Comparative analysis of fungal communities between herbicide-resistant and -susceptible Alopecurus aequalis
- College of Plant Protection, Hunan Agricultural University, Changsha, China
Introduction: Alopecurus aequalis is a grass species invading Chinese canola and wheat fields. An A. aequalis KMN-R population surviving mesosulfuron-methyl treatment with recommended rates was acquired from wheatland. Here, we aimed to confirm the resistance profiles of KMN-R to acetolactate synthetase (ALS) inhibiting herbicides and explore the possible resistance mechanisms to mesosulfuron-methyl in this weed population.
Methods: The dose-response tests performed in our study were used to test the toxicity of A. aequalis to ALS-inhibiting herbicides. Sanger sequencing was used to analyze the ALS gene of mesosulfuron-methyl -resistant and -susceptible A. aequalis. RNA sequencing analysis was used to find candidate genes that may confer metabolic resistance to the mesosulfuron-methyl in resistant A. aequalis population. Mesosulfuron-methyl -resistant and -susceptible A. aequalis populations fungal composition was measured via Illumina MiSeq Sequencing.
Results: Dose-response results indicated that KMN-R population evolved resistance to mesosulfuron-methyl and other tested ALS-inhibiting herbicides. Known resistance-conferring Trp-574-Leu gene mutation in A. aequalis ALS was detected in the KMN-R population. Pretreatment with 4-chloro-7-nitrobenzoxadiazole reversed mesosulfuron-methyl resistance in KMN-R. Glutathione S-transferases (GST) gene GSTZ2 and GSTT3 were highly expressed in KMN-R population. In addition, we evaluated the alpha diversity in A. aequalis, centering on OTU abundance, equality, and multiplicity, and found that the fungal community composition had more unexplained variance between KMN-R and KMN-S A. aequalis. We also observed higher abundances of specific fungi in KMN-R A. aequalis.
Discussion: The results proved that resistance to mesosulfuron-methyl in A. aequalis KMN-R population is probably caused by target site- and non-target site-based relating GST and provided the basis for further research between fungal interaction and herbicide resistance.
1 Introduction
Alopecurus aequalis is an annual winter grass weed widespread in the temperate zone of north earth (Hashim et al., 2012). A. aequalis can germinate across a broad range of environment terms, making it a serious predominant weed in several Chinese wheat and canola fields (Zhao et al., 2018a). Strong stooling of A. aequalis strengthens its competitive capacity with crops, reducing crop productivity (Zhao et al., 2018a). Universal methods practicable for controlling A. aequalis still rely significantly on herbicides, particularly acetolactate synthetase (ALS)-inhibiting herbicides. Plant ALS is a crucial enzyme for branched-chain amino acid biosynthesis (Durner et al., 1991). ALS-inhibiting herbicide has been wildly used to control weeds. Nevertheless, more and more weeds are resistant to ALS-inhibiting herbicides due to their extensive use. So far, ALS-inhibiting herbicide resistance has been reported in 166 weeds (Heap, 2022). Figuring out the resistance mechanisms is significant to develop valid treatment techniques to slow down the weeds’ developing resistance.
Herbicide resistance mechanisms can be divided into two types: target site resistance (TSR) and non-target site resistance (NTSR) (Powles and Yu, 2010). TSR can just simply be confirmed through exploring target site gene mutations or overexpression. For ALS, amino acid changes at eight ALS gene sites (Ala122, Pro197, Ala205, Asp376, Arg377, Trp574, Ser653, and Gly654) evolve ALS-inhibiting herbicide resistance in various weeds (Powles and Yu, 2010; Yu and Powles, 2014b). Different from TSR, NTSR results from mechanisms reducing herbicides to reach the target site (Délye et al., 2013). Metabolic resistance is a predominantly known NTSR so far, though it is still in the process of being studied as related to multiple gene families, such as glutathione S-transferases (GSTs), ATP-binding cassette (ABC) transporters, cytochrome P450 monooxygenases (P450s), and glucosyltransferases (GTs) (Yuan et al., 2007). Recently, metabolic resistance to ALS-inhibiting herbicides was increasingly reported in some weed species, for instance, Echinochloa crus-galli (Riar et al., 2013), Amaranthus tuberculatus (Shergill et al., 2018), Amaranthus palmeri (Nakka et al., 2018), Echinochloa phyllopogon (Iwakami et al., 2014), Bromus rigidus (Owen et al., 2012), Beckmannia syzigachne (Wang et al., 2022), and Myosoton aquaticum (Bai et al., 2019).
Mesosulfuron-methyl is an ALS-inhibiting herbicide commonly applied to control grassy weed. Long-standing usage of mesosulfuron-methyl makes it less effective. Lately, mesosulfuron-methyl susceptibility in A. aequalis also decreased (Guo et al., 2015b). As documented, known TSR mutation sites of ALS in A. aequalis, such as Pro-197-Arg/Ser/Tyr/Thr and Trp-574-Leu, responsible for mesosulfuron-methyl resistance, have been characterized (Guo et al., 2015a; Xia et al., 2015; Guo et al., 2018; Zhao et al., 2019b). Although metabolic resistance has been recognized in mesosulfuron-methyl-resistant A. aequalis, identification of resistance-related metabolic enzyme gene has proceeded slowly. Three CYP450 contigs (CYP71A4, CYP94A1, and CYP709C56), one GST contig (GSTU1), and one GT contig in A. aequalis were reported to be involved in mesosulfuron-methyl resistance (Zhao et al., 2019a; Zhao et al., 2019b; Zhao et al., 2022), and only the CYP709C56 was explicitly identified to have considerable effect, endowing metabolic herbicide resistance in A. aequalis (Zhao et al., 2022).
Plants form mutualistic symbioses with fungi in natural systems (Rodriguez and Redman, 2008). Adaptability benefit given by fungi expressing mutualistic lifestyles contained abiotic and biotic stress tolerances, enhanced reproduction, and promoted growth (Yan et al., 2019; Ganie et al., 2022). Plants under stress conditions will employ a “cry for help” strategy, producing “chemical messengers” to activate microbial endophytes to alleviate the stress-induced damage (Liu et al., 2020a). Fungi, such as Epichloë coenophiala in Lolium arundinaceum and Epichloë in Hordeum brevisubulatum, are capable of tolerating abiotic stress (Dinkins et al., 2017; Chen et al., 2021). Currently, in weeds, only endophytes in Polypogon fugax enhance quizalofop-p-ethyl resistance (Liu et al., 2020b). Bioactive secondary metabolites, particularly bio-synthesizing using fungi, have been found to be promising new compounds to exploit in agriculture for use in controlling weeds (Macías-Rubalcava and Garrido-Santos, 2022).
In 2019, farmers in Anhui province discovered that the mesosulfuron-methyl-recommended rate cannot control A. aequalis in the wheat field. Thus, the objectives of the present study were to 1) confirm the resistance level to mesosulfuron-methyl in putative resistant A. aequalis populations; 2) investigate the TSR mechanism in mesosulfuron-methyl-resistant A. aequalis populations; 3) investigate the NTSR mechanisms in mesosulfuron-methyl-resistant A. aequalis populations; and 4) investigate and quantify the fungi between the mesosulfuron-methyl-resistant and -susceptible A. aequalis populations.
2 Materials and methods
2.1 Plant material and chemicals
The resistant A. aequalis population (KMN-R) was gathered in the Chinese Anhui wheat field where mesosulfuron-methyl was applied for at least 5 years. The susceptible population (KMN-S) was gathered from the vacant field where mesosulfuron-methyl was never applied. Seeds from 60 mature seedlings were gathered stochastically manually in each population. All collected seeds were dried and preserved in plastic bags until use.
Herbicides applied in the response test were shown in Table S1. GST inhibitor 4-chloro-7-nitrobenzoxadiazole (NBD-Cl, 97%) and cytochrome P450 inhibitor malathion (95%) were bought from Sigma.
2.2. Whole plant response to acetolactate synthetase-inhibiting herbicides
About 30 A. aequalis seeds were seeded in each pot containing nutrient soil, and the pot was moved to artificial climate chambers with a 14-h day of 20°C and 10-h night of 15°C at 75% humidity. After growing for 4 weeks, seedlings were thinned to 20 in each pot and sprayed with tested ALS-inhibiting herbicides (Table S1) and returned to the greenhouse. After treating for 3 weeks, aboveground seedlings were cut to determine the fresh weight.
2.3 Cloning and sequencing of A. aequalis ALS genes
Fresh leaf tissue (100 mg) in each plant was used to extract genomic DNA (gDNA) using a Tiangen Biotech Co. DNA Kit with instructions. Primers (Table S2) were designed and used to amplify A. aequalis ALS gene fragment involving all known ALS-inhibiting herbicide resistance-related mutations. Polymerase chain reaction (PCR) was conducted, and the PCR run was set as described (Wang et al., 2022). Amplified PCR products were also purified, cloned, and sequenced as described (Wang et al., 2022). Ten plants randomly selected from each population were used for sequencing. Sequences were aligned using BioEdit sequence alignment editor software and DNAMAN.
