Natural products, including a new caboxamycin, from Streptomyces and other Actinobacteria isolated in Spain from storm clouds transported by Northern winds of Arctic origin
- 1Departamento de Biología Funcional Área de Microbiología Universidad de Oviedo, Oviedo, Spain
- 2Instituto Universitario de Oncología del Principado de Asturias, Universidad de Oviedo, Oviedo, Spain
- 3Instituto de Investigación Sanitaria del Principado de Asturias (ISPA), Universidad de Oviedo, Oviedo, Spain
- 4Fundación MEDINA Centro de Excelencia en Investigación de Medicamentos Innovadores en Andalucía, Granada, Spain
- 5Departamento de Ingeniería Química y Tecnología del Medio Ambiente Área de Ingeniería Química Universidad de Oviedo, Oviedo, Spain
Actinobacteria, mostly Streptomyces species, are the main source of natural products essential in medicine. While the majority of producer microorganisms of secondary metabolite are reported from terrestrial or marine environments, there are limited reports of their isolation from atmospheric precipitations. Clouds are considered as atmospheric oases for microorganisms and there is a recent paradigm shift whereby atmospheric-derived Actinobacteria emerge as an alternative source for drug discovery. In this context, we studied a total of 18 bioactive Actinobacteria strains, isolated by sampling nine precipitation events with prevailing Northern winds in the Cantabrian Sea coast, Northern Spain. Backward trajectories meteorological analyses indicate that air masses were originated mostly in the Arctic Ocean, and their trajectory to downwind areas involved the Atlantic Ocean and also terrestrial sources from continental Europe, and in some events from Canada, Greenland, Mauritania and Canary Islands. Taxonomic identification of the isolates, by 16S rRNA gene sequencing and phylogenetic analyses, revealed that they are members of three Actinobacteria genera. Fifteen of the isolates are Streptomyces species, thus increasing the number of bioactive species of this genus in the atmosphere to a 6.8% of the total currently validated species. In addition, two of the strains belong to the genus Micromonospora and one to genus Nocardiopsis. These findings reinforce a previous atmospheric dispersal model, extended herein to the genus Micromonospora. Production of bioactive secondary metabolites was screened in ethyl acetate extracts of the strains by LC-UV-MS and a total of 94 secondary metabolites were detected after LC/MS dereplication. Comparative analyses with natural products databases allowed the identification of 69 structurally diverse natural products with contrasted biological activities, mostly as antibiotics and antitumor agents, but also anti-inflammatory, antiviral, antiparasitic, immunosuppressant and neuroprotective among others. The molecular formulae of the 25 remaining compounds were determined by HRMS. None of these molecules had been previously reported in natural product databases indicating potentially novel metabolites. As a proof of concept, a new metabolite caboxamycin B (1) was isolated from the culture broth of Streptomyces sp. A-177 and its structure was determined by various spectrometric methods. To the best of our knowledge, this is the first novel natural product obtained from an atmospheric Streptomyces, thus pointing out precipitations as an innovative source for discovering new pharmaceutical natural products.
Introduction
Members of the Phylum Actinobacteria, also known as actinomycetes, are major producers of secondary metabolites of medical and biotechnological use, being the Streptomyces genus the most prolific source of natural products of pharmaceutical interest. At present, there is an urgent need of new secondary metabolites, essentially antibiotics to combat pathogenic resistant bacteria, but also compounds with applications as anticancer agents, antivirals, antiparasitics and immunosuppresants, among others (Ramirez-Rendon et al., 2022).
New trends in natural products discovery are now focused in the search for novel producers in the less explored environments of our planet, including deep-sea habitats and the atmosphere. Regarding the biogeography of the Streptomyces genus, it is known that they are ubiquitous since they are widespread not only in terrestrial habitats, as was established in the last century, but also in oceanic and atmospheric environments (Braña et al., 2015; Sarmiento-Vizcaíno et al., 2017a). An atmospheric dispersal model, coupled with the main Earth hydrological cycle, was proposed to explain the biogeography of Streptomyces species (Sarmiento-Vizcaíno et al., 2016).
Highly diverse bioactive Streptomyces and Nocardiopsis species were isolated from multiple precipitation events happened in the Cantabrian Sea Coast, Northern Spain, with prevalent Western and North Western winds (Sarmiento-Vizcaíno et al., 2021, 2018). It has been suggested that during different precipitation events and by changing the latitude of the sampling place, it is possible to isolate a great diversity of Actinobacteria producing a remarkable reservoir of natural products with relevant biological activities, thus revealing the pharmaceutical and biotechnological potential of the atmosphere as a relevant source for natural products discovery (Sarmiento-Vizcaíno et al., 2021).
As a result of a multidisciplinary approach, we explored here the phylogenetic and biosynthetic diversity of Actinobacteria obtained in the Cantabrian Sea Cost, Spain, over 3 years’ time in precipitation events with Northern winds, the still remaining unexplored source of precipitations in this geographical region. This culture-dependent approach involved taxonomical and phylogenetic analyses as well as meteorological analyses. Antimicrobial assays, metabolic profiling by LC-UV-MS, followed by identification by comparison to natural products databases, purification and structural elucidation, were used to uncover the biosynthetic diversity of these atmospheric-derived Actinobacteria.
Materials and methods
Sampling of atmospheric precipitations
Rainwater, hailstone and snow samples were collected within the years 2013–2016 in Northern Spain, at the Asturias Cantabrian Sea coastal region, a very wet and rainy region whose climate is influenced by the Atlantic Ocean (Figure 1). Samples of 2–3 ml were taken in sterile recipients mainly at the coastal locality of Gijón (43° 32′ N, 5° 39′ W), but also in Oviedo (43° 21′ N, 5° 52′ W), and plated on selective media as has been described (Braña et al., 2015; Sarmiento-Vizcaíno et al., 2016). During most of the precipitation events sampled the prevailing wind direction was Northern.
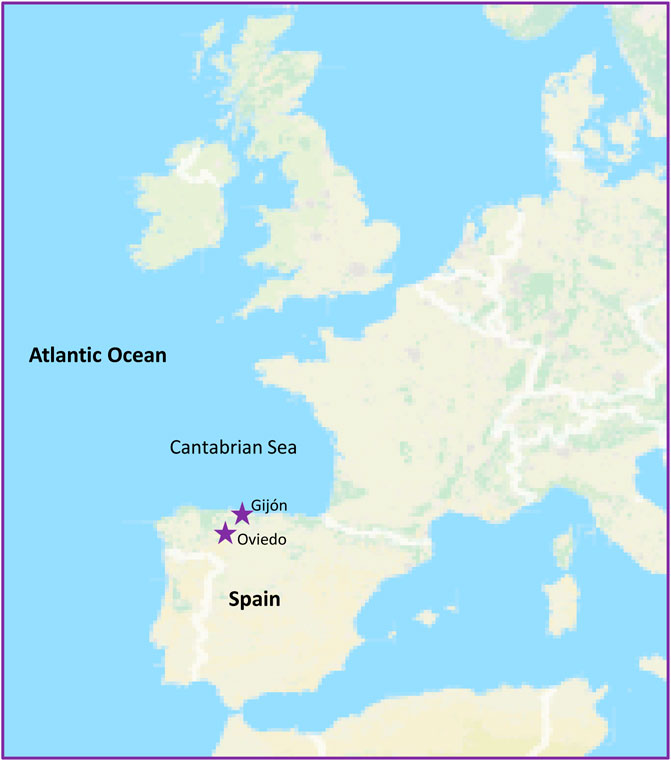
FIGURE 1. Sampling locations. Overview of the Western Atlantic Ocean and the sampling locations in Northern Spain indicated by stars.
Actinobacteria strains isolation and culture
Actinobacteria strains were isolated after plating of atmospheric samples on selective agar media, prepared with cycloheximide (80 μg mL–1) as antifungal and nalidixic acid (20 μg mL–1) as anti-Gram negative bacteria, using MOPS BLEB 1/6 (Oxoid) basal medium as previously reported (Sarmiento-Vizcaíno et al., 2016). The selection plates were prepared either with distilled water or with a supplement of 3.5% NaCl. Colonies were selected based on different morphological features and pigment production on R5A agar plates after incubation for 2–3 weeks at 28 °C. Pure cultures were conserved in 20% glycerol at −20°. MOPS BLEB 1/6 was also used as the basal medium for halotolerance studies, adding NaCl at 0, 3.5, 7.0, and 10.5% (w/v) final concentrations. For secondary metabolite production R5A medium was used as previously described (Sarmiento-Vizcaíno et al., 2018).
Air mass backward trajectories analyses
Backward trajectories of the air masses were generated using the HYSPLIT model (Hybrid Single Particle Lagrangian Integrated Trajectory) from the Global Data Assimilation System of National Oceanic and Atmospheric Administration, USA (Stein et al., 2015) to estimate the long-range transport journey of air masses that originated the precipitation events herein studied. Five-day backward trajectories were obtained using the NOAA model (http://ready.arl.noaa.gov/hypub-bin/trajtype.pl?runtype=archive) to track the transport pathways of air masses and determine the origin of diverse air parcels. Sampling locations were used as the backward trajectory start point with altitudes over the sea level of 30, 1,000 and 3,000 m (Gijón), and 300, 1,000 and 3,000 m (Oviedo) as previously reported (Sarmiento-Vizcaíno et al., 2021).
Antimicrobial bioassays
To determine the antimicrobial activities of isolates, agar diffusion methods were used against a panel of the following indicator microorganisms: the Gram-positive bacteria Micrococcus luteus ATCC 14452 and Streptomyces 85E ATCC 55824, the Gram-negative Escherichia coli ESS, and the yeast Saccharomyces cerevisiae var. Carlsbergensis. Analyses were performed in TSA1/2 (Merck) against bacteria and in Sabouraud 1/2 (Pronadisa) against yeast, and bioassays were carried out both with agar plugs (7 mm diameter) and also with 6-mm-diameter AA Discs (Whatman), loaded with ethyl acetate extracts of bioactive isolates, as previously reported (Sarmiento-Vizcaíno et al., 2021). Agar plugs assays detect all diffusible compounds produced by actinobacterial strains, both polar and apolar, whereas the AA discs bioassays only detect diffusible apolar molecules extracted with ethyl acetate.
