Toward Food Freshness Monitoring: Coordination Binding–Based Colorimetric Sensor Array for Sulfur-Containing Amino Acids
- 1Institute of Industrial Science, The University of Tokyo, Tokyo, Japan
- 2Key Laboratory of Green Chemistry and Chemical Processes, Department of Chemistry, School of Chemistry and Molecular Engineering, East China Normal University, Shanghai, China
Herein, a self-assembled colorimetric chemosensor array composed of off-the-shelf catechol dyes and a metal ion (i.e., Zn2+) has been used for the sulfur-containing amino acids (SCAAs; i.e., glutathione, glutathione disulfide, L–cysteine, DL–homocysteine, and L–cystine). The coordination binding–based chemosensor array (CBSA) fabricated by a competitive assay among SCAAs, Zn2+ ions, and catechol dyes [i.e., pyrocatechol violet (PV), bromopyrogallol red (BPR), pyrogallol red (PR), and alizarin red S (ARS)] yielded fingerprint-like colorimetric changes. We succeeded in the qualification of SCAAs based on pattern recognition [i.e., a linear discrimination analysis (LDA)] with 100% correct classification accuracy. The semiquantification of reduced/oxidized forms of SCAAs was also performed based on LDA. Furthermore, we carried out a spike test of glutathione in food samples using the proposed chemosensor array with regression analysis. It is worth mentioning that we achieved a 91–110% recovery rate in real sample tests, which confirmed the accuracy of the constructed model. Thus, this study represents a step forward in assessing food freshness based on supramolecular analytical methods.
Introduction
To date, there is an increasing demand from consumers to evaluate the safety of food products. Freshness is the main standard for food quality assessment, including the physical form (Luo et al., 2021), the number of microorganisms (Jacxsens et al., 2003), and biochemical components (Lonchamp et al., 2009). Among the common biochemical components, sulfur-containing amino acids (SCAAs) including L–cysteine (Cys) (Cebi et al., 2017; Garcia et al., 2015), L–cystine (CySS) (Chen and Li, 2019), DL–homocysteine (HCys) (Hoey et al., 2007), glutathione reduced form (GSH) (Xu et al., 2015), and glutathione oxidized form (GSSG) (Moreira et al., 2011) have been used as analyte markers in food samples, including wine (Valero et al., 2003), wheat flour (Reinbold et al., 2008), and fruit juice (Fracassetti et al., 2011), to evaluate food quality. The reduced form of SCAAs acts as antioxidants in food (Nikolantonaki et al., 2018), which is oxidized by air over time. Thus, by quantifying the reduced form and the oxidized form of SCAAs, the freshness of food samples can be assessed.
SCAAs are currently quantified by instrumental methods (i.e., high-performance liquid chromatography (HPLC) (Zhu et al., 2020) and mass spectrometry (MS) (Kuster et al., 2008), or enzyme-linked immunosorbent assay (ELISA) (Kurose et al., 1997) because of their high reliability and accuracy. However, the requirement of expensive instruments, complex sensing procedures, and trained personnel limits their application for rapid and straightforward analysis. To simplify the sensing procedures, chemosensors have become a promising option, which can exhibit optical property changes based on molecular recognition (Hyman and Franz, 2012; Lee et al., 2015; Wu et al., 2015; Yan et al., 2017; Khorasani et al., 2019; Roy, 2021). In this regard, several optical chemosensors have been developed for natural amino acids (Buryak and Severin, 2005; Ma et al., 2013; Yin et al., 2013; Sener et al., 2014; Ghasemi et al., 2015; Meng et al., 2015; Chao and Zhang, 2017; Gholami et al., 2019; Liu et al., 2019; Xu et al., 2020). However, these chemosensors require complicated synthetic processes (Yang et al., 2011; Song et al., 2016; Yang et al., 2018), which limit their practical usage in real-world scenarios. Chemosensor arrays by supramolecular interactions with pattern recognition can avoid the synthetic processes and simultaneously quantify multiple analytes (Cao et al., 2020; Sasaki et al., 2021a; Sasaki et al., 2021b; Lyu et al., 2021). To the best of our knowledge, the development of a colorimetric sensor array using only a combination of off-the-shelf reagents for simultaneous SCAA detection has not yet been reported.