2.4 Effects of glutathione S-transferases and cytochrome P450 inhibition on the resistance to mesosulfuron-methyl
Seeds from A. aequalis populations were cultured by following the same method as above. A. aequalis seedlings were sprayed with mesosulfuron-methyl, NBD-Cl, NBD-Cl plus mesosulfuron-methyl, malathion, and malathion plus mesosulfuron-methyl. NBD-Cl (270 g a.i. ha-1) was used 48 h before mesosulfuron-methyl treatment, and malathion (1,000 g a.i. ha-1) at 2 h. Mesosulfuron-methyl was applied following the doses as above. Twenty-one days after mesosulfuron-methyl application, photographs were recorded for growth status and aboveground weight per pot was determined.
2.5 RNA sequencing data
TaKaRa Biotech RNAiso Plus was used to extract total RNA from three KMN-R and three KMN-S seedlings with instructions. NanoPhotometer® spectrophotometer was used to analyze the quantity and quality of total RNA. RNA sequencing (RNA-seq) was performed using Illumina platform to generate paired-end reads. Data from KMN-R and KMN-S seedlings were merged to assemble with Trinity (Grabherr et al., 2011) for generating the reference transcriptome. The clean read was filtered from raw read for downstream analyses, and clean data were mapped to reference for obtaining each gene read count. The gene read count for each transcript was normalized to fragments per kilobase of exon per million fragments mapped (FPKM). The expression level of each gene was estimated using RNASeq by expectation maximization (RSEM) (Li and Dewey, 2011). Differential expression between KMN-R and KMN-S was compared using t-test (p < 0.05). Differentially expressed genes (DEGs) were analyzed for Gene Ontology (GO) enrichment using GOseq R packages (Young et al., 2010).
2.6 Gene expression analysis of NTSR contigs in A. aequalis
The candidate NTSR contig was chosen based on the following criteria: upregulated for >2-fold in KMN-R than KMN-S (p < 0.05) and related to GST, ABC transporter, and GT annotation. Samples provided for RNA-seq were used to validate GST, ABC transporter candidate, and GT contig using quantitative real-time PCR (qRT-PCR). Primers (Table S3) for GST, ABC transporter, and GT contig were designed using an online software (https://sg.idtdna.com/scitools/Applications/RealTimePCR/). The eukaryotic translation initiation factor 4A (eIF4A) gene was used as an internal control in A. aequalis (Zhao et al., 2018b). Only a particular PCR band was amplified in each primer, and the negative control did not amplify anything. The amplified PCR product was sequenced to confirm the anticipated gene. qRT-PCR was performed using ABI-7500 Fast Real-Time PCR System with TaKaRa SYBR® Premix Ex Taq™ kits. Primer efficiencies of all studied genes were 94%–115%. qRT-PCR experiments and expression level analyses were conducted as described (Pan et al., 2019).
2.7 Statistical analyses
Each treatment contained three replicates, and every experiment was repeated twice. Herbicide effective rates reducing 50% plant inhibition in fresh weight (GR50) were calculated using four-parameter log-logistic curve for fitting in SigmaPlot software as described (Wang et al., 2022). Resistance indexes (RIs) were determined as the GR50 of KMN-R divided by the GR50 of KMN-S to indicate resistant levels for the KMN-R population.
2.8 DNA extractions and Illumina MiSeq sequencing preparation in A. aequalis
To remove surface fungi in A. aequalis, sterile water, 70% alcohol, and sodium hypochlorite solution (with 2.5% active Cl-1) were used to separate soil from seedlings, then sterile water was used to wash the seedlings five times, and sterile absorbent papers were used to remove water. Ultimately, seedlings were cut into small fragments using flame-sterilized scalpels and stored at -80°C until use. All procedures were conducted under a sterile environment. Power Soil DNA Isolation Kit was used to extract microbial DNA from 1.0 g A. aequalis (wet weight). Primer pairs ITS1F (5’-CTTGGTCATTTAGAGGAAGTAA-3’) and ITS2R (5’-GCTGCGTTCTTCATCGATGC-3’) were used to generate fungal internal transcribed spacer (ITS) amplicon libraries. PCR was conducted, and the PCR run was set as described (Wang et al., 2019). Amplified PCR products were purified on 2% agarose gel. Illumina MiSeq platform was used to paired-end sequence (2 × 300 bp for fungi) purified amplicons by Majorbio Bio-Pharm Technology Co. Ltd. (Shanghai, China). Data were analyzed using Majorbio I-Sanger Cloud Platform (www.i-sanger.com).
2.9 Bioinformatic processing in A. aequalis
Trimmomatic (Bolger et al., 2014) and FLASH (Magoč and Salzberg, 2011) were used to demultiplex, quality-filter, and merge raw fastq files with the following criteria: (a) Reads cut at any site can receive average quality scores <20 over 50-bp moving windows. (b) Primers used were correctly matched, permitting two nucleotide mismatches, and a read involving a blurred base was removed. (c) Sequences with an overlap of >10 bp were merged on account of the overlapped parts. Then, the remaining distinct sequences were calculated for pairwise distance and created a distance matrix. A 0.03 operational taxonomic unit (OTU) definition (97% sequence similarity cutoff level) was used to cluster OTUs using UPARSE, and OTUs contained multitude unity taxonomy. Singleton was removed from the dataset to minimize the effect of sequencing artifacts (Dickie, 2010). UCHIME (Edgar et al., 2011) was used to identify and remove chimeric sequence. The RDP Classifier algorithm against the UNITE v.7 ITS database (fungi) was used to analyze the taxonomy of ITS sequences with a confidence threshold of 70%.
3 Results
3.1 Dose–response to acetolactate synthetase-inhibiting herbicides
Whole plant response tests showed that the KMN-R population evolved resistance to mesosulfuron-methyl (Table 1). The GR50 value of KMN-R was 131.03 g a.i. ha−1, while the GR50 value of KMN-S was 1.14 g a.i. ha−1. The estimated RI for KMN-R was 114.94-fold by dividing the GR50 value of KMN-R to that of KMN-S, indicating high-level mesosulfuron-methyl resistance. KMN-S was susceptible to five tested ALS-inhibiting herbicides (Figure 1). Compared with KMN-S, the resistant KMN-R endowed resistance to the five ALS-inhibiting herbicides, including rimsulfuron, imazamox, pyroxsulam, bispyribac-sodium, and flucarbazone-sodium (Figure 1).
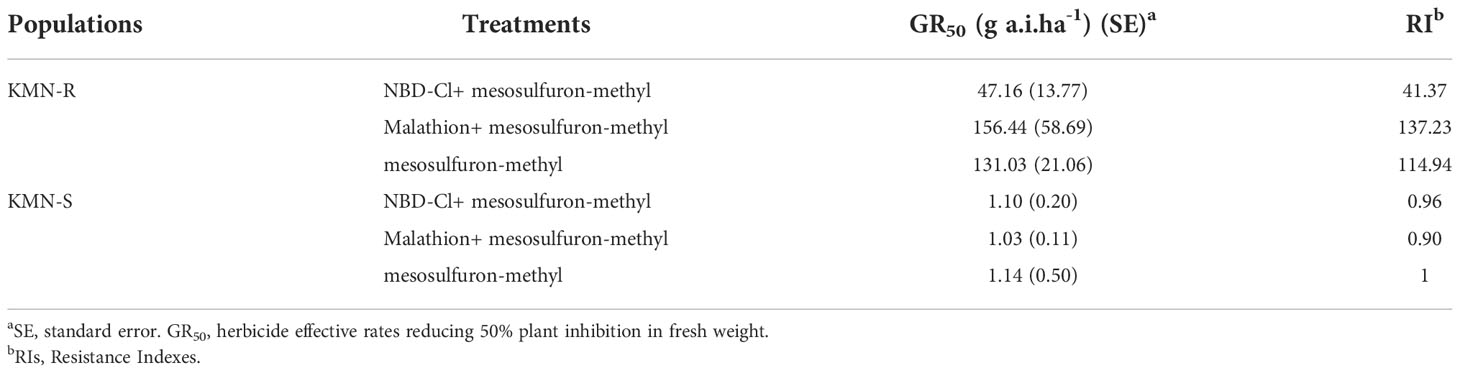
Table 1 Influences of GST inhibitor (NBD-Cl) and cytochrome P450 inhibitor (malathion) on Alopecurus aequalis growth to mesosulfuron-methyl.