16S RNA analysis identification and phylogenetic analysis
DNA was extracted with a microbial isolation kit (Ultra Clean, MoBio Laboratories, Inc.) for taxonomic identification of the strains, using standard methods for checking the purity (Russell and Sambrook, 2001). Partial 16S rRNA gene sequences of the bacterial strains were obtained by PCR amplification using the 616V (forward) and 699R (reverse) primers (Arahal et al., 2008) (Braña et al., 2015), using the BLAST program (Basic Local Alignment Search Tool) against the NCBI (National Centre for Biotechnology Information). The nucleotide sequences were compared to sequences in databases, submitted and deposited in the EMBL database with accession numbers OL587568-OL587585. Phylogenetic analysis of the strains based on 16S rRNA gene sequences was performed as previously reported (Sarmiento-Vizcaíno et al., 2021).
Chromatographic analysis
Plugs of R5A plates (about 7 ml) were extracted using ethyl acetate in neutral and acidic (with 1% formic acid) conditions. After evaporation, the organic fraction residue was redissolved in 100 µL of a mixture of DMSO and methanol (50:50). The analyses of the samples were performed by reversed phase liquid chromatography as previously described (Braña et al., 2015; Sarmiento-Vizcaíno et al., 2016).
Identification of compounds by LC-UV-Vis and LC-UV-HRMS analyses
Samples were first analyzed and evaluated using an in-house HPLC-UV-Vis database. LC-UV-HRMS analyses were carried out as has been described (Pérez-Victoria et al., 2016; Sarmiento Vizcaíno et al., 2018) and major peaks in each chromatogram were searched against the MEDINA’s internal database and also against the Dictionary of Natural Products (DNP) (Chapman and Hall, 2015).
Purification of novel compounds
For purification of the secondary metabolites produced, Streptomyces sp. A-177 was cultured in 40 Erlenmeyer flasks (250 ml), each containing 50 ml of R5A medium supplemented with 3%. DMSO inoculated with spores and incubated in an orbital shaker at 28°C and 250 rpm during 7 days. The cultures were centrifuged and extracted with ethyl acetate acidified with 1% formic acid. The extracts were dried and the residue was subsequently redissolved in a small volume of acetonitrile and DMSO (1:1). The same peaks were also found in the organic extract of the culture pellets, which were dried and redissolved in the same way. The desired compounds were purified by preparative HPLC using a SunFire C18 column (10 μm, 10 × 250 mm, Waters). The purification was performed in two steps. The mobile phase was a mixture of 90% acetonitrile and TFA 0.1% in the first step and 80% acetonitrile and TFA 0.1% in the second one, in isocratic conditions at 5 ml/min. In both cases, the solutions containing the collected peaks were evaporated in rotavapor and finally lyophilized, resulting in 3.9 mg of caboxamycin B (1).
LC-HRMS and NMR analyses
HRMS spectra (ESI-TOF) were acquired using a Bruker maXis QTOF mass spectrometer coupled to an Agilent 1,200 Rapid Resolution HPLC. NMR spectra were recorded at 297 K on a Bruker Avance III spectrometer (500 and 125 MHz for 1H and 13C, respectively) equipped with a 1.7 mm TCI MicroCryoProbeTM. 1H and 13C chemical shifts were reported in ppm using the signals of the residual solvent as internal reference (δH 2.50 and δC 39.5 ppm for DMSO-d6).
Results
Selection of bioactive Actinobacteria from precipitation events in Northern Spain
The strains studied herein were isolated and characterized in Spain by sampling multiple precipitation events, as previously reported (Sarmiento-Vizcaíno et al., 2021). After a dereplication process, involving antimicrobial activity, phenotypical features, metabolic profiling and meteorological analyses, 18 morphologically different bioactive strains, isolated in precipitations events with prevalent Northern winds, were here selected. To analyze antibiotic production, the isolates were initially screened for antimicrobial activity against bacteria and fungi as indicator microorganisms (Table 1), using agar diffusion methods as recently reported (Sarmiento-Vizcaíno et al., 2021). Table 1A shows the results of bioassays with agar plugs and Table 1B displays the results of ethyl acetate extracts using agar diffusion with AA discs. Most of the strains display strong antibiotic activities against Gram-positive bacteria, such as M. luteus and Streptomyces 85E, whereas 10 strains were active against the Gram-negative E. coli ESS and seven strains against the yeast S. cerevisiae.
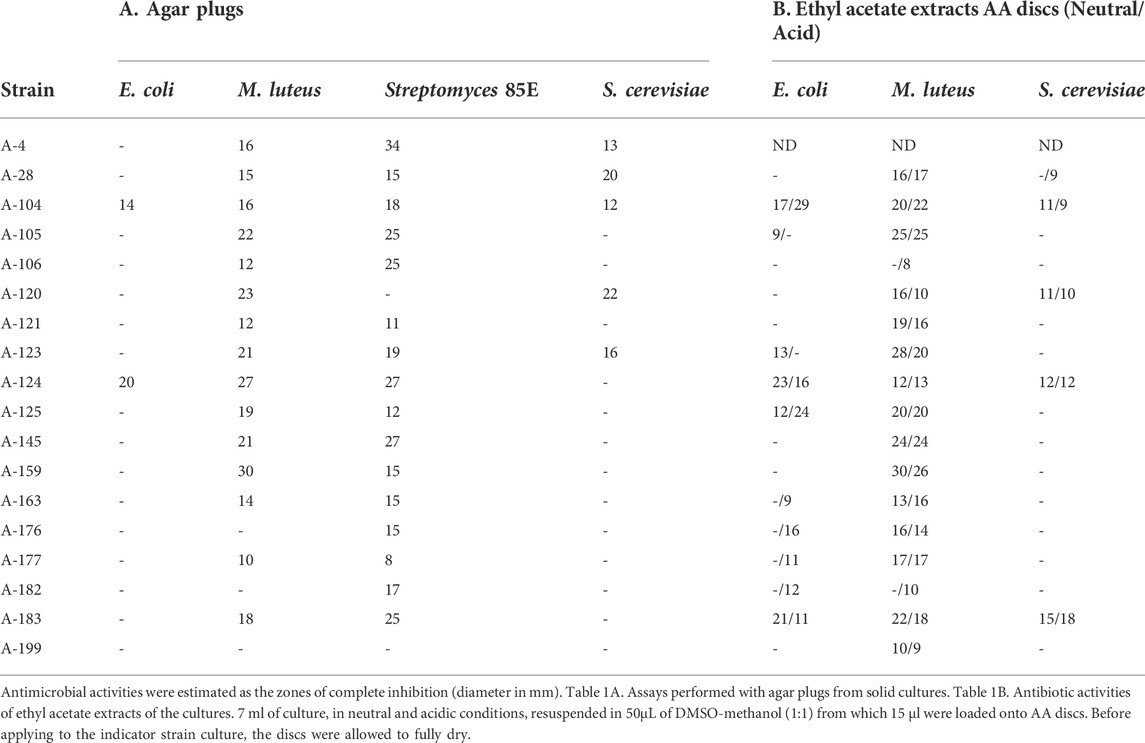
TABLE 1. Antibiotic activities of Actinobacteria against a panel of Gram-negative, Gram-positive bacteria and fungi.
Air mass backward trajectories analyses
Selected bioactive strains were isolated in Northern Spain during years 2013, 2015 and 2016 in nine precipitation events in which the prevailing wind direction was Northern. Samples were mostly collected in Gijón, sampling place, during eight events of rainfall and hailstone, and one snow event was sampled in Oviedo.
NOAA meteorological analyses were addressed to estimate the origin and trajectories of the air masses that caused different precipitation events in which the isolate were obtained. As shown in Figure 2, 5 days HYSPLIT backward trajectories were performed in the sampling locations at three different arriving heights. In all events the air masses were transported by prevalent Northern Winds, and the backward trajectories showed that air masses were mainly originated in the Arctic Ocean, close to the North Pole, and traveled toward South Europe, such as the sampling place in North Spain, thus revealing a main oceanic route from the Arctic and the North Atlantic Oceans. In some events, the air masses trajectory also involves terrestrial routes in Europe, Canada and Greenland to downwind areas.
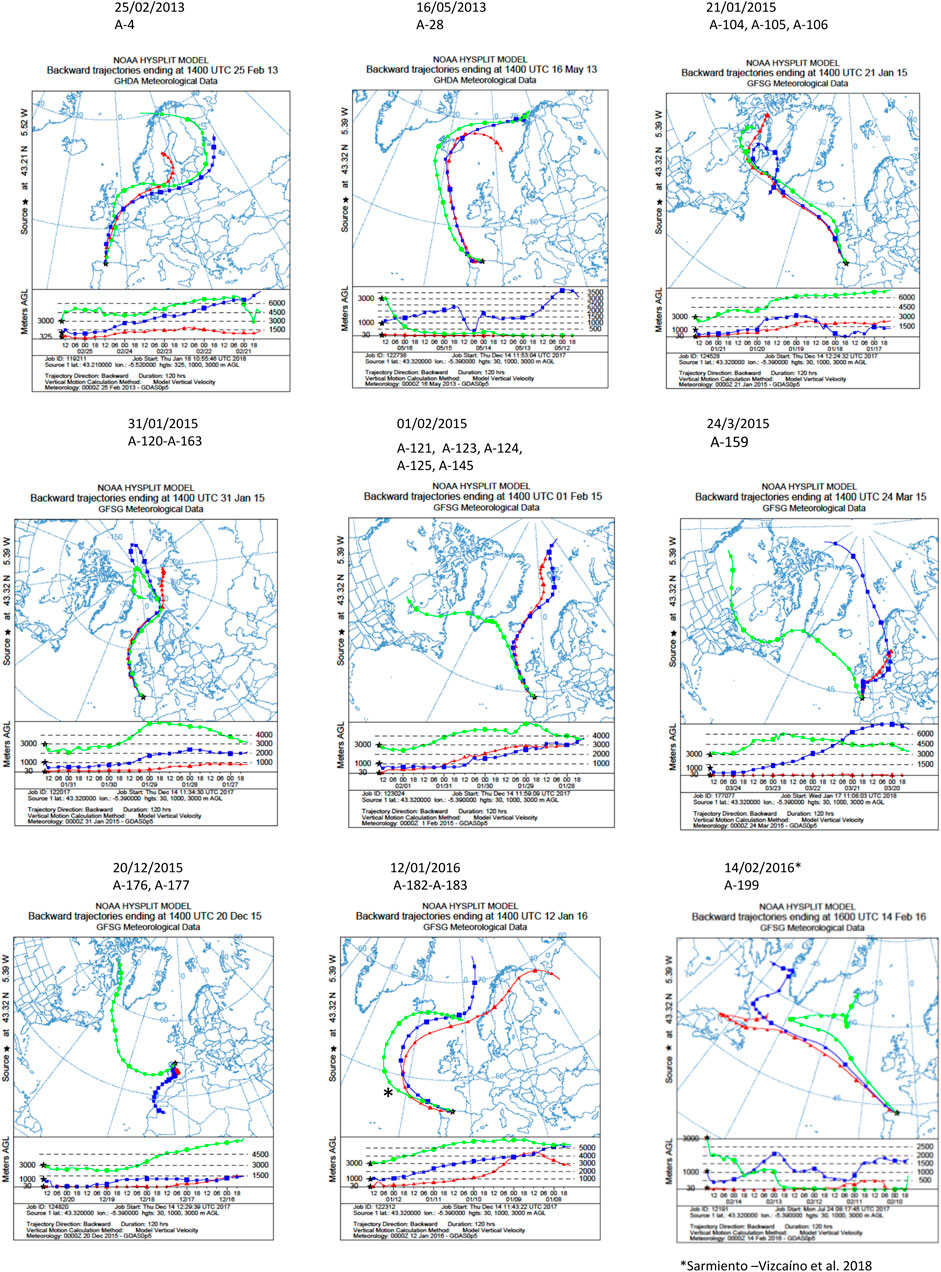
FIGURE 2. Five-day backward trajectories of air masses generating the storms that caused the precipitation events. They were calculated with the NOAA HYSPLIT Model with three different transects with different arriving height as previously reported (Sarmiento-Vizcaíno et al., 2018). The sampling locations were used as the backward trajectory start point with altitudes over sea level of 30, 1,000 and 3,000 m (Gijón), 300, 1,000 and 3,000 m (Oviedo). Black asterisks indicate the sampling places. The strains isolated and the dates of the precipitation events are shown on the top of the figures.
