Research progress in the detection of trace heavy metal ions in food samples
- State Key Laboratory of Chemical Resource Engineering, College of Chemistry, Beijing Advanced Innovation Center for Soft Matter Science and Engineering, Beijing University of Chemical Technology, Beijing, China
Food safety is the basis for ensuring human survival and development. The threat of heavy metal ions to food safety has become a social concern with the rapid growth of the economy and the accompanying environmental pollution. Some heavy metal ions are highly toxic even at trace levels and pose significant health risks to humans. Therefore, ultrasensitive detection of heavy metal ions in food samples is important. In this mini-review, recent advances in the analytical methods based on nanomaterials for detecting trace heavy metal ions in food samples are summarized in three categories: electrochemical, colorimetric, and fluorescent methods. We present the features and sensing mechanisms of these three methods, along with typical examples to illustrate their application in the detection of heavy metal ions in foods. This mini-review ends with a discussion of current challenges and future prospects of these approaches for sensing heavy metal ions. The review will help readers understand the principles of these methods, thereby promoting the development of new analytical methods for the detection of heavy metal ions in food samples.
1 Introduction
Food is the basis for human beings to maintain nutritional balance, carry out growth and development, and maintain physical health. Over the past decades, the development of the modern economy, wastewater irrigation, improper disposal of industrial wastes, and abuse of agrochemicals have led to the massive accumulation of heavy metal ions in agricultural soil and surface water (Ai et al., 2018; Mukherjee et al., 2021; Lai et al., 2023). Heavy metal ions have a long half-life, cannot be naturally degraded, and are enriched in living organisms (Keyster et al., 2020). Heavy metal ions in soil and surface water can easily accumulate in agricultural products through the biological chain. Heavy metal ions can reach a high level in the human body by eating and drinking, endangering human health (Zhang et al., 2017; Meng et al., 2023). Hence, heavy metal ions have become one of the most serious environmental and ecological pollutants.
Heavy metals are broadly defined as metallic elements with a relatively high density (>5 g/cm3). Toxic heavy metal elements mainly refer to mercury (Hg), cadmium (Cd), lead (Pb), chromium (Cr), arsenic (As), zinc (Zn), and et al. (Bansod et al., 2017). Among them, As is a non-metallic element, but it is listed as a toxic heavy element based on its chemical properties and toxicity. In general, even at relatively low concentrations, heavy metal ions can interact with nitrogen, sulfur, and oxygen of the biomolecules such as proteins and DNA. The interactions lead to disruption of the structure and function of these biomolecules, and ultimately inducing irreversible chronic or acute intoxication of the organisms (Shen et al., 2022). For example, Cd(II) disrupts iron homeostasis by inducing hyperactivation of heme oxygenase-1 and harassing lipid metabolism, ultimately leading to iron death (Zeng et al., 2021). Even at ultra-low daily doses, Cd(II) may gradually bioaccumulate along the food chain. The terminal half-life of Cd(II) in human organs is about 10–30 years (Guo et al., 2023). High levels of Cd(II) can cause kidney and liver damage and even induce cancer (Qin et al., 2021). Another highly toxic heavy metal ion is Pb(II), which damages almost all organs in the body (Hemmaphan and Bordeerat, 2022). The guideline value of the World Health Organization for the concentration of Pb(II) in drinking water is 4.83 × 10−8 M. Even if the concentration of Pb(II) in human blood is less than 5 μg/dL, it may trigger mental decline and behavioral difficulties in children. In short, heavy metal ions enter the human body along the food chain by contaminating soil, food, and water, ultimately leading to neurological disorders, digestive disorders, liver and kidney damage, immune system dysfunction, as well as cancer. Monitoring technology is the basis for preventing and combating heavy metal pollution. The quantity of heavy metal ions in foods is generally in the trace range. The maximum limit for heavy metal contamination in foods has been reported to be 0.02 mg/kg (Rachmawati et al., 2024). Therefore, the development of sensitive and efficient analytical methods for the determination of trace heavy metal ions in food samples is important for human health.
A wide variety of techniques have been applied to detect heavy metal ions in foods (Yang et al., 2023). The analytical methods have undergone a process of development from traditional analytical methods to instrumental analytical methods. Conventional analytical methods include inductively coupled plasma-mass spectrometry (ICP-MS) (Shih et al., 2019), atomic absorption spectrometry (AAS) (Kartoğlu et al., 2024) and gas chromatography-mass spectrometry (GC-MS) (Nozari et al., 2023). Many of these techniques offer excellent selectivity and detection limits and are capable of simultaneously measuring multiple heavy metal ions (Ding et al., 2021). However, these traditional analytical methods are complex to operate, requiring expensive and large analytical instruments, as well as experienced researchers (Yu et al., 2021). These methods are not suitable for rapid screening and on-site detection of trace heavy metal ions in foods. To solve these problems, many new analytical methods have been reported, such as electrochemical methods (Pan et al., 2024), colorimetric methods (Sharifi et al., 2022), and fluorescence methods (Chen et al., 2022). Compared with traditional analytical methods, these methods have the advantages of simple operation, high sensitivity, and high accuracy (Lim et al., 2021). Therefore, in the following sections, this mini-review highlights recent advances in these three analytical techniques (electrochemical, colorimetric, and fluorescent methods) for the detection of trace heavy metal ions in food samples from 2021 to 2024. In the end of the review, current challenges and future prospects of these approaches for sensing applications are briefly discussed.