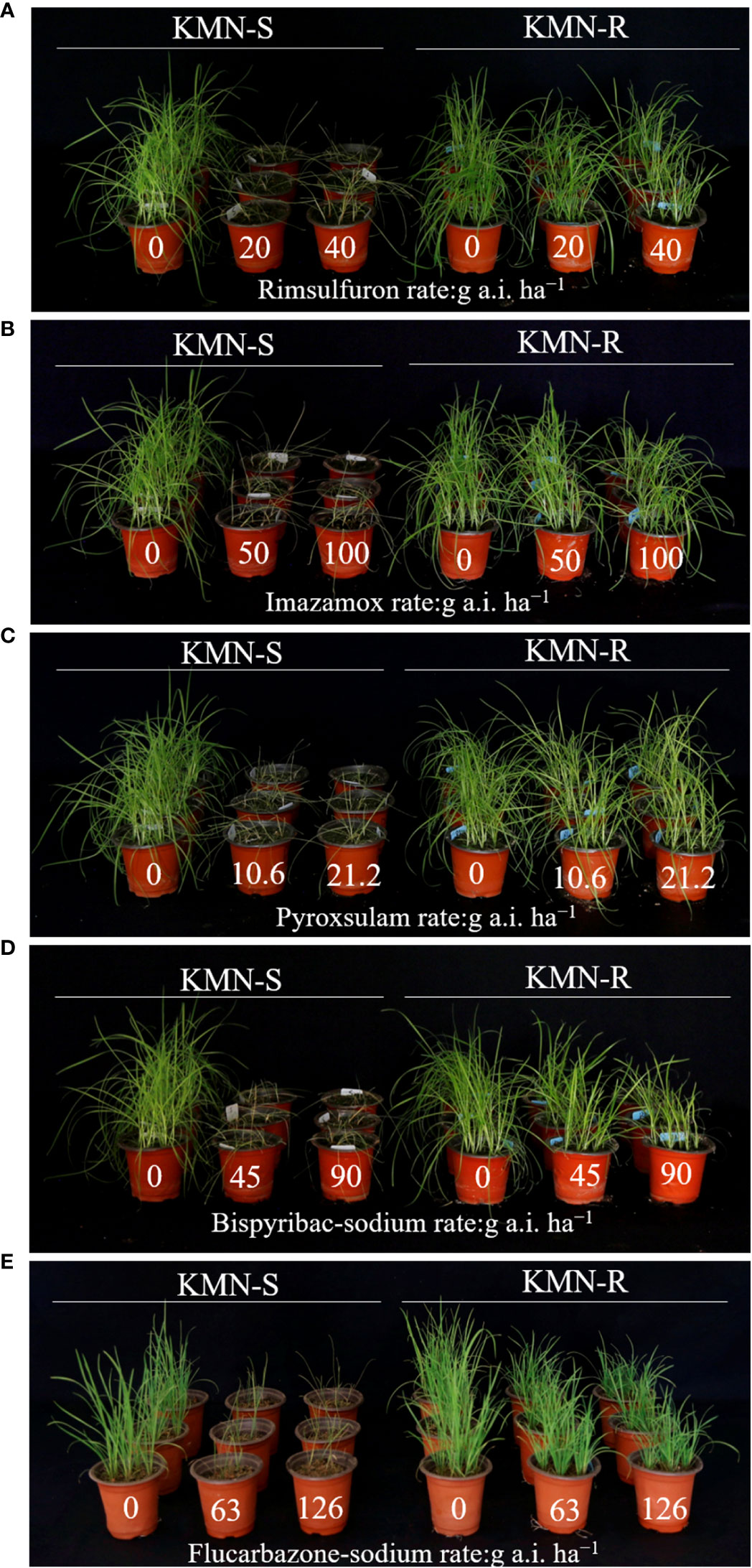
Figure 1 Sensitivities of the susceptible (KMN-S) and resistant (KMN-R) Alopecurus aequalis populations to different ALS-inhibiting herbicides. (A) Rimsulfuron. (B) Imazamox. (C) Pyroxsulam. (D) Bispyribac sodium. (E) Flucarbazone sodium. If seedlings grew well after herbicide application, they were regarded as resistant; if seedlings suffered severe damage or death, they were regarded as sensitive. The numbers mean herbicide application rates (g a.i. ha−1).
3.2 Comparison of ALS gene sequences
The length of the A. aequalis ALS partial gene sequences was 1,917 bp. Sequence alignment between KMN-R and KMN-S samples assumed the TGG to TTG nucleotide variation, causing Trp-574-Leu mutation in KMN-R (Figure 2), confirming that this known resistance-conferring substitution existed in the KMN-R population.
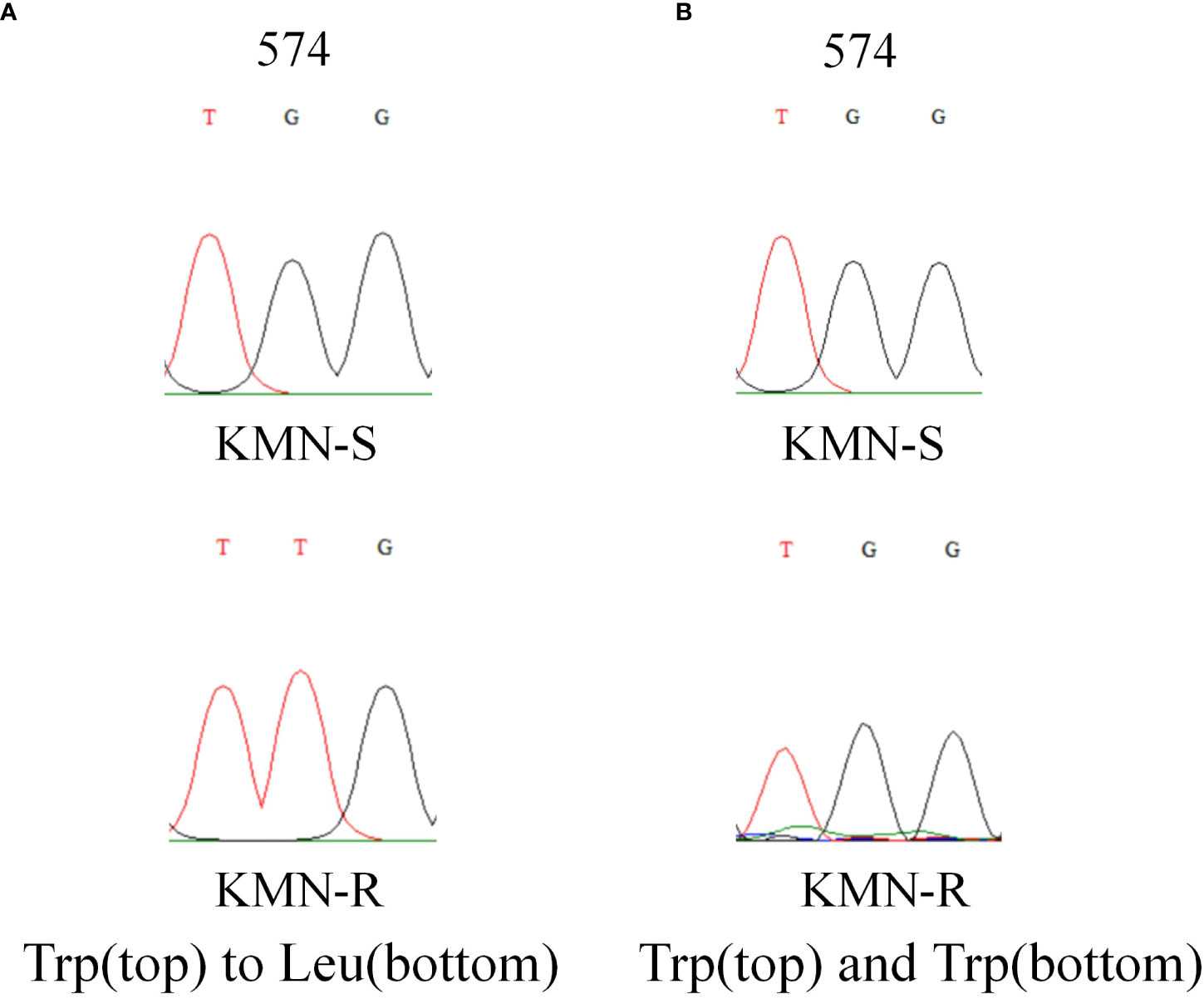
Figure 2 DNA sequencing revealed (A) Trp-574-Leu change in ALS1 and (B) no change in ALS2 in KMN-R Alopecurus aequalis compared with KMN-S. The red and black lines mean the signal intensities of the “T” and “G” bases in Sanger sequencing. ALS1 and ALS2 is the two copies of ALS gene.
3.3 Effects of glutathione S-transferases and cytochrome P450 inhibition on the resistance to mesosulfuron-methyl
Malathion at 1,000 g a.i. ha-1 or NBD-Cl at 270 g a.i. ha-1 did not influence the development of A. aequalis seedlings. NBD-Cl or malathion had no impact on the susceptibility of the plants in susceptible population KMN-S to mesosulfuron-methyl (Table 1). Treatment with malathion did not increase the toxicity of mesosulfuron-methyl to plants in resistant population KMN-R (Table 1). However, treatment with NBD-Cl combined with mesosulfuron-methyl increased mesosulfuron-methyl toxicity to plants in KMN-R (Table 1), with a markedly decreased GR50 value from 131.03 to 47.16 g a.i. ha-1.
3.4 Identification of differentially expressed contigs in A. aequalis
Owing to the absence of the A. aequalis genome, the reference transcriptome in A. aequalis assembled the sequencing data from KMN-R and KMN-S. Then, 36,996 supposed genes with contig N50 size of 1,969 bp were identified from 42,875 transcripts. Assembled sequences were annotated using SwissProt, NCBI non-redundant protein sequences (Nr), protein family (PFAM), clusters of orthologous groups of proteins (KOG), GO, and KEGG ortholog (KO) databases (Table S4). Based on FPKM at t-test (p < 0.05), DEGs in the KMN-R and KMN-S were assessed. A total of 1,484 differentially expressed contigs between KMN-R and KMN-S were confirmed (Figure S1). DEG functions were evaluated using GO enrichment analyses, and metabolic process and cellular process were significantly enriched (Figure S2).
