Interactions Between Gut Microbiota, Host, and Herbal Medicines: A Review of New Insights Into the Pathogenesis and Treatment of Type 2 Diabetes
- 1Department of Endocrinology, Guang'anmen Hospital, China Academy of Chinese Medical Sciences, Beijing, China
- 2Graduate School, Beijing University of Chinese Medicine, Beijing, China
- 3Molecular Biology Laboratory, Guang'anmen Hospital, Chinese Academy of Traditional Chinese Medicine, Beijing, China
Herbal medicines (HMs) are a major subset of complementary and alternative medicine. They have been employed for the efficient clinical management of type 2 diabetes mellitus (T2DM) for centuries. However, the related underlying mechanisms still remain to be elucidated. It has been found out that microbiota is implicated in the pathogenesis and treatment of T2DM. An interplay between gut microbiota and host occurs mainly at the gastrointestinal mucosal barrier. The host movements influence the composition and abundance of gut microbiota, whereas gut microbiota in turn modulate the metabolic and immunological activities of the host. Intestinal dysbiosis, endotoxin-induced metabolic inflammation, immune response disorder, bacterial components and metabolites, and decreased production of short-chain fatty acids are considered significant pathogenic mechanisms underlying T2DM. The interaction between gut microbiota and HMs during T2DM treatment has been investigated in human, animal, and in vitro studies. HMs regulate the composition of beneficial and harmful bacteria and decrease the inflammation caused by gut microbiota. Furthermore, the metabolism of gut microbiota modulates HM biotransformation. In this review, we have summarized such research findings, with the aim to improve our understanding of the pathogenesis and potential therapeutic mechanisms of HMs in T2DM and to provide new insights into specific targeted HM-based therapies and drug discovery.
Introduction
Diabetes mellitus (DM) is a widely prevalent chronic disease associated with significant healthcare problems worldwide. According to the International Diabetes Federation (IDF), ~425 million adults suffered from DM in 2017, and the number is expected to increase to 629 million by 2045 (Cho et al., 2018). The prevalence of DM varies between developing and developed countries and is estimated to increase in these countries by 69 and 20% from 2010 to 2030, respectively (Shaw et al., 2010). Long-term DM can result in multiple complications and comorbidities, affecting the eyes, kidneys, cardiovascular system, and nervous system (Inzucchi et al., 2012). The mortality rates of adult patients with DM are reportedly two to four times higher than in adults without diabetes (Gerrits et al., 2015). Type 2 diabetes mellitus (T2DM) is one of the main types of DM. It is characterized by hyperglycemia resulting from progressive β-cell dysfunction in the presence of insulin resistance (American Diabetes Association, 2019). Furthermore, T2DM accounts for more than 90% of all diabetic patients, leading to a public pandemic (Chatterjee et al., 2017). The prevalence of T2DM has increased markedly over the years, causing a significant global burden of mortality and disability (Zheng et al., 2017). Obesity is the most prominent risk factor for T2DM. Other risk factors include a low-fiber diet and an unhealthy lifestyle, including inactivity, smoking, and alcohol consumption (Wu et al., 2014; Kautzky-Willer et al., 2016). There are various therapeutic approaches to T2DM, such as lifestyle management (Schellenberg et al., 2013) (self-management education and support, nutrition therapy, physical activity, dietary planning, and psychosocial care), oral medications (Qaseem et al., 2017) (metformin, sulfonylureas, thiazolidinediones, α-glucoside inhibitors, dipeptidyl peptidase-4 inhibitors, and sodium-dependent glucose transporter 2), injectable medications (Tran et al., 2015) (insulin and glucagon-like peptide-1), surgery (Maleckas et al., 2015), and complementary and alternative medications (Nahas and Moher, 2009).
With the rising importance of complementary and alternative medicine according to the recommendations of the World Health Organization (WHO) (Zhang Q. et al., 2019), the application and research of alternative medications for the treatment of T2DM have markedly increased in recent years (Al-Eidi et al., 2016; Pang et al., 2019). As one of the paramount types of alternative medicine, herbal medicine (HM) has thousand years of history and includes systematic medical theories based on long periods of phenotype-based and personalized clinical trials (Li and Weng, 2017). The WHO estimated that 70–80% of populations living in developing countries considered HM a primary healthcare approach, while the studies of HM are still at the preliminary stage and need further research on the efficacy mechanisms (Ekor, 2014). During the past two decades, HM has played an active role in the treatment of DM and has proved to be valuable for the prevention of disease progression in both European and Asian countries (Banjari et al., 2019). According to the world ethnobotanical information, ~800 herbs have been applied for the control of DM (Alarcon-Aguilara et al., 1998). A large amount of clinical trials and animal tests have demonstrated the effect of various forms of HM, such as the use of single herbs and their extracts (Pang et al., 2015; Mirfeizi et al., 2016), herbal medicine decoctions (Zhang et al., 2011; Ryuk et al., 2017), and Chinese patent medicines (Pang et al., 2017; Chen et al., 2019). Besides establishing the efficacy of HMs against T2DM, studies have also aimed to identify their therapeutic mechanisms using modern science and technology; however, these mechanisms remain to be elucidated.
The gut represents the largest digestive and immune organ of the human body, which harbors trillions of microbes. The microbes inhabiting the gut, also known as gut microbiota, compose a complex ecological community and greatly impact the host health (Lozupone et al., 2012). Nowadays, an increasing number of researchers have focused on the role of gut microbiota in disease and drug treatment, including the interaction of gut microbiota, T2DM, and HM. New perspectives based on gut microbiota have provided interesting insights into the mechanism of the action of HMs in T2DM treatment.
Here, we aim to provide an overview of the relationship among gut microbiota, host, and T2DM from a pathological perspective, including changes of gut microbiota and how they interact with the host in T2DM. Next, we describe the interaction of gut microbiota, host, and HMs in T2DM treatment, which facilitates the understanding of the potential therapeutic mechanisms of HMs. Finally, we summarize and discuss the HM therapeutic strategy based on gut microbiota and present our perspectives.
Host, Gut Microbiota, and T2DM: Pathogenesis
Interplay Between Gut Microbiota and Host
The human intestine is a complex ecosystem. The microbiota and host share an extensive platform for intercellular signaling and defense against potential pathogens (Sekirov et al., 2010). In healthy humans, ~3.8 × 1013 bacteria colonize the intestine, which together code for over three million genes (Qin et al., 2010). Classifying bacteria by the phylogenetic diversity of variable nucleotide sequences of small subunit ribosomal RNA operons or 16S rRNA genes allows the analysis of the huge microbial community. A diversity of organismal assemblages can yield a core unit at the functional level, and deviations from this core are associated with different physiological states (Turnbaugh et al., 2009).
Microbiome analysis has revealed that disease progression is associated with changes in the fecal microbiome. Research on animal models has indicated that different responses based on host genotypes may contribute to the development of metabolic disorder phenotypes linked with gut microbiota alterations (Miranda-Ribera et al., 2019; Wang J. H. et al., 2019). In healthy individuals, most intestinal bacteria can be classified under five phyla, Firmicutes, Bacteroidetes, Actinobacteria, Proteobacteria, and Verrucomicrobia (Tremaroli and Backhed, 2012). All these microbes express genes for the production short-chain fatty acids (SCFAs) (Krautkramer et al., 2016), ligands for G-protein-coupled receptors (GPCRs) (Cohen et al., 2017), neurotransmitters (Asano et al., 2012), and other metabolites. In turn, the metabolites genetically and epigenetically influence the host responses (Sharkey et al., 2018). As for the hosts, intrinsic and extrinsic factors both influence the gut bacterial composition. Through delivery at birth, infant feeding, genetics, infections, medications, and diet, the host internal environment changes frequently, leading to congruent alterations of the gut microbiota (Wen and Duffy, 2017). Basically, the host vital movements influence the gut microbiota abundance, whereas the gut microbiota control the metabolic physiological state and immunological functions of the host through a series of gene-regulated mechanisms.
Most of the interactions between the host and gut microbiota occur at the gastrointestinal barrier, which consists of two parts: bacterial and mucosal barriers. Both are crucial for the prevention of passage of commensal bacteria, pathogens, and food antigens from the lumen into the gut tissue and host systemic circulation (Sorini et al., 2019). The bacterial barrier is the first line of defense against luminal content penetration and performs numerous biological functions. The gut microbiota differs along the longitudinal axis of the gut. Thus, various bacteria colonize different places in the intestine. Due to the radial oxygen gradient, microbes residing on the colonic mucosa harbor higher oxygen tolerance and catalase expression compared to luminal or stool-associated bacteria (Albenberg et al., 2014). As for the mucosal barrier, the intestinal epithelium contains a large surface that is lined by a monolayer of intestinal epithelial cells (IECs) (Wu et al., 2019). They create mucosal barriers including both physical and chemical barriers for the maintenance of a symbiotic relationship between the gut microbiota and host (Okumura and Takeda, 2017). Once the intestinal barrier is disrupted, some of the intestinal physiological functions will be impaired, which may cause circular responses of intestinal dysbiosis, inflammation, and enzymatic machinery and immune response disorders.
