Devising Mixed-Ligand Based Robust Cd(II)-Framework From Bi-Functional Ligand for Fast Responsive Luminescent Detection of Fe3+ and Cr(VI) Oxo-Anions in Water With High Selectivity and Recyclability
- 1Inorganic Materials and Catalysis Division, CSIR-Central Salt and Marine Chemicals Research Institute (CSMCRI), Bhavnagar, India
- 2Academy of Scientific and Innovative Research (AcSIR), Ghaziabad, India
Environmental issue related applications have globally surfaced as hottest areas of research, wherein luminescent metal-organic frameworks (LMOFs) with functionalized pores put unique signature in real-time monitoring of multiple classes of toxic compounds, and overcome many of the challenges of conventional materials. We report a two-fold interpenetrated, mixed-ligand Cd(II)-organic framework (CSMCRI-11) [Cd1.5(L)2(bpy)(NO3)]·DMF·2H2O (CSMCRI = Central Salt and Marine Chemical Research Institute, HL = 4- (1H-imidazol-1-yl)benzoic acid, bpy = 4,4′-bipyridine) that exemplifies bipillar-layer structure with two different Cd(II) nodes, and displays notable robustness in diverse organic solvents and water. Intense luminescence signature of the activated MOF (11a) is harnessed in extremely selective and fast responsive sensing of Fe3+ ions in aqueous phase with notable quenching constant (1.91 × 104 M−1) and impressive 166 ppb limit of detection (LOD). The framework further serves as a highly discriminative and quick responsive scaffold for turn-off detection of two noxious oxo-anions (Cr2O72− and CrO42−) in water, where individual quenching constants (CrO42−: 1.46 × 104 M−1; Cr2O72−: 2.18 × 104 M−1) and LOD values (CrO42−: 179 ppb; Cr2O72−: 114 ppb) rank among best sensory MOFs for aqueous phase detection of Cr(VI) species. It is imperative to stress the outstanding reusability of the MOF towards detection of all these aqueous pollutants, besides their vivid monitoring by colorimetric changes under UV-light. Mechanism of selective quenching is comprehensively investigated in light of absorption of the excitation/emission energy of the host framework by individual studied analyte.
Introduction
Severe pollution of earth’s hydrosphere by detrimental chemicals has raised global concerns to human health and ecological systems. (Samanta et al., 2019; Yao et al., 2020) For instance, Fe3+ ion is one of the vital ions existing in the living organism and plays essential role in transportation of oxygen molecule through blood. It also has a fundamental role in the formation of haemoglobin, enzyme and proteins. (Xu et al., 2016) Hence, Fe3+ ion is firmly an indicator of health. Both inadequacy and abundance in blood plasma can lead to biological/genetic malfunction. Syndrome like insomnia, cancer, heart and declining immunity is correlated with iron content in the human body. (Dang et al., 2012; Liu et al., 2016; Chandra Rao and Mandal, 2018) Also, in the physiological milieu, iron exists as Fe2+ and Fe3+ are essentially playing a significant role So, it is imperative to detect one from the other because a specific amount of Fe3+ is needed to promote the formation of muscle and haemoglobin. (Barba-Bon et al., 2012; Gogoi and Biswas, 2018) Though a wide range of literature is available for sensing of Fe3+ ions but sensing under aqueous condition is still rare. (Xu et al., 2016; Yang et al., 2019) In this direction, the readiness of new sensors for Fe(III) ion detection is as yet a challenging objective. (Lustig et al., 2017; Sun et al., 2019) Alongside, chromium is also essential for mankind. Cr (III) is an essential biological element while Cr(VI) ion as CrO42− and Cr2O72− oxo-anions is employed as an oxidising agent in manufacturing processes (Lv et al., 2016). Owing to exhaustive use in industrial processes, these oxo-anions are utterly polluting the environment and water bodies as a result of their outstanding solubility in water. These two oxo-anions are both cytotoxic and carcinogenic, and leads to the disruption of various proteins, enzymes and DNAs in the animal body. (Dong et al., 2016; Chen et al., 2017a; Dong et al., 2017; Sun et al., 2019) Thus, it is of vast significance to monitor minute presence of Fe3+ and/or Cr(VI) ions in aqueous media via highly sensitive, fast-responsive and easily portable method. (Xu and Yan, 2015; Li et al., 2019; Singh et al., 2020)
As of now, conventional procedures include high-performance liquid chromatography (HPLC), ion-exchange chromatography, gas chromatography (GC), gas chromatography-mass Spectrometry (GC/MS), ion-exchange chromatography and other detection techniques. Those techniques are time-consuming, quite expensive and require complicated instruments. On the other hand, luminescence-based sensory materials have shown several advantages, for instance, fast response time, ease of performance, distinct signal outputs, low cost, high sensitivity, and low detection limit. (El Rassi, 1997; Zhu et al., 2013; Wang et al., 2015; Senthilkumar et al., 2018; Guo et al., 2019; Yao et al., 2020) Although, several homogenous sensors have been developed, (Ma et al., 2019), quest of heterogeneous sensor is indispensable owing to their advantages like separation, eco-friendly nature and multicyclic regeneration properties. (Liu et al., 2016; Vikrant et al., 2018; Zhao et al., 2018; Yan, 2019) In this milieu, metal-organic frameworks (MOFs) have grown as most fascinating porous, heterogonous scaffolds with well defined-crystalline materials, and recently used as probes for luminescent sensing of diverse lethal pollutants. (Kitagawa et al., 2004; Chen et al., 2007; Cohen, 2010; Cui et al., 2011; Kreno et al., 2012; Zhou et al., 2012; Pettinari et al., 2017; Zhang et al., 2017) In relation to the aforesaid concerns, coupled with our ongoing efforts to develop new sensory LMOFs for minute detection of diverse lethal pollutants, (Goswami et al., 2019a; Goswami et al., 2019b; Singh et al., 2020; Seal et al., 2021a; Seal et al., 2021b) we have synthesised a water stable, Cd(II)-MOF CSMCRI-11 using the bifunctional ligand 4- (1H-imidazol-1-yl) benzoic acid (HL, Supplementary Scheme S1) in combination with pillaring linker bpy (Scheme 1). CSMCRI-11 exhibits two-fold interpenetrated structure with unidirectional pores. This framework shows highly selective and sensitive detection of Fe3+ ion and noxious Cr(VI) oxo-anions (CrO42− and Cr2O72−) at ppb level in the aqueous phase. Fast responsive time and excellent sensitivity along with very low limit of detection render this framework one of the best LMOFs among contemporary reports, and promises its utilization as smart material for detection of hazardous contaminants in the aqueous phase.
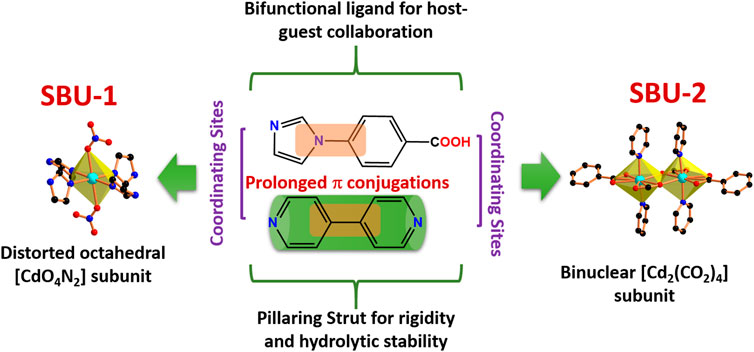
SCHEME 1. Notable Features of the HL (bifunctional ligand), bpy (pillaring strut) and Cd2+ Nodes/Units in CSMCRI-11.
Results and Discussion
Crystal Structure of CSMCRI-11
Framework CSMCRI-11 was synthesized as colourless rectangular crystals under solvothermal conditions by reaction of bifunctional ligand 4- (1H-imidazol-1-yl) benzoic acid (HL), 4,4′-bipyridine (bpy) and Cd(NO3)2·4H2O in the molar ratio of 0.2:0.2:0.16. As-synthesized CSMCRI-11 crystal was examined by single-crystal X-ray diffraction (Supplementary Table S1) which displays monoclinic space group C2/c. The asymmetric unit contains two Cd(II) ion, one bpy linker, two deprotonated ligand (L−1: hereafter L) and one nitrate anion. Both the metal centres exhibit different coordination environments. For instance, Cd(1) is coordinated with four oxygen atoms from the carboxylate group of L and two nitrogen atoms (N atoms N3 and N5) from the pyridyl ring of bpy linker in distorted octahedral geometry (CdO4N2). The Cd-O bond length range from 2.292 to 2.419 Å while Cd-N bond length ranges from 2.285 to 2.297 Å. (Zhai et al., 2019).