Seven upregulated contigs of >2-fold (p < 0.05) in KMN-R than KMN-S in relation to GST, GT, and ABC transporter annotations were validated using prepared RNA-seq samples (Table 2). Two contigs (TRINITY_DN14492_c0_g1 with a GSTZ2 annotation and TRINITY_DN438_c0_g1 with a GSTT3 annotation) were markedly upregulated using qRT-PCR, fold changes of which displayed no differences with RNA-seq results (Table 2). These two highly expressed GST contigs appear to be involved in mesosulfuron-methyl resistance in KMN-R.
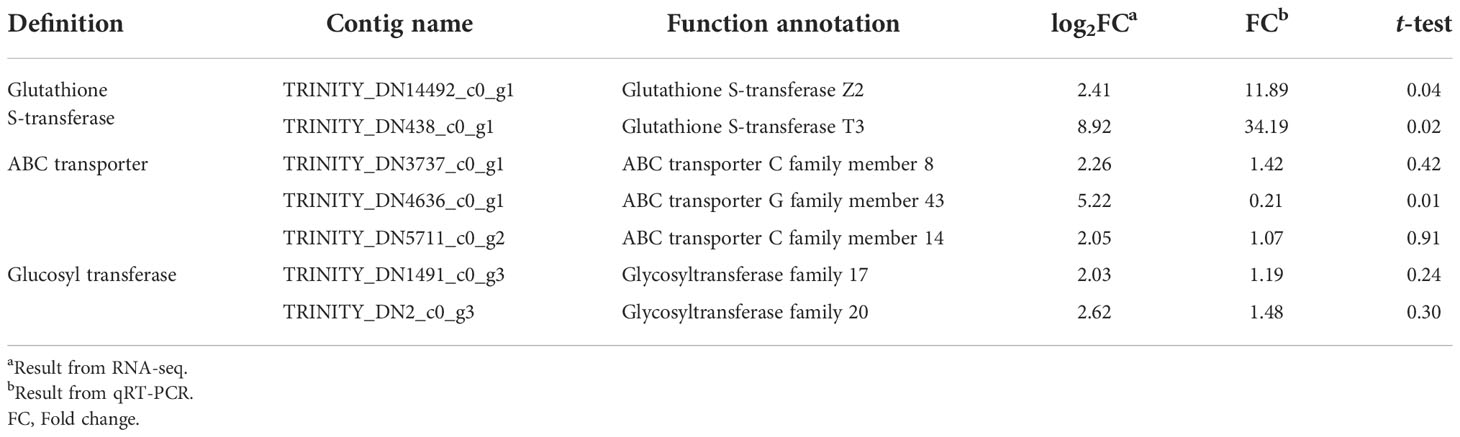
Table 2 Upregulated contigs annotated to metabolism identified by RNA-seq and qRT-PCR in Alopecurus aequalis.
3.5 Richness of fungal species in A. aequalis
A total of 405,386 fungal sequences with 290 bp average length were obtained after filtering and qualifying raw reads. A total of 155 A. aequalis fungal OTUs with 97% similarity were inferred in 5 phyla, 19 classes, 37 orders, 67 families, and 90 genera (Table S5). At the level of the phylum of A. aequalis fungal, the fungal community was dominated by Ascomycota (relative abundance 9.09%), Basidiomycota (relative abundance 3.07%), and Glomeromycota (relative abundance 0.12%) (Figure 3A). At the level of the class of A. aequalis fungal, the community was dominated by Dothideomycetes (relative abundance 2.96%), Sordariomycetes (relative abundance 2.05%), Tremellomycetes (relative abundance 1.69%), Eurotiomycetes (relative abundance 1.50%), and unclassified Ascomycota (relative abundance 1.30%) (Figure 3B). At the level of the family of A. aequalis fungal, the fungal community was dominated by Mycosphaerellaceae (relative abundance 1.77%), unclassified Ascomycota (relative abundance 1.30%), Trichosporonaceae (relative abundance 1.28%), and Nectriaceae (relative abundance 1.15%) (Figure 3C). At the level of the genus of A. aequalis fungal, the fungal community was dominated by unclassified Mycosphaerellaceae (relative abundance 1.77%), unclassified Ascomycota (relative abundance 1.30%), Apiotrichum (relative abundance 1.27%), and Fusarium (relative abundance 1.10%) (Figure 3D).
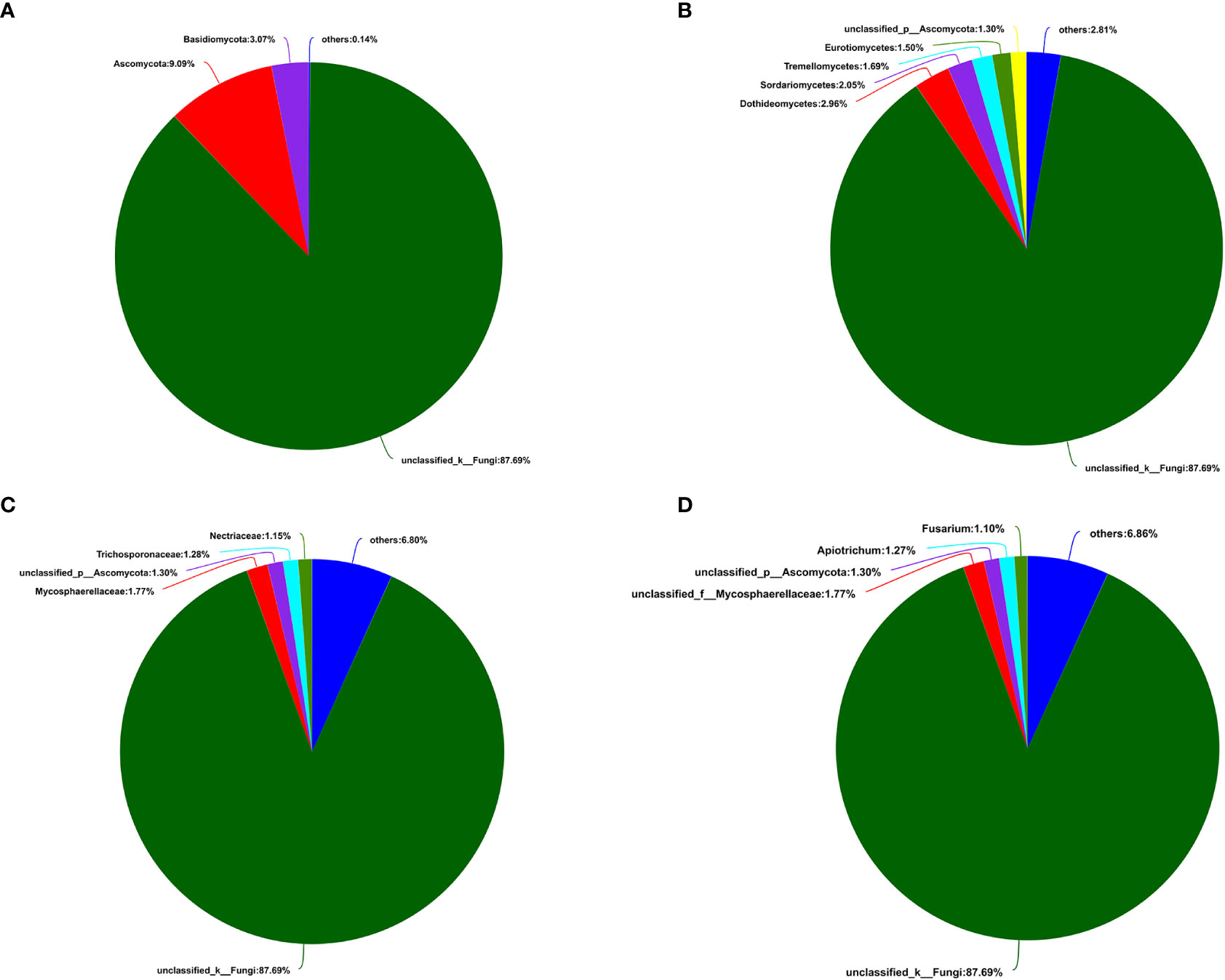
Figure 3 Compositions and relative plenties of fungal endophytes in Alopecurus aequalis on diverse taxonomic levels. (A) Phylum. (B) Class. (C) Family. (D) Genus.
3.6 Diversity of fungal species between KMN-R and KMN-S A. aequalis
The alpha diversity indices (Simpson and Shannon) computed from fungal OTUs of KMN-R and KMN-S A. aequalis populations indicated that less varied fungal OTUs were found in KMN-S than KMN-R (Table S6). At the phylum level of A. aequalis fungal, ANOVA was used to evaluate all detected phyla to test the relative abundance (%) of KMN-R and KMN-S (Figure 4A). Fungal community in KMN-R and KMN-S A. aequalis populations was dominated in four phyla, including Ascomycota, Basidiomycota, and Glomeromycota. It was observed that Ascomycota in KMN-R was significantly more abundant (p < 0.05) than that in KMN-S at the phylum level (Table 3). Particularly, Mortierellomycota was only found with a high relative abundance in the KMN-R A. aequalis.
