Emergence of Multidrug-Resistant Escherichia coli Producing CTX-M, MCR-1, and FosA in Retail Food From Egypt
- 1Bacterial Epidemiology and Antimicrobial Resistance Research Unit, U.S. National Poultry Research Center, U.S. Department of Agriculture, Agricultural Research Service (USDA-ARS), Athens, GA, United States
- 2Hygiene and Zoonoses Department, Faculty of Veterinary Medicine, Mansoura University, Mansoura, Egypt
- 3Department of Microbiology and Immunology, Faculty of Pharmacy, Kafrelsheikh University, Kafr El-Sheikh, Egypt
- 4Animal Health Research Institute, Agriculture Research Center, Cairo, Egypt
- 5Institute of Preventive Veterinary Sciences & Department of Veterinary Medicine, College of Animal Sciences, Zhejiang University, Hangzhou, China
- 6Gastrointestinal Bacteria Reference Unit, Public Health England, London, United Kingdom
In this study, multidrug-resistant (MDR) Escherichia coli isolates from retail food and humans assigned into similar Multilocus Sequence Types (MLST) were analyzed using whole genome sequencing (WGS). In silico analysis of assembled sequences revealed the existence of multiple resistance genes among the examined E. coli isolates. Of the six CTX-M-producing isolates from retail food, blaCTX-M-14 was the prevalent variant identified (83.3%, 5/6). Two plasmid-mediated fosfomycin resistance genes, fosA3, and fosA4, were detected from retail food isolates (one each from chicken and beef), where fosA4 was identified in the chicken isolate 82CH that also carried the colistin resistance gene mcr-1. The blaCTX-M-14 and fosA genes in retail food isolates were located adjacent to insertion sequences ISEcp1 and IS26, respectively. Sequence analysis of the reconstructed mcr-1 plasmid (p82CH) showed 96–97% identity to mcr-1-carrying IncI2 plasmids previously identified in human and food E. coli isolates from Egypt. Hierarchical clustering of core genome MLST (HierCC) revealed clustering of chicken isolate 82CH, co-harboring mcr-1 and fosA4 genes, with a chicken E. coli isolate from China at the HC200 level (≤200 core genome allelic differences). As E. coli co-harboring mcr-1 and fosA4 genes has only been recently reported, this study shows rapid spread of this genotype that shares similar genetic structures with regional and international E. coli lineages originating from both humans and food animals. Adopting WGS-based surveillance system is warranted to facilitate monitoring the international spread of MDR pathogens.
Introduction
The emergence of multidrug resistance (MDR) in Escherichia coli has become a global health concern (Klemm et al., 2018). The continuous administration of antimicrobials in animals, either for treatment or prophylaxis and growth promotion purposes when fed to animals at sub-therapeutic doses, has led to the development of antimicrobial resistances that are able to disseminate to humans through the food chain (Pokharel et al., 2020; Van et al., 2020). The blaCTX-M gene encodes an extended spectrum β-lactamase responsible for the hydrolysis of most β-lactams except cephamycins and carbapenems, and often co-exists with other genes conferring resistance to different antimicrobial classes such as aminoglycosides, tetracyclines, sulfonamides and fluroquinolones (Cantón et al., 2012; Lupo et al., 2018). Treatment of these bacterial infections is more complicated if resistance to colistin and fosfomycin is also present. These older antimicrobials have been reintroduced for the treatment of severe infections caused by MDR E. coli (Sherry and Howden, 2018; Karaiskos et al., 2019). Fosfomycin resistance in Gram-negative bacteria is frequently associated with the glutathione S-transferase-encoding gene fosA (Liu et al., 2020). Mobilized colistin resistance (mcr) gene acts by adding phosphoethanolamine to lipid A in lipopolysaccharides, modifying the bacterial cell wall and reducing susceptibility to colistin (Liu et al., 2016). The horizontal transfer of blaCTX-M as well as mcr and fosA resistance genes is primarily linked to plasmids. However, other mobile genetic elements (MGEs) such as transposons and insertion sequences (IS) have also contributed to the transmission of these genes (Li et al., 2017; Zhang et al., 2019). Thus, studying the genetic context of these resistance genes would provide better understanding of the mechanisms responsible for their transmission.
The clonal diversity of E. coli has been determined using molecular typing techniques such as pulsed-field gel electrophoresis (PFGE) and multilocus sequence typing (MLST), to investigate and monitor the potential reservoirs of these isolates, their antimicrobial resistance and virulence traits (Nemoy et al., 2005). MLST assigned E. coli isolates into distinct sequence types (STs) based on the sequences of seven housekeeping genes (Tartof et al., 2005), that could indicate a possible epidemiological relationship if isolates from different hosts belong to the same STs (Ramadan et al., 2020b). The global spread of E. coli lineages, particularly those carrying emerging resistance genes, requires the implementation of whole genome sequencing (WGS)-based phylogenetic analyses for the discrimination of closely related isolates at higher resolution, compared to the seven-gene based MLST (Uelze et al., 2020; Zhou et al., 2020).
Previous studies from Egypt have reported the existence of antimicrobial-resistant E. coli isolates from retail food that could possibly disseminate to humans, yet there are few reports, to the best of our knowledge, that have analyzed the draft genome sequences of these isolates. Recently, we reported the circulation of shared MLST among the MDR E. coli isolated from retail food and humans in Egypt (Ramadan et al., 2020b). This study aimed to further characterize these isolates using WGS to explore the genetic environment of resistance genes they harbor, the associated plasmids, and to compare these isolates with the available E. coli genomes from Egypt, as well as the international lineages co-harboring colistin, mcr-1, and fosfomycin, fosA, resistance genes.
Materials and Methods
E. coli Isolates for Whole Genome Sequencing
Thirteen E. coli isolates from humans (n = 2) and retail food (n = 11; five isolates from whole chicken carcasses and six isolates from ground beef) were chosen from our previous study (Ramadan et al., 2020b) for further genetic characterization using WGS. E. coli isolates from i) food samples purchased from retail live chicken shops and supermarkets, and ii) stool of diarrheic patients admitted to Mansoura University Hospitals, were all recovered between April and July 2017 from Mansoura, Egypt. Isolates were selected to represent the shared eight STs belonging to four clonal complexes (CCs) identified from chicken and beef (ST224, ST1011, ST48/CC10, ST156/CC156, ST155/CC155 and ST58/CC155), human and chicken (ST10/CC10), and human and beef (ST226/CC226). The enrolled isolates were MDR as previously determined (Ramadan et al., 2020b) (Table S1). Antimicrobials and their minimum inhibitory concentrations (MICs) were to: ampicillin (≥32 μg/ml), amoxicillin/clavulanic acid (≥32/16 μg/ml), ceftriaxone (≥4 μg/ml), azithromycin (≥32 μg/ml), chloramphenicol (≥32 μg/ml), nalidixic acid (≥32 μg/ml), ciprofloxacin (≥4 μg/ml), sulfisoxazole (≥512 μg/ml), trimethoprim/sulfamethoxazole (≥4/76 μg/ml), tetracycline (≥16 μg/ml), gentamicin (≥16 μg/ml) and streptomycin (≥64 μg/ml).
