Enhancement of microwave absorption performance of porous carbon induced by Ce (CO3) OH
- 1Chinese People’s Liberation Army, Beijing, China
- 2Institute of Defense Engineering, Academy of Military Sciences, Beijing, China
In recent years, electromagnetic pollution has become more and more serious, resulting in a very negative impact on people’s health. Therefore, it is important to develop efficient microwave absorbers to reduce electromagnetic pollution. Here, we construct a novel absorbing material of the polymer gel-derived porous carbon decorated by rare earth compounds (Ce (CO3) OH). When the thickness is 2.2 mm, the composite exhibits excellent microwave absorption performance with the optimal RLmin value and EAB reached up to −47.67 dB and 5.52 GHz, respectively, covering the Ku band. The high-efficiency microwave absorption is mainly attributed to the synergistic effect of dipole polarization, defect polarization and interfacial polarization. This work not only provides a new view for designing superior absorber materials, but also lay a foundation for their real applications.
1 Introduction
With the widespread use of electronic equipment in our daily lives, microwave absorbers have become totally essential to protect human health from serious electromagnetic pollution and improve the apparatus’ communication signal quality (Xu et al., 2019; Zhang et al., 2020; Wang et al., 2021; Nan et al., 2022). So far, much effort have been devoted into the development of target absorbers and the core requirements were identified as light weight, strong absorption, thin layer and broad bandwidth (Phang et al., 2008; Wan et al., 2015; Mo et al., 2019). To achieve these goals, various nano-structural materials have been reported, including magnetic metal oxides (Liu et al., 2013; Zhou et al., 2015), MXene (Han et al., 2017; Huang et al., 2022a), metal alloy (Lutsev and Shutkevich, 2016; Xu et al., 2018; Huang et al., 2022b) and carbonaceous substances (Wu et al., 2021; Wu et al., 2022), etc. Among them, carbon-based nanomaterials derived from gel structure attracted much attention as a result of low density, abundant porosity and adjustable dielectric property (Wang et al., 2022a). By using agar gel containing NiCO3 as precursors, Guo et al. synthesized a Ni/C composite consisting of three-dimensional carbon structure with accommodated nickel nanoparticles, which showed an excellent absorbing capability with a broad bandwidth in the range of 13.2 GHz–18 GHz. (Xie et al., 2018). Sun et al. proposed different magnetic metals and porous carbon composite structure on the basis of sol-gel method and subsequent in situ pyrolysis process. The prepared composites provided multiple loss pathway of electromagnetic wave and the minimum value of reflection loss was −33.5 dB at 7.7 GHz (Shen et al., 2022). Combining a typical sol-gel approach with supercritical drying and carbonization process, Yang et al. reported a resorcinol-formaldehyde based carbon aerogel with pore structure and good impedance matching. The wide effective bandwidth was estimated to be 4.5 GHz and minimum reflection loss value was determined to be −37.5 dB (Wang et al., 2022b). It follows that further improvement of absorbing performance from gel-derived carbonaceous materials is still limited by the narrow effective bandwidth, which may hinder their practical applications.
In recent, optimization of impedance matching has gradually been proved to expand the absorption bandwidth, and then increase electromagnetic wave attenuation performance (Rehman et al., 2019; Qiao et al., 2020; Jin et al., 2022). Several strategies have been proposed to regulate the dielectric properties of carbon materials, realizing the broadband and strong absorption (Wang et al., 2022c; He et al., 2022; Xu et al., 2022). For example, to reach a minimum reflection loss of −61.2 dB and an effective absorption bandwidth covering 5.2 GHz, Zhu et al. constructed a tubular structured carbon nanofibers coated with titanium dioxide layers, which showed the wave-transparent behavior to change the complex permittivity of carbonaceous constituent and facilitate the composites’ impedance matching (Kang et al., 2020). Similarly, Che et al. optimized the conductivity by anchoring the carbon nanotubes on TiO2 nanospheres or designing a multiple structure of vesicle-like-shell TiO2@carbon via the pyrolyzing the bimetallic zeolitic imidazolate framework encapsulating TiO2 nanoparticles or metal-polydopamine-coated TiO2, respectively. The obtained absorption performance presented a reflection loss of −44.0 dB and an effective absorption bandwidth of 5.4 GHz at the thickness of 2.0 mm (Ding et al., 2020). To improve its poor impedance-matching characteristic, Zhang et al. modified the ordered mesoporous carbon with silica (OMC-5@SiO2) through a self-assemble method and heat treatment process. With the impedance ratio around 1 from 8.56 GHz to 13.2 GHz, the OMC-5@SiO2 reached an effective absorption bandwidth of 4.8 GHz and the minimum reflection value of −40.7 dB at 10.8 GHz (Zhou et al., 2020). Nevertheless, the reported strategies are still limited, and new approaches are expected.
In this work, a new composite consisting of the polymer gel-derived porous carbon decorated by rare earth compounds [Ce (CO3) OH] was designed and synthesized. Compared to pure carbon materials, the prepared composites [Ce (CO3) OH/C] presented the significant higher pyrrolic N and 1.02 ID/IG value. The electromagnetic parameters analysis showed that the modified composites’ conductivity occurred downshift with the filling ratio of 10% and the thickness of 1.85 mm. For the electromagnetic wave absorption, by changing the absorbers’ thickness to 2.2 mm, the optimal RLmin value and EAB reached up to −47.67 dB and 5.52 GHz, respectively, covering the Ku band. It is believed that the results reported in this work will not only provide a new thought for controlling the impedance matching for carbon-based materials, but also lay a foundation for their real applications.
