5′, and NH-6′ to C3′/C4′/C5′ revealed the presence of a 1,2,3,4-tetrasubstituted pyrrole moiety (unit A, Figure 2). The remaining NMR data of 2 (unit B, Figure 2) were highly similar to those for SEK43 (Meurer et al., 1997), including a characteristic 1,2,3-trisubstituted phenyl fragment (δH 6.82, 1H, d, J = 8.8 Hz; 7.24, 1H, dd, J = 8.2, 8.8 Hz; δH 6.83, 1H, d, J = 8.2 Hz; Table 1) and meta couplings spin system of H16 (δH 6.11, 1H, d, J = 2.3 Hz) and H18 (δH 6.07, 1H, d, J = 2.3 Hz) (Figure 2, Table 1). In addition, presence of the β-oxo-δ-lactone moiety was supported by HMBC correlations from H4 (δH 5.59) to C2/C3/C5, and from H1′ (δH 7.84) to C1/C2/C3 (Figure 2, Supplementary Figure 2). Finally, the two units A and B were linked by C2–C1′, which was supported by HMBC correlations (Figure 2, Supplementary Figure 2) from H1′ (δH 7.84) to C3′ and C1/C2/C3. However, the Z/E configuration of Δ2,1′ in 2 couldn't be determined solely by NMR data. Similar phenomena were observed for hybrubins (Zhao et al., 2016).
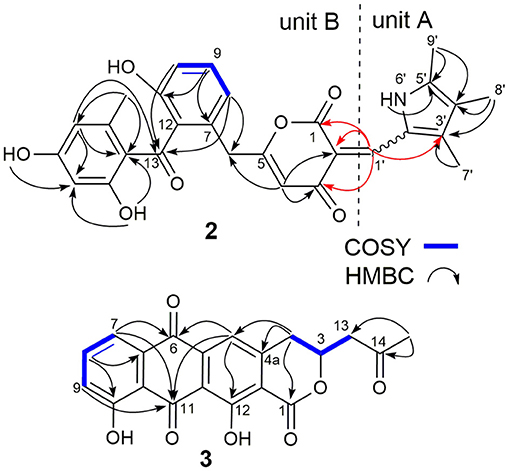
Figure 2. Selected key COSY and HMBC correlations of SEK43F (2) and fluoquinone (3). Key HMBC correlations connecting units A and B of 2 are in red.
The molecular formula of fluoquinone (3) was determined to be C20H14O7 (m/z 365.0672 [M - H]−, calcd for 365.0667) by HRESIMS, requiring 14 degrees of unsaturation. The 1H, 13C, and HSQC NMR data for 3 displayed a singlet methyl, two sp3 methylenes, one sp3 methine, four sp2 methines, and 12 sp2 quaternary carbons (Table 1, Supplementary Figure 3). The 1H-1H COSY spectrum of 3 (Figure 2, Supplementary Figure 3, Table 1) showed a characteristic aromatic ABC spin system (δH 7.70/7.79/7.40) and a CH2-CH(O)-CH2 fragment (δH 3.31/4.12/5.02 and 3.09/3.13/5.02). HMBC correlations (Figure 2, Supplementary Figure 3) from H5/H7 to C6, from H8 to C10, together with four-bond HMBC correlations (Figure 2, Supplementary Figure 3) from H5/H7/H9 to C11, and from H5 to C12 supported the presence of a 1,8-dihydroxyanthraquinone moiety, highly similar to that of the fungal metabolite dermolactone (Gill and Gimenez, 1990; Cotterill et al., 1995). Comparison of NMR data revealed that 3 was different from dermolactone by the absence of a methoxy group at C8 (COSY correlations between H7/H8/H9 in 3) and the presence of an additional acetyl group at C13 (HMBC correlations from H3-15 to C14/C13 in 3). Thus, the planar structure of 3 was determined as shown in Figure 1. The optical rotation value of 3 ([α]25 D + 1.6, CHCl3; c 0.22) was quite different from that of the synthesized (S)-(+)-dermolactone ([α]22 D + 169.3, c 0.21) (Cotterill et al., 1995), but comparable to that of the naturally isolated dermolactone ([α]22 D + 22.0, CHCl3; c 0.07) (Gill and Gimenez, 1990). Natural dermolactone had been confirmed as a mixture of the (S)-(+)- and (R)-(-)-enantiomers (Cotterill et al., 1995). It was thus deduced that fluoquinone (3) should also contain a pair of racemic (S)-(+)- and (R)-(-)-enantiomers (almost in a ration of 1:1) due to its negligible optical rotation. Analysis of the 1H NMR and 13C NMR data of compounds 4–6 revealed that they were identical to 2-acetylchrysophanol (4) (Supplementary Figure 4, Supplementary Table 1) (Abdelfattah, 2009), 4-acetylchrysophanol (5) (Supplementary Figure 5, Supplementary Table 1) (Shaaban et al., 2007), and 3,3′,4,4′,5,5′-hexamethyl-2,2′-dipyrrolylmethene (6) (Supplementary Figure 6, Supplementary Table 1) (Guseva et al., 2008; Lund and Thompson, 2014).
