Relationship and Interconversion Between Superhydrophilicity, Underwater Superoleophilicity, Underwater Superaerophilicity, Superhydrophobicity, Underwater Superoleophobicity, and Underwater Superaerophobicity: A Mini-Review
- 1State Key Laboratory for Manufacturing System Engineering and Shaanxi Key Laboratory of Photonics Technology for Information, School of Electronic Science and Engineering, Xi'an Jiaotong University, Xi'an, China
- 2School of Mechanical Engineering, Xi'an Jiaotong University, Xi'an, China
Superwetting surfaces have received increasing attention because of their rich practical applications. Although various superwettabilities are independently achieved, the relationship between those superwettabilities is still not well-clarified. In this mini-review, we show that superhydrophilicity, underwater superoleophilicity, underwater superaerophilicity, superhydrophobicity, underwater superoleophobicity, and underwater superaerophobicity can be obtained on a same structured surface by the combination of hierarchical surface microstructures and proper chemistry. The relationship and interconversion between the above-mentioned different superwettabilities are also well-discussed. We believe that the current discussion and clarification of the relationship and interconversion between different superwettabilities has important significance in the design, fabrication, and applications of various superwetting materials.
Introduction
As three common states of matter, solid, liquid, and gas form different kinds of solid/liquid/gas interfaces. The materials with extreme wettability have received increasing attention because of their wide practical applications in waterproof coating (Yong et al., 2017c), anti-icing/snowing/fogging (Lv et al., 2014; Kreder et al., 2016; Chu et al., 2019), self-cleaning coating (Nishimoto and Bhushan, 2013; Yong et al., 2013c, 2014b; Ragesh et al., 2014), the manipulation of small droplets (Wang et al., 2011; Yong et al., 2013b, 2015b), corrosion resistance (Pan et al., 2013; Zhan et al., 2018), oil/water separation (Xue et al., 2014; Wang B. et al., 2015; Yong et al., 2016a,b, 2018e, 2019c; Bian et al., 2020), fog collection (Zhang et al., 2017), cell engineering (Stratakis et al., 2011; Shen et al., 2012), anti-biological adhesion (Genzer and Efimenko, 2006; Yong et al., 2018f), drag reduction in water (Shi et al., 2007), lab on a chip (Kwon et al., 2007; Vitale et al., 2013), microfluidic system (Songok et al., 2014; Wang S. et al., 2015), liquid patterning (Jokinen et al., 2008; Yong et al., 2015c), enhanced buoyancy (Yong et al., 2014c; Zhan et al., 2019), submarine gas collection (Yong et al., 2018c,d). After billions of years of evolution, creatures in nature have nearly perfect structure and function. Wherein, many organisms have evolved special surface wettability. For example, lotus leaf has the self-cleaning function (Barthlott and Neinhuis, 1997), water strider can walk on water surface (Gao and Jiang, 2004), the butterfly can shake off raindrops and fly in the rain (Zheng et al., 2007), the eyes of mosquito can repel fog (Gao et al., 2007), the fish scale cannot be polluted by oil in water (Liu et al., 2009; Yong et al., 2018b), and desert beetle, cacti, and spider silk have the capacity of harvesting water in dry air (Parker and Lawrence, 2001; Zheng et al., 2010; Ju et al., 2012). It is demonstrated that the surface wettability is primarily determined by the surface composition and the surface morphology of a solid substrate (Yong et al., 2013a; Bellanger et al., 2014; Jiang et al., 2015; Wen et al., 2015; Su et al., 2016; Bai et al., 2020). The study related to the surface wettability becomes a current research focus. Inspired by animals and plants in nature, various kinds of superwettabilities have been achieved by different microfabrication methods, such as superhydrophobicity, superhydrophilicity, underwater superoleophobicity and superoleophilicity, and underwater superaerophobicity and superaerophilicity (Teisala et al., 2014; Tian et al., 2014; Yong et al., 2014a, 2019b; Wang J. N. et al., 2015; Liu et al., 2017). Water droplet, oil droplet, or gas bubble on the material surfaces with superhydrophilicity, superoleophilicity, or superaerophilicity has a contact angle (CA) <10°, while it has a CA larger than 150° on the material surfaces with superhydrophobicity, superoleophobicity, or superaerophobicity, respectively (Tian et al., 2014; Wen et al., 2015; Yong et al., 2015a, 2017a,c; Su et al., 2016; Liu et al., 2017). Although these superwettabilities are independently achieved, the relationship between different superwettabilities is still not well-discussed. The clear relationship between different superwettabilities is important for the design of various superwetting materials and the interconversion between different superwettabilities.
In this review, the relationship and the interconversion between different superwettabilities are discussed and summarized. Taking the hydrophilic Al substrate and the hydrophobic polydimethylsiloxane (PDMS) substrate as the examples, we show that various kinds of superwettabilities can be obtained on the same structured surface. The formation mechanism of different superwettabilities and their interconversion are well-discussed and clarified.
