Supramolecular Fluorescence Probe Based on Twisted Cucurbit[14]uril for Sensing Fungicide Flusilazole
- 1State Key Laboratory Breeding Base of Green Pesticide and Agricultural Bioengineering, Key Laboratory of Green Pesticide and Agricultural Bioengineering, Ministry of Education, Guizhou University, Guiyang, China
- 2Key Laboratory of Macrocyclic and Supramolecular Chemistry of Guizhou Province, Guizhou University, Guiyang, China
- 3College of Chemical and Environmental Engineering, Shandong University of Science and Technology, Qingdao, China
The host-guest complex of the common dye, thioflavin T (ThT), and twisted cucurbit[14]uril (tQ[14]) was selected as a fluorescent probe to determine non-fluorescent triazole fungicides, including flusilazole, azaconazole, triadimefon, tebuconazole, tricyclazole, flutriafol, penconazole, and triadimenol isomer A, in an aqueous solution. The experimental results reveal that the ThT@tQ[14] probe selectively responded to flusilazole with significant fluorescence quenching and a detection limit of 1.27 × 10−8 mol/L. In addition, the response mechanism involves not only a cooperation interaction—ThT occupies a side-cavity of the tQ[14] host and the triazole fungicide occupies another side-cavity of the tQ[14] host—but also a competition interaction in which both ThT and the triazole fungicide occupy the side-cavities of the tQ[14] host.
Introduction
Triazole systemic fungicides, particularly flusilazole (1-{[bis(4-fluorophenyl) (methyl)silyl]methyl}-1H-1,2,4-triazole), are widely used in fruit, vegetable, and grain crops during cultivation and storage with high efficiency and good sterilization ability (Figure 1) (Zhang Y. H. et al., 2016). However, they still have somewhat toxic or other undesirable side effects on non-target organisms. Extensive or inappropriate use can cause soil and water pollution, and thus threaten human health (Ma et al., 2016). Therefore, it is necessary to develop sensitive and selective methods for the analysis of triazole fungicides, which are usually present in trace amounts.
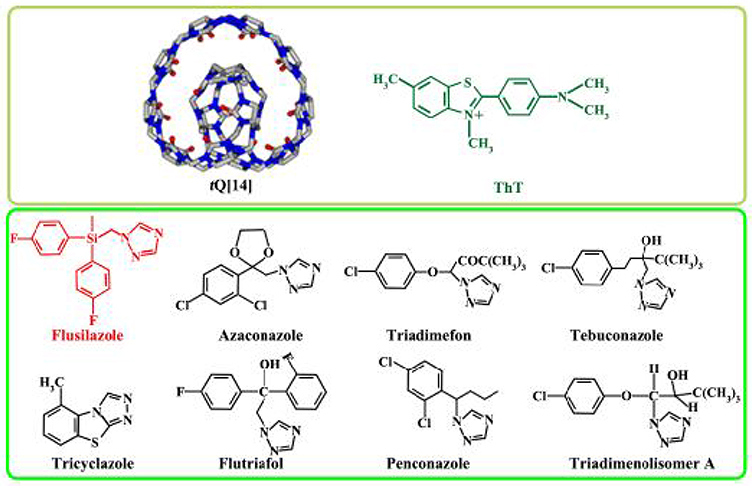
Figure 1. Structures of tQ[14], ThT, and eight fungicide triazoles, namely flusilazole, azaconazole, triadimefon, tebuconazole, tricyclazole, flutriafol, penconazole, and triadimenol isomer A.
The most common analytical method used for the trace determination of triazoles, particularly flusilazole residues in water, is chromatography e.g., liquid chromatography-tandem mass spectrometry (LC-MS/MS) (García-Valcárcel and Tadeo, 2011; Fu et al., 2017), high-performance liquid chromatography (HPLC) with ultraviolet light, diode-array detection (DAD), photodiode-array (PDA) detection (Bordagaray et al., 2013, 2014; Qi et al., 2014; Ma et al., 2016; Zhang Y. H. et al., 2016), gas chromatography (GC) with nitrogen-phosphorous detection (NPD) or electron capture detection (ECD) (Lozowicka et al., 2015; Im et al., 2016), and GC-mass spectrometry (GC-MS) (Tseng et al., 2014; Chu et al., 2015), and with tandem MS (GC-MS/MS) (Xu et al., 2013). Nevertheless, these chromatographic techniques necessitate experienced workers, costly devices, and lengthy specimen preparation. In comparison with the above-mentioned methods, spectrofluorimetry is the most favorable analytical strategy employed to analyze different biological specimens due to its innate simplicity, high sensitivity, and accessibility in the majority of quality-control and clinical laboratories (Yao et al., 2013). However, determination of the existence of many common triazoles cannot be performed directly using typical fluorimetric techniques since the aqueous solutions with triazoles do not have native fluorescence.