Taxonomic identification, phylogenetic analyses and distribution of bioactive isolates
The nucleotide sequences of the 16S rRNA gene fragments of bioactive strains were deposited in the EMBL database and accession numbers are displayed in Table 2, which also shows the closest phylogenetic relatives with indication of their isolation source. A generalized feature in all strains is their salt tolerance (Table 2). Most of the isolates tolerate NaCl concentrations, up to 7%, which is in agreement with previous reports (Sarmiento-Vizcaíno et al., 2021, 2018).
The results of the phylogenetic analyses based on 16S rRNA gene alignments, revealed that all 18 strains share 99.4–100% identity with known actinobacterial species, belonging to three different genera among the Phylum Actinobacteria. Among 18 studied strains, 15 belonged to the Streptomyces genus, having their closest homologues in species previously isolated from terrestrial, marine and atmospheric environments (Table 2), in association with lichens, plants, terrestrial invertebrates (honeybees, beetles), marine macroalgae and marine invertebrates. In addition, two strains belonging to the Micromonospora genus were obtained in two precipitation events (Table 2). This genus has been proposed as a source for bioactive natural products discovery (Hifnawy M. S. et al., 2020; Hifnawy M. et al., 2020). Also a strain belonging to the genus Nocardiopsis was identified in one precipitation event. A neighbour-joining phylogenetic tree (Figure 3) was built to estimate the relationship between the strains and their nearest phylogenetic relatives.
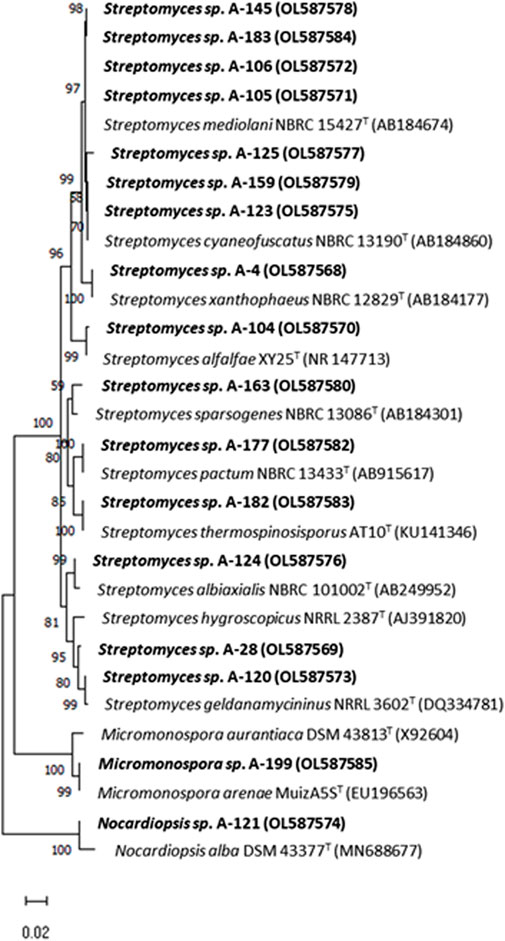
FIGURE 3. Neighbor-joining phylogenetic tree generated by distance matrix analysis of 16S rRNA gene sequences from atmospheric Actinobacteria, Streptomyces, Micromonospora and Nocardiopsis (highlighted) and their nearest phylogenetic relatives. The numbers on branch nodes indicate bootstrap values (1,000 resamplings; only values > 50% are shown). Bar represents 1% sequence divergence.
Identification of bioactive secondary metabolites
The biosynthetic potential of the strains studied herein was uncovered by metabolite profiling analyses of ethyl acetate extracts of strains. Extracts were analyzed by LC-UV and LC/HRMS in combination with searches in UV and MS databases or the Dictionary of Natural Products (DNP), after generation of a molecular formula of each peek based on HRMS results. Complex metabolic profiles were obtained, with most of the strains producing multiple natural products in R5A medium (Supplementary Material S1). As an example, Figure 4 displays UV210 nm chromatograms corresponding to three samples.
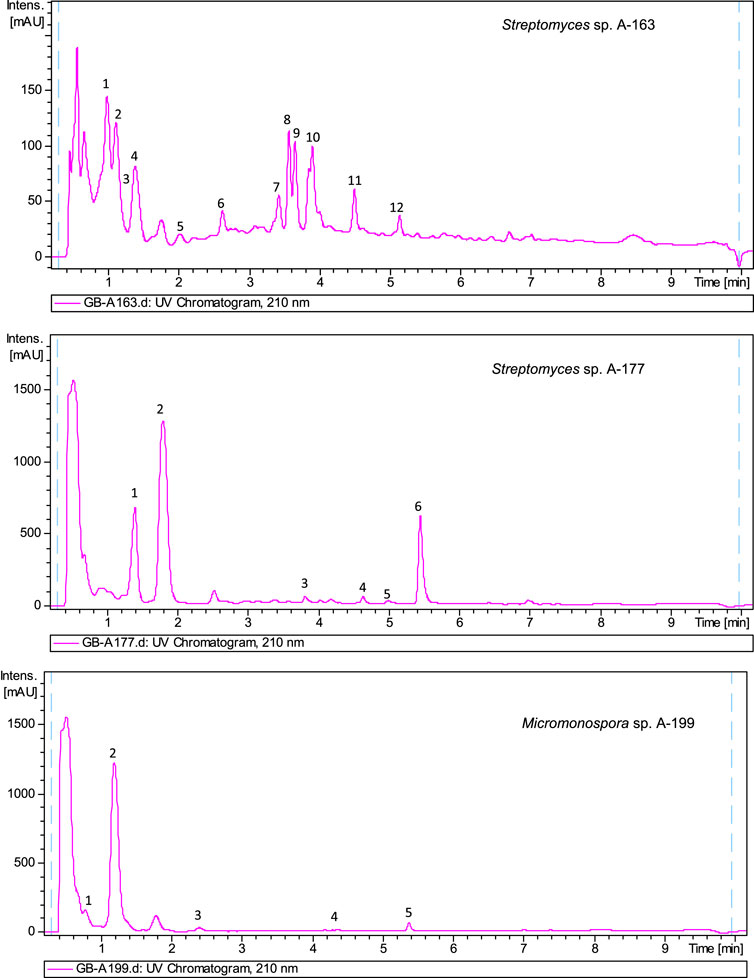
FIGURE 4. UV210nmchromatogram of ethyl acetate extracts from Streptomyces sp A-163, Streptomyces sp A-177 and Micromonospora sp. A-199. Dereplicated components in sample A-163: 1) Hydroxystreptazolin, 2) Streptazolin, 3) Cyclo (leucylprolyl), 4) C12H24O4 (molecular formula not found in the Dictionary of Natural Products for prokaryotes), 5) C18H16O4 (no UV coincidence in the Dictionary of Natural Products for prokaryotes), 6) C11H13NO2 (no UV coincidence in the Dictionary of Natural Products for prokaryotes), 7) C12H20O3 (no UV coincidence in the Dictionary of Natural Products for prokaryotes) (8 and 9) BE 14348B (10) BE 14348D/E (11) WS 9761B (12) Antibiotic W 007. Dereplicated components in sample A-177: 1) Cyclo (phenylalanylprolyl), 2) C13H14O4 (no UV coincidence in the Dictionary of Natural Products for prokaryotes), 3) Alteramide A, 4) Clifednamide A, 5) C15H10ClNO4 new caboxamycin derivative, 6) Caboxamycin B (1). Dereplicated components in sample A-199: (1) Cyclo (4-hydroxyprolylphenylalanyl), (2) N-[2-(1H-Indol-3-yl)-2-oxoethyl]acetamide, (3) C14H12N2O5 (molecular formula not found in the Dictionary of Natural Products for prokaryotes), (4) Macrolactin N, (5) Diazepinomicin.
Among a total of 94 secondary metabolites detected after LC/MS dereplication and comparative analyses of ethyl acetate extracts of all strains, 69 were identified as having matches in DNP, as shown in Table 3. The remaining 25 compounds had molecular formulae determined by HRMS not previously reported for any molecule included in natural product databases (see Table 4) and are of great pharmaceutical interest since they might be new natural products and thus candidates for novel drugs discovery. Regarding the previously reported biological activities of known natural products identified, 36 display biological activities as antibiotics, both antibacterial and antifungal, 22 as antitumor/cytotoxic, four anti-inflammatory, three immunosuppresant, two antiviral, two antiparasitic, two neuroprotective, one hepatoprotective one immunostimulator, and other compounds of pharmacological interest (Table 3). They belong to remarkably diverse structural families, such as anthracyclines, angucyclinones, ansamycins, macrolactams, polienes, polyketides, alkaloids, macrolides, phenazines and others.