2 Electrochemical sensing
Electrochemical methods are analytical methods based on the electrochemical properties of measured substances in solution and at electrodes. An electrochemical sensing system usually consists of a counter electrode, a reference electrode, and a working electrode (Sardesai et al., 2020). The system indirectly detects heavy metal ions by measuring physical quantities such as electrode potential, charge, current, and conductivity. The principle of the electrochemical sensor is shown in Figure 1A (Amali et al., 2021). In chemical composition analysis, electrochemical analysis is a fast, sensitive, accurate, simple, and low-cost method for micro and trace analysis. Important progress has been made in the development of new electrode modification materials for the electrochemical detection of trace heavy metal ions, among which a series of complexes, such as MOF and MXene, have been widely used (Li et al., 2021).
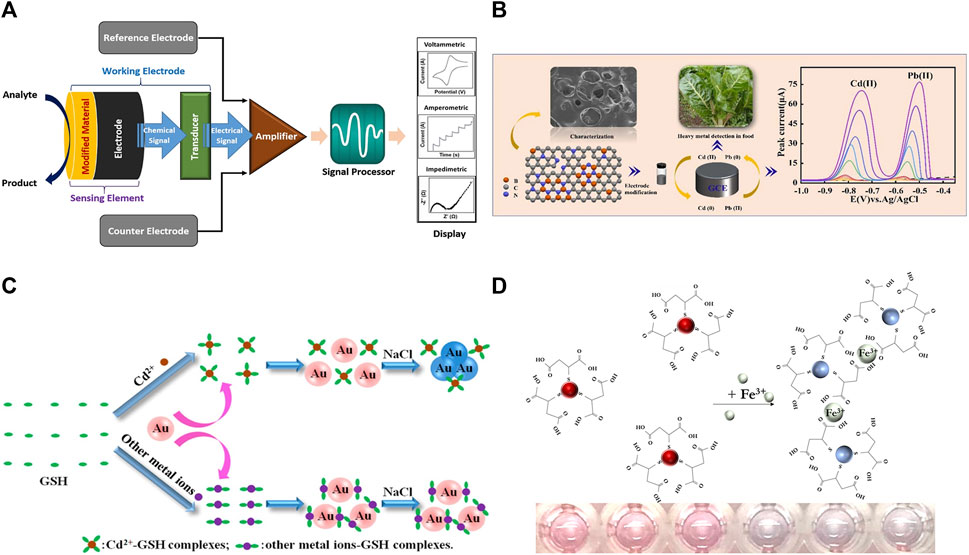
Figure 1. (A) Schematic diagram of an electrochemical sensor, reproduced with permission from (Amali et al., 2021). (B) Schematic diagram of BCN-Nafion/GCE detection of Cd(II) and Pb(II) in food samples, reproduced with permission from (Huang et al., 2023). (C) Schematic representation of the colorimetric detection of heavy metal ions using AuNPs, reproduced with permission from (Guo et al., 2014). (D) Scheme of colorimetric detection of Fe(III) ions using MSA-AuNPs, reproduced with permission from (Komova et al., 2021).
Metal-organic frameworks (MOFs) are an extremely versatile type of super porous nanomaterials. With excellent chemical properties, rich and tunable microporous structure, and large specific surface area, MOFs showed unique advantages in the adsorption of heavy metal ions (Wang et al., 2022; Zhang et al., 2022). Researchers (Ma et al., 2024) synthesized a novel thiacalix [4]arene-based metal-organic framework Co-LTPA. Co-LTPA/GCE had a strong binding affinity for heavy metal ions and was used for the detection of Cd(II) and Pb(II) in foods (milk, honey, and orange juice), with a limit of detection (LOD) of 0.119 and 0.279 nM, respectively. MOFs were complexed with inorganic materials (such as carbon-based materials, metals, and metal oxides) to further improve the performance of electrode materials. For example, Dong et al. (2023) developed a sensing platform by integrating the material of MWCNTs and CeMOF for the simultaneous determination of Cd(II) and Pb(II) in grain and water samples, with the LOD of 2.2 and 0.64 ppb, respectively. Similarly, Huo et al. (2022) developed an electrochemical sensing platform that could simultaneously detect Cd(II), Pb(II), Cu(II), and Hg(II). The electrode was modified with a metal-organic backbone (UiO-66-NH2) and a carbon-based material, three-dimensional graphene (3DGO). UiO-66-NH2 had a large specific surface area and porous structure. The abundant amino groups on the surface of UiO-66-NH2 could bind with Cd(II), Pb(II), Cu(II), and Hg(II). 3DGO could increase the electron transfer rate and further improve the electrochemical properties of the composites. The 3DGO/UiO-66-NH2 electrochemical sensor was capable of simultaneously quantifying these heavy metal ions in food samples (rice, milk, and honey samples). Pang et al. (2023) modified amino-functionalized Co-based metal organoskeletons (Co-MOF-NH2) on electrodes deposited with gold nanoparticles (AuNPs) for the detection of Pb(II) and Cd(II). The developed Co-MOF-NH2/AuNPs/CPE could be used to detect real food samples (drinking water, juice, tea, grain, fruits, vegetables, liver and aquatic products), with the LOD of 7.0 × 10−2 and 1.1 × 10−2 ng mL−1 for Pb(II) and Cd(II), respectively. In order to further improve the reliability of the sensors and minimize the influence from the complex environment, ratiometric electrochemical sensors were developed (Yang et al., 2018). Wang et al. (2024) realized the proportional detection of Pb(II) and Hg(II) using differential pulse voltammetry signals of carbon dots (CDs) and UiO-66-CNTs as response and reference signals. The sensor could be applied for the detection of Pb(II) and Hg(II) in edible vegetables.