Whole Genome Sequencing and Analysis
Extraction of genomic DNA (gDNA) from E. coli isolates was performed using GenElute™ Bacterial Genomic DNA Kit (Sigma-Aldrich, St. Louis, MO). The quality check for gDNA was determined using a NanoDrop™ spectrophotometer, followed by measuring gDNA concentration on an Invitrogen Qubit 2.0 Fluorometer as instructed by the manufacturer (Life Technologies Inc., Carlsbad, CA). DNA libraries were constructed using Nextera™ XT DNA Preparation Kit and Nextera XT index primers (Illumina Inc., San Diego, CA) following the manufacturer’s protocol. Paired-end sequences of 2 × 250 bp length were then generated from DNA libraries using a 500-cycle MiSeq reagent kit version 2 (Illumina Inc., San Diego, CA) on an Illumina MiSeq system. Raw reads were assembled de novo into contigs using A5-miseq assembler (Coil et al., 2015). The assembled sequences were deposited into the National Center for Biotechnology Information (NCBI) under BioProject number PRJNA666443. The assembly statistics and accession numbers are available in Table S2. The assembled sequences of E. coli isolates were analyzed using ResFinder 4.1, PlasmidFinder 2.1, and SerotypeFinder 2.0 available at the Center for Genomic Epidemiology (CGE, https://cge.cbs.dtu.dk/services/) to identify resistance genes, plasmid replicons and serotypes, respectively. BLAST (https://blast.ncbi.nlm.nih.gov/Blast.cgi) search in combination with identification of insertion sequence (IS) elements using ISFinder (https://www-is.biotoul.fr/blast.php) illustrated the genetic environment of blaCTX-M-14, blaCTX-M-15, blaCTX-M-55, fosA3, fosA4 and mcr-1 genes among the examined isolates. Annotation of the assembled sequences was performed using DFAST (https://dfast.nig.ac.jp/), then genetic comparison was drawn using Easyfig (http://mjsull.github.io/Easyfig/) tool (Sullivan et al., 2011). Plasmid reconstruction from the WGS of isolates harboring fosA and mcr-1 genes was performed using PLACNETw (Vielva et al., 2017). The reconstructed plasmids were then aligned to the NCBI database to determine the best plasmid match. Genetic comparison of the reconstructed plasmids and the retrieved plasmid sequences from NCBI was determined using BLAST Ring Image Generator (BRIG) tool (http://sourceforge.net/projects/brig).
Phylogenetic Analysis of the Examined E. coli Isolates
To perform phylogenetic analysis, raw paired-end fastq reads of the thirteen isolates were imported into Enterobase (https://enterobase.warwick.ac.uk/), and compared to the available genomes of E. coli from Egypt updated December 4th, 2020, using single nucleotide polymorphisms (SNPs) and hierarchical clustering of core genome (cg) MLST (HierCC) based on 2,513 core genomic loci (Zhou et al., 2020). The chicken E. coli isolate 82CH co-harboring mcr-1 and fosA4 was specifically compared to the global E. coli lineages, where 59 E. coli from NCBI, that co-harbored mcr-1 and fosA genes and representing different sources were randomly selected for SNPs and HierCC in Enterobase. In both comparisons, isolates were mapped to the reference strain E. coli K-12 MG1655 for SNPs analysis, and designated to HC200 differing by ≤200 core genomic alleles. Metadata for the selected E. coli sequences from Enterobase and NCBI are listed in Tables S3, S4.
Results and Discussion
In Silico Analysis of Whole Genome Sequences of Retail Food and Human E. coli
ResFinder analysis showed the presence of several antimicrobial resistance genes among retail food (chicken and beef) and human isolates (Table 1). All isolates contained at least one β-lactamase gene (blaTEM, blaCTX-M, blaOXA and/or blaSHV) except beef isolate 11M. The blaCTX-M gene was identified in eight isolates: four from chicken (blaCTX-M-14), and two each from beef (blaCTX-M-14 and blaCTX-M-55) and human (blaCTX-M-14 and blaCTX-M-15) isolates. Of the six blaCTX-M identified from retail food (chicken and beef) isolates, blaCTX-M-14 was the prevalent variant that constituted 83.3% (5/6). Recent studies reported high prevalence of blaCTX-M-14 among E. coli isolates from retail chicken meat from Japan (Hayashi et al., 2018) and feces of food animals from South Korea (Song et al., 2020). However, reports from Egypt have determined higher occurrence of other variants than blaCTX-M-14 in isolates from food animals such as blaCTX-M-15 in E. coli from rectal swabs of healthy cattle (Braun et al., 2016), blaCTX-M-1 from chicken and beef meat (Moawad et al., 2017) and blaCTX-M-28 from retail meat products (Sabala et al., 2021).
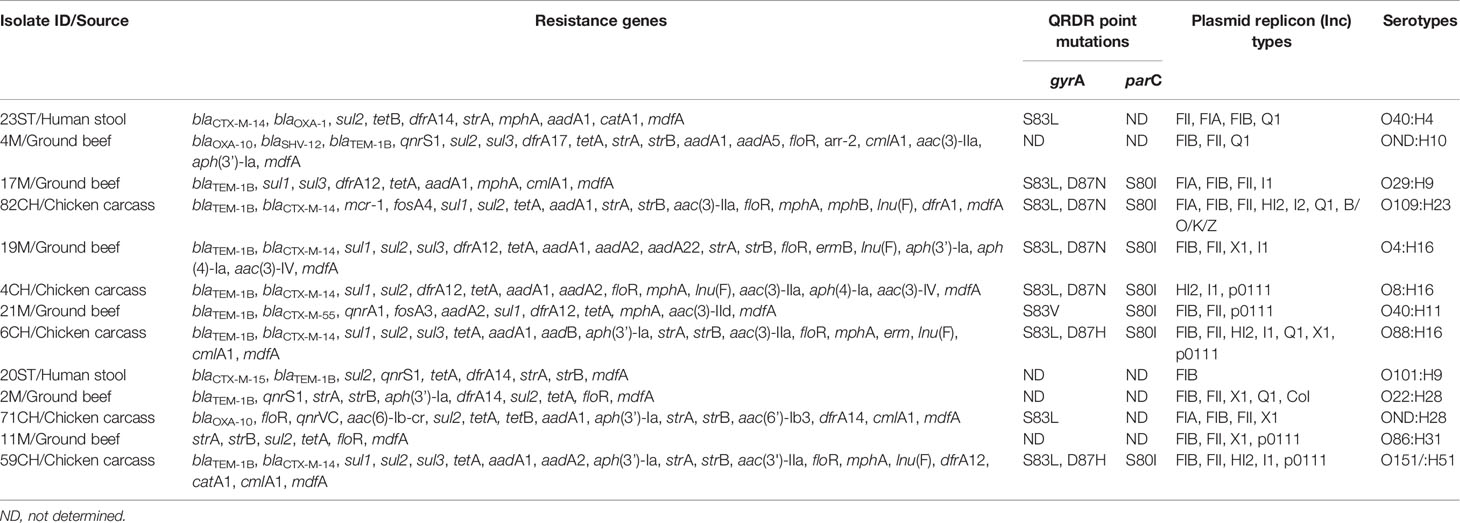
Table 1 Resistance genes, quinolone resistance-determining region (QRDR) point mutations, plasmid replicons, and serotypes of Escherichia coli from humans and retail food in Egypt.
