Proflavine (PFH+): as a photosensitizer (PS) biocatalyst for the visible-light-induced synthesis of pyrano [2,3-d] pyrimidine scaffolds
- Department of Medical Nanotechnology, School of Advanced Medical Sciences and Technologies, Shiraz University of Medical Sciences, Shiraz, Iran
A sustainable methodology for the synthesis of pyrano [2,3-d] pyrimidine scaffolds have been developed, employing the Knoevenagel-Michael tandem cyclocondensation reaction of barbituric acid/1,3-dimethylbarbituric acid, malononitrile, and aryl aldehydes. This study elucidates the advancement of a sustainable and environmentally conscious approach to synthesizing this category of chemical compounds. In the present investigation, a novel photosensitizer comprising proflavine (PFH+) bio-photocatalyst was employed in an aqueous medium, subjected to air atmosphere at room temperature, and stimulated by a blue-light-emitting diode (LED) to harness renewable energy. The fundamental objective of this initiative is to utilize a photosensitizer (PS) biocatalyst that has been recently developed, can be conveniently acquired, and is priced affordably. The proflavine (PFH+) photocatalyst, demonstrates the ability to initiate photoinduced-electron transfer (PET) through exposure to visible light. This property endows the photocatalyst with a practical and efficient method of achieving high effectiveness, energy efficiency, and environmentally friendly outcomes. The current research endeavor has the objective of examining the turnover number (TON) and turnover frequency (TOF) pertaining to pyrano [2,3-d] pyrimidine scaffolds. Moreover, it has been validated that cyclization at the gram-scale is a feasible approach that can be employed in various industrial settings.
Introduction
The utilization of photoredox catalysis offers discernible merits towards sustainability, whilst simultaneously adhering to various tenets of green chemistry. The principal source of energy for light is radiation. Photonic radiation is characterized by its absence of charge, non-toxicity, and ecological compatibility, which renders it an efficient source of energy. The utilization of photons, due to their ability to furnish adequate energy, is a viable approach to attain the coveted reactivity, without resorting to the employment of extreme temperatures or rigorous conditions associated with thermal activation. The utilization of light-absorbing species, also known as photocatalysts, is a viable option for catalysis at low concentrations. The implementation of catalysis at low concentrations is known as the use of catalytic amounts. The term “reagents” refers to the chemical substances used in a reaction to cause a chemical change in the target molecule. By attaining an electronically excited state, they elicit occurrences of electron transfer (ET) either towards or away from inactive/stable substrates. The aforementioned phenomenon engenders notably active entities in a moderate and regulated fashion. There are two favorable outcomes in terms of sustainability that ensue from this situation. Initially, a plausible means of reducing reactivity involves employing low-energy reagents, thereby promoting less dangerous synthetic pathways and facilitating the elimination of less toxic or pollutant byproducts. Photoredox catalysis has the capability to activate typically unreactive groups within molecules. This study demonstrates the enhanced capacity for functional group tolerance exhibited by a particular chemical reaction that involves the cleavage of C-H bonds. Photoredox catalysis is an indispensable tool in the construction of concise synthetic routes with improved atom economies that utilize sustainable feedstock resources (Crisenza and Melchiorre, 2020; Mohamadpour, 2022a; Mohamadpour, 2022b; Mohamadpour, 2023a; Mohamadpour, 2023b).
Proflavine derivatives have garnered significant interest as chemotherapeutic agents owing to their diverse biological activities, which offer promising pharmaceutical potential (Sabolova et al., 2020). Proflavine, which was among the first identified antibacterial agents, was subsequently surpassed by the discovery of penicillin in the 20th century. The utilization of the agent persists in contemporary times as a potent disinfectant (Acheson, 1973). Extensive research on proflavine derivatives has resulted in their prevalent use as agents for antibacterial, anticancer, antimalarial, and antiprotozoal purposes (Denny et al., 1994; Denny, 2002). The significant function of flavins as essential photoreceptors and redox cofactors in various biological processes has subsequently fueled interest in the utilization of flavin derivatives as biomimetic photocatalysts (Ghisla and Massey, 1989; Silva and Edwards, 2006).
The photosensitizer (PS) is a fundamental component involved in the primary processes of light absorption and electron transfer in photocatalytic systems. According to prior reports, a majority of photocatalytic systems employ complexes containing precious metals such as Ru or Ir as a photosensitizer (PS). Unfortunately, the high cost associated with such precious metals has hindered their potential applications (Meng et al., 2021). Proflavine is identified as one of the flavine derivatives. The compound known as proflavine possesses a high degree of photosensitivity, thereby rendering it a subject of significant research interest. In particular, much attention has been devoted to the PFH+ strain of proflavine due to its remarkable photophysical and photochemical characteristics. In the present study, a cost-effective organic dye, namely proflavine (PFH+), characterized by its affordable pricing and relatively high photo absorption capacity (Meng et al., 2021; Ong et al., 2022), has been chosen to facilitate a facile synthesis of heterocyclic compounds through a sustainable and environmentally conscious route.
The considerable reserves of energy, cost-effectiveness along the capacity to access sustainable energy sources render visible light irradiation a dependable means for the development of organic compounds (Mohamadpour, 2021a; Mohamadpour, 2021b; Mohamadpour, 2022c).
It is anticipated that pyranopyrimidines will exhibit compelling pharmacological and biochemical properties, including inhibitor of the antiallergic (Kitamura and Onishi, 1984), antihypertensive (Furuya and Ohtaki, 1994), cardiotonic (Heber et al., 1993), bronchiodilator (Coates, 1990), antibronchitic (Sakuma et al., 1991), and antitumor activities (Broom et al., 1976).