Achievement of Various Superwettabilities
Superwettability can be designed by combining proper surface microstructures and chemistry (Yong et al., 2013a, 2017c; Bellanger et al., 2014; Jiang et al., 2015; Wen et al., 2015; Su et al., 2016; Bai et al., 2020). Al is a typical hydrophilic substrate. Figures 1A,B shows the scanning electron microscopy (SEM) images of the Al surface with rough surface microstructure (Yong et al., 2019a). The surface microstructure is created by laser ablation. There are periodic microgrooves with a width of ~35 μm, a depth of ~21 μm, and a period of 40 μm forming on the Al surface. The top of the ridges between the microgrooves is randomly coated with rich nanoparticles. A small water droplet spreads out on the structured Al surface after touching the surface, with a final water CA (WCA) of 1.7° (Figure 1C). The surface microstructure is fully wet by water, so the rough Al surface shows superhydrophilicity. The underwater wettability of such a superhydrophilic Al surface is investigated by immersing the sample in water. Oil droplets can maintain a ball-like shape on the sample and the oil CA (OCA) is measured to be 155.1° in a water medium (Figure 1D). Once the sample is tilted by 1.9°, the oil droplet can roll away freely, so the sliding angle (SA) is only 1.9°. The result reveals that the rough Al surface exhibits underwater superoleophobicity and very low adhesion to oil. Such an underwater superoleophobic surface has an excellent oil-repellent ability in a water medium. The behavior of the bubble on the superhydrophilic Al surface in water is similar to that of underwater oil droplets. Underwater superaerophobicity is exhibited by the structured Al surface. The bubble on the sample surface has a bubble CA (BCA) of 154° (Figure 1E) and SA of 0.5° in water. Therefore, a hierarchical rough Al substrate simultaneously has superhydrophilicity, underwater superoleophobicity, and underwater superaerophobicity.
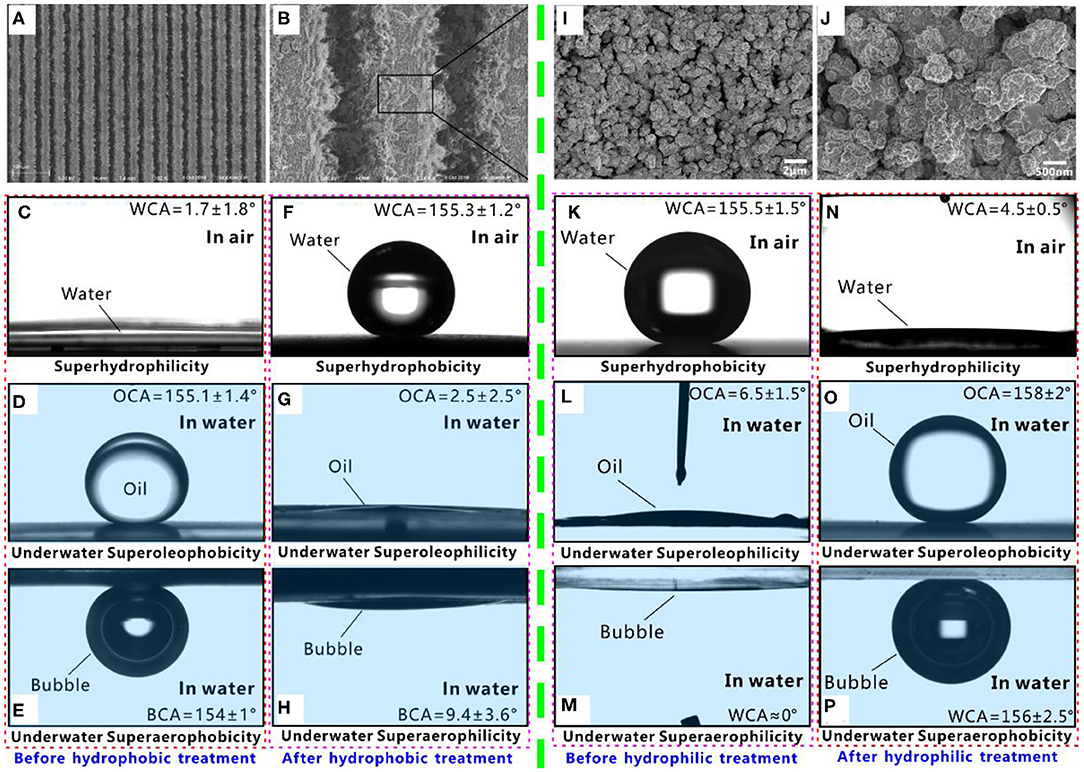
Figure 1. Different superwettabilities on the structured surface. (A,B) SEM images of the hydrophilic Al substrate with a hierarchical surface microstructure. (C,F) Water droplet on the structured Al surface in air. (D,G) Underwater oil droplet on the structured Al surface. (E,H) Underwater gas bubble on the structured Al surface. The samples in (C–E) are originally structured Al surface, while the samples in (F–H) are further treated by hydrophobic modification. (I,J) SEM images of the hydrophobic PDMS substrate with a hierarchical surface microstructure. (K,N) Water droplet on the structured PDMS surface in air. (L,O) Underwater oil droplet on the structured PDMS surface. (M,P) Underwater gas bubble on the structured Al surface. The samples in (K–M) are originally structured PDMS surface, while the samples in (N–P) are further treated by hydrophilic modification. Reproduced from Yong et al. (2019a) with the permission of Guo et al. Reproduced from Yong et al. (2017b) with the permission of Chen et al.
