Synergistic Activity of Niclosamide in Combination With Colistin Against Colistin-Susceptible and Colistin-Resistant Acinetobacter baumannii and Klebsiella pneumoniae
- 1Clinic Unit of Infectious Diseases, Microbiology and Preventive Medicine, Institute of Biomedicine of Seville (IBiS), University Hospital Virgen del Rocío/CSIC/University of Seville, Seville, Spain
- 2Department of Medicine, University of Seville, Seville, Spain
Colistin is among the few antibiotics effective against multidrug-resistant Acinetobacter baumannii and Klebsiella pneumoniae clinical isolates. However, in the last few years, colistin-resistant A. baumannii and K. pneumoniae strains have emerged. Therefore, combination therapies, between colistin and other old drugs, restoring the activity of colistin are required. The main objective of this study was to analyse the activity of niclosamide, an anthelmintic drug, in combination with colistin against colistin-susceptible (Col-S) and colistin-resistant (Col-R) A. baumannii and K. pneumoniae. The MIC were determined by microdilution assay and the time-kill curves were performed. The zeta potential of Col-S and Col-R of A. baumannii and K. pneumoniae in presence of niclosamide was assessed. Niclosamide in combination with colistin showed improved activity against Col-S and Col-R A. baumannii and K. pneumoniae. Time-killing curves showed synergic activity between niclosamide and colistin against Col-S and Col-R A. baumannii and K. pneumoniae, especially when niclosamide or colistin was added for second time at 4 h of the 24 h killing curve. Col-R A. baumannii and K. pneumoniae in presence of niclosamide exhibited a greater negative charge (−34.95 ± 0.35 mV and −38.85 ± 0.92 mV; P < 0.05) than Col-R A. baumannii and K. pneumoniae in absence of niclosamide (−26.85 ± 3.65 mV and −35.27 ± 0.72 mV). These data suggest that niclosamide might be combined with colistin, being a potential alternative for treatment of Col-R Gram-negative bacilli infections.
Introduction
A number of infections caused by multidrug-resistant Acinetobacter baumannii and Klebsiella pneumoniae required the use of colistin, but both pathogens may rapidly acquire specific resistance mechanisms against colistin (Bonnin et al., 2013; Ah et al., 2014). Nowadays, the rates of colistin resistance worldwide vary between 3 and 28% for A. baumannii, and 2.8 and 10.5% for K. pneumoniae (Fernández-Cuenca et al., 2013; Ah et al., 2014). In this context, combination therapies between colistin and other drugs are among the new promising strategies to treat bacterial infections (Vila and Pachón, 2012; Cassir et al., 2014).
Classical combinations between colistin and other antimicrobial agents to which the isolate is resistant have been reported (Vila and Pachón, 2012; Paul et al., 2014). Their use has remained wide in vitro and in animal experimental model of infections (Vila and Pachón, 2012; Zusman et al., 2013). Few randomized controlled trials examining specific combinations have been completed or are ongoing, and are not sufficient to guide clinical practice (Paul et al., 2014; Poulikakos et al., 2014). This joins with the relatively short window of therapeutic application for severely ill patients for some combinations, and for the rapid emergence of drug resistance (Poulikakos et al., 2014).
In this context, “repurposing drugs,” defined as investigating new uses for already existing drugs, have gained renewed interest, as reflected by several recent studies (Chopra et al., 2010; Younis et al., 2015; Tharmalingam et al., 2018), and using them associated with colistin (Antunes et al., 2012; Zemke et al., 2014).
Niclosamide is an anthelmintic drug widely used for treating tape worm infection in humans and has lately been shown to possess anti-cancer and anti-diabetic activities (Tao et al., 2014; Ye et al., 2014). Niclosamide has also been identified as a potent anti-bacterial drug against Helicobacter pylori (Tharmalingam et al., 2018) and Pseudomonas aeruginosa by the inhibition of quorum sensing and various virulence genes, and by the reduction of elastase and pyocyanin levels (Imperi et al., 2013; Costabile et al., 2015). Moreover, Rajamuthiah et al., have reported that niclosamide present bacteriostatic activity against Staphylococcus aureus probably due the damage in their bacterial membrane but not against A. baumannii, P. aeruginosa, E. coli, and K. pneumoniae (Rajamuthiah et al., 2015; Gwisai et al., 2017). Currently there is no study regarding the combination between colistin and niclosamide to restore the activity of colistin against Gram-negative bacilli.
The aim of this study was to determine the in vitro activity of niclosamide in combination with colistin against colistin-susceptible and colistin-resistant A. baumannii and K. pneumoniae strains.
Materials and Methods
Bacterial Strains
Reference colistin-susceptible (Col-S) A. baumannii ATCC 17978 strain (Baumann et al., 1968), and 13 clinical colistin-resistant (Col-R) A. baumannii strains isolated from an hospital outbreak in 2000 in Spain (Valencia et al., 2009) were used in this study. We also used reference Col-S K. pneumoniae CECT 997 strain (Reading and Cole, 1977), one Col-S and 2 Col-R clinical K. pneumoniae strains (Pachón-Ibáñez et al., 2018).
Pmra, Pmrb, Mgrb, Phop, and Phoq Genes Amplification and Sequencing
In order to investigate the possible contribution of pmrAB, mgrB, and phoPQ to the colistin resistance in A. baumannii and K. pneumoniae, these genes were analyzed to detect any genetic alteration. DNA samples were obtained from the isolates by heating the colonies in water at 96°C. The genes were amplified using the primers listed in Table 1. The obtained bands were purified with the kit MEGAquick-spin plus (iNtRON Biotechnology, USA) and sequenced at the Institute of Biomedicine of Seville. The nucleotide and deduced protein sequences were analyzed using the Serial Cloner program (http://serialbasics.free.fr/Serial_Cloner.html).