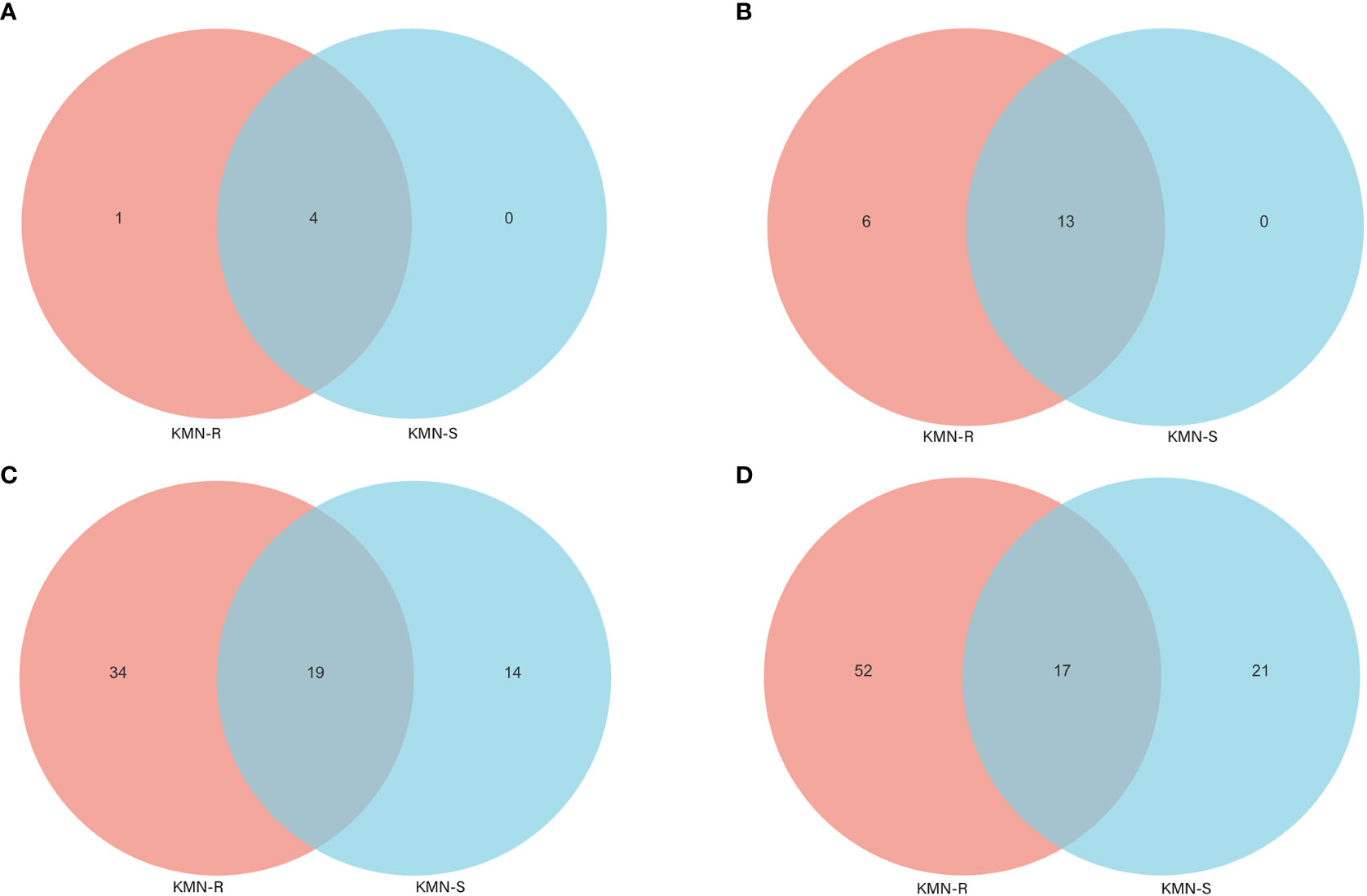
Figure 4 Venn diagram of fungal endophyte communities in KMN-R and KMN-S of Alopecurus aequalis at diverse taxonomic levels. (A) Phylum. (B) Class. (C) Family. (D) Genus.

Table 3 Relative plenties percentage of sequence number in common phyla between KMN-R and KMN-S of Alopecurus aequalis.
At the level of the class of A. aequalis fungal, ANOVA was used to evaluate all detected classes to test the relative abundance (%) of KMN-R and KMN-S (Figure 4B). The fungal community in KMN-R and KMN-S A. aequalis populations was dominated by 13 classes. Significant enrichments (p < 0.05) were observed in Dothideomycetes, Eurotiomycetes, and unclassified Ascomycota between the KMN-R and KMN-S A. aequalis at the class level (Table 4). Specifically for Taphrinomycetes, Lecanoromycetes, Mortierellomycetes, Cystobasidiomycetes, Leotiomycetes, and Orbiliomycetes, we only observed a high relative abundance in the KMN-R A. aequalis (Figure 5A).
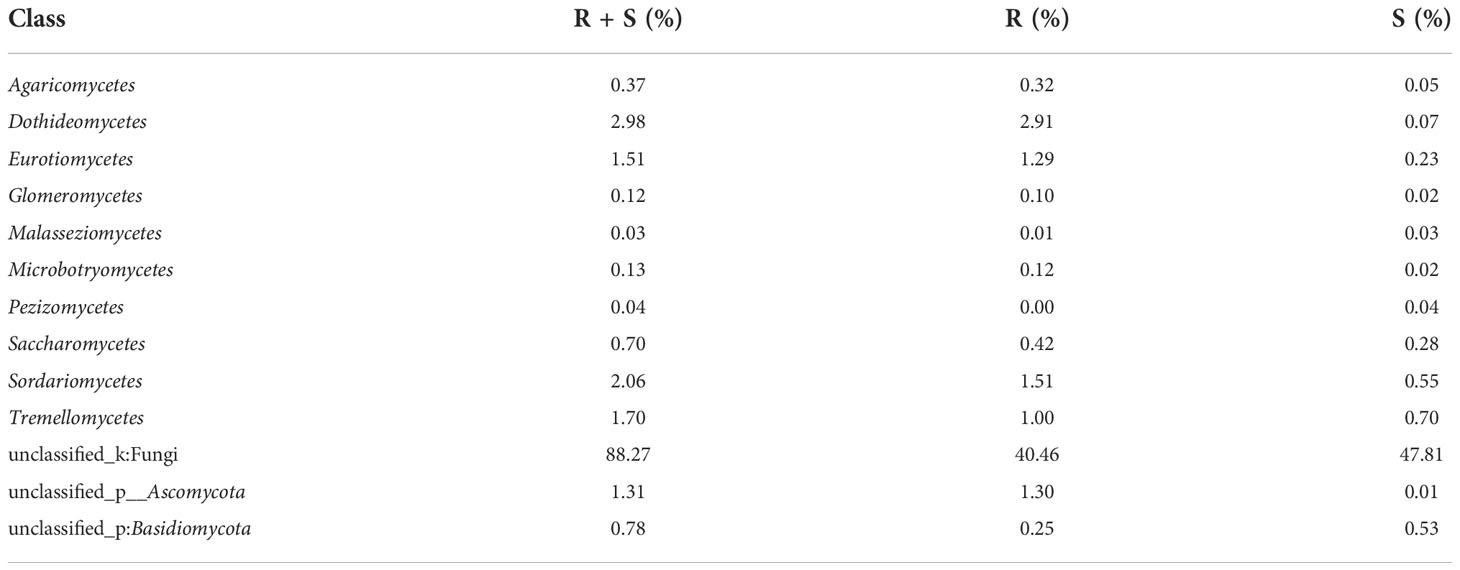
Table 4 Relative plenties percentage of sequence number in common classes between KMN-R and KMN-S of Alopecurus aequalis.
At the level of the family of A. aequalis fungal, ANOVA was used to evaluate all detected families to test the relative abundance (%) of KMN-R and KMN-S (Figure 4C). Fungal community in KMN-R and KMN-S A. aequalis populations was dominated in 19 families. Significant enrichments (p < 0.05) were observed in Mycosphaerellaceae and unclassified Ascomycota between the KMN-R and KMN-S A. aequalis at the family level (Table 5). In addition, 34 families were only observed with a high relative abundance in the KMN-R A. aequalis, including Taphrinaceae, Didymellaceae, and unclassified Eurotiomycetes (Figure 5B), and 14 families were only observed with a high relative abundance in the KMN-S A. aequalis, including Taphrinaceae, Didymellaceae, unclassified Saccharomycetales, Bulleribasidiaceae, and Diatrypaceae (Figure 6A).