Fluoroalkylsilane modification is usually adopted to lower the surface energy of a material. The fluoroalkylsilane treatment switches the structured Al surface from a superhydrophilic state to a superhydrophobic state. The water droplet on the resultant surface has a WCA of 155.3° (Figure 1F) and can roll off easily with a SA of 6.3°. The fluoroalkylsilane-modified rough Al surface exhibits ultralow adhesive superhydrophobicity and excellent repellence to water. As the superhydrophobic surface is dipped into water, a mirror-like reflectance appears on the sample surface, because a layer of air persists the superhydrophobic surface and the water (Larmour et al., 2007; Zhao et al., 2010). When an oil droplet touches the microstructure of the superhydrophobic surface in water, it will spread out along the sample surface and wet the surface microstructure. The OCA is as low as 2.5° to this oil droplet, indicating that the surface shows superoleophilicity underwater (Figure 1G). Similar to the underwater oil wettability, if a small bubble is dispensed on the superhydrophobic Al surface in water, it will also spread out and like being absorbed by the surface, with the BCA of 9.4° (Figure 1H). The surface exhibits underwater superaerophilicity to bubbles. Therefore, the fluoroalkylsilane-modified structured Al surface simultaneously has superhydrophobicity, underwater superoleophilicity, and underwater superaerophilicity.
Different from the inherently hydrophilic Al substrate, the PDMS is a kind of intrinsic hydrophobic substrate. Figures 1I,J shows the SEM images of a structured PDMS surface (Yong et al., 2017b). The surface texture is also induced by laser microfabrication. The structured surface is coated with a large number of microscale coral-like structures with several micrometers in size. The surface of the microcorals is further decorated with rich nanoscale protrusions. The hierarchical rough microstructure endows the PDMS surface with excellent superhydrophobicity. The water droplet on the structured surface has a WCA of 155.5° (Figure 1K) and a SA of 2°. In a water medium, when an oil droplet or a bubble is released onto the superhydrophobic PDMS substrate, the oil droplet or a bubble can spread out immediately and be completely absorbed by the sample surface. The measured OCA and the BCA are only 6.5° (Figure 1L) and ~0° (Figure 1M), respectively. Therefore, the underwater superoleophilicity and superaerophilicity are also exhibited by the structured PDMS surface. The surface energy of the PDMS can be increased by short-time oxygen plasma irradiation (Wu et al., 2011; Cai et al., 2014). Oxygen plasma irradiation switches the structured PDMS surface from a superhydrophobic state to a superhydrophilic state. The water droplet can fully wet the surface with a WCA of 4.5° (Figure 1N). Such a superhydrophilic PDMS surface has great repellence to both oil droplets and gas bubbles in water. Underwater oil droplet and bubble have a spherical shape on such PDMS surface, with the OCA of 158° (Figure 1O) and BCA of 156° (Figure 1P), respectively. Both oil droplets and bubbles can easily roll away from a 3° tilted sample surface (SA = 3°). Therefore, the superhydrophilic PDMS surface also exhibits underwater superoleophobicity and superaerophobicity.
Relationship Between Different Superwettabilities
Different superwettabilities (e.g., superhydrophilicity, underwater superoleophilicity, underwater superaerophilicity, superhydrophobicity, underwater superoleophobicity, and underwater superaerophobicity) have been achieved by combing hierarchical microstructure and proper chemistry. The relationship between these different superwettabilities is summarized in Figure 2 (Yong et al., 2017b). The rough surface microstructure can amplify the natural wettability of a substrate (Yong et al., 2013a; Bellanger et al., 2014; Jiang et al., 2015; Wen et al., 2015; Su et al., 2016; Bai et al., 2020). The intrinsic hydrophilicity of a substrate can be enhanced to extreme state (i.e., superhydrophilicity) by surface microstructure; that is, the synergistic effect of the rough microstructure and high-surface-energy chemical composition produces a superhydrophilic surface (Figure 2A). Water droplets can completely wet the superhydrophilic surface microstructure at the Wenzel state in the air (Wang and Jiang, 2007; Yong et al., 2017c, 2018a). After the immersion in water, the superhydrophilicity allows the surface to be fully wet by water and the space of the microstructure to be filled with water (Figures 2B,E). The water likes being trapped by the surface microstructures, forming a tapped water cushion. As an oil droplet or a bubble is released on the superhydrophilic surfaces in water, the trapped water cushion filled in the interspaces of the surface microstructures will prevent the oil droplet/bubble from effectively touching the surface microstructure, because of the inherent repellence between water and oil droplet/bubble. The oil and bubble are only allowed to touch the peak part of the surface microstructure (Figures 2C,F). The underwater oil droplet and bubble just maintain near-spherical shapes to reach minimum free energy. Their shapes are not changed over time (Figures 2D,G). In such a three-phase (solid/water/oil or solid/water/gas) system, the underwater oil droplet (bubble) is at the underwater version of Cassie state on the structured surface (Wang and Jiang, 2007; Yong et al., 2017c, 2018a). As a result, the superhydrophilic microstructure presents superoleophobicity and superaerophobicity underwater.