Herein, we report a simple, rapid, and accurate coordination binding–based chemosensor array (CBSA) for the high-throughput colorimetric detection of SCAAs (GSH, GSSG, Cys, hCys, and CySS). Four catechol dyes [i.e., pyrocatechol violet (PV), bromopyrogallol red (BPR), pyrogallol red (PR), and alizarin red S (ARS)] were employed as colorimetric indicators (Sasaki et al., 2019), and Zn2+ ions were used as the color and binding manipulator (Kaushik et al., 2015). The catechol dyes produce the dye–Zn2+ coordination complex upon the addition of Zn2+ ions, which exhibit colorimetric changes (Hamedpour et al., 2019). Subsequently, colorimetric changes can be observed by the addition of SCAAs because of the generation of coordination complexes of Zn2+ and SCAAs, which dissociate the dye–Zn2+ complex with a colorimetric recovery (Figure 1). The use of commercially available reagents avoids complex synthesis, which is a major advantage for the simple establishment of a sensor array (Sasaki et al., 2021c). In this study, various cross-reactive colorimetric responses demonstrated by the CBSA were analyzed using chemometric methods, including a linear discrimination analysis (LDA) (Anzenbacher et al., 2010) and regression analysis (SVM) (Hamel, 2009; Minami et al., 2012). The LDA is a mathematical method used in statistics to establish a linear combination to characterize two or more groups of objects, which could be used for qualitative and semiquantitative analyses of the targets. The SVM is a supervised learning model for data classification and regression analysis. To predict the unknown concentrations of the target samples, we established the SVM model with the calibration dataset using standard solutions. Notably, we quantified the pseudo-oxidation processes of SCAAs in aqueous media and predicted the GSH concentration in tomato and grapefruit juice (Minich and Brown, 2019). These results indicate that our simple preparation and user-friendly sensing system could achieve high-throughput analysis for SCAAs, which would be a step forward for assessing food freshness based on supramolecular analytical methods.
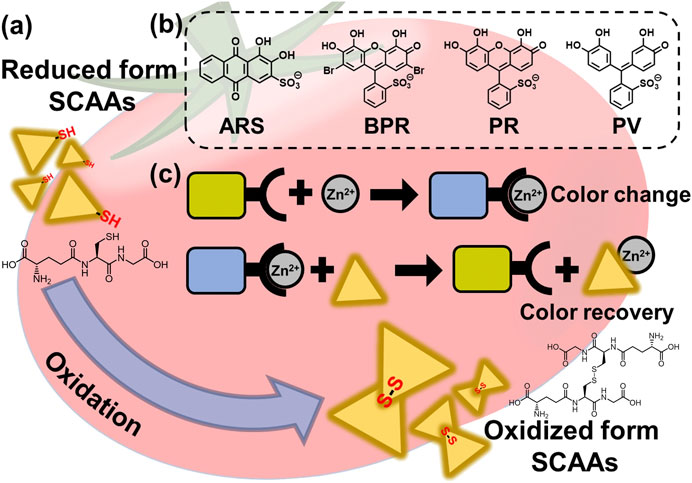
FIGURE 1. (A) Schematic illustration of the SCAA oxidation (e.g., glutathione). (B) Chemical structure of catechol dyes (PV, BPR, PR, and ARS). (C) Illustrated detection mechanism of SCAAs.
Materials and Methods
Materials
SCAAs (Cys, CySS, GSH, and HCys), 1,4-benzoquinone (pBQ), 3-mercaptopropionic acid (3-MPA), PR, BPR, PV, and other amino acids [L–alanine (Ala), L–arginine (Arg), L–aspartic acid (Asp), L–glutamine (Gln), L–glutamic acid (Gln), glycine (Gly), L–histidine (His), L–isoleucine (Ile), L–leucine (Leu), L–lysine (Lys), L–methionine (Met), L–phenylalanine (Phe), L–proline (Pro), L–serine (Ser), L–threonine (Thr), L–tryptophan (Trp), L–tyrosine (Tyr), and L–valine (Val)] were purchased from Tokyo Chemical Industries Co. Inc. (Tokyo, Japan). Additionally, ARS, sodium chloride, methanol, and zinc nitrate hexahydrate were purchased from FUJIFILM Wako Pure Chemical Co. Inc. (Osaka, Japan). N-(2-hydroxyethyl)-1-piperazineethanesulfonic acid (HEPES) was purchased from Dojindo Laboratories (Kumamoto, Japan). All chemicals were used without further purification. All aqueous samples were prepared with Milli-Q water (18.2 MΩcm) (Millipore, Bedford, MA, United States).
Measurements
The UV-Vis spectra were measured within the wavelength range of 350–800 nm at a scan rate of 240 nm/min using a Shimadzu UV-2600 UV-Vis spectrophotometer. The dye (40 μM) was mixed with Zn2+ solutions at various concentrations and incubated at room temperature (25°C) for 10–60 min (i.e., ARS, BPR, and PR for 60 min, and PV for 10 min) in HEPES buffer (50 mM) with 10 mM NaCl at pH 7.4. Titration isotherms were prepared from the variations with maximum absorption at 615 nm for PV, 557 nm for BPR, 543 nm for PR, and 515 nm for ARS, respectively.
Qualitative and quantitative analyses were carried out by array experiments in 384-well microplates using a microplate reader (SYNERGY H1, Biotek, Winooski, United States). For the array experiments, the dye–Zn2+ complex solution (90 μL) was first added to each well. Subsequently, the analyte and buffer solutions (10 μL) were mixed with the sensor solution. The microplate was then shaken for 3 min for incubation. Furthermore, a spike test using food samples was performed to evaluate the accuracy of the chemosensor array for real-world applications. A commercial tomato juice (Ito En tomato juice) was centrifuged at 14,000 rpm for 30 min to remove any insoluble matter. The supernatant fluid was diluted 40-fold and applied to a 384-well microplate without any further treatment. A series of standard GSH samples was calibrated with a concentration of 0–2 mM.