Resistome findings also revealed the presence of two plasmid-mediated fosfomycin resistance genes: fosA3 and fosA4 from beef 21M and chicken 82CH isolates, respectively (Table 1). Furthermore, the chicken E. coli isolate 82CH that carried fosA4 also co-harbored mcr-1 responsible for colistin resistance. Recent reports from Egypt have shown the co-existence of mcr-1 and fosA4 in E. coli isolated from chicken feces (Soliman et al., 2021) and retail chicken carcass (Sadek et al., 2021). This signifies the role of poultry as potential reservoirs for the persistence and dissemination of these antimicrobial resistances in Egypt.
Five E. coli isolates carried plasmid-mediated quinolone resistance (PMQR) genes; three isolates were from beef (2M, qnrS1; 4M, qnrS1; 21M, qnrA1) and one each from chicken (71CH, qnrVC) and human (20ST, qnrS1). Only in chicken isolate 71CH, acetyl transferase gene, aac(6)-Ib-cr, was co-harbored with qnrVC. The plasmid-mediated qnr genes encode pentapeptide repeat proteins that are responsible for quinolone resistance via protecting bacterial DNA gyrase and topoisomerase IV from quinolone inhibition (Strahilevitz et al., 2009). A recent study from Egypt reported high prevalence of qnr genes among Gram-negative clinical pathogens including E. coli, Klebsiella pneumoniae, Serratia marcescens, Salmonella enterica subsp. arizonae and Pseudomonas aeruginosa; 58.7% of these isolates carried at least one qnr gene (Khalifa et al., 2019). The existence of qnr-producing E. coli in retail chicken and beef meat as reported in recent studies from different countries including Egypt (Moawad et al., 2017), the United States (Tyson et al., 2019) and Philippines (Belotindos et al., 2021), poses a serious public health threat. The functional qnrVC gene had been commonly identified in Vibrionaceae family (Zhang et al., 2018) and in P. aeruginosa isolates in China (Lin et al., 2020) playing a crucial role in quinolone resistance. To our knowledge, this is the first report of qnrVC in an E. coli isolate globally. Chromosomal mutations to gyrA and parC were also detected, where gyrA S83L was the prevalent point mutation (8/13, 61.5%) followed by parC S80I (7/13, 53.8%) and gyrA D87N (4/13, 30.8%). All the examined thirteen isolates carried at least one resistance gene to the aminoglycosides [strA, strB, aadA, aac(3), aph(3’), aph(4), aac(6’)], folate pathway inhibitors (sul, dfrA), and tetracycline (tetA, tetB) (Table 1).
Our findings showed that plasmid incompatibility (Inc) types, IncFIB (12/13, 92.3%) and IncFII (11/13, 84.6%) were the predominant plasmid replicons identified from retail food and human E. coli (Table 1). This was in agreement with previous studies that determined higher frequencies of IncF replicon types among E. coli isolates, especially those that were MDR (Carattoli, 2009; Yang et al., 2015; Adenipekun et al., 2019). A recent study from Egypt demonstrated the existence of IncF replicon types in NDM-producing E. coli isolates from humans and dogs (Ramadan et al., 2020a). The wide dissemination of IncF replicons among E. coli isolates from different sources, and the association of resistance traits, e.g. genes encoding extended spectrum β-lactamases (ESBLs), carbapenemases, aminoglycoside-modifying enzymes, and PMQR genes, to this replicon type, could be responsible for possible interspecies dissemination of these plasmid-mediated resistance genes between humans and animals (Rozwandowicz et al., 2018; Adenipekun et al., 2019).
Genetic Overview of blaCTX-M, mcr-1 and fosA Genes and Associated Plasmids
Genetic mapping of blaCTX-M variants (blaCTX-M-14, blaCTX-M-15, and blaCTX-M-55), mcr-1 and fosA has been determined as described in Figure 1. The blaCTX-M-55 identified in beef isolate 21M, was bracketed with insertion sequence (IS) IS6 and wbuC (for a cupin fold metalloprotein), whereas blaCTX-M-15 in human isolate 20ST was located upstream to ISEcp1 (Figures 1A, B). The contigs carrying blaCTX-M-55 and blaCTX-M-15 exhibited 100% sequence similarity to the corresponding parts of the plasmid pZY-1 identified from Citrobacter freundii in China (accession no. CP055248.1) and plasmid pCFSAN061768 identified from E. coli in Egypt (accession no. CP042974.1), respectively. Six E. coli isolates, four from chicken (6CH, 82CH, 4CH, 59CH) and one each from beef (19M) and human (23ST) harbored blaCTX-M-14. In chicken and beef isolates, blaCTX-M-14 was located upstream to ISEcp1 similar to blaCTX-M-15 in human isolate 20ST. This was in concordance with previous studies reporting the association of ISEcp1 with different blaCTX-M variants such as blaCTX-M-1, -2, -9, -25 (Rossolini et al., 2008), blaCTX-M-15 (Casella et al., 2018) and blaCTX-M-14 (Liao et al., 2015; Tadesse et al., 2018). The contigs carrying blaCTX-M-14 in the four chicken isolates showed high similarity to each other and to the backbone of the IncHI2 plasmid, while beef isolate 19M harbored a chromosomal blaCTX-M-14 (Figure 1C). This highlights the potential role of ISEcp1 in mobilization of blaCTX-M-14 regardless of whether the gene was located on plasmids or the chromosome (Zhao et al., 2020).