Two alike Cd (1) ions are bridged through two carboxylate groups in syn-anti fashion to form a binuclear [Cd2(CO2)4] subunit (Scheme 1) with Cd(1)-Cd(1) spacing of 3.986 Å. On the other hand, Cd (2) atom is in entirely different coordination milieu, and reveals distorted octahedral geometry (CdO4N2) via ligation with four imidazolyl nitrogen atoms of L in the equatorial plane, and two oxygen atoms of nitrate anions. Here, Cd-O bond length is 2.358 Å, and Cd-N bond length varies from 2.296 to 2.318 Å. Every deprotonated ligand is in coordination with [Cd2(CO2)4] subunits and Cd (2) ions forming two-dimensional (2D) layer along the crystallographic b axis.
The weavy layer acts as a roof with rhombus window (Figure 1A) of size 16.4 × 18.8 Å2 (considering atom to atom connection). Two bpy linkers connects every [Cd2(CO2)4] units, leading to a double-pillar layered three-dimensional (3D) structure with the formation of large voids (dimension: 11.4 × 24.5 Å2) along the c axis. Such huge pores mutually instigate two-fold interpenetration (Figure 1D) to the overall structure of CSMCRI-11. Nevertheless, rectangular cavities of dimension 6.54 × 18.09 Å2 still exists along ab plane. Also, pillaring bpy linkers are accompanied by string π–π interaction (centroid–centroid distances in the range: 3.794 Å), imparting high rigidity to the 3D porous network.
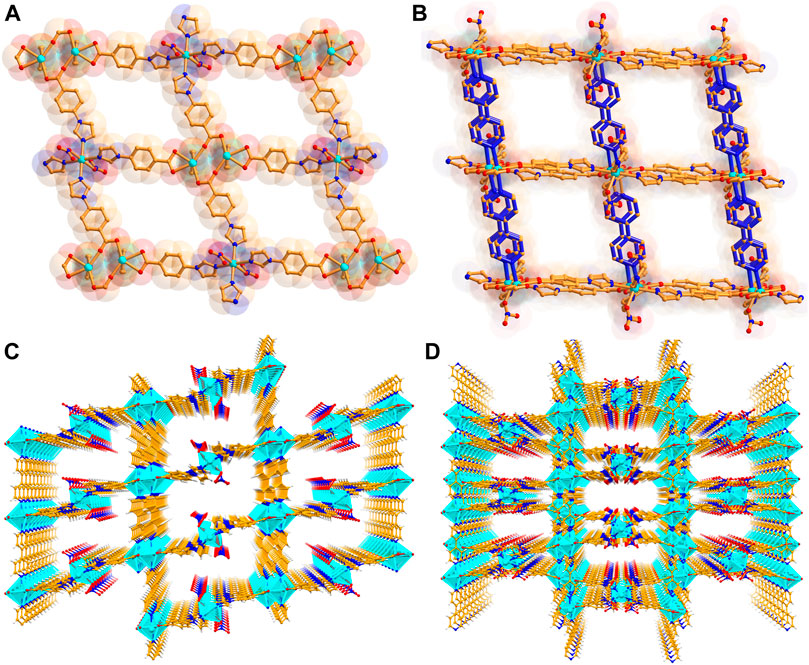
FIGURE 1. (A) A view of the 2D layer structure along ac plane, and (B) simplified demonstration of bipillar-layer structure, (C) tilted view of the one-dimensional porous channel in non-interpenetrated and (D) interpenetrated structure along c axis.
These porous channels accommodate DMF and H2O solvent molecules, which are highly disordered. However, their presences were alternatively ascribed from a combination of PLATON calculation, thermogravimetric weight loss, elemental analysis, and IR spectral data that corresponds to the molecular formula of CSMCRI-11 [Cd1.5(L)2(bpy)(NO3)]·DMF·2H2O. Regardless of two-fold interpenetration, the solvent accessible voids of CSMCRI-11 is estimated to be 31.6% of the total crystal volume.
Assessment of Thermal and Moisture Stability of CSMCRI-11
The purity of bulk phase and structural integrity of CSMCRI-11 was established from strong correlations of peaks between powder X-ray diffraction (PXRD) pattern of as synthesised framework and simulated one (Figure 2A), obtained from crystallographic data. The FT-IR spectral data exhibits IR band of the >C═O (carbonyl) for guest DMF solvent at 1663 cm−1 (Supplementary Figure S4). Also, a broad absorption peak centred around 3300 cm−1 corresponds to lattice water molecules. Thermal stability of the framework was assessed from thermogravimetric analysis (TGA) under inert atmosphere (Supplementary Figure S5), showing a two-step weight-loss. The first weight loss was observed in the temperature range 30–100 oC and corresponded to 3.89% of the initial mass, accounting for the removal of lattice water. The second weight loss of 8.89% features loss of DMF solvents. A sharp weight loss beyond 350 oC relates to framework decomposition. For, generation of the guest-free (activated) framework (hereafter 11a), the as-synthesised crystals of CSMCRI-11 were immersed in methanol for three days (by exchanging the solvent three times a day), and solvent exchanged framework was heated under vacuum at 120 °C for overnight. The FT-IR spectra analysis of 11a shows absence of all the peaks related to H2O or DMF solvents. Furthermore, the TGA curve of 11a did not reveal any weight loss up to 320 °C, supporting its robust nature at high temperature (Supplementary Figure S5). To further corroborate stability of the structure, 50 mg of as-made CSMCRI-11 was taken in a vial and exposed to common organic solvents (methanol, acetonitrile, dichloromethane, tetrahydrofuran, acetone), and water. Importantly, PXRD patterns of these exposed samples remained unchanged (Figure 2B). We further investigated the hydrolytic stability of the framework by exposing it to saturated water vapours at room temperature. A time-dependent PXRD (Supplementary Figure S6) study up to 10 days was carried out at a regular interval, which revealed maintenance of structural integrity throughout, and substantiates to sufficient hydrolytic stability of the MOF. The stability aspect was further assessed by leaving 11a in the open air for 15 days. Remarkably, PXRD pattern (Figure 2B) remained unchanged and corroborates to its high robustness.
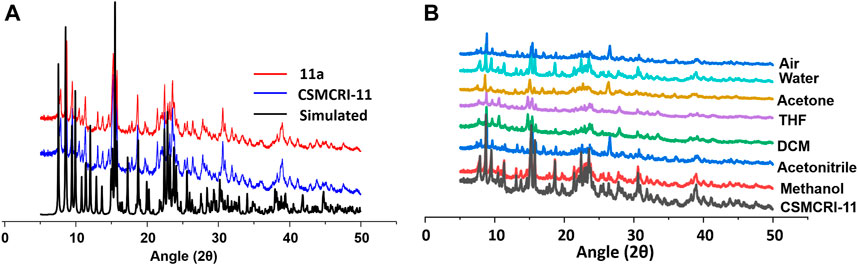
FIGURE 2. (A) PXRD pattern of CSMCRI-11 calculated, synthesised and activated framework. (B) PXRD curves of the pristine framework CSMCRI-11 in diverse solvents and air.
Photoluminescence Studies
Strategic incorporation of electronically passive transition metal in association with conjugated organic struts is one of the potential strategies for the synthesis of luminescent MOFs. (Zhu et al., 2015; Zhang et al., 2018; Zhou et al., 2018) Owing to the excellent thermal and hydrolytic stability, together with its highest luminescence intensity in water (Supplementary Figure S7c), CSMCRI-11 was employed as a fluorescent probe in activated form for aqueous phase minute detection of water contaminants. Upon excitation at 265 nm, aqueous dispersion of 11a showed strong emission band at 426 nm that is attributed to mixed contribution of the intra-ligand charge transfer of π-electron-rich links, (Chen et al., 2007) ligand-to-ligand charge transfer (LLCT) (π−π* and n−π* transitions) (Luo et al., 2012; Wan et al., 2015) and adequate π−π stacking interaction between the ligands in the structure. (Zhao et al., 2016; Liu et al., 2019) Additional experiment showed that after filtration of MOF particles, no fluorescence (Supplementary Figure S7d) could be observed in the remaining solution supporting the fact that above emission originates as a result of dispersed MOF particles only. UV-visible spectrum (Supplementary Figure S7a) of 11a shows a redshift of 9 and 3 nm in comparison to bpy and HL, respectively that are associated with binding of linkers to the Cd(II) metal ions. Remarkably, the intensity of the photoluminescent (PL) spectrum of 11a was found to be > 90% higher than constituting ligands. Coordination interactions through Cd(II) centres enhance the rigidity of the structure and boosts the PL intensity to such extent.