How the Gut Microbiota Affect T2DM
Intestinal Dysbiosis
Dysbiosis is characterized by a loss of beneficial microorganisms, an expansion of potentially harmful microbes and/or a loss of overall microbial diversity (Olesen and Alm, 2016). Obesity and polymetabolic disorders lead to an imbalance of gut microbiota, which is considered a characteristic of T2DM. Intestinal dysbiosis leads to the translocation of bacterial metabolites, such as trimethylamine (TMA), mediators of metabolic dysregulation, and pathogen-associated molecular patterns (PAMPs) (Tilg et al., 2020). Bacteroidetes and Firmicutes are the two dominant bacterial phyla in T2DM patients gut microbiota. Interestingly, the ratio of Bacteroidetes to Firmicutes has been positively and significantly correlated with plasma glucose concentrations in many previous studies (Turnbaugh et al., 2009; Larsen et al., 2010; Schwiertz et al., 2010). The Bacteroidetes content rises with weight loss and low-calorie diet, which is beneficial to the recovery from T2DM (Ley et al., 2006). In T2DM patients, the abundance of lipopolysaccharide-producing Escherichia coli (phylum Proteobacteria) increases. They contribute to enhanced systemic inflammation of the intestine (Qin et al., 2012). Contrarily, Akkermansia muciniphila and Faecalibacterium prausnitzii are highly abundant human gut microbes in healthy individuals, and their reduced levels are associated with inflammation and alterations of metabolic processes involved in the development of T2DM (Verhoog et al., 2019). The significance of Akkermansia muciniphila for the maintenance of the gastrointestinal tract integrity has recently been identified. Its metabolites affect a number of transcription factors and genes involved in cellular lipid metabolism, which is crucial for the precession of metabolic syndrome and T2DM. Akkermansia muciniphila and its metabolite propionate modulate the expression of Fiaf, GPR43, histone deacetylases (HDACs), and peroxisome proliferator-activated receptor gamma (PPAR gamma), and they play an important role in the regulation of transcription factor function, cell cycle, lipolysis, and satiety (Lukovac et al., 2014). Intestinal dysbiosis alters the microbiome to upregulate the membrane transport of sugars and transport of branched-chain amino acids and to downregulate butyrate biosynthesis (Luca et al., 2019). The enriched microbial genes mapped to oxidative stress signaling suggest a direct link between the altered microbiota composition and inflammatory state in patients with T2DM (Tilg et al., 2020). The dysbiosis-provoked rupture of the gut barrier leads to local and systemic inflammation, which is relevant to the development of T2DM (Belizario et al., 2018).
Endotoxin-Promoted Metabolic Inflammation
T2DM is, to a certain degree, an inflammatory disease, and several inflammatory molecules are indicative of the development of T2DM (Zhou W. Y. et al., 2019). Microbiota modulate the expression of PPARs in intestinal epithelial and immune modulatory cells and alter the host inflammatory responses (Hasan et al., 2019). Meanwhile, homeostatic imbalance or disruption facilitates the translocation of endotoxins like lipopolysaccharide (LPS) into the circulation, which results in enhanced systemic and intestinal inflammation and gastrointestinal wall permeability. In diabetes, the gut microbiota contribute to the pathophysiological regulation of endotoxemia and increase the intestinal permeability due to malfunction of tight junction proteins, such as occuludin and ZO-1 (Cani et al., 2007b, 2008). This increases the plasma levels of LPS, which causes low-grade inflammation in the circulation and eventually, insulin resistance (IR) (Cani et al., 2007a). As a coreceptor for the monocyte differentiation antigen CD14+, TLR4 mediates various LPS-induced inflammatory cascades and the development of the innate immune response, which consists of recognition receptors (PRRs), antimicrobial peptides, and secreted IgA (Creely et al., 2007; Jialal and Rajamani, 2014). Chronic low-grade inflammation ensues with the activation of proinflammatory pathways, contributing to obesity, IR, pancreatic β-cell decline, and eventually T2DM (Lew et al., 2019). LPS is one of the PAMPs, which are recognized by the PRRs, including the Toll-like receptors (TLRs) and Nod-like receptors (NLRs). The interaction between PRRs and LPS induces cytokine and interferon production. In turn, they trigger proinflammatory signaling cascades in human peripheral tissues (Zhao C. et al., 2019). Inflammation promotes an oxidative state, which enhances the enrichment of aerotolerant phyla. It also increases the production of terminal electron acceptors for facultative anaerobes. This means that the inflammatory state contributes to the severity of the intestinal dysbiosis, which promotes the destruction of the bacterial barrier (Winter et al., 2013).
Reduced Short-Chain Fatty Acid Production
SCFAs are byproducts of anaerobic microbial fermentation of undigested food in the large intestine (Wisniewski et al., 2019). They can modulate the host energy homeostasis through interactions between chemosensory enteroendocrine cells, which belong to epithelial cells and can supply energy themselves (Kuwahara, 2014). There is evidence that SCFAs increase the pancreatic β-cell mass and insulin secretion, reduce glucagon secretion, and regulate glucose metabolism (Mandaliya and Seshadri, 2019b). Intestinal dysbiosis may change the ratio of anaerobic and aerobic bacteria, which leads directly to SCFA reduction. SCFAs, including propionate, butyrate, and acetate, can trigger the local release of peptide YY (PYY) and GLP1. SCFA receptors are highly expressed on GLP1-producing L cells in the distal ileum and colon (Mandaliya and Seshadri, 2019a). Propionate is a substrate for gluconeogenesis that protects the host from diet-induced obesity and glucose intolerance (Ohira et al., 2017). Butyrate, a SCFA fermented by microbiota, is an energy substrate for colonocytes that decreases the permeability of the intestinal barrier by promoting GLP-2 release and increasing mucus secretion (Sonnenburg and Backhed, 2016). Butyrate may suppress the abnormally increased proliferation of colonic epithelial cells in diabetes by targeting HMGB1 (Wang S. Y. et al., 2019). Furthermore, as a histone deacetylase (HDAC) inhibitor, butyrate can promote β-cell differention, proliferation, function and improve insulin, which has a close link with diabetes (Khan and Jena, 2015). Researchers also demonstrated that butyrate and propionate activate intestinal gluconeogenesis (IGN) via cAMP-dependent mechanism and a gut-brain neural circuit involving the fatty acid receptor FFARS, which had a metabolic benefits on glucose control in mice (De Vadder et al., 2016). As for acetate, it's found that increased production of this SCFA promotes increased glucose-stimulated insulin secretion through activation of the parasympathetic nervous system (Perry et al., 2016).
In summary, due to the intestinal dysbiosis, the endotoxin-mediated promotion of metabolic inflammation and reduction of SCFA levels are the two main mechanisms underlying the interplay between gut microbiota and host in T2DM (Figure 1).
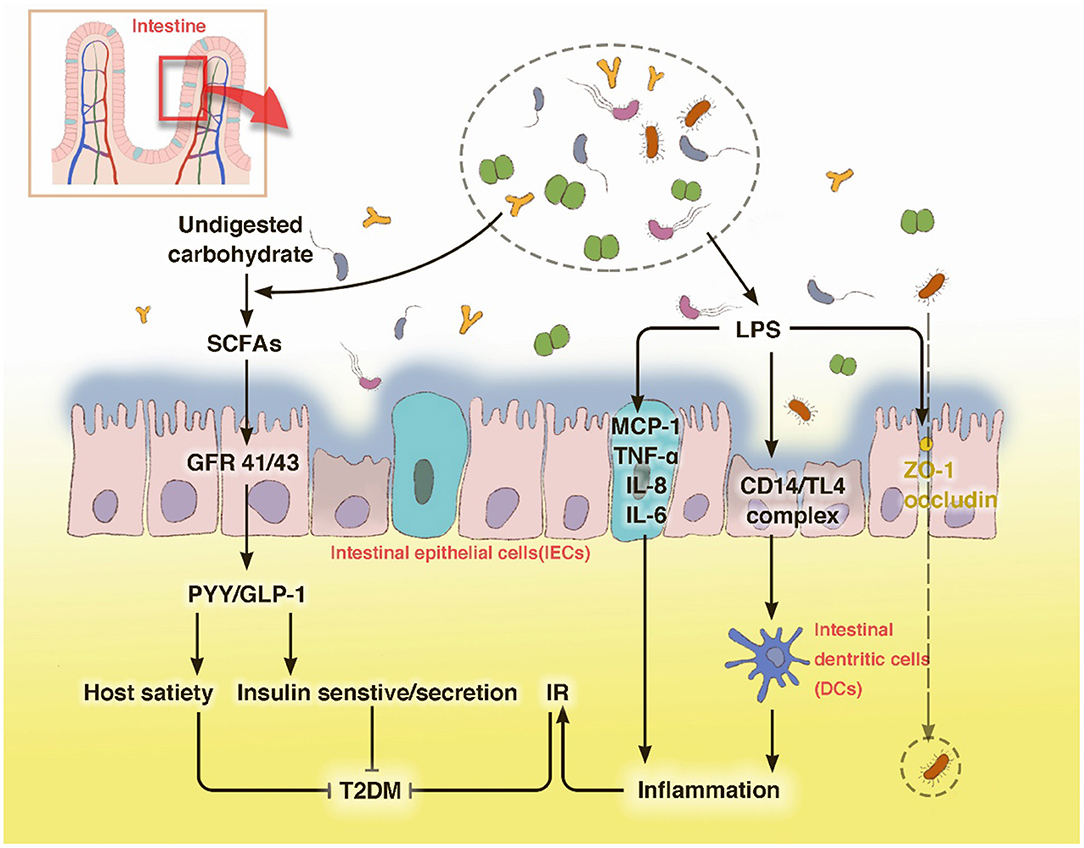
Figure 1. T2DM modulates the abundance of specific gut bacteria to decrease mucus-producing species and several short chain fatty acid (SCFA)-producing bacteria. These bacteria then produce LPS, which can destroy the barrier function of the gut and leak out to the internal environment through occludin and ZO-1, leading to an inflammatory status. The low circulating levels of SCFAs can foster host satiety disorder and a decrease in insulin sensitivity or secretion through GPRs. The disturbance of carbohydrate metabolism may cause an intestinal barrier disruption, which leads to a severer intestinal dysbiosis. With LPS stimulation, IECs secrete the pro-inflammatory cytokines MCP-1, TNF-α, IL-18, and IL-6. Innate immune cells, such as macrophages and DCs, use pattern recognition receptors to attach to pathogens or toxins, such as LPS, which leads to an activation of the inflammatory cascade.