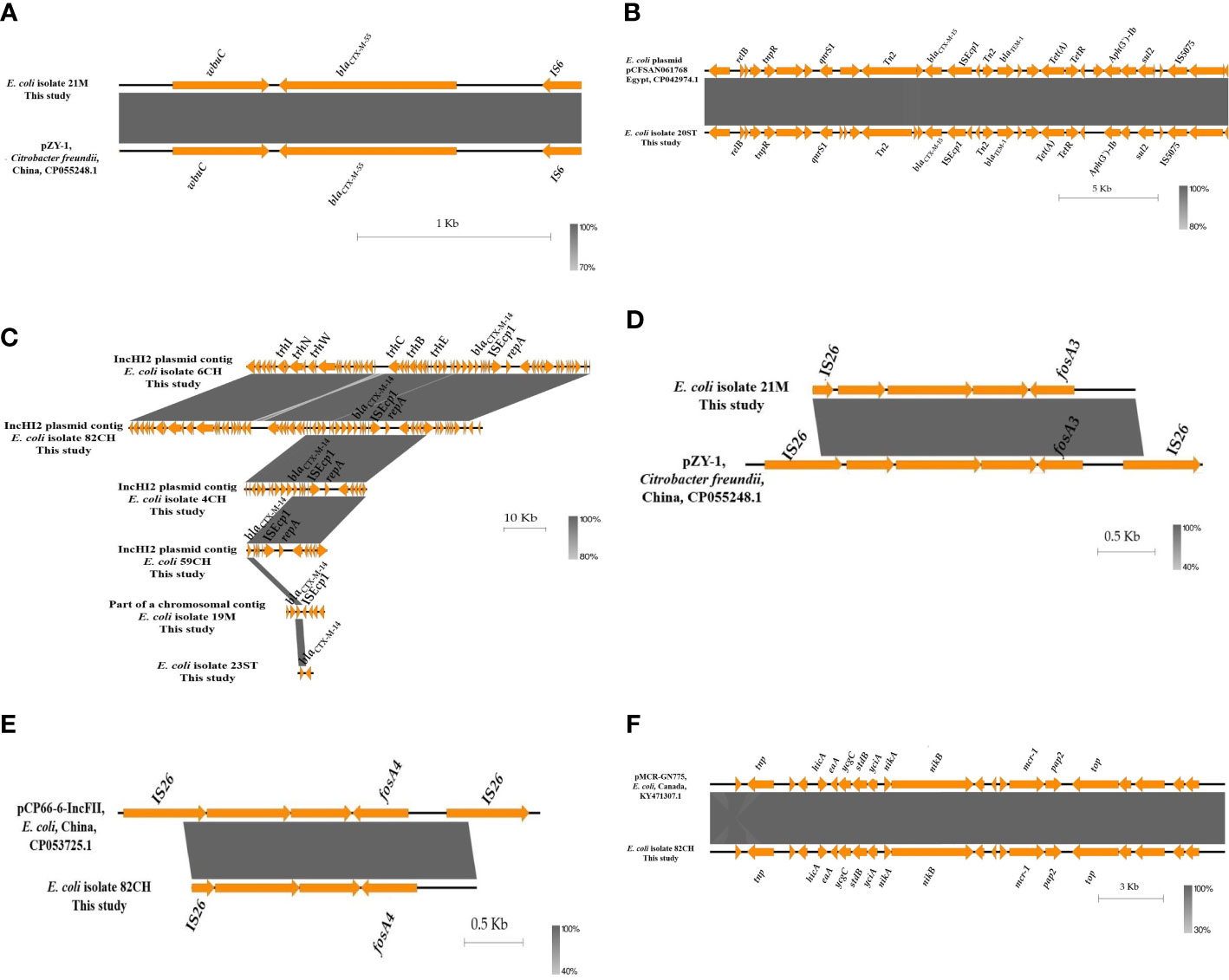
Figure 1 Schematic representation of the genetic environments of blaCTX-M-55 (A), blaCTX-M-15 (B), blaCTX-M-14 (C), fosA3 (D), fosA4 (E) and mcr-1 (F) identified from the whole genome sequences of the examined Escherichia coli isolates from retail food and human. The figure was drawn using the EasyFig tool (http://mjsull.github.io/Easyfig/).
Both variants of fosA, fosA3 in beef isolate 21M and fosA4 in chicken isolate 82CH, were located downstream to IS26 belonging to the IS6 family (Figures 1D, E), which might be involved in their mobilization. Sequences of fosA plasmids were recovered from WGS of beef (21M) and chicken (82CH) E. coli isolates using PLACNETw. The reconstructed fosA3 (p21M-IncF) and fosA4 (p82CH-IncF) plasmids showed approximately 72% sequence similarities to the entire IncFII plasmids pZY-1 (accession no. CP055248.1, size 89.6 kb) identified from C. freundii in China and pCP66-6-IncFII (accession no. CP053725.1, size 74.8 kb) identified from E. coli in China, respectively. The maintenance (repA, replicase; parM/stbA, partition and stability; psiB, SOS inhibition) and transfer (tra) genes of p21M-IncF and p82CH-IncF plasmids were identical to the sequences of the corresponding genes of pZY-1 and pCP66-6-IncFII plasmids, respectively (Figures 2A, B). The existence of fosA in both isolates (21M and 82CH) adjacent to IS26 borne by IncFII plasmid, a widely known plasmid type for the dissemination of fosA worldwide (Benzerara et al., 2017), is alarming and could suggest retail chicken and beef as potential sources of this resistance gene to humans in Egypt. In our mcr-1 isolate (82CH) from chicken carcass, the genetic context (nikA- nikB [encoding relaxase)- mcr-1- pap2- top (encoding a DNA topoisomerase III)] was detected without ISApl1, a commonly associated IS with mcr-1 (Figure 1F). A similar mcr-1 context has been identified previously in E. coli from beef sausage in Egypt (Sadek et al., 2020). BLAST analysis of the reconstructed mcr-1 plasmid (p82CH) from this study isolate showed 97% sequence similarity to the mcr-1-carrying IncI2 plasmid pMCR-GN775 (accession no. KY471307.1, size 64.6 kb) detected from an E. coli ST624 isolated from a Canadian patient with a history of hospitalization in Egypt (Tijet et al., 2017), and 96% similarity to IncI2 plasmid pEGMCR (accession no. MT499885.1, size ~64.1 kb) from a retail chicken isolate from Egypt (Figure 2C). IncI2 plasmid p82CH carried pilus and conjugative transfer proteins pilQ, pilR, pilS and pilV which are responsible for the conjugal transfer of that plasmid (Darphorn et al., 2021). This provides evidence that mcr-1-carrying IncI2 plasmid could be circulating among food animals and humans in Egypt.