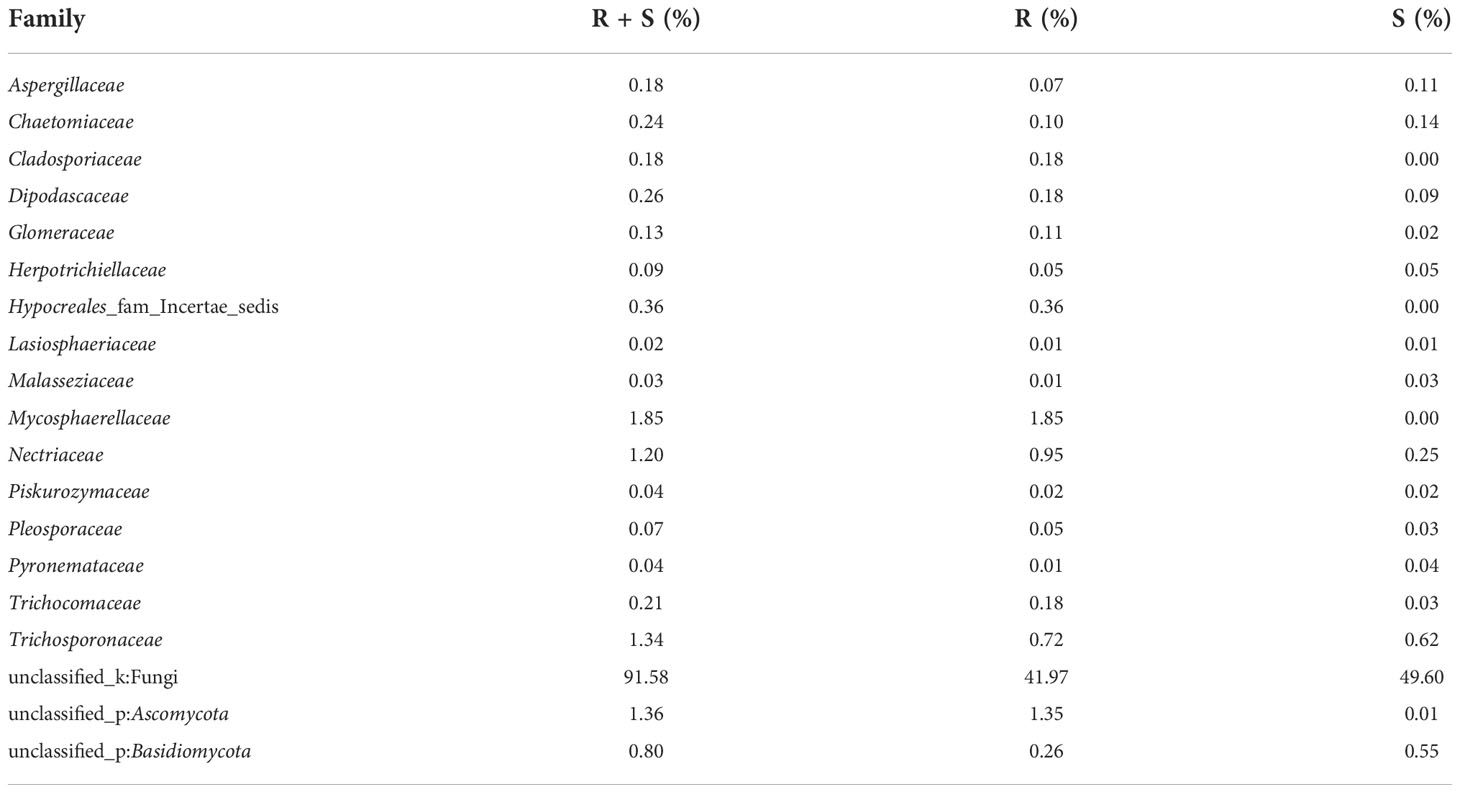
Table 5 Relative plenties percentage of sequence number in common families between KMN-R and KMN-S of Alopecurus aequalis.
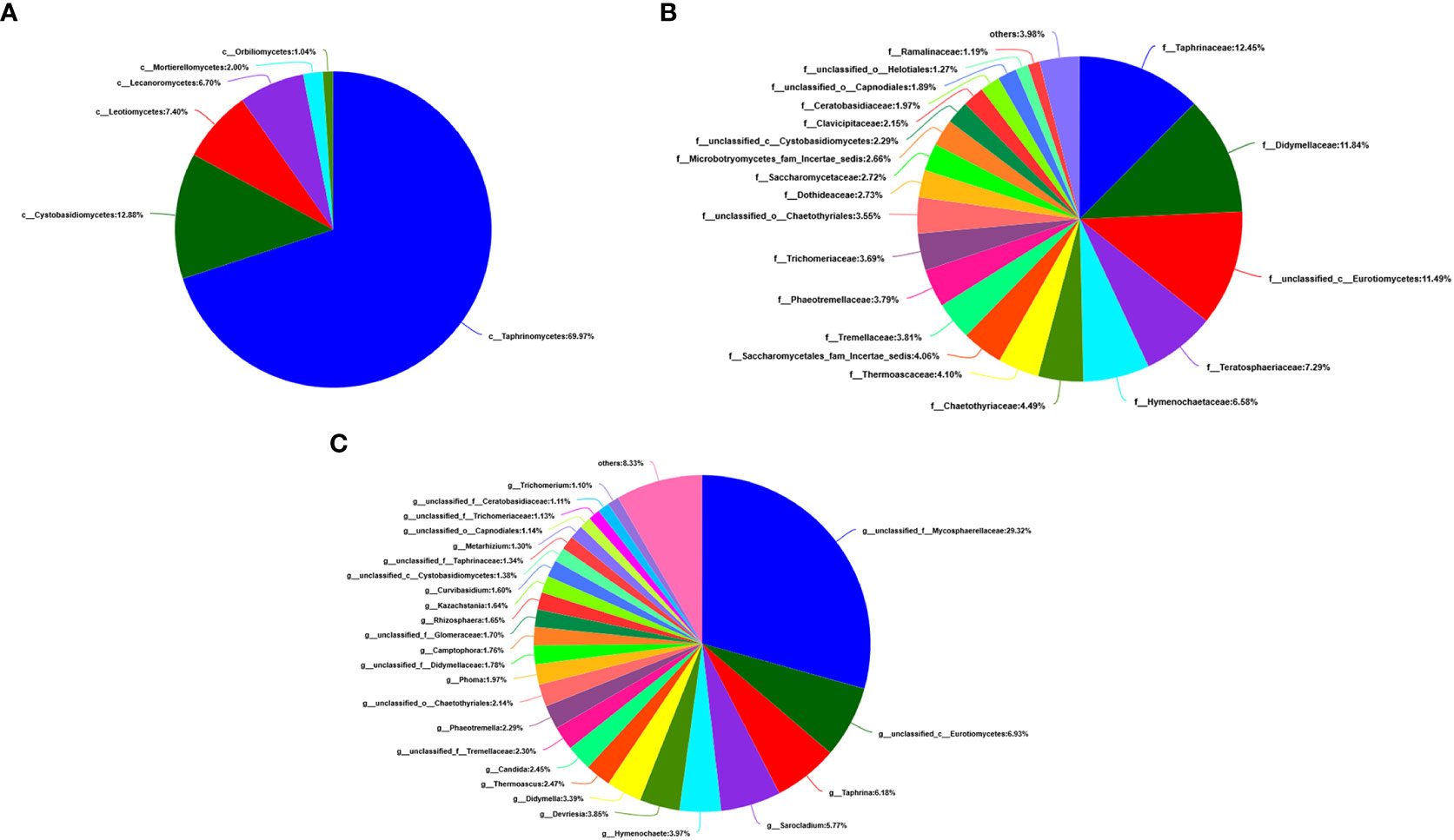
Figure 5 Compositions and relative plenties of unique fungal endophytes in KMN-R of Alopecurus aequalis on diverse taxonomic levels. (A) Class. (B) Family. (C) Genus.
At the genus level of A. aequalis fungal, ANOVA was used to evaluate all detected genera to test the relative abundance (%) (Figure 4D). The fungal community in KMN-R and KMN-S A. aequalis populations was dominated by 17 genera. It was observed that unclassified Ascomycota in KMN-R was significantly more abundant (p < 0.05) than that in KMN-S at the genus level (Table 6). In addition, 52 genera were only observed with a high relative abundance in the KMN-R A. aequalis, including unclassified Mycosphaerellaceae and unclassified Eurotiomycetes (Figure 5C), and 21 genera were only observed with a high relative abundance in the KMN-S A. aequalis, including unclassified Saccharomycetales, Humicola, and Dioszegia (Figure 6B).