Metabolic Dysfunction Induced by Bacterial Components and Metabolites
During food digestion, xenobiotic metabolism and vital movements of the host activate a series of events via enzymatic pathways to function in combination with the gut (Tremaroli and Backhed, 2012). Bacterial components contribute to the production of many bioactive molecule types like bile acids and adipokines, which are essential for the interconnected pathways of glycolysis, tricarboxylic acid/Krebs cycle, oxidative phosphorylation (OXPHOS), and amino acid and fatty acid metabolism (Belizario et al., 2018). Bile acids are molecules generated from cholesterol by microbiota of the lower small intestine and colon. They reportedly inhibit diet-induced obesity and prevent the development of IR by activating the bile acid receptor (farnesoid × receptor, FXR) and membrane G protein coupled receptor TGR5, indicating their effects on energy homeostasis (Gastaldelli et al., 2010; Gerard and Vidal, 2019). The ability to metabolize the naturally occurring FXR antagonist tauro-b-muricholic acid is an essential step toward impaired tolerance to glucose and insulin (Lazar et al., 2019). Adipose tissue is commonly recognized as an active organ presenting key metabolic and endocrine functions by secreting bioactive peptides and proteins, referred to as adipokines, which play a critical role in host metabolism. With the impact of gut microbiota it releases inflammatory adipokines, such as fatty acid binding protein 4 (FABP-4), acylation-stimulating protein (ASP), retinol-binding protein 4 (RBP4), lipocalin-2 (LCN2), and chemerin, etc., which are associated with increased inflammation, obesity, insulin resistance, and eventually T2DM (Lee et al., 2019) (Figure 2).
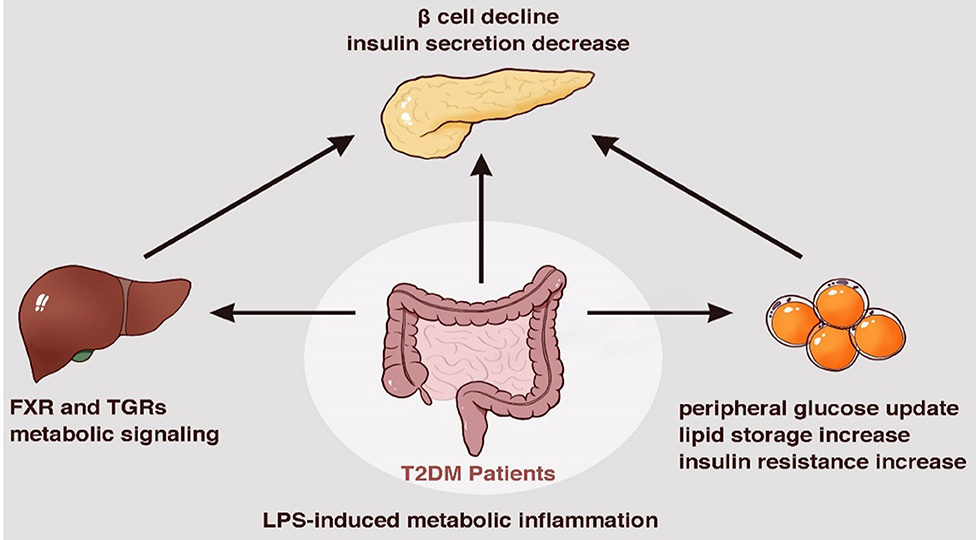
Figure 2. Metabolic dysfunction induced by bacterial components and metabolites a series of enzymatic machinery reactions, leading to insulin resistance.
Host-Gut Microbiota-Herbal Medicine Interaction During T2DM Treatment
HMs Regulate the Composition of Beneficial and Maleficent Bacteria
A significant difference between the gut microbiota composition of T2DM patients and healthy individuals was discovered as early as in 2010. The ratios of Bacteroidetes to Firmicutes and the Bacteroides-Prevotella group to C. coccoides-E. rectale group were closely related to blood glucose concentration; furthermore, members of Betaproteobacteria were highly enriched in T2DM patients (Larsen et al., 2010). With the increasing evidence from research, the imbalance of beneficial and maleficent bacteria is considered important for the pathogenesis of T2DM. SCFAs, including butyrate, propionate, and acetate, are associated with attenuated obesity and T2DM (Ju et al., 2019). The SCFA butyrate is especially beneficial for the improvement of T2DM by increasing insulin sensitivity and enhancing mitochondrial function (Gao et al., 2009). The increase in the abundance of SCFA-producing, and especially, butyrate-producing bacteria and the decline of the abundance of opportunistic pathogens are crucial mechanisms underlying T2DM treatment (Zhang B. et al., 2019).
Rhubarb is a perennial herb used for the therapy of inflammatory diseases including acute pancreatitis and gastroenteritis and of diabetes and its complications in combination with other herbs (Li et al., 2004; Zhou et al., 2016; Cao et al., 2017). Anthraquinone, which contains free anthraquinones and glycosides, is one of its major components (Arvindekar et al., 2015). A recent animal study found that the therapeutic mechanism of a purified anthraquinone-glycoside preparation from rhubarb (RAGP) for T2DM involves an improvement of gut dysbiosis with an enrichment of probiotic Lactobacillus and short-chain fatty acid-producing bacteria and decreased abundance of the Lachnospiraceae NK4A136 group and LPS-producing bacteria Desulfovibrio (Cui et al., 2019).
Water extract of Caulis Spatholobi (WECS) effectively maintained blood glucose homeostasis and reduced insulin resistance in a study using a diet-introduced obesity (DIO) mouse model. It improved not only laboratory indicators related to diabetes but also microbiota dysbiosis, mainly by increasing the abundance of anti-obesity and anti-diabetes-related bacterial genera, including Parabacteroides, Bacteroidetes, Anaerotruncus, and Bifidobacterium (Zhang C. et al., 2019).
Xiexin Tang (XXT) is a Chinese herbal formula commonly applied for the treatment of diabetes. In an in vivo study, XXT notably shifted the gut microbiota of T2DM rats. It increased the abundance of SCFA-producing and anti-inflammatory bacteria, such as Adlercreutzia, Alloprevotella, Barnesiella, [Eubacterium] Ventriosum group, Blautia, Lachnospiraceae UCG-001, Papillibacter, and Prevotellaceae NK3B31 group to different degrees. The changes of gut microbiota composition were consistent with the amelioration of T2DM rat hyperglycemia, lipid metabolism disorder, and inflammatory activities (Wei et al., 2018).
Huang-Lian-Jie-Du decoction (HLJDD), a famous Chinese herbal formula originating from the Tang Dynasty, has been widely applied in T2DM treatment for thousands of years (Zhang et al., 2014). Despite its clinical hypoglycemic effect, the underlying mechanism is unclear. Recently, an animal study found that HLJDD ameliorated blood glucose and restored the dysregulated microbiota composition. HLJDD-induced hyperglycemia improvement was mainly related to an increase in the abundance of SCFA-producing bacteria, such as Adlercreutzia, Porphyromonadaceae (including Parabacteroides), Lachnospiraceae (including Blautia), and a decrease of conditioned pathogenic bacteria, such as Corynebacteriaceae (including Corynebacterium), Staphylococcaceae (including Staphylococcus), and Aerococcaceae (including Aerococcus and Facklamia) (Chen et al., 2018).
Besides animal experiments, gradually accumulating clinical studies exploring and verifying the intestinal microbial mechanisms behind the hypoglycemic effect of HM in T2DM treatment have also emerged. They have mainly focused on the modulation of gut microbiota composition. A clinical trial on the anti-diabetic effects of a traditional Chinese herbal formula named Gegen Qinlian decoction (GQD) found a correlation between the increase in the abundance of beneficial gut microbiota and its clinical efficacy, including blood glucose levels and islet β-cell function. Furthermore, the butyrate-producing bacteria Faecalibacterium prausnitzii were significantly enriched in the feces of T2DM patients after 12 weeks of intervention (Xu et al., 2015). A randomized clinical trial on the efficacy of a specially designed Chinese herbal formula named AMC for T2DM with hyperlipidemia indicated that AMC significantly ameliorated blood glucose and lipid levels and improved HOMA-IR and triglyceride levels with higher efficacy than metformin. The study confirmed that these effects were associated with butyrate-producing bacteria, such as Faecalibacterium spp. and Blautia (Tong et al., 2018) (Table 1).
HMs Reduce Inflammation Caused by Gut Microbiota and Host Immunity
The pathogenesis of T2DM is closely associated with a low-grade inflammatory state and an activation of the host immune response, also called an ongoing cytokine-induced acute-phase response (Pickup, 2004; Donath and Shoelson, 2011). In T2DM pathogenesis, LPS released by gram-negative bacteria enters the enterohepatic circulation due to an intestinal microecological disorder, which can initiate the immune response in adipose tissue. The expression of TLRs is activated and proinflammatory adipocytokines, such as IL-1, IL-6, and TNF-α are released, resulting in a low-grade inflammatory state (Creely et al., 2007). T cells, as a critical effector of cell-mediated immunity, are crucial for the development of T2DM and the associated inflammation. T cell metabolism is closely related to insulin and its downstream signaling through the insulin receptor, and the lack of insulin receptors on T cells can inhibit glycolysis (Tsai et al., 2018). Besides, accumulating evidence links CD4+ T cells to obesity and insulin resistance, which are major risk factors for T2DM (Xia et al., 2017). Subtypes of CD4+ T cells, including Th1 and Th2 cells, can produce large amounts of proinflammatory cytokines after their activation to regulate inflammatory processes (Kahn et al., 2006; Raphael et al., 2015). With the accumulating evidence of gut microbiota playing a significant role in host immunity and subsequent inflammation, the related mechanisms of HMs in T2DM treatment are gradually emerging. The therapeutic mechanisms underlying their efficacy have been associated with intestinal anti-inflammation and immunomodulation.
Scutellaria Radix (SR) and Coptidis Rhizome (CR) are well-known herbs applied for diabetes treatment since thousands of years and have demonstrated hypoglycemic effects in both clinical trials and basic experiments (Zhang et al., 2018; Ran et al., 2019). The combination of SR and CR (SC) exerted anti-diabetic activities through the TLR4 signaling pathway involved in anti-inflammation and gut microbiota regulation. The administration of SC in a T2DM KK-Ay mouse model was associated with significantly decreased LPS, IL-6, TNF-α, TLR4, and MyD88 protein levels and improved blood glucose, insulin, and blood lipid content; moreover, Lactobacillus intestinalis was considered a possible targeted probiotic (Zhang C. H. et al., 2019).
Potentilla discolor Bunge (PDB) is a perennial herb usually utilized as an anti-diabetic agent. In an animal study, its hypoglycemic effect was closely related with the regulation of intestinal endotoxemia and inflammation. Compared with control mice, T2D mice treated with PDB presented a significant decrease in TNF-α, IL-1β, and IL-6 serum levels and LPS in feces and serum. Furthermore, the abundance of the Bacteroidales_S24-7_group was increased after PDB administration, accompanied by a decreased abundance of Helicobacteraceae (Han et al., 2019).