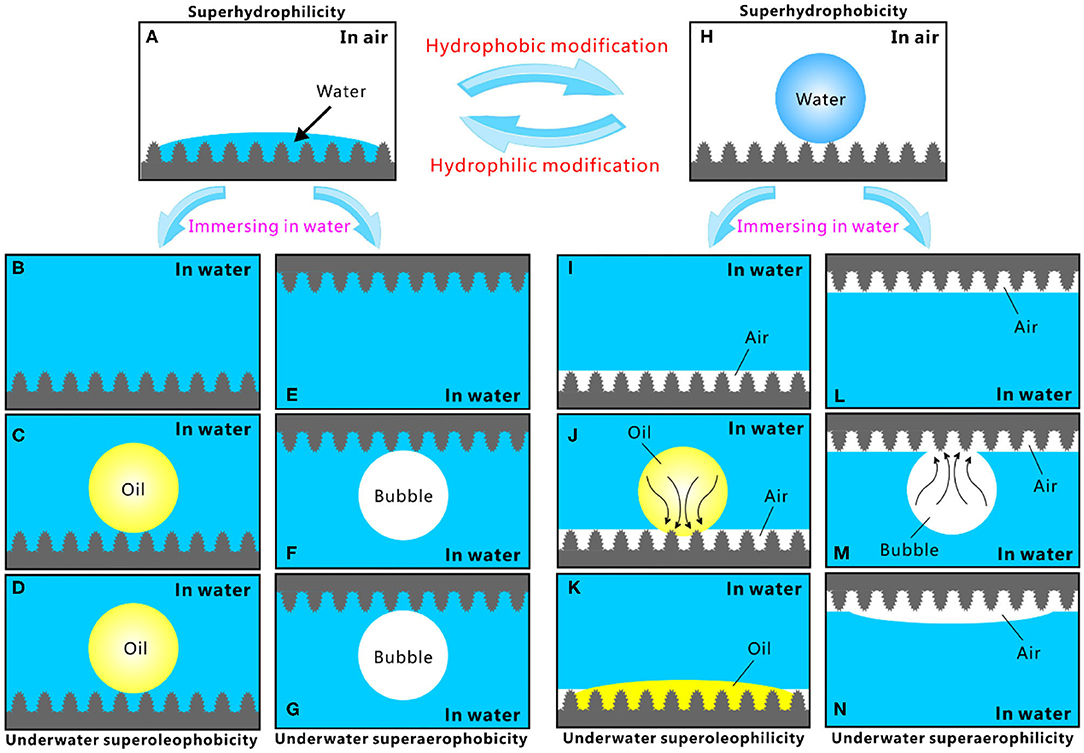
Figure 2. Relationship and interconversion of different superwettabilities. (A) Water droplet on the superhydrophilic microstructure. (B,E) Immersion of the superhydrophilic surface in water. (C) Placing an oil droplet on the superhydrophilic microstructure underwater. (D) Variation of the oil droplet in (C) over time. (F) Releasing a gas bubble on the superhydrophilic microstructure underwater. (G) Variation of the bubble in (F) over time. (H) Water droplet on the superhydrophobic microstructure. (I,L) Immersion of the superhydrophobic surface in water. (J) Placing an oil droplet on the superhydrophobic microstructure underwater. (K) Variation of the oil droplet in (J) over time. (M) Releasing a gas bubble onto the superhydrophobic microstructure underwater. (N) Variation of the bubble in (M) over time. Reproduced from Yong et al. (2017b) with the permission of Chen et al.
By lowering surface energy (such as fluoroalkylsilane modification), the superhydrophilic microstructure can be switched to a superhydrophobic state. The superhydrophobicity results from the synergistic action of the rough hierarchical microstructures and the low-surface-energy chemical composition. Water droplets cannot wet the superhydrophobic surface and can just touch the peaks of the surface microstructure (Figure 2H). The wetting between the water droplet and the superhydrophobic surfaces belongs to the Cassie state (Wang and Jiang, 2007; Yong et al., 2017c, 2018a). An air cushion trapped in the superhydrophobic microstructure forms underneath the water droplet. The trapped air layer will develop to a surrounding air layer on the sample surface once the superhydrophobic surface is dipped into water, no matter the superhydrophobic surface faces down or up (Figures 2I,L). In a water medium, if an oil droplet is dispensed onto the superhydrophobic surfaces, the capillary action and pressure will drive the oil to enter into the trapped air layer and immediately spread out along this air gap (Figure 2J). As the oil droplet fully spread out, a very small OCA value is obtained (Figure 2K). Therefore, the superhydrophobic surface reveals underwater superoleophilicity. The behavior of an underwater bubble is very similar to that of oil droplets. Once a small bubble touches the superhydrophobic surface underwater, the gas in the bubble will enter into the trapped air layer under pressure (Figure 2M) and finally merge with the air previously trapped on the surface microstructure (Figure 2N). The bubble likes being completely absorbed by the superhydrophobic surface, resulting in the underwater superaerophilicity of the sample surface.
It is demonstrated that the superhydrophobic surface usually exhibits both underwater superoleophilicity and superaerophilicity. By contrast, the superhydrophilic surface usually shows both underwater superoleophobicity and superaerophobicity. The superhydrophilicity and superhydrophobicity of a structured surface can be transformed from one state to another state by simple hydrophobic or hydrophilic modification. As a result, the reversible transformation between different wettabilities can be achieved. For example, the laser-structured PDMS surface shows superhydrophobicity, underwater superoleophilicity, and underwater superaerophilicity (Yong et al., 2017b, 2018c). Hydrophilic modification (e.g., oxygen plasma irradiation) changes the surface wettability to superhydrophilicity, underwater superoleophobicity, and underwater superaerophobicity. Interestingly, after hydrophobic modification (e.g., storage in the air), the original superhydrophobicity, underwater superoleophilicity, and underwater superaerophilicity can completely recover. Therefore, the same rough microstructure can have various superwettabilities. The superhydrophilicity, superoleophilicity, and superaerophilicity enable the materials to have the capacity of capturing, absorbing, and collecting water droplets, oil droplets, and gas bubbles. On the contrary, the superhydrophobicity, superoleophobicity, and superaerophobicity allow the materials to greatly repel water, oil, and bubble.