LDA was applied for qualitative analysis based on the raw dataset without any further treatment using SYSTAT 13. Moreover, a semiquantitative assay of the mixture of GSH/GSSG and Cys/CySS was also carried out using LDA. Student’s t-test was used to eliminate outlier data points. The quantitative analyses and real sample tests were conducted by a regression analysis based on a supporting vector machine (SVM) with Solo 7.5.2. Two parameters [root-mean-square errors for calibration (RMSEC) and prediction (RMSEP)] were applied to confirm the accuracy of the constructed models.
Discussions
The complexation of catechol dyes and Zn2+ ions in a HEPES buffer (50 mM) with NaCl (10 mM) at pH 7.4 and at 25°C was evaluated according to a previous study (Hamedpour et al., 2019). To maintain the ionic strength, sodium chloride (10 mM) was added. Each dye complex showed specific colorimetric changes upon the addition of SCAAs (GSH, GSSG, Cys, and hCys) (see Supplementary Material). The spectral shift accompanying the color recovery was observed by the ascension of the concentration, indicating the decomposition of the Zn2–catechol dye complex. Because of the low solubility of CySS in the buffer solution, we could not apply UV-Vis titration for CySS in this study. Association constants (Kassoc) for the SCAAs with Zn2+ ions were calculated by titration isotherms according to a nonlinear regression fitting method (see supporting material) (Hargrove et al., 2010). The Kassoc for GSH were determined as follows: PV: (4.7 ± 0.3) × 103 M−1; BPR: (2.8 ± 0.8) × 104 M−1; PR: (1.5 ± 0.3) × 105 M−1; and ARS: (6.5 ± 0.6) × 105 M−1. In the case of GSSG, the Kassoc were calculated as (1.6 ± 0.1) × 104 M−1, (9.6 ± 1.8) × 103 M−1, (2.4 ± 0.6) × 104 M−1, and (9.2 ± 1.7) × 106 M−1 PV for, BPR, PR, and ARS, respectively. With the various binding affinities for the targets, our proposed chemosensor array could be applied for simultaneous qualitative and quantitative SCAA detection. Interestingly, the reduced/oxidized form of glutathione showed different isotherms toward the dye–Zn2+ complex (Figure 2), which indicates that we could monitor the oxidizing process of SCAAs based on colorimetric changes.
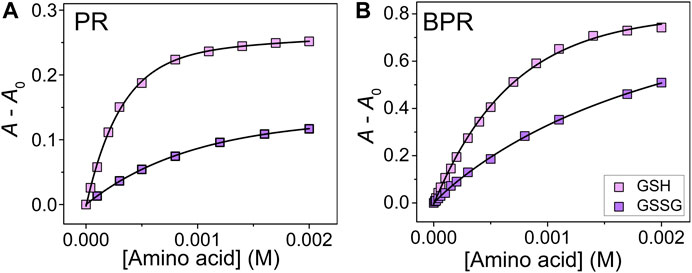
FIGURE 2. UV-Vis titration isotherm of (A) PR–Zn2+ and (B) BPR–Zn2+ to GSH and GSSG (0–2.0 mM) at 25°C. The concentrations of dye and Zn2+ were 40 μM.
Subsequently, we applied natural amino acids to our proposed chemosensor array for the selectivity test. Most of the natural amino acids [i.e., valine (Val), tyrosine (Tyr), tryptophan (Trp), threonine (Thr), serine (Ser), proline (Pro), phenylalanine (Phe), methionine (Met), lysine (Lys), leucine (Leu), isoleucine (Ile), glycine (Gly), glutamic acid (Glu), glutamine (Gln), aspartic acid (Asp), asparagine (Asn), arginine (Arg), and alanine (Ala)] showed slight or almost no response, whereas histidine (His) caused significant absorbance changes with the peak wavelength shifts. This response was due to the high association constant of Zn2+ ions and His compared to the aforementioned natural amino acids (Krężel and Maret, 2016). In addition, the selectivity test indicated that the sulfur group contributes to the sensing mechanism based on CBSA (see Supplementary Material) (Namuswe and Berg, 2012). Thus, we attempted to apply a high-throughput assay to the aforementioned five analytes (i.e., GSH, GSSG, hCys, Cys, and His). We selected LDA among the pattern recognition methods because it can reduce the dimensionality and evaluate classification accuracy based on a leave-one-out cross-validation protocol (i.e., the jackknife method). The classification of the six cluster groups (one control group and five analyte groups) achieved 100% accuracy (Supplementary Figure S24). Thus, we can conclude that the proposed chemosensor array can discriminate between similar structural amino acids and reduced/oxidized forms of SCAAs.