Luminescent Sensing of Fe3+ Ions in ppb Level
Owing to high photoluminescence intensity, 11a has been employed as a probe for selective and sensitive detection of metal ions in the aqueous phase. 1 mg of powdered 11a was dispersed in 2 ml water and sonicated for 2 h to generate a uniform suspension (1 mg/2 ml dispersion of 11a for each titration) Photoluminescence spectra of the aqueous phase dispersion was recorded by incremental addition of a series of MCly metal salts (M: Ba2+, Fe3+, Fe2+, K+, Mn2+, Ca2+, Cd2+, Pd2+, Co2+, Al3+, Ni2+, Cu2+, Cr3+, Mg2+, and La3+) in water (2.5 mM). For all these cases, the luminescence spectra were recorded at an emission wavelength of 426 nm under constant stirring at room temperature upon incremental addition of analyte (20–120 µl), and every titration was performed in triplicates to ensure the reproducibility of the results. Surprisingly, a rapid and significant decrease in the luminescence intensity of 11a was detected (Figure 3A) only in case of Fe3+ ion solution, while remaining metal ions did not show any significant changes. The quenching efficiency of Fe3+ ion is found to be 91.6% (Supplementary Figure S8), whilst the rest of the metal ions showed almost nominal values. The quenching competence follows the trend (Supplementary Figure S8): Fe3+>Cr3+>Cu2+>Ni2+>Fe2+>La3+>Cd2+>Mg2+>Al3+>Mn2+>Ba2+>Ca2+>Co2+>Pd2+>K+.
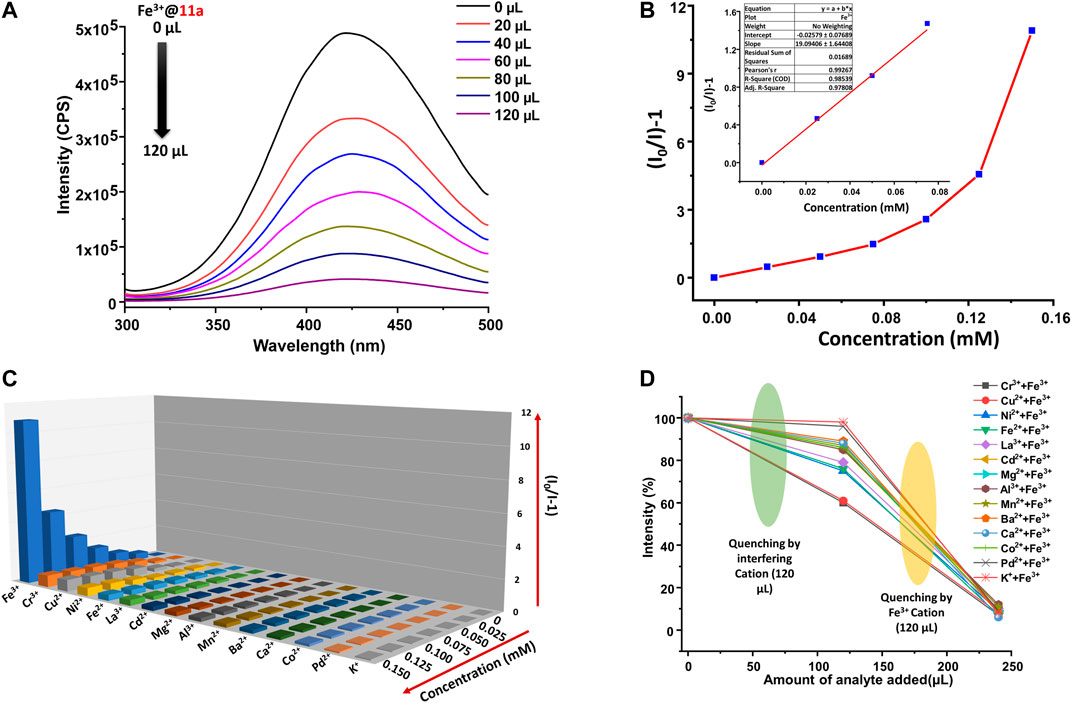
FIGURE 3. (A) Photoluminescence spectra of 11a upon gradual addition of aqueous solution Fe3+ ions (2.5 mM), (B) Stern-Volmer plot for 11a upon incremental addition of Fe3+ ions (Inset: linear region of the plot), (C) S-V plots for all studied metal ions (2.5 mM) upon titration with well-dispersed suspension of 11a (λmax of emission 11a ca. 426 nm; 5 nm slit width), (D) Interference plot presenting the decrease in PL intensities upon addition of aqueous solution of several metal ions (2.5 mM, 120 μl), followed by Fe3+ ions (2.5 mM, 120 μl).
Quantitative analysis of the fluorescence quenching of 11a by Fe3+ ions was determined by Stern-Volmer (S-V) equation (Parmar et al., 2017a; Parmar et al., 2017b): I0/I = 1 + Ksv [C] (I and I0 are photoluminescence intensities of the 11a after and before titration with analytes, respectively; [C] is the molar concentration of analytes ions in mM; Ksv is known to be S-V constant in M−1). Change in the PL intensity was recorded upon incremental addition of an aqueous solution of Fe3+ ions (2.5 mM) to the aforesaid MOF dispersion in water (Figure 3B). The bent S-V plot at higher Fe3+ concentration might be attributed to the dynamic and static quenching process. (Chen et al., 2018) For the calculation of KSV, a linear correlation between the PL intensity and Fe3+ concentration was considered at lower concentration range (0–0.08 mM), which resulted remarkable value of 1.91 × 104 M−1 (Table 1) that stands well in literature reports for detection for Fe3+ ions (Supplementary Table S6). Further, limit of detection (LOD) was obtained from changes in the emission intensity on incremental addition of 10 µM solution of Fe3+ ions. By employing the standard equation 3σ/K (Nagarkar et al., 2014; Karmakar et al., 2017) (σ = standard deviation of initial intensity of the MOF without analyte for five consecutive blank measurements at 2 min intervals, K = slope of the linear curve in Supplementary Figure S9) the LOD value for Fe3+ turned out (Supplementary Table S3) to be 0.219 μM (corresponding to 166 ppb). Given quenching efficiency, KSV value, and LOD are important and desired criteria for sensing application, Fe3+ ion sensing by 11a truly corroborates ultra-sensitive detection in comparison to literature reports (Supplementary Table S6).
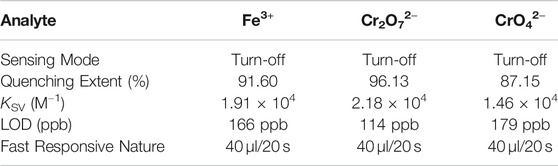
TABLE 1. Performance Characteristics of 11a for Detection of Fe3+, CrO42− and Cr2O72− ions in Water.
To further corroborate real-time applicability, visual detection of Fe3+ ions was targeted in the solution phase. As divulged in Figure 7B, the high fluorescence of aqueous dispersion of 11a under UV light (365 nm) immediately quenches upon one drop addition of Fe3+ solution, and authenticates to solution-phase visual detection of Fe3+ions.