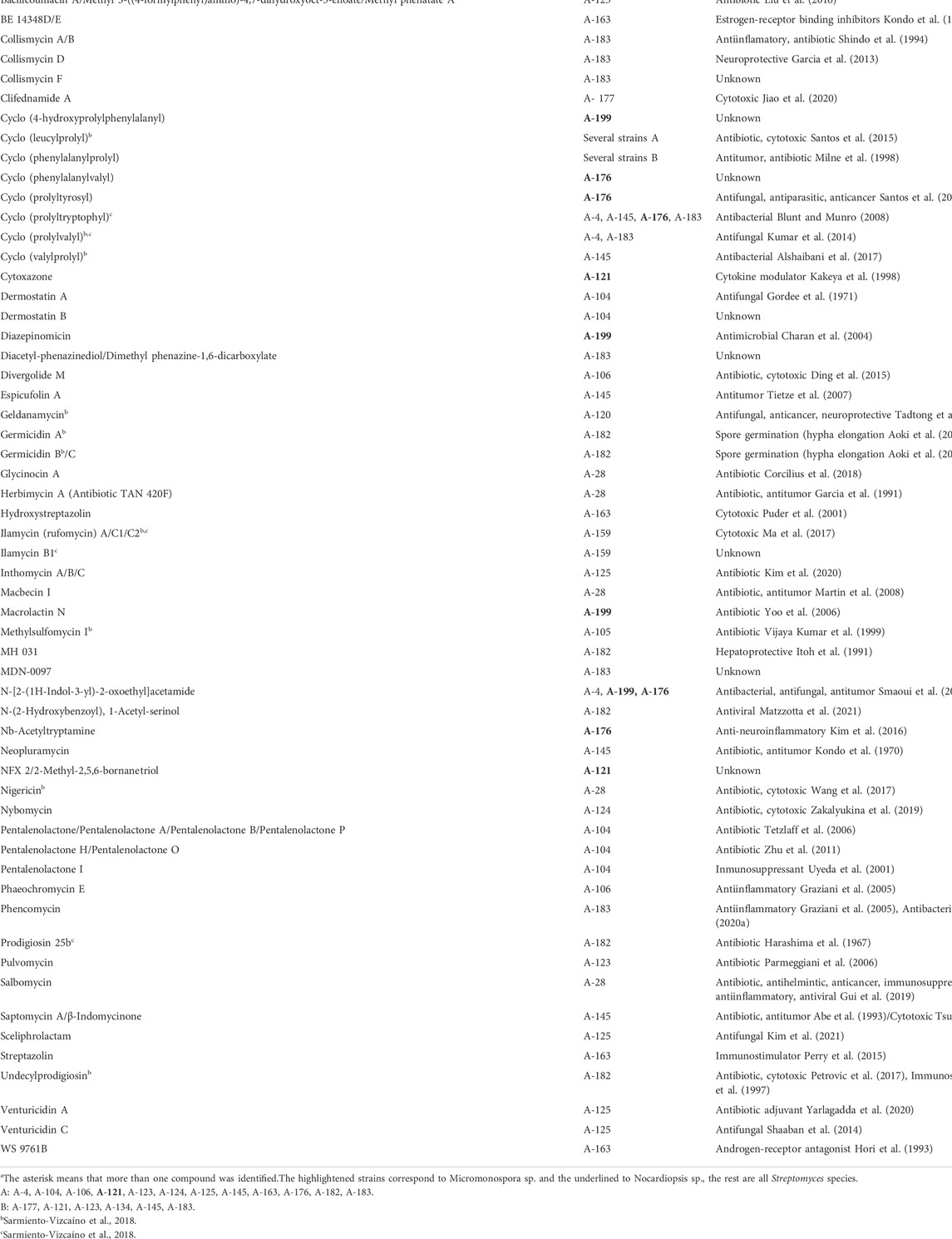
TABLE 3. Identified compounds produced by bioactive Streptomyces, Micromonospora and Nocardiopsis strains and their biological activities.
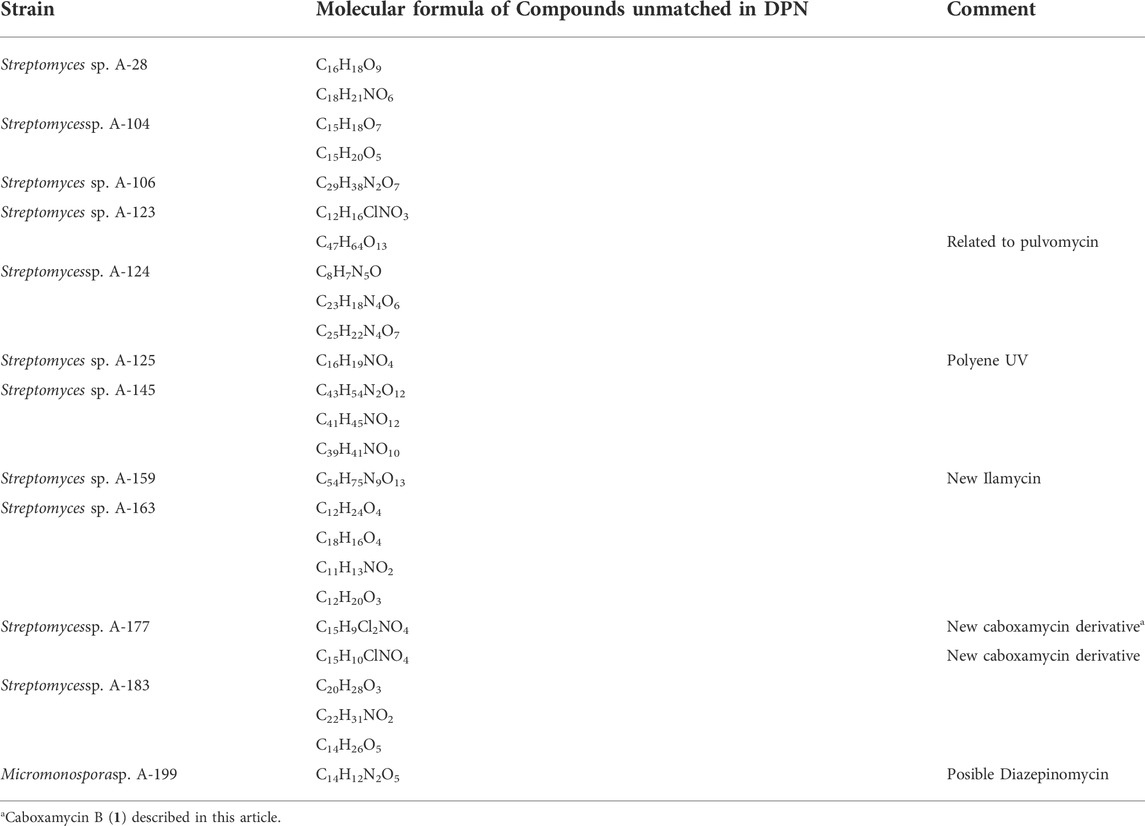
TABLE 4. Molecular formulae of secondary metabolites not previously found in the Dictionary of natural products.
Concerning the sources of compounds, Table 5 displays the number of identified and unidentified compounds produced by each producing strain, and the results of meteorological analyses to know the sources and trajectories of the air masses causing the precipitation events in which they were isolated, estimated with a 5-day NOAA HYSPLIT Model, (Figure 2). According to backward transport trajectories analyses, the air masses mainly originated in the Arctic Ocean and travelled southward to downwind areas, such as the sampling places in Europe in a trajectory that also involves the Atlantic Ocean. As shown in Table 5, the unidentified molecules were produced by 12 strains, 11 Streptomyces and one Micromonospora, and were sampled in winter events mostly sourced around the North Pole. Nine unidentified molecules were obtained in events sourced in Svalbard archipelago, Norway (01/02/2015), four in the North Pole (31/01/2015), three in the White Sea (12/01/2016), two in Lofoten Islands, Norway (16/05/2013). The remaining events in which unidentified molecules were obtained were sourced in the Davies Strait, in the Arctic Ocean, three in the 21/01/2015 event, one in the 24/03/2015 and one in 14/02/2016. Also, 2 novel molecules were obtained in the 20/12/2015 event, in which the air mass at 3,000 m is sourced in the Davies Strait, whereas at 1,000 m originated in Mauritania and Canary Islands, and at 30 m were sourced in Spain (Iberian Peninsula).
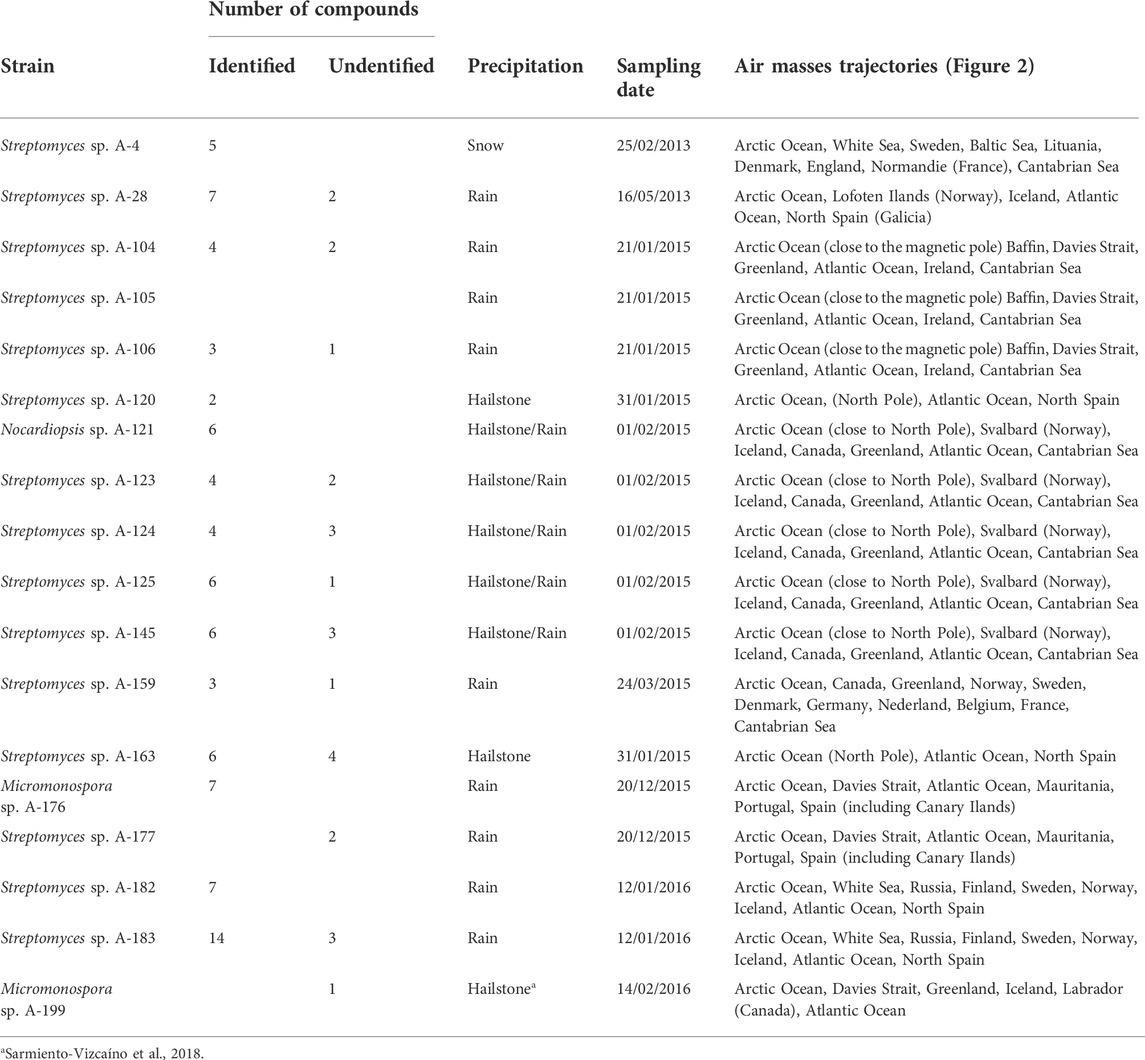
TABLE 5. Sources of atmospheric Actinobacteria producing new natural products. Number of compounds, air masses sources and trajectories of the precipitation events. Air masses backward trajectories analyses were estimated as indicated in Figure 2.