In vitro Susceptibility Testing
MIC of colistin (Sigma, Spain), MIC of niclosamide (Sigma, Spain), and MIC of colistin in presence of different concentrations of niclosamide (between 0.5 and 4 μM) against Col-S and Col-R references and clinical A. baumannii and K. pneumoniae strains were determined in two independent experiments by broth microdilution assay according to CLSI recommendations for A. baumannii and EUCAST recommendations for K. pneumoniae (Clinical and Laboratory Standards Institute, 2016 European Committee on Antimicrobial Susceptibility European Committee on Antimicrobial Susceptibility Testing [EUCAST], 2016). The initial inoculum of 5 x 105 CFU/mL for each strain was used in microtiter plates V (Deltlab, Spain) in presence of colistin, niclosamide, or colistin plus niclosamide, and incubated for 16–18 h at 37°C. Escherichia coli ATCC 25922 was used as control strain.
Time-Kill Kinetic Assays
Time-kill curves of A. baumannii ATCC 17978 and Col-R #11 strains and K. pneumoniae CECT 997 and KPc21 strains with starting inoculum of 1 × 106 CFU/mL, conducted on Mueller Hinton broth cation-adjusted, in presence of 2 μM niclosamide and colistin (sub-MIC) alone or in combination were performed in two independent experiments as previously described (Smani et al., 2011).
Moreover, in some conditions niclosamide was added for a second time 4 h after bacterial inoculation in order to avoid the antagonism effect of niclosamide with colistin observed in the first 4 h. In the same way colistin was added for a second time 4 h after bacterial inoculation because the half-life of colistin in bacterial culture broth is 4 h (Owen et al., 2007; Bergen et al., 2010). Bacterial cultures without drugs were carried out in parallel as controls. Tubes of each condition were incubated at 37°C with shaking and samples were taken at 0, 2, 4, 8, and 24 h and serially diluted. Viable counts were determined by plating 100 μL of control, test cultures, or dilutions at the indicated times onto sheep blood agar plates (Beckton Dickinson, USA). Plates were incubated for 24 h, and after colony counts, the log of viable cells (CFU/mL) was determined. Synergy was defined as a reduction ≥ 2 log CFU/mL with the combination respect to the more active drug (Pachón-Ibáñez et al., 2018). Thus, niclosamide was considered synergistic when in combination with colistin reduced the bacterial concentration ≥ 2 log CFU/mL with respect to colistin alone.
Zeta Potential Measurements
Zeta potential measurements were performed as previously described with minor modifications (Soon et al., 2011). Briefly, the bacterial surface was cleansed by washing twice with Milli-Q water, resuspended in Milli-Q water at 108 CFU/mL, and diluted 10-fold in the same medium immediately prior to zeta potential measurement. The resulting suspensions were used to fill clear disposable folded capillary zeta cells (Malvern, UK).
To examine the effect of niclosamide treatment on Col-S and Col-R A. baumannii and K. pneumoniae strains, 2 μM niclosamide was added to 5 mL of bacterial culture at 108 CFU/mL, then incubated in a shaking bath (37°C, 180 rpm) for 20 min and prepared for zeta potential analysis as described above. The zeta potential measurement (mV) of bacterial cells was measured at 25°C with a zeta potential analyzer at 150 V (Zetasizer Nano ZS; Malvern Instruments, Malvern, UK).
Statistical Analysis
Group data are presented as mean ± SEM. Student t-test was used to determine differences between means. Differences were considered significant at P < 0.05. The SPSS (version 17.0) statistical package was used (SPSS Inc.).
Results
Colistin MIC and Resistance Mechanisms
The MIC for the Col-R A. baumannii and K. pneumoniae strains ranged from 32 to > 256 μg/mL, while those for the susceptible reference strains were 0.5 μg/mL. The analysis of the pmrA, pmrB, mgrB, phoP, and phoQ sequences showed that Col-R A. baumannii strains presented only different amino acids substitution in pmrB (López-Rojas et al., 2016). Col-R K. pneumoniae strains presented IS1 transposase insertion in mgrB or different amino acids substitution in pmrA and pmrB, without substituting amino acid in phoP and phoQ (Table 2).
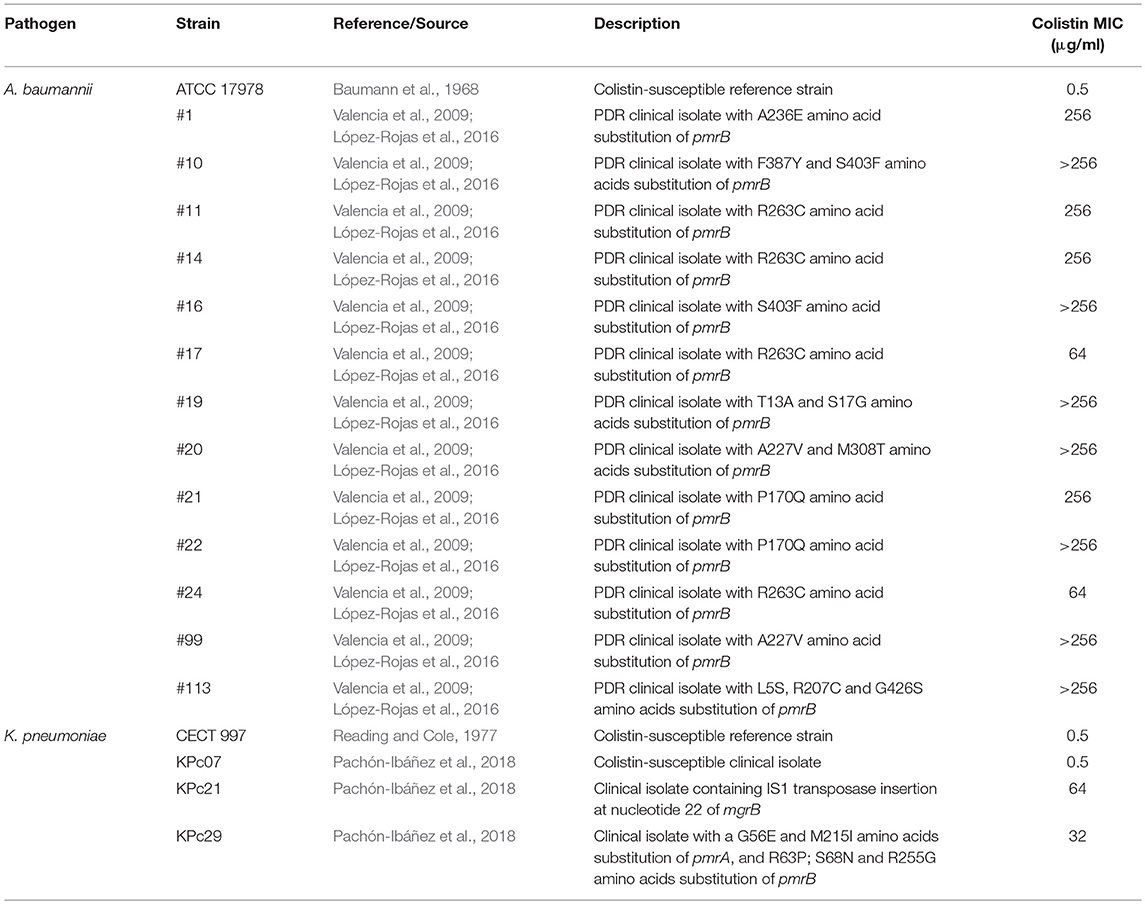
Table 2. Colistin MICs and description of Col-S and Col-R A. baumannii and K. pneumoniae strains used in this study.