Conclusions
In conclusion, we discuss and clarify the relationship and the interconversion of superhydrophilicity, underwater superoleophilicity, underwater superaerophilicity, superhydrophobicity, underwater superoleophobicity, and underwater superaerophobicity. These different superwettabilities can be designed on a same structured surface by the combination of hierarchical surface microstructures and proper chemistry. It is revealed that the superhydrophobic surfaces usually exhibit both underwater superoleophilicity and superaerophilicity, whereas the superhydrophilic surfaces usually show underwater superoleophobicity and superaerophobicity. The superhydrophilicity and superhydrophobicity of a structured surface can be transformed from one state to another state by simple hydrophobic or hydrophilic modification. Therefore, various superwettabilities can be achieved on a same rough microstructure and reversibly convert from one state to other states. We believe that the relationship between different superwettabilities has great guiding significance for the design of superwetting materials and the applications of the artificial superwetting materials.
Author Contributions
FC directed and supervised the research project. JY wrote the manuscript. QY and XH contributed toward significant discussions and revised the paper. All authors contributed to the article and approved the submitted version.
Funding
This work was supported by the National Key Research and Development Program of China (2017YFB1104700), the National Science Foundation of China (61805192 and 61875158), the International Joint Research Laboratory for Micro/Nano Manufacturing and Measurement Technologies, and the Fundamental Research Funds for the Central Universities.
Conflict of Interest
The authors declare that the research was conducted in the absence of any commercial or financial relationships that could be construed as a potential conflict of interest.
References
Bai, X., Yang, Q., Fang, Y., Zhang, J., Yong, J. L., Hou, X., et al. (2020). Superhydrophobicity-memory surfaces prepared by a femtosecond laser. Chem. Eng. J. 383, 123143. doi: 10.1016/j.cej.2019.123143
Barthlott, W., and Neinhuis, C. (1997). Purity of the sacred lotus, or escape from contamination in biological surfaces. Planta 202, 1–8. doi: 10.1007/s004250050096
Bellanger, H., Darmanin, T., Givenchy, E. T., and Guittard, F. (2014). Chemical and physical pathways for the preparation of superoleophobic surfaces and related wetting theories. Chem. Rev. 114, 2694–2716. doi: 10.1021/cr400169m
Bian, H., Yong, J., Yang, Q., Hou, X., and Chen, F. (2020). Simple and low-cost oil/water separation based on the underwater superoleophobicity of the existing materials in our life or nature. Front. Chem. 8:507. doi: 10.3389/fchem.2020.00507
Cai, Y., Lin, L., Xue, Z., Liu, M., Wang, S., and Jiang, L. (2014). Filefish-inspired surface design for anisotropic underwater oleophobicity. Adv. Funct. Mater. 24, 809–816. doi: 10.1002/adfm.201302034
Chu, D., Singh, S. C., Yong, J., Zhan, Z., Sun, X., Duan, J., et al. (2019). Superamphiphobic surfaces with controllable adhesion fabricated by femtosecond laser bessel beam on PTFE. Adv. Mater. Interfaces 6:1900550. doi: 10.1002/admi.201900550
Gao, X., and Jiang, L. (2004). Water-repellent legs of water striders Nature 432:36. doi: 10.1038/432036a
Gao, X., Yan, X., Yao, X., Xu, L., Zhang, K., Zhang, J., et al. (2007). The dry-style antifogging properties of mosquito compound eyes and artificial analogues prepared by soft lithography. Adv. Mater. 19, 2213–2217. doi: 10.1002/adma.200601946
Genzer, J., and Efimenko, K. (2006). Recent developments in superhydrophobic surfaces and their relevance to marine fouling: a review. Biofouling 22, 339–360. doi: 10.1080/08927010600980223
Jiang, T., Guo, Z., and Liu, W. (2015). Biomimetic superoleophobic surfaces: focusing on their fabrication and applications. J. Mater. Chem. A 3, 1811–1827. doi: 10.1039/C4TA05582A
Jokinen, V., Sainiemi, L., and Franssila, S. (2008). Complex droplets on chemically modified silicon nanograss. Adv. Mater. 20, 3453–3456. doi: 10.1002/adma.200800160
Ju, J., Bai, H., Zheng, Y., Zhao, T., Fang, R., and Jiang, L. (2012). A multi-structural and multi-functional integrated fog collection system in cactus. Nat. Commun. 3:1247. doi: 10.1038/ncomms2253