Structural elucidation of caboxamycin B
LC-UV-HRMS analyses of extracts from Streptomyces sp. A-177 revealed the presence of two new natural products structurally related to caboxamycin, an antibiotic belonging to the benzoxazole family produced by a deep-sea Streptomyces isolated in the Canary Basin (Hohmann et al., 2009), and whose biosynthetic pathway was elucidated (Losada et al., 2017) (Table 4). One of these compounds, caboxamycin B (1), was isolated by HPLC as a peak with a characteristic UV spectrum (SI, Fig SX and its structure was determined as shown in Figure 5, based on HRMS and 1D/2D NMR spectroscopy. The (+)ESI-TOF spectrum of 1 (Supplementary Figure S1) showed a [M + H]+ ion at m/z337.9980, with an isotopic pattern corresponding to the presence of two chlorine atoms, indicative of the molecular formula C15H9Cl2NO4 (Δ = -0.30 ppm) which was not found in any natural products database. Interestingly, another ion present in the spectrum at m/z727.9016 and its isotopic distribution fitted very well with the molecular formula C30H15Cl4N2O8Fe ([2M-2H + Fe]+; calcd, 727.9005, Δ = +1.52 ppm), which suggests that one is able to complex iron, as previously reported for caboxamycin (Supplementary Figure S2).
The 1H-NMR spectrum of 1 (Supplementary Table S1, Supplementary Figure S3) showed four aromatic hydrogen signals, two doublets (δH 7.19 and 7.60 ppm; both with 3JH,H = 8.7 Hz) and two broad singlets (δH7.96 and 8.13 ppm), as well as one singlet methyl at δH2.66 ppm and one additional singlet at δH11.66 ppm. Also, a weak signal as a broad singlet is observed at δH13.51 ppm, suggesting the presence of a carboxylic acid group. Interpretation of the 13C-NMR (Supplementary Figure S4) and HSQC (Supplementary Figure S6) spectra confirmed the presence of four aromatic methines and one methyl group and further revealed the presence often quaternary carbons in the structure. These data, along with the molecular formula, clarified that proton signal at 11.66 ppm should be bound to heteroatom and further supported the presence of a carboxylate group in 1.
The benzoxazole-type structure of one was fully elucidated based on COSY, HSQC and HMBC correlations (Supplementary Figures S5–S8). The COSY (Supplementary Figure S5) spectrum revealed the presence of a single spin system comprising vicinal hydrogens H-3 and H-4 (δH 7.19 and 7.60 ppm, respectively). HMBC correlations from H-3 to C-1 and C-5, from H-4 to C-2 and C-6, from H-6 to C-2, C-4 and C-2´ (all of them weak but genuine cross-peaks; SI, Fig SX)and from 2-OH to C-1, C-2 and C-3, together with the chemical shift of C-5 (δC 123.6 ppm), jointly established the presence of a 5-chloro-salicyclic acid moiety (Figure 5).
On the other hand, the 4-methyl-5-chloro-3-hydroxy-anthranilic acid moiety was determined based on HMBC correlations form H-5′ to C-3′a, C-7′ and C-1´´ as well as from 7′-Me to C-6′, C-7′ and C-7′a, along with the characteristic chemical shifts of C-3′a and C-7′a (δC 137.2 and 149.4 ppm respectively) (Hohmann et al., 2009), confirming the structure of caboxamycin B as shown in Figure 5.
Discussion
We provide here further evidence of the potential of the atmosphere in the discovery of natural products of interest in medicine and biotechnology. Antibiotic producing members of three genera of the phylum Actinobacteria were herein isolated in Spain during precipitation events with prevalent Northern winds over a 3 years period. Taxonomic identification and phylogenetic analyses revealed that atmospheric isolates belong mainly to Streptomyces genus, the largest of the phylum, with 690 currently validated species, but also to the Actinobacteria genus Micromonospora, with 110 validated species, and genus Nocardiopsis with 46 validated species. Bearing in mind previous reports on precipitations from Western and NorthWestern winds (Sarmiento-Vizcaíno et al., 2018, 2021), the results provided here from events with Northern winds further increases the number of Streptomyces homologues producing bioactive natural products so far isolated from atmospheric precipitations to a total of 47, which represents a 6.8% of the of the total number of currently validated species in this genus (http://www.bacterio.net/streptomyces.html).
Since atmosphere is an extreme environment, very selective for microorganisms, isolation of Actinobacteria from precipitations resulted much easier than from traditional terrestrial sources. In this innovative approach sampling is a straightforward process, precipitations literally fall from the sky and can bring in a great diversity of novel strains from sources that are potentially out of reach. Although many samples were herein analyzed throughout the years to explore the diversity of Actinobacteria in atmospheric environments, for an efficient natural product discovery approach it would be more convenient to analyze a higher volume from a single precipitation event. This way of proceeding has been successfully reported when sampling a hailstone storm in the same geographical region, where 38 potential novel molecules were found (Sarmiento-Vizcaino et al., 2018).
Meteorological analyses revealed that the air masses involving 5 days backward trajectories point to an Arctic Ocean origin and their diverse downwind pathways. These Actinobacteria remain viable after their atmospheric transport by winds across oceans and continents and are dispersed via the atmosphere before they fall down by precipitation. These findings provide further support for the Streptomyces atmospheric dispersal model (Sarmiento-Vizcaíno et al., 2016, 2021), which is herein extended to the genus Micromonospora. This cycle may have been occurring over geological time scales being of relevance for the biogeography and evolutionary history of these Actinobacteria.
In oceanic environments, among Actinobacteria associated to marine organisms, it has been reported that the dominant genera of producers of secondary metabolites of pharmaceutical relevance are Streptomyces (68%), followed by Micromonospora (6%) and Nocardiopsis (3%) (Chen et al., 2021). The strains herein reported are closely related to bioactive oceanic species isolated in the Cantabrian Sea, North Atlantic Ocean. Among Streptomyces, of particular interest are S. cyaneofuscatus homolog strains (A-123, A-125 and A-159) which are homologues to highly producers of biologically active compounds, isolated during an oceanographic expedition to the submarine Avilés Canyon, Cantabrian Sea (Sarmiento-Vizcaíno et al., 2017b), where novel natural products with antibiotic and cytotoxic activities were discovered (Ortiz-López et al., 2018; Rodríguez et al., 2018). In addition, Micromonospora and Nocardiopsis species, known as a source for structurally diverse novel products (Subramani and Sipkema. 2019), were also isolated herein. Micromonospora species were previously found in snow from Svalbard, Norway, in the Arctic Circle at 78° N (Amato et al., 2007) and also more than 30 isolates with Micromonopora-like phenotype were obtained in other precipitation events (Sarmiento-Vizcaíno, 2016). Furthermore, bioactive species of this genus were also found to colonize deep sea environments, such as the Avilés Canyon up to 4,700 depth, in cold waters of Arctic origin (Sarmiento-Vizcaíno, 2016; Sarmiento-Vizcaíno et al., 2017a), where a novel compound displaying cytotoxic activities against human tumour cell lines was discovered (Sarmiento-Vizcaíno et al., 2017b). Concerning Nocardiopsis, bioactive members of this genus were previously isolated in atmospheric precipitation events with prevalent Western winds (Sarmiento-Vizcaíno et al., 2021).
Comparative analyses of secondary metabolites detected herein with natural products databases, led to the identification of a total of 69 structurally diverse compounds. Concerning their biological activity, after a literature search, 52% of the identified natural products are antibiotics (both against Gram-positive and Gram-negative bacteria and against fungi) and 32% are antitumor/cytotoxic agents. There also compounds with anti-inflamatory, antiviral, antiparasitic, immunosuppresant, immunostimulator, neurprotective, hepatoprotective and other pharmaceutical properties (Table 3). Of great relevance are 25 compounds with molecular formulae not previously reported in Natural product Databases (Table 4), since they are candidates for drugs discovery. Taken into account previous data (Sarmiento-Vizcaíno et al., 2018, 2021), the results here provided increase up to 93 the number of unidentified compounds so far reported to be produced by atmospheric Actinobacteria.
As a proof of concept a novel caboxamycin, caboxamycin B, the second of a family, was herein obtained from cultures of Streptomyces sp. A-177, and its structure established after HPLC purification and HRMS and NMR analysis. This new molecule is structurally related to caboxamycin, an antibiotic of the benzoxazole family initially obtained in the Canary Basin (Hohmann et al., 2009) and later on from Streptomyces halstedii M-204, asociated to an equinoderm in the Avilés Canyon (Sarmiento-Vizcaíno et al., 2017a). Remarkably, caboxamycin B constitutes the first halogenated caboxamycin reported in the literature. Our findings provide further support of the potential of atmospheric-derived Actinobacteria in the discovery of pharmaceutical natural products, particularly antibiotics, which are urgently needed for fighting against pathogenic bacteria resistant to antibiotics in currently in clinical use.