Numerous catalysts have demonstrated the ability to generate synthetic pyrano [2,3-d] pyrimidine scaffolds, for instance, DABCO-based ionic liquids (Seyyedi et al., 2016), L-proline (Bararjanian et al., 2009), iron ore pellet (Sheihhosseini et al., 2016), nano-sawdust-OSO3H (Sadeghi et al., 2015), Al-HMS-20 (Sabour et al., 2015), TSA/B(OH)3 (Khazaei et al., 2015a), Mn/ZrO2 (Maddila et al., 2015), cellulose-based nanocomposite (Maleki et al., 2017), DBA (Bhat et al., 2016), TBAB (Mobinikhaledi and Bodaghi Fard, 2010), Fe3O4@SiO2@(CH2)3-Urea-SO3H/HCl (Zolfigol et al., 2016), Et3N-Ultrasonic (Azarifar et al., 2012), ZnFe2O4 nanoparticles (Khazaei et al., 2015b), microwave (Devi et al., 2003), nickel nanoparticles (Khurana and Vij, 2013), CaHPO4 (Bodaghifard et al., 2016), Zn [(L)proline]2 (Heravi et al., 2010), theophylline (Mohamadpour, 2021c), β-CD (Mohamadpour, 2022d), and CuO/ZnO nanocatalyst (Albadi et al., 2015). Numerous determinants, including extended reaction periods, utilization of expensive compounds, intricate chemical reactions, and reduced yields, have noteworthy implications for the management and disposal procedures of waste products. Additionally, the process of extracting homogeneous catalysts from reaction mixtures can pose a significant difficulty. Recently, the use of visible-light-induced photochemical reactions has attracted the attention of researchers and many advances have been made so far (Ma C. H. et al., 2022; Ma C. et al., 2022; Danfeng et al., 2022; Mohamadpour, 2022e; Mohamadpour, 2023c). The current investigation delineates the use of photocatalysts in the synthesis of heterocyclic compounds, with a particular emphasis on the incorporation of eco-friendly methodologies. Through the examination that was conducted, it was discovered that organic dye photo-redox catalysts can be easily obtained and are also economically viable. The technique discussed above entails the utilization of a photosensitizer (PS) biocatalyst as a highly effective organo-photocatalyst.
The present investigation has successfully discerned a new type of photosensitizer (PS) biocatalyst, denoted as proflavine (PFH+) as a photocatalyst that functions through a photoinduced-electron transfer (PET) pathway induced by visible light. The present protocol employs the Knoevenagel-Michael cyclocondensation reaction, which involves the application of barbituric acid/1,3-dimethylbarbituric, malononitrile, and aryl aldehydes. Moreover, the aforementioned process has the potential to utilize a blue-light-emitting-diode (LED) as a sustainable and environmentally conscious means of renewable sourcing energy in an air atmosphere at room temperature, within a water-based environment.
Experimental
General
The melting points of the diverse compounds were ascertained utilizing an electrothermal apparatus denoted as the 9100. The acquisition of 1HNMR spectra using DMSO-d6 was accomplished through the usage of the Bruker DRX-300 Avance instruments. The aforementioned compounds were generously supplied in significant amounts by Fluka, Merck, and Acros, and were promptly utilized.
The present study introduces a methodology for the sustainable synthesis of pyrano [2,3-d] pyrimidines with eco-friendly attributes (4a-t)
A solution consisting of 3 mL of water and 0.2 mol% of proflavine (PFH+) was prepared at room temperature. The mixture was afterward amalgamated with 1,3-dimethylbarbituric acid/barbituric acid (3, 1.0 mmol), malononitrile (2, 1.0 mmol), and aryl aldehydes (1, 1.0 mmol). The reactions were recorded utilizing thin-layer chromatography (TLC). After the chemical reaction, the crude solid underwent a screening process and was subsequently washed with water. The resulting product was then subjected to crystallization using ethanol, which effectively eliminated the need for any additional purification techniques. The current investigation concerns the possibility of synthesizing the aforementioned substances on a gram-scale, utilizing the domain of pharmaceutical research and development (R&D). A singular experimental procedure entailed the utilization of a combination consisting of 2,4-dimethoxybenzaldehyde, malononitrile, and barbituric acid at a molar quantity of 50 mmol. After a reaction time of 6 min, the resultant product was recovered through the use of a conventional filtration technique. According to the 1HNMR spectroscopy data, the chemical compound under investigation demonstrates a substantial level of spectroscopic purity. The spectroscopic data are presented in the Supplementary Material.
Results and discussion
The current investigation ascertained the response of benzaldehyde, malononitrile, and barbituric acid in a 3 mL aqueous solution. Under laboratory conditions, a 15-min incubation of 3 mL of water without the implementation of a photocatalyst resulted in the generation of 4i, which accounted for a proportion of 36%, at ambient temperature. Table 1, entry 8 provides a comprehensive record of the aforementioned observation. The addition of multiple adjunct photocatalysts augmented the reaction rate. The constituent substances depicted in Figure 1 are proflavine, acriflavine, lumichrome, riboflavin tetraacetate, and lumiflavin. The current methodology facilitates the generation of 4i with diversified rates of yield. The aforementioned findings have provided a basis for improved operational productivity within the context of proflavine. According to the information presented in Table 1, entry 1, a reaction involving 0.2 mol% of proflavine led to a yield of 93%. Table 2 showcases markedly subpar outcomes for THF, DCM, EtOH, MeOH, CH3CN, toluene, EtOAc, CHCl3, DMSO, and conditions devoid of solvents exhibited a noteworthy improvement in productivity and expedited the process. In the current study, the reaction within the H2O context was found to demonstrate a markedly elevated rate and consequential yield. The yield of 93% was attained as per the statistical information furnished in Table 2, with specific reference to entry 3. An assortment of light sources has been utilized in research endeavors pertaining to the evaluation of the effects of blue light on crop productivity, as evidenced in Table 2. During the assessment conducted in the absence of an illuminating apparatus, the existence of 4i was observed in a minuscule quantity. The current study provides evidence that the simultaneous presence of proflavine (PFH+) and visible light is a crucial requirement for achieving the successful synthesis of product 4i. To establish the most favorable setups, various grades of blue-light-emitting-diode (LED) intensities, namely, 10 W, 12 W, 18 W, and 24 W were utilized. This study’s results elucidate that the utilization of 18 W blue-light-emitting-diodes (LEDs) achieved the most advantageous outcomes. Several substrates were subjected to experiments under idealized conditions, as illustrated in Table 3 and Scheme 1. The incorporation of a benzaldehyde moiety was found to not significantly influence the ultimate reaction outcome. In the current chemical reaction, the replacement of the halide functional group was deemed to be an acceptable course of action. The present state of the reaction accommodates both reactions that entail functional groups possessing the ability to donate electrons and those that entail functional groups that display electron withdrawal properties. The potential productivity of ortho-, meta-, and para-substituted aromatic aldehydes is markedly heightened in the natural setting. The similarity in reactivity between barbituric acid and 1,3-dimethylbarbituric acid was observed.