Dendrobium is a HM clinically used for the treatment of T2DM and its complications, and dendrobium polyphenols are its main constituents that exert diverse pharmacological effects (Paudel et al., 2018). A study on the effects of a polyphenol-rich extract of Dendrobium loddigesii (DJP) in diabetic mice identified anti-inflammatory activities of DJP for T2DM treatment through a reduction of IL-6 and TNF-α expression, which was correlated with the modulation of gut microbiota, including an increase of the Bacteroidetes to Firmicutes ratio and Prevotella/Akkermansia abundance and a decrease in the abundance of S24-7/Rikenella/Escherichia coli (Li et al., 2018) (Table 1).
Gut Microbiota-Mediated Metabolism Modulates the Biotransformation of HMs
The clinical application of HMs for T2DM treatment has been widely accepted globally. However, there is still a huge gap in terms of an approval by the US Food and Drug Administration and drug discovery due to the low bioavailability and bioactivity of HMs after oral administration (Kesarwani et al., 2013). In recent years, scientists have found that gut microbiota critically modulate the biotransformation of hypoglycemic HMs, transforming polar and poor lipophilic compounds to less polar and more lipophilic compounds and improving their oral absorption rate for T2DM treatment. Specifically, gut microbiomes encode various enzymes, which can metabolize HMs, modify the structure of the original chemicals, and produce new compounds (Koppel et al., 2017). After the metabolism of gut microbiomes, the bioavailability and bioactivity of newly produced chemical compounds differ from those of the original HM chemicals, and compounds with higher bioavailability and bioactivity can be easily absorbed by the intestine and produce therapeutic effects in the host. During the process of HM biotransformation, a gut bacterial strain can transform various chemical compounds. Meanwhile, a compound can also be transformed by the synergistic effects of various bacteria.
Berberine (BBR) is a representative constituent of a commonly used hypoglycemic HM CR (Wang J. et al., 2019). Numerous studies have demonstrated the hypoglycemic effects of BBR in T2DM (Zhang et al., 2010; Liang et al., 2019). However, as an isoquinoline alkaloid with poor water solubility, BBR is poorly absorbed by intestinal epithelial cells, which leads to its extremely low bioavailability (Liu et al., 2016). Recent studies have found that gut microbiota play an important role in BBR biotransformation and its antidiabetic effects. In the host intestine, gut microbiota convert BBR to a more easily absorbable but inactive metabolite, dihydroberberine (dhBBR), through catalysis of nitroreductases. After dhBBR absorption into intestinal wall tissue, it is oxidized immediately to BBR and exerts pharmacological activities in blood circulation (Feng et al., 2015).
Ginseng is a HM, which has been applied for T2DM treatment for thousands of years. Ginseng beneficial effects in clinical trials include improved blood glucose control and insulin sensitivity in T2DM patients (Gui et al., 2016). Ginsenosides, the main active components of ginseng, exert hypoglycemic effects in type 2 diabetic rats, including lowering blood glucose levels, modulating insulin response, and decreasing body weight (Tian et al., 2018). The metabolism of gut microbiota has a marked influence on ginsenoside pharmacological effects. Ginsenoside Rb1, an anti-diabetic agent belonging to tetracyclic triterpenoid saponins, has low bioavailability (Yu et al., 2018; Zhou P. et al., 2019). However, after catalysis of β-glucosidases produced by gut bacteria, ginsenoside Rb1 can be metabolized to more bioactive compounds, such as ginsenoside 20(S)-Rg3 and compound K (Jung et al., 2012; Quan et al., 2012; Kim, 2013). Ginsenosides Re and Rg1 are also transformed to new metabolites like the rare ginsenosides Rd, GypXVII, Rg2, and protopanaxatriol, which exhibit higher biological and pharmacological activities (Yu et al., 2017).
Curcumin, one of the primary active constituents of the HM turmeric, possesses a range of pharmacological activities including anti-diabetic, anti-inflammatory, and antioxidant effects and is protective against diabetes and its complications (Nabavi et al., 2015; Parsamanesh et al., 2018). However, as a polyphenolic compound, curcumin's poor oral bioavailability represents a big barrier to its clinical efficacy (Lopresti, 2018). Gut microbiota have been identified as a key point in the biotransformation of curcumin. Curcumin can be metabolized through the metabolism of human intestinal bacterium Blautia sp. MRG-PMF1 is converted to demethylcurcumin and bisdemethylcurcumin by a methyl aryl ether cleavage reaction (Burapan et al., 2017). Besides, in an in vitro human fecal incubation experiment three metabolites, tetrahydrocurcumin (THC), dihydroferulic acid (DFA), and a metabolite tentatively identified as 1-(4-hydroxy-3-methoxyphenyl)-2-propanol, were detected in the mixture containing curcumin after human fecal fermentation (Tan et al., 2015). The bacterium Escherichia coli from human feces was discovered in the microbial biotransformation of curcumin. Through a two-step reduction by an unique enzyme purified from Escherichia coli, which was named “NADPH-dependent curcumin/dihydrocurcumin reductase”, curcumin could be converted into dihydrocurcumin as an intermediate metabolite and tetrahydrocurcumin as the end product (Hassaninasab et al., 2011).
Quercitrin is a bioflavonoid present in various anti-diabetic HMs, such as Mori Folium, Bupleurum Radix, and hawthorn that has a positive effect on carbohydrate metabolism and antioxidant activities during diabetic treatment (Babujanarthanam et al., 2010, 2011). However, due to its relatively poor bioavailability, the key factors mediating its beneficial effects and underlying mechanism remain elusive. An in vivo experiment incubating quercetin with human gut bacteria found that metabolites with higher bioactivity were produced, including quercetin, 3,4-dihydroxyphenylacetic acid, and 4-hydroxybenzoic acid. Fusobacterium K-60 was detected as the main bacterium, which transformed quercitrin to quercetin (Kim et al., 1999).
Discussion and Perspectives
In recent years, the mechanisms of T2DM-associated alterations in gut microbiota are being constantly explored. Intestinal dysbiosis, endotoxin-induced metabolic inflammation, immune response disorder, bacterial components and metabolites, and a decreasing production of SCFAs are closely associated with the pathogenesis of T2DM and represent a mechanism of gut microbiota-host interplay. However, research on the hypoglycemic effects of HMs targeting gut microbiota is only in its infancy. Our analysis of relevant clinical studies showed that most merely analyzed the modulation of gut microbiota composition by HMs, whereas the underlying mechanisms, including the interaction mode of floras with HMs, the affected host pathways, and the therapeutic targets were rarely studied. Therefore, we believe that from the perspective of gut microbiota, investigating the therapeutic mechanisms of hypoglycemic HMs has great potential.
HM is the major component of the traditional Chinese medicine (TCM) system and is considered the “basis of all other medicines” (An et al., 2019). For thousands of years, it has not been scientifically recognized, because this system and its theory are totally different from modern science. The core of the TCM system is the balance and imbalance of Yin and Yang, which is difficult to quantify and characterize, whereas modern science focuses on objective and quantifiable evidence. Although prescriptions of multiple HM combinations based on the TCM theory have achieved notable clinical efficacy, the poor knowledge of their mechanisms has greatly limited TCM and HM progress. However, research on the interaction between gut microbiota and HM presents scientific evidence for TCM and HM utility and rational compatibility, which greatly increases the optimism for its use in the clinical practice. In TCM prescriptions, multiple HM combinations exert synergistic or antagonistic effects to obtain better efficacy or reduce toxicity and adverse effects. For example, for the treatment of T2DM, CR, and SR are commonly combined to lower blood glucose, because according to the TCM theory, they both have a bitter flavor and can combat high sugar. As obscure as the theory is, gut microbiota can be a perfect explanation why the main components of CR and SR, namely berberine and baicalin, can improve the imbalance of gut microbiome in the host, increase the abundance of various SCFA-producing bacteria, and decrease the abundance of harmful bacteria (Zhang et al., 2015; Ju et al., 2019). However, an antagonism between CR and SR in the intestine also exists. In an in vitro experiment using rat fecal suspensions, CR decreased the bioavailability of anthraquinones by inhibiting the transformation of conjugated anthraquinones to free anthraquinones mediated by gut microbiota, whereas SR confronted CR by inhibiting the glucuronidation of anthraquinones in the intestine (Yan et al., 2015).
The exploration of the interplay of gut microbiota-host-HM also offers new insights into precision HM therapy and drug discovery. Metformin is universally acknowledged as a first-line anti-diabetic agent for the management of T2DM and improves the intestinal bacterial dysbiosis. It has been confirmed that one of the therapeutic effects of metformin in T2DM is through SCFA production as well as a increase of Eschericha abundance (Forslund et al., 2015). Metformin exert anti-hyperglycemic effect through an increase in the bile acid gycoursodeoxycholic acid in the intestine by decreasing the abundance of species of B. fragilis and its bile salt hydrolase activity (Sun et al., 2018). However, it is less well-known that the origins of metformin can be traced back to a traditional European HM, namely Galega officinalis (also known as goat's rue) (Bailey, 2017). This classic and successful example demonstrates that herbs are very valuable for medicine and contribute significantly to drug discovery. With the recent increasing interest and accumulating research evidence on HMs and their pharmacologic effects and related mechanisms, new drug discovery and development based on HMs are expected to be an important future trend. As a definite target, gut microbiota may be a direction for precisely targeted HM therapy and new drug discovery using extracts from natural HMs.
Furthermore, many herbs in the Chinese Pharmacopeia enriched in fibers and botanicals are also present in our daily diet and are named herbal food supplements (Di Giorgi Gerevini et al., 2005). For example, Momordica charantia and Chinese yam are common foods in the daily diet and are also used as HMs to treat diabetes and modulate gut microbiota (Li et al., 2017; Wang et al., 2017). Diet is intimately associated with T2DM, and gut microbiota is considered an intersection of diet and disease (Sonnenburg and Backhed, 2016). Furthermore, numerous studies have confirmed that the daily structure is important for the management of diabetes (Nie et al., 2019). Therefore, the exploration of herbal foods regulating the flora may aid the development of hypoglycemic dietary supplements and medications to reach the objective of “Let food be the medicine and medicine be the food.”