In addition, semiquantitative LDA was carried out using the proposed chemosensor array for the mixture of oxidized/reduced SCAAs (i.e., glutathione and cysteine). For example, GSH–GSSG was selected as the representative analyte pair at various concentration ratios (Figure 3). The dynamic concentration changing rate of GSH and GSSG was selected as 2:1 to mimic the natural oxidizing process of glutathione (Supplementary Table S5). It is worth mentioning that the semiquantitative LDA of the mixture of oxidized/reduced SCAAs achieved a 100% correct classification rate. Furthermore, quantitative analyses were employed without sample preprocessing to investigate their capability for practical applications. Quantitative analyses were performed in a mixture containing GSH–GSSG or Cys–CySS in different molar ratios. An SVM-based regression analysis was carried out to establish a rapid and accurate assay (Figure 4). The SVM process includes two steps: the first step for the calibration of the measured data set and the second step for feature prediction of the unknown samples. More importantly, the limits of detection (LoDs) based on the 3σ method (Miller and Miller, 2018) were calculated as follows: 2.4 ppm for Cys, 2.0 ppm for hCys, 0.4 ppm for GSH, and 2.6 ppm for GSSG. As aforementioned, the constructed SVM model demonstrated the prediction of unknown concentrations of SCAAs with high accuracy, which indicates our array system could be applied to real sample analyses.
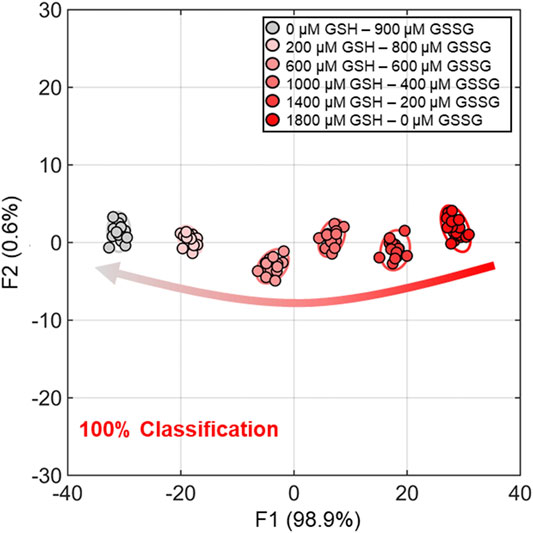
FIGURE 3. Semiquantitative LDA result for dynamic molar ratios of GSH and GSSG. The measurements were performed with eighteen repetitions for each concentration group for 100% classification accuracy. The confidence ellipses indicate 95% confidence rate.
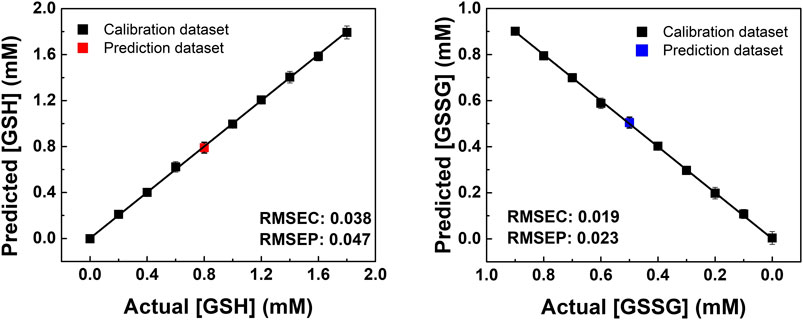
FIGURE 4. SVM regression analysis for quantitative analysis of the GSH and GSSG mixtures in various concentration ratios. The RMSEC and RMSEP values (shown as insets) represent the accuracy of the constructed model and the prediction.
Finally, we performed a spike test with GSH using concentrated tomato juice, grapefruit juice, and fresh tomato samples (Minich and Brown, 2019). Different concentrations of GSH were mixed with the diluted tomato juice and chemosensors, which were estimated by the previously calibrated SVM model (Figure 5). As shown in the Supplementary Table S6, the prediction of the GSH concentration in the real sample was successfully performed with a recovery rate of 91–110%. Moreover, the semiquantitative analysis offered 100% accurate classification of the fresh and oxidized tomato samples (Figure 6). The decrease in GSH concentration in the oxidized tomato sample was confirmed by HPLC–electrospray ionization MS (ESI-MS, see Supplementary Figures S32, S33). The LDA results indicated that the oxidized tomato sample demonstrated a closer distance to the low GSH concentration group, rather than the fresh sample (Figure 6). The result of the semiquantitative analysis and the spike test indicated that the chemosensor array combined with pattern recognition could be applied for quantifying unknown GSH concentrations, which suggests that the proposed method would become a rapid and promising method for the detection of SCAAs in food samples.