Conclusion
Identification of Actinobacteria in precipitation events with prevalent Northern winds in Spain reveals that, besides Streptomyces and Nocardiopsis species previously reported in samples from Western and North Western winds, bioactive members of the genus Micromonospora are also present in atmospheric environments. In total, almost a hundred of potential novel natural products have been establish in these Actinobacteria isolated from precipitation events with different winds so far. These findings confirm that atmospheric Actinobacteria deserve further research, since they produce a remarkable diversity of bioactive compounds of pharmacological and biotechnological relevance. In this line of thought, the discovery of a novel molecule structurally related to the antibiotic caboxamycin, such as caboxamycin B, is to the best of our knowledge the first novel natural product reported in a Streptomyces strain isolated from the atmosphere.
Data availability statement
The datasets presented in this study can be found in online repositories. The names of the repository/repositories and accession number(s) can be found in the article/Supplementary Material.
Author contributions
AS-V and GB isolated the strains. AS-V performed the bioactivity assays, taxonomic identification, phylogenetic analyses of the strains, extraction of cultures and isolation of caboxamycin B. GB analyzed the air masses backward trajectories. AS-V analyzed the compounds by LC-UV. JM and FR performed the metabolite profiling analysis and identified the compounds produced by LC-MS. FJO-L performed the structural elucidation of caboxamycin B. LG and GB conceived and coordinated the project. GB wrote the manuscript which has been revised, and approved by all the authors.
Acknowledgments
The authors want to thank the Universidad de Oviedo for financial support, Ayuda PAPI-18-PUENTE-6. We are grateful to José L. Martínez and Daniel Serna (Servicios científico-técnicos, edificio Severo Ochoa, Universidad de Oviedo) for their help in strains identification.
Conflict of interest
The authors declare that the research was conducted in the absence of any commercial or financial relationships that could be construed as a potential conflict of interest.
Publisher’s note
All claims expressed in this article are solely those of the authors and do not necessarily represent those of their affiliated organizations, or those of the publisher, the editors and the reviewers. Any product that may be evaluated in this article, or claim that may be made by its manufacturer, is not guaranteed or endorsed by the publisher.
Supplementary material
The Supplementary Material for this article can be found online at: https://www.frontiersin.org/articles/10.3389/fchem.2022.948795/full#supplementary-material
References
Abe, N., Nakakita, Y., Nakamura, T., Enoki, N., Uchida, H., and Munekata, M. (1993). Novel antitumor antibiotics, saptomycins. I. Taxonomy of the producing organism, fermentation, HPLC analysis and biological activities. J. Antibiot. (Tokyo). 46 (10), 1530–1535. doi:10.7164/antibiotics.46.1530
Al-Zereini, W., Fondja, F., Yao, C. B., Laatsch, H., and AnkeAqabamycins, H. A-G. (2010). Aqabamycins A-G: Novel nitro maleimides from a marine Vibrio species. I. Taxonomy, fermentation, isolation and biological activities. J. Antibiot. (Tokyo). 63 (6), 297–301. doi:10.1038/ja.2010.34
Alshaibani, M., Zin, N. M., Jalil, J., Sidik, N., Ahmad, S. J., Kamal, N., et al. (2017). Isolation, purification, and characterization of five active diketopiperazine derivatives from endophytic streptomyces SUK 25 with antimicrobial and cytotoxic activities. J. Microbiol. Biotechnol. 27, 1249–1256. doi:10.4014/jmb.1608.08032
Amato, P., Hennebelle, R., Magand, O., Sancelme, M., Delort, A. M., Barbante, C., et al. (2007). Bacterial characterization of the snow cover at Spitzberg, Svalbard. FEMS Microbiol. Ecol. 59 (2), 255–264. doi:10.1111/j.1574-6941.2006.00198.x
Aoki, Y., Matsumoto, D., Kawaide, H., and Natsume, M. (2011). Physiological role of germicidins in spore germination and hyphal elongation in Streptomyces coelicolor A3(2). J. Antibiot. 64, 607–611. doi:10.1038/ja.2011.59
Arahal, D. R., Sánchez, E., Macián, M. C., and Garay, E. (2008). Value of recN sequences for species identification and as a phylogenetic marker within the family “Leuconostocaceae”. Int. Microbiol. 11, 33–39.
Bell, R., Carmeli, S., and Sar, N. (1994). Vibrindole A, a metabolite of the marine bacterium, Vibrio parahaemolyticus, isolated from the toxic mucus of the boxfish Ostracion cubicus. J. Nat. Prod. (Gorakhpur). 57 (11), 1587–1590. doi:10.1021/np50113a022
Blunt, J., and Munro, M. H. G. (2008). Dictionary of marine naturalproducts, with CD-ROM. Boca Raton, FL: Chapman & Hall/CRC Press.
Braña, A. F., Fiedler, H. P., Nava, H., González, V., Sarmiento-Vizcaíno, A., Molina, A., et al. (2015). Two Streptomyces species producing antibiotic, antitumor, and anti-inflammatory compounds are widespread among intertidal macroalgae and deep-sea coral reef invertebrates from the central Cantabrian Sea. Microb. Ecol. 69, 512–524. doi:10.1007/s00248-014-0508-0
Chapman, E., and Hall, W. (2015). Dictionary of marine natural products (DMNP 2014). Boca Raton, FL: Chapman & Hall/CRC.
Charan, R. D., Schlingmann, G., Janso, J., Bernan, V., Feng, X., and Carter, G. T. (2004). Diazepinomicin, a new antimicrobial alkaloid from a marine Micromonospora sp. J. Nat. Prod. (Gorakhpur). 67 (8), 1431–1433. doi:10.1021/np040042r
Chen, J., Xu, L., Zhou, Y., and Han, B. (2021). Natural products from actinomycetes associated with marine organisms. Mar. Drugs 19 (11), 629. doi:10.3390/md19110629
Corcilius, L., Liu, D. Y., Ochoa, J. L., Linington, R. G., and Payne, R. J. (2018). Synthesis and evaluation of analogues of the glycinocin family of calcium-dependent antibiotics. Org. Biomol. Chem. 16 (29), 5310–5320. doi:10.1039/c8ob01268g
Ding, L., Franke, J., and Hertweck, C. (2015). Divergolide congeners illuminate alternative reaction channels for ansamycin diversification. Org. Biomol. Chem. 13 (6), 1618–1623. doi:10.1039/c4ob02244k
Garcia, I., Vior, N. M., González-Sabín, J., Braña, A. F., Rohr, J., Moris, F., et al. (2013). Engineering the biosynthesis of the polyketide-nonribosomal peptide collismycin A for generation of analogs with neuroprotective activity. Chem. Biol. 20 (8), 1022–1032. doi:10.1016/j.chembiol.2013.06.014
Garcia, R., Parikh, N. U., Saya, H., and Gallick, G. E. (1991). Effect of herbimycin A on growth and pp60c-src activity in human colon tumor cell lines. Oncogene 6 (11), 1983–1989.
Goodfellow, M., Kumar, Y., Labeda, D. P., and Sembiring, L. (2007). The Streptomyces violaceusniger clade: A home for streptomycetes with rugose ornamented spores. Ant. Van Leeuwenhoek 92, 173–199. doi:10.1007/s10482-007-9146-6
Gordee, R. S., Butler, T. F., and Narasimhachari, N. (1971). The antifungal activity of dermostatin. J. Antibiot. (Tokyo). 24 (8), 561–565. doi:10.7164/antibiotics.24.561
Graziani, E. I., Ritacco, F. V., Bernan, V. S., and Telliez, J. B. (2005). Phaeochromycins A-E, anti-inflammatory polyketides isolated from the soil actinomycete Streptomyces phaeochromogenes LL-P018. J. Nat. Prod. (Gorakhpur). 68 (8), 1262–1265. doi:10.1021/np0500629
Gui, M., Zhang, M. X., Wu, W. H., and Sun, P. (2019). Natural occurrence, bioactivity and biosynthesis of elaiophylin analogues. Molecules 24 (21), 3840. doi:10.3390/molecules24213840
Harashima, K., Tsuchida, N., Tanaka, T., and Nagatsu, J. (1967). Prodigiosin-25 C isolation and the chemical structure. Agric. Biol. Chem. 31 (4), 481–489. doi:10.1080/00021369.1967.10858824
Hifnawy, M., Hassan, H. M., Mohammed, R., Sayed, A. M., Hamed, A. A., AbouZid, F. S., et al. (2020b). Induction of antibacterial metabolites by Co-cultivation of two red-sea-sponge-associated actinomycetes Micromonospora sp. UR56 and actinokinespora sp. EG49. Mar. Drugs 18 (5), 243. doi:10.3390/md18050243
Hifnawy, M. S., Fouda, M. M., Sayed, A. M., Mohammed, R., Hassan, H. M., AbouZid, S. F., et al. (2020a). The genus Micromonospora as a model microorganism for bioactive natural product discovery. RSC Adv. 10 (35), 20939–20959. doi:10.1039/d0ra04025h
Hohmann, C., Schneider, K., Bruntner, C., Irran, E., Nicholson, G., Bull, A. T., et al. (2009). Caboxamycin, a new antibiotic of the benzoxazole family produced by the deep-sea strain Streptomyces sp. NTK 937. J. Antibiot. (Tokyo). 62 (2), 99–104. doi:10.1038/ja.2008.24
Hori, Y., Abe, Y., Nakajima, H., Shigematsu, N., Takase, S., Goto, T., et al. (1993). WS9761 A and B: New non-steroidal androgen-receptor antagonists produced by a streptomyces. J. Antibiot. (Tokyo). 46 (12), 1901–1903. doi:10.7164/antibiotics.46.1901
Itoh, Y., Shimura, H., Ito, M., Watanabe, N., Yamagishi, M., Tamai, M., et al. (1991) A novel hepatoprotective .NU.-lactone, MH-031. I. Discovery, isolation, physico-chemical properties and structural elucidation. J. Antibiot. (Tokyo). 44 (8), 832–837. doi:10.7164/antibiotics.44.832
Jensen, H. L. (1931). Contributions to our knowledge of the Actinomycetales. II. The definition and subdivision of the genus Actinomyces, with a preliminary account of Australian soil Actinomycetes. Proc. Linn. Soc. N. S. W. 56, 345–370.