2 Experimental section
2.1 Materials
The P123 (PEO-PPO-PEO) material was purchased from sigma-Aldrich. The hexamethylenetetetramine (C6H12N4) and hydrochloric acid (HCl) were bought from Sinopharm Group. The 2,4-dihydroxybenzoic acid (C7H6O4) and cerium nitrate hexahydrate (Ce (NO3)2·6H2O) were purchased from Aladdin Reagent. The ethylenediamine was bought from General Reagent. All chemicals were utilized without further purification.
2.2 Preparation of polymer gel
Firstly, 3.56 g P123 was dissolved in 60 ml deionized water and sonicated for 30 min. Then, 0.936 g hexamethylenetetetramine, 3.115 g 2,4-dihydroxybenzoic acid and 415 μl ethylenediamine were added into the above solution, sonicated and dispersed, and transferred to a 100 Teflon-lined stainless autoclave and heatd at 130°C for 4 h. Finally, orange-red product was filtered and washed, and dried in a freeze dryer for 24 h.
2.3 Preparation of carbon materials
First, 1 g of polymer gel and 4 g of calcium carbonate nanoparticles were well ground and calcined in a tube furnace under nitrogen atmosphere at 900°C for 2 h. Then, the calcination product was immersed in 40 ml of 3 M HCl and stirred for 1 h to remove the generated calcium oxide. Finally, the product was filtered, washed with deionized water until neutral, and dried overnight at 70°C under vacuum.
2.4 Preparation of porous carbon loaded cerium nanomaterials (Ce/PC)
First, 100 mg of the above carbon materials and 200 mg of Ce (NO3)2·6H2O were added to 30 ml deionized water, sonicated for 2 h and stirred for 24 h, and then dried under vacuum for 24 h. Finally, the product was calcined at 1,000°C for 1 h under N2 with a heating rate of 5°C min−1. In addition, the blank sample PC was obtained by direct pyrolysis of a carbon material without adsorbed Ce.
2.5 Characterization and tests
The crystal structure was measured by X-ray diffraction (Bruker, D8 Advance). The micro-morphology and micro-structure were observed by scanning electron microscope (SEM) (Tescan MIRA3 LMU) and transmission electron microscopy (TEM) (JEOL JEM-2100F). Chemical composition was studied by X-ray photoelectron spectroscopy (XPS) on a K-Alpha 1063 X-ray photoelectron spectrometer. Raman tests were recorded on Renishaw Raman spectroscopy. The microwave absorption (MA) performance was investigated by a microwave vector network analyzer (VNA, Agilent N5230A).
3 Results and discussion
3.1 Structural and morphological analysis
The surface morphology and elemental composition of the prepared samples were analyzed by scanning electron microscopy (SEM). As shown in Figures 1(A–C), there is no significant difference in the micro-morphology between Ce/PC and PC samples, which are composed of irregular bulk particles. Ce grows in porous carbon to form Ce/PC sample, which is confirmed by EDS pattern of Ce/PC sample (Figure 1D). The TEM image (Figure 1E) show granular Ce growing on a porous carbon skeleton substrate. Additionally, the related HR-TEM (Figure 1F) image for Ce/PC shows clear lattice space of 0.403 nm, which is attributed to (1 2 2) plane of Ce (CO3) OH, further demonstrating the successful addition of Ce. The selected area electron diffraction (SAED) exhibits a ring-shape pattern corresponding to the Ce (CO3) OH.
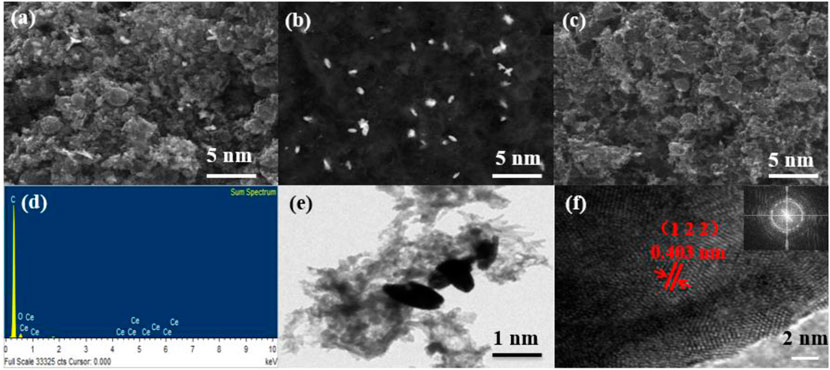
FIGURE 1. (A) Bright-field SEM picture for Ce/PC; (B) Dark field SEM picture for Ce/PC; (C) Bright-field SEM picture for PC; (D) EDS picture of Ce/PC; (E) TEM image of Ce/PC; (F) HR-TEM and SAED images of Ce/PC.
It can be seen from the X-ray powder diffraction (XRD) pattern in Figure 2A that both Ce/PC and PC samples have two broad diffraction peaks at 26.5°C and 43.3°C, which are attributed to (0 0 2) and (1 0 1) planes of carbon. The characteristic peaks of Ce/PC is the same as that of the standard comparison card PDF # 41-0013, which proves that Ce in Ce/PC sample exists in the form of Ce (CO3) OH. Normally, the degree of graphitization of a material can be characterized by Raman spectroscopy. From Figure 2B, both Ce and Ce/PC exhibit two peaks located at around 1,333 cm−1 (D band) and 1,587 cm−1 (G band) The D band signifies the sp3 defects or disorder, and G band indicates the sp2 hybridization. The ID/IG is usually used to reflect the degree of disorder (Shu et al., 2018a; Wu et al., 2019). It can be seen from Figure 3B that the ID/IG of the prepared material decreases slightly with the doping of Ce, which indicates that the degree of defect or disorder is reduced in Ce/PC sample.