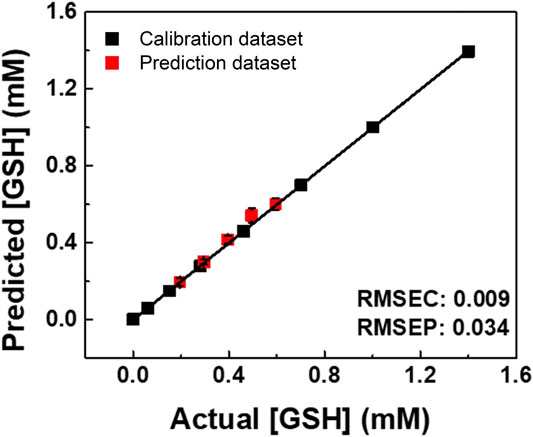
FIGURE 5. SVM regression for real sample analysis of GSH in a juice (Ito En tomato juice). The RMSEC and RMSEP values (shown as insets) represent the accuracy of the constructed model and the prediction.
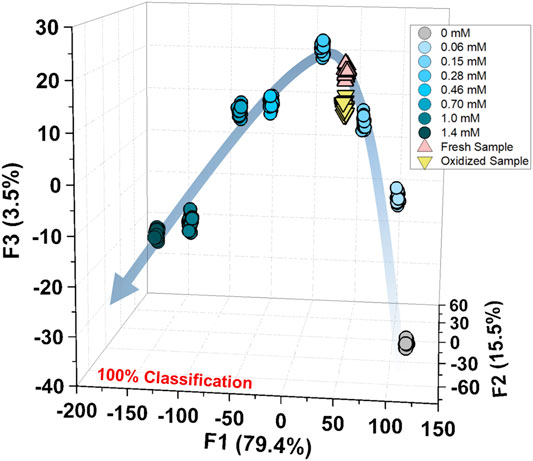
FIGURE 6. Semiquantitative analysis for the fresh and oxidized tomato samples with the calibration of GSH (0–1.4 mM). The measurements were repeated twenty times, resulting in 100% accurate classification.
Conclusion
In summary, we proposed a self-assembled colorimetric chemosensor array system for the qualitative and quantitative detection of SCAAs. The proposed array system was fabricated by CBSA utilizing off-the-shelf reagents, which avoided complicated synthetic processes. The reversible coordination binding of dye–Zn2+ complexes offered significant color changes upon the addition of the analytes. The LDA results reflected a clear classification of six groups (i.e., control, non-SCAAs, and reduced/oxidized SCAAs) with 100% classification accuracy. Moreover, quantitative analyses with high accuracy were achieved by the SVM, which allowed for the prediction of reduced/oxidized SCAAs in the mixtures. Most importantly, the spike test of GSH was performed in juice samples with high recovery rates. This study would lead to the application of supramolecular chemosensors for food freshness monitoring in the general society.
Data Availability Statement
The original contributions presented in the study are included in the article/Supplementary Material, further inquiries can be directed to the corresponding author.
Author Contributions
XL and YS performed the spectroscopic and high-throughput array experiments and wrote the manuscript. WT and XL performed the HPLC/ESI-MS analyses. JZ performed the spectroscopic experiments. TZ and YT wrote the manuscript. TM conceived the entire project.
Funding
TM acknowledges the financial support from JST CREST (Grant No. JPMJCR 2011) and JSPS KAKENHI (Grant Numbers JP21H01780 and JP20K21204). YS thanks JSPS KAKENHI (Grant No. JP18J21190). TM and YT also acknowledge the Japan–China Joint Research Cooperative Program (Joint Project).
Conflict of Interest
The authors declare that the research was conducted in the absence of any commercial or financial relationships that could be construed as a potential conflict of interest.
Supplementary Material
The Supplementary Material for this article can be found online at: https://www.frontiersin.org/articles/10.3389/fchem.2021.685783/full#supplementary-material
References
Anzenbacher, P., Lubal, P., Buček, P., Palacios, M. A., and Kozelkova, M. E. (2010). A Practical Approach to Optical Cross-Reactive Sensor Arrays. Chem. Soc. Rev. 39, 3954–3979. doi:10.1039/B926220M
Buryak, A., and Severin, K. (2005). A Chemosensor Array for the Colorimetric Identification of 20 Natural Amino Acids. J. Am. Chem. Soc. 127, 3700–3701. doi:10.1021/ja042363v
Cao, Z., Cao, Y., Kubota, R., Sasaki, Y., Asano, K., Lyu, X., et al. (2020). Fluorescence Anion Chemosensor Array Based on Pyrenylboronic Acid. Front. Chem. 8, 414. doi:10.3389/fchem.2020.00414
Cebi, N., Dogan, C. E., Develioglu, A., Yayla, M. E. A., and Sagdic, O. (2017). Detection of L-Cysteine in Wheat Flour by Raman Microspectroscopy Combined Chemometrics of HCA and PCA. Food Chem. 228, 116–124. doi:10.1016/j.foodchem.2017.01.132
Chao, D., and Zhang, Y. (2017). Aggregation Enhanced Luminescent Detection of Homocysteine in Water with Terpyridine-Based Cu2+ Complexes. Sens. Actuators, B 245, 146–155. doi:10.1016/j.snb.2017.01.176
Chen, S.-H., and Li, C.-W. (2019). Detection and Characterization of Catechol Quinone-Derived Protein Adducts Using Biomolecular Mass Spectrometry. Front. Chem. 7, 571. doi:10.3389/fchem.2019.00571
Fracassetti, D., Lawrence, N., Tredoux, A. G. J., Tirelli, A., Nieuwoudt, H. H., and Du Toit, W. J. (2011). Quantification of Glutathione, Catechin and Caffeic Acid in Grape Juice and Wine by a Novel Ultra-performance Liquid Chromatography Method. Food Chem. 128, 1136–1142. doi:10.1016/j.foodchem.2011.04.001
Garcia, I., Romero, L. C., and Gotor, C. (2015). “Cysteine Homeostasis,” in Amino Acids in Higher Plants. Editor J. P. F. D'Mello (Wallingford: CABI), 219–233.