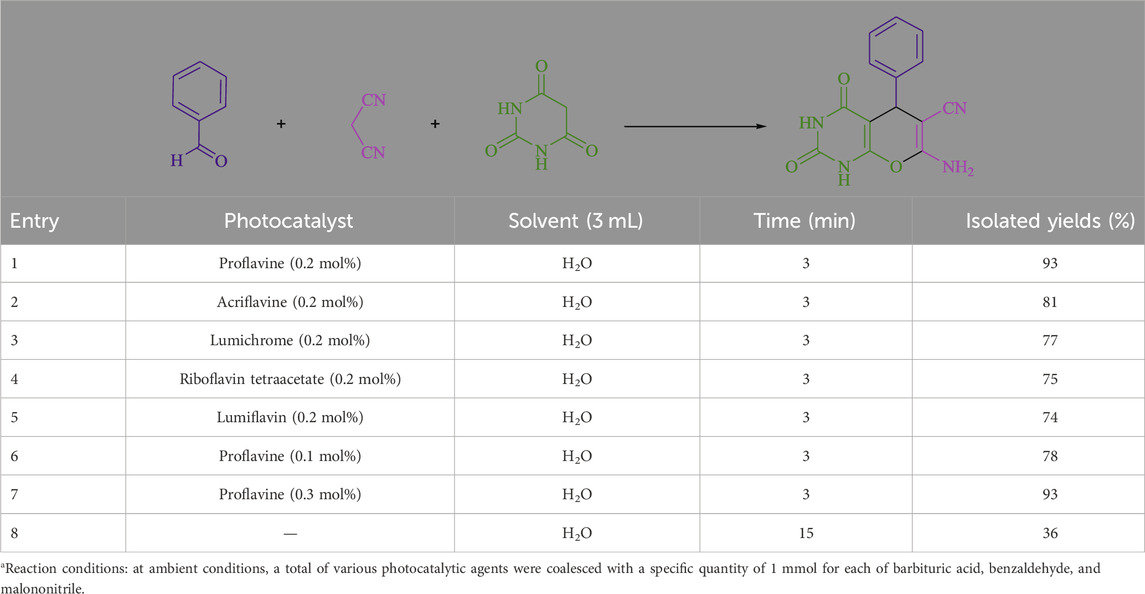
Table 1. The present work provides a table of optimized photocatalysts for the synthesis of 4ia.
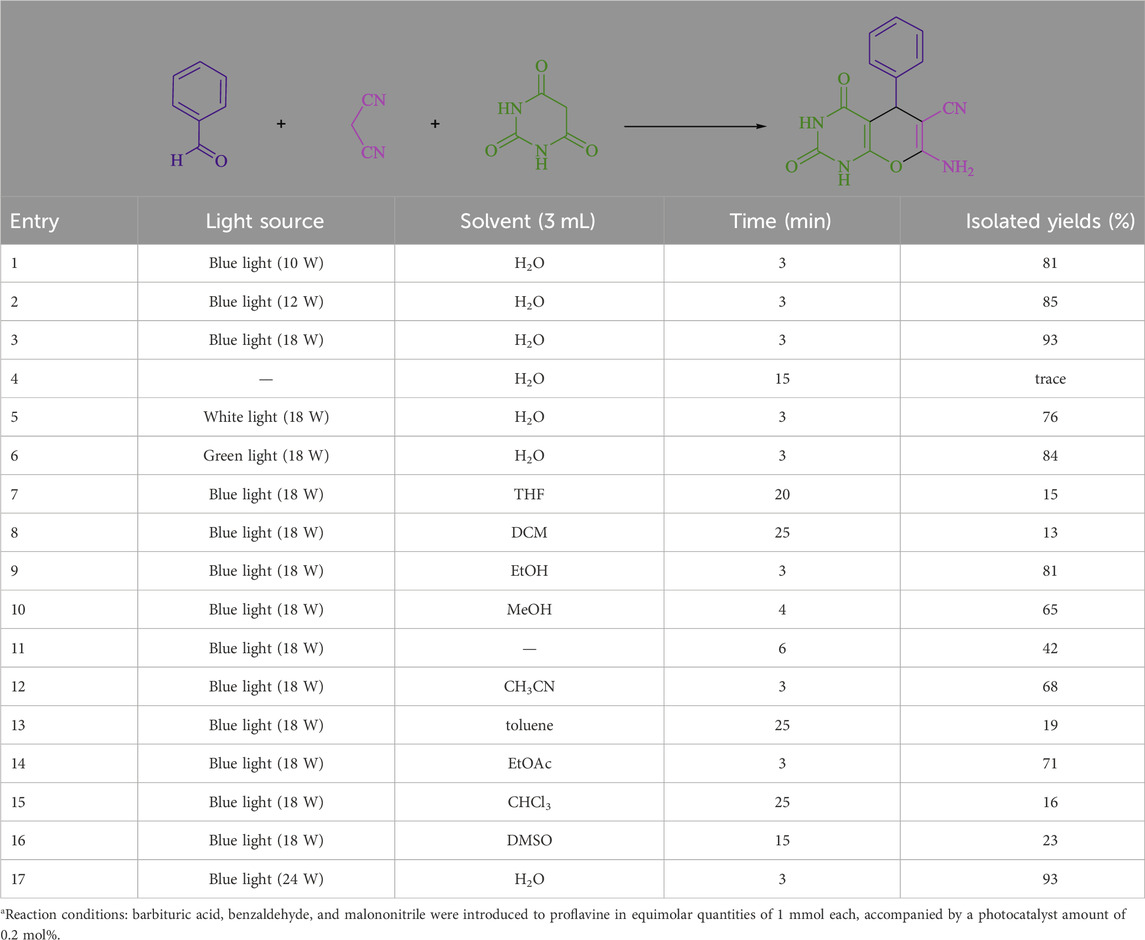
Table 2. The present study aims to investigate the optimization table of solvent and visible light conditions for the synthesis of 4ia.