Kreder, M. J., Alvarenga, J., Kim, P., and Aizenberg, J. (2016). Design of anti-icing surfaces: smooth, textured or slippery? Nat. Rev. Mater. 1:15003. doi: 10.1038/natrevmats.2015.3
Kwon, K. W., Choi, S. S., Lee, S. H., Kim, B., Lee, S. N., Park, M. C., et al. (2007). Label-free, microfluidic separation and enrichment of human breast cancer cells by adhesion difference. Lab Chip 7, 1461–1468. doi: 10.1039/b710054j
Larmour, I. A., Bell, S. E. J., and Saunders, G. C. (2007). Remarkably simple fabrication of superhydrophobic surfaces using electroless galvanic deposition. Angew. Chem. 119, 1740–1742. doi: 10.1002/ange.200604596
Liu, M., Wang, S., and Jiang, L. (2017). Nature-inspired superwettability systems. Nat. Rev. Mater. 2, 17036. doi: 10.1038/natrevmats.2017.36
Liu, M., Wang, S., Wei, Z., Song, Y., and Jiang, L. (2009). Bioinspired design of a superoleophobic and low adhesive water/solid interface. Adv. Mater. 21, 665–669. doi: 10.1002/adma.200801782
Lv, J., Song, Y., Jiang, L., and Wang, J. (2014). Bio-Inspired Strategies for Anti-Icing. ACS Nano 8, 3152–3169. doi: 10.1021/nn406522n
Nishimoto, S., and Bhushan, B. (2013). Bioinspired self-cleaning surfaces with superhydrophobicity, superoleophobicity, and superhydrophilicity. RSC Adv. 3, 671–690. doi: 10.1039/C2RA21260A
Pan, S., Kota, A. K., Mabry, J. M., and Tuteja, A. (2013). Superomniphobic surfaces for effective chemical shielding. J. Am. Chem. Soc. 135, 578–581. doi: 10.1021/ja310517s
Parker, R., and Lawrence, C. R. (2001). Water capture by a desert beetle. Nature 414, 33–34. doi: 10.1038/35102108
Ragesh, P., Ganesh, V. A., Nair, S. V., and Nair, A. S. (2014). A review on “self-cleaning and multifunctional materials”. J. Mater. Chem. A 2, 14773–14797. doi: 10.1039/C4TA02542C
Shen, L., Wang, B., Wang, J., Fu, J., Picart, C., and Ji, J. (2012). Asymmetric free-standing film with multifunctional anti-bacterial and self-cleaning properties. ACS Appl. Mater. Interfaces 4, 4476–4483. doi: 10.1021/am301118f
Shi, F., Niu, J., Liu, J., Liu, F., Wang, Z., Feng, X., et al. (2007). Towards understanding why a superhydrophobic coating is needed by water striders. Adv. Mater. 19, 2257–2261. doi: 10.1002/adma.200700752
Songok, J., Tuominen, M., Teisala, H., Haapanen, J., Mäkel,ä, J., Kuusipalo, J., et al. (2014). Paper-based microfluidics: fabrication technique and dynamics of capillary-driven surface flow. ACS Appl. Mater. Interfaces 6, 20060–20066. doi: 10.1021/am5055806
Stratakis, E., Ranella, A., and Fotakis, C. (2011). Biomimetic micro/nanostructured functional surfaces for microfluidic and tissue engineering applications. Biomicrofluidics 5:013411. doi: 10.1063/1.3553235
Su, B., Tian, Y., and Jiang, L. (2016). Bioinspired interfaces with superwettability: from materials to chemistry. J. Am. Chem. Soc. 138, 1727–1748. doi: 10.1021/jacs.5b12728
Teisala, H., Tuominen, M., and Kuusipalo, J. (2014). Superhydrophobic coatings on cellulose-based materials: fabrication, properties, and applications. Adv. Mater. Interfaces 1:1300026. doi: 10.1002/admi.201300026
Tian, Y., Su, B., and Jiang, L. (2014). Interfacial material system exhibiting superwettability. Adv. Mater. 26, 6872–6897. doi: 10.1002/adma.201400883
Vitale, A., Quaglio, M., Marasso, S. L., Chiodoni, A., Cocuzza, M., and Bongiovanni, R. (2013). Direct photolithography of perfluoropolyethers for solvent-resistant microfluidics. Langmuir 29, 15711–15718. doi: 10.1021/la402755q
Wang, B., Liang, W., Guo, Z., and Liu, W. (2015). Biomimetic super-lyophobic and super-lyophilic materials applied for oil/water separation: a new strategy beyond nature. Chem. Soc. Rev. 44, 336–361. doi: 10.1039/C4CS00220B
Wang, J. N., Zhang, Y. L., Liu, Y., Zheng, W., Lee, L. P., and Sun, H. B. (2015). Recent developments in superhydrophobic graphene and graphene-related materials: from preparation to potential applications. Nanoscale 7, 7101–7114. doi: 10.1039/C5NR00719D
Wang, M., Chen, C., Ma, J., and Xu, J. (2011). Preparation of superhydrophobic cauliflower-like silica nanospheres with tunable water adhesion. J. Mater. Chem. 21, 6962–6967. doi: 10.1039/c1jm10283d
Wang, S., and Jiang, L. (2007). Definition of superhydrophobic states. Adv. Mater. 19, 3423–3424. doi: 10.1002/adma.200700934
Wang, S., Wang, T., Ge, P., Xue, P., Ye, S., Chen, H., et al. (2015). Controlling flow behavior of water in microfluidics with a chemically patterned anisotropic wetting surface. Langmuir 31, 4032–4039. doi: 10.1021/acs.langmuir.5b00328