Ghasemi, F., Hormozi-Nezhad, M. R., and Mahmoudi, M. (2015). A Colorimetric Sensor Array for Detection and Discrimination of Biothiols Based on Aggregation of Gold Nanoparticles. Anal. Chim. Acta 882, 58–67. doi:10.1016/j.aca.2015.04.011
Gholami, M. D., Manzhos, S., Sonar, P., Ayoko, G. A., and Izake, E. L. (2019). Dual Chemosensor for the Rapid Detection of Mercury(II) Pollution and Biothiols. Analyst 144, 4908–4916. doi:10.1039/c9an01055f
Hamedpour, V., Sasaki, Y., Zhang, Z., Kubota, R., and Minami, T. (2019). Simple Colorimetric Chemosensor Array for Oxyanions: Quantitative Assay for Herbicide Glyphosate. Anal. Chem. 91, 13627–13632. doi:10.1021/acs.analchem.9b02822
Hargrove, A. E., Zhong, Z., Sessler, J. L., and Anslyn, E. V. (2010). Algorithms for the Determination of Binding Constants and Enantiomeric Excess in Complex Host : Guest Equilibria Using Optical Measurements. New J. Chem. 34, 348–354. doi:10.1039/B9NJ00498J
Hoey, L., Mcnulty, H., Askin, N., Dunne, A., Ward, M., Pentieva, K., et al. (2007). Effect of a Voluntary Food Fortification Policy on Folate, Related B Vitamin Status, and Homocysteine in Healthy Adults. Am. J. Clin. Nutr. 86, 1405–1413. doi:10.1093/ajcn/86.5.1405
Hyman, L. M., and Franz, K. J. (2012). Probing Oxidative Stress: Small Molecule Fluorescent Sensors of Metal Ions, Reactive Oxygen Species, and Thiols. Coord. Chem. Rev. 256, 2333–2356. doi:10.1016/j.ccr.2012.03.009
Jacxsens, L., Devlieghere, F., Ragaert, P., Vanneste, E., and Debevere, J. (2003). Relation between Microbiological Quality, Metabolite Production and Sensory Quality of Equilibrium Modified Atmosphere Packaged Fresh-Cut Produce. Int. J. Food Microbiol. 83, 263–280. doi:10.1016/s0168-1605(02)00376-8
Kaushik, R., Kumar, P., Ghosh, A., Gupta, N., Kaur, D., Arora, S., et al. (2015). Alizarin Red S-Zinc(II) Fluorescent Ensemble for Selective Detection of Hydrogen Sulphide and Assay with an H2S Donor. RSC Adv. 5, 79309–79316. doi:10.1039/C5RA11901D
Khorasani, M. Y., Langari, H., Sany, S. B. T., Rezayi, M., and Sahebkar, A. (2019). The Role of Curcumin and its Derivatives in Sensory Applications. Mater. Sci. Eng. C 103, 109792. doi:10.1016/j.msec.2019.109792
Krężel, A., and Maret, W. (2016). The Biological Inorganic Chemistry of Zinc Ions. Arch. Biochem. Biophys. 611, 3–19. doi:10.1016/j.abb.2016.04.010
Kurose, I., Higuchi, H., Miura, S., Saito, H., Watanabe, N., Hokari, R., et al. (1997). Oxidative Stress-Mediated Apoptosis of Hepatocytes Exposed to Acute Ethanol Intoxication. Hepatology 25, 368–378. doi:10.1002/hep.510250219
Küster, A., Tea, I., Sweeten, S., Rozé, J.-C., Robins, R. J., and Darmaun, D. (2008). Simultaneous Determination of Glutathione and Cysteine Concentrations and 2H Enrichments in Microvolumes of Neonatal Blood Using Gas Chromatography-Mass Spectrometry. Anal. Bioanal. Chem. 390, 1403–1412. doi:10.1007/s00216-007-1799-5
Lee, M. H., Kim, J. S., and Sessler, J. L. (2015). Small Molecule-Based Ratiometric Fluorescence Probes for Cations, Anions, and Biomolecules. Chem. Soc. Rev. 44, 4185–4191. doi:10.1039/C4CS00280F
Liu, L., Zhang, Q., Wang, J., Zhao, L., Liu, L., and Lu, Y. (2019). A Specific Fluorescent Probe for Fast Detection and Cellular Imaging of Cysteine Based on a Water-Soluble Conjugated Polymer Combined with Copper(II). Talanta 198, 128–136. doi:10.1016/j.talanta.2019.02.003
Lonchamp, J., Barry-Ryan, C., and Devereux, M. (2009). Identification of Volatile Quality Markers of Ready-To-Use Lettuce and Cabbage. Food Res. Int. 42, 1077–1086. doi:10.1016/j.foodres.2009.05.002