In vitro Activity of Niclosamide in Combination With Colistin Against Col-S and Col-R A. Baumannii and K. Pneumoniae
Niclosamide alone or in combination with colistin was tested against reference and clinical Col-S and Col-R A. baumannii and K. pneumoniae strains. The MIC is shown in Table 3. Niclosamide alone showed a range of MIC from 6.25 to 400 μM for Col-S and Col-R A. baumannii strains, and from 400 to >800 μM for Col-S and Col-R K. pneumoniae strains.
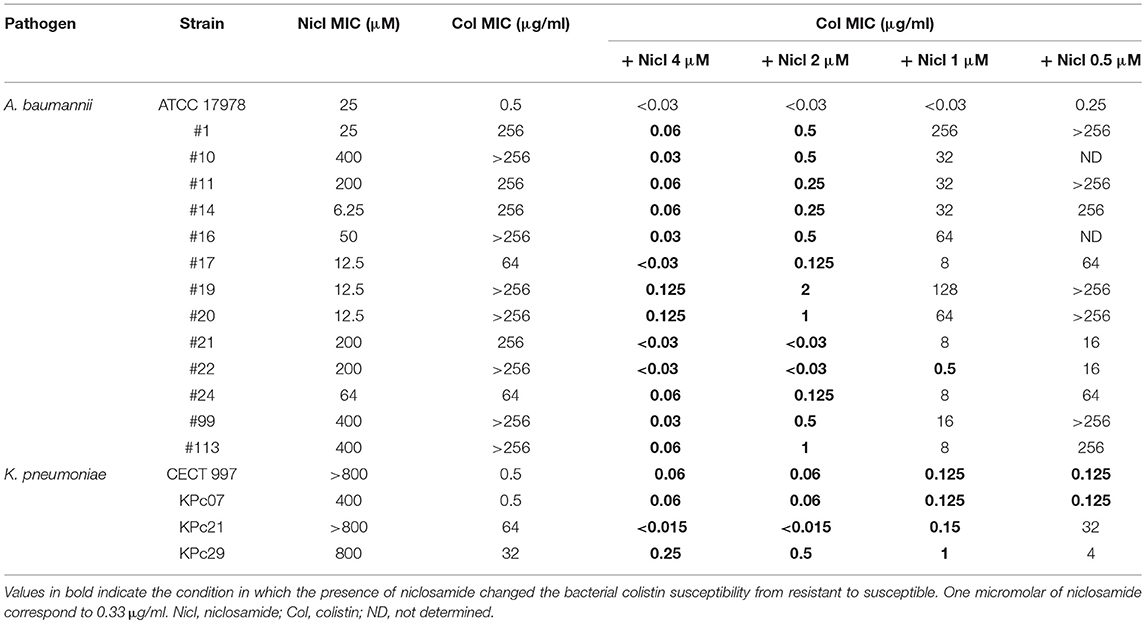
Table 3. Determination of MIC of niclosamide and colistin alone or in combination against Col-S and Col-R A. baumannii and K. pneumoniae.
Niclosamide at 1, 2, and 4 μM in combination with colistin increased significantly the activity of colistin against all Col-S and Col-R strains. In contrast niclosamide, at 0.5 μM in combination with colistin didn't increased the activity of colistin against most of Col-R A. baumannii. For the rest of experiments, we chose 2 μM as niclosamide optimal concentration.
Time-Killing Curves
We examined the ability of niclosamide in combination with colistin to kill Col-S and Col-R A. baumannii strains (ATCC 17978 and #11) and Col-S and Col-R K. pneumoniae strains (CECT 997 and KPc21) in time course assays. Two micromolar niclosamide in combination with 0.25 μg/mL colistin, a colistin sub-MIC of ATCC 17978 strain, showed in the first 4 h no synergistic activity with respect to colistin alone, followed by later synergistic activity decreasing the bacterial cell count by 3.14 log CFU/mL with respect to colistin alone at 24 h (Figure 1A). Combination of 2 μM niclosamide with 8 μg/mL colistin, a colistin sub-MIC of #11 strain, showed higher synergistic activity, decreasing the bacterial cell count with respect to colistin alone by 5.93 log CFU/mL at 24 h (Figure 1A).