It is noteworthy that the complexation of a fluorescent dye with a macrocycle host can induce significant change in its fluorescence (Zhou et al., 2008; Zhang et al., 2012; Kogawa et al., 2014). Such assays depend on an indicator-displacement technique in which the analyte competitively supplants a fluorescent guest that results in an alteration in its fluorescence intensity (You et al., 2015; Sayed and Pal, 2016). Various macrocyclic compounds, including cyclodextrins, calix[n]arenes, and pillar[n]arenas, have long been employed as the hosts in several macrocyclic-dye supramolecular systems (Choudhury et al., 2010; Lau and Heyne, 2010; Liu et al., 2015b; Sun et al., 2015). In particular, cucurbit[n]urils (Q[n]s) are made up of n glycoluril units connected via 2n methylene bridges (Day et al., 2000; Kim et al., 2000; Cong et al., 2016). These hosts have highly polar carbonyl-fringed portals with hydrophobic cavities that can create remarkably stable complexes with different guest molecules (Kim, 2002; Dsouza et al., 2011; Gao et al., 2017; Liu J. et al., 2017; Murray et al., 2017; Yang et al., 2018). The origination of inclusion complexes frequently improves or interrupts the photo-physical and photo-chemical characteristics of the guest molecules. For example, using different Q[n]s to encapsulate some dyes can change the fluorescent characteristics of the guest molecules (Praetorius et al., 2008; Baumes et al., 2009, 2011; Choudhury et al., 2009). The dye@Q[n] complexes can be composed of supramolecular fluorescent probes with high sensitivity and selectivity to recognize and detect analytes, such as metal ions, amines, pesticides, drugs, and DNA (Wheate, 2008; Mohanty et al., 2009; Nau et al., 2009; Zhou et al., 2009; Day and Collins, 2012; Xing et al., 2013; Elbashir and Aboul-Enein, 2015; Liu W. Q. et al., 2017; Xi et al., 2017; Tang et al., 2018; Wang et al., 2018).
Thioflavin T, 3,6-dimethyl-2-(4-dimethylaminophenyl) benzthiazolium cation (ThT) is a benzthiazolium dye (Figure 1). An aqueous solution of ThT reveals a weak native fluorescence. Nevertheless, as revealed by previous evaluations, the fluorescence of ThT in an aqueous solution can be vastly improved in the presence of Q[7], Q[8], and twisted cucurbit[14]uril (tQ[14]). It was found that ThT can form ThT@Q[n] inclusion complexes with different stoichiometric ratios, which can be applied to identify both alkali-metal and alkaline-earth-metal ions (Choudhury et al., 2009, 2010; Mohanty et al., 2009; Wang et al., 2018). In the present study, a procedure was proposed to determine the residues of triazole fungicides, such as azaconazole, triadimefon, tebuconazole, tricyclazole, flutriafol, penconazole, and triadimenol isomer A in aqueous solution (Figure 1).
Results and Discussion
Fluorescence Quenching of ThT@tQ[14] With Flusilazole
Previous studies have proven that the fluorescence of ThT in an aqueous solution over a wide range of interaction ratios can be enhanced by its interaction with tQ[14] via different interaction models due to the multiple cavity features of the tQ[14] molecule and two active moieties (the benzothiazole and dimethylaminobenzene moieties) in ThT (Wang et al., 2018). In the current work, we focused on the inclusion complexes of ThT@tQ[14] with 1:1 and 1:5 interaction ratios in the presence of the eight selected triazoles, the corresponding fluorescence spectra of which can be seen in Figure 2 and Figures S1–S3. A significant fluorescence quenching of the ThT@tQ[14] inclusion complex probe (1:1) was only observed upon increasing the amount of flusilazole; ~60% of the fluorescence intensity was lost when Cflusilazole/CThT@tQ[14] was ~4 (Figure 2A). Figure 2B shows the plot of the fluorescence intensity at λem = 488 nm upon increasing the flusilazole concentration; Kobserved was calculated by the nonlinear-least-squares method to be (8.2 ± 0.4) × 105 L/mol obtained (Figure S4), which is likely due to the influence of the interaction of tQ[14] with flusilazole on the interaction of tQ[14] with ThT. Moreover, the ThT@tQ[14] fluorescent probe presented high selectivity for flusilazole because the probe showed little or almost no response to the other seven triazole fungicides studied (Figure 3 and Figure S1). The ThT@tQ[14] inclusion complex probe at a molar ratio of 1:5 was also selected to investigate its response to triazole pesticides with the addition of flusilazole also leading to a fluorescence quenching of the ThT@tQ[14] inclusion complex probe; only ~22% of the fluorescence intensity was lost when Cflusilazole/CThT@tQ[14] was ~4 (Figure S2). However, the ThT@tQ[14] fluorescent probe also showed little or almost no response to the other triazole fungicides studied (Figure S3).
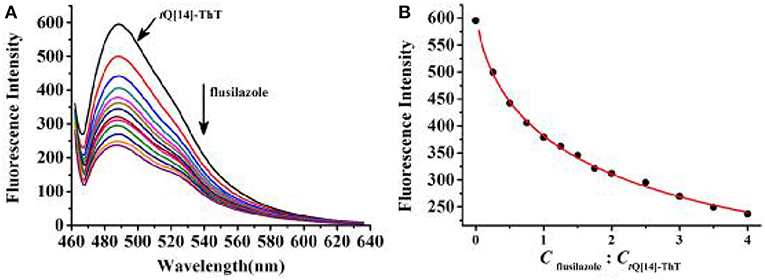
Figure 2. (A) Fluorescence titration spectra (λex = 448 nm) for ThT@tQ[14] (1:1, 1 μM) in the presence of flusilazole of the following different stoichiometries in μM: 0, 0.25, 0.5, 0.75, 1.0, 1.25, 1.5, 1.75, 2.0, 2.5, 3.0, 3.5, and 4.0; (B) Plot obtained for fluorescence intensity of ThT@tQ[14] vs. Cflusilazole/CThT@tQ[14].