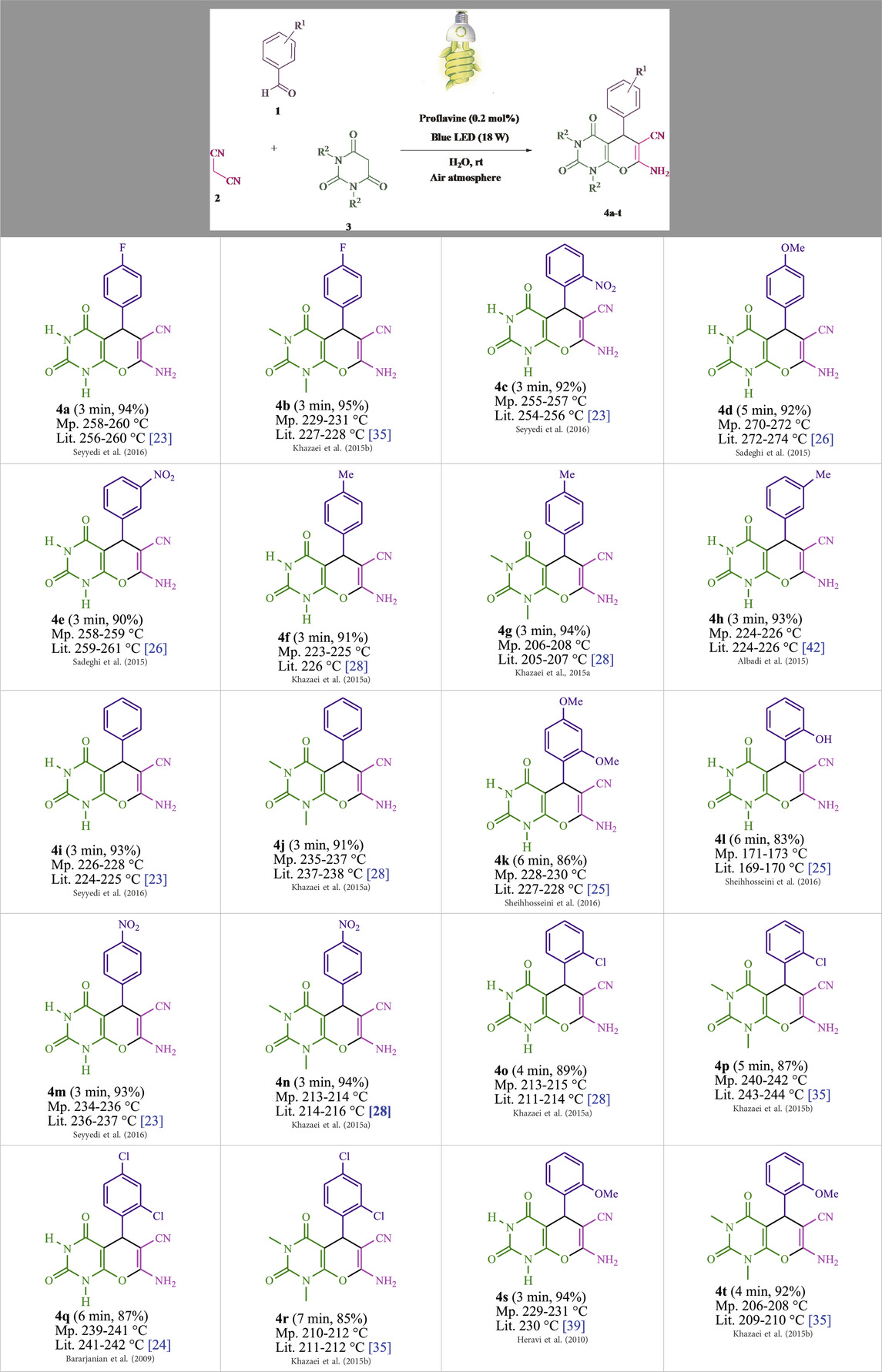
Table 3. The synthesis of pyrano [2,3-d]pyrimidine scaffolds is accomplished via the utilization of the photosensitizer (PS) biocatalyst; proflavine (PFH+).
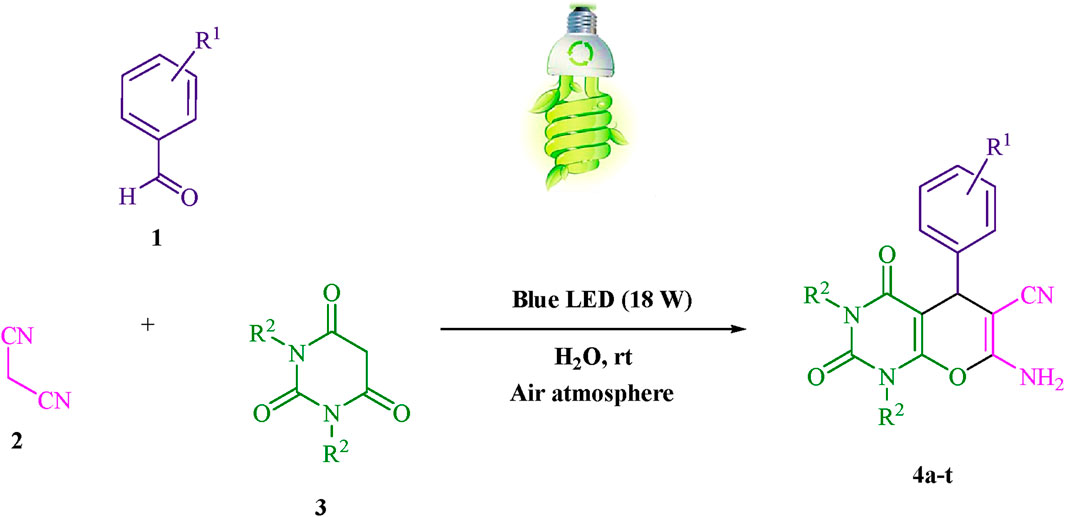
Scheme 1. The current study presents a methodology for the synthesis of pyrano [2,3-d] pyrimidine scaffolds in a radical manner.
The presented data in Table 4 conveys definitive measures of both turnover frequency (TOF) and turnover number (TON). In academic writing, the distinct types of yield, namely the Yield/Amount of catalyst (mol) and the Yield/Time/Amount of catalyst (mol), are commonly denoted as TON and TOF, respectively. Elevated turnover number (TON) and turnover frequency (TOF) values possess the potential to augment the efficiency of a catalyst system by decreasing the quantity of catalyst necessary to optimize desired yields. Concerning 4i, a TOF measuring 155 is deemed to be elevated, whereas a TON measuring 465 is also considered to be elevated.