Jiao, Y. J., Liu, Y., Wang, H. X., Zhu, D. Y., Shen, Y. M., and Li, Y. Y. (2020). Expression of the Clifednamide biosynthetic pathway in streptomyces generates 27, 28-seco-Derivatives. J. Nat. Prod. (Gorakhpur). 83 (9), 2803–2808. doi:10.1021/acs.jnatprod.0c00900
Jiménez, J. T., Sturdíková, M., Brezová, V., Svajdlenka, E., and Novotová, M. (2012). Screening of mutant strain Streptomyces mediolani sp. AC37 for (-)-8-O-methyltetrangomycin production enhancement. J. Microbiol. 50 (6), 1014–1023. doi:10.1007/s12275-012-2025-5
Kakeya, H., Morishita, M., Kobinata, K., Osono, M., Ishizuka, M., and Osada, H. (1998). Isolation and biological activity of a novel cytokine modulator, cytoxazone. J. Antibiot. (Tokyo). 51 (12), 1126–1128. doi:10.7164/antibiotics.51.1126
Khan, S. T., Komaki, H., Motohashi, K., Kozone, I., Mukai, A., Takagi, M., et al. (2011). Streptomyces associated with a marine sponge Haliclona sp.; biosynthetic genes for secondary metabolites and products. Environ. Microbiol. 13 (2), 391–403. doi:10.1111/j.1462-2920.2010.02337.x
Kim, D. C., Quang, T. H., Yoon, C. S., Ngan, N. T. T., Lim, S. I., Lee, S. Y., et al. (2016). Anti-neuroinflammatory activities of indole alkaloids from kanjang (Korean fermented soy source) in lipopolysaccharide-induced BV2 microglial cells. Food Chem. x. 213, 69–75. doi:10.1016/j.foodchem.2016.06.068
Kim, S. B., and Goodfellow, M. (2002). Streptomyces thermospinisporus sp. nov., a moderately thermophilic carboxydotrophic streptomycete isolated from soil. Int. J. Syst. Evol. Microbiol. 52 (4), 1225–1228. doi:10.1099/00207713-52-4-1225
Kim, J. H., Song, Y., Kim, M. J., and Kim, S. (2020). Asymmetric total synthesis of inthomycins A, B, and C. J. Org. Chem. 85 (7), 4795–4806. doi:10.1021/acs.joc.0c00017
Kim, S. H., Park, G., Park, J. S., and Kwon, H. C. (2021). Antifungal streptomyces spp., plausible partners for brood-caring of the dung beetle copris tripartitus. Microorganisms 9 (9), 1980. doi:10.3390/microorganisms9091980
Kirby, B. M., and Meyers, P. R. 2007. "Micromonospora arenae sp. nov., isolated from marine sediment collected from Muizenberg Beach, South Africa and Micromonospora tulbaghiae sp. nov., isolated from the leaves of wild garlic, Tulbaghia violacea."
Kondo, H., Nakajima, S., Yamamoto, N., Okura, A., Satoh, F., Suda, H., et al. (1990). BE-14348 substances, new specific estrogen-receptor binding inhibitors. Production, isolation, structure determination and biological properties. J. Antibiot. (Tokyo). 43 (12), 1533–1542. doi:10.7164/antibiotics.43.1533
Kondo, S., Wakashiro, T., Hamada, M., Maeda, K., Takeuchi, T., and Umezawa, H. (1970). Isolation and characterization of a new antibiotic, neopluramycin. J. Antibiot. (Tokyo). 23 (7), 354–359. doi:10.7164/antibiotics.23.354
Kumar, S. N., Nambisan, B., Sundaresan, A., Mohandas, C., and Anto, R. J. (2014). Isolation and identification of antimicrobial secondary metabolites from Bacillus cereus associated with a rhabditid entomopathogenic nematode. Ann. Microbiol. 64, 209–218. doi:10.1007/s13213-013-0653-6
Liu, S., Han, X., Jiang, Z., Wu, G., Hu, X., You, X., et al. (2016). Hetiamacin B-D, new members of amicoumacin group antibiotics isolated from Bacillus subtilis PJS. J. Antibiot. (Tokyo) 69, 769–772. doi:10.1038/ja.2016.3
Losada, A. A., Cano-Prieto, C., García-Salcedo, R., Braña, A. F., Méndez, C., Salas, J. A., et al. (2017). Caboxamycin biosynthesis pathway and identification of novel benzoxazoles produced by cross-talk in Streptomyces sp. NTK 937. Microb. Biotechnol. 10 (4), 873–885. doi:10.1111/1751-7915.12716
Luzhetskyy, A., Hoffmann, J., Pelzer, S., Wohlert, S. E., Vente, A., and Bechthold, A. (2008). Aranciamycin analogs generated by combinatorial biosynthesis show improved antitumor activity. Appl. Microbiol. Biotechnol. 80 (1), 15–19. doi:10.1007/s00253-008-1515-1
Ma, J., Huang, H., Xie, Y., Liu, Z., Zhao, J., Zhang, C., et al. (2017). Biosynthesis of ilamycins featuring unusual building blocks and engineered production of enhanced anti-tuberculosis agents. Nat. Commun. 8, 391. doi:10.1038/s41467-017-00419-5
Maehr, H., Liu, C. M., Liu, M., Perrotta, A., Smallheer, J. M., Williams, T. H., et al. (1982). Microbial products. VI Five novel metabolites related to benz[a]anthracene from an unidentified actinomycete designated X-14881. J. Antibiot. (Tokyo). 35 (12), 1627–1631. doi:10.7164/antibiotics.35.1627
Martin, C. J., Gaisser, S., Challis, I. R., Carletti, I., Wilkinson, B., Gregory, M., et al. (2008). Molecular characterization of macbecin as an Hsp90 inhibitor. J. Med. Chem. 51 (9), 2853–2857. doi:10.1021/jm701558c
Mazzotta, S., Berastegui-Cabrera, J., Vega-Holm, M., García-Lozano, M. D. R., Carretero-Ledesma, M., Aiello, F., et al. (2021). Design, synthesis and in vitro biological evaluation of a novel class of anti-adenovirus agents based on 3-amino-1, 2-propanediol. Bioorg. Chem. 114, 105095. doi:10.1016/j.bioorg.2021.105095
Milne, P. J., Hunt, A. L., Rostoll, K., Van Der Walt, J. J., and Graz, C. J. (1998). The biological activity of selected cyclic dipeptides. J. Pharm. Pharmacol. 50 (12), 1331–1337. doi:10.1111/j.2042-7158.1998.tb03355.x
Moree, W. J., McConnell, O. J., Nguyen, D. D., Sanchez, L. M., Yang, Y. L., Zhao, X., et al. (2014). Microbiota of healthy corals are active against fungi in a light-dependent manner. ACS Chem. Biol. 9, 2300–2308. doi:10.1021/cb500432j
Ortiz-López, F. J., Alcalde, E., Sarmiento-Vizcaíno, A., Díaz, C., Cautain, B., García, L. A., et al. (2018). New 3-hydroxyquinaldic acid derivatives from cultures of the marine derived actinomycete Streptomyces cyaneofuscatus M-157. Mar. Drugs 16, 371. doi:10.3390/md16100371
Parmeggiani, A., Krab, I. M., Okamura, S., Nielsen, R. C., Nyborg, J., and Nissen, P. (2006). Structural basis of the action of pulvomycin and GE2270 A on elongation factor Tu. Biochemistry 45 (22), 6846–6857. doi:10.1021/bi0525122
Paściak, M., Pawlik, K., Gamian, A., Szponar, B., Skóra, J., and Gutarowska, B. (2014). An airborne actinobacteria Nocardiopsis alba isolated from bioaerosol of a mushroom compost facility. Aerobiologia 30, 413–422. doi:10.1007/s10453-014-9336-4
Pérez-Victoria, I., Martín, J., and Reyes, F. (2016). Combined LC/UV/MS and NMR strategies for the dereplication of marine natural products. Planta Med. 82, 857–871. doi:10.1055/s-0042-101763
Perry, J. A., Koteva, K., Verschoor, C. P., Wang, W., Bowdish, D. M., and Wright, G. D. (2015). A macrophage-stimulating compound from a screen of microbial natural products. J. Antibiot. (Tokyo). 68 (1), 40–46. doi:10.1038/ja.2014.83
Petrović, S., Vasić, V., Mitrović, T., Lazović, S., and Leskovac, A. (2017). The impact of concentration and administration time on the radiomodulating properties of undecylprodigiosin in vitro. Archives Industrial Hyg. Toxicol. 68, 1–8. doi:10.1515/aiht-2017-68-2897
Puder, C., Loya, S., Hizi, A., and Zeeck, A. (2001). New co-metabolites of the streptazolin pathway. J. Nat. Prod. (Gorakhpur). 64 (1), 42–45. doi:10.1021/np000377i
Qiao, J., Chen, L., Li, Y., Wang, J., Zhang, W., and Chen, S. (2012). Whole-genome sequence of Nocardiopsis alba strain ATCC BAA-2165, associated with honeybees. J. Bacteriol. 194, 6358–6359. doi:10.1128/JB.01522-12
Ramírez-Rendon, D., Passari, A. K., Ruiz-Villafán, B., Rodríguez-Sanoja, R., Sánchez, S., and Demain, A. L. (2022). Impact of novel microbial secondary metabolites on the pharma industry. Appl. Microbiol. Biotechnol. 106, 1855–1878. doi:10.1007/s00253-022-11821-5
Rodríguez, V., Martín, J., Sarmiento-Vizcaíno, A., de la Cruz, M., García, L. A., Blanco, G., et al. (2018). Anthracimycin B, a potent antibiotic against gram-positive bacteria isolated from cultures of the deep-sea actinomycete streptomyces cyaneofuscatus M-169. Mar. Drugs 16, 406. doi:10.3390/md16110406
Russell, D. W., and Sambrook, J. F. (2001). Molecular cloning: A laboratory manual. 3rd Edn. New York, NY: Cold Spring Harbor Laboratory Press.
Ruttanasutja, P., and Pathom-aree, W. (2015). Selective isolation of cultivable actinomycetes from Thai coastal marine sediment. Available at: http://cmuir.cmu.ac.th/jspui/handle/6653943832/66826.