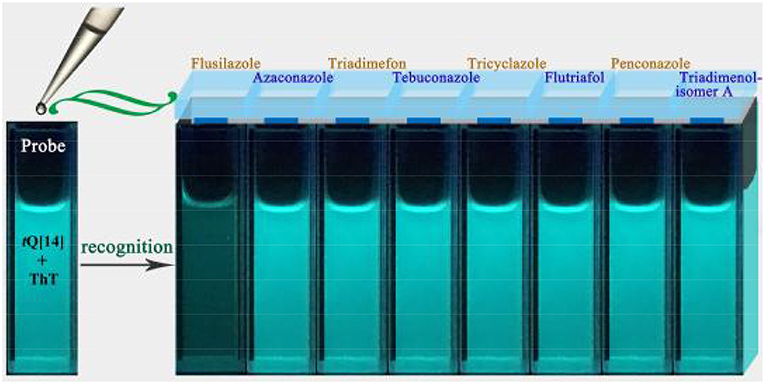
Figure 3. Fluorescence images of ThT@tQ[14] (50 μM) upon addition of eight triazole pesticides (50 μM) under UV-lamp irradiation (365 nm).
Analytical Characteristics of ThT@tQ[14] Probe
The standard calibration curve (Figure S5) of the complexes of flusilazole with ThT@tQ[14] (1:1) was obtained using the plot of fluorescence intensity F compared to flusilazole concentration. The linear range was (0.0–1.0) × 10−6 mol/L for flusilazole with a correlation coefficient of 0.9888. For flusilazole, the limit of detection (LOD) was 1.27 × 10−8 mol/L and the linear regression equation F = −216.24C+576.26. Although the ThT@tQ[14] (1:5) inclusion complex was less sensitive to flusilazole, it still exhibited a very low LOD (2.80 × 10−8 mol/L) and the linear regression equation was F = −98.25C+863.26 (Figure S6).
Effects of Interfering Substances
In the present work, the impacts of different common interfering substances on the determination of flusilazole using the ThT@tQ[14] probe were also investigated. The tolerance limit was established as the concentration of an added interfering substance that causes a relative error of < ±5% in the flusilazole determination. The samples consisted of a fixed amount of flusilazole (1.0 μM) and ThT@tQ[14] (1.0 μM) with an increasing amount of different interfering substances; the corresponding results are shown in Table 1 and Figure S7. It is clear that the determination was free from interference in the presence of the common metal ions and anions in aqueous solutions, except Ca2+. Structural analysis revealed that tQ[14] had a similar portal size to those of Q[6] and Q[7] (Cheng et al., 2013; Liu et al., 2015a; Li et al., 2016; Zhang J. et al., 2016; Zhang et al., 2017), and a higher portal carbonyl intensity (Cheng et al., 2013; Liu et al., 2015a; Zhang J. et al., 2016; Zhang et al., 2017), which could exhibit a higher affinity for metal cations, particularly the Ca2+ cation (Cheng et al., 2013; Qiu et al., 2017; Wang et al., 2018). The titration fluorescence spectra recorded for the tQ[14]-ThT-flusilazole (1:1:1) system upon increasing the amount of Ca2+ showed that the fluorescence of the ternary interaction system could be further decreased due to the influence of the Ca2+ cation (Figure S8). The titration 1H nuclear magnetic resonance (NMR) spectra for the tQ[14]-ThT-flusilazole (1:1:1) system in the presence of Ca2+ also showed that the guest molecules, ThT and flusilazole, were gradually pushed out from the cavity area upon increasing the amount of Ca2+ (Figure S9).
Preliminary Exploration of the Response Mechanism of ThT@tQ[14] Fluorescent Probe Toward Flusilazole
Titration 1H NMR Spectra
We have found that the ThT@tQ[14] fluorescent probe was selectively sensitive toward flusilazole and could be used to detect flusilazole via a significant fluorescence quenching process. Why is the ThT@tQ[14] fluorescent probe selectively sensitive to flusilazole? How does the flusilazole molecule influence the interaction between the ThT molecule with the tQ[14] host molecule? What could the interaction mode be? To understand the selectivity and response mechanism of the ThT@tQ[14] fluorescent probe toward flusilazole, titration 1H NMR spectra were recorded upon the gradual addition of one of the selected triazole fungicides into the solution of the ThT@tQ[14] (1:1) fluorescent probe. The detailed interaction of ThT and tQ[14] has been discussed in a previous work (Figures 4A,B) (Wang et al., 2018). Figure 4F shows the 1H NMR spectrum for the flusilazole in the presence of tQ[14]. When compared with the 1H NMR spectrum for the unbound flusilazole molecule (Figure 4G), the proton resonances corresponding to H5 and H6 on the triazole moiety of the bound flusilazole molecule underwent slightly down-field shifts (0.01 and 0.01 ppm, respectively), whereas the other proton resonances experienced slightly up-field shifts, suggesting that a weak interaction existed between tQ[14] and the flusilazole guest molecule or π··· π stacking of the aryl groups of the flusilazole guest molecules. Figures 4C–E show the titration 1H NMR spectra for the tQ[14]-ThT complex upon increasing the amount of flusilazole. The addition of flusilazole appeared to pull the bound ThT out of the interaction area of the tQ[14] host because the proton resonances were closer to those of the unbound ThT molecule. However, the proton resonances of flusilazole, in particular, the H5 and H6 experienced down-field shift, suggesting that ThT was still interacting with the host and flusilazole was located in the de-shielding region of tQ[14] (probably at portal area). Similar phenomena could be observed for the other tQ[14]-ThT-triazole fungicide systems, in which the addition of the triazole fungicide could lead to a change in the interaction between tQ[14] and ThT. Moreover, both ThT and the triazole fungicide exhibited an interaction with the tQ[14] host (Figures S10–S16). At first, it seemed that it was difficult to obtain the required information using the titration 1H NMR method. A closer inspection revealed that the addition of different triazole fungicides could lead to different chemical shifts for the proton resonances corresponding to the ThT molecule, and the effects of the different triazole fungicides on the bound ThT were compared with the chemical shift corresponding to proton Hg. The data in Table 2 reveal that flusilazole resulted in the larger change in the chemical-shift values (Δδ = 0.05) when the tQ[14]-ThT-triazole fungicide ratio was 1:1:2.
