The Tick Microbiota Dysbiosis Promote Tick-Borne Pathogen Transstadial Transmission in a Babesia microti–Infected Mouse Model
- Key Laboratory of Animal Parasitology of Ministry of Agriculture, Shanghai Veterinary Research Institute, Chinese Academy of Agricultural Sciences, Shanghai, China
Ticks are obligate hematophagous ectoparasites. They are important vectors for many pathogens, of both medical and veterinary importance. Antibiotic residues in animal food are known, but very little is known about the effects of antibiotic residues in animals on the microbiome diversity of ticks and tick-borne pathogen transmission. We used a Haemaphysalis longicornis–infested mouse model to evaluate the effect of antibiotic usage on tick microbiome. Nymphal ticks were fed on an antibiotic cocktail-treated or water control mice. Adult ticks molted from nymphs fed on the antibiotic cocktail-treated mouse had a dysbiosed microbiota. Nymphal ticks were also fed on a B. microti–infected mice that had been treated with antibiotic cocktail or water. We found that the B. microti infection in adult ticks with a dysbiosed microbiota (44.7%) was increased compared with the control adult ticks (24.2%) by using qPCR targeting 18S rRNA gene. This may increase the risk of tick-borne pathogens (TBPs) transmission from adult ticks to a vertebrate host. These results show that an antibiotic-treated mouse can induce tick microbiota dysbiosis. Antibiotic treatment of B. microti-infected mouse poses the possibility of increasing transstadial transmission of B. microti from the nymph to the adult H. longicornis. These findings suggest that B. microti transmission may be exacerbated in high antibiotic usage areas.
Introduction
Ticks are obligate hematophagous ectoparasites of vertebrates, which feed on a wide range of hosts, including mammals, birds, and reptiles. Ticks are major vectors of many pathogens, especially zoonotic pathogens. Ticks are important vectors of human infectious diseases worldwide and are second only to mosquitoes in terms of public health importance. Ticks are responsible for the transmission of infectious agents, such as bacteria (Coxiella, Rickettsia, Borrelia, and Anaplasma), viruses (severe fever with thrombocytopenia syndrome virus, tick-borne encephalitis virus, and Alongshan virus), and parasites (Babesia, and Theileria).
The four development stages of ticks are eggs, larvae, nymphs, and adults. All mobile life stages bite humans and animals. Nymphs and adult females are most commonly encountered. Nymphs and adults are also the most important stages for the transmission of tick-borne pathogens (TBPs). Nymphs are one of the most dangerous tick stage, because they are small and difficult to detect. Few TBPs are transmitted transovarially, and most TBDs are transmitted by nymphs or adult ticks (Bonnet et al., 2017). The ixodid ticks typically feed only once during each developmental stage, after which they detach from the host. Unfed adult ticks do not acquire pathogens from nymphs. Pathogens are transstadially transmitted from nymphs to adults. Furthermore, adult ticks effectively transmit pathogens to vertebrate hosts during blood feeding. The study of transstadial transmission of TBPs from nymphs to adults is significant for tick and TBD control.
Microbial communities within vectors are important in pathogen colonization. They subsist in vectors and can influence pathogen transmission from vectors to hosts (Gall et al., 2016; Abraham et al., 2017; Rodgers et al., 2017). The bacterial microbiome of Dermacentor andersoni ticks influences pathogen susceptibility (Gall et al., 2016). The blood meal source significantly influences tick microbiomes and may impact pathogen transmission (Swei and Kwan, 2017). Ixodes scapularis ticks do not harbor a stable midgut microbiome (Ross et al., 2018). In Anopheles mosquitoes, malaria parasite infection increased when gut microbiota colonization was impaired by feeding on an antibiotic-treated sugar solution (Dong et al., 2009; Meister et al., 2009). Antimicrobial agents and antibiotics have been widely used on animal farms for treatment of animal diseases. Antibiotics are also commonly used to treat skin lesions and TBD associated with tick bites, such as Lyme disease on humans. Tick populations and TBD are increasing, and newly emerging TBPs are being reported. The antibiotics present in the blood meal of ticks could potentially interfere with the tick microbiome and result in tick gut dysbiosis. This may enhance tick susceptibility to pathogens and promote TBP transmission.
To test this possibility, we used an H. longicornis infestation model and a B. microti infection model in mice. H. longicornis is an important tick species that maintains and transmits a variety of zoonotic pathogen agents, including B. microti, severe fever with thrombocytopenia virus (SFTSV), Rickettsia raoultii, Ehrlichia chaffeensis, and Borrrlia garinii. H. longicornis is a new and emerging threat to public health in US states (Tanne, 2018). Babesiosis is a worldwide, malaria-like tick-borne zoonotic infectious disease that can lead to livestock losses and health issues for humans. The genus Babesia, including obligate intraerythrocytic protozoans, includes the causative agents of babesiosis. B. microti is the most prevalent species associated with human babesiosis in the USA and in study regions of the People’s Republic of China (Vannier and Krause, 2012; Ord and Lobo, 2015).
We previously demonstrated that tick nymphs fed on B. microti–infected mice could molt into infected adult ticks (Wu et al., 2017). B. microti is typically transmitted to the host during the blood meal. This feeding strategy provides an ideal pathogen model to study the tick microbiome and its effects on pathogen transmission by the tick vector. In our antibiotic model for mice treatment, we used a cocktail of ampicillin, metronidazole, neomycin trisulfate, and vancomycin, which are all important antimicrobials in human and veterinary medicine (World Health Organization, 2017).
Materials and Methods
Ethics Statement
All animal experimental protocols in this study were approved by the Institutional Animal Care and Use Committee of Shanghai Veterinary Research Institute (IACUC approval number: SHVRI-SZ-20200608-01). After approval, the experiment was carried out in compliance with the Guidelines on the Humane Treatment of Laboratory Animals.
Mice, Ticks, and B. microti
Six- to 8-week-old female BALB/c mice (18-20 g/mouse) were purchased from SPF (Beijing) Biotechnology Co., LTD (Beijing, China). The mice were housed under specific pathogen free conditions at the Animal Center of the Shanghai Veterinary Research Institute for 3 to 5 days for acclimation under standard 12-h light cycle with free access to food and water. H. longicornis ticks (parthenogenetic strain) were obtained from a tick colony maintained in the laboratory at 25°C with 90% relative humidity in a dark incubator. H. longicornis ticks used in this study were fed on BALB/c mice to keep the life cycle. The B. microti strain was obtained from the American Type Culture Collection (ATCC PRA-99TM; Manassas, VA, USA) and maintained in our laboratory by serial passage in BALB/c mice (Zhang et al., 2016).
Antibiotic Administration in Mice
After the acclimation, the mice were treated with the antibiotic cocktail by intraperitoneal injection and drinking water according to a previous study with some modifications (Rodrigues et al., 2017). The control mice received only filtered water, following the same regimen. The antibiotic cocktail (1.5 g/L ampicillin, 1.5 g/L metronidazole, 1.5 g/L neomycin trisulfate, and 1 g/L vancomycin) was administered in drinking water and 100 µL of antibiotic cocktail was intraperitoneally injected at the concentration described above once each day. After about 2 weeks, nymphs were placed to feed on the mice, and the mice continued to be treated with the same antibiotic or water control administration described above until the ticks detached. Each group consisted of five mice per experiment.