Wen, L., Tian, Y., and Jiang, L. (2015). Bioinspired super-wettability from fundamental research to practical applications. Angew. Chem. Int. Ed. 54, 3387–3399. doi: 10.1002/anie.201409911
Wu, D., Wu, S., Chen, Q.-D., Zhao, S., Zhang, H., Jiao, J., et al. (2011). Facile creation of hierarchical PDMS microstructures with extreme underwater superoleophobicity for anti-oil application in microfluidic channels. Lab Chip 11, 3873–3879. doi: 10.1039/c1lc20226j
Xue, Z., Cao, Y., Liu, N., Feng, L., and Jiang, L. (2014). Special wettable materials for oil/water separation. J. Mater. Chem. A 2, 2445–2460. doi: 10.1039/C3TA13397D
Yong, J., Chen, F., Fang, Y., Huo, J., Yang, Q., Zhang, J., et al. (2017a). Bioinspired design of underwater superaerophobic and superaerophilic surfaces by femtosecond laser ablation for anti- or capturing bubbles. ACS Appl. Mater. Interfaces 9, 39863–39871. doi: 10.1021/acsami.7b14819
Yong, J., Chen, F., Huo, J., Fang, Y., Yang, Q., Bian, H., et al. (2018b). Biodegradable, underwater superoleophobic wood sheet for efficient oil/water separation. ACS Omega 3, 1395–1402. doi: 10.1021/acsomega.7b02064
Yong, J., Chen, F., Huo, J., Fang, Y., Yang, Q., Zhang, J., et al. (2018c). Femtosecond laser induced underwater superaerophilic and superaerophobic PDMS sheet with through-microholes for air bubbles selectively passing through and further collecting underwater gas. Nanoscale 10, 3688–3696. doi: 10.1039/C7NR06920K
Yong, J., Chen, F., Li, M., Yang, Q., Fang, Y., Huo, J., et al. (2017b). Remarkably simple achievement of superhydrophobicity, superhydrophilicity, underwater superoleophobicity, underwater superoleophilicity, underwater superaerophobicity, and underwater superaerophilicity on femtosecond laser ablated PDMS surfaces. J. Mater. Chem. A 5, 25249–25257. doi: 10.1039/C7TA07528F
Yong, J., Chen, F., Li, W., Huo, J., Fang, Y., Yang, Q., et al. (2018d). Underwater superaerophobic and superaerophilic nanoneedles-structured meshes for water/bubbles separation: removing or collecting gas bubbles in water. Global Challenges 2:1700133. doi: 10.1002/gch2.201700133
Yong, J., Chen, F., Yang, Q., Bian, H., Du, G., Shan, C., et al. (2016a). Oil-water separation: a gift from the desert. Adv. Mater. Interfaces 3:1500650. doi: 10.1002/admi.201500650
Yong, J., Chen, F., Yang, Q., Farooq, U., and Hou, X. (2015a). Photoinduced switchable underwater superoleophobicity-superoleophilicity on laser modified titanium surfaces. J. Mater. Chem. A 3, 10703–10709. doi: 10.1039/C5TA01782C
Yong, J., Chen, F., Yang, Q., Huo, J., and Hou, X. (2017c). Superoleophobic surfaces. Chem. Soc. Rev. 46, 4168–4217. doi: 10.1039/C6CS00751A
Yong, J., Chen, F., Yang, Q., Jiang, Z., and Hou, X. (2018a). A review of femtosecond-laser-induced underwater superoleophobic surfaces. Adv. Mater. Interfaces 5:1701370. doi: 10.1002/admi.201701370
Yong, J., Chen, F., Yang, Q., Zhang, D., Bian, H., Du, G., et al. (2013a). Controllable adhesive superhydrophobic surfaces based on PDMS microwell arrays. Langmuir 29, 3274–3279. doi: 10.1021/la304492c
Yong, J., Chen, F., Yang, Q., Zhang, D., Du, G., Si, J., et al. (2013b). Femtosecond laser weaving superhydrophobic patterned PDMS surfaces with tunable adhesion. J. Phys. Chem. C 117, 24907–24912. doi: 10.1021/jp408863u
Yong, J., Chen, F., Yang, Q., Zhang, D. S., Farooq, U., Du, G. Q., et al. (2014a). Bioinspired underwater superoleophobic surface with ultralow oil-adhesion achieved by femtosecond laser microfabrication. J. Mater. Chem. A 2, 8790–8795. doi: 10.1039/C4TA01277A
Yong, J., Fang, Y., Chen, F., Huo, J., Yang, Q., Bian, H., et al. (2016b). Femtosecond laser ablated durable superhydrophobic PTFE films with micro-through-holes for oil/water separation: separating oil from water and corrosive solutions. Appl. Surf. Sci. 389, 1148–1155. doi: 10.1016/j.apsusc.2016.07.075
Yong, J., Huo, J., Chen, F., Yang, Q., and Hou, X. (2018e). Oil/water separation based on natural materials with super-wettability: recent advances. Phys. Chem. Chem. Phys. 20, 25140–25163. doi: 10.1039/C8CP04009E
Yong, J., Huo, J., Yang, Q., Chen, F., Fang, Y., Wu, X., et al. (2018f). Femtosecond laser direct writing of porous network microstructures for fabricating super-slippery surfaces with excellent liquid repellence and anti-cell proliferation. Adv. Mater. Interfaces 5:1701479. doi: 10.1002/admi.201701479