Zeolitic imidazolate frameworks (ZIFs) are a special class of MOF materials. Surface functionalization and heteroatom doping of ZIFs are important ways to improve their physicochemical properties. As illustrated in Figure 1B, Huang et al. (2023) prepared a boron and nitrogen co-doped porous carbon material (BCN) to improve the physicochemical properties of ZIF-8. The BCN-Nafion/GCE could be used for the detection of trace Cd(II) and Pb(II) in Beta vulgaris var. cicla L samples. Moreover, two-dimensional antimonene could be synthesized on the surface of ZIFs to improve the stability and biocompatibility of electrode materials. Zhang et al. (2023) prepared an electrochemical sensor based on ZIF-67@AMNFs by loading antimonene sheet on the surface of ZIF-67 MOF. ZIF-67@AMNFs electrochemical sensor was used for the simultaneous determination of Cd(II), Pb(II), and Hg(II) in milk, honey, and tea samples with the LOD of 0.01, 0.042, and 0.031 pM, respectively.
Novel transition metal carbides/carbonitrides (MXenes) have attracted researchers in the field of electrochemistry. Among all the discovered MXenes, titanium carbide (Ti3C2Tx) aroused great attention (Qiu et al., 2022). Chen et al. (2023a) prepared amino-functionalized multilayer titanium carbide (NH2-Ti3C2Tx). NH2-Ti3C2Tx was used to detect Cd(II) and Pb(II) in food samples including rice, wheat, sorghum and corn, with the LOD of 0.41 and 0.31 μg L−1, respectively. Researchers modified the composition and structure of MXene material to further enhance the electrode properties (Yu et al., 2019). A new strategy was applied to create highly interconnected porous structures, assembling 2D MXene nanosheets into 3D structures. Chen et al. (2023b) combined reduced graphene oxide (rGO) with melamine-doped MXene to obtain 3D composite aerogels with good electrochemical properties. The 3D melamine/MXene/rGO composite aerogel modified SPCE electrode could simultaneously detect Zn(II), Cd(II), and Pb(II) in foods (sorghum, rice, wheat and corn), with the LOD of 0.48, 0.45, and 0.29 μg L−1, respectively. Wen et al. (2022) constructed a MXA-CuO/CC electrochemical sensor for the simultaneous detection of Cd(II) and Pb(II) by combining MXene aerogels and CuO on the surface of flexible carbon cloth. In addition, this work utilized the synergistic adsorption of oxygen vacancies and Bi (III) to enhance the performance of the sensor. MXA-CuO/CC showed high reliability and efficiency for the detection of Cd(II) and Pb(II) in grain. Dong et al. (2024) coupled UiO-66-NH2 and MXene-rGO aerogel to prepare a novel sensing electrode for the simultaneous detection of ultra-trace Cd(II) and Pb(II) in grain and water samples.
3 Colorimetric sensing
The naked-eye detectable color change is an attractive feature of colorimetric sensors, and colorimetry can be detected quickly and conveniently (Nazifi et al., 2021). Heavy metal ions undergo a series of reactions with the substances, resulting in a change in the color of the solution. Qualitative and quantitative detection of heavy metal ions can be achieved by visual analysis or using an ultraviolet-visible spectrophotometer. Since each colorimetric material has its unique optical and chemical properties, they have completely different sensing mechanisms. In recent years, colorimetric sensors based on metal nanomaterials and nanoenzymes have been widely used for the detection of heavy metal ions.
The metal nanoparticles as colorimetric signaling probes has been applied on the basis of their localized surface plasmon resonance (Sönnichsen et al., 2005). The reasons that localized surface plasmon resonance sensor can cause color changes for two reasons. On the one hand, heavy metal ions can induce aggregation or dispersion of nanoparticles; on the other hand, heavy metal ions can induce morphological changes of nanoparticles. The basic principle is shown in Figure 1C (Guo et al., 2014). AuNPs are easily functionalized, visualized and coordinable than other metal nanoparticles, AuNPs have been extensively investigated for the detection of heavy metal ions with colorimetric signals. Hua et al. (2023) developed two convenient, instrument-free new strategies for the instantaneous determination of Cu(II) and Hg(II). The analytes promoted the reduction of chloroauric acid to different forms of AuNPs, resulting in different color variations. The nanosensors could be applied to detect Cu(II) and Hg(II) in drinking water with the LOD of 3.5 and 0.1 nM, respectively. Bhattacharyya and Hossain (2024) synthesized LGC-AuNPs using Lannea Grandis Coromandelica bark extract as reducing and stabilizing agent. The LGC-AuNPs-based sensor could produce color changes within 20 s for speedy and sensitive response to Zn(II) and Hg(II). LGC-AuNPs probe was used for the accurate detection of Zn(II) and Hg(II) in drinking water with extremely low detection limits. Some organic molecules were commonly used for the functionalization of metal nanoparticles to recognize heavy metal ions. As illustrated in Figure 1D, researchers (Komova et al., 2021) developed a simple method to prepare mercaptosuccinic-acid-functionalized gold nanoparticles (MSA-AuNPs). When Fe(III) was present, the functional groups (MSA) on the surface of AuNPs bind with these metal ions to form a large number of cross-linked structures, resulting in the aggregation of AuNPs, along with the color of the solution changing from red to blue-gray. MSA-AuNPs can be used to determine Fe(III) in drinking water, with a detection limit lower than the maximum allowable concentration for drinking water defined by the World Health Organization.
Recently, rapid detection of heavy metal ions based on nanozymes showed great potential. Nanozymes are a class of nanomaterials with biocatalytic function, which has the advantages of stable performance and are easy to be mass-produced (Jiang et al., 2019; Li et al., 2019). Hu et al. (2024) explored a colorimetric method based on a phosphatase mimic (MPA-CeO2) to analyze toxic Hg(II). When Hg(II) was present, the catalytically hydrolysis of colorless p-nitrophenyl phosphate to yellow p-nitrophenol by MPA-CeO2 was blocked. The method achieved the detection of Hg(II) in milk samples with the LOD as low as 0.16 nM. However, structural diversity and uneven elemental distribution of nanozymes resulted in low activity of nanozymes. To solve the problem, novel single atom nanozymes (SAzymes) have been continuously developed (Cheng et al., 2019). Song et al. (2022) demonstrated the oxidase-like activity of the prepared SAzyme (SACe-N-C nanozyme). Fe(III) and Cr(VI) significantly expedited the electron transfer rate of Ce(III) and Ce(IV) on the surface of the SACe-N-C nanozyme. Therefore, Fe(III) and Cr(VI) accelerated the SACe-N-C nanoenzymes-catalyzed process of substrate 3,3′,5,5′–tetramethylbenzidine. The sensor enabled rapid detection of Fe(III) and Cr(VI) in drinking water and spinach samples with the LOD of 34.72 and 93.65 ng mL−1, respectively.