Collection of H. longicornis Adults
Each mouse was collared with a plastic tube with central drilling to prevent grooming and then ticks were allowed to feed to repletion on infected mice. About 35 nymphs fed to repletion on each mouse that had been treated with the antibiotic cocktail or control water. The engorged nymphs that detached from mice on the same day were collected. To prevent environmental microbial contamination, the collected ticks were surfaced sterilized with 70% ethanol twice, followed by three washes with antibiotic solution (200 µg/mL streptomycin, 200 U/mL penicillin, and 0.5 µg/mL amphotericin B), and three washes with sterilized water in a Laminar Flow Biosafety Cabinet according to the previous study with some modifications (Narasimhan et al., 2014; Swei and Kwan, 2017). They were then wicked free of excess fluid on sterile Whatman paper, placed in sterile tubes, and maintained at 25°C with 90% relative humidity in a dark sterile incubator. After two weeks, the first molted ticks were observed. The adult ticks that molted from nymphs feeding on the antibiotic or control water treated mice were designated AT adults and CT adults, respectively, in this study.
Tick Samples Preparation and DNA Extraction
The newly molted adult ticks were collected and surface sterilized as described above to remove any surface microbial contamination. Adult ticks were dissected with sterile blades, and the guts were pooled (8 ticks) and homogenized. The supernatant was collected after centrifugation at 12,000 g for 5 min. The supernatant was used for DNA extraction using a genomic DNA Isolation Mini Kit according to manufacturer instructions (Vazyme Biotech Co., Ltd., China). DNA concentrations and purity were measured via a NanoDrop ND-1000 spectrophotometer (Thermo Fisher Scientific, Waltham, MA, USA). The extracted DNA was divided into two parts and stored at −80°C until further use.
16S rRNA Gene Profile
Sequencing of the 16S rRNA genes was performed using the Illumina MiSeq platform. Universal 16S primers (341F/806R) with a sample-specific 12 bp barcode were used to amplify the hypervariable V3-V4 region of bacterial 16S rRNA genes. PCR was performed using thermal cycler Model C1000 (Bio-Rad, Richmond, CA, USA). Microbiome bioinformatics were performed with QIIME2 2019.4 with slight modification (Bolyen et al., 2019) according to the official tutorials (https://view.qiime2.org/). Briefly, raw sequences were quality filtered, denoised, merged, and chimera filtered using the DADA2 plugin with DADA2 pipeline (Callahan et al., 2016). On average, we obtained 107445 clean reads/sample. Taxa abundances at the ASV levels were statistically compared among groups by MetagenomeSeq, and visualized as Manhattan plots (Zgadzaj et al., 2016). LEfSe (Linear discriminant analysis effect size) was performed to detect differentially abundant taxa across groups using the default parameters (Segata et al., 2011).
Acquisition of B. microti by H. longicornis Nymphs
Blood containing B. microti-infected erythrocytes was collected from the mice infected with B. microti. Thin blood smears with Giemsa staining were used to examine the Babesia parasites in infected red blood cells (RBCs). About 2 weeks after the antibiotic cocktail or control water treatment, each mouse was inoculated intraperitoneally with 100 µL of B. microti positive blood. The parasitemia density of each mouse was monitored daily, and 35 nymph ticks were applied to feed on each mouse with B. microti parasitemia of 20%. The engorged nymphs were collected and surfaced sterilized as described above. The engorged nymphs from each treated group were maintained in two different dark sterile incubators until they molted into adult ticks. Fourteen days later, the first molted tick was observed.
Detection of B. microti Infection in Adult Ticks
DNA was extracted from each adult tick using the method described above. Negative controls (blank samples) were used during DNA extraction. The B. microti infection in adult ticks was tested by qPCR targeting 18S rRNA gene to detect B. microti according to the previously reported method (Wu et al., 2017). Briefly, qPCR was performed to quantify B. microti using absolute quantification under conditions 2 min at 37°C, 30 s at 95°C, 40 cycles of 95°C for 10 s, and 60°C for 30s. A B. microti–positive control constructed by cloning the 429-bp PCR amplicon into a pMD18-T plasmid was used to run standard curve. All qPCR amplifications were performed in triplicate on a QuantStudio 5 Real-Time PCR machine (Applied Biosystems, Foster City, CA, USA). The 20-μl PCR mixture contained 10 μl of 2 × Probe Master Mix (QN114, Vazyme Biotech Co., Ltd., China), 1 μl of each primer, and 0.5 μl of probe at 10 μM, 3.5 μl of DNA sample, and 4 μl of water. Forward and reverse primers and probe sequences are as follows: Bm18Sf-AACAGGCATTCGCCTTGAAT, Bm18Sr-CCAACT GCTCCTATTAACCATTACTCT, and Bm18Sp-6FAM-CTA CAGCATGGAATAATGA-MGBNFQ, respectively.
Sectioning and Staining of Adult Ticks
Adult ticks were fed for 48 hours and then dissected in 2.5% glutaraldehyde with sterile blades. The midguts were rinsed and placed in 2.5% glutaraldehyde for one hour at room temperature and overnight at 4°C. Then they were washed in graded alcohol, followed by three washes in xylene, paraffin embedded, and sectioned at about 5-μm thickness. Sections were stained with Periodic acid Schiff base (PAS) and visualized under a light microscope. The thickness of PAS positive PM-like layer was calculated in three different regions in each microscopic filed, and the arithmetic average was determined. At least three fields/section were calculated, and five to eight individual sections were examined in each treated group.
RNAseq Library Preparation and Sequencing
Ticks were dissected and midguts were pooled (eight adult ticks) and homogenized in TRIzol (Invitrogen, USA) according to the method described above. Total RNA for RNA-seq was extracted according to the manufacturer’s instructions. Library preparation was performed using a VAHTS mRNA-seq V3 Library Prep Kit (Illumina, San Diego, CA, USA). PolyA mRNA was enriched from 1 μg of total RNA using magnetic beads with Oligo (dT). Purified mRNA was disrupted into 200 to 300 bp short fragments and primed with random hexamers for cDNA synthesis. cDNA was then subjected to end-repair and dA base addition and then connected with sequencing adapters. Suitable fragments were purified using VAHTS DNA Clean Beads (Illumina) and were selected as templates for PCR amplification. Libraries were validated with an Agilent 2100 Bioanalyzer (Agilent, Santa Clara, CA, USA) to check size distribution. Samples were qualified and pooled by qRT-PCR. Sequencing was done on Illumina novaqseq pe150.
Low-quality reads were filtered and clean reads were mapped to H. longicornis genome using HISAT2 software (http://ccb.jhu.edu/software/hisat2/index.shtml). Differential expression analysis was performed with the DESeq package. Genes with adjusted |log2Fold Change| > 1 and P value<0.05 were considered significantly differentially expressed. KEGG and GO analyses for differentially expressed genes were performed using topGO and clusterProfiler.
Statistical Analysis
The statistical differences between experimental and control groups were determined using GraphPad Prism 8.0 software (GraphPad Software, San Jose, CA, USA). The significant differences between experiment and control groups were analyzed using Student’s t-test or one-way ANOVA with Dunnett’s multiple comparison post-test. A P value < 0.05 was considered significant.