The Biological Evaluation
Compounds 1–6 exhibited negligible antibacterial activity against seven indicator strains: S. aureus ATCC 29213, E. faecalis ATCC 29212, E. coli ATCC 25922, A. baumannii ATCC 19606, B. subtilis SCSIO BS01, Micrococcus Luteus SCSIO ML01, and methicillin resistant S. aureus ATCC 43300. Compound 2 showed weak cytotoxic activities against four human cancer cell lines (SF-268, MCF-7, NCI-H460, and HepG-2) with IC50 values at the range of 36–57 μM (Table 2).
Plausible Biosynthetic Pathway
The heterologous expression of the intact fls-gene cluster from M. rosaria SCSIO N160 in S. albus J1074 afforded a set of APKs 1–5. These aromatic compounds should be derived from the fls gene cluster-encoded enzymes because that the host S. albus J1074 is known for the absence of type II PKS gene clusters (Olano et al., 2014). Previously, a biosynthetic pathway of fluostatins has been proposed (Yang et al., 2015). Briefly, the type II PKS enzymes FlsA (the KSα unit), FlsB (the KSβ unit) and FlsC (the dissociated ACP) assemble one unit of acetyl-CoA and 9 units of malonyl-CoA to generate a linear decaketide, which undergoes the FlsE-catalyzed C9 ketoreduction to give M1 (Figure 3A). Subsequently, the aromatic cyclase FlsD catalyzes the “norm” C7/C12 first-ring cyclization to convert M1 to M2. The downstream enzymes FlsI (a second cyclase analogous to JadI in jadomycin biosynthesis; Kulowski et al., 1999) and FlsO3 (oxygenases) converted M2 to prejadomycin (cyclization pattern I: C7/C12, C5/C14, C4/C17, C2/C19), an established precursor to undergo oxidative modifications to produce diverse FST derivatives (e.g., 1, Figure 3A) (Huang et al., 2018). Alternatively, M2 could undergo spontaneous cyclization (pattern II: C7/C12, C14/C19, C1-OH/C5) to produce SEK43 (a shunt product often encountered in bacterial type II PKS pathways) (McDaniel et al., 1995; Hertweck et al., 2007), which is a key precursor leading to SEK43F (2). The tri-methylated pyrrole unit in 2, which is also present in 6, should be biosynthesized by the host strain, because that the production of 6 was also observed in S. albus J1074 harboring the void vector pSET152 (Supplementary Figure 1). We propose that 6 is produced by the coupling of two putative subunits 6a and 6b (Figure 3B), through a condensation reaction similar to RedH catalysis in the biosynthesis of undecylprodigiosin and hybrubins (Williamson et al., 2005; Zhao et al., 2016). The formation of 2 should be produced from a “pathway crosstalk” event via a condensation reaction by connecting C2 of SEK43 (generated by the heterologous fls type II PKS) and the aldehyde group of 6b (produced by the host), either spontaneously or catalyzed by a host-derived RedH-like enzyme (Figures 3A,B) (Zhao et al., 2016).