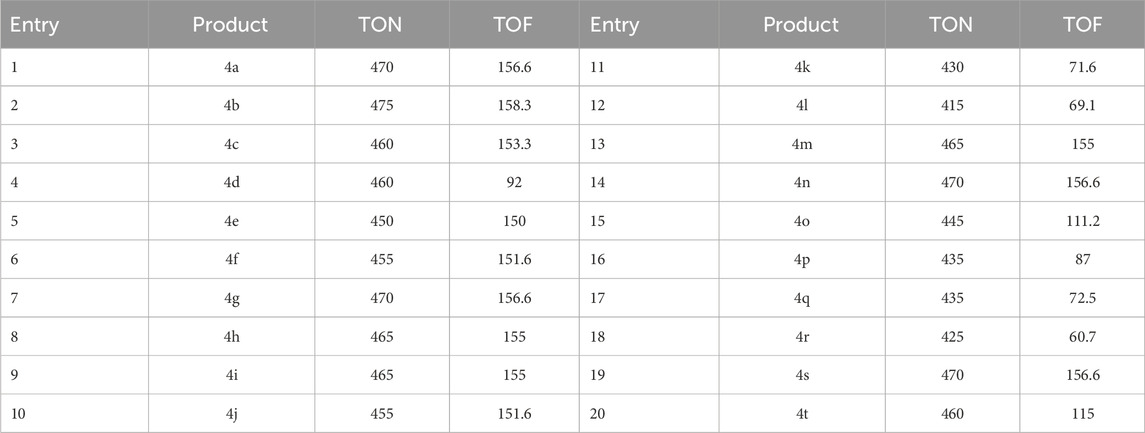
Table 4. The aim is to determine the numerical values of turnover number (TON) and turnover frequency (TOF) through rigorous analysis and evaluation.
The suggested mechanism
Scheme 2 illustrates a comprehensive depiction of the proposed methodology. The present study employs a photoinduced-electron transfer (PET) pathway in conjunction with the bio-photocatalyst; proflavine (PFH+), to create photocatalytic instruments capable of utilizing visible light energy in a sustainable manner. The implementation of visible light expedites the procedural course. The generation of the malononitrile radical is facilitated by a method that originates from the mechanism of photoinduced-electron transfer (PET), thereby enhancing the performance of the [PFH+]* and activated by visible light. The electron transfer process initiated by the radical adduct (C) and the radical anion of proflavine results in the formation of intermediates (D) as well as the ground state PFH+. The intermediate (F) is generated through a process of hydrogen atom abstraction from intermediate (E) by the malononitrile radical. This mechanism involves the formation of an intermediate (F) which is essential in the overall process. The intermediate species designated as (F) and (D) engage in a Michael acceptor reaction, resulting in the production of (G). Subsequently, the generation of (4) is induced by intramolecular cyclization and tautomerization mechanisms.
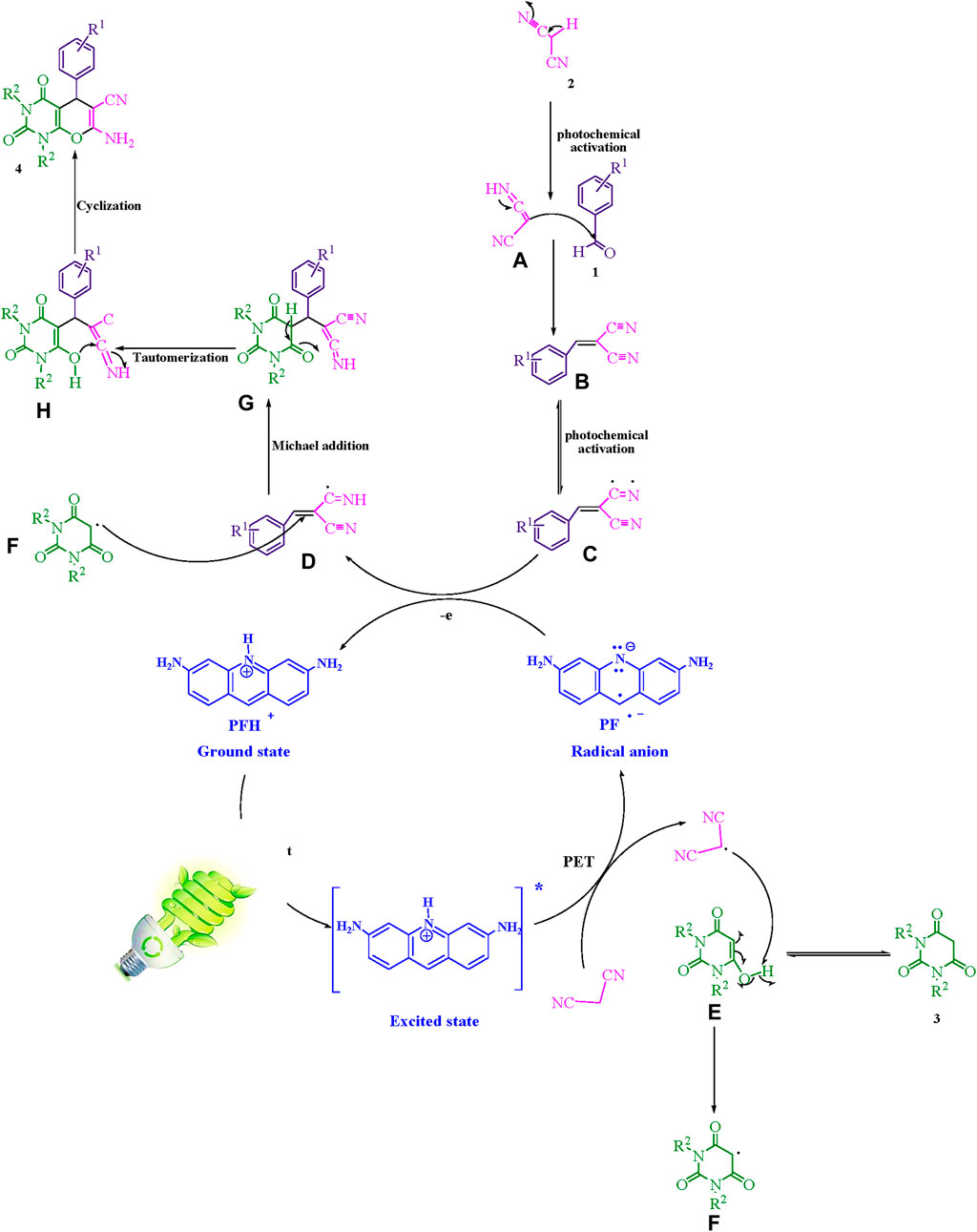
Scheme 2. This manuscript proposes a thorough exposition of the synthetic methodology employed to produce pyrano [2,3-d] pyrimidine scaffolds.