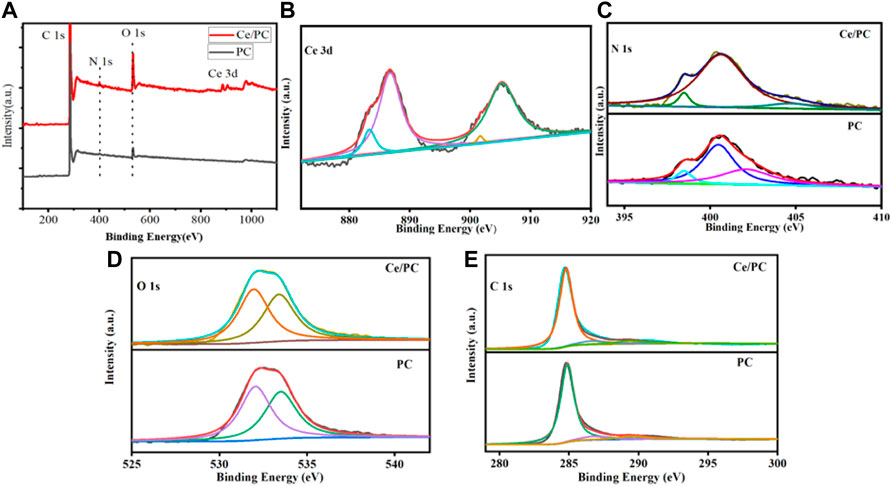
FIGURE 3. (A) XPS survey spectra for Ce/PC and PC; (B) Ce 3d XPS spectrum for Ce/PC; (C) N 1s XPS spectra, (D) O 1s XPS spectra and (E) C 1s XPS spectra for Ce/PC and PC.
The chemical composition of PC and Ce/PC sample was determined by X-ray photoelectron spectroscopy (XPS). The peaks of Cand Ce elements were found in the XPS survey spectrum of Ce/PC (Figure 3A), further confirming the presence of these elements in the Ce/PC sample. In Ce 3d spectra (Figure 3B), Ce/PC sample shows four characteristic peaks. The Ce 3d3/2 is marked as U and U′, and Ce 3d5/2 is marked as V and V′. The characteristic peaks U′ and V represent Ce3+, and the characteristic peaks U and V′ represent Ce4+ (Wu et al., 2020).Therefore, Ce4+ and Ce3+ coexist in Ce-PC, indicating the presence of oxygen vacancies. The electric conductivity increases with the increase of oxygen vacancy defects (Shu et al., 2018a; Wu et al., 2019). The enhanced electric conductivity is beneficial to enhancing the conduction loss and charge polarization relaxation of Ce-PC sample (Chen et al., 2020; Zhao et al., 2020). The N 1s spectra (Figure 3C) demonstrated the presence of pyridinic-N (398.4 eV), graphitic-N (400.5 eV) and oxide-N (402.1 eV,404.7 eV) in PC and Ce/PC samples (Yang et al., 2020). From Figure 3D, the O 1s spectra can be fitted into two peaks of -COOH (531.9 eV) and -OH (533.4 eV). (Soren et al., 2016). As shown in Figure 3E, the peaks of C 1s at 289.3 eV, 286.9 eV and 284.8 eV can be assigned to O-C=O, C-O and C=N bonds, respectively.
3.2 Microwave absorption properties
The microwave absorption properties of microwave absorbing materials can be evaluated by reflection loss (RL). According to the transmission line theory, the RL is calculated by the following formula (Wang et al., 2019a; Liu et al., 2019; Luo et al., 2020):
Herein Zin is the input impedance of absorber, Z0 is the impedance of free space,
As described in Figures 4A, 4A', the RLmin of PC samples is less than 10 dB, indicating that pure PC materials have weak microwave absorption properties. From Figures 4B, B', compared with PC samples, the RLmin of Ce/PC samples at 13.76 GHz is −47.67 dB, showing significantly improved microwave absorption properties, the corresponding thickness is 2.2 mm, and the effective absorption bandwidth (EAB, RL < −10 dB) can reach 5.52 GHz. In addition, more than 10 dB can be achieved by adjusting the matching thickness from 1 mm to 3 mm, covering almost the entire Ku and X-band.
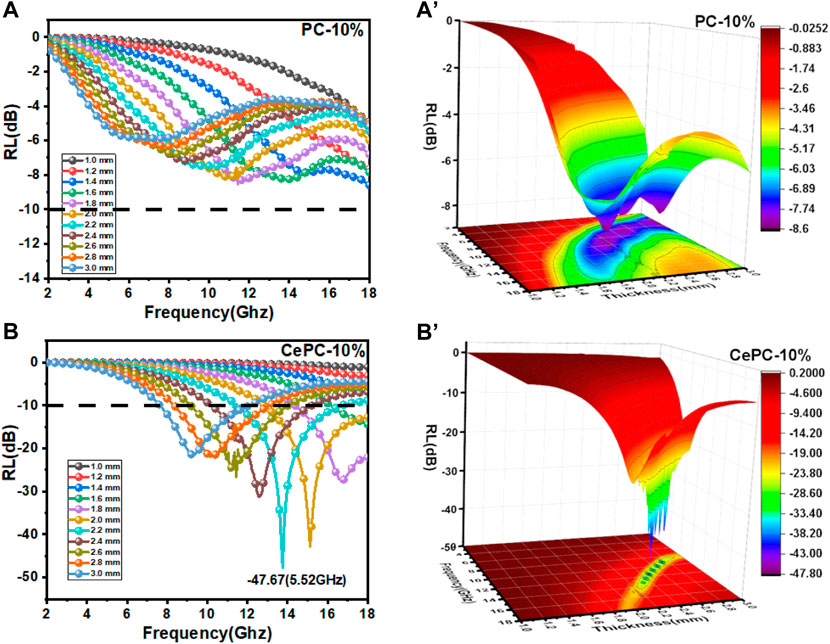
FIGURE 4. Frequency dependence of reflection loss with different thicknesses and 3D plots of reflection loss: (A) and (A') PC, (B) and (B') Ce/PC.