Author Contributions
XT and ML provided the idea. YZ, XG, JT, and LZ wrote the draft of the manuscript. LZ, HG, and YW searched the data and helped with the figures. XY and BP analyzed the data. All authors contributed to the article and approved the submitted version.
Funding
This work was supported by grants from The State Key Program of National Natural Science of China (No. 81430097), Sino-Austrian Cooperation Project (GH2017-03-06), The National Natural Science Foundation of China (No. 81904187), and Capital Health Development Research Project (CD2020-4-4155).
Conflict of Interest
The authors declare that the research was conducted in the absence of any commercial or financial relationships that could be construed as a potential conflict of interest.
References
Alarcon-Aguilara, F. J., Roman-Ramos, R., Perez-Gutierrez, S., Aguilar-Contreras, A., Contreras-Weber, C. C., and Flores-Saenz, J. L. (1998). Study of the anti-hyperglycemic effect of plants used as antidiabetics. J. Ethnopharmacol. 61, 101–110. doi: 10.1016/S0378-8741(98)00020-8
Albenberg, L., Esipova, T. V., Judge, C. P., Bittinger, K., Chen, J., Laughlin, A., et al. (2014). Correlation between intraluminal oxygen gradient and radial partitioning of intestinal microbiota. Gastroenterology 147, 1055–63. doi: 10.1053/j.gastro.2014.07.020
Al-Eidi, S., Tayel, S., Al-Slail, F., Qureshi, N. A., Sohaibani, I., Khalil, M., et al. (2016). Knowledge, attitude and practice of patients with type 2 diabetes mellitus towards complementary and alternative medicine. J. Integr. Med. 14, 187–96. doi: 10.1016/s2095-4964(16)60244-3
American Diabetes Association (2019). Standards of medical care in diabetes-2019 abridged for primary care providers. Clin. Diabetes 37, 11–34. doi: 10.2337/cd18-0105
An, X., Bao, Q., Di, S., Zhao, Y., Zhao, S., Zhang, H., et al. (2019). The interaction between the gut microbiota and herbal medicines. Biomed. Pharmacother. 118:109252. doi: 10.1016/j.biopha.2019.109252
Arvindekar, A. U., Pereira, G. R., and Laddha, K. S. (2015). Assessment of conventional and novel extraction techniques on extraction efficiency of five anthraquinones from rheum emodi. J. Food Sci. Technol. 52, 6574–6582. doi: 10.1007/s13197-015-1814-3
Asano, Y., Hiramoto, T., Nishino, R., Aiba, Y., Kimura, T., Yoshihara, K., et al. (2012). Critical role of gut microbiota in the production of biologically active, free catecholamines in the gut lumen of mice. Am. J. Physiol. Gastrointest. Liver Physiol. 303, G1288–G1295. doi: 10.1152/ajpgi.00341.2012
Babujanarthanam, R., Kavitha, P., Mahadeva, R. U., and Pandian, M. R. (2011). Quercitrin a bioflavonoid improves the antioxidant status in streptozotocin: induced diabetic rat tissues. Mol. Cell. Biochem. 358, 121–129. doi: 10.1007/s11010-011-0927-x
Babujanarthanam, R., Kavitha, P., and Pandian, M. R. (2010). Quercitrin, a bioflavonoid improves glucose homeostasis in streptozotocin-induced diabetic tissues by altering glycolytic and gluconeogenic enzymes. Fundam. Clin. Pharmacol. 24, 357–364. doi: 10.1111/j.1472-8206.2009.00771.x
Bailey, C. J. (2017). Metformin: historical overview. Diabetologia 60, 1566–1576. doi: 10.1007/s00125-017-4318-z
Banjari, I., Misir, A., Pavlic, M., Herath, P. N., and Waisundara, V. Y. (2019). Traditional herbal medicines for diabetes used in Europe and Asia: remedies from croatia and Sri Lanka. Altern. Ther. Health Med. 25, 40–52.
Belizario, J. E., Faintuch, J., and Garay-Malpartida, M. (2018). Gut microbiome dysbiosis and immunometabolism: new frontiers for treatment of metabolic diseases. Mediators Inflamm. 2018, 1–12. doi: 10.1155/2018/2037838
Burapan, S., Kim, M., and Han, J. (2017). Curcuminoid demethylation as an alternative metabolism by human intestinal microbiota. J. Agric. Food Chem. 65, 3305–3310. doi: 10.1021/acs.jafc.7b00943
Cani, P. D., Amar, J., Iglesias, M. A., Poggi, M., Knauf, C., Bastelica, D., et al. (2007a). Metabolic endotoxemia initiates obesity and insulin resistance. Diabetes 56, 1761–1772. doi: 10.2337/db06-1491
Cani, P. D., Bibiloni, R., Knauf, C., Neyrinck, A. M., Neyrinck, A. M., Delzenne, N. M., et al. (2008). Changes in gut microbiota control metabolic endotoxemia-induced inflammation in high-fat diet-induced obesity and diabetes in mice. Diabetes 57, 1470–1481. doi: 10.2337/db07-1403
Cani, P. D., Neyrinck, A. M., Fava, F., Knauf, C., Burcelin, R. G., Tuohy, K. M., et al. (2007b). Selective increases of bifidobacteria in gut microflora improve high-fat-diet-induced diabetes in mice through a mechanism associated with endotoxaemia. Diabetologia 50, 2374–2383. doi: 10.1007/s00125-007-0791-0
Cao, Y. J., Pu, Z. J., Tang, Y. P., Shen, J., Chen, Y. Y., Kang, A., et al. (2017). Advances in bio-active constituents, pharmacology and clinical applications of rhubarb. Chin. Med. 12:36. doi: 10.1186/s13020-017-0158-5
Chatterjee, S., Khunti, K., and Davies, M. J. (2017). Type 2 diabetes. Lancet 389, 2239–2251. doi: 10.1016/S0140-6736(17)30058-2
Chen, J., Zheng, L., Hu, Z., Wang, F., Huang, S., Li, Z., et al. (2019). Metabolomics reveals effect of zishen jiangtang pill, a chinese herbal product on high-fat diet-induced type 2 diabetes mellitus in mice. Front. Pharmacol. 10:256. doi: 10.3389/fphar.2019.00256
Chen, M. Y., Liao, Z. Q., Lu, B. Y., Wang, M. X., Lin, L., Zhang, S. B., et al. (2018). Huang-lian-jie-du-decoction ameliorates hyperglycemia and insulin resistant in association with gut microbiota modulation. Front. Microbiol. 9:2380. doi: 10.3389/fmicb.2018.02380
Cho, N. H., Shaw, J. E., Karuranga, S., Huang, Y., Da, R. F. J., Ohlrogge, A. W., et al. (2018). IDF diabetes atlas: global estimates of diabetes prevalence for 2017 and projections for 2045. Diabetes Res. Clin. Pract. 138, 271–281. doi: 10.1016/j.diabres.2018.02.023
Cohen, L. J., Esterhazy, D., Kim, S. H., Lemetre, C., Aguilar, R. R., Gordon, E. A., et al. (2017). Commensal bacteria make GPCR ligands that mimic human signalling molecules. Nature 549, 48–53. doi: 10.1038/nature23874
Creely, S. J., McTernan, P. G., Kusminski, C. M., Fisher, F. M., Da Silva, N. F., Khanolkar, M., et al. (2007). Lipopolysaccharide activates an innate immune system response in human adipose tissue in obesity and type 2 diabetes. Am. J. Physiol. Endocrinol. Metab. 292, E740–E747. doi: 10.1152/ajpendo.00302.2006
Cui, H. X., Zhang, L. S., Luo, Y., Yuan, K., Huang, Z. Y., and Guo, Y. (2019). A purified anthraquinone-glycoside preparation from rhubarb ameliorates type 2 diabetes mellitus by modulating the gut microbiota and reducing inflammation. Front. Microbiol. 10:1423. doi: 10.3389/fmicb.2019.01423
De Vadder, F., Kovatcheva-Datchary, P., Zitoun, C., Duchampt, A., Bäckhed, F., and Mithieux, G. (2016). Microbiota-produced succinate improves glucose homeostasis via intestinal gluconeogenesis. Cell. Metab. 24, 151–157. doi: 10.1016/j.cmet.2016.06.013
Di Giorgi Gerevini, V., Copparoni, R., Dalfrà, S, Leonardi, M., and Guidarelli, L. (2005). Herbal food supplements. Ann. Super Sanita 41, 55–59.