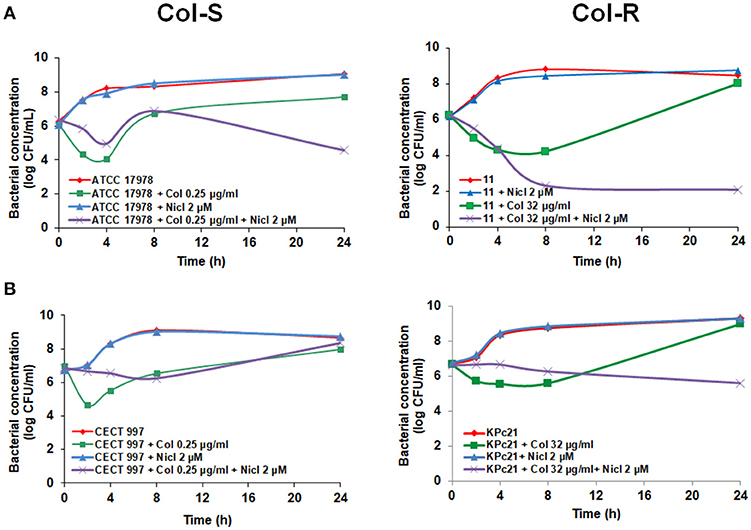
Figure 1. Early addition of niclosamide potentiates the colistin activity against Col-S and Col-R A. baumannii and K. pneumoniae strains. Time-kill curves of A. baumannii ATCC 17978 and #11 strains (A) and K. pneumoniae CECT 997 and KPc21 strains (B) in presence of niclosamide (0 or 2 μM), and colistin (sub-MIC) alone or in combination with niclosamide for 24 h. 1 μM of niclosamide correspond to 0.33 μg/ml. Nicl, niclosamide, Col, colistin.
In the case of K. pneumoniae, 2 μM niclosamide in combination with 0.25 μg/mL colistin, a colistin sub-MIC of CECT 997 strain, didn't showed synergistic effect with colistin during 24 h (Figure 1B). In contrast, 2 μM niclosamide in combination with 32 μg/mL colistin, a colistin sub-MIC of KPc21 strain, showed synergistic activity with respect to colistin alone decreasing the bacterial cell count by 3.38 log CFU/mL at 24 h (Figure 1B). In a control experiment, 2 μM niclosamide had no effect on the growth of Col-S and Col-R A. baumannii and K. pneumoniae strains (Figure 1A,B).
With these results, since the half-life of colistin in bacterial culture broth is 4 h (Owen et al., 2007; Bergen et al., 2010) and can be degraded during time-kill curve experiments (Mohamed et al., 2014), we cannot rule out the possibility that the absence of synergistic activity of niclosamide in combination with colistin observed especially with K. pneumoniae CECT 997 strain (Figure 1B) would be due to colistin degradation in the bacterial culture broth.
Consequently, to maintain the colistin concentration in the medium, in the following experiments we added 0.25 μg/mL colistin after 4 h post-incubation with the initial 2 μM niclosamide and 0.25 μg/mL colistin, or with 0.25 μg/mL colistin alone. This approach produced synergy between niclosamide and colistin, decreasing the bacterial cell count of CECT 997 strain by 4.62 log CFU/mL with respect to colistin plus colistin at 24 h (Figure 2B). Similarly, the addition of 32 μg/mL colistin after 4 h post-incubation with niclosamide and 32 μg/mL colistin decreased the growth of KPc21 strain by 4.38 log CFU/mL with respect to colistin plus colistin at 24 h (Figure 2B). In the case of A. baumannii ATCC 17978 strain, the addition of colistin for second time at 4 h increased the synergy between niclosamide and colistin plus colistin decreasing the bacterial cell count by 2.37 log CFU/mL with respect to niclosamide plus colistin, without the addition of colistin at 4 h, at 8 h (Figure 2A). In contrast, with #11 strain the addition of colistin for second time did not improve the synergy between niclosamide and colistin observed in the (Figures 1, 2A).
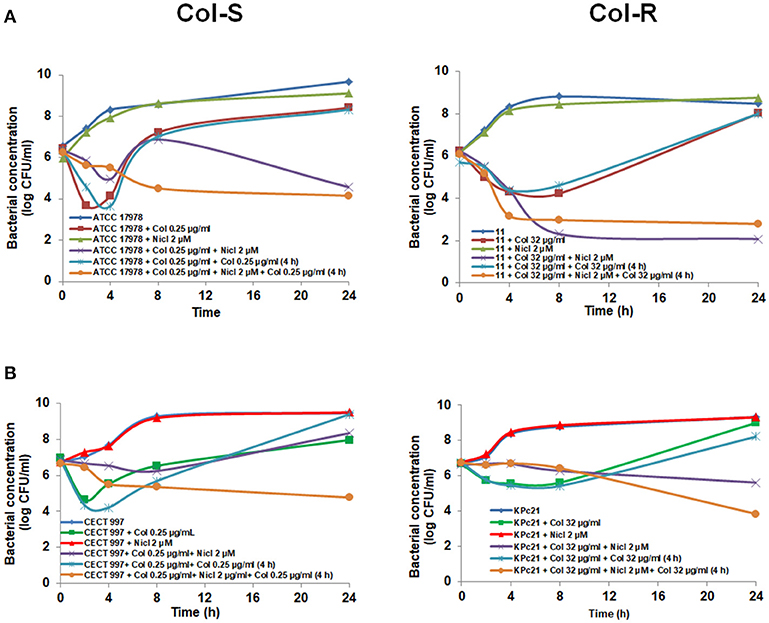
Figure 2. Niclosamide potentiates the colistin activity against Col-S and Col-R A. baumannii and K. pneumoniae strains after second time addition of colistin. Time-kill curves of A. baumannii ATCC 17978 and #11 strains (A), K. pneumoniae CECT 997 and KPc21 strains (B), in presence of 2 μM niclosamide and colistin (sub-MIC) alone, or in combination with or without addition of colistin for second time 4 h after bacterial addition. One micromolar of niclosamide correspond to 0.33 μg/ml. Nicl, niclosamide; Col, colistin.
Furthermore, to avoid the antagonism effect of niclosamide with colistin observed in the first 4 h (Figures 1, 2), we added 2 μM niclosamide after 4 h post-incubation with 0.25 and 8 μg/mL colistin, sub-MIC of A. baumannii ATCC 17978 and #11 strains, respectively. We observed a decrease in the growth of ATCC 17978 and #11 strains by 5.06 and 3.38 log CFU/mL, respectively, with respect to colistin alone at 24 h (Figure 3A).