4 Fluorescence sensing
The interaction between fluorescent probes and heavy metal ions can change the fluorescence characteristics of the probes, thus achieving the purpose of metal detection. Compared with traditional techniques, the fluorescence spectroscopic methods are characterized with fast response time, simple operation, high selectivity and sensitivity. Fluorescence spectroscopy is more suitable for the analysis of trace metal ions in complex matrices (Lian et al., 2020). For fluorescence analysis, the choice of fluorescent probes is a key factor. A wide variety of fluorescent probes have been applied. In this section, we briefly discuss advances in fluorescent nanomaterials for the detection of heavy metal ions in food samples.
Fluorescent nanomaterials with unique photoluminescent properties have gained considerable interest. The fluorescent probes can be categorized into: semiconductor quantum dots (QDs), carbon nanomaterials, and metal nanoparticles. Semiconductor QDs including CdS, CdSe, CdTe, CdS and ZnSe were widely used in the fields of metal ion detection (Anas et al., 2019). He et al. (2022) prepared an “on-off” sensor based on the glutathione (GSH)-modified CdS QDs for the detection of Cu(II), Hg(II), and Mg(II) in foods. The fluorescence of GSH-CdS QDs sensor was linearly quenched by Cu(II), Hg(II), and Mg(II). The LOD were 0.22, 5.8, and 1.6 ng mL−1, respectively. Hu et al. (2023) used GSH-CdTe QDs for Cu(II) detection, where the concentration of Cu(II) showed a good linear relationship with the fluorescence attenuation of the sensor in the range of 20–1100 nM. GSH-CdTe QDs had been successfully applied to the detection of real samples such as hawthorn, American ginseng and honeysuckle, allowing detection of Cu(II) with the LOD of 10.12 nM. However, the cytotoxicity and hydrophobicity of semiconductor QDs still face significant challenges as ideal fluorescent probes in food samples.
Carbon nanomaterials present unique properties due to quantum size effect, surface effect, and macroscopic quantum tunneling effect. Since the first fluorescent carbon nanoparticles were extracted from the cleavage of carbon nanotubes in 2004, carbon dot-like materials had attracted much attention (Xu et al., 2004). Heteroatom chemical doping could effectively modulate the physicochemical and photochemical properties of CDs. Zou et al. (2022) employed nitrogen-doped carbon quantum dots (N-CQDs) for highly selective detection of Hg(II). Based on the joint of dynamic and static quenching effect, the sensing system realized practical detection in sorghum and rice samples with the LOD of 0.8 μM. Zhang et al. (2023a) synthesized an N-doped blue-light CDs (N-BCDs) with high quantum yield. Cr(VI) quenched the fluorescence of N-BCDs through the internal filter effect. N-BCDs were used for the instantaneous detection of trace Cr(VI) in drinking water. As shown in Figure 2A, Tan (Tan et al., 2022) et al. prepared nitrogen-doped CDs (N-CDs) using a one-step hydrothermal method. The fluorescence of N-CDs could be quenched by Cd(II) and Hg(II) within 5 minutes, allowing the rapid and sensitive detection of Cd(II) and Hg(II) in fruits and vegetables, with the LOD of 0.20 and 0.188 μM, respectively.
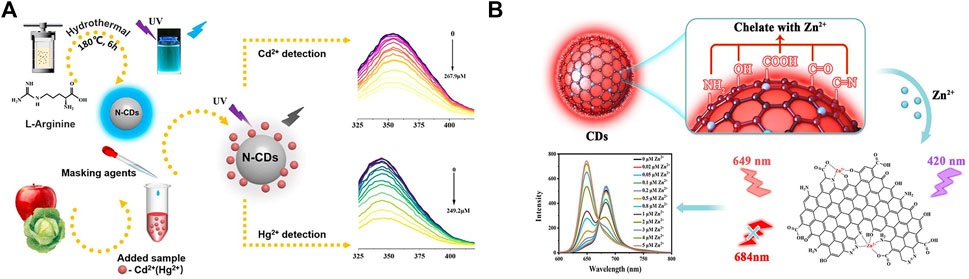
Figure 2. (A) The process of preparation of N-CDs, and schematic diagram of the detection of Cd(II) and Hg(II) in food samples by N-CDs, reproduced with permission from (Tan et al., 2022). (B) Mechanism illustration of the CDs for the detection of zinc ion, reproduced with permission from (Lu et al., 2023).
The aforementioned N-CDs fluorescent sensors were based on turn-off methodologies. Wang et al. (2022) constructed a three-channel fluorescent array sensor based on N-doped CDs (NCDs) and gold nanoclusters for the rapid and accurate detection of Cd(II), Pb(II), and Hg(II) in traditional Chinese medicine, with the LOD of 0.15, 0.20, and 0.09 μM, respectively. In addition, owing to the complexity of food samples, the development of ratiometric sensors for the detection of heavy metal ions is necessary to attenuate or eliminate the effects of the external environment. As shown in Figure 2B, Lu et al. (2023) developed a ratiometric fluorescent sensor based on double-emitting fluorescent CDs, which could specifically recognize Zn(II). In the presence of Zn(II), the fluorescence intensity of CDs decreased at 684 nm and increased at 649 nm, enabling the accurate determination of Zn(II) in milk powder and zinc gluconate oral solution, with the LOD of 5 nM.