Santos, J. D., Vitorino, I., De la Cruz, M., Diaz, C., Cautain, B., Annang, F., et al. (2019). Bioactivities and extract dereplication of actinomycetales isolated from marine sponges. Front. Microbiol. 10, 727. doi:10.3389/fmicb.2019.00727
Santos, O. C. S., Soares, A. R., Machado, F. L. S., Romanos, M. T. V., Muricy, G., Giambiagi-deMarval, M., et al. (2015). Investigation of biotechnological potential of sponge-associated bacteria collected in Brazilian coast. Lett. Appl. Microbiol. 60, 140–147. doi:10.1111/lam.12347
Sarmiento-Vizcaíno, A., Espadas, J., Martín, J., Braña, A. F., Reyes, F., García, L. A., et al. (2018). Atmospheric precipitations, hailstone and rainwater, as a novel source of streptomyces producing bioactive natural products. Front. Microbiol. 9, 773. doi:10.3389/fmicb.2018.00773
Sarmiento-Vizcaíno, A., Martín, J., Reyes, F., García, L. A., and Blanco, G. (2021). Bioactive natural products in actinobacteria isolated in rainwater from storm clouds transported by western winds in Spain. Front. Microbiol. 12, 773095. doi:10.3389/fmicb.2021.773095
Sarmiento-Vizcaíno, A., Braña, A. F., González, V., Nava, H., Molina, A., Llera, E., et al. (2016). Atmospheric dispersal of bioactive Streptomyces albidoflavus strains among terrestrial and marine environments. Microb. Ecol. 71, 375–386. doi:10.1007/s00248-015-0654-z
Sarmiento-Vizcaíno, A., Braña, A. F., Pérez-Victoria, I., Martín, J., de Pedro, N., Cruz, M., et al. (2017b). Paulomycin G, a new natural product with cytotoxic activity against tumor cell lines produced by deep-sea sediment derived Micromonospora matsumotoense M-412 from the Avilés Canyon in the Cantabrian Sea. Mar. Drugs 15, 271. doi:10.3390/md15090271
Sarmiento-Vizcaíno, A., González, V., Braña, A. F., Palacios, J. J., Otero, L., Fernández, J., et al. (2017a). Pharmacological potential of phylogenetically diverse actinobacteria isolated from deep-sea coral ecosystems of the submarine Avilés Canyon in the Cantabrian Sea. Microb. Ecol. 73, 338–352. doi:10.1007/s00248-016-0845-2
Sarmiento-Vizcaíno, A. (2016). Pharmacological potential of actinobacteria isolated from terrestrial, marine and atmospheric environments in the Cantabrian. Oviedo: Dissertation thesis. University of Oviedo. Available at: http://hdl.handle.net/10651/39315.
Seiichi, T., Masayuki, M., and Toru, H. (1983). Antibiotic TAN-420, its production and use. European Patent EP0110710A2.
Shaaban, K. A., Singh, S., Elshahawi, S. I., Wang, X., Ponomareva, L. V., Sunkara, M., et al. (2014). Venturicidin C, a new 20-membered macrolide produced by Streptomyces sp. TS-2-2. J. Antibiot. (Tokyo). 67 (3), 223–230. doi:10.1038/ja.2013.113
She, W., Sun, Z., Yi, L., Zhao, S., and Liang, Y. (2016). Streptomyces alfalfae sp. nov. and comparisons with its closest taxa Streptomyces silaceus, Streptomyces flavofungini and Streptomyces intermedius. Int. J. Syst. Evol. Microbiol. 66 (1), 44–49. doi:10.1099/ijsem.0.000671
Shigemori, H., Bae, M. A., Yazawa, K., Sasaki, T., and Kobayashi, J. (1992). Alteramide A, a new tetracyclic alkaloid from a bacterium Alteromonas sp. associated with the marine sponge Halichondria okadai. J. Org. Chem. 57, 4317–4320. doi:10.1021/jo00041a053
Shindo, K., Yamagishi, Y., Okada, Y., and Kawai, H. (1994). Collismycins A and B, novel non-steroidal inhibitors of denamethasone-glucocorticoid receptor binding. J. Antibiot. (Tokyo). 47 (9), 1072–1074. doi:10.7164/antibiotics.47.1072
Singh, V., Haque, S., Singh, H., Verma, J., Vibha, K., Singh, R., et al. (2016). Isolation, screening, and identification of novel isolates of actinomycetes from India for antimicrobial applications. Front. Microbiol. 7, 1921. doi:10.3389/fmicb.2016.01921
Smaoui, S., Mathieu, F., Elleuch, L., Coppel, Y., Merlina, G., Karray-Rebai, I., et al. (2012). Taxonomy, purification and chemical characterization of four bioactive compounds from new Streptomyces sp. TN256 strain. World J. Microbiol. Biotechnol. 28 (3), 793–804. doi:10.1007/s11274-011-0872-6
Songia, S., Mortellaro, A., Taverna, S., Fornasiero, C., Scheiber, E. A., Erba, E., et al. (1997). Characterization of the new immunosuppressive drug undecylprodigiosin in human lymphocytes: Retinoblastoma protein, cyclin-dependent kinase-2, and cyclin-dependent kinase-4 as molecular targets. J. Immunol. 158, 3987–3995.
Stein, A. F., Draxler, R. R., Rolph, G. D., Stunder, B. J. B., Cohen, M. D., and Ngan, F. (2015). NOAA’s HYSPLIT atmospheric transport and dispersion modeling system. Bull. Am. Meteorol. Soc. 96, 2059–2077. doi:10.1175/BAMS-D-14-00110.1
Subramani, R., and Sipkema, D. (2019). Marine rare actinomycetes: A promising source of structurally diverse and unique novel natural products. Mar. Drugs 17 (5), 249. doi:10.3390/md17050249
Tadtong, S., Meksuriyen, D., Tanasupawat, S., Isobe, M., and Suwanborirux, K. (2007). Geldanamycin derivatives and neuroprotective effect on cultured P19-derived neurons. Bioorg. Med. Chem. Lett. 17, 2939–2943. doi:10.1016/j.bmcl.2006.12.041
Tetzlaff, C. N., You, Z., Cane, D. E., Takamatsu, S., Omura, S., and Ikeda, H. (2006). A gene cluster for biosynthesis of the sesquiterpenoid antibiotic pentalenolactone in Streptomyces avermitilis. Biochemistry 45 (19), 6179–6186. doi:10.1021/bi060419n
Tietze, L. F., Gericke, K. M., Singidi, R. R., and Schuberth, I. (2007). Novel strategies for the synthesis of anthrapyran antibiotics: Discovery of a new antitumor agent and total synthesis of (S)-espicufolin. Org. Biomol. Chem. 5 (8), 1191–1200. doi:10.1039/b700838d
Tindall, B. J. (2014). The epithet aurantiaca in micromonospora aurantiaca Sveshnikova et al. 1969 (Approved Lists 1980) is illegitimate and requires a replacement epithet. Opinion 89. Judicial commission of the international committee on systematics of prokaryotes. Int. J. Syst. Evol. Microbiol. 64 (10), 3580–3581. doi:10.1099/ijs.0.069153-0
Tsukahara, K., Toume, K., Ito, H., Ishikawa, N., and Ishibashi, M. (2014). Isolation of β-indomycinone guided by cytotoxicity tests from Streptomyces sp. IFM11607 and revision of its double bond geometry. Nat. Prod. Commun. 9 (9), 1934578X1400900–8. doi:10.1177/1934578x1400900927
Uyeda, M., Mizukami, M., Yokomizo, K., and Suzuki, K. (2001). Pentalenolactone I and hygromycin A, immunosuppressants produced by Streptomyces filipinensis and Streptomyces hygroscopicus. Biosci. Biotechnol. Biochem. 65 (5), 1252–1254. doi:10.1271/bbb.65.1252
Vijaya Kumar, E. K., Kenia, J., Mukhopadhyay, T., and Nadkarni, S. R. (1999). Methylsulfomycin I, a new cyclic peptide antibiotic from a Streptomyces sp. HIL Y-9420704. J. Nat. Prod. 62, 1562–1564. doi:10.1021/np990088y
Whitman, W., Goodfellow, M., Kämpfer, P., Busse, H.-J., Trujillo, M., Ludwig, W., et al. (2012). Bergey’s manual of systematic bacteriology 5 The Actinobacteria (2 ed.). Springer Science & Business Media.
Wu, C. Z., Jang, J. H., Ahn, J. S., and Hong, Y. S. (2012). New geldanamycin analogs from Streptomyces hygroscopicus. J. Microbiol. Biotechnol. 22, 1478–1481. doi:10.4014/jmb.1206.06026
Yarlagadda, V., Medina, R., and WrightVenturicidin, G. D. A. (2020). Venturicidin A, A membrane-active natural product inhibitor of atp synthase potentiates aminoglycoside antibiotics. Sci. Rep. 10 (1), 8134. doi:10.1038/s41598-020-64756-0
Yoo, J. S., Zheng, C. J., Lee, S., Kwak, J. H., and KimMacrolactin, W. G. N. (2006). Macrolactin N, a new peptide deformylase inhibitor produced by Bacillus subtilis. Bioorg. Med. Chem. Lett. 16 (18), 4889–4892. doi:10.1016/j.bmcl.2006.06.058
Zakalyukina, Y. V., Birykov, M. V., Lukianov, D. A., Shiriaev, D. I., Komarova, E. S., Skvortsov, D. A., et al. (2019). Nybomycin-producing Streptomyces isolated from carpenter ant Camponotus vagus. Biochimie 160, 93–99. doi:10.1016/j.biochi.2019.02.010
Zhang, H., Wang, H., Wang, Y., Cui, H., Xie, Z., Pu, Y., et al. (2012). Genomic sequence-based discovery of novel angucyclinone antibiotics from marine Streptomyces sp. W007. FEMS Microbiol. Lett. 332 (2), 105–112. doi:10.1111/j.1574-6968.2012.02582.x
Keywords: caboxamycin B, antibiotic, antitumor, streptomyces, micromonospora, nocardiopsis, actinomycete
Citation: Sarmiento-Vizcaíno A, Martín J, Ortiz-López FJ, Reyes F, García LA and Blanco G (2022) Natural products, including a new caboxamycin, from Streptomyces and other Actinobacteria isolated in Spain from storm clouds transported by Northern winds of Arctic origin. Front. Chem. 10:948795. doi: 10.3389/fchem.2022.948795
Received: 20 May 2022; Accepted: 17 October 2022;
Published: 03 November 2022.
Edited by:
Yong Huang, Central South University, ChinaReviewed by:
Madan Kharel, University of Maryland Eastern Shore, United StatesSatya P. Singh, Saurashtra University, India
Copyright © 2022 Sarmiento-Vizcaíno, Martín, Ortiz-López, Reyes, García and Blanco. This is an open-access article distributed under the terms of the Creative Commons Attribution License (CC BY). The use, distribution or reproduction in other forums is permitted, provided the original author(s) and the copyright owner(s) are credited and that the original publication in this journal is cited, in accordance with accepted academic practice. No use, distribution or reproduction is permitted which does not comply with these terms.
*Correspondence: Gloria Blanco, gbb@uniovi.es