Generally, the electromagnetic parameters (
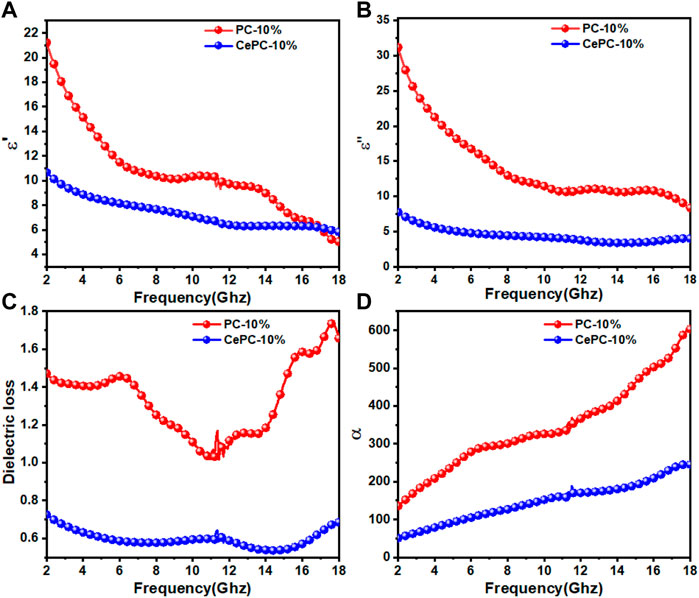
FIGURE 5. Electromagnetic parameters of PC and Ce/PC: (A) the real part and (B) the imaginary part of complex permittivity, (C) dielectric loss and (D) attenuation constant α.
The attenuation ability of electromagnetic wave is usually reflected by the attenuation constant (
Figure 5D shows the frequency dependence of the attenuation constant an of the prepared samples. As can be seen from Figure 5D, compared with PC materials, Ce/PC shows relatively weak electromagnetic wave attenuation ability. The change of attenuation capacity mainly comes from the change of dielectric loss. However, PC does not show the best microwave absorption performance, as shown in Figure 4. Therefore, the impedance matching characteristics need to be further considered.
As shown in Figure 6, the Z value of pure PC material is far from 1, which indicates that the impedance matching is poor. The Ce/PC material is closer to the optimal impedance matching line (Z = 1), which indicates that the impedance matching has been greatly improved. Due to the realization of the best impedance matching, most of the incident microwaves can enter the material (Liu et al., 2017a; Wang et al., 2019c). At the same time, the moderate electromagnetic attenuation ability can effectively convert electromagnetic energy into thermal energy (Shu et al., 2019a; Wang et al., 2019c; Zhang et al., 2019). Therefore, Ce/PC shows better microwave absorption properties.
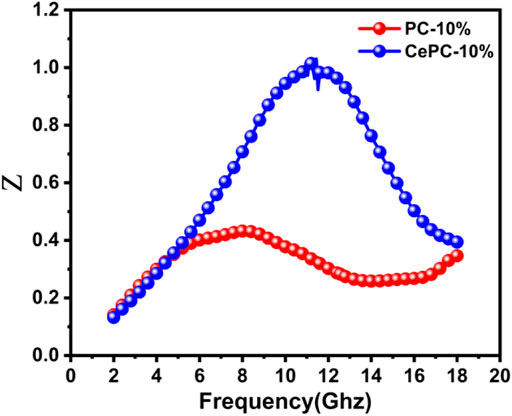
FIGURE 6. Frequency dependence of normalized impedance matching (Z) for the samples of PC and Ce/PC.
According to Debye theory, dielectric loss includes conduction loss and polarization loss (Liu et al., 2017b).
Herein,ԑs,
We analyzed the possible microwave absorption mechanism of Ce (CO3) OH/C nanocomposites. First of all, under the action of alternating electromagnetic field, the residual groups (- COOH and-OH) and structural defects on the surface or edge of porous carbon will cause dipole polarization and defect polarization (Suresh et al., 2013; Shu et al., 2018a), respectively. At the same time, Ce (CO3) OH nanoparticles can also be used as polarization centers to further enhance dipole polarization relaxation (Wu et al., 2019). Secondly, a large number of heterogeneous interfaces between paraffin matrix, porous carbon and Ce (CO3) OH can arrange polar bonds or charges under the action of alternating electromagnetic field to attenuate the power of incident microwave, like the same kind of capacitor structure (Cao et al., 2012). Third, according to Cao’s electron hopping model (Song et al., 2009; Cao et al., 2010), electrons can absorb electromagnetic energy and migrate on the porous carbon surface, and Ce (CO3) OH nanoparticles can be used as a bridge for electron hopping, thus improving the conductive loss, and further converting electromagnetic energy into thermal energy through collision with the lattice (Cao et al., 2018).