Selectivity, Recyclability and Fast Responsive Fe3+ Sensing
Given presence of other metal ions cannot be neglected in real systems, competitive analysis test (CAT) was considered. To a 2 ml suspension of MOF, 120 µl of interfering analyte (2.5 mM) was added first, followed by similar amount of Fe3+ ion. While PL intensity did not change during the addition of interfering analytes, the solution exhibited more than 90% decrease of emission upon second addition. Further, to ensure the reusability of the material, MOF particles were separated via centrifugation after sensing experiments, washed thoroughly with water followed by methanol, and air-dried. The intensity of the recycled material was restored up to five consecutive sensing-recovery cycles (Figure 4A). Structural integrity and purity of reused 11a were verified from unchanged PXRD patterns to that of pristine ones. In addition, the response time of 11a towards sensing of Fe3+ was studied by recording the time-dependent change in the luminescence intensity. (Goswami et al., 2019b). For this, 40 μl of Fe3+ solution (2.5 mM) was added to the MOF dispersion, wherein luminescence intensity (Figure 4B) dropped to 61.9%. The change in PL intensity was recorded for 120 s after a regular interval of 20 s. Remarkably, no significant change in the photoluminescence intensity was observed beyond 20 s, and affirms fast responsive Fe3+ ion detection in aqueous phase.
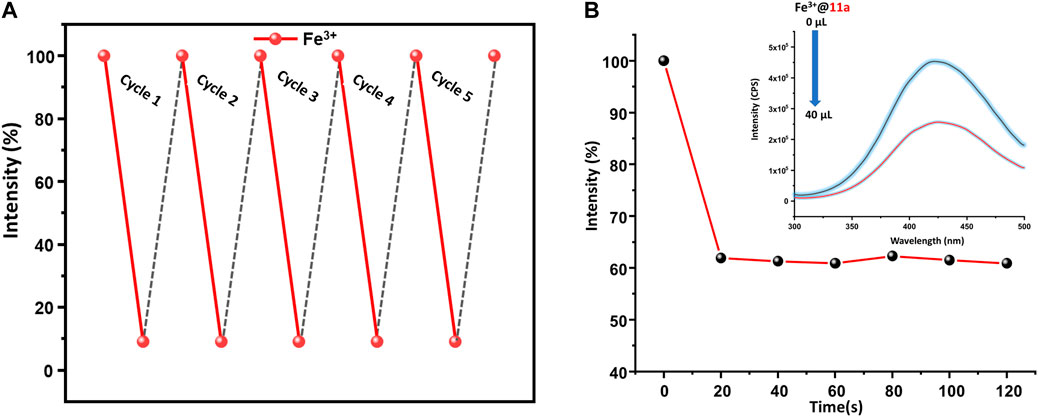
FIGURE 4. (A) Regeneration of pristine fluorescence intensity of 11a towards 2.5 mM Fe3+ solution used for 5 cycles, (B) Time dependent change in fluorescence intensity of 11a, upon addition of Fe3+ ion (recorded up to 120 s after a regular interval of 20 s).
Luminescence Detection of Cr(VI) oxo-anions (Cr2O72-/CrO42-)
Selective luminescent detection of Fe3+ in highly sensitive and fast responsive manner inspired us to explore further application of this framework towards sensing of anions. We performed anion detection considering potassium salts of a series of anions (NO3−, SO42−, MoO42−, NO2−, Cl−, F−, PO43−, SCN−, I−, MnO4−, Br−, CrO42− and Cr2O72−) in water (2.5 mM). These solutions were separately added to the aqueous dispersions of 11a. Among others, a significant quenching of PL intensity was observed by hexavalent oxo-chromium anions (Cr2O72−, CrO42−), whereas rest of the anions did not show any major changes in luminescence intensity. The order of quenching (Supplementary Figure S10) was found to be Cr2O72−>CrO42−>MnO4−>SCN−>MoO42−>I−>NO3−>NO2−>SO42–>Cl−>Br−>PO43−. The quenching efficiency for Cr2O72− and CrO42− are 96.13 and 87.15%, respectively. For quantitative determination of the extent of quenching, changes in luminescence intensity of 11a were recorded by incremental addition of anionic solution. Stern–Volmer constant was obtained (vide supra) via plotting [(I0/I)-1] vs. concentration of the analyte that revealed linear curve at the lower concentration (Figure 5C,D) and deviation from linearity occurs as the concentration raises. Ksv values were calculated from linear portion of the curve and found to be 1.46 × 104 and 2.18 × 104 M−1 for CrO42− and Cr2O72−, respectively. In addition, LOD (vide supra) was evaluated by the gradual addition of 10 μM aqueous anionic solutions to 2 ml MOF dispersion. From linear fitting of the graph (Supplementary Figure S11, Supplementary Figure S12) involving intensity against analyte concentration, LOD was calculated to be 179 and 114 ppb for CrO42− and Cr2O72−, individually. A comparison of MOF-based fluorescence sensors for the detection of lethal oxo-anionic Cr(VI) pollutants in water is tabulated in Supplementary Table S7, which indicates that quenching constants from this study rank one of the best values among existing reports. Essentially, such low detection limits render 11a a suitable candidate for monitoring of these lethal oxo anions in water.
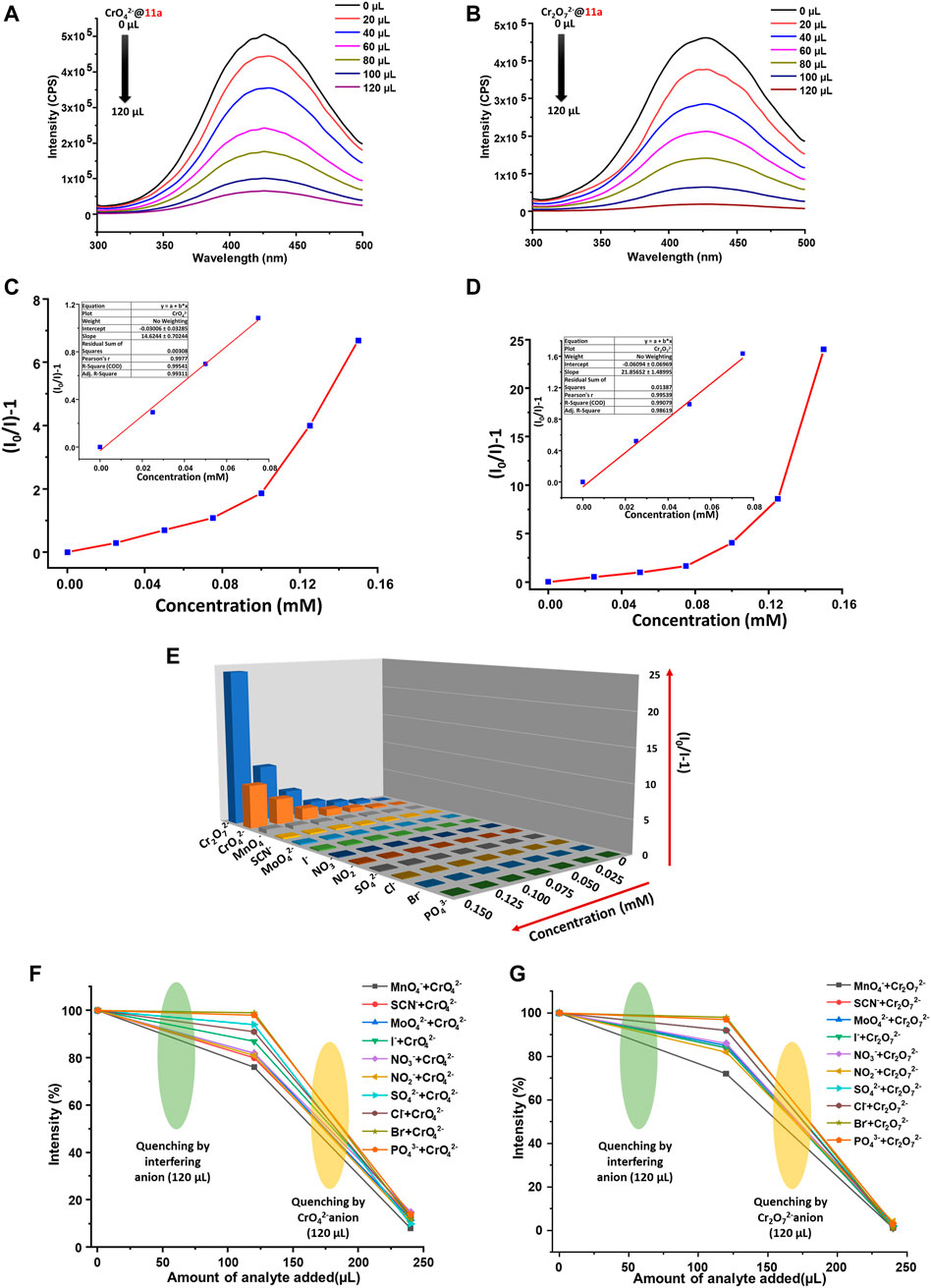
FIGURE 5. Photoluminescence spectra of 11a upon gradual addition of aqueous solution (2.5 mM) (A) CrO42− and (B) Cr2O72−, Stern-Volmer plot for 11a upon incremental addition of (C) CrO42− and (D) Cr2O72−(Inset: linear region of the plot), (E) S-V plots for all studied anions (2.5 mM) upon titration with well-dispersed suspension of 11a (λmax of emission 11a ca. 423 nm; 5 nm slit width) Interference plot showing the decrease in photoluminescence intensity intensities upon the addition of aqueous solution of various anions (2.5 mM) followed by (F) CrO42- and (G) Cr2O72-.