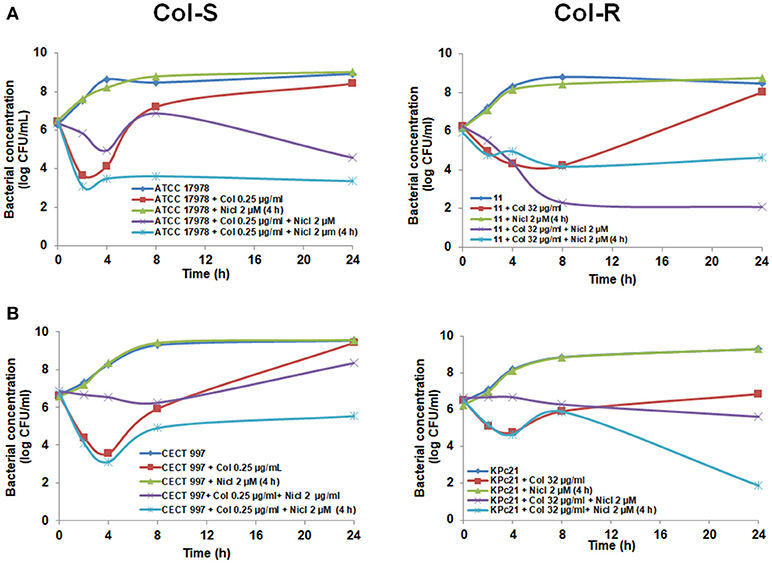
Figure 3. Late addition of niclosamide potentiates the colistin activity against Col-S and Col-R A. baumannii and K. pneumoniae strains. Time-kill curves of A. baumannii ATCC 17978 and #11 strains (A), K. pneumoniae CECT 997 and KPc21 strains (B), in presence of colistin (sub-MIC) alone with or without addition of 2 μM niclosamide 4 h after bacterial addition. One micromolar of niclosamide correspond to 0.33 μg/ml. Nicl, niclosamide; Col, colistin.
In the case of K. pneumoniae, we added 2 μM niclosamide after 4 h post-incubation with 0.25 and 32 μg/mL colistin, sub-MIC of CECT 997 and KPc21 strains, respectively. We observed a decrease in the growth of CECT 997 and KPc21 strain by 3.89 and 4.98 log CFU/mL, respectively, with respect to colistin alone at 24 h (Figure 3B). In the control experiment, addition of 2 μM niclosamide after 4 h post-bacterial incubation had no effect on the growth of Col-S and Col-R A. baumannii and K. pneumoniae strains (Figures 3A, 3B).
Zeta Potential
Figure 4 illustrates the zeta potential of A. baumannii ATCC 17978 and #11 strains in presence and absence of niclosamide. Analysis of zeta potential revealed that treatment of ATCC 17978 and #11 strains with 2 μM niclosamide exhibited significantly high negative surface charge by −35.62 ± 1.32 mV and −34.95 ± 0.35 mV, respectively with respect to ATCC 17978 and #11 strains without treatment with niclosamide, −32.33 ± 0.63 and −26.85 ± 3.65 mV, respectively. Similarly, analysis of zeta potential revealed that treatment of K. pneumoniae CECT 997 and KPc21 strains with 2 μM niclosamide exhibited significantly high negative surface charge by −40.23 ± 0.88 mV and −38.85 ± 0.93 mV, respectively with respect to CECT 997 and KPc21 strains without treatment with niclosamide, −36.7 ± 0.88 and −35.27 ± 0.72 mV, respectively (Figure 4).
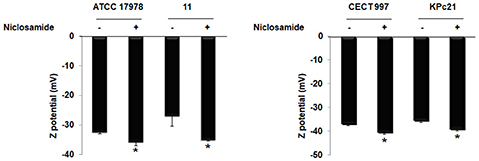
Figure 4. Zeta potential of Col-S and Col-R A. baumannii and K. pneumoniae strains in presence and absence of niclosamide. Data are the mean ± SEM. *P < 0.05 untreated vs. treated with niclosamide.
Discussion
Colistin resistance, although uncommon, is increasingly being reported among clinical Gram-negative bacilli isolates, and an understanding of its impact on the activity of antimicrobials is now evolving (Olaitan et al., 2014). Modification of LPS is one of the colistin resistance mechanisms in Gram negative bacilli that result in the increase of positive surface charge of the bacterial outer membrane (Olaitan et al., 2014).
Niclosamide's ability to carrier proton has been previously used to investigate the potential of niclosamide for blocking the acidification of endosomes in eukaryotic cells (Jurgeit et al., 2012). Thus, the use of niclosamide to carrier the proton in the outer membrane of Col-R strains could be helpful to restore the activity of colistin against Gram negative bacilli.
In the present study, the combination of niclosamide with colistin potentiates the activity of colistin against Col-S and especially Col-R A. baumannii and K. pneumoniae strains at 24 h, despite of the antagonist effect of niclosamide on the antibacterial effect of colistin against these strains in the first 4 h. Similar results regarding the antagonism of the colistin effect were observed in the first 4–8 h when colistin was combined with doripenem or ertapenem against a clinical isolate of K. pneumoniae resistant to colistin, doripenem, and ertapenem (Hong et al., 2013), and with levofloxacin against a clinical isolate of A. baumannii resistant to levofloxacin (Safarika et al., 2015). In the present study, this antagonism has been corrected when niclosamide was added 4 h after bacterial and colistin incubation.
It is noteworthy to mention that the use of niclosamide alone did not affect the growth of Col-S and Col-R A. baumannii and K. pneumoniae strains, which is consistent with previously published data in which niclosamide was not effective against Gram-negative bacilli including A. baumannii and K. pneumoniae (Rajamuthiah et al., 2015). In addition, the synergy between niclosamide and colistin was increased when colistin was added for second time 4 h post-bacterial due to a possible compensation of colistin degradation in the broth culture (Owen et al., 2007; Bergen et al., 2010).