Results
Antibiotic Usage on Mice Changed the Microbiota of the Newly Molted H. longicornis Adults
The host blood meal source can significantly influence tick microbiomes (Swei and Kwan, 2017). Antimicrobial agents and antibiotics are widely used on animal farms to prevent and treat animal diseases. In this study, we tested the effect of antibiotics used on animals on the microbiome diversity of ticks. The tick gut is the first organ to come in contact with pathogens during blood meal uptake. It is a pivotal microbial entry point and determines pathogen colonization, survival, and infectivity (Narasimhan et al., 2014). Ticks can harbor variety of bacteria, especially in their guts. Therefore, in the present study, the gut microbiome difference between the adult ticks from different groups was determined, and the experimental workflow for microbiome analysis is shown in Figure 1A. Proteobacteria, Firmicutes, Actinobacteria, and Gemmatimonadetes dominated the tick gut bacterial community (Figure 1B), and the microbial composition of the guts of AT adults and CT adults showed differences at the phylum and genus levels (Figures 1C, D). The gut microbiota in AT adults was dominated by Acinetobacter and Bacillaceae_Bacillus at the genus level. However, the most abundant genera in the CT adults were Ammoniphilus and Coxiella (Figure 1D).
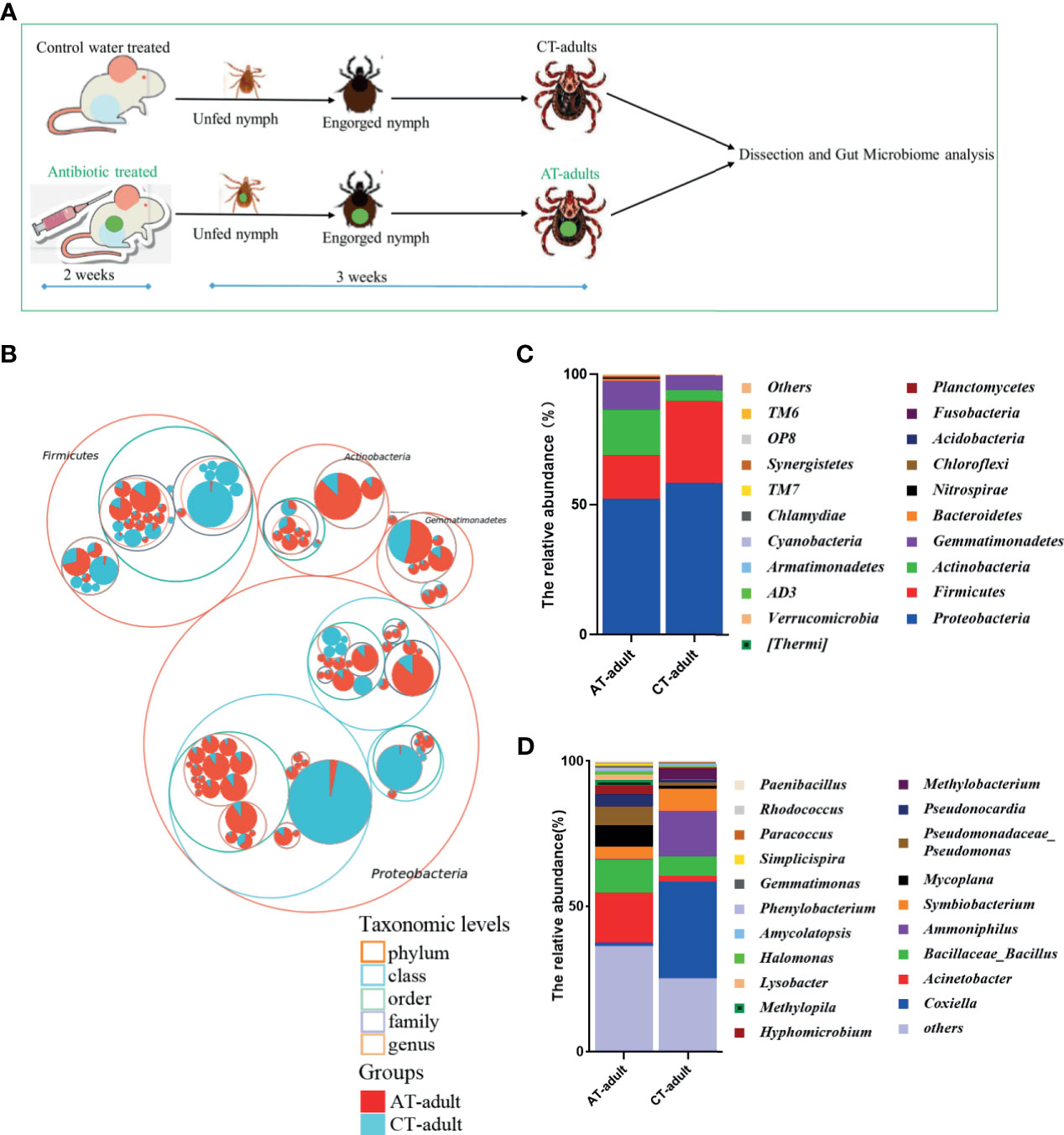
Figure 1 Antibiotics use on mice affects the gut microbiota community of H. longicornis. (A) The experimental workflow for microbiome analysis. Nymphs were fed on an antibiotic cocktail- treated or water control mice to generate AT adults and CT adults, respectively. The 2-week scale represents the time it takes to build an antibiotic-treated mouse model. The 3-week scale represents the time it required to obtain the newly molted adult ticks from the unfed nymphs in this study. The green dot represents perform antibiotic treatment. (B) Taxonomic differences are based on 16S rRNA sequencing. Taxonomic composition visualized by circular packing. The largest circles represent phylum level, and the inner circles represent class, family, and genus. The circle sizes represent the mean relative abundance of the taxa. The taxa were colored by sample groups (red for AT adults and blue for CT adults), whereas the area of the group corresponded to the mean relative abundance of the taxa in each group. (C, D) Total gut bacterial relative abundance at the taxonomic rank of phylum (C) and genus (D) of CT adults and AT adults.
Rarefaction curves showed that a higher bacterial diversity was observed in the guts of AT adults than in the guts of CT adults (Figure 2A and Supplement Table S1). LEfSe analysis showed that Acinetobacter and Coxiella were divided between the AT adult and CT adult groups: Acinetobacter in the AT adult group, and Coxiella in the CT adult group (Figures 2B, C). MetagenomeSeq analysis showed that Nocardioides, Pseudonocardia, Bacillus, Paenibacillus, Nitrospira, Reyranella, Sphingomonas, Ralstonia, Acinetobacter, and Pseudomonas were significantly enriched in the AT adults at the genus level (Figure 2D), and Pseudonocardia, Bifidobacterium, Bacillus, Lactobacillus, Faecalibacterium, Rhodoplanes, Cupriavidus, Massilia, Neisseria, and Coxiella were enriched in the CT adults (Figure 2E). These results showed that antibiotic usage on mice changed the gut microbiota of the newly molted H. longicornis adults, mainly causing an increase in bacterial diversity but a decrease in bacterial abundance. The abundance of Coxiella and Acinetobacter within gut samples showed a significant difference between the two groups (Figure 2C), and there were different iconic species between AT adults and CT adults (Figures 2D, E).