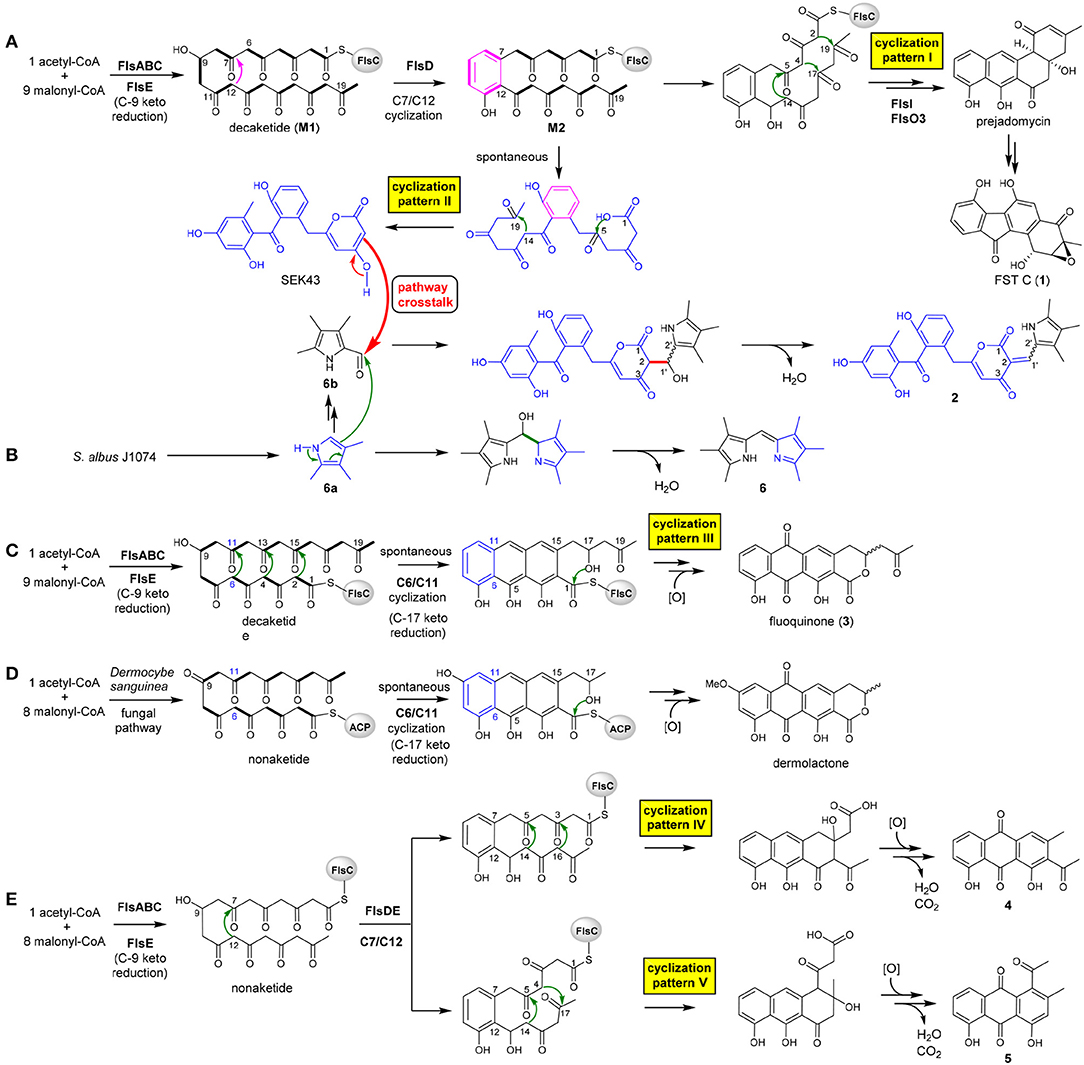
Figure 3. Proposed biosynthesis scheme leading to diversified APKs by taking 5 different cyclization strategies (A–E) and a pathway crosstalk between the host S. albus and the heterologous fls gene cluster (A,B).