Donath, M. Y., and Shoelson, S. E. (2011). Type 2 diabetes as an inflammatory disease. Nat. Rev. Immunol. 11, 98–107. doi: 10.1038/nri2925
Ekor, M. (2014). The growing use of herbal medicines: issues relating to adverse reactions and challenges in monitoring safety. Front. Pharmacol. 4:177. doi: 10.3389/fphar.2013.00177
Feng, R., Shou, J. W., Zhao, Z. X., He, C. Y., Ma, C., Huang, M., et al. (2015). Transforming berberine into its intestine-absorbable form by the gut microbiota. Sci. Rep. 5:12155. doi: 10.1038/srep12155
Forslund, K., Hildebrand, F., Nielsen, T., Falony, G., Le Chatelier, E., Sunagawa, S., et al. (2015). Disentangling type 2 diabetes and metformin treatment signatures in the human gut microbiota. Nature 528, 262–266. doi: 10.1038/nature15766
Gao, Z., Yin, J., Zhang, J., Ward, R. E., Martin, R. J., Lefevre, M., et al. (2009). Butyrate improves insulin sensitivity and increases energy expenditure in mice. Diabetes 58, 1509–1517. doi: 10.2337/db08-1637
Gastaldelli, A., Natali, A., Vettor, R., and Corradini, S. G. (2010). Insulin resistance, adipose depots and gut: interactions and pathological implications. Digest. Liver Dis. 42, 310–319. doi: 10.1016/j.dld.2010.01.013
Gerard, C., and Vidal, H. (2019). Impact of gut microbiota on host glycemic control. Front. Endocrinol. 10:29. doi: 10.3389/fendo.2019.00029
Gerrits, E. G., Landman, G. W., and Bilo, H. J. (2015). Mortality trends in diabetes mellitus. JAMA Intern. Med. 175:469. doi: 10.1001/jamainternmed.2014.7985
Gui, Q. F., Xu, Z. R., Xu, K. Y., and Yang, Y. M. (2016). The efficacy of ginseng-related therapies in type 2 diabetes mellitus: an updated systematic review and meta-analysis. Medicine (Baltimore) 95:e2584. doi: 10.1097/MD.0000000000002584
Han, L. H., Li, T. G., Du, M., Chang, R., Zhan, B. Y., and Mao, X. Y. (2019). Beneficial effects of potentilla discolor bunge water extract on inflammatory cytokines release and gut microbiota in high-fat diet and streptozotocin-induced type 2 diabetic mice. Nutrients 11:670. doi: 10.3390/nu11030670
Hasan, A. U., Rahman, A., and Kobori, H. (2019). Interactions between host PPARs and gut microbiota in health and disease. Int. J. Mol. Sci. 20:387. doi: 10.3390/ijms20020387
Hassaninasab, A., Hashimoto, Y., Tomita-Yokotani, K., and Kobayashi, M. (2011). Discovery of the curcumin metabolic pathway involving a unique enzyme in an intestinal microorganism. Proc. Natl. Acad. Sci. U.S.A. 108, 6615–6620. doi: 10.1073/pnas.1016217108
Inzucchi, S. E., Bergenstal, R. M., Buse, J. B., Diamant, M., Ferrannini, E., Nauck, M., et al. (2012). Management of hyperglycemia in type 2 diabetes: a patient-centered approach: position statement of the American diabetes association (ADA) and the European association for the study of diabetes (EASD). Diabetes Care 35, 1364–1379. doi: 10.2337/dc12-0413
Jialal, I., and Rajamani, U. (2014). Endotoxemia of metabolic syndrome: a pivotal mediator of meta-inflammation. Metab. Syndr. Relat. Disord. 12, 454–6. doi: 10.1089/met.2014.1504
Ju, M., Liu, Y., Li, M., Cheng, M., Zhang, Y., Deng, G., et al. (2019). Baicalin improves intestinal microecology and abnormal metabolism induced by high-fat diet. Eur. J. Pharmacol. 857:172457. doi: 10.1016/j.ejphar.2019.172457
Jung, I. H., Lee, J. H., Hyun, Y. J., and Kim, D. H. (2012). Metabolism of ginsenoside Rb1 by human intestinal microflora and cloning of its metabolizing beta-D-glucosidase from Bifidobacterium longum H-1. Biol. Pharm. Bull. 35, 573–581. doi: 10.1248/bpb.35.573
Kahn, S. E., Hull, R. L., and Utzschneider, K. M. (2006). Mechanisms linking obesity to insulin resistance and type 2 diabetes. Nature 444, 840–846. doi: 10.1038/nature05482
Kautzky-Willer, A., Harreiter, J., and Pacini, G. (2016). Sex and gender differences in risk, pathophysiology and complications of type 2 diabetes mellitus. Endocr. Rev. 37, 278–316. doi: 10.1210/er.2015-1137
Kesarwani, K., Gupta, R., and Mukerjee, A. (2013). Bioavailability enhancers of herbal origin: an overview. Asian Pac. J. Trop. Biomed. 3, 253–266. doi: 10.1016/S2221-1691(13)60060-X
Khan, S., and Jena, G. (2015). The role of butyrate, a histone deacetylase inhibitor in diabetes mellitus: experimental evidence for therapeutic intervention. Epigenomics 7, 669–680. doi: 10.2217/epi.15.20
Kim, D. H., Kim, S. Y., Park, S. Y., and Han, M. J. (1999). Metabolism of quercitrin by human intestinal bacteria and its relation to some biological activities. Biol. Pharm. Bull. 22, 749–751. doi: 10.1248/bpb.22.749
Kim, H. K. (2013). Pharmacokinetics of ginsenoside Rb1 and its metabolite compound K after oral administration of Korean red ginseng extract. J. Ginseng. Res. 37, 451–456. doi: 10.5142/jgr.2013.37.451
Koppel, N., Maini, R. V., and Balskus, E. P. (2017). Chemical transformation of xenobiotics by the human gut microbiota. Science 356:eaag2770. doi: 10.1126/science.aag2770
Krautkramer, K. A., Kreznar, J. H., Romano, K. A., Vivas, E. I., Barrett-Wilt, G. A., Rabaglia, M. E., et al. (2016). Diet-microbiota interactions mediate global epigenetic programming in multiple host tissues. Mol. Cell. 64, 982–992. doi: 10.1016/j.molcel.2016.10.025
Kuwahara, A. (2014). Contributions of colonic short-chain fatty acid receptors in energy homeostasis. Front. Endocrinol. (Lausanne) 5:144. doi: 10.3389/fendo.2014.00144
Larsen, N., Vogensen, F. K., van den Berg, F. W., Nielsen, D. S., Andreasen, A. S., Pedersen, B. K., et al. (2010). Gut microbiota in human adults with type 2 diabetes differs from non-diabetic adults. PLoS ONE 5:e9085. doi: 10.1371/journal.pone.0009085
Lazar, V., Ditu, L. M., Pircalabioru, G. G., Picu, A., Petcu, L., Cucu, N., et al. (2019). Gut microbiota, host organism, and diet trialogue in diabetes and obesity. Front. Nutr. 6:21. doi: 10.3389/fnut.2019.00021
Lee, M. W., Lee, M., and Oh, K. J. (2019). Adipose tissue-derived signatures for obesity and type 2 diabetes: adipokines, batokines and microRNAs. J. Clin. Med. 8:854. doi: 10.3390/jcm8060854
Lew, K. N., Starkweather, A., Cong, X. M., and Judge, M. (2019). A mechanistic model of gut-brain axis perturbation and high-fat diet pathways to gut microbiome homeostatic disruption, systemic inflammation, and type 2 diabetes. Biol. Res. Nurs. 21, 384–399. doi: 10.1177/1099800419849109
Ley, R. E., Turnbaugh, P. J., Klein, S., and Gordon, J. I. (2006). Microbial ecology–human gut microbes associated with obesity. Nature 444, 1022–1023. doi: 10.1038/4441022a
Li, F. S., and Weng, J. K. (2017). Demystifying traditional herbal medicine with modern approach. Nat. Plants 3:17109. doi: 10.1038/nplants.2017.109
Li, Q., Li, W., Gao, Q., and Zou, Y. (2017). Hypoglycemic effect of chinese yam (dioscorea opposita rhizoma) polysaccharide in different structure and molecular weight. J. Food Sci. 82, 2487–2494. doi: 10.1111/1750-3841.13919
Li, W. L., Zheng, H. C., Bukuru, J., and De Kimpe, N. (2004). Natural medicines used in the traditional Chinese medical system for therapy of diabetes mellitus. J. Ethnopharmacol. 92, 1–21. doi: 10.1016/j.jep.2003.12.031
Li, X. W., Chen, H. P., He, Y. Y., Chen, W. L., Chen, J. W., Gao, L., et al. (2018). Effects of rich-polyphenols extract of dendrobium loddigesii on anti-diabetic, anti-inflammatory, anti-oxidant, and gut microbiota modulation in db/db mice. Molecules 23:3245. doi: 10.3390/molecules23123245
Liang, Y., Xu, X., Yin, M., Zhang, Y., Huang, L., Chen, R., et al. (2019). Effects of berberine on blood glucose in patients with type 2 diabetes mellitus: a systematic literature review and a meta-analysis. Endocr. J. 66, 51–63. doi: 10.1507/endocrj.EJ18-0109
Liu, C. S., Zheng, Y. R., Zhang, Y. F., and Long, X. Y. (2016). Research progress on berberine with a special focus on its oral bioavailability. Fitoterapia 109, 274–282. doi: 10.1016/j.fitote.2016.02.001
Lopresti, A. L. (2018). The problem of curcumin and its bioavailability: could its gastrointestinal influence contribute to its overall health-enhancing effects? Adv. Nutr. 9, 41–50. doi: 10.1093/advances/nmx011
Lozupone, C. A., Stombaugh, J. I., Gordon, J. I., Jansson, J. K., and Knight, R. (2012). Diversity, stability and resilience of the human gut microbiota. Nature 489, 220–230. doi: 10.1038/nature11550
Luca, M., Di Mauro, M., Di Mauro, M., and Luca, A. (2019). Gut microbiota in alzheimer's disease, depression, and type 2 diabetes mellitus: the role of oxidative stress. Oxid. Med. Cell. Longev. 2019:4730539. doi: 10.1155/2019/4730539
Lukovac, S., Belzer, C., Pellis, L., Keijser, B. J., de Vos, W. M., Montijn, R. C., et al. (2014). Differential modulation by akkermansia muciniphila and faecalibacterium prausnitzii of host peripheral lipid metabolism and histone acetylation in mouse gut organoids. Mbio 5:e01438-14. doi: 10.1128/mBio.01438-14
Maleckas, A., Venclauskas, L., Wallenius, V., Lonroth, H., and Fandriks, L. (2015). Surgery in the treatment of type 2 diabetes mellitus. Scan. J. Surg. 104, 40–47. doi: 10.1177/1457496914561140
Mandaliya, D. K., and Seshadri, S. (2019a). Short chain fatty acids, pancreatic dysfunction and type 2 diabetes. Pancreatology 19, 280–284. doi: 10.1016/j.pan.2019.01.021
Mandaliya, D. K., and Seshadri, S. (2019b). Short chain fatty acids, pancreatic dysfunction and type 2 diabetes. Pancreatology 19, 617–622. doi: 10.1016/j.pan.2019.04.013
Miranda-Ribera, A., Ennamorati, M., Serena, G., Cetinbas, M., Lan, J., Sadreyev, R. I., et al. (2019). Exploiting the zonulin mouse model to establish the role of primary impaired gut barrier function on microbiota composition and immune profiles. Front. Immunol. 10:2233. doi: 10.3389/fimmu.2019.02233
Mirfeizi, M., Mehdizadeh, T. Z., Mirfeizi, S. Z., Asghari, J. M., Rezvani, H. R., and Afzali, M. (2016). Controlling type 2 diabetes mellitus with herbal medicines: a triple-blind randomized clinical trial of efficacy and safety. J. Diabetes 8, 647–656. doi: 10.1111/1753-0407.12342
Nabavi, S. F., Thiagarajan, R., Rastrelli, L., Daglia, M., Sobarzo-Sanchez, E., Alinezhad, H., et al. (2015). Curcumin: a natural product for diabetes and its complications. Curr. Top. Med. Chem. 15, 2445–2455. doi: 10.2174/1568026615666150619142519
Nahas, R., and Moher, M. (2009). Complementary and alternative medicine for the treatment of type 2 diabetes. Can. Fam. Physician. 55, 591–596.