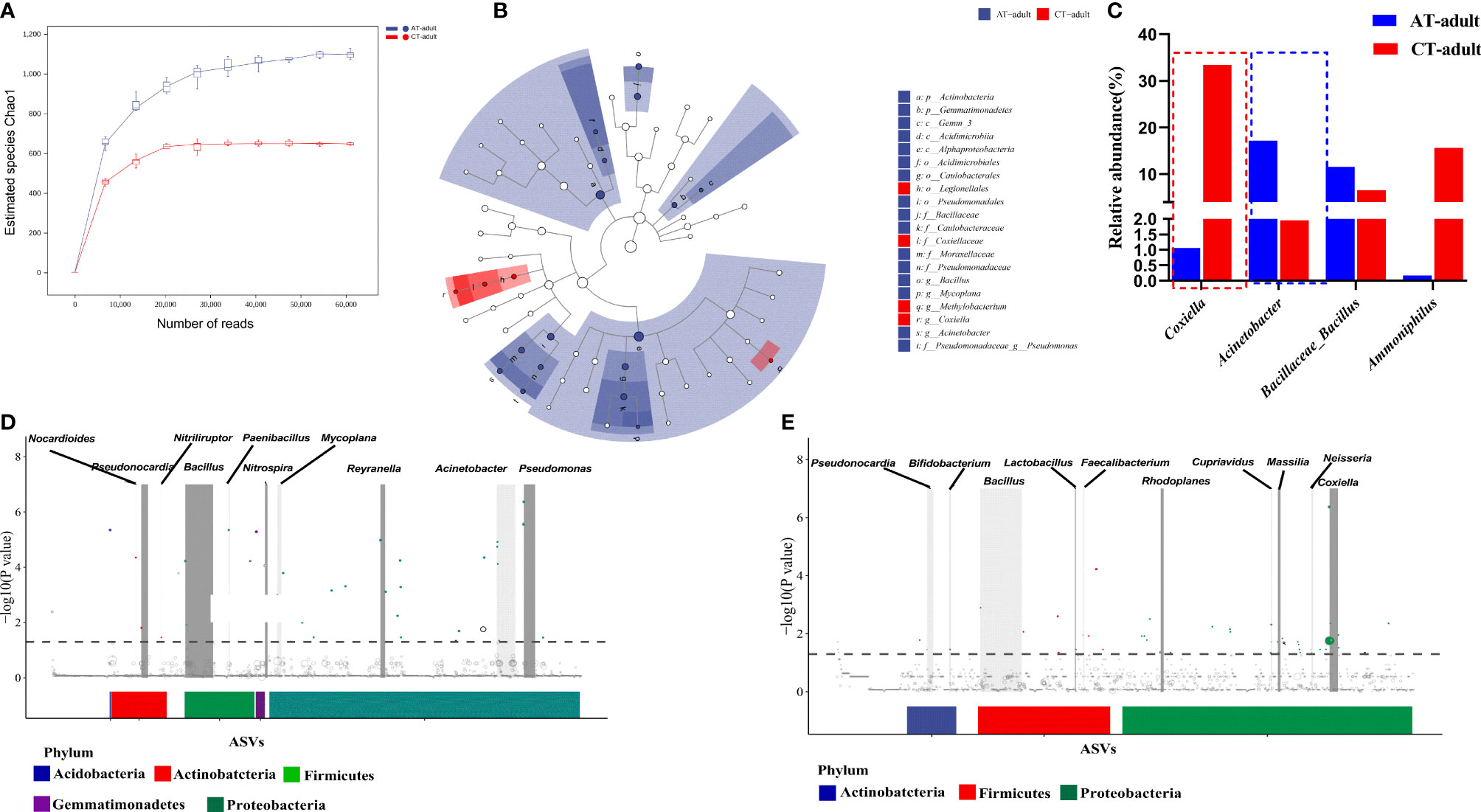
Figure 2 Antibiotics used on mice resulted in tick microbiota dysbiosis. (A) Rarefaction curve of the estimated number of genera using the Chao1 method. (B) Cladogram for taxonomic representation of significant differences between two groups. (C) Percentages of the most abundant genera in AT adults and CT adults. (D, E) Manhattan plots showing the abundance enrichment in the AT adults (D) and CT adults (E). The dashed line represents the P = 0.05 threshold of significance. The color represents the different taxonomic affiliation of the ASVs (phylum level), and the dot size corresponds to their relative abundance in the respective samples. Gray boxes denote the different taxonomic groups (genus level).
Tick Microbiota Dysbiosis Influenced B. microti Transstadial Transmission From Nymphs to Adult Ticks
Nymphal ticks were fed on B. microti-positive mice treated with the antibiotic cocktail or water control, and the nymphs were collected once engorged. The infection in AT adults and CT adults was evaluated by counting the proportion of ticks carrying B. microti genome (prevalence) and the B. microti genome copies of per tick (intensity) (Melendez and Forlano, 1996). The experimental design for the B. microti transstadial transmission analysis is shown in Figure 3A. The AT adults showed a significantly increased B. microti prevalence and infection intensity compared to CT adults, as indicated by qPCR (Figures 3B, C, and Supplement Table S2). A 200-fold increase in B. microti infectivity and 1.5- to 2-fold increase in parasite prevalence occurred in AT adults (Figures 3B, C).
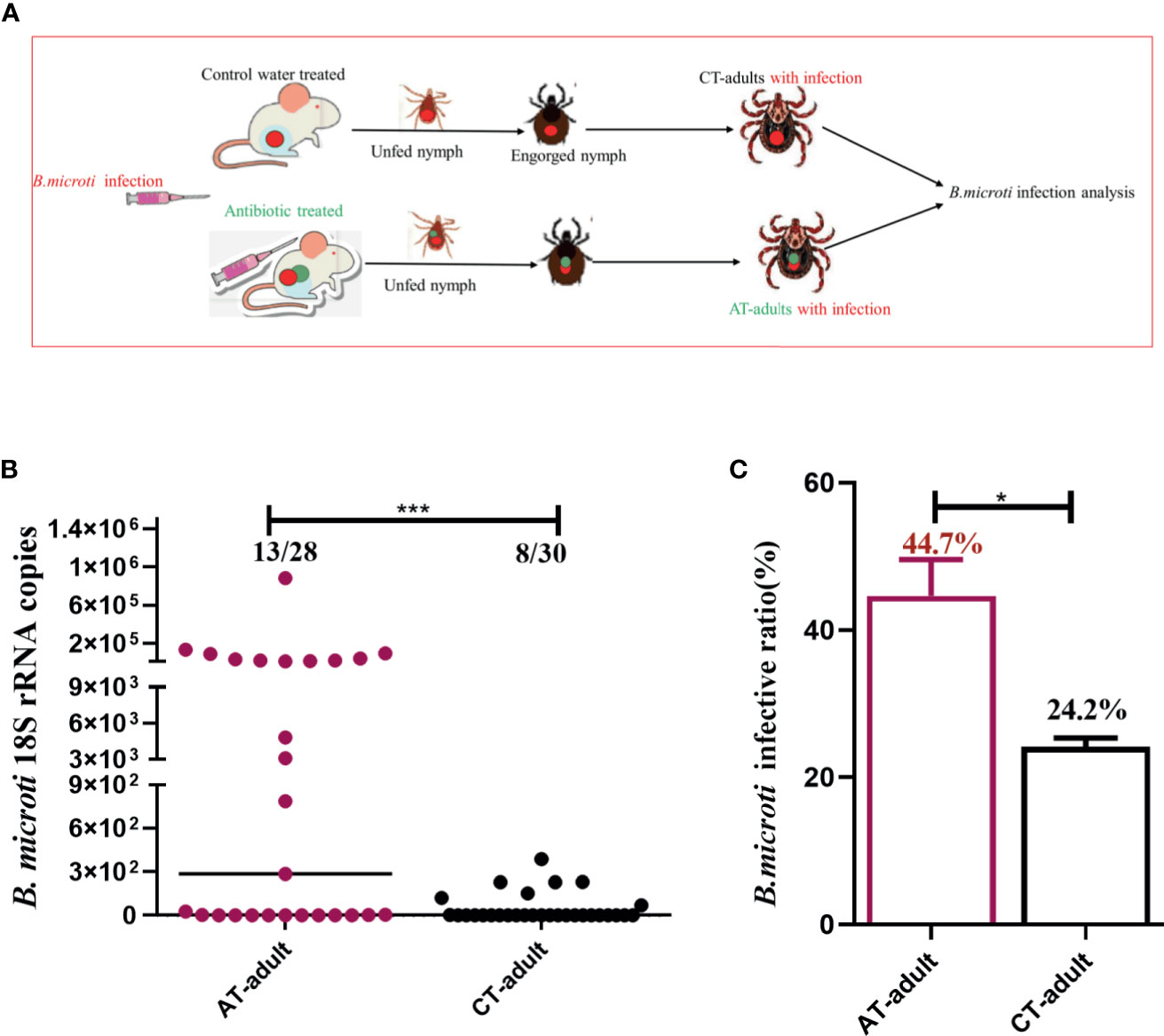
Figure 3 Tick microbiota dysbiosis impairs capacity to transstadially transmit B. microti from nymphs to adult ticks. (A) The experimental workflow for B. microti infection analysis in adult ticks. Nymphs were fed on an antibiotic cocktail-treated or water control mice with B. microti infection to generate AT adults with infection and CT adults with infection, respectively. (B, C) Assessment of the antibiotic-mediated microbiota dysbiosis regulation of tick capacity to transstadially transmit B. microti. * represents p < 0.05, *** means p < 0.001.