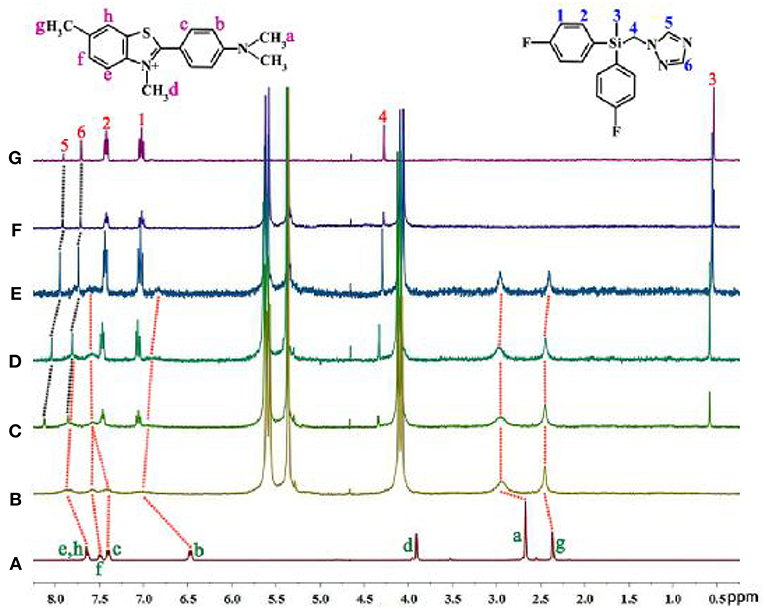
Figure 4. 1H NMR spectra (400 MHz, D2O) for (A) ThT, (B) tQ[14]-ThT (1:1), (C) tQ[14]-ThT-flusilazole (1:1:0.5), (D) tQ[14]-ThT-flusilazole (1:1:1), (E) tQ[14]-ThT-flusilazole (1:1:2), (F) tQ[14]-flusilazole, and (G) flusilazole.
2D diffusion-ordered NMR spectroscopy (DOSY) experiments were performed to afford further evidence for the formation of the tQ[14]-ThT-flusilazole (1:1:1) ternary interaction species. Figure S17 depicts the DOSY spectra of tQ[14], ThT, flusilazole, and tQ[14]-ThT-flusilazole (1:1:1) ternary interaction species in D2O at 298 K and the corresponding diffusion coefficients (D) are 3.49 × 10−10, 5.46 × 10−10, 3.88 × 10−10, and 2.92 × 10−10, respectively. According to the values of four species, the tQ[14]-ThT-flusilazole (1:1:1) ternary interaction species is the smallest, suggesting that the ternary species could be the largest species, moreover, all the proton signals of the host and the guest display the same diffusion coefficient (D = 2.92 × 10−10 · m2 · s−1), indicating that they are part of the same species.
Isothermal Titration Calorimetry
Isothermal titration calorimetry (ITC) experiments were conducted to determine the association constants and thermodynamic parameters of the host-guest interaction between tQ[14] and ThT with the triazole fungicides in an aqueous solution to further explore the fluorescence-quenching mechanism of ThT@tQ[14] with flusilazole (Figures S18–S26). From the data acquired (Table 3), all the association constants obtained for the triazole fungicides@tQ[14] complexes [(1.46–8.39) × 105 L/mol] were slight larger than that of the ThT@tQ[14] complex (1.28 × 105 L/mol), but there are no significant differences. The titration 1H NMR study has proved that these pesticides could not replace ThT to form pesticide@tQ[14] inclusion complexes.
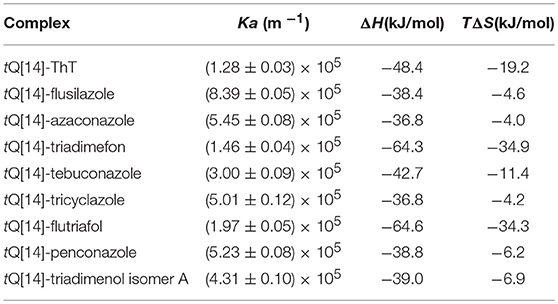
Table 3. Thermodynamic parameters of tQ[14] and ThT with eight triazole pesticides in aqueous solution at 298.15 K.
The fluorescence enhancement mechanism of ThT could be due to the restriction of the freely rotating dimethylamine group on the benzene moiety, making the lone pair electrons on nitrogen atoms conjugate to the ThT aromatic system. The shell-like cavity structure of tQ[14] can provide such controlled environment, the inclusion of dimethylamino phenyl moiety of ThT inhibited dimethylamino free rotation, moreover, the shell-like cavity structure of tQ[14] could prevent ThT from threading through the side cavity of tQ[14] (Liu et al., 2015a; Li et al., 2016). Therefore, we can observe ThT fluorescence enhancement. Unlike HMeQ[6] and Q[7] which have similar portal sizes to that of the side portals of tQ[14], ThT could thread through their cavities, and show a weak fluorescence emission (referring the fluorescence spectra as shown in Figure S27). On the other hand, the fluorescence emission of ThT is also affected by its electron-pushing group (dimethylamine group) and electron-withdrawing group (quaternary ammonium moiety). The titration 1H NMR spectra could provide the interaction images of this ternary system: the flusilazole was located in the de-shielding region of tQ[14] (probably at portal area), which caused the chemical shift change of ThT proton resonance to move toward the free ThT, in particular, the interaction of the azole moiety and quaternary ammonium moiety could weaken the electron-withdrawing capacity of quaternary ammonium moiety, resulting in partial quenching of the fluorescence of the tQ[14]-ThT-flusilazole interaction system (Figure 5). According to the suggested interaction mode, whether the fluorescence quenching of ThT@tQ[14] is caused mainly depends on the interaction of the azole moiety of pesticides and quaternary ammonium moiety of ThT and the ability of pesticides to pull ThT out of the side cavity of tQ[14]. While the NMR and ITC measurement results showed that the flusilazole has the biggest impact, although the differences from other triazole fungicides are subtle.