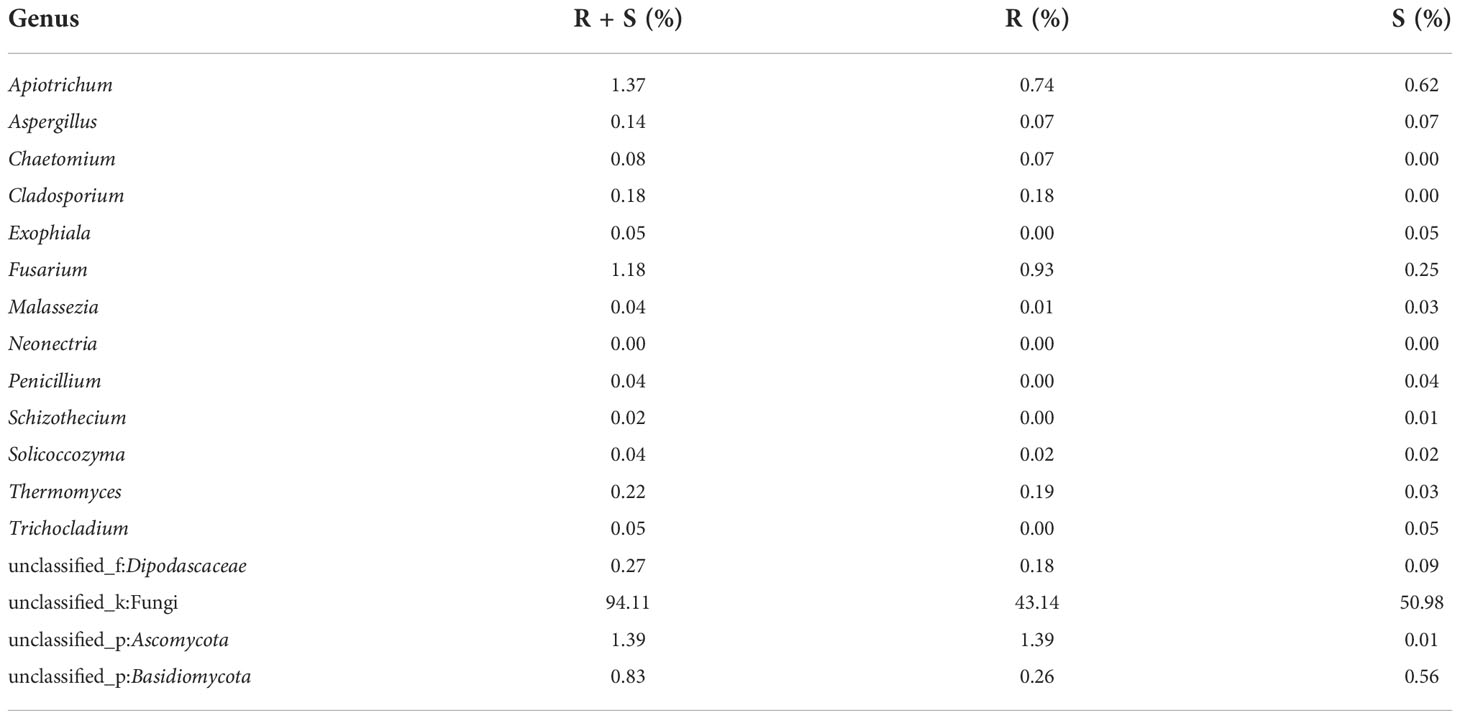
Table 6 Relative plenties percentage of sequence number in common genera between KMN-R and KMN-S of Alopecurus aequalis.
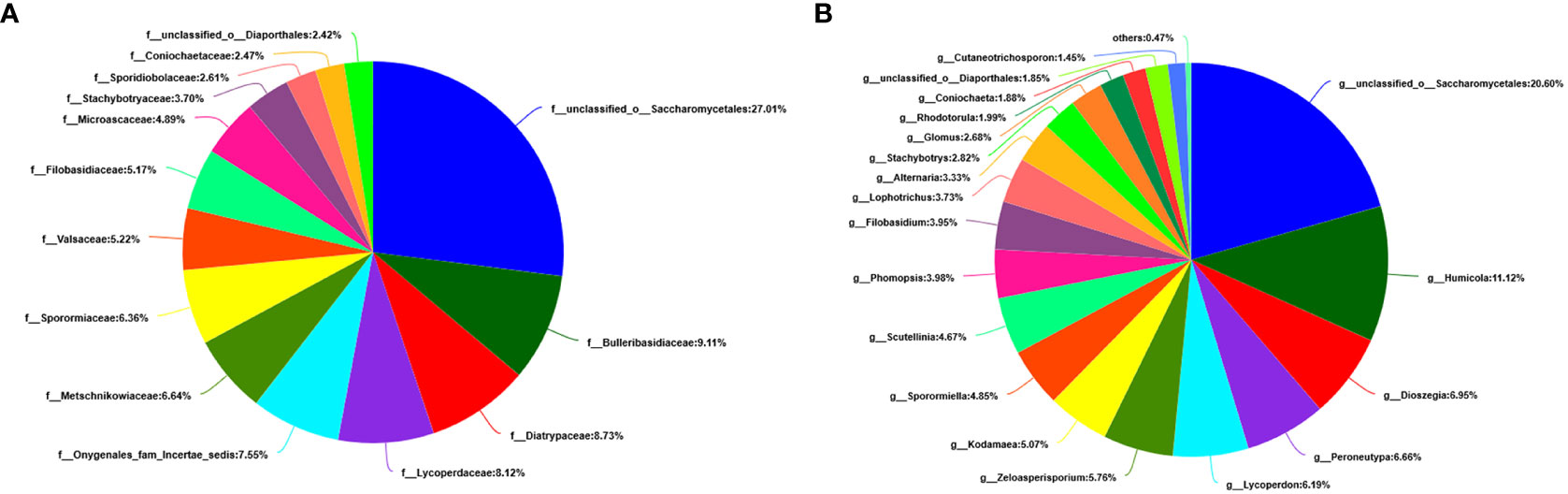
Figure 6 Compositions and relative plenties of unique fungal endophytes in KMN-S of Alopecurus aequalis on diverse taxonomic levels. (A) Family. (B) Genus.
4 Discussion
A. aequalis has evolved mesosulfuron-methyl resistance because of enhanced selective pressures applied to wheat fields in China. Since mesosulfuron-methyl was brought in, it is consumingly applied to control A. aequalis and other grassy weeds. Nevertheless, resistance to mesosulfuron-methyl happened in A. aequalis (Guo et al., 2015b), and it has been reported that high-level mesosulfuron-methyl resistance existed in A. aequalis populations (Guo et al., 2015a; Xia et al., 2015; Guo et al., 2018; Zhao et al., 2019b). In this study, the KMN-R population gathered from an Anhui wheat field has also developed mesosulfuron-methyl resistance. In the light of local farmer households, mesosulfuron-methyl has been applied in the wheat field where KMN-R gathered for >5 years. This result shows again that long-term usage of the same herbicide or herbicides with similar resistance mechanisms is the biggest risk factor to promote herbicide resistance evolutions (Norsworthy et al., 2012).
GST-mediated herbicide metabolism was confirmed in both weeds and crops (Cummins et al., 2011; Cummins et al., 2013; Evans et al., 2017; Nakka et al., 2017). NBD-Cl has been indicated to inhibit the expressed pi class GST in human and expressed phi (F) class GST in resistant Alopecurus myosuroides strongly (Ricci et al., 2005; Cummins et al., 2013). In this study, to investigate NTSR, NBD-Cl treatment decreased the resistance level of KMN-R to mesosulfuron-methyl to a great extent (Table 1). Especially, the consequent GR50 value of NBD-Cl plus mesosulfuron-methyl was higher than the GR50 value of KMN-S, showing a GST-participated enhanced metabolism and other resistance mechanisms presented in the KMN-R population and contributed to its resistance phenotype. Malathion is known to goal diverse P450 genes (Oliveira et al., 2017). In our study, pretreatment with malathion did not increase the toxicity of mesosulfuron-methyl to plants in resistant population KMN-R, indicating that there might not be any additional benefit from P450-mediated enhanced metabolism.
Even though enhanced herbicide metabolism has been found in several weeds, limited genes related to metabolic resistance are identified (Cummins et al., 2013; Iwakami et al., 2014; Iwakami et al., 2019). Here, A. aequalis GSTZ2 and GSTT3 genes were upregulated in the KMN-R population (Table 2). In weeds, GSTF2 and GSTF1 genes have major roles in herbicide resistance of A. myosuroides (Cummins et al., 2013) and waterhemp (Amaranthus tuberculatus) (Evans et al., 2017). In crops, tau glutathione transferases from Citrus sinensis (CsGSTU) overexpression evolved tolerance to fluorodifen and alachlor in tobacco (Lo Cicero et al., 2015; Lo Cicero et al., 2017), and GSTBZ2 conducts the key last step in the biosynthesis of anthocyanins in corn (Marrs et al., 1995). These findings showed that A. aequalis GSTZ2 and GSTT3 also can have crucial roles in evolving mesosulfuron-methyl resistance. Notably, the resistant and susceptible A. aequalis populations in the present research were collected in the same province and with a distance of <3 km. Therefore, we ensured the conformance of the studied populations in regard to genetic background and decreased the “false-positive” alleles, irrelevant to metabolic resistance, due to this factor. Additional research is needed to evaluate the functions of the two GST genes in mesosulfuron-methyl resistance.