To examine the possibility that antibiotic treatment influenced B. microti infection and proliferation within the mice host, the parasitemia density of B. microti in mice at different time points after infestation with ticks was monitored. The parasitemia density of mice on the 0 and 3rd days of tick infestation was similar between the two groups (Supplement Table S3). These results demonstrated that the antibiotic treatment had no influence on B. microti infection in mice, but it did have a significant influence on the transstadial transmission from nymphs to adult ticks.
Tick Microbiota Dysbiosis Altered Tick Gut Morphology
The tick gut is the first organ to come in contact with pathogens. We examined the gross morphology of the tick gut. The peritrophic matrix (PM) is a glycoprotein-rich acellular structure that is located between the gut lumen and the epithelium. The tick PM is considered a barrier to parasite infection and plays an important role in host–bacteria–pathogen interactions. We determined the PM layer of adult ticks (48 h after the start of feeding on mice) using PAS staining. AT adults showed a significant decrease in the thickness of the PM compared with CT adults (Figures 4A, B). B. microti infection resulted in disruption of the PM layer in AT adults but not in CT adults (Figure 4A). These results indicate that tick microbiota dysbiosis induced by antibiotic usage on the mice host altered tick gut morphology. This gut morphology change may help facilitate B. microti transstadial transmission from nymph to adult by modulating the PM layer.
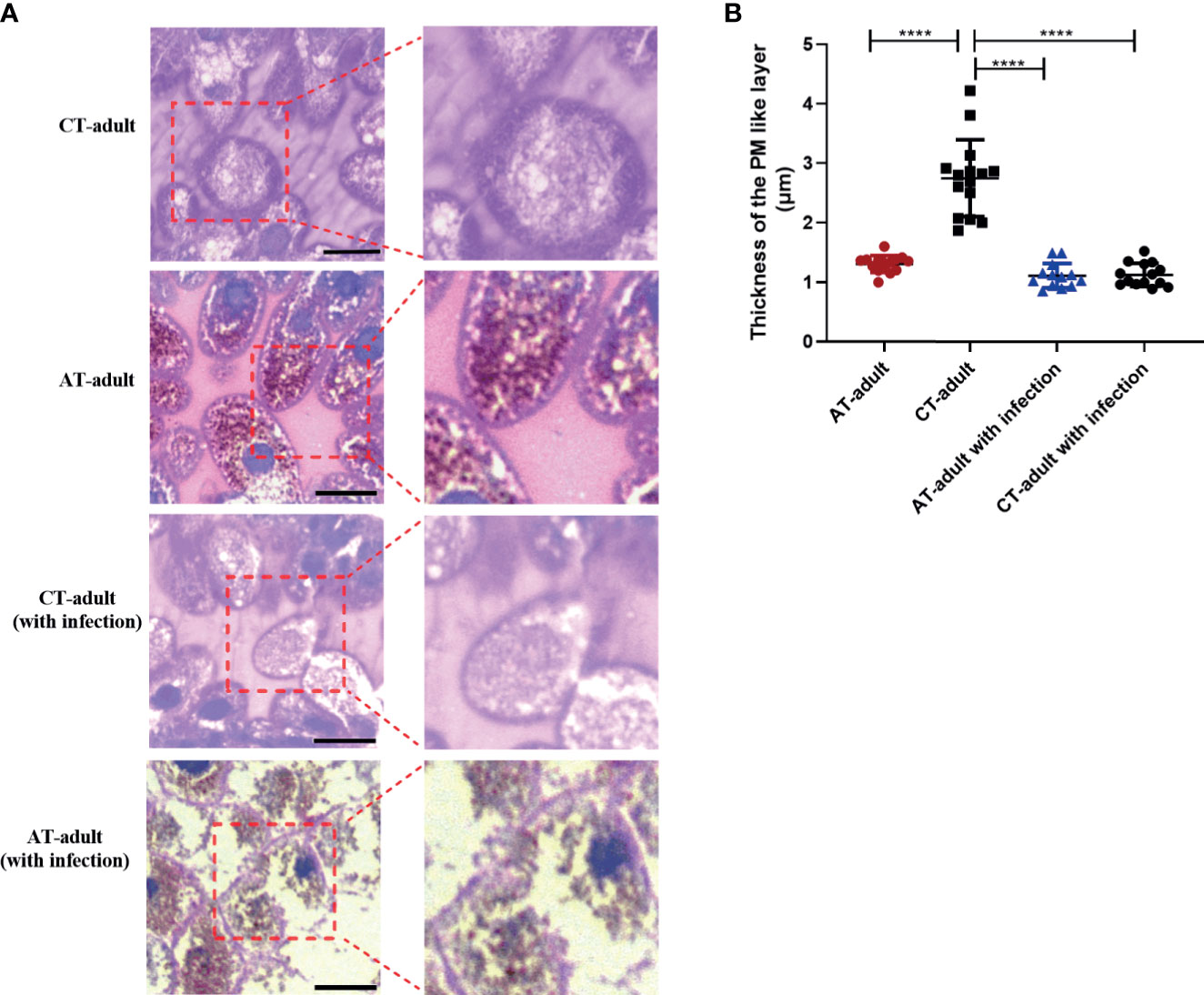
Figure 4 Tick microbiota dysbiosis impairs gut barrier integrity. (A) PAS stain and sectioned 48 h fed guts of AT adults and CT adults (under negative and positive mode). Boxed outlines within the images on the left have been magnified. The scale bar represents 50 μm. (B) Quantification of relative thickness of the PM-like layer of among different groups. **** means p < 0.0001.