5 Conclusion and outlook
Due to the harmful effects of heavy metal ions even at low concentrations, ultra-sensitive and selective methods for the detection of heavy metal ions in food samples are highly desirable. In this manuscript, we review the recent progress and development trends in the nanomaterial-based sensors for detecting trace heavy metal ions in food samples. The design principles, sensing mechanisms, and applications of electrochemical, colorimetric, and fluorescent methods are presented in details. Even though these assays are promising in the detection of trace heavy metal ions, there is still space for improvement, especially in the practical applications. On the one hand, some of the sensors used to detect trace heavy metal ions were only applied to simple samples, such as drinking water rather than food samples. This limitation was due to the various interfering substances in complex food samples, which would bring some interferences in the sensing system. Therefore, apart from improving the sensitivity of the sensor, the anti-interference and selectivity of the sensor in complex matrices needs to be improved, so that these methods can be extensively applied to the detection of trace heavy metal ions in various foods. On the other hand, most of the currently developed sensors could only be applied to detect one or a few heavy metal ions. Sensors that can simultaneously detect various heavy metal ions are relatively lacking. We envision that with the development of different sensing arrays and the assistance of deep-learning algorithms, the sensitive and accurate detection of these heavy metal ions in high throughput will be achieved in the near future. The advancement of these sensing technologies will benefit the on-site precise and rapid screening of trace heavy metal ions for food safety concerns.
Author contributions
LS: Conceptualization, Data curation, Investigation, Visualization, Writing–original draft. QW: Conceptualization, Writing–review and editing. YJ: Funding acquisition, Supervision, Writing–review and editing. ZW: Conceptualization, Funding acquisition, Supervision, Writing–review and editing.
Funding
The author(s) declare that financial support was received for the research, authorship, and/or publication of this article. This work is supported by National Key Research and Development Program of China (2022YFC2106100), Beijing Natural Science Foundation (No. 7232342 and 2242027), Academy of Medical Sciences Newton Advanced Fellowship (NAFR13\1015).
Conflict of interest
The authors declare that the research was conducted in the absence of any commercial or financial relationships that could be construed as a potential conflict of interest.
Publisher’s note
All claims expressed in this article are solely those of the authors and do not necessarily represent those of their affiliated organizations, or those of the publisher, the editors and the reviewers. Any product that may be evaluated in this article, or claim that may be made by its manufacturer, is not guaranteed or endorsed by the publisher.
References
Ai, S., Liu, B., Yang, Y., Ding, J., Yang, W., Bai, X., et al. (2018). Temporal variations and spatial distributions of heavy metals in a wastewater-irrigated soil-eggplant system and associated influencing factors. Ecotoxicol. Environ. Saf. 153, 204–214. doi:10.1016/j.ecoenv.2018.02.026
Amali, R. K. A., Lim, H. N., Ibrahim, I., Huang, N. M., Zainal, Z., and Ahmad, S. A. A. (2021). Significance of nanomaterials in electrochemical sensors for nitrate detection: a review. Trends Environ. Anal. Chem. 31, e00135. doi:10.1016/j.teac.2021.e00135
Anas, N. A. A., Fen, Y. W., Omar, N. A. S., Daniyal, W. M. E. M. M., Ramdzan, N. S. M., and Saleviter, S. (2019). Development of graphene quantum dots-based optical sensor for toxic metal ion detection. Sensors 19 (18), 3850. doi:10.3390/s19183850
Bhattacharyya, M., and Hossain, M. (2024). Picomolar level sensorial dual colorimetric gold nanoparticle sensor for Zn2+ and Hg2+ ions synthesized from bark extract of Lannea Grandis Coromandelica and its wide range applications in real sample analysis. Spectrochimica Acta Part A Mol. Biomol. Spectrosc. 308, 123682. doi:10.1016/j.saa.2023.123682
Chen, W., Guan, Y., Chen, Q., Ren, J., Xie, Y., and Yin, J. (2022). The mark of Mercury(II) in living animals and plants through using a BODIPY-based near-infrared fluorescent probe. Dyes Pigments 200, 110134. doi:10.1016/j.dyepig.2022.110134
Chen, Y., Zhao, P., Hu, Z., Liang, Y., Han, H., Yang, M., et al. (2023a). Amino-functionalized multilayer Ti3C2Tx enabled electrochemical sensor for simultaneous determination of Cd2+ and Pb2+ in food samples. Food Chem. 402, 134269. doi:10.1016/j.foodchem.2022.134269
Chen, Y., Zhao, P., Liang, Y., Ma, Y., Liu, Y., Zhao, J., et al. (2023b). A sensitive electrochemical sensor based on 3D porous melamine-doped rGO/MXene composite aerogel for the detection of heavy metal ions in the environment. Talanta 256, 124294. doi:10.1016/j.talanta.2023.124294