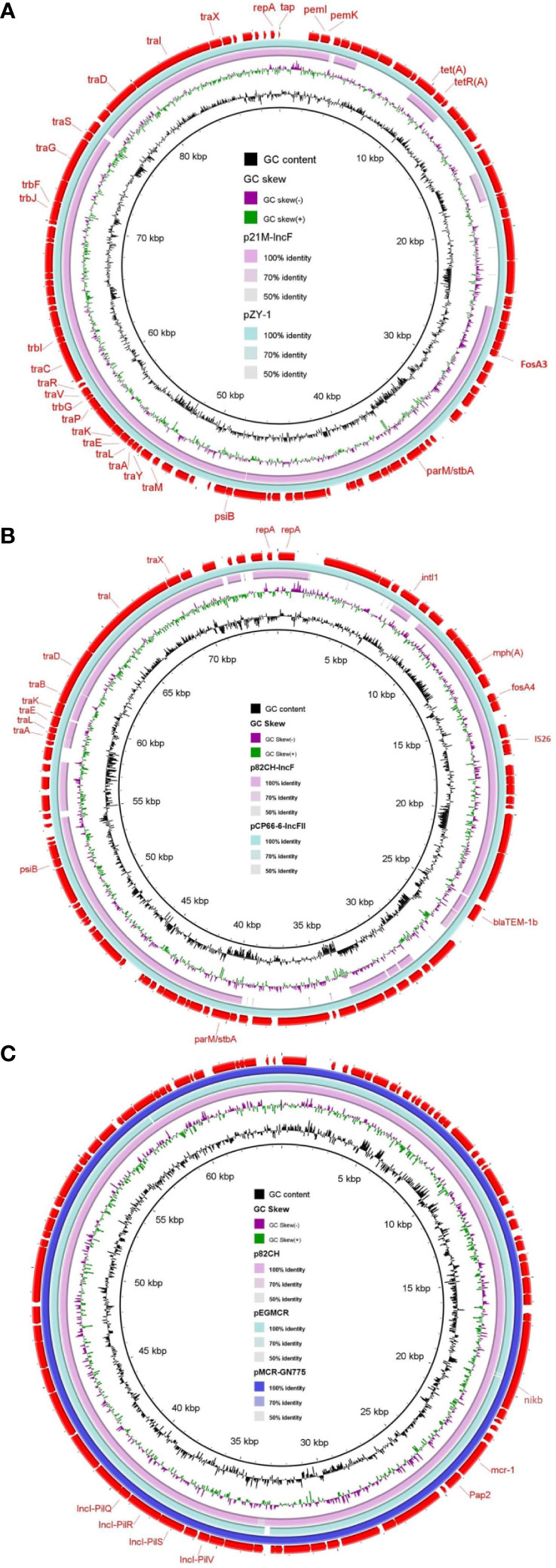
Figure 2 Comparative sequence analysis of fosA3-, fosA4- and mcr-1-carrying plasmids from the examined Escherichia coli isolates. Sequence comparison of reconstructed fosA3 plasmid (p21M-IncF) from whole-genome sequence (WGS) of beef isolate 21M (A). Sequence comparison of reconstructed fosA4 plasmid (p82CH-IncF) from WGS of chicken isolate 82CH (B). Sequence comparison of reconstructed mcr-1 plasmid (p82CH) from WGS of chicken isolate 82CH (C). The out-layer circle (red color) in (A–C) represents the reference plasmids used for sequence comparisons: pZY-1 plasmid (accession no. CP055248.1) (A), pCP66-6-IncFII plasmid (accession no. CP053725.1) (B), and pMCR-GN775 plasmid (accession no. KY471307.1) (C). The figure was generated using BLAST Ring Image Generator (BRIG) tool (http://sourceforge.net/projects/brig).
Phylogenetic Analysis of the Examined E. coli Using SNPs and HierCC
SNPs and HierCC integrated into Enterobase were performed to investigate the phylogenetic relatedness of the examined E. coli isolates with publicly available E. coli genomes from Egypt (Figure 3A and Table S3). In Enterobase, HierCC designations have been set based on differences of 2,513 core genes among isolates with eleven designations: HC0, HC2, HC5, HC10, HC20, HC50, HC100, HC200, HC400, HC1100, HC2350; HC0 corresponds to indistinguishable isolates, HC1100 to ST lineage and HC2350 to Escherichia species (Zhou et al., 2020). Chicken isolate 59CH was clustered and assigned to similar HC200 (HC200_1157) with E. coli previously isolated from chicken feces (A-1-4-1 and A-1-8-1), which possibly explains the circulation of this clone among poultry in Egypt. When the chicken isolate 82CH was compared to the global E. coli co-harboring mcr-1 and fosA, our isolate was clustered and shared a similar HC200 (HC200_2281) with an E. coli (XM1416) isolated from diseased broiler from China (Figure 3B and Table S4). This could indicate the wide circulation of this lineage co-harboring mcr-1 and fosA4 across different continents that might be attributed to the global trade in food animals and food products (Ludden et al., 2020).
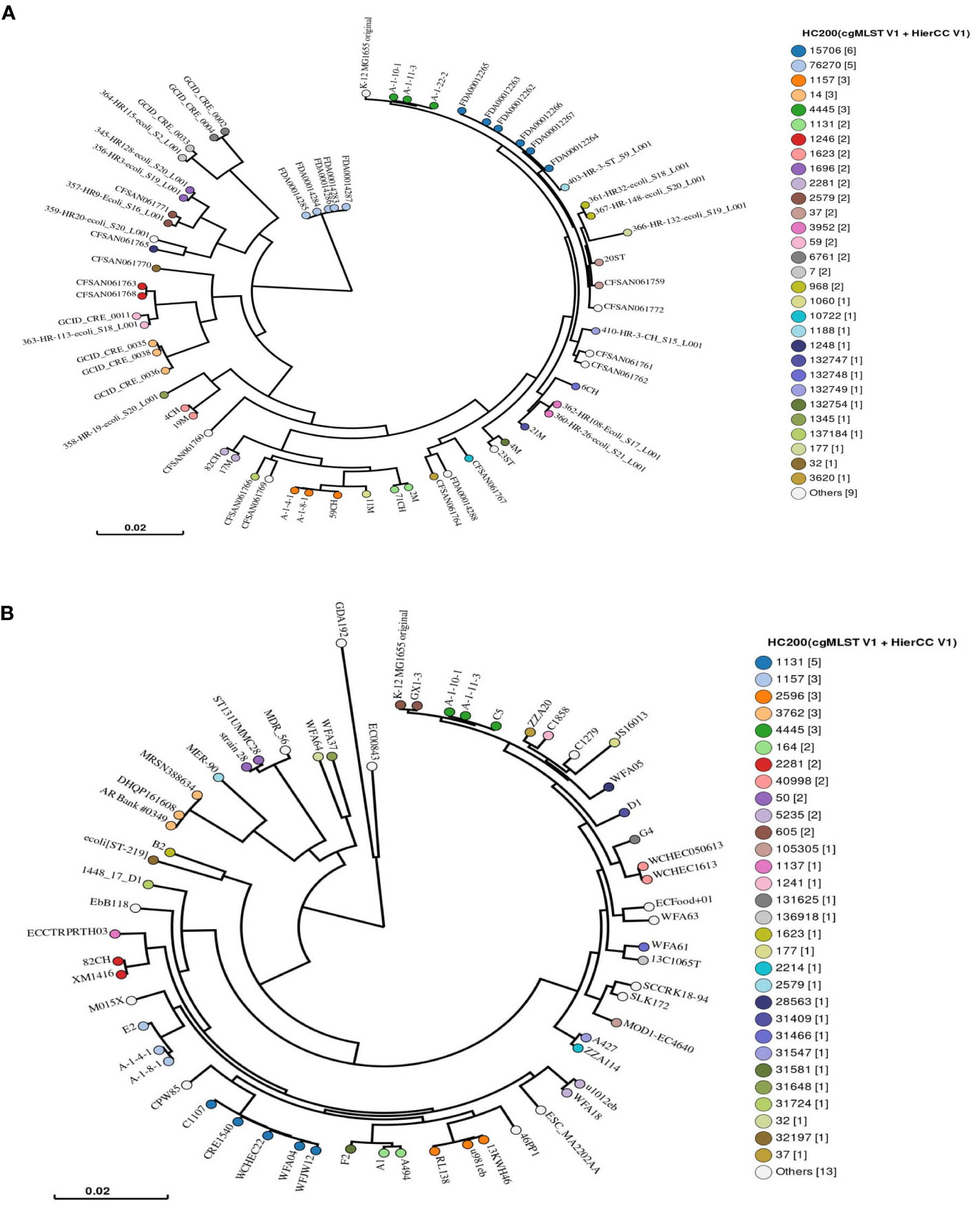
Figure 3 Single nucleotide polymorphisms (SNPs) and hierarchical clustering of cgMLST (HierCC) of the examined Escherichia coli isolates with the publicly available E. coli isolates from Egypt (A) in Enterobase (https://enterobase.warwick.ac.uk/). The chicken isolate 82CH co-harboring mcr-1 and fosA4 genes was compared to 59 E. coli isolates from NCBI representing different sources using SNPs and HierCC analysis (B). The legend shows the cgMLST HC200 that indicates allelic differences no more than 200 of 2,513 core genomic alleles among isolates.