Yong, J., Singh, S. C., Zhan, Z., Chen, F., and Guo, C. (2019a). How to obtain six different superwettabilities on a same microstructured pattern: relationship between various superwettabilities in different solid/liquid/gas systems. Langmuir 35, 921–927. doi: 10.1021/acs.langmuir.8b03726
Yong, J., Singh, S. C., Zhan, Z., Mohamed, E., Chen, F., and Guo, C. (2019b). Femtosecond laser-produced underwater “superpolymphobic” nanorippled surfaces: repelling liquid polymers in water for application of controlling polymer shape and adhesion. ACS Appl. Nano Mater. 2, 7362–7371. doi: 10.1021/acsanm.9b01869
Yong, J., Yang, Q., Chen, F., Bian, H., Du, G. Q., Farooq, U., et al. (2015b). Reversible underwater lossless oil droplet transportation. Adv. Mater. Interfaces 2:1400388. doi: 10.1002/admi.201400388
Yong, J., Yang, Q., Chen, F., Du, G., Shan, C., Farooq, U., et al. (2015c). Using “underwater superoleophobic pattern” to make liquid lens array. RSC Adv. 5, 40907–40911. doi: 10.1039/C5RA04671H
Yong, J., Yang, Q., Chen, F., Zhang, D., Du, G., Si, J., et al. (2014c). A bioinspired planar superhydrophobic microboat. J. Micromech. Microeng. 24:035006. doi: 10.1088/0960-1317/24/3/035006
Yong, J., Yang, Q., Chen, F., Zhang, D. S., Bian, H., Ou, Y., et al. (2013c). Stable superhydrophobic surface with hierarchical mesh-porous structure fabricated by a femtosecond laser. Appl. Phys. A 111, 243–249. doi: 10.1007/s00339-013-7572-z
Yong, J., Yang, Q., Chen, F., Zhang, D. S., Farooq, U., Du, G. Q., et al. (2014b). A simple way to achieve superhydrophobicity, controllable water adhesion, anisotropic sliding, and anisotropic wetting based on femtosecond-laser-induced line-patterned surfaces. J. Mater. Chem. A 2, 5499–5507. doi: 10.1039/C3TA14711H
Yong, J., Yang, Q., Guo, C., Chen, F., and Hou, X. (2019c). A review of femtosecond laser-structured superhydrophobic or underwater superoleophobic porous surfaces/materials for efficient oil/water separation. RSC Adv. 9, 12470–12495. doi: 10.1039/C8RA10673H
Zhan, Z., EIKabbash, M., Cheng, J., Zhang, J., Singh, S., and Guo, C. (2019). Highly floatable superhydrophobic metallic assembly for aquatic applications. ACS Appl. Mater. Interfaces 11, 48512–48517. doi: 10.1021/acsami.9b15540
Zhan, Z., Li, Z., Yu, Z., Singh, S., and Guo, C. (2018). Superhydrophobic Al surfaces with properties of anticorrosion and reparability. ACS Omega 3, 17425–17429. doi: 10.1021/acsomega.8b02631
Zhang, S., Huang, J., Chen, Z., and Lai, Y. (2017). Bioinspired special wettability surfaces: from fundamental research to water harvesting applications. Small 13:1602992. doi: 10.1002/smll.201602992
Zhao, Y., Tang, Y., Wang, X., and Lin, T. (2010). Superhydrophobic cotton fabric fabricated by electrostatic assembly of silica nanoparticles and its remarkable buoyancy. Appl. Surf. Sci. 256, 6736–6742. doi: 10.1016/j.apsusc.2010.04.082
Zheng, Y., Bai, H., Huang, Z., Tian, X., Nie, F. Q., Zhao, Y., et al. (2010). Directional water collection on wetted spider silk. Nature 463, 640–643. doi: 10.1038/nature08729
Keywords: superhydrophilicity, superhydrophobicity, underwater superoleophilicity, underwater superoleophobicity, underwater superaerophilicity, underwater superaerophobicity
Citation: Yong J, Yang Q, Hou X and Chen F (2020) Relationship and Interconversion Between Superhydrophilicity, Underwater Superoleophilicity, Underwater Superaerophilicity, Superhydrophobicity, Underwater Superoleophobicity, and Underwater Superaerophobicity: A Mini-Review. Front. Chem. 8:828. doi: 10.3389/fchem.2020.00828
Received: 02 June 2020; Accepted: 05 August 2020;
Published: 10 September 2020.
Edited by:
Doo Soo Chung, Seoul National University, South KoreaReviewed by:
Bharat Bhushan, Indian Agricultural Research Institute (ICAR), IndiaTae Joo Shin, Ulsan National Institute of Science and Technology, South Korea
Copyright © 2020 Yong, Yang, Hou and Chen. This is an open-access article distributed under the terms of the Creative Commons Attribution License (CC BY). The use, distribution or reproduction in other forums is permitted, provided the original author(s) and the copyright owner(s) are credited and that the original publication in this journal is cited, in accordance with accepted academic practice. No use, distribution or reproduction is permitted which does not comply with these terms.
*Correspondence: Feng Chen, chenfeng@mail.xjtu.edu.cn