Selective, Multicyclic and Fast-Responsive Detection of Cr (VI) Anions
Keeping in mind that real-system possesses many other interfering anions, we next probed selectivity of 11a towards detection of Cr2O72− and CrO42− ions by performing CAT, maintaining standard protocol (vide supra). In a distinctive experiment, 120 µl of interfering analyte solution (2.5 mM) was added, which resulted almost insignificant changes to the emission behaviour of 11a. Quite in contrast, successive addition of same amount of individual oxo-anionic Cr(VI) solution to the above mixture (MOF + interfering anions) leads to a drastic turn-off response to the PL intensity (Figures 5F,G). It is imperative to state that we repeated this experiment three times to ensure the chronology of results. Such remarkably selective sensing of Cr(VI) oxo-anion is of true elegance and validates 11a as a potential and reliable probe for detection of Cr(VI) oxo-anions in aqueous phase.
Given reclamation and reusability of a heterogeneous sensory material is of crucial importance, 11a was centrifuged after sensing experiments, washed thoroughly with water, followed by methanol and finally air-dried. It was observed that PL intensity could be restored up to five sensing-recovery cycles (Figure 6A) and indicative of consistency of its performance towards hexavalent chromium ion detection. Structural robustness of 11a during repetitive sensing was additionally assured from intact positions of all peaks in its PXRD pattern (Supplementary Figure S13) after sensing experiment. Further, fast-responsive analyte test (FRAT) was conducted to confirm the response time. Decrease in the intensity was recorded upon addition of 40 µl of Cr(VI) oxo anion solution to the MOF dispersion as a function of time. Emission response decreased to 69.9 and 58.2% for CrO42− and Cr2O72− ions (Figures 6B,C), respectively. Change in PL intensity was recorded up to 120 s after a regular interval of 20 s, which revealed no significant change in luminescence intensity beyond 20 s. These results further validated fast responsive sensing of both the Cr(VI) anionic species in water. Motivated by excellent solution-phase of detection, an attempt was made to visually detect Cr(VI) ions in the aqueous phase. MOF dispersion was exposed to UV light (265 nm), which resulted bright blue emission. Addition of just one drop of either CrO42− or Cr2O72− ions instantaneously diminished (Figure 7B) this emission and supports real-time visual monitoring as well.
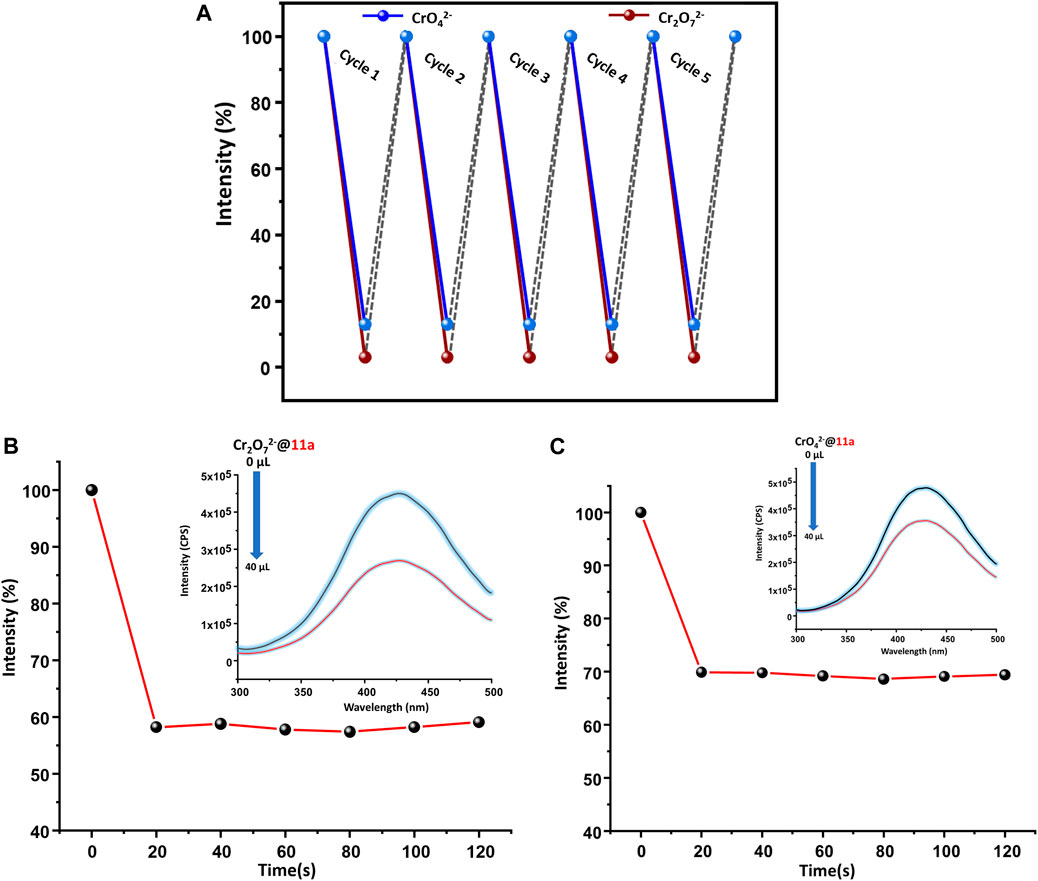
FIGURE 6. (A) Regeneration of pristine fluorescence intensity of 11a towards CrO42− /Cr2O72− used for 5 cycles, Time dependent change in fluorescence intensity of 11a, upon addition of (B) Cr2O72− /(C) CrO42−ions (recorded up to 120 s after a regular interval of 20 s).
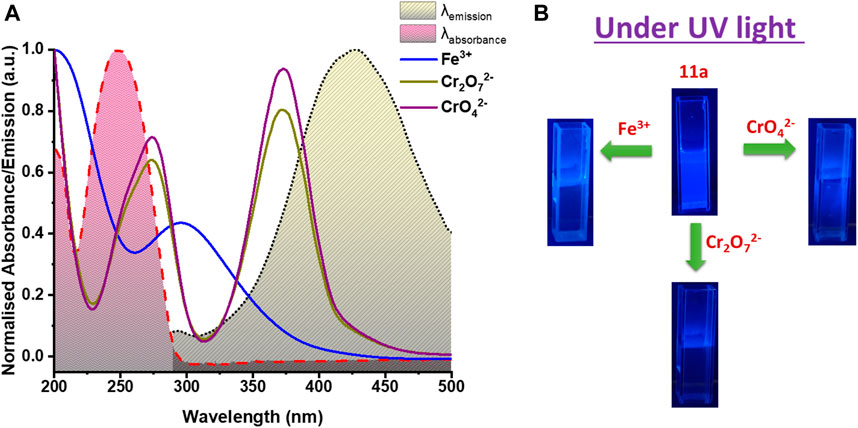
FIGURE 7. (A) Spectral overlap between absorbance spectra of studied analytes (Fe3+, Cr2O72 − and CrO42 −) and emission as well as excitation spectrum of 11a, (B) Visible change under UV light in luminescence intensity of 11a on addition of Fe3+, Cr2O72− and CrO42− solution.