Conclusions
In order to further expand the application of Q[n]-based host-guest chemistry, especially the host-guest complexes with fluorescent properties, in detection and recognition, a known host-guest complex of ThT@tQ[14] (1:1) was used as a fluorescent probe to determine non-fluorescence triazole fungicides, including flusilazole, azaconazole, triadimefon, tebuconazole, tricyclazole, flutriafol, penconazole, and triadimenol isomer A. This new and simple fluorometry method proved to be highly selective and sensitive to one of triazole fungicides—flusilazole, and the determination was free from interference by the common metal ions and anions in aqueous solutions, except Ca2+. The investigation of the response mechanism revealed that a side-cavity of the tQ[14] host includes the dimethylamino phenyl moiety of ThT, resulting in the ThT fluorescence enhancement; the addition of flusilazole results in the interaction of the azole moiety of flusilazole and quaternary ammonium moiety of ThT, which could weaken the electron-withdrawing capacity of quaternary ammonium moiety, resulting in partial quenching of the fluorescence of the tQ[14]-ThT-flusilazole interaction system. This unusual phenomenon results from the novel structural feature of tQ[14], namely that tQ[14] possesses a central-cavity and two of the same side-cavities.
Experiment
Materials
tQ[14] was set up and purified in our laboratory according to a procedure detailed in the literature (Cheng et al., 2013). Analytical grade flusilazole (99.5%), azaconazole (99.5%), triadimefon (99.0%), tebuconazole (99.0%), tricyclazole (99.0%), flutriafol (98.6%), penconazole (99.5%), and triadimenol isomer A (95.4%) were purchased from Dr. Ehrenstorfer GmbH (Augsburg, Germany) and used as-received without any further purification. Double-distilled water was used for each of the experiments.
1H NMR
Each of the 1H NMR spectra, including those for titration experiments, were documented at 25°C on a JEOL JNM-ECZ400s spectrometer using SiMe4 as an internal reference. D2O was utilized as a field-frequency lock and the chemical shifts documented in parts per million (ppm).
ITC
Microcalorimetric experiments were conducted with a Nano ITC (TA, USA) isothermal titration calorimeter. Then, 25 consecutive 10-μL aliquots of a 1 mM tQ[14] solution were introduced into the microcalorimetric reaction cell, which contained 1.3 mL of a 0.1-mM guest molecule solution at 25°C. The heat of reaction was corrected for the heat of dilution of the guest molecule solution, which was determined in a separate experiment. Each of the solutions were de-gassed before the titration via ultrasonication. Computer simulations (curve fitting) were conducted with Nano ITC analysis software.
Fluorescence Titration
The fluorescence spectra of the host-guest complexes were documented at 25°C using a Varian Cary Eclipse spectrofluorometer (Varian, Inc., Palo Alto, CA, USA). Stock solutions of tQ[14] (1 × 10−3 mol L−1), ThT (1 × 10−3 mol L−1), and flusilazole (1 × 10−4 mol L−1) were set up in water. Working solutions were set up by diluting the stock solutions to the necessary concentrations.
A tQ[14]-ThT (1:1) complex solution was set up at a fixed concentration of 1 × 10−6 mol·L−1 in H2O, which was subsequently combined with flusilazole at guest/host ratios of 0, 0.25:1, 0.5:1, 0.75:1, 1:1, …, and 4:1. Fluorescence spectrophotometric titrations were established as detailed prior (λex = 448 nm and λem = 488 nm). For each experiment, three replicate measurements were recorded.
LOD Measurement
The calculation technique used for the LOD was based on the standard derivation of 10 measurements without the guest molecule (σ) and the slope of the linear calibration curve (K) based on the formula LOD = 3σ/K.
The standard deviation of 10 measurements without the guest molecule could be determined based on the following relationship: , where n is the number of measurements (n = 11).
Author Contributions
YF, R-HG, YH, and BB undertook the acquisition and analysis of data for the work. ZT and XX drafted the work and revised it critically for important intellectual content.
Conflict of Interest Statement
The authors declare that the research was conducted in the absence of any commercial or financial relationships that could be construed as a potential conflict of interest.
Acknowledgments
We acknowledge the support of the National Natural Science Foundation of China (Grant No. 21562015), the Creative Research Groups of Guizhou Provincial Education Department (2017-028), and the Innovation Program for High-level Talents of Guizhou Province (No. 2016-5657).