It is well known that niclosamide does not cause significant bacterial cell envelope damage in Gram-positive pathogens (Rajamuthiah et al., 2015), and Gram-negative bacilli may have intrinsic resistance to niclosamide due to their functional and structural characteristics (Blair et al., 2014). Interestingly, despite the fact that niclosamide does not appear to inhibit A. baumannii and K. pneumoniae growth, we demonstrated, for the first time, that niclosamide increased the negative surface charge of Col-S and Col-R A. baumannii and K. pneumoniae strains. This effect was higher with Col-R than with Col-S A. baumannii and K. pneumoniae strains. This fact would be due to the proportion of negative and positive surface charge in these strains. Indeed, we observed that Col-R strains contain less negative surface charges than Col-S strains. These data are consistent with previously published reports, showing less negative surface charges in Col-R A. baumannii and K. pneumoniae (Soon et al., 2011; Velkov et al., 2014). Recently, it was reported that salicylanilide analogs inhibit Clostridioides difficile growth via membrane depolarization by dissipation of the bacterial membrane potential (Gooyit and Janda, 2016).
Furthermore, we showed that Col-R A. baumannii strain did not improve the synergy with niclosamide while adding the second colistin dose. A explanation for this data would be that the binding of colistin to the cell wall of this strain is saturated after the first addition of colistin in medium. Indeed, this strain, in presence of niclosamide, has presented lower zeta potential level (−34.95 ± 0.35 mV) than with K. pneumoniae CECT 997 strain (−40.23 ± 0.88 mV), K. pneumoniae KPc21 strain (−38.85 ± 0.93 mV), and A. baumannii ATCC 17978 strain (−35.62 ± 1.32 mV) which suggest the colistin binding saturation.
We have not shown that the incubation of A. baumannii and K. pneumoniae with niclosamide affect their OMPs profiles (data not shown). Thus, further investigations, including the integrity of bacterial membrane by transmission electron microscopy, are necessary to better understand how niclosamide acts synergistically with colistin against Col-S and Col-R A. baumannii and K. pneumoniae strains.
Concerning future developments of niclosamide as potent synergic drug with colistin, niclosamide derivative or same structural class of niclosamides, i.e., the salicylanilide oxyclozanide, need to be evaluated in combination with colistin. Previous studies showed that oxyclozanide present same activities as niclosamide against P. aeruginosa and S. aureus (Imperi et al., 2013; Rajamuthiah et al., 2015). Furthermore, It should be a very interesting exercise to assay in vitro the anti-quorum sensing of niclosamide (Imperi et al., 2013; Costabile et al., 2015) in combination with colistin and in vivo the colistin niclosamide combination in an infection model like the silk worm Galleria mellonella.
Conclusions
Niclosamide has potentiated the effect of colistin against Col-S and Col-R A. baumannii and K. pneumoniae strains. This effect might be due to the alteration of negative surface charge proportion in the outer membrane of these strains. The results of this study provide new insights into the use of niclosamide in combination with colistin to treat the infections by Gram negative bacilli.
Author Contributions
JP and YS conceived the study. RA-A, MG-M, VS-E, RP-M, and MP-I carried out the experiments. RA-A and YS analyzed the data. MJ-M, MP-I, and JP have reviewed the manuscript and the experiments. RA-A and YS wrote the manuscript. All authors read and approved the final manuscript.
Funding
This study was supported by the Instituto de Salud Carlos III, Proyectos de Investigación en Salud (grant PI16/01378). YS is supported by the Subprograma Miguel Servet Tipo I from the Ministerio de Economía y Competitividad of Spain (CP15/01358).
Conflict of Interest Statement
The authors declare that the research was conducted in the absence of any commercial or financial relationships that could be construed as a potential conflict of interest.
Acknowledgments
We thank Dr. Alvaro Pascual for the kind gift of the K. pneumoniae strains KPc07, KPc21 and KPc29. We thank Dr. Javier Sánchez-Cespedes for helping with anthelmintic drug screening.
References
European Committee on Antimicrobial Susceptibility Testing [EUCAST] (2016). European Society of Clinical Microbiology and Infectious Diseases. Clinical Breakpoints. Bochum: EUCAST.
Ah, Y. M., Kim, A. J., and Lee, J. Y. (2014). Colistin resistance in Klebsiella pneumoniae. Int. J. Antimicrob. Agents 44, 8–15. doi: 10.1016/j.ijantimicag.2014.02.016
Antunes, L. C., Imperi, F., Minandri, F., and Visca, P. (2012). in vitro and in vivo antimicrobial activities of gallium nitrate against multidrug-resistant Acinetobacter baumannii. Antimicrob. Agents Chemother. 56, 5961–5970. doi: 10.1128/AAC.01519-12
Baumann, P., Doudoroff, M., and Stanier, R. Y. (1968). A study of the Moraxella grouP. II. Oxidative-negative species (genus Acinetobacter). J. Bacteriol. 95, 1520–1541.
Bergen, P. J., Bulitta, J. B., Forrest, A., Tsuji, B. T., Li, J., and Nation, R. L. (2010). Pharmacokinetic/pharmacodynamic investigation of colistin against Pseudomonas aeruginosa using an in vitro model. Antimicrob. Agents Chemother. 54, 3783–3789. doi: 10.1128/AAC.00903-09
Blair, J. M., Webber, M. A., Baylay, A. J., Ogbolu, D. O., and Piddock, L. J. V. (2014). Molecular mechanisms of antibiotic resistance. Nat. Rev. Microbiol. 13, 42–51. doi: 10.1038/nrmicro3380
Bonnin, A. R., Nordmann, P., and Poirel, L. (2013). Screening and deciphering antibiotic resistance in Acinetobacter baumannii: a state of the art. Expert Rev. Anti. Infect. Ther. 11, 571–583. doi: 10.1586/eri.13.38
Cassir, N., Rolain, J. M., and Brouqui, P. (2014). A new strategy to fight antimicrobial resistance: the revival of old antibiotics. Front. Microbiol. 5:551. doi: 10.3389/fmicb.2014.00551
Chopra, S., Torres-Ortiz, M., Hokama, L., Madrid, P., Tanga, M., Mortelmans, K., et al. (2010). Repurposing FDA-approved drugs to combat drug-resistant Acinetobacter baumannii. J. Antimicrob. Chemother. 65, 2598–2601. doi: 10.1093/jac/dkq353
Clinical and Laboratory Standards Institute (2016). Performance Standards for Antimicrobial Susceptibility Testing. Twenty-Sixth Informational Supplement. Document M100-S26. CLSI. Wayne, PA: Clinical and Laboratory Standards Institute.