Cheng, N., Li, J.-C., Liu, D., Lin, Y., and Du, D. (2019). Single-Atom nanozyme based on nanoengineered Fe–N–C catalyst with superior peroxidase-like activity for ultrasensitive bioassays. Small 15 (48), 1901485. doi:10.1002/smll.201901485
Ding, Q., Li, C., Wang, H., Xu, C., and Kuang, H. (2021). Electrochemical detection of heavy metal ions in water. Chem. Commun. 57 (59), 7215–7231. doi:10.1039/D1CC00983D
Dong, J., Li, X., Wen, L., Ma, Y., Xu, J., Luo, H., et al. (2024). A novel electrochemical strategy based on MXene@rGO composite aerogel-doped UiO-66-NH2 for simultaneous detection of cadmium and lead in grain and water samples. Food Chem. 437, 137835. doi:10.1016/j.foodchem.2023.137835
Dong, J., Wen, L., Zhao, D., Yang, H., Zhao, J., Hu, Z., et al. (2023). Flexible carbon fiber cloth supports decorated with cerium metal- organic frameworks and multi-walled carbon nanotubes for simultaneous on-site detection of Cd2+ and Pb2+ in food and water samples. Food Chem. 418, 135869. doi:10.1016/j.foodchem.2023.135869
Guo, Y., Zhang, Y., Shao, H., Wang, Z., Wang, X., and Jiang, X. (2014). Label-free colorimetric detection of cadmium ions in rice samples using gold nanoparticles. Anal. Chem. 86 (17), 8530–8534. doi:10.1021/ac502461r
Guo, Z., Chen, P., Yosri, N., Chen, Q., Elseedi, H. R., Zou, X., et al. (2023). Detection of heavy metals in food and agricultural products by surface-enhanced Raman spectroscopy. Food Rev. Int. 39 (3), 1440–1461. doi:10.1080/87559129.2021.1934005
He, K., Yu, X., Qin, L., and Wu, Y. (2022). CdS QDs: facile synthesis, design and application as an “on–off” sensor for sensitive and selective monitoring Cu2+, Hg2+ and Mg2+ in foods. Food Chem. 390, 133116. doi:10.1016/j.foodchem.2022.133116
Hemmaphan, S., and Bordeerat, N. K. (2022). Genotoxic effects of lead and their impact on the expression of DNA repair genes. Int. J. Environ. Res. Public Health 19 (7), 4307. doi:10.3390/ijerph19074307
Hu, P., Liu, J., Xia, C., Liu, B., Zhu, H., and Niu, X. (2024). Matrix redox interference-free nanozyme-amplified detection of Hg2+ using thiol-modified phosphatase-mimetic nanoceria. Sensors Actuators B Chem. 401, 135030. doi:10.1016/j.snb.2023.135030
Hu, Z., Long, W., Liu, T., Guan, Y., Lei, G., Suo, Y., et al. (2023). A sensitive fluorescence sensor based on a glutathione modified quantum dot for visual detection of copper ions in real samples. Spectrochimica Acta Part A Mol. Biomol. Spectrosc. 294, 122517. doi:10.1016/j.saa.2023.122517
Hua, F., Pan, F., Yang, J., Yan, Y., Huang, X., Yuan, Y., et al. (2023). Quantitative colorimetric sensing of heavy metal ions via analyte-promoted growth of Au nanoparticles with timer or smartphone readout. Anal. Bioanal. Chem. 415 (14), 2705–2713. doi:10.1007/s00216-023-04669-9
Huang, R., Lv, J., Chen, J., Zhu, Y., Zhu, J., Wågberg, T., et al. (2023). Three-dimensional porous high boron-nitrogen-doped carbon for the ultrasensitive electrochemical detection of trace heavy metals in food samples. J. Hazard. Mater. 442, 130020. doi:10.1016/j.jhazmat.2022.130020
Huo, D., Zhang, Y., Li, N., Ma, W., Liu, H., Xu, G., et al. (2022). Three-dimensional graphene/amino-functionalized metal–organic framework for simultaneous electrochemical detection of Cd(II), Pb(II), Cu(II), and Hg(II). Anal. Bioanal. Chem. 414 (4), 1575–1586. doi:10.1007/s00216-021-03779-6
Jiang, D., Ni, D., Rosenkrans, Z. T., Huang, P., Yan, X., and Cai, W. (2019). Nanozyme: new horizons for responsive biomedical applications. Chem. Soc. Rev. 48 (14), 3683–3704. doi:10.1039/C8CS00718G
Kartoğlu, B., Bodur, S., Zeydanlı, D., Göver, T., Özaydın, E., Gülhan Bakırdere, E., et al. (2024). Determination of copper in rose tea samples using flame atomic absorption spectrometry after emulsification liquid–liquid microextraction. Food Chem. 439, 138140. doi:10.1016/j.foodchem.2023.138140
Keyster, M., Niekerk, L.-A., Basson, G., Carelse, M., Bakare, O., Ludidi, N., et al. (2020). Decoding heavy metal stress signalling in plants: towards improved food security and safety. Plants 9 (12), 1781. doi:10.3390/plants9121781
Komova, N. S., Serebrennikova, K. V., Berlina, A. N., Pridvorova, S. M., Zherdev, A. V., and Dzantiev, B. B. (2021). Mercaptosuccinic-acid-functionalized gold nanoparticles for highly sensitive colorimetric sensing of Fe(III) ions. Chemosensors 9 (10), 290. doi:10.3390/chemosensors9100290
Lai, L., Yan, F., Chen, G., Huang, Y., Huang, L., and Li, D. (2023). Recent progress on fluorescent probes in heavy metal determinations for food safety: a review. Molecules 28 (15), 5689. doi:10.3390/molecules28155689
Li, Q., Zheng, S., Du, M., and Pang, H. (2021). Ultrathin nanosheet metal–organic framework-NiO/Ni nanorod composites. Chem. Eng. J. 417, 129201. doi:10.1016/j.cej.2021.129201