Supplementary Material
The Supplementary Material for this article can be found online at: https://www.frontiersin.org/articles/10.3389/fchem.2019.00154/full#supplementary-material
References
Baumes, L. A., Buaki, M., Jolly, J., Corma, A., and Garcia, H. (2011). Fluorimetric detection and discrimination of a-amino acids based on tricyclic basic dyes and cucurbiturils supramolecular assembly. Tetrahedron Lett. 52, 1418–1421. doi: 10.1016/j.tetlet.2011.01.071
Baumes, L. A., Sogo, M. B., Montes-Navajas, P., Corma, A., and Garcia, H. (2009). First colorimetric sensor array for the identification of quaternary ammonium salts. Tetrahedron Lett. 50, 7001–7004. doi: 10.1016/j.tetlet.2009.09.165
Bordagaray, A., García-Arrona, R., and Millán, E. (2013). Development and application of a screening method for triazole fungicide determination in liquid and fruit samples using solid-phase microextraction and HPLC-DAD. Anal. Methods 5, 2565–2571. doi: 10.1039/c3ay26433e
Bordagaray, A., García-Arrona, R., and Millán, E. (2014). Determination of triazole fungicides in liquid samples using ultrasound-assisted emulsification microextraction with solidification of floating organic droplet followed by high-performance liquid chromatography. Food Anal. Methods 7, 1195–1203. doi: 10.1007/s12161-013-9733-2
Cheng, X. J., Liang, L. L., Chen, K., Ji, N. N., Xiao, X., Zhang, J. X., et al. (2013). Twisted cucurbit[14]uril. Angew. Chem. Int. Ed. 52, 7252–7255. doi: 10.1002/anie.201210267
Choudhury, S. D., Mohanty, J., Pal, H., and Bhasikuttan, A. C. (2010). Cooperative metal ion binding to a cucurbit[7]uril-thioflavin T complex: demonstration of a stimulus-responsive fluorescent supramolecular capsule. J. Am. Chem. Soc. 132, 1395–1401. doi: 10.1021/ja908795y
Choudhury, S. D., Mohanty, J., Upadhyaya, H. P., Bhasikuttan, A. C., and Pal, H. (2009). Photophysical studies on the noncovalent interaction of thioflavin T with cucurbit[n]uril macrocycles. J. Phys. Chem. B 113, 1891–1898. doi: 10.1021/jp8103062
Chu, S. P., Tseng, W. C., Kong, P. H., Huang, C. K., Chen, J. H., Chen, P. S., et al. (2015). Up-and-down-shaker-assisted dispersive liquid–liquid microextraction coupled with gas chromatography–mass spectrometry for the determination of fungicides in wine. Food Chem. 185, 377–382. doi: 10.1016/j.foodchem.2015.04.015
Cong, H., Ni, X. L., Xiao, X., Huang, Y., Zhu, Q. J., Xue, S. F., et al. (2016). Synthesis and separation of cucurbit[n]urils and their derivatives[J]. Org. Biomol. Chem. 14, 4335–4364. doi: 10.1039/C6OB00268D
Day, A. I., Arnold, A. P., and Blanch, R. J. (2000). Method for synthesis of cucurbiturils. PCT Int. Appl. 112.
Day, A. I., and Collins, J. G. (2012). Cucurbituril receptors and drug delivery. Supramol. Chem. 3, 983–1000. doi: 10.1002/9780470661345.smc056
Dsouza, R. N., Pischel, U., and Nau, W. M. (2011). Fluorescent dyes and their supramolecular host/guest complexes with macrocycles in aqueous solution. Chem. Rev. 111, 7941–7980. doi: 10.1021/cr200213s
Elbashir, A., and Aboul-Enein, H. Y. (2015). Supramolecular analytical application of cucurbit[n]urils using fluorescence spectroscopy. Crit. Rev. Anal. Chem. 45, 52–61. doi: 10.1080/10408347.2013.876354
Fu, Y., Yang, T., Zhao, J., Zhang, L., Chen, R. X., and Wu, Y. L. (2017). Determination of eight pesticides in Lycium barbarum by LC-MS/MS and dietary risk assessment. Food Chem. 218, 192–198. doi: 10.1016/j.foodchem.2016.09.014
Gao, R. H., Chen, L. X., Chen, K., Tao, Z., and Xiao, X. (2017). Development of hydroxylated cucurbit[n]urils, their derivatives and potential applications. Coordinat. Chem. Rev. 348, 1–24. doi: 10.1016/j.ccr.2017.07.017
García-Valcárcel, A. I., and Tadeo, J. L. (2011). Determination of azoles in sewage sludge from Spanish wastewater treatment plants by liquid chromatography-tandem mass spectrometry. J. Sep. Sci. 34, 1228–1235. doi: 10.1002/jssc.201000814
Im, S. J., Rahman, M. M., El-Aty, A. M. A., Kim, S. W., Kabir, H., Farha, W., et al. (2016). Simultaneous detection of fluquinconazole and flusilazole in lettuce using gas chromatography with a nitrogen phosphorus detector: decline patterns at two different locations. Biomed. Chromatogr. 30, 946–952. doi: 10.1002/bmc.3634
Kim, J., Jung, I. S., Kim, S. Y., Lee, E., Kang, J. K., Sakamoto, S., et al. (2000). New cucurbituril homologues: syntheses, isolation, characterization, and X-ray crystal structures of cucurbit[n]uril (n = 5, 7, and 8). J. Am. Chem. Soc. 122, 540–541. doi: 10.1021/ja993376p
Kim, K. (2002). Mechanically interlocked molecules incorporating cucurbituril and their supramolecular assemblies. Chem. Soc. Rev. 31, 96–107. doi: 10.1039/a900939f