To conclude, this study reported the emergence of blaCTX-M, fosA and mcr-1 genes among retail food isolates in Egypt using WGS. Coupling our findings with recent reports from Egypt, we found that chicken could be the potential source for the emergence of E. coli co-harboring mcr-1 and fosA4 genes. Moreover, comparative sequence analyses of blaCTX-M, fosA and mcr-1 genes and their associated plasmids among the examined E. coli from retail food, showed the existence of genetic features such as insertion sequences (IS) and certain plasmid Inc types, that are responsible for the mobilization and horizontal transfer of these genes. The global expansion of E. coli co-harboring mcr-1 and fosA from different continents, requires the implementation of WGS for surveillance and control interventions.
Author's Note
The mention of trade names or commercial products in this manuscript is solely for the purpose of providing specific information and does not imply recommendation or endorsement by the U.S. Department of Agriculture. USDA is an equal opportunity provider and employer.
Data Availability Statement
The datasets presented in this study can be found in online repositories. The names of the repository/repositories and accession number(s) can be found below: https://www.ncbi.nlm.nih.gov/, PRJNA666443/.
Author Contributions
HR and CRJ conceived and designed the study. HR performed whole genome sequencing of isolates, analyzed the data and wrote the original draft of the manuscript. AMS participated in data analysis and to the writing of the manuscript. LMH and TAW contributed to the laboratory work, whole genome sequencing and data analysis. ME contributed to genome analysis. CRJ and JGF secured funding and provided project administration. HR, AMS, ME, MAC, CJ, JGF and CRJ reviewed and edited the manuscript. All authors contributed to the article and approved the submitted version.
Funding
This work has been funded by the U.S. Department of Agriculture (USDA) project 6040-32000-079-00-D. The Science, Technology and Innovation Fund Authority (STIFA) of Egypt (Short term fellowship, ID 25449) partially supported this work.
Conflict of Interest
The authors declare that the research was conducted in the absence of any commercial or financial relationships that could be construed as a potential conflict of interest.
Acknowledgments
The authors acknowledge the Daniel Turnberg UK/Middle East Travel Fellowship for funding the visit of Dr. Hazem Ramadan to Public Health England, UK.
Supplementary Material
The Supplementary Material for this article can be found online at: https://www.frontiersin.org/articles/10.3389/fcimb.2021.681588/full#supplementary-material
References
Adenipekun, E. O., Jackson, C. R., Ramadan, H., Iwalokun, B. A., Frye, J. G., Barrett, J. B., et al. (2019). Plasmid Replicons and β-Lactamase-Encoding Genes of Multidrug-Resistant Escherichia Coli Isolated From Humans and Food Animals in Lagos, Southwest Nigeria. Microb. Drug Resist. 25 (10), 1410–1423. doi: 10.1089/mdr.2018.0305
Belotindos, L., Villanueva, M., Miguel, J., Bwalya, P., Harada, T., Kawahara, R., et al. (2021). Prevalence and Characterization of Quinolone-Resistance Determinants in Escherichia Coli Isolated From Food-Producing Animals and Animal-Derived Food in the Philippines. Antibiotics 10 (4), 413. doi: 10.3390/antibiotics10040413
Benzerara, Y., Gallah, S., Hommeril, B., Genel, N., Decré, D., Rottman, M., et al. (2017). Emergence of Plasmid-Mediated Fosfomycin-Resistance Genes Among Escherichia Coli Isolates, France. Emerg. Infect. Dis. 23 (9), 1564–1567. doi: 10.3201/eid2309.170560
Braun, S. D., Ahmed, M. F. E., El-Adawy, H., Hotzel, H., Engelmann, I., Weiß, D., et al. (2016). Surveillance of Extended-Spectrum Beta-Lactamase-Producing Escherichia Coli in Dairy Cattle Farms in the Nile Delta, Egypt. Front. Microbiol. 7, 1020. doi: 10.3389/fmicb.2016.01020
Cantón, R., González-Alba, J., Galán, J. (2012). CTX-M Enzymes: Origin and Diffusion. Front. Microbiol. 3, 110. doi: 10.3389/fmicb.2012.00110
Carattoli, A. (2009). Resistance Plasmid Families in Enterobacteriaceae. Antimicrob. Agents Chemother. 53 (6), 2227. doi: 10.1128/AAC.01707-08
Casella, T., Haenni, M., Madela, N. K., Andrade, L. K. D., Pradela, L. K., Andrade, L. N. D., et al. (2018). Extended-Spectrum Cephalosporin-Resistant Escherichia Coli Isolated From Chickens and Chicken Meat in Brazil Is Associated With Rare and Complex Resistance Plasmids and Pandemic ST Lineages. J. Antimicrob. Chemother. 73 (12), 3293–3297. doi: 10.1093/jac/dky335
Coil, D., Jospin, G., Darling, A. E. (2015). A5-Miseq: An Updated Pipeline to Assemble Microbial Genomes From Illumina MiSeq Data. Bioinformatics 31 (4), 587–589. doi: 10.1093/bioinformatics/btu661
Darphorn, T. S., Bel, K., Koenders-van Sint Anneland, B. B., Brul, S., Ter Kuile, B. H. (2021). Antibiotic Resistance Plasmid Composition and Architecture in Escherichia Coli Isolates From Meat. Sci. Rep. 11 (1), 2136. doi: 10.1038/s41598-021-81683-w
Hayashi, W., Ohsaki, Y., Taniguchi, Y., Koide, S., Kawamura, K., Suzuki, M., et al. (2018). High Prevalence of blaCTX-M-14 Among Genetically Diverse Escherichia Coli Recovered From Retail Raw Chicken Meat Portions in Japan. Int. J. Food Microbiol. 284, 98–104. doi: 10.1016/j.ijfoodmicro.2018.08.003