Table 5 provides a comparative evaluation of the efficiency of different catalysts in assisting the synthesis of pyrano [2,3-d] pyrimidines. The proposed methodology utilizes minuscule quantities of photocatalyst, facilitating rapid chemical conversions, while avoiding the generation of residual products. The aforementioned modality demonstrates the potential for utilization in circumstances where wavelengths of light can be feasibly observed. Atom-economy demonstrates significant efficacy and exerts considerable impact on the industrial sector at the scale of multigrams.
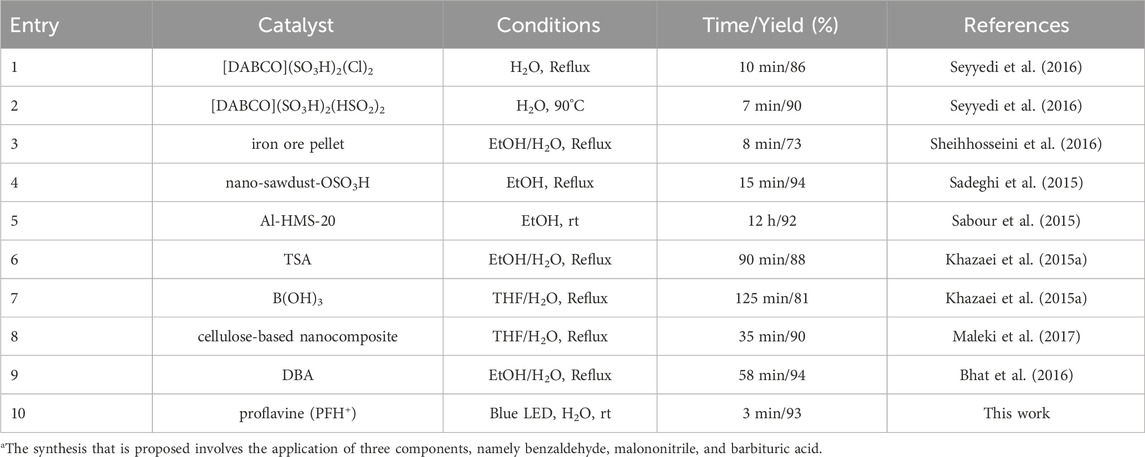
Table 5. The research at hand seeks to scrutinize the catalytic efficacy of various catalysts employed in the production of 4ia.
Conclusion
The Knoevenagel-Michael cyclocondensation reaction, which is initiated by radicals, has been applied in the green synthesis of pyrano [2,3-d] pyrimidine frameworks via the reaction of aryl aldehydes, malononitrile, and either barbituric acid or 1,3-dimethylbarbituric acid. The current investigation employed a novel photosensitizer (PS) biocatalyst; proflavine (PFH+), as an organo-photocatalyst via a photoinduced-electron transfer (PET). At ambient temperature and under air atmosphere conditions, the efficacy of blue-light-emitting-diode (LED) technology has been found to generate a sustainable mechanism of energy production in an aqueous environment. The method presented in this study confers several advantages to the domain of chemical synthesis. The advantages of this approach pertain to several aspects, including rapid reaction times, elimination of hazardous solvents, increased product yields, optimized reaction pathways, robust operating conditions, and utilization of sustainable energy sources. The employment of chromatography was not deemed indispensable for the process of separation. By safeguarding the outcome, it is possible to expedite a multigram-scale reaction involving various substrates. Henceforth, the proposed methodology can be effectively executed within a framework that adheres to the principles of sustaining long-term ecological and financial sustenance.
Data availability statement
The original contributions presented in the study are included in the article/Supplementary Material, further inquiries can be directed to the corresponding authors.
Author contributions
FM: Conceptualization, Data curation, Formal Analysis, Investigation, Methodology, Project administration, Resources, Software, Supervision, Validation, Visualization, Writing–original draft, Writing–review and editing. AA: Conceptualization, Data curation, Formal Analysis, Investigation, Methodology, Project administration, Resources, Software, Supervision, Validation, Visualization, Writing–original draft, Writing–review and editing.
Funding
The author(s) declare that no financial support was received for the research, authorship, and/or publication of this article.
Acknowledgments
This work is supported by Iran National Science Foundation (INSF) (no. 4015618), supported by Iran’s National Elites Foundation (no. 4015618), and also, Shiraz University of Medical Sciences.
Conflict of interest
The authors declare that the research was conducted in the absence of any commercial or financial relationships that could be construed as a potential conflict of interest.
Publisher’s note
All claims expressed in this article are solely those of the authors and do not necessarily represent those of their affiliated organizations, or those of the publisher, the editors and the reviewers. Any product that may be evaluated in this article, or claim that may be made by its manufacturer, is not guaranteed or endorsed by the publisher.
Supplementary material
The Supplementary Material for this article can be found online at: https://www.frontiersin.org/articles/10.3389/fchem.2024.1304850/full#supplementary-material
References
Acheson, R. M. (1973). The antibacterial action of acridines. 2nd ed. New York - London - Sydney - Toronto: Interscience Publishers, John Wiley & Sons, 789–814.