Kogawa, C., Zoppi, A., Quevedo, M. A., Salgado, H. R. N., and Longhi, M. R. (2014). Increasing doxycycline hyclate photostability by complexation with beta-cyclodextrin. AAPS PharmSciTech. 15, 1209–1217. doi: 10.1208/s12249-014-0150-7
Lau, V., and Heyne, B. (2010). Calix[4]arene sulfonate as a template for forming fluorescent thiazole orange H-aggregates. Chem. Commun. 46, 3595–3597. doi: 10.1039/c002128h
Li, Q., Qiu, S. C., Chen, K., Zhang, Y., Wang, R. B., Huang, Y., et al. (2016). Encapsulation of alkyldiammonium ions within two different cavities of twisted cucurbit[14]uril. Chem. Commun. 52, 2589–2592. doi: 10.1039/C5CC08642F
Liu, J., Lan, Y., Yu, Z. Y., Tan, C. S. Y., Parker, R. M., Abell, C., et al. (2017). Cucurbit[n]uril-based microcapsules self-assembled within microfluidic droplets: a versatile approach for supramolecular architectures and materials. Acc. Chem. Res. 50, 208–217. doi: 10.1021/acs.accounts.6b00429
Liu, Q., Li, Q., Cheng, X. J., Xi, Y. Y., Xiao, B., Xiao, X., et al. (2015a). A novel shell-like supramolecular assembly of 4,4-bipyridyl derivatives and a twisted cucurbit[14]uril molecule. Chem. Commun. 51, 9999–10001. doi: 10.1039/C5CC02653A
Liu, Q., Yang, Y., Li, H., Zhu, R., Shao, Q., Yang, S., et al. (2015b). NiO nanoparticles modified with 5,10,15,20-tetrakis(4-carboxyl pheyl)-porphyrin: promising peroxidase mimetics for H2O2 and glucose detection. Biosens Bioelectron. 64, 147–153. doi: 10.1016/j.bios.2014.08.062
Liu, W. Q., Samanta, S. K., Smith, B. D., and Isaacs, L. (2017). Synthetic mimics of biotin/(strept)avidin. Chem. Soc. Rev. 46, 2391–2403. doi: 10.1039/C7CS00011A
Lozowicka, B., Abzeitova, E., Sagitov, A., Kaczynski, P., Toleubayev, K., and Li, A. (2015). Studies of pesticide residues in tomatoes and cucumbers from Kazakhstan and the associated health risks. Environ. Monit. Assess. 187:609. doi: 10.1007/s10661-015-4818-6
Ma, J. P., Yao, Z. D., Hou, L. W., Lu, W. H., Yang, Q. P., Li, J. H., et al. (2016). Metal organic frameworks (MOFs) for magnetic solid-phase extraction of pyrazole/pyrrole pesticides in environmental water samples followed by HPLC-DAD determination. Talanta 161, 686–692. doi: 10.1016/j.talanta.2016.09.035
Mohanty, J., Choudhury, S. D., Upadhyaya, H. P., Bhasikuttan, A. C., and Pal, H. (2009). Control of the supramolecular excimer formation of thioflavin T within acucurbit[8]uril host: a fluorescence on/off mechanism. Chem. Eur. J. 15, 5215–5219. doi: 10.1002/chem.200802686
Murray, J., Kim, K., Ogoshi, T., Yao, W., and Gibb, B. C. (2017). The aqueous supramolecular chemistry of cucurbit[n]urils, pillar[n]arenes and deep-cavity cavitands. Chem. Soc. Rev. 46, 2479–2496. doi: 10.1039/C7CS00095B
Nau, W. M., Ghale, G., Hennig, A., Bakirci, H., and Bailey, D. M. (2009). Substrate-selective supramolecular tandem assays: monitoring enzyme inhibition of arginase and diamine oxidase by fluorescent dye displacement from calixarene and cucurbituril macrocycles. J. Am. Chem. Soc. 131, 11558–11570. doi: 10.1021/ja904165c
Praetorius, A., Bailey, D. M., Schwarzlose, T., and Nau, W. M. (2008). Design of a fluorescent dye for indicator displacement from cucurbiturils: a macrocycle-responsive fluorescent switch operating through a pKa shift. Org. Lett. 10, 4089–4092. doi: 10.1021/ol8016275
Qi, R. F., Jiang, H. C., Liu, S. X., and Jia, Q. (2014). Preconcentration and determination of pesticides with graphene-modified polymer monolith combined with high performance liquid chromatography. Anal. Methods 6, 1427–1434. doi: 10.1039/c3ay41688g
Qiu, S. C., Chen, K., Wang, Y., Hua, Z. Y., Li, F., Huang, Y., et al. (2017). Crystal structure analysis of twisted cucurbit [14]uril conformations. Inorg. Chem. Commun. 86, 49–53. doi: 10.1016/j.inoche.2017.09.024
Sayed, M., and Pal, H. (2016). Supramolecularly assisted modulations in chromophoric properties and their possible applications: an overview. J. Mater. Chem. C 4, 2685–2706. doi: 10.1039/C5TC03321G
Sun, N. N., Xiao, X., Li, W., and Jiang, J. Z. (2015). Multi-stimuli sensitive behavior of novel bodipy-involved pillar[5]arene-based fluorescent [2]rotaxane and its supramolecular gel. Adv. Sci. 2, 1500082–1500090. doi: 10.1002/advs.201500082
Tang, Q., Zhang, J., Sun, T., Wang, C. H., Huang, Y., Zhou, Q. D., et al. (2018). A turn-on supramolecular fluorescent probe for sensing benzimidazole fungicides and its application in living cell imaging. Spectrochim. Acta Part A Mol. Biomol. Spectrosc. 191, 372–376. doi: 10.1016/j.saa.2017.10.042