Luminescent Quenching Mechanism
To look into the sensing mechanism of Fe3+ ions and Cr(VI) oxo-anions, a series of experimental studies were performed. In all the cases, quenching of the PL intensity due to collapse of the framework was discarded on the basis of unaltered PXRD patterns (Supplementary Figure S13) after sensing. The possibility of exchange of Fe3+ ions with the constituting Cd(II) ions is nullified from fast-responsive detection, in a sense that trans metalation reaction in such a short time span is not possible (Figure 4A). Another probability includes adsorption of Fe3+ and/or Cr2O72−/CrO42− ions within the framework. To this end, 11a was separately immersed to aqueous solutions of Fe3+, Cr2O72−and CrO42− for about a day. Resultant products Fe3+@11a, CrO42−@11a and Cr2O72−@11a were analysed by inductively coupled plasma optical emission spectrometry (ICP-OES), which validated no encapsulation of these ions even after prolonged exposure from the ratio of Cd (II) and other ions (Supplementary Table S2). Moreover, ICP-OES of the supernatant solution showed absence of Cd2+ ions and further corroborated no leaching of Cd(II) ions. Similarly, FT-IR analysis (Supplementary Figure S14) of the samples did not show any additional peak and eliminates the chances of encapsulation of these ions. As a matter of fact, the easy recyclability of 11a after every detection through simple washing also indicates weak surface interaction between the studied analytes and the MOF.
In this milieu, the competition for the excitation energy absorption between 11a and other ions may lead to the change of the luminescent signals. (Chen et al., 2017b; Dong et al., 2017). Figure 7A shows the UV absorption spectra of Fe3+, CrO42− and Cr2O72− plotted together with absorption and emission spectra of 11a. For Fe3+, relatively broad and moderate adsorption was perceived covering both excitation and emission peak of 11a. Of note, other metal ions have no such conspicuous absorption overlapping with the framework. This leads us to conclude that Fe3+ ions can absorb the excitation and emission light of 11a, thereby decreasing its luminescence intensity. (Guo et al., 2019) On the other hand, competitive energy absorption was envisioned as a valid, alternative reason for optical response quenching. CrO42−/Cr2O72− have two absorption bands in the range 220– 450 nm that cover a wide range of excitation and emission bands of 11a. In contrast, other anions do not absorb in this particular wavelength region. So excitation energy will be strongly absorbed only by these hexavalent Cr(VI) anions, reducing UV-vis absorption of the 11a, and resulting in substantial quenching. (Yi et al., 2015) Conversely, absorption spectra overlap of other metal ion and anions with absorption spectra of 11a show no significant overlap (Supplementary Figure S15a, Supplementary Figure S15b) and validates selective detection of studied analytes. Indeed, the excellent extent of overlap between the absorption spectra of Fe3+, Cr(VI) oxo-ions and emission spectrum of 11a helps in producing fluorescence quenching owing to the resonance energy transfer (RET).
Conclusion
In conclusion, mixed-ligand approach has been effectively harnessed in synthesizing a hydrolytically robust, bipillar-layer Cd(II)-framework from the combination of bifunctional ligand 4-(4-carboxyphenyl)-1,2,4-triazole (HL) and linker bpy. The 2-fold interpenetrated structure with one-dimensional porous channels shows high open-air stability and retains its network integrity in common organic solvents. The π-electrons rich organic struts, suitably arranged through Cd(II) metal centres, allows high fluorescence intensity to the activated structure, which has been successfully utilized in sensitive and selective luminescent monitoring of Fe3+ ions in water with a 166 ppb limit of detection (LOD) and 20 s response time. The framework further reveals repetitive detection of two lethal Cr(VI) oxo-anions with fast-responsive emission quenching, where every quenching constants (CrO42−: 1.73 × 104 M−1; Cr2O72−: 5.42 × 104 M−1) and LOD values (CrO42−: 179 ppb; Cr2O72−: 114 ppb) rank among the best sensory MOFs for detection of Cr(VI) ions in water. Apart from solution-titration based turn-off responses, visual detection of these ions under UV light has been observed that validates real-time applicability of the material. Owing to selective, multi-cyclic, fast responsive and aqueous phase luminescent based detection of contaminating ions, this material promises its suitability for sustainable applications. Detailed experimental studies have been carried out to understand the mechanism of selective quenching, including competitive absorption of the excitation energy and the resonance energy transfer between the host framework and individual analytes. Given aqueous phase acute luminescent detection of water contaminating ions belongs to important global agendas for sustainability, this robust MOF highlights the importance of structure–property synergies and represents a futuristic material for sensing applications.
Experimental Section
Synthesis of 4- (1H-imidazol-1-yl) benzoic acid (HL)
The ligand was synthesised and characterised by previously reported method (Refer to SI).
Synthesis of CSMCRI-11
A mixture of Cd(NO3)2∙4H2O (48.4 mg, 0.156 mmol), 4,4ʹ-bipyridyl (31.25 mg, 0.2 mmol) and 4-(1H-imidazol-1-yl)benzoic acid (HL) (37.23 mg, 0.19 mmol) was dissolved in N,N-dimethylformamide (DMF; 7 ml) sealed in 15 ml glass vial, and heated at 120 °C for 2 days. The colourless, rectangular crystals were isolated (Supplementary Scheme S2) in 65% yield.
Anal.Calcd. for C33H33N8O10Cd1.5 = [Cd1.5(L)2(bpy)(NO3)]·DMF·2H2O: C, 45.54; H, 3.82; N, 12.88%; found: C, 44.23; H, 3.92; N, 12.43%. FT-IR (KBr) analysis: 1303, 1399, 1563, 1612, 1663, & 3414 cm−1 (Supplementary Figure S4).
Synthesis of 11a
As synthesised crystals were washed with fresh DMF and finally dried in air. The guest solvents in CSMCRI-11 were exchanged with methanol by soaking the crystals in methanol for 3 days followed by exchanging the solvent 3 times a day. The crystals were dried overnight under vacuum at 120 °C to generate a solvent-free framework 11a.
Anal.Calcd. for C30H22N7O7Cd1.5 = [Cd1.5(L)2(bpy)(NO3)] C, 47.34; H, 2.91; N, 12.88%. found: C, 46.17; H, 3.01; N, 12.45%.
Data Availability Statement
The datasets presented in this study can be found in online repositories. CCDC 2054352 contains the supplementary crystallographic data for this paper. The data can be obtained free of charge from The Cambridge Crystallographic Data Centre via www.ccdc.cam.ac.uk/data_request/cif.
Author Contributions
All authors listed have made a substantial, direct and intellectual contribution to the work, and approved it for publication.
Funding
CSMCRI communication number is 28/2021. SN acknowledges financial support from DST-SERB (Grant No. ECR/2016/000156) and CSIR (Grant No. MLP-0028). MS acknowledges UGC, Delhi, for providing senior research fellowship. GK acknowledges CSIR, Delhi for fellowship. The analytical support from AESD&CIF is greatly acknowledged.
Conflict of Interest
The authors declare that the research was conducted in the absence of any commercial or financial relationships that could be construed as a potential conflict of interest.