Tick Microbiota Dysbiosis Affected the Tick Gut Transcriptional Response
Transstadial transmission, exhibited by tick-borne pathogens, is a major adaptation promoting long-lasting persistence, and this can increase transmission efficiency. The molecular mechanisms that TBP uses to maintain transstadial transmission are not known. We examined the differential gene expression profiles of tick gut between the AT adults and CT adults under B. microti infection. In total, 58 and 219 differentially expressed genes (DEGs) were identified under negative (without B. microti infection) and positive mode (with B. microti infection), respectively, between AT adults and CT adults (Figures 5A, B).
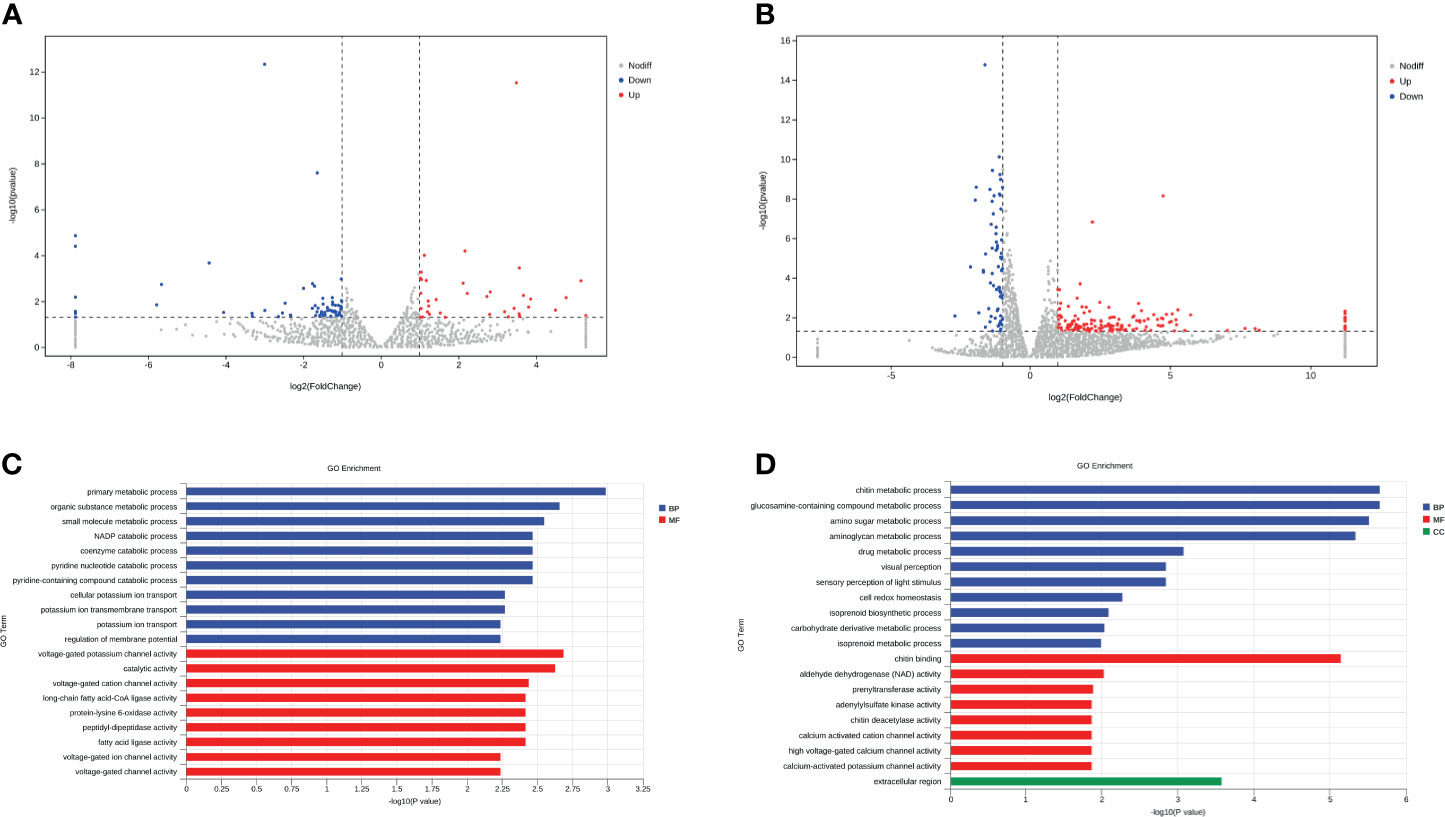
Figure 5 Tick microbiota dysbiosis regulates the gene expression of the tick gut. (A, B) Volcano plots of differentially expressed genes between the AT adults and CT adults under negative mode (A) and positive mode (B). The up-regulated genes and down-regulated genes are shown in red and blue, respectively. (C, D) GO term assignments for DEGs between AT adults and CT adults under negative mode (C) and positive mode (D). The abscissa indicates the number of unigenes in the secondary GO classification, and the ordinate indicates the secondary GO classification.
We found that DEGs between AT adult and CT adult under negative mode are mainly involved in primary metabolic, organic substance metabolic, small molecule metabolic and NADP catabolic process (Biological Process, BP), voltage-gated channel activity, and ligase activity (Molecular Function, MF) (Figure 5C). Babesia enters ticks via multiple mechanisms and alters the tick gut microenvironment and the tick gut transcriptome. The DEGs between AT adults and CT adults under positive mode were mainly involved in chitin metabolic process (BP), chitin binding (MF), and the extracellular region (CC) (Figure 5D and Supplement Table S4). Chitin is a necessary component that helps maintain the barrier function of the PM in the tick gut (Kariu et al., 2013). According to the results of transcriptome and PAS staining, it is possible that this transcriptional response is microbiota dysbiosis induced by either direct or indirect action from specific bacteria. These actions thin or disrupt the PM, and pathogen infection could magnify this effect.
Discussion
In mosquitoes, malaria parasite infection increases if the gut microbiota colonization is hindered by feeding on an antibiotic-treated sugar solution (Dong et al., 2009; Meister et al., 2009). In contrast to mosquitoes, ticks have a long-term and large-volume intake of blood. The tick microbiome can be altered by host blood meal (Swei and Kwan, 2017). Large amounts of antimicrobial agents and antibiotics have been used on animal farms to prevent and treat animal diseases, and antibiotics are widely used to treat tick bites and TBDs, such as Lyme disease, in humans. Our data showed that antibiotic used on a mouse host could alter the microbiome of adult ticks (Figures 1, 2). These results suggest that antibiotics should be used reasonably to avoid the emergence and spread of antibiotic-resistant bacteria (ARB) via arthropods, such as ticks. If possible, antibiotics that do not promote or prevent TBDs transmission should be prioritized. We are currently studying the relationship between ARBs and the blood meal from an antibiotic treated host.