It is intriguing to discover the anthraquinone derivative 3 in a bacterium. It was speculated that fundamentally different cyclization strategies were employed by bacterial and fungal type II PKSs to biosynthesize structurally similar APKs with fused-rings (Thomas, 2001, 2016). The first-ring cyclization modes were classified into F-mode for fungi to contain two intact acetate units, and S-mode for bacteria to contain three intact acetate units in the first cyclized phenol ring (Supplementary Figure 7) (Thomas, 2001, 2016; Zhou et al., 2010). This fundamental difference was reinforced by the discovery of three divergent first-ring cyclization strategies to converge in generating the same metabolite chrysophanol, namely F-mode (C6/C11) in fungi and plants (Bringmann et al., 2006), and S-mode (C5/C10) or S′-mode (C7/C12) in bacteria (Bringmann et al., 2006, 2009). Several bacterial fused-ring APKs were found as exceptions to be putatively derived from fungal F-mode cyclizations, such as TW93f and Tw93g (Shen et al., 1999), piloquinone (Polonsky and Lederer, 1963), murayaquinone (Gould et al., 1997), and haloquinone (Krone et al., 1981). However, it was recently discussed that evidence should be provided for these exceptions to either validate the structures (TW93f and Tw93g), or confirm the folding pattern by detailed labeling studies (Thomas, 2016). In contrast, the presence of the six-membered lactone ring in fluoquinone (3) strongly suggested that 3 should be derived from the first-ring cyclization via C6/C11 (cyclization pattern III: C6/C11, C4/C13, C2/C15, C17-OH/C1, Figure 3C). The fungal natural product dermolactone, highly similar to 3, was confirmed to be derived from the F-mode of first ring cyclization (Figure 3D) (Gill and Gimenez, 1990). Thus, fluoquinone (3) represents an exception as a bacterial F-mode (fungal mode) to the F and S biosynthetic classifications of fused ring APKs (Thomas, 2001, 2016).
Compounds 4 and 5 were proposed to be derived from a common non-aketide, which was putatively assembled by FlsABC from one unit of acetyl-CoA and eight units of malonyl-CoA (Figures 3E,F). The discovery of 4 and 5 indicated that the CLF enzyme FlsB should exhibit a loose control in the chain length, allowing the formation of both decaketides (Figures 3A,C) and non-aketides (Figure 3E). The promiscuous chain length control has been reported for native type II PKS enzymes (McDaniel et al., 1993; Meurer et al., 1997; Shen et al., 1999), or can be altered by enzyme engineering (Burson and Khosla, 2000; Tang et al., 2003). Subsequently, the non-aketide was divergent in the third ring cyclization to provide two products 4 (cyclization pattern IV: C7/C12, C5/C14, C3/C16) and 5 (cyclization pattern V: C7/C12, C5/C14, C4/C17).
Although there are different cyclization patterns involved in the formation of 1–5, the cyclization mechanisms are quite common and well-known in type II PKS biosynthesis: (i) enzyme-catalyzed or spontaneous cyclization via aldol condensation (Shen et al., 1999; Zhou et al., 2010), e.g., C7/C12, C6/C11, C5/C14, C4/C17, C4/C13, C3/C16, C2/C15, C2/C19, and C14/C19 (Figure 3); (ii) spontaneous cyclization via lactonization (Meurer et al., 1997), e.g., C1-OH/C5, C17-OH/C1 (Figure 3).
Conclusion
This study expands our knowledge on the power of type II PKSs in diversifying APKs with the occurrence of five different cyclization patterns by a single set of type II PKSs. SEK43F (2) represents an unusual carbon skeleton resulting from a pathway crosstalk, in which a pyrrole-like moiety derived from the host S. albus J1074 is fused to an APK SEK43 generated from the heterologous fls type II PKSs. The occurrence of 3 highlights a bacterial APK that is exceptionally derived from a fungal F-mode first-ring cyclization.
Author Contributions
CZ and YZ designed the study protocol and directed the research. CH, WZ, and CheY performed compound isolation and structure determination. CH carried out the biological assays. ChuY conducted the in vivo genetic studies. CH, YZ, and CZ analyzed the data and wrote the manuscript.
Funding
This work is supported in part by the National Natural Science Foundation of China (31820103003, 41676165, 31700042), Guangdong Province (GDME-2018C005, 2015A030308013), the Chinese Academy of Sciences (QYZDJ-SSW-DQC004).
Conflict of Interest Statement
The authors declare that the research was conducted in the absence of any commercial or financial relationships that could be construed as a potential conflict of interest.
Acknowledgments
We are grateful to Y. Gao, Z. Xiao, A. Sun, C. Li, and Y. Zhang in the analytical facilities of SCSIO.