Costabile, G., d'Angelo, I., Rampioni, G., Bondi, R., Pompili, B., Ascenzioni, F., et al. (2015). Towards repositioning niclosamide for anti-virulence therapy of Pseudomonas aeruginosa lung infections: development of inhalable formulations through nanosuspension technology. Mol. Pharm. 12, 2604–2617. doi: 10.1021/acs.molpharmaceut.5b00098
Fernández-Cuenca, F., Tomás-Carmona, M., Caballero-Moyano, F., Bou, G., Martínez-Martínez, L., Vila, J., et al. (2013). in vitro activity of 18 antimicrobial agents against clinical isolates of Acinetobacter spp.: multicenter national study GEIH-REIPI-Ab 2010. Enferm Infecc Microbiol. Clin. 31, 4–9. doi: 10.1016/j.eimc.2012.06.010
Gooyit, M., and Janda, K. M. (2016). Reprofiled anthelmintics bate hypervirulent stationary-phase Clostridium difficile. Sci. Rep. 6:33642. doi: 10.1038/srep33642
Gwisai, T., Hollingsworth, N. R., Cowles, S., Tharmalingam, N., Mylonakis, E., Burgwyn Fuchs, B., et al. (2017). Repurposing niclosamide as a versatile antimicrobial surface coating against device-associated. Hospital-acquired bacterial infections. Biomed. Mater. 12:045010. doi: 10.1088/1748-605X/aa7105
Haeili, M., Javani, A., Moradi, J., Jafari, Z., Feizabadi, M. M., and Babaei, E. (2017). MgrB alterations mediate colistin resistance in Klebsiella pneumoniae isolates from Iran. Front. Microbiol. 8, 1–8. doi: 10.3389/fmicb.2017.02470
Hong, J. H., Clancy, C. J., Cheng, S., Shields, R. K., Chen, L., Doi, Y., et al. (2013). Characterization of porin expression in Klebsiella pneumoniae Carbapenemase (KPC)-producing K. pneumoniae identifies isolates most susceptible to the combination of colistin and carbapenems. Antimicrob. Agents Chemother. 57, 2147–2153. doi: 10.1128/AAC.02411-12
Imperi, F., Massai, F., Ramachandran Pillai, C., Longo, F., Zennaro, E., Rampioni, G., et al. (2013). New life for an old drug: the anthelmintic drug niclosamide inhibits Pseudomonas aeruginosa quorum sensing. Antimicrob. Agents Chemother. 57, 996–1005. doi: 10.1128/AAC.01952-12
Jurgeit, A., McDowell, R., Moese, S., Meldrum, E., Schwendener, R., and Greber, U. F. (2012). Niclosamide is a proton carrier and targets acidic endosomes with broad antiviral effects. PLoS Pathog. 8:e1002976. doi: 10.1371/journal.ppat.1002976
López-Rojas, R., Domínguez-Herrera, J., McConnell, M. J., Docobo-Peréz, F., Smani, Y., Fernández-Reyes, M., et al. (2011). Impaired virulence and in vivo fitness of colistin-resistant Acinetobacter baumannii. J. Infect. Dis. 203, 545–548. doi: 10.1093/infdis/jiq086
López-Rojas, R., García-Quintanilla, M., Labrador-Herrera, G., Pachón, J., and McConnell, M. J. (2016). Impaired growth under iron-limiting conditions associated with the acquisition of colistin resistance in Acinetobacter baumannii. Int. J. Antimicrob. Agents 47, 473–477. doi: 10.1016/j.ijantimicag.2016.03.010
Mohamed, A. F., Cars, O., and Friberg, L. E. (2014). A pharmacokinetic/pharmacodynamic model developed for the effect of colistin on Pseudomonas aeruginosa in vitro with evaluation of population pharmacokinetic variability on simulated bacterial killing. J. Antimicrob. Chemother. 69, 1350–1361. doi: 10.1093/jac/dkt520
Olaitan, A. O., Morand, S., and Rolain, J. M. (2014). Mechanisms of polymyxin resistance: acquired and intrinsic resistance in bacteria. Front. Microbiol. 5:643. doi: 10.3389/fmicb.2014.00643
Owen, R. J., Li, J., Nation, R. L., and Spelman, D. (2007). in vitro pharmacodynamics of colistin against Acinetobacter baumannii clinical isolates. J. Antimicrob. Chemother. 59, 473–477. doi: 10.1093/jac/dkl512
Pachón-Ibáñez, M. E., Labrador-Herrera, G., Cebrero-Cangueiro, T., Díaz, C., Smani, Y., Del Palacio, J. P., et al. (2018). Efficacy of colistin and its combination with rifampin in vitro and in experimental models of infection caused by carbapenemase-producing clinical isolates of Klebsiella pneumoniae. Front. Microbiol. 9:912. doi: 10.3389/fmicb.2018.00912
Paul, M., Carmeli, Y., Durante-Mangoni, E., Mouton, J. W., Tacconelli, E., Theuretzbacher, U., et al. (2014). Combination therapy for carbapenem-resistant Gram-negative bacteria. J. Antimicrob. Chemother. 69, 2305–2309. doi: 10.1093/jac/dku168
Poirel, L., Jayol, A., Bontron, S., Villegas, M.V, Ozdamar, R., Tükkoglu, S., et al. (2015). The mgrB gene as a key target for acquired resistance to colistin in Klebsiella pneumoniae. J. Antimicrob. Chemother. 70, 75–80. doi: 10.1093/jac/dku323
Poulikakos, P., Tansarli, G. S., and Falagas, M. E. (2014). Combination antibiotic treatment versus monotherapy for multidrug-resistant, extensively drug-resistant, and pandrug-resistant Acinetobacter infections: a systematic review. Eur. J. Clin. Microbiol. Infect. Dis. 33, 1675–1685. doi: 10.1007/s10096-014-2124-9