Luo, X., Masuda, T., Matsubara, K., Wada, Y., and Ikehata, A. (2021). The Relationship between Perceived Freshness and Water Content of Cabbage Leaves: A Near Infrared Imaging Survey of Substance Distribution Underlying Product Appearance. Lwt 139, 110523. doi:10.1016/j.lwt.2020.110523
Lyu, X., Hamedpour, V., Sasaki, Y., Zhang, Z., and Minami, T. (2021). 96-Well Microtiter Plate Made of Paper: A Printed Chemosensor Array for Quantitative Detection of Saccharides. Anal. Chem. 93, 1179–1184. doi:10.1021/acs.analchem.0c04291
Ma, Y., Zheng, B., Zhao, Y., Yuan, H., Cai, Y., Du, J., et al. (2013). A Sensitive and Selective Chemosensor for GSSG Detection Based on the Recovered Fluorescence of NDPA-Fe3O4@SiO2-Cu(II) Nanomaterial. Biosens. Bioelectron. 48, 138–144. doi:10.1016/j.bios.2013.04.006
Meng, Q., Jia, H., Succar, P., Zhao, L., Zhang, R., Duan, C., et al. (2015). A Highly Selective and Sensitive ON-OFF-ON Fluorescence Chemosensor for Cysteine Detection in Endoplasmic Reticulum. Biosens. Bioelectron. 74, 461–468. doi:10.1016/j.bios.2015.06.077
Miller, J., and Miller, J. C. (2018). Statistics and Chemometrics for Analytical Chemistry. (Upper Saddle River: Pearson education).
Minami, T., Esipenko, N. A., Zhang, B., Kozelkova, M. E., Isaacs, L., Nishiyabu, R., et al. (2012). Supramolecular Sensor for Cancer-Associated Nitrosamines. J. Am. Chem. Soc. 134, 20021–20024. doi:10.1021/ja3102192
Minich, D. M., and Brown, B. I. (2019). A Review of Dietary (Phyto)Nutrients for Glutathione Support. Nutrients 11, 2073. doi:10.3390/nu11092073
Moreira, A. R. S., Benedi, J., Gonzalez-Torres, L., Olivero-David, R., Bastida, S., Sanchez-Reus, M. I., et al. (2011). Effects of Diet Enriched with Restructured Meats, Containing Himanthalia Elongata, on Hypercholesterolaemic Induction, CYP7A1 Expression and Antioxidant Enzyme Activity and Expression in Growing Rats. Food Chem. 129, 1623–1630. doi:10.1016/j.foodchem.2011.06.019
Namuswe, F., and Berg, J. M. (2012). Secondary Interactions Involving Zinc-Bound Ligands: Roles in Structural Stabilization and Macromolecular Interactions. J. Inorg. Biochem. 111, 146–149. doi:10.1016/j.jinorgbio.2011.10.018
Nikolantonaki, M., Julien, P., Coelho, C., Roullier-Gall, C., Ballester, J., Schmitt-Kopplin, P., et al. (2018). Impact of Glutathione on Wines Oxidative Stability: A Combined Sensory and Metabolomic Study. Front. Chem. 6, 182. doi:10.3389/fchem.2018.00182
Reinbold, J., Rychlik, M., Asam, S., Wieser, H., and Koehler, P. (2008). Concentrations of Total Glutathione and Cysteine in Wheat Flour as Affected by Sulfur Deficiency and Correlation to Quality Parameters. J. Agric. Food Chem. 56, 6844–6850. doi:10.1021/jf800880n
Roy, P. (2021). Fluorescent Chemosensors Based on 4-Methyl-2,6-Diformylphenol. Coord. Chem. Rev. 427, 213562. doi:10.1016/j.ccr.2020.213562
Sasaki, Y., Kubota, R., and Minami, T. (2021a). Molecular Self-Assembled Chemosensors and Their Arrays. Coord. Chem. Rev. 429, 213607. doi:10.1016/j.ccr.2020.213607
Sasaki, Y., Lyu, X., Kubota, R., Takizawa, S.-Y., and Minami, T. (2021b). Easy-to-Prepare Mini-Chemosensor Array for Simultaneous Detection of Cysteine and Glutathione Derivatives. ACS Appl. Bio Mater. 4, 2113–2119. doi:10.1021/acsabm.0c01275
Sasaki, Y., Lyu, X., Zhou, Q., and Minami, T. (2021c). Indicator Displacement Assay-Based Chemosensor Arrays for Saccharides Using Off-The-Shelf Materials toward Simultaneous On-Site Detection on Paper. Chem. Lett. 50, 987–995. doi:10.1246/cl.200962
Sasaki, Y., Zhang, Z., and Minami, T. (2019). A Saccharide Chemosensor Array Developed Based on an Indicator Displacement Assay Using a Combination of Commercially Available Reagents. Front. Chem. 7, 49. doi:10.3389/fchem.2019.00049