Supplementary material
The Supplementary Material for this article can be found online at: https://www.frontiersin.org/articles/10.3389/fchem.2018.00528/full#supplementary-material
References
Abdelfattah, M. S. (2009). Mansoquinone: isolation and structure elucidation of new antibacterial aromatic polyketides from terrestrial Streptomyces Sp. Eg5. Nat. Prod. Res. 23, 212–218. doi: 10.1080/14786410801961550
Akiyama, T., Harada, S., Kojima, F., Takahashi, Y., Imada, C., Okami, Y., et al. (1998a). Fluostatins, A., and B, new inhibitors of dipeptidyl peptidase III, produced by Streptomyces sp. TA-3391-I. Taxonomy of producing strain, production, isolation, physico-chemical properties and biological properties. J. Antibiot. 51, 553–559.
Akiyama, T., Nakamura, K. T., Takahashi, Y., Naganawa, H., Muraoka, Y., Aoyagi, T., et al. (1998b). Fluostatins, A., and B, new inhibitors of dipeptidyl peptidase III, produced by Streptomyces sp. TA-3391-II. Structure determination. J. Antibiot. 51, 586–588.
Baur, S., Niehaus, J., Karagouni, A. D., Katsifas, E. A., Chalkou, K., Meintanis, C., et al. (2006). Fluostatins C~E, novel members of the fluostatin family produced by Streptomyces strain Acta 1383. J. Antibiot. 59, 293–297. doi: 10.1038/ja.2006.41
Bringmann, G., Gulder, T. A. M., Hamm, A., Goodfellow, M., and Fiedler, H. P. (2009). Multiple convergence in polyketide biosynthesis: a third folding mode to the anthraquinonechrysophanol. Chem. Commun. 6810–6812. doi: 10.1039/b910501h
Bringmann, G., Noll, T. F., Gulder, T. A., Grune, M., Dreyer, M., Wilde, C., et al. (2006). Different polyketide folding modes converge to an identical molecular architecture. Nat. Chem. Biol. 2, 429–433. doi: 10.1038/nchembio805
Burson, K. K., and Khosla, C. (2000). Dissecting the chain length specificity in bacterial aromatic polyketide synthases using chimeric genes. Tetrahedron 56, 9401–9408. doi: 10.1016/S0040-4020(00)00824-3
Cotterill, A. S., Gill, M., and Milanovic, N. M. (1995). Pigments of Fungi. 41. Synthesis of (S)-(+)-dermolactone and (+/-)-dermolactone: stereochemistry of dermolactone from the Australian fungus Dermocybe sanguinea (Wulf. Ex Fr.) Wunsche sensu Cleland. J. Chem. Soc. Perkin Trans. 1, 1215–1223.
Das, A., and Khosla, C. (2009). Biosynthesis of aromatic polyketides in bacteria. Acc. Chem. Res. 42, 631–639. doi: 10.1021/ar8002249
Feng, Z., Kim, J. H., and Brady, S. F. (2010). Fluostatins produced by the heterologous expression of a TAR reassembled environmental DNA derived type II PKS gene cluster. J. Am. Chem. Soc. 132, 11902–11903. doi: 10.1021/ja104550p
Gill, M., and Gimenez, A. (1990). Pigments of fungi. Part 17. (S)-(+)-dermochrysone, (+)-dermolactone, dermoquinone, and related pigments; new nonaketides from the fungus Dermocybe sanguinea (sensu Cleland). J. Chem. Soc. Perkin Trans. 1, 2585–2591.