Supplementary Material
The Supplementary Material for this article can be found online at: https://www.frontiersin.org/articles/10.3389/fchem.2021.651866/full#supplementary-material
References
Barba-Bon, A., Costero, A. M., Gil, S., Parra, M., Soto, J., Martínez-Máñez, R., et al. (2012). A new selective fluorogenic probe for trivalent cations. Chem. Commun. 48, 3000–3002. doi:10.1039/C2CC17184H
Chandra Rao, P., and Mandal, S. (2018). Europium-based metal–organic framework as a dual luminescence sensor for the selective detection of the phosphate anion and Fe3+ ion in aqueous media. Inorg. Chem. 57, 11855–11858. doi:10.1021/acs.inorgchem.8b02017
Chen, B., Yang, Y., Zapata, F., Lin, G., Qian, G., and Lobkovsky, E. B. (2007). Luminescent open metal sites within a metal–organic framework for sensing small molecules. Adv. Mater. 19, 1693–1696. doi:10.1002/adma.200601838
Chen, C.-H., Wang, X.-S., Li, L., Huang, Y.-B., and Cao, R. (2018). Highly selective sensing of Fe3+ by an anionic metal–organic framework containing uncoordinated nitrogen and carboxylate oxygen sites. Dalton Trans. 47, 3452–3458. doi:10.1039/C8DT00088C
Chen, M., Xu, W.-M., Tian, J.-Y., Cui, H., Zhang, J.-X., Liu, C.-S., et al. (2017a). A terbium(III) lanthanide–organic framework as a platform for a recyclable multi-responsive luminescent sensor. J. Mater. Chem. C 5, 2015–2021. doi:10.1039/C6TC05615F
Chen, S., Shi, Z., Qin, L., Jia, H., and Zheng, H. (2017b). Two new luminescent Cd(II)-Metal–Organic frameworks as bifunctional chemosensors for detection of cations Fe3+, anions CrO42–, and Cr2O72– in aqueous solution. Cryst. Growth Des. 17, 67–72. doi:10.1021/acs.cgd.6b01197
Cohen, S. M. (2010). Modifying MOFs: new chemistry, new materials. Chem. Sci. 1, 32–36. doi:10.1039/C0SC00127A
Cui, Y., Yue, Y., Qian, G., and Chen, B. (2011). Luminescent functional metal–organic frameworks. Chem. Rev. 112, 1126–1162. doi:10.1021/cr200101d
Dang, S., Ma, E., Sun, Z.-M., and Zhang, H. (2012). A layer-structured Eu-MOF as a highly selective fluorescent probe for Fe3+ detection through a cation-exchange approach. J. Mater. Chem. 22, 16920–16926. doi:10.1039/C2JM32661B
Dong, C., Wu, G., Wang, Z., Ren, W., Zhang, Y., Shen, Z., et al. (2016). Selective colorimetric detection of Cr(III) and Cr(VI) using gallic acid capped gold nanoparticles. Dalton Trans. 45, 8347–8354. doi:10.1039/C5DT04099J
Dong, J., Xu, H., Hou, S.-L., Wu, Z.-L., and Zhao, B. (2017). Metal–organic frameworks with Tb4 clusters as nodes: luminescent detection of chromium(VI) and chemical fixation of CO2. Inorg. Chem. 56, 6244–6250. doi:10.1021/acs.inorgchem.7b00323
El Rassi, Z. (1997). Capillary electrophoresis of pesticides. Electrophoresis 18, 2465–2481. doi:10.1002/elps.1150181236
Gogoi, C., and Biswas, S. (2018). A new quinoline based luminescent Zr(IV) metal–organic framework for the ultrasensitive recognition of 4-nitrophenol and Fe(III) ions. Dalton Trans. 47, 14696–14705. doi:10.1039/C8DT03058H
Goswami, R., Mandal, S. C., Pathak, B., and Neogi, S. (2019a). Guest-induced ultrasensitive detection of multiple toxic organics and Fe3+ ions in a strategically designed and regenerative smart fluorescent metal–organic framework. ACS Appl. Mater. Inter. 11, 9042–9053. doi:10.1021/acsami.8b20013
Goswami, R., Mandal, S. C., Seal, N., Pathak, B., and Neogi, S. (2019b). Antibiotic-triggered reversible luminescence switching in amine-grafted mixed-linker MOF: exceptional turn-on and ultrafast nanomolar detection of sulfadiazine and adenosine monophosphate with molecular keypad lock functionality. J. Mater. Chem. A. 7, 19471–19484. doi:10.1039/C9TA06632B
Guo, X.-Y., Dong, Z.-P., Zhao, F., Liu, Z.-L., and Wang, Y.-Q. (2019). Zinc(II)–organic framework as a multi-responsive photoluminescence sensor for efficient and recyclable detection of pesticide 2,6-dichloro-4-nitroaniline, Fe(III) and Cr(VI). New J. Chem. 43, 2353–2361. doi:10.1039/C8NJ05647A
Karmakar, A., Samanta, P., Desai, A. V., and Ghosh, S. K. (2017). Guest-responsive metal–organic frameworks as scaffolds for separation and sensing applications. Acc. Chem. Res. 50, 2457–2469. doi:10.1021/acs.accounts.7b00151
Kitagawa, S., Kitaura, R., and Noro, S. (2004). Functional porous coordination polymers. Angew. Chem. Int. Ed. 43, 2334–2375. doi:10.1002/anie.200300610
Kreno, L. E., Leong, K., Farha, O. K., Allendorf, M., Van Duyne, R. P., and Hupp, J. T. (2012). Metal–organic framework materials as chemical sensors. Chem. Rev. 112, 1105–1125. doi:10.1021/cr200324t
Li, Y., Wei, Z., Zhang, Y., Guo, Z., Chen, D., Jia, P., et al. (2019). Dual-emitting EY@Zr-MOF composite as self-calibrating luminescent sensor for selective detection of inorganic ions and nitroaromatics. ACS Sustainable Chem. Eng. 7, 6196–6203. doi:10.1021/acssuschemeng.8b06500
Liu, G., Lu, Y.-K., Ma, Y.-Y., Wang, X.-Q., Hou, L., and Wang, Y.-Y. (2019). Syntheses of three new isostructural lanthanide coordination polymers with tunable emission colours through bimetallic doping, and their luminescence sensing properties. Dalton Trans. 48, 13607–13613. doi:10.1039/C9DT02733E
Liu, J.-Q., Li, G.-P., Liu, W.-C., Li, Q.-L., Li, B.-H., Gable, R. W., et al. (2016). Two unusual nanocage-based ln-MOFs with triazole sites: highly fluorescent sensing for Fe3+ and Cr2O72−, and selective CO2 capture. ChemPlusChem 81, 1299–1304. doi:10.1002/cplu.201600289
Luo, L., Wang, P., Xu, G.-C., Liu, Q., Chen, K., Lu, Y., et al. (2012). Zinc(II) and cadmium(II) complexes with rigid 3,3′,5,5′-tetra(1H-imidazol-1-yl)-1,1′-biphenyl and varied carboxylate ligands. Cryst. Growth Des. 12, 2634–2645. doi:10.1021/cg300220q
Lustig, W. P., Mukherjee, S., Rudd, N. D., Desai, A. V., Li, J., and Ghosh, S. K. (2017). Metal–organic frameworks: functional luminescent and photonic materials for sensing applications. Chem. Soc. Rev. 46, 3242–3285. doi:10.1039/C6CS00930A
Lv, R., Wang, J., Zhang, Y., Li, H., Yang, L., Liao, S., et al. (2016). An amino-decorated dual-functional metal–organic framework for highly selective sensing of Cr(III) and Cr(VI) ions and detection of nitroaromatic explosives. J. Mater. Chem. A. 4, 15494–15500. doi:10.1039/C6TA05965A
Ma, T., Zhao, X., Matsuo, Y., Song, J., Zhao, R., Faheem, M., et al. (2019). Fluorescein-based fluorescent porous aromatic framework for Fe3+ detection with high sensitivity. J. Mater. Chem. C 7, 2327–2332. doi:10.1039/C8TC06288A
Nagarkar, S. S., Desai, A. V., and Ghosh, S. K. (2014). A fluorescent metal–organic framework for highly selective detection of nitro explosives in the aqueous phase. Chem. Commun. 50, 8915–8918. doi:10.1039/C4CC03053B
Parmar, B., Rachuri, Y., Bisht, K. K., Laiya, R., and Suresh, E. (2017a). Mechanochemical and conventional synthesis of Zn(II)/Cd(II) luminescent coordination polymers: dual sensing probe for selective detection of chromate anions and TNP in aqueous phase. Inorg. Chem. 56, 2627–2638. doi:10.1021/acs.inorgchem.6b02810
Parmar, B., Rachuri, Y., Bisht, K. K., and Suresh, E. (2017b). Mixed-ligand LMOF fluorosensors for detection of Cr(VI) oxyanions and Fe3+/Pd2+ cations in aqueous media. Inorg. Chem. 56, 10939–10949. doi:10.1021/acs.inorgchem.7b01130
Pettinari, C., Marchetti, F., Mosca, N., Tosi, G., and Drozdov, A. (2017). Application of metal − organic frameworks. Polym. Int. 66, 731–744. doi:10.1002/pi.5315