As shown in Table 1, we summarize the literature on Ce (CO3) OH/C composites as microwave absorbing materials and Ce as filling materials reported in this work (Wang et al., 2016; Xing et al., 2017; Ge et al., 2019). Obviously, among the reported Ce filled carbon matrix composites, the prepared Ce (CO3) OH/C nanocomposites have good microwave absorption properties, strong absorption and thin thickness.

TABLE 1. Typical Ce-based composites as microwave absorbers reported in this work and recent literatures.
4 Conclusion
In summary, a new composite material composed of porous carbon derived from polymer gel decorated with rare earth compound [Ce (CO3) OH] was successfully synthesized by one-pot hydrothermal method. The results show that compared with pure carbon materials, the prepared composites [Ce (CO3) OH/C] show significantly higher pyrroline N value, and the dispersion of the particles is better. In addition, the introduction of Ce (CO3) OH can improve the impedance matching of porous carbon materials, which has a significant effect on electromagnetic parameters and microwave absorption properties. When the filling ratio is 10 wt%, the prepared nanocomposites exhibit the best RL of −47.67 dB and EAB of 5.52 GHz at a thickness of only 2.2 mm. By adjusting the matching thickness from 1 mm to 3 mm, the matching thickness can be below 10 dB, covering almost the entire Ku and X-band. In addition, the possible microwave absorption mechanism of nanocomposites is proposed, which can be attributed to the synergistic effect of dipole polarization, defect polarization and interfacial polarization. In addition, the conductive loss is increased due to electron migration. Therefore, the prepared nanocomposites can be used as efficient absorbing materials in the field of electromagnetic wave absorption.
Data availability statement
The original contributions presented in the study are included in the article/Supplementary Material, further inquiries can be directed to the corresponding authors.
Author contributions
JW: Material preparation, electrochemical measurements, data analysis, writing-original draft. YC: tests. YW: measurements. YL: analysis. FL: analysis. BL: review. QW: review. JZ: Review.
Conflict of interest
The authors declare that the research was conducted in the absence of any commercial or financial relationships that could be construed as a potential conflict of interest.
Publisher’s note
All claims expressed in this article are solely those of the authors and do not necessarily represent those of their affiliated organizations, or those of the publisher, the editors and the reviewers. Any product that may be evaluated in this article, or claim that may be made by its manufacturer, is not guaranteed or endorsed by the publisher.
References
Cao, M., Wang, X., Cao, W., Fang, X., Wen, B., and Yuan, J. (2018). Thermally driven transport and relaxation switching self-powered electromagnetic energy conversion. Small 14, 1800987. doi:10.1002/smll.201800987
Cao, M-S., Song, W-L., Hou, Z-L., Wen, B., and Yuan, J. (2010). The effects of temperature and frequency on the dielectric properties, electromagnetic interference shielding and microwave-absorption of short carbon fiber/silica composites. Carbon 48, 788–796. doi:10.1016/j.carbon.2009.10.028
Cao, M-S., Yang, J., Song, W-L., Zhang, D. Q., Wen, B., Jin, H. B., et al. (2012). Ferroferric oxide/multiwalled carbon nanotube vs polyaniline/ferroferric oxide/multiwalled carbon nanotube multiheterostructures for highly effective microwave absorption. ACS Appl. Mat. Interfaces 4, 6949–6956. doi:10.1021/am3021069
Chen, W. S., Xue, J., Bao, Y. F., and Feng, L. (2020). Surface engineering of nano-ceria facet dependent coupling effect on Pt nanocrystals for electro-catalysis of methanol oxidation reaction. Chem. Eng. J. 381, 122752. doi:10.1016/j.cej.2019.122752
Ding, J. J., Wang, L., Zhao, Y. H., Yu, X., Xing, L., Ding, G., et al. (2020). Rutile TiO2 nanoparticles encapsulated in a zeolitic imidazolate framework-derived hierarchical carbon framework with engineered dielectricity as an excellent microwave absorber. ACS Appl. Mat. Interfaces 12, 48140–48149. doi:10.1021/acsami.0c12764
Ge, C., Wang, L., Liu, G., and Chen, H. (2019). Synthesis and electromagnetic absorption properties of CeO2@Fe composites with core-shell structure. J. Magnetism Magnetic Mater. 485, 228–235. doi:10.1016/j.jmmm.2019.04.087
Han, M. K., Yin, X. W., Li, X. L., Anasori, B., Zhang, L., Cheng, L., et al. (2017). Laminated and two-dimensional carbon-supported microwave absorbers derived from mxenes. ACS Appl. Mat. Interfaces 9, 20038–20045. doi:10.1021/acsami.7b04602