Gould, S. J., Melville, C. R., and Chen, J. (1997). The biosynthesis of murayaquinone, a rearranged polyketide. Tetrahedron 53, 4561–4568. doi: 10.1016/S0040-4020(97)00226-3
Guseva, G. B., Antina, E. V., V'yugin, A. I., and Loginova, A. E. (2008). Complex formation of Cu(II), Ni(II), Zn(II), Co(II), and Cd(II) acetates with 3,3',4,4',5,5'-hexamethyldipyrrolylmethene. R. J. Coord. Chem. 34, 599–605. doi: 10.1134/S1070328408080071
Hertweck, C., Luzhetskyy, A., Rebets, Y., and Bechthold, A. (2007). Type II polyketide synthases: gaining a deeper insight into enzymatic teamwork. Nat. Prod. Rep. 24, 162–190. doi: 10.1039/B507395M
Huang, C., Yang, C., Zhang, W., Zhang, L., De, B. C., Zhu, Y., et al. (2018). Molecular basis of dimer formation during the biosynthesis of benzofluorene-containing atypical angucyclines. Nat. Commun. 9:2088. doi: 10.1038/s41467-018-04487-z
Jiang, X., Zhang, Q., Zhu, Y., Nie, F., Wu, Z., Yang, C., et al. (2017). Isolation, structure elucidation and biosynthesis of benzo[b]fluorene nenestatin A from deep-sea derived Micromonospora echinospora SCSIO 04089. Tetrahedron 73, 3585–3590. doi: 10.1016/j.tet.2017.03.054
Jin, J., Yang, X., Liu, T., Xiao, H., Wang, G., Zhou, M., et al. (2018). Fluostatins M–Q featuring a 6-5-6-6 ring skeleton and high oxidized A-rings from marine Streptomyces sp. PKU-MA00045. Mar. Drugs 16, 87–100. doi: 10.3390/md16030087
Krone, B., Hinrichs, A., and Zeeck, A. (1981). Metabolic products of microorganisms. 208. Haloquinone, a new antibiotic active against halobacteria. 2. Chemical-structure and derivatives. J. Antibiot. 34, 1538–1543. doi: 10.7164/antibiotics.34.1538
Kulowski, K., Wendt-Pienkowski, E., Han, L., Yang, K., Vining, L. C., and Hutchinson, C. R. (1999). Functional characterization of the jadI gene as a cyclase forming angucyclinones. J. Am. Chem. Soc. 121, 1786–1794. doi: 10.1021/ja982707f
Lund, K. L. A. R., and Thompson, A. (2014). Synthesis of symmetric meso-H-dipyrrin hydrobromides from 2-formylpyrroles. Synlett 25, 1142–1144. doi: 10.1055/s-0033-1341066
McDaniel, R., Ebertkhosla, S., Hopwood, D. A., and Khosla, C. (1993). Engineered biosynthesis of novel polyketides: manipulation and analysis of an aromatic polyketide synthase with unproved catalytic specificities. J. Am. Chem. Soc. 115, 11671–11675. doi: 10.1021/ja00078a002
McDaniel, R., Ebertkhosla, S., Hopwood, D. A., and Khosla, C. (1995). Rational design of aromatic polyketide natural-products by recombinant assembly of enzymatic subunits. Nature 375, 549–554. doi: 10.1038/375549a0
Meurer, G., Gerlitz, M., WendtPienkowski, E., Vining, L. C., Rohr, J., and Hutchinson, C. R. (1997). Iterative type II polyketide synthases, cyclases and ketoreductases exhibit context-dependent behavior in the biosynthesis of linear and angular decapolyketides. Chem. Biol. 4, 433–443. doi: 10.1016/S1074-5521(97)90195-2
Mosmann, T. (1983). Rapid colorimetric assay for cellular growth and survival: application to proliferation and cytotoxicity assays. J. Immunol. Methods 65, 55–63. doi: 10.1016/0022-1759(83)90303-4
Olano, C., Garcia, I., Gonzalez, A., Rodriguez, M., Rozas, D., Rubio, J., et al. (2014). Activation and identification of five clusters for secondary metabolites in Streptomyces albus J1074. Microb. Biotechnol. 7, 242–256. doi: 10.1111/1751-7915.12116
Polonsky, J., and Lederer, E. (1963). Piloquinone: a new phenanthrene-o-quinone isolated from mycelium of Streptomyces pilosus. Nature 199, 285–286. doi: 10.1038/199285a0
Shaaban, K. A., Shaaban, M., Grün-Wollny, I., Maier, A., Fiebig, H. H., and Laatsch, H. (2007). Julichrome Q6 glucuronide, a monomeric subunit of the julimycin B-I complex from a terrestrial Streptomyces sp. J. Nat. Prod. 70, 1545–1550. doi: 10.1021/np070196h
Shen, B. (2000). Biosynthesis of aromatic polyketides. Top. Curr. Chem. 209, 1–51. doi: 10.1007/3-540-48146-X_1