Albadi, J., Mansournezhad, A., and Sadeghi, T. (2015). Eco-friendly synthesis of pyrano [2,3-d] pyrimidinone derivatives catalyzed by a novel nanocatalyst of ZnO-supported copper oxide in water. Res. Chem. Intermed. 41, 8317–8326. doi:10.1007/s11164-014-1894-0
Azarifar, D., Nejat-Yami, R., Sameri, F., and Akrami, Z. (2012). Ultrasonic-Promoted One-Pot Synthesis of 4H-chromenes, pyrano[2,3-d]pyrimidines, and 4H-pyrano[2,3-c]pyrazoles. Lett. Org. Chem. 9, 435–439. doi:10.2174/157017812801322435
Bararjanian, M., Balalaei, S., Movassagh, B., and Amani, A. M. (2009). One-pot synthesis of pyrano [2, 3-d] pyrimidinone derivatives catalyzed by L-proline in aqueous media. J. Iran. Chem. Soc. 6, 436–442. doi:10.1007/BF03245854
Bhat, A. R., Shalla, A. H., and Dongre, R. S. (2016). Dibutylamine (DBA): a highly efficient catalyst for the synthesis of pyrano [2, 3-d] pyrimidine derivatives in aqueous media. J. Taibah Univ. Sci. 10, 9–18. doi:10.1016/j.jtusci.2015.03.004
Bodaghifard, M. A., Solimannejad, M., Asadbegi, S., and Dolatabadifarahani, S. (2016). Mild and green synthesis of tetrahydrobenzopyran, pyranopyrimidinone and polyhydroquinoline derivatives and DFT study on product structures. Res. Chem. Intermed. 42, 1165–1179. doi:10.1007/s11164-015-2079-1
Broom, A. D., Shim, J. L., and Anderson, G. L. (1976). Pyrido[2,3-d]pyrimidines. IV. Synthetic studies leading to various oxopyrido[2,3-d]pyrimidines. J. Org. Chem. 41, 1095–1099. doi:10.1021/jo00869a003
Crisenza, G. E., and Melchiorre, P. (2020). Chemistry glows green with photoredox catalysis. Nat. Commun. 11, 803. doi:10.1038/s41467-019-13887-8
Danfeng, W., Jin, W., Chunhua, M., Yuqin, J., and Bing, Y. (2022). C-3 functionalization of 2-aryl-2H-indazoles under photo/electrocatalysis. Chin. J. Org. Chem. 42, 4024–4036. doi:10.6023/cjoc202208039
Denny, W. A. (2002). Acridine derivatives as chemotherapeutic agents. Curr. Med. Chem. 9, 1655–1665. doi:10.2174/0929867023369277
Denny, W. A., and Bagulley, B. C. (1994). in Molecular aspects of anticancer drug-DNA interactions. Editors S. Neidle, and M. Waring (London: Palgrave Chapter), 7, 270–311. doi:10.1007/978-1-349-13330-7
Devi, I., Kumar, B. S. D., and Bhuyan, P. J. (2003). A novel three-component one-pot synthesis of pyrano [2, 3-d] pyrimidines and pyrido [2, 3-d] pyrimidines using microwave heating in the solid state. Tetrahedron Lett. 44, 8307–8310. doi:10.1016/j.tetlet.2003.09.063
Ghisla, S., and Massey, V. (1989). Mechanisms of flavoprotein-catalyzed reactions. Eur. J. Biochem. 181, 1–17. doi:10.1111/j.1432-1033.1989.tb14688.x
Heber, D., Heers, C., and Ravens, U. (1993). Positive inotropic activity of 5-amino-6-cyano-1,3-dimethyl-1,2,3,4-tetrahydropyrido[2,3-d]pyrimidine-2,4-dione in cardiac muscle from Guinea-pig and man. Part 6: compounds with positive inotropic activity. Die Pharm. 48, 537–541.
Heravi, M. M., Ghods, A., Bakhtiari, K., and Derikvand, F. (2010). Zn[(L)proline]2: an efficient catalyst for the synthesis of biologically active pyrano[2,3-d]pyrimidine derivatives. Synth. Commun. 40, 1927–1931. doi:10.1080/00397910903174390
Khazaei, A., Alavi Nik, H. A., and Moosavi-Zare, A. R. (2015a). Water mediated domino knoevenagel-michael-cyclocondensation reaction of malononitrile, various aldehydes and barbituric acid derivatives using boric acid aqueous solution system compared with nano-titania sulfuric acid. J. Chin. Chem. Soc. 62, 675–679. doi:10.1002/jccs.201500115
Khazaei, A., Ranjbaran, A., Abbasi, F., Khazaei, M., and Moosavi-Zare, A. R. (2015b). Synthesis, characterization and application of ZnFe2O4 nanoparticles as a heterogeneous ditopic catalyst for the synthesis of pyrano[2,3-d] pyrimidines. RSC Adv. 5, 13643–13647. doi:10.1039/c4ra16664g
Khurana, J. M., and Vij, K. (2013). Nickel nanoparticles as semiheterogeneous catalyst for one-pot, three-component synthesis of 2-amino-4H-pyrans and pyran annulated heterocyclic moieties. Synth. Commun. 43, 2294–2304. doi:10.1080/00397911.2012.700474
Ma, C., Meng, H., Li, J., Yang, X., Jiang, Y., and Yu, B. (2022b). Photocatalytic transition-metal-free direct 3-acetalation of quinoxaline-2(1H)-ones. Chin. J. Chem. 40, 2655–2662. doi:10.1002/cjoc.202200386
Ma, C. H., Zhao, L., He, X., Jiang, Y. Q., and Yu, B. (2022a). Visible-light-induced direct 3-ethoxycarbonylmethylation of 2-aryl-2H-indazoles in water. Org. Chem. Front. 9, 1445–1450. doi:10.1039/D1QO01870A
Maddila, S. N., Maddila, S., van Zyl, W. E., and Jonnalagadda, S. B. (2015). Mn doped ZrO2 as a green, efficient and reusable heterogeneous catalyst for the multicomponent synthesis of pyrano [2, 3-d]-pyrimidine derivatives. RSC Adv. 5, 37360–37366. doi:10.1039/C5RA06373F
Maleki, A., Jafari, A. A., and Yousefi, S. (2017). Green cellulose-based nanocomposite catalyst: design and facile performance in aqueous synthesis of pyranopyrimidines and pyrazolopyranopyrimidines. Carbohydr. Polym. 175, 409–416. doi:10.1016/j.carbpol.2017.08.019
Meng, X., Chen, X., Sun, W., and Gao, Y. (2021). Highly efficient photocatalytic CO2 reduction with an organic dye as photosensitizer. Inorg. Chem. Commun. 129, 108617. doi:10.1016/j.inoche.2021.108617
Mobinikhaledi, A., and Bodaghi Fard, M. A. (2010). Tetrabutylammonium bromide in water as a green media for the synthesis of pyrano[2,3-d]pyrimidinone and tetrahydrobenzo[b]pyran derivatives. Acta Chim. Slov. 57, 931–935.