TBDs are increasing, and emerging TBPs are commonly reported. A previous study reported that the blood meal source can influence pathogen transmission (Swei and Kwan, 2017). Only the feeding stage has been examined, not the newly molted stage (Gall et al., 2016; Narasimhan et al., 2017; Swei and Kwan, 2017). Pathogens inhabiting molted unfed adult ticks mainly originate from engorged nymph ticks (similar to vertical transmission in mammals) and are transmitted to a new host during blood feeding. In this study, we investigated the microbiome and transstadial transmission of TBP in newly molted adult ticks. Our findings show that the disruption of the tick microbiome enhances tick-borne B. microti transstadial transmission from nymphs to adult ticks (Figure 3). Adult ticks are readily identified, but nymphs are easily overlooked. Our findings suggest that relatively more effort should be directed to nymph tick stage control to reduce TBD transmission.
Bacterial diversity is increased, but bacterial abundance is decreased in AT adults compared to the CT adults (Figures 2A, C). Coxiella abundance was significantly decreased in AT adults compared to CT adults. The genus Coxiella in ticks includes both symbiotic and pathogenic bacteria (Nardi et al., 2021), and non-pathogenic symbionts may be important in the transmission of TBPs (Bonnet et al., 2017). Li et al. (2018) suggested that Coxiella was likely an important defensive endosymbiont, and TBPs prevalence was decreased, with this being dependent on reduced Coxiella endosymbiont in ticks. We showed that the Coxiella abundance was decreased and B. microti infection increased in AT adults compared to the CT adults, which is consistent with the results of Li et al. However, the correlation between the dysbiosis of Coxiella in ticks and the TBPs transmission needs further study and may lead to a potential biological strategy for TBPs control.
The tick PM is considered to be a barrier to pathogen infection, although many TBPs have evolved to overcome the barrier. We found a thinner PM layer in AT adults compared to CT adults by using PAS staining (Figure 4). The PM is a key regulator of mosquito gut homeostasis and prevents systemic infection of malaria vector mosquitoes (Rodgers et al., 2017). In this study, the tick gut microbiota dysbiosis influenced the thickness of the PM, and the integrity of the PM was seriously disrupted after B. microti infection in the AT adults (Figure 4). The integrity of the I. scapularis PM affected Borrelia burgdorferi colonization (Narasimhan et al., 2014). We found an increase of B. microti infection in AT adults accompanied with a disrupted PM structure. We also compared the PM structure between the AT adults (without infection) and AT adults (with infection) and found that only the thickness but not the integrity of PM was changed in AT adults (without infection), whereas the integrity of PM was disrupted after B. microti infection. These results indicated that antibiotic-induced microbiome dysbiosis may affect B. microti infection by altering the PM structure. Furthermore, we found differential expression of PM-associated genes between AT adults and CT adults using RNA sequencing (Figure 5). According to these, we speculate the antibiotic disrupt the microbial homeostasis in tick midguts, that influence the PM structure in a way that regulates the expression of genes associated with PM formation, thereby resulting in a greater permissiveness to B. microti. The presence of gut bacteria in mosquitoes is necessary for synthesis of complete type I PM, and microbiota-induced PM prevents systemic infection of bacteria (Rodgers et al., 2017). Oral bacterial infection induced the formation of adult Drosophila PM, whose presence protects against intestinal bacterial infection (Kuraishi et al., 2011). These results suggest that the PM may be an important structure that is regulated by tick dysbiosis to affect B. microti transmission. A limitation here is that the direct association of B. microti and PM is not showed due to the difficulty of isolation of PM and specific bacterial. In the tick I. scapularis, the presence of the gut microbiota has also been shown to be necessary for production of PM proper thickness, with this being dependent on STAT-signalling (Narasimhan et al., 2014). In the mosquito, the disruption of the PM induced a systemic immune response, which is associated with the translocation of bacteria of Enterobacteriaceae into the body cavity (Rodgers et al., 2017). According to these reports and our present data, we speculate it is possible that tick innate immunity maybe altered due to the change of certain bacteria, and this alteration may infulence the PM structure. However, given limitations of some experimens (including the isolation and identification of specific strains, the blood feeding of ticks in vitro), further investigation is needed to examine how tick immunity induced by the specific bacterial modulate PM using other substitute method.
Conclusions
Antibiotics usage in vertebrate hosts can lead to microbiota dysbiosis in ticks using these hosts for a blood meal. This dysbiosis can promote nymph-to-adult transstadial transmission of B. microti in H. longicornis. Our results indicated that PM maybe a pivotal structure which is regulated by tick dysbiosis to affect B. microti transstadial transmission. More studies are needed to observe the specific microbial strains in microbiota dysbiosis that influence nymph-to-adult transmission of B. microti and to explore the potentially safe and effective biological control strategies.
Data Availability Statement
The data presented in the study are deposited in the Sequence Read Archive (SRA) repository, accession number (SRP322057 and SRP323180).
Ethics Statement
The animal study was reviewed and approved by the Institutional Animal Care and Use Committee of Shanghai Veterinary Research Institute.
Author Contributions
NW designed the projects, collected and analyzed the data, and drafted the manuscript. YZ and JC helped in the mouse housed experiments. HZ participated in the collection of tick samples. JZ participated in the design of this study and revision the manuscript. All authors contributed to the article and approved the submitted version.
Funding
This work was sponsored by Shanghai Sailing Program (19YF1458600) and the China Postdoctoral Science Foundation (2018M641567).
Conflict of Interest
The authors declare that the research was conducted in the absence of any commercial or financial relationships that could be construed as a potential conflict of interest.
Publisher’s Note
All claims expressed in this article are solely those of the authors and do not necessarily represent those of their affiliated organizations, or those of the publisher, the editors and the reviewers. Any product that may be evaluated in this article, or claim that may be made by its manufacturer, is not guaranteed or endorsed by the publisher.
Acknowledgments
We thank Zhibing Lin, Mengmeng Cai, and Jinmiao Lu for their help and support. We thank Accdon (www. accdon.com) for linguistic assistance during manuscript preparation.