Nie, Q. X., Chen, H. H., Hu, J. L., Fan, S. T., and Nie, S. P. (2019). Dietary compounds and traditional Chinese medicine ameliorate type 2 diabetes by modulating gut microbiota. Crit. Rev. Food Sci. Nutr. 59, 848–863. doi: 10.1080/10408398.2018.1536646
Ohira, H., Tsutsui, W., and Fujioka, Y. (2017). Are short chain fatty acids in gut microbiota defensive players for inflammation and atherosclerosis? J. Atheroscler. Thromb. 24, 660–672. doi: 10.5551/jat.RV17006
Okumura, R., and Takeda, K. (2017). Roles of intestinal epithelial cells in the maintenance of gut homeostasis. Exp. Mol. Med. 49:e338. doi: 10.1038/emm.2017.20
Olesen, S. W., and Alm, E. J. (2016). Dysbiosis is not an answer. Nat. Microbiol. 1:16228. doi: 10.1038/nmicrobiol.2016.228
Pang, B., Lian, F. M., Zhao, X. Y., Zhao, X. M., Jin Lin, Y. Q., Zheng, Y. J., et al. (2017). Prevention of type 2 diabetes with the traditional Chinese patent medicine: a systematic review and meta-analysis. Diabetes Res. Clin. Pract. 131, 242–259. doi: 10.1016/j.diabres.2017.07.020
Pang, B., Zhao, L. H., Zhou, Q., Zhao, T. Y., Wang, H., Gu, C. J., et al. (2015). Application of berberine on treating type 2 diabetes mellitus. Int. J. Endocrinol. 2015:905749. doi: 10.1155/2015/905749
Pang, G. M., Li, F. X., Yan, Y., Zhang, Y., Kong, L. L., Zhu, P., et al. (2019). Herbal medicine in the treatment of patients with type 2 diabetes mellitus. Chin. Med. J. (Engl). 132, 78–85. doi: 10.1097/CM9.0000000000000006
Parsamanesh, N., Moossavi, M., Bahrami, A., Butler, A. E., and Sahebkar, A. (2018). Therapeutic potential of curcumin in diabetic complications. Pharmacol. Res. 136, 181–193. doi: 10.1016/j.phrs.2018.09.012
Paudel, M. R., Chand, M. B., Pant, B., and Pant, B. (2018). Antioxidant and cytotoxic activities of dendrobium moniliforme extracts and the detection of related compounds by GC-MS. BMC Complement Altern. Med. 18:134. doi: 10.1186/s12906-018-2197-6
Perry, R. J., Peng, L., Barry, N. A., Cline, G. W., Zhang, D., Cardone, R. L., et al. (2016). Acetate mediates a microbiome-brain-β-cell axis to promote metabolic syndrome. Nature 534, 213–217. doi: 10.1038/nature18309
Pickup, J. C. (2004). Inflammation and activated innate immunity in the pathogenesis of type 2 diabetes. Diabetes Care 27, 813–823. doi: 10.2337/diacare.27.3.813
Qaseem, A., Barry, M. J., Humphrey, L. L., and Forciea, M. A. (2017). Oral pharmacologic treatment of type 2 diabetes mellitus: a clinical practice guideline update from the american college of physicians. Ann. Intern. Med. 166, 279–290. doi: 10.7326/M16-1860
Qin, J., Li, R., Raes, J., Arumugam, M., Burgdorf, K. S., Manichanh, C., et al. (2010). A human gut microbial gene catalogue established by metagenomic sequencing. Nature 464, 59–65. doi: 10.1038/nature08821
Qin, J., Li, Y., Cai, Z., Li, S., Zhu, J., Zhang, F., et al. (2012). A metagenome-wide association study of gut microbiota in type 2 diabetes. Nature 490, 55–60. doi: 10.1038/nature11450
Quan, L. H., Min, J. W., Yang, D. U., Kim, Y. J., and Yang, D. C. (2012). Enzymatic biotransformation of ginsenoside Rb1 to 20(S)-Rg3 by recombinant beta-glucosidase from microbacterium esteraromaticum. Appl. Microbiol. Biotechnol. 94, 377–384. doi: 10.1007/s00253-011-3861-7
Ran, Q., Wang, J., Wang, L., Zeng, H. R., Yang, X. B., and Huang, Q. W. (2019). Rhizoma coptidis as a potential treatment agent for type 2 diabetes mellitus and the underlying mechanisms: a review. Front. Pharmacol. 10:805. doi: 10.3389/fphar.2019.00805
Raphael, I., Nalawade, S., Eagar, T. N., and Forsthuber, T. G. (2015). T cell subsets and their signature cytokines in autoimmune and inflammatory diseases. Cytokine 74, 5–17. doi: 10.1016/j.cyto.2014.09.011
Ryuk, J. A., Lixia, M., Cao, S., Ko, B. S., and Park, S. (2017). Efficacy and safety of gegen qinlian decoction for normalizing hyperglycemia in diabetic patients: a systematic review and meta-analysis of randomized clinical trials. Complement Ther. Med. 33, 6–13. doi: 10.1016/j.ctim.2017.05.004
Schellenberg, E. S., Dryden, D. M., Vandermeer, B., Ha, C., and Korownyk, C. (2013). Lifestyle interventions for patients with and at risk for type 2 diabetes: a systematic review and meta-analysis. Ann. Intern. Med. 159, 543–551. doi: 10.7326/0003-4819-159-8-201310150-00007
Schwiertz, A., Taras, D., Schafer, K., Beijer, S., Bos, N. A., Donus, C., et al. (2010). Microbiota and SCFA in lean and overweight healthy subjects. Obesity (Silver Spring) 18, 190–195. doi: 10.1038/oby.2009.167
Sekirov, I., Russell, S. L., Antunes, L. C. M., and Finlay, B. B. (2010). Gut microbiota in health and disease. Physiol. Rev. 90, 859–904. doi: 10.1152/physrev.00045.2009
Sharkey, K. A., Beck, P. L., and McKay, D. M. (2018). Neuroimmunophysiology of the gut: advances and emerging concepts focusing on the epithelium. Nat. Rev. Gastroenterol. Hepatol. 15, 765–784. doi: 10.1038/s41575-018-0051-4
Shaw, J. E., Sicree, R. A., and Zimmet, P. Z. (2010). Global estimates of the prevalence of diabetes for 2010 and 2030. Diabetes Res. Clin. Pract. 87, 4–14. doi: 10.1016/j.diabres.2009.10.007
Sonnenburg, J. L., and Backhed, F. (2016). Diet-microbiota interactions as moderators of human metabolism. Nature 535, 56–64. doi: 10.1038/nature18846
Sorini, C., Cosorich, I., Lo Conte, M., De Giorgi, L., Facciotti, F., Lucianò, R., et al. (2019). Loss of gut barrier integrity triggers activation of islet-reactive T cells and autoimmune diabetes. Proc. Natl. Acad. Sci. U. S. A. 116, 15140–15149. doi: 10.1073/pnas.1814558116
Sun, L., Xie, C., Wang, G., Wu, Y., Wu, Q., Wang, X., et al. (2018). Gut microbiota and intestinal FXR mediate the clinical benefits of metformin. Nat. Med. 24, 1919–1929. doi: 10.1038/s41591-018-0222-4
Tan, S., Calani, L., Bresciani, L., Dall'Asta, M., Faccini, A., Augustin, M. A., et al. (2015). The degradation of curcuminoids in a human faecal fermentation model. Int. J. Food Sci. Nutr. 66, 790–796. doi: 10.3109/09637486.2015.1095865
Tian, Z., Ren, N., Wang, J., Zhang, D., and Zhou, Y. (2018). Ginsenoside ameliorates cognitive dysfunction in type 2 diabetic goto-kakizaki rats. Med. Sci. Monit. 24, 3922–3928. doi: 10.12659/MSM.907417
Tilg, H., Zmora, N., Adolph, T. E., and Elinav, E. (2020). The intestinal microbiota fuelling metabolic inflammation. Nat. Rev. Immunol. 20, 40–54. doi: 10.1038/s41577-019-0198-4
Tong, X. L., Xu, J., Lian, F. M., Yu, X. T., Zhao, Y. F., Xu, L. P., et al. (2018). Structural alteration of gut microbiota during the amelioration of human type 2 diabetes with hyperlipidemia by metformin and a traditional chinese herbal formula: a multicenter, randomized, open label clinical trial. Mbio 9:e02392–17. doi: 10.1128/mBio.02392-17
Tran, L., Zielinski, A., Roach, A. H., Jende, J. A., Householder, A. M., Cole, E. E., et al. (2015). Pharmacologic treatment of type 2 diabetes: injectable medications. Ann. Pharmacother. 49, 700–714. doi: 10.1177/1060028015573010
Tremaroli, V., and Backhed, F. (2012). Functional interactions between the gut microbiota and host metabolism. Nature 489, 242–249. doi: 10.1038/nature11552
Tsai, S., Clemente-Casares, X., Zhou, A. C., Lei, H., Ahn, J. J., Chan, Y. T., et al. (2018). Insulin receptor-mediated stimulation boosts T cell immunity during inflammation and infection. Cell. Metab. 28, 922–934.e4. doi: 10.1016/j.cmet.2018.08.003
Turnbaugh, P. J., Hamady, M., Yatsunenko, T., Cantarel, B. L., Duncan, A., Ley, R. E., et al. (2009). A core gut microbiome in obese and lean twins. Nature 457, 480–484. doi: 10.1038/nature07540
Verhoog, S., Taneri, P. E., Diaz, Z. M. R., Marques-Vidal, P., Troup, J. P., Bally, L., et al. (2019). Dietary factors and modulation of bacteria strains of akkermansia muciniphila and faecalibacterium prausnitzii: a systematic review. Nutrients 11:1565. doi: 10.3390/nu11071565
Wang, J., Wang, L., Lou, G. H., Zeng, H. R., Hu, J., Huang, Q. W., et al. (2019). Coptidis rhizoma: a comprehensive review of its traditional uses, botany, phytochemistry, pharmacology and toxicology. Pharm. Biol. 57, 193–225. doi: 10.1080/13880209.2019.1577466