Samanta, P., Desai, A. V., Let, S., and Ghosh, S. K. (2019). Advanced porous materials for sensing, capture and detoxification of organic pollutants toward water remediation. ACS Sustainable Chem. Eng. 7, 7456–7478. doi:10.1021/acssuschemeng.9b00155
Seal, N., Goswami, R., Singh, M., Pillai, R. S., and Neogi, S. (2021a). An ultralight charged MOF as fluoro-switchable monitor for assorted organo-toxins: size-exclusive dye scrubbing and anticounterfeiting applications via Tb3+ sensitization. Inorg. Chem. Front. 8, 296–310. doi:10.1039/D0QI01091J
Seal, N., Singh, M., Das, S., Goswami, R., Pathak, B., and Neogi, S. (2021b). Dual-functionalization actuated trimodal attribute in an ultra-robust MOF: exceptionally selective capture and effectual fixation of CO2 with fast-responsive, nanomolar detection of assorted organo-contaminants in water. Mater. Chem. Front. 5, 979–994. doi:10.1039/D0QM00721H
Senthilkumar, S., Goswami, R., Smith, V. J., Bajaj, H. C., and Neogi, S. (2018). Pore wall-functionalized luminescent Cd(II) framework for selective CO2 adsorption, highly specific 2,4,6-trinitrophenol detection, and colorimetric sensing of Cu2+ ions. ACS Sustainable Chem. Eng. 6, 10295–10306. doi:10.1021/acssuschemeng.8b01646
Singh, M., Senthilkumar, S., Rajput, S., and Neogi, S. (2020). Pore-functionalized and hydrolytically robust Cd(II)-Metal–Organic framework for highly selective, multicyclic CO2 adsorption and fast-responsive luminescent monitoring of Fe(III) and Cr(VI) ions with notable sensitivity and reusability. Inorg. Chem. 59, 3012–3025. doi:10.1021/acs.inorgchem.9b03368
Sun, J., Guo, P., Liu, M., and Li, H. (2019). A novel cucurbit[6]Uril-based supramolecular coordination assembly as a multi-responsive luminescent sensor for Fe3+, Cr2O72− and isoquinoline antibiotics in aqueous medium. J. Mater. Chem. C 7, 8992–8999. doi:10.1039/C9TC02666E
Vikrant, K., Tsang, D. C. W., Raza, N., Giri, B. S., Kukkar, D., and Kim, K.-H. (2018). Potential utility of metal–organic framework-based platform for sensing pesticides. ACS Appl. Mater. Inter. 10, 8797–8817. doi:10.1021/acsami.8b00664
Wan, X.-Y., Jiang, F.-L., Chen, L., Pan, J., Zhou, K., Su, K.-Z., et al. (2015). Structural variability, unusual thermochromic luminescence and nitrobenzene sensing properties of five Zn(II) coordination polymers assembled from a terphenyl-hexacarboxylate ligand. CrystEngComm 17, 3829–3837. doi:10.1039/C5CE00420A
Wang, Y., Cheng, L., Liu, Z.-Y., Wang, X.-G., Ding, B., Yin, L., et al. (2015). An ideal detector composed of two-dimensional Cd(II)–Triazole frameworks for nitro-compound explosives and potassium dichromate. Chem. Eur. J. 21, 14171–14178. doi:10.1002/chem.201502167
Xu, H., Gao, J., Qian, X., Wang, J., He, H., Cui, Y., et al. (2016). Metal–organic framework nanosheets for fast-response and highly sensitive luminescent sensing of Fe3+. J. Mater. Chem. A. 4, 10900–10905. doi:10.1039/C6TA03065C
Xu, X.-Y., and Yan, B. (2015). Eu(III)-functionalized MIL-124 as fluorescent probe for highly selectively sensing ions and organic small molecules especially for Fe(III) and Fe(II). ACS Appl. Mater. Inter. 7, 721–729. doi:10.1021/am5070409
Yan, B. (2019). Photofunctional MOF-based hybrid materials for the chemical sensing of biomarkers. J. Mater. Chem. C 7, 8155–8175. doi:10.1039/C9TC01477B
Yang, W., Li, J., Xu, Z., Yang, J., Liu, Y., and Liu, L. (2019). A Eu-MOF/EDTA-NiAl-CLDH fluorescent micromotor for sensing and removal of Fe3+ from water. J. Mater. Chem. C 7, 10297–10308. doi:10.1039/C9TC03328A
Yao, C.-X., Zhao, N., Liu, J.-C., Chen, L.-J., Liu, J.-M., Fang, G.-Z., et al. (2020). Recent progress on luminescent metal-organic framework-involved hybrid materials for rapid determination of contaminants in environment and food. Polymers(Basel). 12, 691. doi:10.3390/polym12030691
Yi, F.-Y., Li, J.-P., Wu, D., and Sun, Z.-M. (2015). A series of multifunctional metal–organic frameworks showing excellent luminescent sensing, sensitization, and adsorbent abilities. Chem. Eur. J. 21, 11475–11482. doi:10.1002/chem.201500595
Zhai, Z.-W., Yang, S.-H., Lv, Y.-R., Du, C.-X., Li, L.-K., and Zang, S.-Q. (2019). Amino functionalized Zn/Cd-Metal–Organic frameworks for selective CO2 adsorption and knoevenagel condensation reactions. Dalton Trans. 48, 4007–4014. doi:10.1039/C9DT00391F
Zhang, J., Gong, L., Feng, J., Wu, J., and Zhang, C. (2017). Two luminescent Zn(II)/Cd(II) metal–organic frameworks as rare multifunctional sensors. New J. Chem. 41, 8107–8117. doi:10.1039/C7NJ01072A
Zhang, Q., Wang, J., Kirillov, A. M., Dou, W., Xu, C., Xu, C., et al. (2018). Multifunctional ln–MOF luminescent probe for efficient sensing of Fe3+, Ce3+, and acetone. ACS Appl. Mater. Inter. 10, 23976–23986. doi:10.1021/acsami.8b06103
Zhao, H., Ni, J., Zhang, J.-J., Liu, S.-Q., Sun, Y.-J., Zhou, H., et al. (2018). A trichromatic MOF composite for multidimensional ratiometric luminescent sensing. Chem. Sci. 9, 2918–2926. doi:10.1039/C8SC00021B
Zhao, X., Li, Y., Chang, Z., Chen, L., and Bu, X.-H. (2016). A four-fold interpenetrated metal–organic framework as a fluorescent sensor for volatile organic compounds. Dalton Trans. 45, 14888–14892. doi:10.1039/C6DT02169G
Zhou, E.-L., Qin, C., Tian, D., Wang, X.-L., Yang, B.-X., Huang, L., et al. (2018). A difunctional metal–organic framework with Lewis basic sites demonstrating turn-off sensing of Cu2+ and sensitization of Ln3+. J. Mater. Chem. C 6, 7874–7879. doi:10.1039/c8tc02425a
Zhou, H.-C., Long, J. R., and Yaghi, O. M. (2012). Introduction to metal–organic frameworks. Chem. Rev. 112, 673–674. doi:10.1021/cr300014x
Zhu, A.-X., Qiu, Z.-Z., Yang, L.-B., Fang, X.-D., Chen, S.-J., Xu, Q.-Q., et al. (2015). A luminescent cadmium(ii) metal–organic framework based on a triazolate–carboxylate ligand exhibiting selective gas adsorption and guest-dependent photoluminescence properties. CrystEngComm 17, 4787–4792. doi:10.1039/C5CE00405E
Keywords: metal-organic frameworks (MOFs), hydrolytic stability, luminescent sensing, water contaminants, fast responsive detection
Citation: Singh M, Kumar G and Neogi S (2021) Devising Mixed-Ligand Based Robust Cd(II)-Framework From Bi-Functional Ligand for Fast Responsive Luminescent Detection of Fe3+ and Cr(VI) Oxo-Anions in Water With High Selectivity and Recyclability. Front. Chem. 9:651866. doi: 10.3389/fchem.2021.651866
Received: 11 January 2021; Accepted: 15 February 2021;
Published: 05 May 2021.
Edited by:
Sukhendu Mandal, Indian Institute of Science Education and Research, IndiaReviewed by:
Giuseppina La Ganga, University of Messina, ItalyShyam Biswas, Indian Institute of Technology Guwahati, India
Copyright © 2021 Singh, Kumar and Neogi. This is an open-access article distributed under the terms of the Creative Commons Attribution License (CC BY). The use, distribution or reproduction in other forums is permitted, provided the original author(s) and the copyright owner(s) are credited and that the original publication in this journal is cited, in accordance with accepted academic practice. No use, distribution or reproduction is permitted which does not comply with these terms.
*Correspondence: Subhadip Neogi, sneogi@csmcri.res.in
†ORCID: Subhadip Neogi, orcid.org/0000-0002-3838-4180; Manpreet Singh, orcid.org/0000-0002-0335-6147