Rajamuthiah, R., Fuchs, B. B., Conery, A. L., Kim, W., Jayamani, E., Kwon, B., et al. (2015). Repurposing Salicylanilide anthelmintic drugs to combat drug resistant Staphylococcus aureus. PLoS ONE 10:e0124595. doi: 10.1371/journal.pone.0124595
Reading, C., and Cole, M. (1977). Clavulanic acid: a beta-lactamase-inhibiting beta-lactam from Streptomyces clavuligerus. Antimicrob. Agents Chemother. 11, 852–857. doi: 10.1128/AAC.11.5.852
Safarika, A., Galani, I., Pistiki, A., and Giamarellos-Bourboulis, E. J. (2015). Time-kill effect of levofloxacin on multidrug-resistant Pseudomonas aeruginosa and Acinetobacter baumannii: synergism with imipenem and colistin. Eur. J. Clin. Microbiol. Infect. Dis. 34, 317–323. doi: 10.1007/s10096-014-2231-7
Smani, Y., Domínguez-Herrera, J., and Pachón, J. (2011). Rifampin protects human lung epithelial cells against cytotoxicity induced by clinical multi and pandrug-resistant Acinetobacter baumannii. J. Infect. Dis. 203, 1110–1119. doi: 10.1093/infdis/jiq159
Soon, R. L., Nation, R. L., Cockram, S., Moffatt, J. H., Harper, M., Adler, B., et al. (2011). Different surface charge of colistin-susceptible and -resistant Acinetobacter baumannii cells measured with zeta potential as a function of growth phase and colistin treatment. J. Antimicrob. Chemother. 66, 126–133. doi: 10.1093/jac/dkq422
Tao, H., Zhang, Y., Zeng, X., Shulman, G. I., and Jin, S. (2014). Niclosamide ethanolamine-induced mild mitochondrial uncoupling improves diabetic symptoms in mice. Nat. Med. 20, 1263–1269. doi: 10.1038/nm.3699
Tharmalingam, N., Port, J., Castillo, D., and Mylonakis, E. (2018). Repurposing the anthelmintic drug niclosamide to combat Helicobacter pylori. Sci. Rep. 8:3701. doi: 10.1038/s41598-018-22037-x
Valencia, R., Arroyo, L. A., Conde, M., Aldana, J. M., Torres, M. J., Fernández-Cuenca, F., et al. (2009). Nosocomial outbreak of infection with pan-drug-resistant Acinetobacter baumannii in a tertiary care university hospital. Infect. Control HosP. Epidemiol. 30, 257–263. doi: 10.1086/595977
Velkov, T., Deris, Z. Z., Huang, J. X., Azad, M. A., Butler, M., Sivanesan, S., et al. (2014). Surface changes and polymyxin interactions with a resistant strain of Klebsiella pneumoniae. Innate Immun. 20, 350–363. doi: 10.1177/1753425913493337
Vila, J., and Pachón, J. (2012). Therapeutic options for Acinetobacter baumannii infections: an update. Expert Opin. Pharmacother. 13, 2319–2336. doi: 10.1517/14656566.2012.729820
Ye, T., Xiong, Y., Yan, Y., Xia, Y., Song, X., Liu, L., et al. (2014). The anthelmintic drug niclosamide induces apoptosis, impairs metastasis and reduces immunosuppressive cells in breast cancer model. PLoS ONE 9:e85887. doi: 10.1371/journal.pone.0085887
Younis, W., Thangamani, S., and Seleem, M. N. (2015). Repurposing Non-antimicrobial drugs and clinical molecules to treat bacterial infections. Curr. Pharm. Des. 21, 4106–41011. doi: 10.2174/1381612821666150506154434
Zemke, A. C., Shiva, S., Burns, J. L., Moskowitz, S. M., Pilewski, J. M., Gladwin, M. T., et al. (2014). Nitrite modulates bacterial antibiotic susceptibility and biofilm formation in association with airway epithelial cells. Free Radic. Biol. Med. 77, 307–316. doi: 10.1016/j.freeradbiomed.2014.08.011
Keywords: repurposing drug, niclosamide, colistin, synergistic effect, Acinetobacter baumannii, Klebsiella pneumoniae
Citation: Ayerbe-Algaba R, Gil-Marqués ML, Jiménez-Mejías ME, Sánchez-Encinales V, Parra-Millán R, Pachón-Ibáñez ME, Pachón J and Smani Y (2018) Synergistic Activity of Niclosamide in Combination With Colistin Against Colistin-Susceptible and Colistin-Resistant Acinetobacter baumannii and Klebsiella pneumoniae. Front. Cell. Infect. Microbiol. 8:348. doi: 10.3389/fcimb.2018.00348
Received: 09 August 2018; Accepted: 11 September 2018;
Published: 03 October 2018.
Edited by:
Rodolfo García-Contreras, Universidad Nacional Autónoma de México, MexicoReviewed by:
Rafael Franco, Instituto Nacional de Rehabilitación, MexicoYael González Tinoco, Centro de Investigación Científica y de Educación Superior de Ensenada (CICESE), Mexico
Riti Sharan, Texas A&M Health Science Center, United States
Copyright © 2018 Ayerbe-Algaba, Gil-Marqués, Jiménez-Mejías, Sánchez-Encinales, Parra-Millán, Pachón-Ibáñez, Pachón and Smani. This is an open-access article distributed under the terms of the Creative Commons Attribution License (CC BY). The use, distribution or reproduction in other forums is permitted, provided the original author(s) and the copyright owner(s) are credited and that the original publication in this journal is cited, in accordance with accepted academic practice. No use, distribution or reproduction is permitted which does not comply with these terms.
*Correspondence: Jerónimo Pachón, pachon@us.es
Younes Smani, ysmani-ibis@us.es