Wang, J. H., Shin, N. R., Lim, S. K., Im, U., Song, E. J., Nam, Y. D., et al. (2019). Diet control more intensively disturbs gut microbiota than genetic background in wild type and ob/ob mice. Front. Microbiol. 10:1292. doi: 10.3389/fmicb.2019.01292
Wang, S., Li, Z., Yang, G., Ho, C. T., and Li, S. (2017). Momordica charantia: a popular health-promoting vegetable with multifunctionality. Food Funct. 8, 1749–1762. doi: 10.1039/C6FO01812B
Wang, S. Y., Li, J. Y., Xu, J. H., Xia, Z. S., Cheng, D., Zhong, W., et al. (2019). Butyrate suppresses abnormal proliferation in colonic epithelial cells under diabetic state by targeting HMGB1. J. Pharmacol. Sci. 139, 266–274. doi: 10.1016/j.jphs.2018.07.012
Wei, X. Y., Tao, J. H., Xiao, S. W., Jiang, S., Shang, E. X., Zhu, Z. H., et al. (2018). Xiexin tang improves the symptom of type 2 diabetic rats by modulation of the gut microbiota. Sci. Rep. 8:3685. doi: 10.1038/s41598-018-22094-2
Wen, L., and Duffy, A. (2017). Factors influencing the gut microbiota, inflammation, and type 2 diabetes. J. Nutr. 147, 1468S−1475S. doi: 10.3945/jn.116.240754
Winter, S. E., Lopez, C. A., and Baumler, A. J. (2013). The dynamics of gut-associated microbial communities during inflammation. Embo. Rep. 14, 319–327. doi: 10.1038/embor.2013.27
Wisniewski, P. J., Dowden, R. A., and Campbell, S. C. (2019). Role of dietary lipids in modulating inflammation through the gut microbiota. Nutrients 11:117. doi: 10.3390/nu11010117
Wu, Y., Ding, Y., Tanaka, Y., and Zhang, W. (2014). Risk factors contributing to type 2 diabetes and recent advances in the treatment and prevention. Int. J. Med. Sci. 11, 1185–1200. doi: 10.7150/ijms.10001
Wu, Y. P., Tang, L., Wang, B. K., Sun, Q. M., Zhao, P. W., and Li, W. F. (2019). The role of autophagy in maintaining intestinal mucosal barrier. J. Cell. Physiol. 234, 19406–19419. doi: 10.1002/jcp.28722
Xia, C., Rao, X. Q., and Zhong, J. X. (2017). Role of T lymphocytes in type 2 diabetes and diabetes-associated inflammation. J. Diabetes Res. 2017:6494795. doi: 10.1155/2017/6494795
Xu, J., Lian, F. M., Zhao, L. H., Zhao, Y. F., Chen, X. Y., Zhang, X., et al. (2015). Structural modulation of gut microbiota during alleviation of type 2 diabetes with a Chinese herbal formula. Isme J. 9, 552–562. doi: 10.1038/ismej.2014.177
Yan, D., Ma, B., Shi, R., Wang, T., and Ma, Y. (2015). Involvement of herb-herb interactions in the influences of radix scutellaria and coptis chinensis on the bioavailability of the anthraquinones form rhei rhizoma in rats. Eur. J. Drug Metab. Pharmacokinet. 40, 103–10. doi: 10.1007/s13318-014-0188-7
Yu, H., Wang, Y., Liu, C., Yang, J., Xu, L., Li, G., et al. (2018). Conversion of ginsenoside Rb1 into six types of highly bioactive ginsenoside Rg3 and its derivatives by FeCl3 catalysis. Chem. Pharm. Bull. (Tokyo) 66, 901–906. doi: 10.1248/cpb.c18-00426
Yu, S., Zhou, X., Li, F., Xu, C., Zheng, F., Li, J., et al. (2017). Microbial transformation of ginsenoside Rb1, Re and Rg1 and its contribution to the improved anti-inflammatory activity of ginseng. Sci. Rep. 7:138. doi: 10.1038/s41598-017-00262-0
Zhang, B., Yue, R., Chen, Y., Yang, M., Huang, X., Shui, J., et al. (2019). Gut microbiota, a potential new target for chinese herbal medicines in treating diabetes mellitus. Evid. Based Complement Alternat. Med. 2019:2634898. doi: 10.1155/2019/2634898
Zhang, C., Liu, J., He, X., Sheng, Y., Yang, C., Li, H., et al. (2019). Caulis spatholobi ameliorates obesity through activating brown adipose tissue and modulating the composition of gut microbiota. Int. J. Mol. Sci. 20:5150. doi: 10.3390/ijms20205150
Zhang, C. H., Sheng, J. Q., Sarsaiya, S., Shu, F. X., Liu, T. T., Tu, X. Y., et al. (2019). The anti-diabetic activities, gut microbiota composition, the anti-inflammatory effects of scutellaria-coptis herb couple against insulin resistance-model of diabetes involving the toll-like receptor 4 signaling pathway. J. Ethnopharmacol. 237, 202–214. doi: 10.1016/j.jep.2019.02.040
Zhang, H., Wei, J., Xue, R., Wu, J. D., Zhao, W., Wang, Z. Z., et al. (2010). Berberine lowers blood glucose in type 2 diabetes mellitus patients through increasing insulin receptor expression. Metabolism 59, 285–292. doi: 10.1016/j.metabol.2009.07.029
Zhang, Q., Shaan, A., Espinosa, S. A., Gallego-Perez, D., and Weeks, J. (2019). The path toward integration of traditional and complementary medicine into health systems globally: the world health organization report on the implementation of the 2014–2023 strategy. J. Altern. Complement Med. 25, 869–871. doi: 10.1089/acm.2019.29077.jjw
Zhang, X., Zhao, Y. F., Xu, J., Xue, Z. S., Zhang, M. H., Pang, X. Y., et al. (2015). Modulation of gut microbiota by berberine and metformin during the treatment of high-fat diet-induced obesity in rats. Sci. Rep. 5:14405. doi: 10.1038/srep14405
Zhang, X. J., Deng, Y. X., Shi, Q. Z., He, M. Y., Chen, B., and Qiu, X. M. (2014). Hypolipidemic effect of the Chinese polyherbal huanglian jiedu decoction in type 2 diabetic rats and its possible mechanism. Phytomedicine 21, 615–623. doi: 10.1016/j.phymed.2013.11.004
Zhang, X. J., Liu, S., Xing, J. P., Liu, Z. Q., and Song, F. R. (2018). Effect of type 2 diabetes mellitus on flavonoid pharmacokinetics and tissue distribution after oral administration of radix scutellaria extract in rats. Chin. J. Nat. Med. 16, 418–427. doi: 10.1016/S1875-5364(18)30075-X
Zhang, Z., Xue, H. L., Liu, Y., and Wang, W. J. (2011). Yi-Qi-Zeng-Min-Tang, a Chinese medicine, ameliorates insulin resistance in type 2 diabetic rats. World J. Gastroenterol. 17, 987–995. doi: 10.3748/wjg.v17.i8.987
Zhao, C., Yang, C., Wai, S. T. C., Zhang, Y. M. P. P, Paoli, P, Wu, Y., et al. (2019). Regulation of glucose metabolism by bioactive phytochemicals for the management of type 2 diabetes mellitus. Crit. Rev. Food Sci. Nutr. 59, 830–847. doi: 10.1080/10408398.2018.1501658
Zheng, Y., Ley, S. H., and Hu, F. B. (2017). Global aetiology and epidemiology of type 2 diabetes mellitus and its complications. Nat. Rev. Endocrinol. 14, 88–98. doi: 10.1038/nrendo.2017.151
Zhou, P., Xie, W., He, S., Sun, Y., Meng, X., Sun, G., et al. (2019). Ginsenoside Rb1 as an anti-diabetic agent and its underlying mechanism analysis. Cells 8:204. doi: 10.3390/cells8030204
Zhou, W. Y., Sailani, M. R., Contrepois, K., Zhou, Y. J., Ahadi, S., Leopold, S. R., et al. (2019). Longitudinal multi-omics of host-microbe dynamics in prediabetes. Nature 569, 663–671. doi: 10.1038/s41586-019-1236-x
Keywords: herbal medicines, type 2 diabetes mellitus, gut microbiota, bacterial metabolites, therapeutic mechanisms
Citation: Zheng Y, Gou X, Zhang L, Gao H, Wei Y, Yu X, Pang B, Tian J, Tong X and Li M (2020) Interactions Between Gut Microbiota, Host, and Herbal Medicines: A Review of New Insights Into the Pathogenesis and Treatment of Type 2 Diabetes. Front. Cell. Infect. Microbiol. 10:360. doi: 10.3389/fcimb.2020.00360
Received: 11 February 2020; Accepted: 10 June 2020;
Published: 17 July 2020.
Edited by:
Yongqun Oliver He, University of Michigan, United StatesReviewed by:
Giusi Desirè Sciumè, University of Pisa, ItalyJun Wang, Institute of Microbiology (CAS), China
Copyright © 2020 Zheng, Gou, Zhang, Gao, Wei, Yu, Pang, Tian, Tong and Li. This is an open-access article distributed under the terms of the Creative Commons Attribution License (CC BY). The use, distribution or reproduction in other forums is permitted, provided the original author(s) and the copyright owner(s) are credited and that the original publication in this journal is cited, in accordance with accepted academic practice. No use, distribution or reproduction is permitted which does not comply with these terms.
*Correspondence: Jiaxing Tian, tina_yai@126.com; Xiaolin Tong, tongxiaolin@vip.163.com; Min Li, limin-72114@163.com
†These authors have contributed equally to this work and share first authorship