Tseng, W. C., Chu, S. P., Kong, P. H., Huang, C. K., Chen, J. H., Chen, P. S., et al. (2014). Water with low concentration of surfactant in dispersed solvent-assisted emulsion dispersive liquid–liquid microextraction for the determination of fungicides in wine. J. Agric. Food Chem. 62, 9059–9065. doi: 10.1021/jf5036096
Wang, H., Tang, Q., Zhang, J., Yao, Y. Q., Xiao, X., Huang, Y., et al. (2018). Alkaline earth cation-mediated photoluminescent complexes of thioflavin T with twisted cucurbit[14]uril. N. J. Chem. 42, 9244–9251. doi: 10.1039/C7NJ04115B
Wheate, N. J. (2008). Improving platinum(II)-based anticancer drug delivery using cucurbit[n]urils. J. Inorgan. Biochem. 102, 2060–2066. doi: 10.1016/j.jinorgbio.2008.06.005
Xi, Y. Y., Tang, Q., Huang, Y., Tao, Z., Xue, S. F., Zhou, Q. D., et al. (2017). A novel fluorescent indicator displacement assay for sensing the anticancer drug gefitinib. Supramol. Chem. 29, 229–235. doi: 10.1080/10610278.2016.1202413
Xing, X. Q., Zhou, Y. Y., Sun, J. Y., Tang, D. B., Li, T., and Wu, K. (2013). Determination of paraquat by cucurbit[7]uril sensitized fluorescence quenching method. Anal. Lett. 46, 694–705. doi: 10.1080/00032719.2012.729240
Xu, M., Lu, S. Y., Chen, D. J., Lan, J. C. G, Zhang, Z., et al. (2013). Determination of ten pesticides of pyrazoles and pyrroles in tea by accelerated solvent extraction coupled with gas chromatography-tandem mass spectrometry. Chinese J. Chromatogr. 31, 218–222. doi: 10.3724/SP.J.1123.2012.10003
Yang, L. G., Kan, J. L., Wang, X., Zhang, Y. H., Tao, Z., Liu, Q. Y., et al. (2018). Study on the binding interaction of the α,α′,δ,δ′-tetramethylcucurbit[6]uril with biogenic amines in solution and the solid state. Front. Chem. 6:289. doi: 10.3389/fchem.2018.00289
Yao, F. H., Liu, H. L., Wang, G. Q., Du, L. M., Yin, X. F., and Fu, Y. L. (2013). Determination of paraquat in water samples using a sensitive fluorescent probe titration method. J. Environ. Sci. 25, 1245–1251. doi: 10.1016/S1001-0742(12)60124-7
You, L., Zha, D. J., and Anslyn, E. V. (2015). Recent advances in supramolecular analytical chemistry using optical sensing. Chem. Rev. 115, 7840–7892. doi: 10.1021/cr5005524
Zhang, H. Y., Liu, L. L., Gao, C., Sun, R. Y., and Wang, Q. C. (2012). Enhancing photostability of cyanine dye by cucurbituril encapsulation. Dyes Pigm. 94, 266–270. doi: 10.1016/j.dyepig.2012.01.022
Zhang, J., Tang, Q., Gao, Z. Z., Huang, Y., Xiao, X., and Tao, Z. (2017). Stimuli-responsive supramolecular assemblies between twisted cucurbit[14]uril and hemicyanine dyes and their analysis application. J. Phys. Chem. B 121, 11119–11123. doi: 10.1021/acs.jpcb.7b10285
Zhang, J., Xi, Y. Y., Li, Q., Tang, Q., Wang, R. B., Huang, Y., et al. (2016). Supramolecular recognition of amino acids by twisted cucurbit[14]uril. Chem. Asian J. 11, 2250–2254. doi: 10.1002/asia.201600803
Zhang, Y. H., Zhang, Y., Zhao, Q. Y., Chen, W. J., and Jiao, B. N. (2016). Vortex-assisted ionic liquid dispersive liquid-liquid microextraction coupled with high-performance liquid chromatography for the determination of triazole fungicides in fruit juices. Food Anal. Methods 9, 596–604. doi: 10.1007/s12161-015-0223-6
Zhou, Y. Y. P, Yu, H., Zhang, L., Xu, H. W., Wu, L., et al. (2009). A new spectrofluorometric method for the determination of nicotine base on the inclusion interaction of methylene blue and cucurbit[7]uril. Microchim. Acta 64, 63–68. doi: 10.1007/s00604-008-0032-3
Keywords: twisted cucurbit[14]uril, thioflavin T, fluorescent probe, triazole fungicides, flusilazole
Citation: Fan Y, Gao R-H, Huang Y, Bian B, Tao Z and Xiao X (2019) Supramolecular Fluorescence Probe Based on Twisted Cucurbit[14]uril for Sensing Fungicide Flusilazole. Front. Chem. 7:154. doi: 10.3389/fchem.2019.00154
Received: 30 August 2018; Accepted: 01 March 2019;
Published: 21 March 2019.
Edited by:
Angela Casini, Cardiff University, United KingdomReviewed by:
Dong-Sheng Guo, Nankai University, ChinaSimin Liu, Wuhan University of Science and Technology, China
Copyright © 2019 Fan, Gao, Huang, Bian, Tao and Xiao. This is an open-access article distributed under the terms of the Creative Commons Attribution License (CC BY). The use, distribution or reproduction in other forums is permitted, provided the original author(s) and the copyright owner(s) are credited and that the original publication in this journal is cited, in accordance with accepted academic practice. No use, distribution or reproduction is permitted which does not comply with these terms.
*Correspondence: Zhu Tao, gzutao@263.net
Xin Xiao, gyhxxiaoxin@163.com