Sener, G., Uzun, L., and Denizli, A. (2014). Colorimetric Sensor Array Based on Gold Nanoparticles and Amino Acids for Identification of Toxic Metal Ions in Water. ACS Appl. Mater. Inter. 6, 18395–18400. doi:10.1021/am5071283
Song, K., Kai, R., Lu, C., Gao, L., Wang, G., and Guo, L. (2016). Towards Two Up-Conversion Optical Sensing Systems for Cysteine Detection: Synthesis, Characterization and Sensing Performance. Sens. Actuators, B 236, 249–256. doi:10.1016/j.snb.2016.06.005
Valero, E., Millán, C., Ortega, J. M., and Mauricio, J. C. (2003). Concentration of Amino Acids in Wine after the End of Fermentation by Saccharomyces Cerevisiae strains. J. Sci. Food Agric. 83, 830–835. doi:10.1002/jsfa.1417
Wu, J., Kwon, B., Liu, W., Anslyn, E. V., Wang, P., and Kim, J. S. (2015). Chromogenic/Fluorogenic Ensemble Chemosensing Systems. Chem. Rev. 115, 7893–7943. doi:10.1021/cr500553d
Xu, W., Feng, H., Zhao, W., Huang, C., Redshaw, C., Tao, Z., et al. (2020). Amino Acid Recognition by a Fluorescent Chemosensor Based on Cucurbit[8]uril and Acridine Hydrochloride. Anal. Chim. Acta 1135, 142–149. doi:10.1016/j.aca.2020.09.028
Xu, Y., Niu, X., Zhang, H., Xu, L., Zhao, S., Chen, H., et al. (2015). Switch-on Fluorescence Sensing of Glutathione in Food Samples Based on a Graphitic Carbon Nitride Quantum Dot (g-CNQD)-Hg2+ Chemosensor. J. Agric. Food Chem. 63, 1747–1755. doi:10.1021/jf505759z
Yan, F., Fan, K., Bai, Z., Zhang, R., Zu, F., Xu, J., et al. (2017). Fluorescein Applications as Fluorescent Probes for the Detection of Analytes. Trends Analyt. Chem. 97, 15–35. doi:10.1016/j.trac.2017.08.013
Yang, X., Guo, Y., and Strongin, R. M. (2011). Conjugate Addition/Cyclization Sequence Enables Selective and Simultaneous Fluorescence Detection of Cysteine and Homocysteine. Angew. Chem. Int. Ed. 50, 10690–10693. doi:10.1002/anie.201103759
Yang, Z., Wang, X., Wu, Y., Li, X., and Chen, M. (2018). Two Emission Turn-On Optical Chemosensors for Cysteine Detection Using Up-Conversion Nanocrystals as Excitation Host: Synthesis, Characterization and Performance. Sens. Actuators, B 255, 1587–1594. doi:10.1016/j.snb.2017.08.182
Yin, C., Huo, F., Zhang, J., Martínez-Máñez, R., Yang, Y., Lv, H., et al. (2013). Thiol-addition Reactions and Their Applications in Thiol Recognition. Chem. Soc. Rev. 42, 6032–6059. doi:10.1039/c3cs60055f
Zhu, B., Li, L., Wei, H., Zhou, W., Zhou, W., Li, F., et al. (2020). A Simultaneously Quantitative Profiling Method for 40 Endogenous Amino Acids and Derivatives in Cell Lines Using Hydrophilic Interaction Liquid Chromatography Coupled with Tandem Mass Spectrometry. Talanta 207, 120256. doi:10.1016/j.talanta.2019.120256
Keywords: glutathione, cysteine, chemosensor array, food analysis, regression analysis, colorimetric sensing
Citation: Lyu X, Tang W, Sasaki Y, Zhao J, Zheng T, Tian Y and Minami T (2021) Toward Food Freshness Monitoring: Coordination Binding–Based Colorimetric Sensor Array for Sulfur-Containing Amino Acids. Front. Chem. 9:685783. doi: 10.3389/fchem.2021.685783
Received: 25 March 2021; Accepted: 31 May 2021;
Published: 17 June 2021.
Edited by:
Tony D. James, University of Bath, United KingdomCopyright © 2021 Lyu, Tang, Sasaki, Zhao, Zheng, Tian and Minami. This is an open-access article distributed under the terms of the Creative Commons Attribution License (CC BY). The use, distribution or reproduction in other forums is permitted, provided the original author(s) and the copyright owner(s) are credited and that the original publication in this journal is cited, in accordance with accepted academic practice. No use, distribution or reproduction is permitted which does not comply with these terms.
*Correspondence: Tsuyoshi Minami, tminami@iis.u-tokyo.ac.jp