He, M., Liao, Q., Zhou, Y. M., Song, Z., Wang, Y., Feng, S., et al. (2022). Lightweight TiO2@C/carbon fiber aerogels prepared from ti3c2tx/cotton for high-efficiency microwave absorption. Langmuir 38, 945–956. doi:10.1021/acs.langmuir.1c02237
Huang, M. Q., Wang, L., Liu, Q., You, W., and Che, R. (2022). Interface compatibility engineering of multi-shell Fe@c@ TiO2@MoS2 heterojunction expanded microwave absorption bandwidth. Chem. Eng. J. 429, 132191. doi:10.1016/j.cej.2021.132191
Huang, Y. F., Xie, Y. L., Zhao, J., Yin, X., and Chai, C. (2022). Variety of zif-8/Mxene-based lightweight microwave-absorbing materials: Preparation and performances of zno/mxene nanocomposites. J. Phys. Chem. C 126, 13847–13853. doi:10.1021/acs.jpcc.2c04026
Jin, C., Wu, Z. C., Yang, C. D., Wang, L., Zhang, R., Xu, H., et al. (2022). Impedance amelioration of coaxial-electrospun TiO2@Fe/C@ TiO2 vesicular carbon microtubes with dielectric-magnetic synergy toward highly efficient microwave absorption. Chem. Eng. J. 433, 133640. doi:10.1016/j.cej.2021.133640
Kang, S., Qiao, S. Y., Cao, Y. T., Hu, Z., Yu, J., Wang, Y., et al. (2020). Hyper-cross-linked polymers-derived porous tubular carbon nanofibers@ TiO2 toward a wide-band and lightweight microwave absorbent at a low loading content. ACS Appl. Mat. Interfaces 12, 46455–46465. doi:10.1021/acsami.0c11839
Liu, J. W., Xu, J. J., Che, R. C., Chen, H., Liu, M., and Liu, Z. (2013). Hierarchical Fe3O4@TiO2 yolk-shell microspheres with enhanced microwave-absorption properties. Chem. Eur. J. 19, 6746–6752. doi:10.1002/chem.201203557
Liu, P., Zhang, Y., Yan, J., Huang, Y., Xia, L., and Guang, Z. (2019). Synthesis of lightweight n-doped graphene foams with open reticular structure for high-efficiency electromagnetic wave absorption. Chem. Eng. J. 368, 285–298. doi:10.1016/j.cej.2019.02.193
Liu, Q., Liu, X., Feng, H., Shui, H., and Yu, R. (2017). Metal organic framework-derived fe/carbon porous composite with low fe content for lightweight and highly efficient electromagnetic wave absorber. Chem. Eng. J. 314, 320–327. doi:10.1016/j.cej.2016.11.089
Liu, W., Shao, Q., Ji, G., Liang, X., Cheng, Y., Quan, B., et al. (2017). Metal–organic-frameworks derived porous carbon-wrapped ni composites with optimized impedance matching as excellent lightweight electromagnetic wave absorber. Chem. Eng. J. 313, 734–744. doi:10.1016/j.cej.2016.12.117
Luo, J., Zhang, K., Cheng, M., Gu, M., and Sun, X. (2020). MoS2 spheres decorated on hollow porous zno microspheres with strong wideband microwave absorption. Chem. Eng. J. 380, 122625. doi:10.1016/j.cej.2019.122625
Lutsev, L., and Shutkevich, V. (2016). Sharp increase of microwave absorption in nonequilibrium mnzn- and nizn-nanoferrites. J. Phys. D. Appl. Phys. 49, 505002. doi:10.1088/0022-3727/49/50/505002
Mo, Z. C., Yang, R. L., Lu, D. W., Yang, L., Hu, Q., Li, H., et al. (2019). Lightweight, three-dimensional carbon nanotube@ TiO2 sponge with enhanced microwave absorption performance. Carbon 144, 433–439. doi:10.1016/j.carbon.2018.12.064
Nan, H. Y., Luo, F., Jia, H. Y., Deng, H., Qing, Y., Huang, Z., et al. (2022). Balancing between polarization and conduction loss toward strong electromagnetic wave absorption of hard carbon particles with morphology heterogeneity. ACS Appl. Mat. Interfaces 14, 19836–19846. doi:10.1021/acsami.2c01171
Phang, S. W., Tadokoro, M., Watanabe, J., and Kuramoto, N. (2008). Synthesis, characterization and microwave absorption property of doped polyaniline nanocomposites containing TiO2 nanoparticles and carbon nanotubes. Synth. Met. 158, 251–258. doi:10.1016/j.synthmet.2008.01.012
Qiao, J., Zhang, X., Xu, D. M., Kong, L., Lv, L., Yang, F., et al. (2020). Design and synthesis of TiO2/Co/carbon nanofibers with tunable and efficient electromagnetic absorption. Chem. Eng. J. 380, 122591. doi:10.1016/j.cej.2019.122591
Quan, B., Liang, X., Xu, G., Cheng, Y., Zhang, Y., Liu, W., et al. (2017). A permittivity regulating strategy to achieve high-performance electromagnetic wave absorbers with compatibility of impedance matching and energy conservation. New J. Chem. 41, 1259–1266. doi:10.1039/c6nj03052a
Rehman, S. U., Liu, J., Fang, Z. B., Wang, J., Ahmed, R., Wang, C., et al. (2019). Heterostructured TiO2/C/Co from zif-67 frameworks for microwave-absorbing nanomaterials. ACS Appl. Nano Mat. 2, 4451–4461. doi:10.1021/acsanm.9b00841
Shen, Z. T., Zu, Y. P., Chen, Y. Q., Ma, S., Zhang, Z., Gong, J., et al. (2022). A novel synthesis method of magnetic porous carbon composites for microwave absorption. Synth. Met. 291, 117184. doi:10.1016/j.synthmet.2022.117184