Mohamadpour, F. (2021a). Catalyst-free, visible light irradiation promoted synthesis of spiroacenaphthylenes and 1H-pyrazolo[1,2-b]phthalazine-5,10-diones in aqueous ethyl lactate. J. Photochem. Photobiol. A Chem. 407, 113041. doi:10.1016/j.jphotochem.2020.113041
Mohamadpour, F. (2021b). Catalyst-free and solvent-free visible light irradiation-assisted Knoevenagel–Michael cyclocondensation of aryl aldehydes, malononitrile, and resorcinol at room temperature. Monatsh. für Chemie-Chemical Mon. 152, 507–512. doi:10.1007/s00706-021-02763-1
Mohamadpour, F. (2021c). Synthesis of pyran-annulated heterocyclic systems catalyzed by theophylline as a green and bio-based catalyst. Polycycl. Aromat. Compd. 41, 160–172. doi:10.1080/10406638.2019.1575246
Mohamadpour, F. (2022a). The development of imin-based tandem Michael–Mannich cyclocondensation through a single-electron transfer (SET)/energy transfer (EnT) pathway in the use of methylene blue (MB+) as a photo-redox catalyst. RSC Adv. 12, 10701–10710. doi:10.1039/D2RA01190E
Mohamadpour, F. (2022b). Visible-light-induced radical condensation cyclization to synthesize 3, 4-dihydropyrimidin-2-(1H)-ones/thiones using photoexcited Na2 eosin Y as a direct hydrogen atom transfer (HAT) catalyst. ACS omega 7, 8429–8436. doi:10.1021/acsomega.1c05808
Mohamadpour, F. (2022c). Catalyst-Free and solvent-free visible light assisted synthesis of tetrahydrobenzo[b]Pyran scaffolds at room temperature. Polycycl. Aromat. Compd. 42, 7607–7615. doi:10.1080/10406638.2021.2006244
Mohamadpour, F. (2022d). Supramolecular β-cyclodextrin as a biodegradable and reusable catalyst promoted environmentally friendly synthesis of pyrano[2,3-d]pyrimidine ScaffoldsviaTandem knoevenagel–michael–cyclocondensation reaction in aqueous media. Polycycl. Aromat. Compd. 42, 2805–2814. doi:10.1080/10406638.2020.1852274
Mohamadpour, F. (2022e). The development of Friedländer heteroannulation through a single electron transfer and energy transfer pathway using methylene blue (MB+). Sci. Rep. 12, 7253. doi:10.1038/s41598-022-11349-8
Mohamadpour, F. (2023a). Carbazole-based photocatalyst (4CzIPN) as a novel donor-acceptor (DA) fluorophore catalyzed gram-scale 2-amino-4H-chromene scaffolds photosynthesis via a proton-coupled electron transfer (PCET) process. J. Taiwan Inst. Chem. Eng. 144, 104699. doi:10.1016/j.jtice.2023.104699
Mohamadpour, F. (2023b). Radical synthesis of dihydropyrano[2,3-c]pyrazoles using acridine yellow G as a photo-induced electron transfer photocatalyst. J. Heterocycl. Chem. 60, 504–512. doi:10.1002/jhet.4607
Mohamadpour, F. (2023c). Acridine yellow G as a photo-induced electron transfer catalyzed radical metal-free synthesis of tetrahydrobenzo[b]pyran scaffolds in an aqueous media. Curr. Res. Green Sustain. Chem. 6, 100356. doi:10.1016/j.crgsc.2023.100356
Ong, J., Loke, J. W., Koh, H. L., and Fan, W. Y. (2022). Proflavine-catalysed trifluoromethylation of α,β-unsaturated carbonyls. Mol. Catal. 530, 112587. doi:10.1016/j.mcat.2022.112587
Sabolova, D., Kristian, P., and Kozurkova, M. (2020). Proflavine/acriflavine derivatives with versatile biological activities. J. Appl. Toxicol. 40, 64–71. doi:10.1002/jat.3818
Sabour, B., Hassan Peyrovi, M., and Hajimohammadi, M. (2015). Al-HMS-20 catalyzed synthesis of pyrano [2, 3-d] pyrimidines and pyrido [2, 3-d] pyrimidines via three-component reaction. Res. Chem. Intermed. 41, 1343–1350. doi:10.1007/s11164-013-1277-y
Sadeghi, B., Bouslik, M., and Shishehbore, M. R. (2015). Nano-sawdust-OSO3H as a new, cheap and effective nanocatalyst for one-pot synthesis of pyrano [2, 3-d] pyrimidines. J. Iran. Chem. Soc. 12, 1801–1808. doi:10.1007/s13738-015-0655-3
Sakuma, Y., Hasegawa, M., Kataoka, K., Hoshina, K., Yamazaki, N., Kadota, T., et al. (1991). WO 91/05785 PCT int. Appl., 1989Chem. Abstr, 115, 71646.
Seyyedi, N., Shirini, F., Safarpoor, M., and Langarudi, N. (2016). DABCO-based ionic liquids: green and recyclable catalysts for the synthesis of barbituric and thiobarbituric acid derivatives in aqueous media. RSC Adv. 6, 44630–44640. doi:10.1039/C6RA05878G
Sheihhosseini, E., Sattaei Mokhatari, T., Faryabi, M., Rafiepour, A., and Soltaninejad, S. (2016). Iron ore pellet, A natural and reusable catalyst for synthesis of pyrano [2, 3-d] pyrimidine and dihydropyrano[c]chromene derivatives in aqueous media. Iran. J. Chem. Chem. Eng. 35, 43–50.
Silva, E., and Edwards, A. M. (2006). Flavins: photochemistry and photobiology, 6. Cambridge: Royal Society of Chemistry.
Keywords: proflavine (PFH+), photosensitizer (PS) biocatalyst, photoinduced-electron transfer (PET), renewable energy source, aqueous media, pyrano [2, 3-d] pyrimidine scaffolds
Citation: Mohamadpour F and Amani AM (2024) Proflavine (PFH+): as a photosensitizer (PS) biocatalyst for the visible-light-induced synthesis of pyrano [2,3-d] pyrimidine scaffolds. Front. Chem. 12:1304850. doi: 10.3389/fchem.2024.1304850
Received: 30 September 2023; Accepted: 22 February 2024;
Published: 26 March 2024.
Edited by:
James Clark, University of York, United KingdomCopyright © 2024 Mohamadpour and Amani. This is an open-access article distributed under the terms of the Creative Commons Attribution License (CC BY). The use, distribution or reproduction in other forums is permitted, provided the original author(s) and the copyright owner(s) are credited and that the original publication in this journal is cited, in accordance with accepted academic practice. No use, distribution or reproduction is permitted which does not comply with these terms.
*Correspondence: Farzaneh Mohamadpour, f_mohamadpour@sums.ac.ir, mohamadpour.f.7@gmail.com; Ali Mohammad Amani, amani_a@sums.ac.ir, aliamani@sums.ac.ir