Karaiskos, I., Lagou, S., Pontikis, K., Rapti, V., Poulakou, G. (2019). The “Old” and the “New” Antibiotics for MDR Gram-Negative Pathogens: For Whom, When, and How. Front. Public Health 7, 151. doi: 10.3389/fpubh.2019.00151
Khalifa, H. O., Soliman, A. M., Ahmed, A. M., Shimamoto, T., Nariya, H., Matsumoto, T., et al. (2019). High Prevalence of Antimicrobial Resistance in Gram-Negative Bacteria Isolated From Clinical Settings in Egypt: Recalling for Judicious Use of Conventional Antimicrobials in Developing Nations. Microb. Drug Resist. 25 (3), 371–385. doi: 10.1089/mdr.2018.0380
Klemm, E. J., Wong, V. K., Dougan, G. (2018). Emergence of Dominant Multidrug-Resistant Bacterial Clades: Lessons From History and Whole-Genome Sequencing. Proc. Natl. Acad. Sci. 115 (51), 12872. doi: 10.1073/pnas.1717162115
Liao, X.-P., Xia, J., Yang, L., Li, L., Sun, J., Liu, Y.-H., et al. (2015). Characterization of CTX-M-14-Producing Escherichia Coli From Food-Producing Animals. Front. Microbiol. 6, 1136. doi: 10.3389/fmicb.2015.01136
Lin, J., Chen, D.-Q., Hong, J., Huang, H., Xu, X. (2020). Prevalence of qnrVC Genes in Pseudomonas Aeruginosa Clinical Isolates From Guangdong, China. Curr. Microbiol. 77 (8), 1532–1539. doi: 10.1007/s00284-020-01974-9
Liu, P., Chen, S., Wu, Z.-Y., Qi, M., Li, X.-Y., Liu, C.-X. (2020). Mechanisms of Fosfomycin Resistance in Clinical Isolates of Carbapenem-Resistant Klebsiella Pneumoniae. J. Glob. Antimicrob. Resist. 22, 238–243. doi: 10.1016/j.jgar.2019.12.019
Liu, Y.-Y., Wang, Y., Walsh, T. R., Yi, L.-X., Zhang, R., Spencer, J., et al. (2016). Emergence of Plasmid-Mediated Colistin Resistance Mechanism MCR-1 in Animals and Human Beings in China: A Microbiological and Molecular Biological Study. Lancet Infect. Dis. 16 (2), 161–168. doi: 10.1016/S1473-3099(15)00424-7
Li, R., Xie, M., Zhang, J., Yang, Z., Liu, L., Liu, X., et al. (2017). Genetic Characterization of mcr-1-bearing Plasmids to Depict Molecular Mechanisms Underlying Dissemination of the Colistin Resistance Determinant. J. Antimicrob. Chemother. 72 (2), 393–401. doi: 10.1093/jac/dkw411
Ludden, C., Decano, A. G., Jamrozy, D., Pickard, D., Morris, D., Parkhill, J., et al. (2020). Genomic Surveillance of Escherichia Coli ST131 Identifies Local Expansion and Serial Replacement of Subclones. Microb. Genomics 6 (4), e000352. doi: 10.1099/mgen.0.000352
Lupo, A., Saras, E., Madec, J.-Y., Haenni, M. (2018). Emergence of blaCTX-M-55 Associated With fosA, rmtB and Mcr Gene Variants in Escherichia Coli From Various Animal Species in France. J. Antimicrob. Chemother. 73 (4), 867–872. doi: 10.1093/jac/dkx489
Moawad, A. A., Hotzel, H., Awad, O., Tomaso, H., Neubauer, H., Hafez, H. M., et al. (2017). Occurrence of Salmonella Enterica and Escherichia Coli in Raw Chicken and Beef Meat in Northern Egypt and Dissemination of Their Antibiotic Resistance Markers. Gut Pathog. 9, 57–57. doi: 10.1186/s13099-017-0206-9
Nemoy, L. L., Kotetishvili, M., Tigno, J., Keefer-Norris, A., Harris, A. D., Perencevich, E. N., et al. (2005). Multilocus Sequence Typing Versus Pulsed-Field Gel Electrophoresis for Characterization of Extended-Spectrum Beta-Lactamase-Producing Escherichia Coli Isolates. J. Clin. Microbiol. 43 (4), 1776–1781. doi: 10.1128/JCM.43.4.1776-1781.2005
Pokharel, S., Shrestha, P., Adhikari, B. (2020). Antimicrobial Use in Food Animals and Human Health: Time to Implement ‘One Health’ Approach. Antimicrob. Resist. Infect. Control 9 (1), 181. doi: 10.1186/s13756-020-00847-x
Ramadan, H., Gupta, S. K., Sharma, P., Ahmed, M., Hiott, L. M., Barrett, J. B., et al. (2020a). Circulation of Emerging NDM-5-Producing Escherichia Coli Among Humans and Dogs in Egypt. Zoonoses Public Health 67 (3), 324–329. doi: 10.1111/zph.12676
Ramadan, H., Jackson, C. R., Frye, J. G., Hiott, L. M., Samir, M., Awad, A., et al. (2020b). Antimicrobial Resistance, Genetic Diversity and Multilocus Sequence Typing of Escherichia Coli From Humans, Retail Chicken and Ground Beef in Egypt. Pathogens 9 (5), 357. doi: 10.3390/pathogens9050357
Rossolini, G. M., D’Andrea, M. M., Mugnaioli, C. (2008). The Spread of CTX-M-Type Extended-Spectrum β-Lactamases. Clin. Microbiol. Infect. 14, 33–41. doi: 10.1111/j.1469-0691.2007.01867.x
Rozwandowicz, M., Brouwer, M. S. M., Fischer, J., Wagenaar, J. A., Gonzalez-Zorn, B., Guerra, B., et al. (2018). Plasmids Carrying Antimicrobial Resistance Genes in Enterobacteriaceae. J. Antimicrob. Chemother. 73 (5), 1121–1137. doi: 10.1093/jac/dkx488
Sabala, R. F., Usui, M., Tamura, Y., Abd-Elghany, S. M., Sallam, K. I., Elgazzar, M. M. (2021). Prevalence of Colistin-Resistant Escherichia Coli Harbouring Mcr-1 in Raw Beef and Ready-to-Eat Beef Products in Egypt. Food Control 119, 107436. doi: 10.1016/j.foodcont.2020.107436
Sadek, M., Ortiz de la Rosa, J. M., Abdelfattah Maky, M., Korashe Dandrawy, M., Nordmann, P., Poirel, L. (2021). Genomic Features of MCR-1 and Extended-Spectrum β-Lactamase-Producing Enterobacterales From Retail Raw Chicken in Egypt. Microorganisms 9 (1), 195. doi: 10.3390/microorganisms9010195
Sadek, M., Poirel, L., Nordmann, P., Nariya, H., Shimamoto, T., Shimamoto, T. (2020). Draft Genome Sequence of an Mcr-1/IncI2-carrying Multidrug-Resistant Escherichia Coli B1:ST101 Isolated From Meat and Meat Products in Egypt. J. Glob. Antimicrob. Resist. 20, 41–42. doi: 10.1016/j.jgar.2019.11.015