Shu, R., Li, W., Wu, Y., Zhang, J., and Zhang, G. (2019). Nitrogen-doped Co-C/MWCNTs nanocomposites derived from bimetallic metal–organic frameworks for electromagnetic wave absorption in the x-band. Chem. Eng. J. 362, 513–524. doi:10.1016/j.cej.2019.01.090
Shu, R., Li, W., Wu, Y., Zhang, J., Zhang, G., and Zheng, M. (2019). Fabrication of nitrogen-doped cobalt oxide/cobalt/carbon nanocomposites derived from heterobimetallic zeolitic imidazolate frameworks with superior microwave absorption properties. Compos. Part B Eng. 178, 107518. doi:10.1016/j.compositesb.2019.107518
Shu, R., Li, W., Zhou, X., Tian, D., Zhang, G., Gan, Y., et al. (2018). Facile preparation and microwave absorption properties of RGO/MWCNTS/ZnFe2O4 hybrid nanocomposites. J. Alloys Compd. 743, 163–174. doi:10.1016/j.jallcom.2018.02.016
Shu, R., Wu, Y., Li, Z., Zhang, J., Wan, Z., Liu, Y., et al. (2019). Facile synthesis of cobalt-zinc ferrite microspheres decorated nitrogen-doped multi-walled carbon nanotubes hybrid composites with excellent microwave absorption in the x-band. Compos. Sci. Technol. 184, 107839. doi:10.1016/j.compscitech.2019.107839
Shu, R., Zhang, G., Wang, X., Gao, X., Wang, M., Gan, Y., et al. (2018). Fabrication of 3d net-like MWCNTS/ZnFe2O4 hybrid composites as high-performance electromagnetic wave absorbers. Chem. Eng. J. 337, 242–255. doi:10.1016/j.cej.2017.12.106
Shu, R., Zhang, G., Zhang, J., Wang, X., Wang, M., Gan, Y., et al. (2018). Fabrication of reduced graphene oxide/multi-walled carbon nanotubes/zinc ferrite hybrid composites as high-performance microwave absorbers. J. Alloys Compd. 736, 1–11. doi:10.1016/j.jallcom.2017.11.084
Song, W-L., Cao, M-S., Hou, Z-L., Fang, X. Y., Shi, X. L., and Yuan, J. (2009). High dielectric loss and its monotonic dependence of conducting-dominated multiwalled carbon nanotubes/silica nanocomposite on temperature ranging from 373 to 873 k in x-band. Appl. Phys. Lett. 94, 233110. doi:10.1063/1.3152764
Soren, S., Mohaptra, B. D., Mishra, S., Debnath, A. K., Aswal, D. K., Varadwaj, K. S. K., et al. (2016). Nano ceria supported nitrogen doped graphene as a highly stable and methanol tolerant electrocatalyst for oxygen reduction. RSC Adv. 6, 77100–77104. doi:10.1039/c6ra13218a
Suresh, R., Ponnuswamy, V., and Mariappan, R. (2013). Effect of annealing temperature on the microstructural, optical and electrical properties of CeO2 nanoparticles by chemical precipitation method. Appl. Surf. Sci. 273, 457–464. doi:10.1016/j.apsusc.2013.02.062
Wan, G. P., Yu, L., Peng, X. G., Wang, G., Huang, X., Zhao, H., et al. (2015). Preparation and microwave absorption properties of uniform tio2@c core-shell nanocrystals. RSC Adv. 5, 77443–77448. doi:10.1039/c5ra14344f
Wang, B. L., Fu, Y. G., Li, J., and Liu, T. (2022). Yolk-shelled Co@SiO2@mesoporous carbon microspheres: Construction of multiple heterogeneous interfaces for wide-bandwidth microwave absorption. J. Colloid Interface Sci. 607, 1540–1550. doi:10.1016/j.jcis.2021.09.028
Wang, J., Zhu, P., Wang, J., Ho, S. L., and Tan, J. (2016). Interchange core/shell assembly of diluted magnetic semiconductor CeO2 and ferromagnetic ferrite fe3o4 for microwave absorption. AIP Adv. 7, 055811. doi:10.1063/1.4973204
Wang, K. J., Ye, Z. W., Li, X. Q., and Yang, J. (2022). Nanoporous resorcinol-formaldehyde based carbon aerogel for lightweight and tunable microwave absorption. Mater. Chem. Phys. 278, 125718. doi:10.1016/j.matchemphys.2022.125718
Wang, L., Bai, X., Wen, B., Du, Z., and Lin, Y. (2019). Honeycomb-like Co/c composites derived from hierarchically nanoporous zif-67 as a lightweight and highly efficient microwave absorber. Compos. Part B Eng. 166, 464–471. doi:10.1016/j.compositesb.2019.02.054
Wang, T., Chen, G., Zhu, J. H., Gong, H., Zhang, L., and Wu, H. (2021). Deep understanding of impedance matching and quarter wavelength theory in electromagnetic wave absorption. J. Colloid Interface Sci. 595, 1–5. doi:10.1016/j.jcis.2021.03.132
Wang, Y., Gao, X., Lin, C., Shi, L., and Wu, G. (2019). Metal organic frameworks-derived Fe-Co nanoporous carbon/graphene composite as a high-performance electromagnetic wave absorber. J. Alloys Compd. 785, 765–773. doi:10.1016/j.jallcom.2019.01.271
Wang, Y., Gao, X., Wu, X., Zhang, W., Luo, C., and Liu, P. (2019). Facile design of 3d hierarchical NiFe2O4/N-GN/ZnO composite as a high performance electromagnetic wave absorber. Chem. Eng. J. 375, 121942. doi:10.1016/j.cej.2019.121942
Wang, Y. Y., Zhu, J. L., Li, N., Shi, J. F., Tang, J. H., Yan, D. X., et al. (2022). Carbon aerogel microspheres with in-situ mineralized TiO2 for efficient microwave absorption. Nano Res. 15, 7723–7730. doi:10.1007/s12274-022-4494-0