Supplementary Material
The Supplementary Material for this article can be found online at: https://www.frontiersin.org/articles/10.3389/fcimb.2021.713466/full#supplementary-material
References
Abraham, N. M., Liu, L., Jutras, B. L., Yadav, A. K., Narasimhan, S., Gopalakrishnan, V., et al (2017). Pathogen-Mediated Manipulation of Arthropod Microbiota to Promote Infection. Proc. Natl. Acad. Sci. U. S. A. 114, E781–EE90. doi: 10.1073/pnas.1613422114
Bolyen, E., Rideout, J. R., Dillon, M. R., Bokulich, N., Abnet, C. C., Al-Ghalith, G. A., et al (2019). Reproducible, Interactive, Scalable and Extensible Microbiome Data Science Using QIIME 2. Nat. Biotechnol. 37, 852–857. doi: 10.1038/s41587-019-0209-9
Bonnet, S. I., Binetruy, F., Hernandez-Jarguin, A. M., Duron, O. (2017). The Tick Microbiome: Why Non-Pathogenic Microorganisms Matter in Tick Biology and Pathogen Transmission. Front. Cell Infect. Microbiol. 7:236. doi: 10.3389/fcimb.2017.00236
Callahan, B. J., McMurdie, P. J., Rosen, M. J., Han, A. W., Johnson, A. J., Holmes, S. P. (2016). DADA2: High-Resolution Sample Inference From Illumina Amplicon Data. Nat. Methods 13, 581–583. doi: 10.1038/nmeth.3869
Dong, Y., Manfredini, F., Dimopoulos, G. (2009). Implication of the Mosquito Midgut Microbiota in the Defense Against Malaria Parasites. PloS Pathog. 5, e1000423. doi: 10.1371/journal.ppat.1000423
Gall, C. A., Reif, K. E., Scoles, G. A., Mason, K. L., Mousel, M., Noh, S. M., et al (2016). The Bacterial Microbiome of Dermacentor Andersoni Ticks Influences Pathogen Susceptibility. ISME J. 10, 1846–1855. doi: 10.1038/ismej.2015.266
Kariu, T., Smith, A., Yang, X. L., Pal, U. (2013). A Chitin Deacetylase-Like Protein is a Predominant Constituent of Tick Peritrophic Membrane That Influences the Persistence of Lyme Disease Pathogens Within the Vector. PloS One 8, e78376. doi: 10.1371/journal.pone.0078376
Kuraishi, T., Binggeli, O., Opota, O., Buchon, N., Lemaitre, B. (2011). Genetic Evidence for a Protective Role of the Peritrophic Matrix Against Intestinal Bacterial Infection in Drosophila Melanogaster. Proc. Natl. Acad. Sci. U. S. A. 108, 15966–15971. doi: 10.1073/pnas.1105994108
Li, L. H., Zhang, Y., Zhu, D., Zhou, X. N. (2018). Endosymbionts Alter Larva-to-Nymph Transstadial Transmission of Babesia Microti in Rhipicephalus Haemaphysaloides Ticks. Front. Microbiol. 9, 1415. doi: 10.3389/fmicb.2018.01415
Meister, S., Agianian, B., Turlure, F., Relogio, A., Morlais, I., Kafatos, F. C., et al (2009). Anopheles Gambiae PGRPLC-Mediated Defense Against Bacteria Modulates Infections With Malaria Parasites. PloS Pathog. 5, e1000542. doi: 10.1371/journal.ppat.1000542
Melendez, R. D., Forlano, M. (1996). Incidence and Intensity of Babesia Spp. Sporokinetes in Engorged Boophilus Microplus From a Dairy Herd in Venezuela. Ann. N. Y. Acad. Sci. 791, 148–156. doi: 10.1111/j.1749-6632.1996.tb53521.x
Narasimhan, S., Rajeevan, N., Liu, L., Zhao, Y. O., Heisig, J., Pan, J., et al (2014). Gut Microbiota of the Tick Vector Ixodes Scapularis Modulate Colonization of the Lyme Disease Spirochete. Cell Host Microbe 15, 58–71. doi: 10.1016/j.chom.2013.12.001
Narasimhan, S., Schuijt, T. J., Abraham, N. M., Rajeevan, N., Coumou, J., Graham, M., et al (2017). Modulation of the Tick Gut Milieu by a Secreted Tick Protein Favors Borrelia Burgdorferi Colonization. Nat. Commun. 8, 184. doi: 10.1038/s41467-017-00208-0
Nardi, T., Olivieri, E., Kariuki, E., Sassera, D., Castelli, M. (2021). Sequence of a Coxiella Endosymbiont of the Tick Amblyomma Nuttalli Suggests a Pattern of Convergent Genome Reduction in the Coxiella Genus. Genome Biol. Evol. 13. doi: 10.1093/gbe/evaa253
Ord, R. L., Lobo, C. A. (2015). Human Babesiosis: Pathogens, Prevalence, Diagnosis and Treatment. Curr. Clin. Microbiol. Rep. 2, 173–181. doi: 10.1007/s40588-015-0025-z
Rodgers, F. H., Gendrin, M., Wyer, C. A. S., Christophides, G. K. (2017). Microbiota-Induced Peritrophic Matrix Regulates Midgut Homeostasis and Prevents Systemic Infection of Malaria Vector Mosquitoes. PloS Pathog. 13, e1006391. doi: 10.1371/journal.ppat.1006391
Rodrigues, R. R., Greer, R. L., Dong, X. X., DSouza, K. N., Gurung, M., Wu, J. Y., et al (2017). Antibiotic-Induced Alterations in Gut Microbiota are Associated With Changes in Glucose Metabolism in Healthy Mice. Front. Microbiol. 8, 2306. doi: 10.3389/fmicb.2017.02306
Ross, B. D., Hayes, B., Radey, M. C., Lee, X., Josek, T., Bjork, J., et al (2018). Ixodes Scapularis Does Not Harbor a Stable Midgut Microbiome. ISME J. 12, 2596–2607. doi: 10.1038/s41396-018-0161-6
Segata, N., Izard, J., Waldron, L., Gevers, D., Miropolsky, L., Garrett, W. S., et al (2011). Metagenomic Biomarker Discovery and Explanation. Genome Biol. 12, R60. doi: 10.1186/gb-2011-12-6-r60
Swei, A., Kwan, J. Y. (2017). Tick Microbiome and Pathogen Acquisition Altered by Host Blood Meal. ISME J. 11, 813–816. doi: 10.1038/ismej.2016.152
Tanne, J. H. (2018). New Tick Seen in Nine US States is an Emerging Disease Threat, Warns CDC. Bmj British Med. J. 363, k5191. doi: 10.1136/bmj.k5191
Vannier, E., Krause, P. J. (2012). Human Babesiosis. N. Engl. J. Med. 366, 2397–2407. doi: 10.1056/NEJMra1202018
World Health Organization (2017). Critically Important Antimicrobials for Human Medicine (Switzerland: Geneva, Switzerland).
Wu, J., Cao, J., Zhou, Y., Zhang, H., Gong, H., Zhou, J. (2017). Evaluation on Infectivity of Babesia Microti to Domestic Animals and Ticks Outside the Ixodes Genus. Front. Microbiol. 8, 1915. doi: 10.3389/fmicb.2017.01915
Zgadzaj, R., Garrido-Oter, R., Jensen, D. B., Koprivova, A., Schulze-Lefert, P., Radutoiu, S. (2016). Root Nodule Symbiosis in Lotus Japonicus Drives the Establishment of Distinctive Rhizosphere, Root, and Nodule Bacterial Communities. Proc. Natl. Acad. Sci. U. S. A. 113, E7996–E8005. doi: 10.1073/pnas.1616564113
Keywords: Haemaphysalis longicornis, microbiota dysbiosis, antibiotic usage, Babesia microti, peritrophic matrix
Citation: Wei N, Cao J, Zhang H, Zhou Y and Zhou J (2021) The Tick Microbiota Dysbiosis Promote Tick-Borne Pathogen Transstadial Transmission in a Babesia microti–Infected Mouse Model. Front. Cell. Infect. Microbiol. 11:713466. doi: 10.3389/fcimb.2021.713466
Received: 23 May 2021; Accepted: 09 July 2021;
Published: 03 August 2021.
Edited by:
Julius Lukes, Academy of Sciences of the Czech Republic (ASCR), CzechiaReviewed by:
Ryan Oliver Marino Rego, Academy of Sciences of the Czech Republic (ASCR), CzechiaAlejandro Cabezas-Cruz, Institut National de recherche pour l’agriculture, l’alimentation et l’environnement (INRAE), France
Copyright © 2021 Wei, Cao, Zhang, Zhou and Zhou. This is an open-access article distributed under the terms of the Creative Commons Attribution License (CC BY). The use, distribution or reproduction in other forums is permitted, provided the original author(s) and the copyright owner(s) are credited and that the original publication in this journal is cited, in accordance with accepted academic practice. No use, distribution or reproduction is permitted which does not comply with these terms.
*Correspondence: Jinlin Zhou, jinlinzhou@shvri.ac.cn