Li, X., Wang, L., Du, D., Ni, L., Pan, J., and Niu, X. (2019). Emerging applications of nanozymes in environmental analysis: opportunities and trends. TrAC Trends Anal. Chem. 120, 115653. doi:10.1016/j.trac.2019.115653
Lian, J., Xu, Q., Wang, Y., and Meng, F. (2020). Recent developments in fluorescent materials for heavy metal ions analysis from the perspective of forensic chemistry. Front. Chem. 8, 593291. doi:10.3389/fchem.2020.593291
Lim, J. W., Kim, T.-Y., and Woo, M.-A. (2021). Trends in sensor development toward next-generation point-of-care testing for mercury. Biosens. Bioelectron. 183, 113228. doi:10.1016/j.bios.2021.113228
Lu, G., Jia, Z., Yu, M., Zhang, M., and Xu, C. (2023). A ratiometric fluorescent sensor based on chelation-enhanced fluorescence of carbon dots for zinc ion detection. Molecules 28 (23), 7818. doi:10.3390/molecules28237818
Ma, L., Pei, W.-Y., Yang, J., and Ma, J.-F. (2024). A new thiacalix[4]arene-based metal-organic framework as an efficient electrochemical sensor for trace detection of Cd2+ and Pb2+. Food Chem. 441, 138352. doi:10.1016/j.foodchem.2023.138352
Meng, R., Zhu, Q., Long, T., He, X., Luo, Z., Gu, R., et al. (2023). The innovative and accurate detection of heavy metals in foods: a critical review on electrochemical sensors. Food control. 150, 109743. doi:10.1016/j.foodcont.2023.109743
Mukherjee, S., Bhattacharyya, S., Ghosh, K., Pal, S., Halder, A., Naseri, M., et al. (2021). Sensory development for heavy metal detection: a review on translation from conventional analysis to field-portable sensor. Trends Food Sci. Technol. 109, 674–689. doi:10.1016/j.tifs.2021.01.062
Nazifi, M., Ramezani, A. M., Absalan, G., and Ahmadi, R. (2021). Colorimetric determination of D-penicillamine based on the peroxidase mimetic activity of hierarchical hollow MoS2 nanotubes. Sensors Actuators B Chem. 332, 129459. doi:10.1016/j.snb.2021.129459
Nozari, M., Esmaili-sari, A., Moradi, A. M., Bahramifar, N., and Taghavi, L. (2023). Contamination, ecological, and health risk assessment of heavy metals and organophosphorus pesticides in single, double, and ratoon cropping of rice: a case study in Mazandaran, North of Iran. Environ. Monit. Assess. 195 (3), 376. doi:10.1007/s10661-023-10916-4
Pan, Y., Wang, L., Chen, S., Wei, Y., and Wei, X. (2024). A target-triggered ultra-sensitive aptasensor for simultaneous detection of Cd2+ and Hg2+ using MWCNTs-Au NPs modified electrode. Food Chem. 440, 138185. doi:10.1016/j.foodchem.2023.138185
Pang, Y.-H., Yang, Q.-Y., Jiang, R., Wang, Y.-Y., and Shen, X.-F. (2023). A stack-up electrochemical device based on metal-organic framework modified carbon paper for ultra-trace lead and cadmium ions detection. Food Chem. 398, 133822. doi:10.1016/j.foodchem.2022.133822
Qin, G., Niu, Z., Yu, J., Li, Z., Ma, J., and Xiang, P. (2021). Soil heavy metal pollution and food safety in China: effects, sources and removing technology. Chemosphere 267, 129205. doi:10.1016/j.chemosphere.2020.129205
Qiu, Z.-M., Bai, Y., Gao, Y.-D., Liu, C.-L., Ru, Y., Pi, Y.-C., et al. (2022). MXenes nanocomposites for energy storage and conversion. Rare Met. 41 (4), 1101–1128. doi:10.1007/s12598-021-01876-0
Rachmawati, S., Candraningtyas, C. F., Putra, C. D. H., Fadhilah, R. N., Muazulfa, T. I., Firmansyah, F., et al. (2024). Analysis of Lead (Pb) and Cadmium (Cd) heavy metals in cow’s milk in Central Java, Indonesia. IOP Conf. Ser. Earth Environ. Sci. 1314 (1), 012005. doi:10.1088/1755-1315/1314/1/012005
Sardesai, A. U., Dhamu, V. N., Paul, A., Muthukumar, S., and Prasad, S. (2020). Design and electrochemical characterization of spiral electrochemical notification coupled electrode (SENCE) platform for biosensing application. Micromachines 11 (3), 333. doi:10.3390/mi11030333
Sharifi, H., Tashkhourian, J., and Hemmateenejad, B. (2022). Identification and determination of multiple heavy metal ions using a miniaturized paper-based optical device. Sensors Actuators B Chem. 359, 131551. doi:10.1016/j.snb.2022.131551
Shen, Y., Nie, C., Wei, Y., Zheng, Z., Xu, Z.-L., and Xiang, P. (2022). FRET-based innovative assays for precise detection of the residual heavy metals in food and agriculture-related matrices. Coord. Chem. Rev. 469, 214676. doi:10.1016/j.ccr.2022.214676
Shih, T.-T., Chen, J.-Y., Luo, Y.-T., Lin, C.-H., Liu, Y.-H., Su, Y.-A., et al. (2019). Development of a titanium dioxide-assisted preconcentration/on-site vapor-generation chip hyphenated with inductively coupled plasma-mass spectrometry for online determination of mercuric ions in urine samples. Anal. Chim. Acta 1063, 82–90. doi:10.1016/j.aca.2019.02.035
Song, G., Zhang, Q., Liang, S., Yao, Y., Feng, M., Majid, Z., et al. (2022). Oxidation activity modulation of a single atom Ce-N-C nanozyme enabling a time-resolved sensor to detect Fe3+ and Cr6+. J. Mater. Chem. C 10 (41), 15656–15663. doi:10.1039/D2TC02476D
Sönnichsen, C., Reinhard, B. M., Liphardt, J., and Alivisatos, A. P. (2005). A molecular ruler based on plasmon coupling of single gold and silver nanoparticles. Nat. Biotechnol. 23 (6), 741–745. doi:10.1038/nbt1100