Shen, Y. M., Yoon, P., Yu, T. W., Floss, H. G., Hopwood, D., and Moore, B. S. (1999). Ectopic expression of the minimal whiE polyketide synthase generates a library of aromatic polyketides of diverse sizes and shapes. Proc. Natl. Acad. Sci. U.S.A. 96, 3622–3627. doi: 10.1073/pnas.96.7.3622
Tang, Y., Tsai, S. C., and Khosla, C. (2003). Polyketide chain length control by chain length factor. J. Am. Chem. Soc. 125, 12708–12709. doi: 10.1021/ja0378759
Thomas, R. (2001). A biosynthetic classification of fungal and Streptomycete fused-ring aromatic polyketides. ChemBioChem 2, 612–627. doi: 10.1002/1439-7633(20010903)2:9<612::AID-CBIC612>3.0.CO;2-Z
Thomas, R. (2016). Examination of potential exceptions to the F and S biosynthetic classification of fused-ring aromatic polyketides. ChemBioChem 17, 2208–2215. doi: 10.1002/cbic.201600315
Williamson, N. R., Simonsen, H. T., Ahmed, R. A., Goldet, G., Slater, H., Woodley, L., et al. (2005). Biosynthesis of the red antibiotic, prodigiosin, in Serratia: identification of a novel 2-methyl-3-n-amyl-pyrrole (MAP) assembly pathway, definition of the terminal condensing enzyme, and implications for undecylprodigiosin biosynthesis in Streptomyces. Mol. Microbiol. 56, 971–989. doi: 10.1111/j.1365-2958.2005.04602.x
Yang, C., Huang, C., Zhang, W., Zhu, Y., and Zhang, C. (2015). Heterologous expression of fluostatin gene cluster leads to a bioactive heterodimer. Org. Lett. 17, 5324–5327. doi: 10.1021/acs.orglett.5b02683
Zhang, W., Liu, Z., Li, S., Lu, Y., Chen, Y., Zhang, H., et al. (2012). Fluostatins I-K from the South China Sea-derived Micromonospora rosaria SCSIO N160. J. Nat. Prod. 75, 1937–1943. doi: 10.1021/np300505y
Zhang, W., Yang, C., Huang, C., Zhang, L., Zhang, H., Zhang, Q., et al. (2017). Pyrazolofluostatins A–C, pyrazole-fused benzo[a]fluorenes from South China Sea-derived Micromonospora rosaria SCSIO N160. Org. Lett. 19, 592–595. doi: 10.1021/acs.orglett.6b03745
Zhang, Z., Pan, H. X., and Tang, G. L. (2017). New insights into bacterial type II polyketide biosynthesis. F1000Research 6:172. doi: 10.12688/f1000research.10466.1
Zhao, Z., Shi, T., Xu, M., Brock, N. L., Zhao, Y.-L., Wang, Y., et al. (2016). Hybrubins: bipyrrole tetramic acids obtained by crosstalk between a truncated undecylprodigiosin pathway and heterologous tetramic acid biosynthetic genes. Org. Lett. 18, 572–575. doi: 10.1021/acs.orglett.5b03609
Zhou, H., Li, Y., and Tang, Y. (2010). Cyclization of aromatic polyketides from bacteria and fungi. Nat. Prod. Rep. 27, 839–868. doi: 10.1039/b911518h
Keywords: heterologous expression, aromatic polyketides, type II polyketide synthase, cyclization modes, pathway crosstalk
Citation: Huang C, Yang C, Zhu Y, Zhang W, Yuan C and Zhang C (2018) Marine Bacterial Aromatic Polyketides From Host-Dependent Heterologous Expression and Fungal Mode of Cyclization. Front. Chem. 6:528. doi: 10.3389/fchem.2018.00528
Received: 04 September 2018; Accepted: 11 October 2018;
Published: 30 October 2018.
Edited by:
Xian-Wen Yang, Third Institute of Oceanography, State Oceanic Administration, ChinaReviewed by:
Shuangjun Lin, Shanghai Jiao Tong University, ChinaSteven Gary Van Lanen, University of Kentucky, United States
Copyright © 2018 Huang, Yang, Zhu, Zhang, Yuan and Zhang. This is an open-access article distributed under the terms of the Creative Commons Attribution License (CC BY). The use, distribution or reproduction in other forums is permitted, provided the original author(s) and the copyright owner(s) are credited and that the original publication in this journal is cited, in accordance with accepted academic practice. No use, distribution or reproduction is permitted which does not comply with these terms.
*Correspondence: Yiguang Zhu, ygzhu@scsio.ac.cn
Changsheng Zhang, czhang2006@gmail.com