Sherry, N., Howden, B. (2018). Emerging Gram Negative Resistance to Last-Line Antimicrobial Agents Fosfomycin, Colistin and Ceftazidime-Avibactam - Epidemiology, Laboratory Detection and Treatment Implications. Expert Rev. Anti Infect. Ther. 16 (4), 289–306. doi: 10.1080/14787210.2018.1453807
Soliman, A. M., Ramadan, H., Zarad, H., Sugawara, Y., Yu, L., Sugai, M., et al. (2021). Co-Production of Tet(X7) Conferring High-Level Tigecycline Resistance, Fosfomycin FosA4 and Colistin Mcr-1.1 Escherichia Coli Strains From Chickens in Egypt. Antimicrob. Agents Chemother. 65 (6), e02084–20. doi: 10.1128/AAC.02084-20
Song, J., Oh, S.-S., Kim, J., Park, S., Shin, J. (2020). Clinically Relevant Extended-Spectrum β-Lactamase-Producing Escherichia Coli Isolates From Food Animals in South Korea. Front. Microbiol. 11, 604. doi: 10.3389/fmicb.2020.00604
Strahilevitz, J., Jacoby, G. A., Hooper, D. C., Robicsek, A. (2009). Plasmid-Mediated Quinolone Resistance: A Multifaceted Threat. Clin. Microbiol. Rev. 22 (4), 664. doi: 10.1128/CMR.00016-09
Sullivan, M. J., Petty, N. K., Beatson, S. A. (2011). Easyfig: A Genome Comparison Visualizer. Bioinformatics 27 (7), 1009–1010. doi: 10.1093/bioinformatics/btr039
Tadesse, D. A., Li, C., Mukherjee, S., Hsu, C.-H., Bodeis Jones, S., Gaines, S. A., et al. (2018). Whole-Genome Sequence Analysis of CTX-M Containing Escherichia Coli Isolates From Retail Meats and Cattle in the United States. Microb. Drug Resist. (Larchmont N. Y.) 24 (7), 939–948. doi: 10.1089/mdr.2018.0206
Tartof, S. Y., Solberg, O. D., Manges, A. R., Riley, L. W. (2005). Analysis of a Uropathogenic Escherichia Coli Clonal Group by Multilocus Sequence Typing. J. Clin. Microbiol. 43 (12), 5860–5864. doi: 10.1128/JCM.43.12.5860-5864.2005
Tijet, N., Faccone, D., Rapoport, M., Seah, C., Pasterán, F., Ceriana, P., et al. (2017). Molecular Characteristics of mcr-1-carrying Plasmids and New Mcr-1 Variant Recovered From Polyclonal Clinical Escherichia Coli From Argentina and Canada. PloS One 12 (7), e0180347. doi: 10.1371/journal.pone.0180347
Tyson, G. H., Li, C., Hsu, C.-H., Bodeis-Jones, S., McDermott, P. F. (2019). Diverse Fluoroquinolone Resistance Plasmids From Retail Meat E. Coli in the United States. Front. Microbiol. 10, 2826. doi: 10.3389/fmicb.2019.02826
Uelze, L., Grützke, J., Borowiak, M., Hammerl, J. A., Juraschek, K., Deneke, C., et al. (2020). Typing Methods Based on Whole Genome Sequencing Data. One Health Outlook 2 (1), 3. doi: 10.1186/s42522-020-0010-1
Van, T. T. H., Yidana, Z., Smooker, P. M., Coloe, P. J. (2020). Antibiotic Use in Food Animals Worldwide, With a Focus on Africa: Pluses and Minuses. J. Glob. Antimicrob. Resist. 20, 170–177. doi: 10.1016/j.jgar.2019.07.031
Vielva, L., de Toro, M., Lanza, V. F., de la Cruz, F. (2017). PLACNETw: A Web-Based Tool for Plasmid Reconstruction From Bacterial Genomes. Bioinformatics 33 (23), 3796–3798. doi: 10.1093/bioinformatics/btx462
Yang, Q.-E., Sun, J., Li, L., Deng, H., Liu, B.-T., Fang, L.-X., et al (2015). IncF Plasmid Diversity in Multi-Drug Resistant Escherichia Coli Strains From Animals in China. Front. Microbiol. 6, 964. doi: 10.3389/fmicb.2015.00964
Zhang, P., Wang, J., Wang, X., Bai, X., Ma, J., Dang, R., et al (2019). Characterization of Five Escherichia Coli Isolates Co-Expressing ESBL and MCR-1 Resistance Mechanisms From Different Origins in China. Front. Microbiol. 10, 1994. doi: 10.3389/fmicb.2019.01994
Zhang, Y., Zheng, Z., Chan, E. W., Dong, N., Xia, X., Chen, S. (2018). Molecular Characterization of qnrVC Genes and Their Novel Alleles in Vibrio Spp. Isolated From Food Products in China. Antimicrob. Agents Chemother. 62 (7), e00529–18. doi: 10.1128/aac.00529-18
Zhao, Q.-Y., Chen, P.-X., Yang, L., Cai, R.-M., Zhu, J.-H., Fang, L.-X., et al. (2020). Transmission of Plasmid-Borne and Chromosomal blaCTX-M-64 Among Escherichia Coli and Salmonella Isolates From Food-Producing Animals Via ISEcp1-Mediated Transposition. J. Antimicrob. Chemother. 75 (6), 1424–1427. doi: 10.1093/jac/dkaa044
Keywords: Escherichia coli, retail food, whole genome sequencing, genetic context, mcr-1, fosA, cgMLST clustering
Citation: Ramadan H, Soliman AM, Hiott LM, Elbediwi M, Woodley TA, Chattaway MA, Jenkins C, Frye JG and Jackson CR (2021) Emergence of Multidrug-Resistant Escherichia coli Producing CTX-M, MCR-1, and FosA in Retail Food From Egypt. Front. Cell. Infect. Microbiol. 11:681588. doi: 10.3389/fcimb.2021.681588
Received: 17 March 2021; Accepted: 07 June 2021;
Published: 13 July 2021.
Edited by:
Nora Lía Padola, National University of Central Buenos Aires, ArgentinaReviewed by:
Andrés González, Institute for Health Research Aragón (IIS Aragón), SpainTiago Casella, Faculty of Medicine of São José do Rio Preto, Brazil
Copyright © 2021 Ramadan, Soliman, Hiott, Elbediwi, Woodley, Chattaway, Jenkins, Frye and Jackson. This is an open-access article distributed under the terms of the Creative Commons Attribution License (CC BY). The use, distribution or reproduction in other forums is permitted, provided the original author(s) and the copyright owner(s) are credited and that the original publication in this journal is cited, in accordance with accepted academic practice. No use, distribution or reproduction is permitted which does not comply with these terms.
*Correspondence: Hazem Ramadan, hazem_hassan@mans.edu.eg; Charlene R. Jackson, charlene.jackson@usda.gov