Tan, Q., Li, X., Wang, L., Zhao, J., Yang, Q., Sun, P., et al. (2022). One-step synthesis of highly fluorescent carbon dots as fluorescence sensors for the parallel detection of cadmium and mercury ions. Front. Chem. 10, 1005231. doi:10.3389/fchem.2022.1005231
Wang, F.-F., Liu, C., Yang, J., Xu, H.-L., Pei, W.-Y., and Ma, J.-F. (2022a). A sulfur-containing capsule-based metal-organic electrochemical sensor for super-sensitive capture and detection of multiple heavy-metal ions. Chem. Eng. J. 438, 135639. doi:10.1016/j.cej.2022.135639
Wang, S., Deng, G., Yang, J., Chen, H., Long, W., She, Y., et al. (2022b). Carbon dot- and gold nanocluster-based three-channel fluorescence array sensor: visual detection of multiple metal ions in complex samples. Sensors Actuators B Chem. 369, 132194. doi:10.1016/j.snb.2022.132194
Wang, X., Xu, M., Kuang, Y., Liu, X., and Yuan, J. (2024). A novel ratiometric electrochemical aptasensor based on M-shaped functional DNA complexes for simultaneous detection of trace lead and mercury ions in series aquatic edible vegetables. J. Hazard. Mater. 465, 133169. doi:10.1016/j.jhazmat.2023.133169
Wen, L., Dong, J., Yang, H., Zhao, J., Hu, Z., Han, H., et al. (2022). A novel electrochemical sensor for simultaneous detection of Cd2+ and Pb2+ by MXene aerogel-CuO/carbon cloth flexible electrode based on oxygen vacancy and bismuth film. Sci. Total Environ. 851, 158325. doi:10.1016/j.scitotenv.2022.158325
Xu, X., Ray, R., Gu, Y., Ploehn, H. J., Gearheart, L., Raker, K., et al. (2004). Electrophoretic analysis and purification of fluorescent single-walled carbon nanotube fragments. J. Am. Chem. Soc. 126 (40), 12736–12737. doi:10.1021/ja040082h
Yang, Q., Nguyen, E. P., Panáček, D., Šedajová, V., Hrubý, V., Rosati, G., et al. (2023). Metal-free cysteamine-functionalized graphene alleviates mutual interferences in heavy metal electrochemical detection. Green Chem. 25 (4), 1647–1657. doi:10.1039/D2GC02978B
Yang, T., Yu, R., Yan, Y., Zeng, H., Luo, S., Liu, N., et al. (2018). A review of ratiometric electrochemical sensors: from design schemes to future prospects. Sensors Actuators B Chem. 274, 501–516. doi:10.1016/j.snb.2018.07.138
Yu, L., Fan, Z., Shao, Y., Tian, Z., Sun, J., and Liu, Z. (2019). Versatile N-doped MXene ink for printed electrochemical energy storage application. Adv. Energy Mater. 9 (34), 1901839. doi:10.1002/aenm.201901839
Yu, L., Pang, Y., Mo, Z., Huang, Y., and Shen, X. (2021). Coordination array for accurate colorimetric sensing of multiple heavy metal ions. Talanta 231, 122357. doi:10.1016/j.talanta.2021.122357
Zeng, L., Zhou, J., Wang, X., Zhang, Y., Wang, M., and Su, P. (2021). Cadmium attenuates testosterone synthesis by promoting ferroptosis and blocking autophagosome-lysosome fusion. Free Radic. Biol. Med. 176, 176–188. doi:10.1016/j.freeradbiomed.2021.09.028
Zhang, H., Hu, X., Li, T., Zhang, Y., Xu, H., Sun, Y., et al. (2022). MIL series of metal organic frameworks (MOFs) as novel adsorbents for heavy metals in water: a review. J. Hazard. Mater. 429, 128271. doi:10.1016/j.jhazmat.2022.128271
Zhang, M., He, H., Huang, Y., Huang, R., Wu, Z., Liu, X., et al. (2023a). Machine learning integrated high quantum yield blue light carbon dots for real-time and on-site detection of Cr(VI) in groundwater and drinking water. Sci. Total Environ. 904, 166822. doi:10.1016/j.scitotenv.2023.166822
Zhang, N., Qiao, R., Su, J., Yan, J., Xie, Z., Qiao, Y., et al. (2017). Recent advances of electrospun nanofibrous membranes in the development of chemosensors for heavy metal detection. Small 13 (16), 1604293. doi:10.1002/smll.201604293
Zhang, Y., Xu, Y., Li, N., Liu, X., Ma, Y., Siyi, Y., et al. (2023b). An ultrasensitive electrochemical sensor based on antimonene simultaneously detect multiple heavy metal ions in food samples. Food Chem. 421, 136131. doi:10.1016/j.foodchem.2023.136131
Keywords: heavy metal ions, detection, food samples, nanomaterials, sensors
Citation: Si L, Wu Q, Jin Y and Wang Z (2024) Research progress in the detection of trace heavy metal ions in food samples. Front. Chem. 12:1423666. doi: 10.3389/fchem.2024.1423666
Received: 26 April 2024; Accepted: 13 May 2024;
Published: 28 May 2024.
Edited by:
Tony D. James, University of Bath, United KingdomReviewed by:
Chunfen Jin, Honeywell UOP, United StatesCopyright © 2024 Si, Wu, Jin and Wang. This is an open-access article distributed under the terms of the Creative Commons Attribution License (CC BY). The use, distribution or reproduction in other forums is permitted, provided the original author(s) and the copyright owner(s) are credited and that the original publication in this journal is cited, in accordance with accepted academic practice. No use, distribution or reproduction is permitted which does not comply with these terms.
*Correspondence: Zhuo Wang, wangzhuo77@mail.buct.edu.cn; Yulong Jin, jinyulong@buct.edu.cn