Wu, L. H., Liu, X., Wan, G. P., Peng, X., He, Z, Shi, S., et al. (2022). Ni/CNTS and carbon coating engineering to synergistically optimize the interfacial behaviors of tio 2 for thermal conductive microwave absorbers. Chem. Eng. J. 448, 137600. doi:10.1016/j.cej.2022.137600
Wu, W. T., Xu, R., Zhou, Y. M., He, M., Lu, P., Wang, R., et al. (2021). Biomimetic 3d coral reef-like GO@TiO2 composite framework inlaid with TiO2-C for low-frequency electromagnetic wave absorption. Carbon 178, 144–156. doi:10.1016/j.carbon.2020.11.085
Wu, Y., Shu, R., Shan, X., Zhang, J., Shi, J., Liu, Y., et al. (2020). Facile design of cubic-like cerium oxide nanoparticles decorated reduced graphene oxide with enhanced microwave absorption properties. J. Alloys Compd. 817, 152766. doi:10.1016/j.jallcom.2019.152766
Wu, Y., Shu, R., Zhang, J., Sun, R., Chen, Y., and Yuan, J. (2019). Oxygen vacancy defects enhanced electromagnetic wave absorption properties of 3d net-like multi-walled carbon nanotubes/cerium oxide nanocomposites. J. Alloys Compd. 785, 616–626. doi:10.1016/j.jallcom.2019.01.227
Xie, P. T., Li, H. Y., He, B., Dang, F., Lin, J., Fan, R., et al. (2018). Bio- gel derived nickel/carbon nanocomposites with enhanced microwave absorption. J. Mat. Chem. C Mat. 6, 8812–8822. doi:10.1039/c8tc02127a
Xing, H., Yin, Q., Liu, Z., and Wang, L. (2017). Excellent microwave absorption behaviors of polyaniline composites containing CeO2 nanorods in the x-band. Nano 12, 1750047. doi:10.1142/s1793292017500473
Xu, J. L., Qi, X. S., Sun, Y., Wang, Z., Liu, Y., Luo, C., et al. (2018). Tuning the electromagnetic synergistic effects for enhanced microwave absorption via magnetic nickel core encapsulated in hydrogenated anatase TiO2 shell. ACS Sustain. Chem. Eng. 6, 12046–12054. doi:10.1021/acssuschemeng.8b02350
Xu, J. L., Sun, L., Qi, X. S., Wang, Z., Fu, Q., and Pan, C. (2019). A novel strategy to enhance the multiple interface effect using amorphous carbon packaged hydrogenated tio2 for stable and effective microwave absorption. J. Mat. Chem. C Mat. 7, 6152–6160. doi:10.1039/c9tc00483a
Xu, L. L., Tao, J. Q., Zhang, X. F., Yao, Z., Zavabeti, A., and Zhou, J. (2022). Co@N-doped double-shell hollow carbon via self-templating-polymerization strategy for microwave absorption. Carbon 188, 34–44. doi:10.1016/j.carbon.2021.11.043
Yang, X., Sun, X., Rauf, M., Mi, H., Sun, L., Deng, L., et al. (2020). N-doped porous tremella-like Fe3C/Celectrocatalysts derived from metal-organic frameworks for oxygen reduction reaction. Dalton Trans. 49, 797–807. doi:10.1039/c9dt03923f
Zhang, X., Wang, J., Su, X., and Huo, S. (2019). Facile synthesis of reduced graphene oxide-wrapped cnfs with controllable chemical reduction degree for enhanced microwave absorption performance. J. Colloid Interface Sci. 553, 402–408. doi:10.1016/j.jcis.2019.06.055
Zhang, Z., Tan, J. W., Gu, W. H., Zhao, H., Zheng, J., Zhang, B., et al. (2020). Cellulose-chitosan framework/polyailine hybrid aerogel toward thermal insulation and microwave absorbing application. Chem. Eng. J. 395, 125190. doi:10.1016/j.cej.2020.125190
Zhao, S. Z., Kang, D. J., Liu, Y. P., Wen, Y., Xie, X., Yi, H., et al. (2020). Spontaneous formation of asymmetric oxygen vacancies in transition-metal-doped CeO2 nanorods with improved activity for carbonyl sulfide hydrolysis. ACS Catal. 10, 11739–11750. doi:10.1021/acscatal.0c02832
Zhou, M., Lu, F., Lv, T. Y., Yang, X., Xia, W., Shen, X., et al. (2015). Loss mechanism and microwave absorption properties of hierarchical nico2o4 nanomaterial. J. Phys. D. Appl. Phys. 48, 215305. doi:10.1088/0022-3727/48/21/215305
Keywords: microwave absorbers, impedance matching, rare earth compounds (Ce (CO3) OH), Ce (CO3) OH/C composite, synergistic effect
Citation: Wang J, Chen Y, Wei Y, Li Y, Li F, Li B, Wu Q and Zhao J (2023) Enhancement of microwave absorption performance of porous carbon induced by Ce (CO3) OH. Front. Chem. 10:1100111. doi: 10.3389/fchem.2022.1100111
Received: 16 November 2022; Accepted: 22 November 2022;
Published: 09 January 2023.
Edited by:
Xiaohui Gao, Central South University, ChinaReviewed by:
Yuhui Peng, Nanchang Hangkong University, ChinaJun He, Hunan Institute of Engineering, China
Copyright © 2023 Wang, Chen, Wei, Li, Li, Li, Wu and Zhao. This is an open-access article distributed under the terms of the Creative Commons Attribution License (CC BY). The use, distribution or reproduction in other forums is permitted, provided the original author(s) and the copyright owner(s) are credited and that the original publication in this journal is cited, in accordance with accepted academic practice. No use, distribution or reproduction is permitted which does not comply with these terms.
*Correspondence: Bingzhen Li, tylerlibz@hotmail.com; Qingqing Wu, 164362808@qq.com; Jinlong Zhao, zjl767688612@163.com