Environmental factors like soil quality are the major drivers of plant microbiome (Podolich et al., 2015; Whitaker et al., 2018). Lots of research indicated that temperature, pH, and soil salinity may affect microbial community structure markedly (Thiem et al., 2018). It was reported that infection with fungi Neotyphodium spp. enhanced herbicide tolerance in ryegrass (Vila-Aiub et al., 2003). In this study, we evaluated alpha diversity, centering on OTU diversity, evenness, and richness (Tables 3–6), and found that fungal community compositions had a more unclear differentiation between KMN-R and KMN-S A. aequalis populations including alpha diversity and taxon abundances because some phyla did not have a distinguishing variation related to herbicide resistance. Notably, we found a higher abundance of Mortierellomycota in the KMN-R A. aequalis (Figure 6). Mortierellomycota was found to be positively correlated with the pH, total organic matter, and cation exchange capacity in plants, which ultimately changed the accumulation of Cd (Shi et al., 2020), indicating that the abundance of Mortierellomycota in the KMN-R A. aequalis might reduce the accumulation of mesosulfuron-methyl. The strain should be isolated from KMN-R A. aequalis for further studies.
Exploring NTSR in A. aequalis is significant, as NTSR can result in unpredictable resistance patterns (Petit et al., 2010). Even though herbicide mixture and rotation are performed as manage solution for TSR, they may be unable to manage NTSR (Yu and Powles, 2014a). Luckily, the NTSR KMN-R can be inhibited with mesosulfuron-methyl plus NBD-Cl. Nevertheless, A. aequalis control requires more extensive solutions instead of only relying on herbicides. Finally, we identified the indicator OTUs and core fungi between GST-involved herbicide-resistant and -susceptible A. aequalis. This may give the basis for further research of herbicide resistance with microbial interaction.
In summary, we found that target site Trp-574-Leu mutation and GST-mediated non-target site evolved mesosulfuron-methyl resistance in an A. aequalis population. The KMN-R population showed broad-spectrum resistance to ALS-inhibiting herbicides of all five chemical families tested. Pretreatment with NBD-Cl reversed the resistance of KMN-R to mesosulfuron-methyl. Two GST genes (GSTZ2 and GSTT3) were constitutively overexpressed in the KMN-R population. Endophytes can promote plant growth and enhance tolerance to abiotic stress. From this perspective, we compared the diversity of endophytic fungi between KMN-R and KMN-S A. aequalis and found that there are some special fungi in the KMN-R population when compared to the KMN-S population. These endophytic fungi may be involved in the resistance of the KMN-R population to mesosulfuron-methyl. Further studies are needed to verify the function of the two GST genes and figure out the role of endophytic fungi in the metabolic processes of herbicide resistance.
Data availability statement
The data presented in the study are deposited in the NCBI Sequence Read Archive repository, accession number PRJNA901208 and PRJNA902298.
Author contributions
YZ, HL, LB, and LP designed the experiments. YZ, HL, ZC, WC, and ZL performed the experiments. YZ, HL, and LP analyzed the data. YZ, LB, and LP wrote and revised the manuscript. All authors read and approved the final manuscript.
Funding
This research was financially supported by the National Key Research and Development Program of China (No. 2021YFD1700101), National Natural Science Foundation of China (32130091, 31901905), Young Elite Scientists Sponsorship Program by CAST (2021QNRC001), the Natural Science Foundation of Hunan Province, China (2021JJ20002), the Science and Technology Innovation Program of Hunan Province (2021RC3087), and China Agriculture Research System (CARS-16-E19).
Conflict of interest
The authors declare that the research was conducted in the absence of any commercial or financial relationships that could be construed as a potential conflict of interest.
Publisher’s note
All claims expressed in this article are solely those of the authors and do not necessarily represent those of their affiliated organizations, or those of the publisher, the editors and the reviewers. Any product that may be evaluated in this article, or claim that may be made by its manufacturer, is not guaranteed or endorsed by the publisher.
Supplementary material
The Supplementary Material for this article can be found online at: https://www.frontiersin.org/articles/10.3389/fcimb.2022.1094853/full#supplementary-material
References
Bai, S., Zhang, F. W., Li, Z. R., Wang, H. Z., Wang, Q., Wang, J. X., et al. (2019). Target-site and non-target-site-based resistance to tribenuron-methyl in multiply-resistant Myosoton aquaticum l. Pestic. Biochem. Phys. 155, 8–14. doi: 10.1016/j.pestbp.2018.12.004
Bolger, A. M., Lohse, M., Usadel, B. (2014). Trimmomatic: A flexible trimmer for illumina sequence data. Bioinformatics 30 (15), 2114–2120. doi: 10.1093/bioinformatics/btu170
Chen, T. X., White, J. F., Li, C. J., Nan, Z. B. (2021). Exogenous spermidine enhances Epichloë endophyte-induced tolerance to NaCl stress in wild barley (Hordeum brevisubulatum). Plant Soil 468 (1-2), 77–95. doi: 10.1007/s11104-021-05109-2
Cummins, I., Dixon, D. P., Freitag-Pohl, S., Skipsey, M., Edwards, R. (2011). Multiple roles for plant glutathione transferases in xenobiotic detoxification. Drug Metab. Rev. 43 (3), 266–280. doi: 10.3109/03602532.2011.552910
Cummins, I., Wortley, D. J., Sabbadin, F., He, Z., Coxon, C. R., Straker, H. E., et al. (2013). Key role for a glutathione transferase in multiple-herbicide resistance in grass weeds. P. Natl. Acad. Sci. U.S.A. 110 (15), 5812–5817. doi: 10.1073/pnas.1221179110
Délye, C., Jasieniuk, M., Le Corre, V. (2013). Deciphering the evolution of herbicide resistance in weeds. Trends Genet. 29 (11), 649–658. doi: 10.1016/j.tig.2013.06.001
Dickie, I. A. (2010). Insidious effects of sequencing errors on perceived diversity in molecular surveys. New Phytol. 188 (4), 916–918. doi: 10.1111/j.1469-8137.2010.03473.x
Dinkins, R. D., Nagabhyru, P., Graham, M. A., Boykin, D., Schardl, C. L. (2017). Transcriptome response of Lolium arundinaceum to its fungal endophyte Epichloë coenophiala. New Phytol. 213 (1), 324–337. doi: 10.1111/nph.14103
Durner, J., Gailus, V., Böger, P. (1991). New aspects on inhibition of plant acetolactate synthase by chlorsulfuron and imazaquin. Plant Physiol. 95 (4), 1144–1149. doi: 10.1104/pp.95.4.1144
Edgar, R. C., Haas, B. J., Clemente, J. C., Quince, C., Knight, R. (2011). UCHIME improves sensitivity and speed of chimera detection. Bioinformatics 27 (16), 2194–2200. doi: 10.1093/bioinformatics/btr381
Evans, A. F., Jr., O’brien, S. R., Ma, R., Hager, A. G., Riggins, C. W., Lambert, K. N., et al. (2017). Biochemical characterization of metabolism-based atrazine resistance in Amaranthus tuberculatus and identification of an expressed GST associated with resistance. Plant Biotechnol. J. 15 (10), 1238–1249. doi: 10.1111/pbi.12711
Ganie, S. A., Bhat, J. A., Devoto, A. (2022). The influence of endophytes on rice fitness under environmental stresses. Plant Mol. Biol. 109 (4-5), 447–467. doi: 10.1007/s11103-021-01219-8
Grabherr, M. G., Haas, B. J., Yassour, M., Levin, J. Z., Thompson, D. A., Amit, I., et al. (2011). Full-length transcriptome assembly from RNA-seq data without a reference genome. Nat. Biotechnol. 29 (7), 644–652. doi: 10.1038/nbt.1883
Guo, W. L., Chi, Y. Y., Feng, L., Tian, X. S., Liu, W. T., Wang, J. X. (2018). Fenoxaprop-p-ethyl and mesosulfuron-methyl resistance status of shortawn foxtail (Alopecurus aequalis sobol.) in eastern China. Pestic. Biochem. Phys. 148, 126–132. doi: 10.1016/j.pestbp.2018.04.013
Guo, W. L., Liu, W. T., Li, L. X., Yuan, G. H., Du, L., Wang, J. X. (2015b). Molecular basis for resistance to fenoxaprop in shortawn foxtail (Alopecurus aequalis) from China. Weed Sci. 63 (2), 416–424. doi: 10.1614/WS-D-14-00105.1
Guo, W. L., Yuan, G. H., Liu, W. T., Bi, Y. L., Du, L., Zhang, C., et al. (2015a). Multiple resistance to ACCase and AHAS-inhibiting herbicides in shortawn foxtail (Alopecurus aequalis sobol.) from China. Pestic. Biochem. Phys. 124, 66–72. doi: 10.1016/j.pestbp.2015.04.006
Hashim, S., Jan, A., Sunohara, Y., Hachinohe, M., Ohdan, H., Matsumoto, H. (2012). Mutation of alpha-tubulin genes in trifluralin-resistant water foxtail (Alopecurus aequalis). Pest Manage. Sci. 68 (3), 422–429. doi: 10.1002/ps.2284
Heap, I. (2022) International survey of herbicide resistant weeds. Available at: http://www.weedscience.org (Accessed 1-5 2018).
Iwakami, S., Endo, M., Saika, H., Okuno, J., Nakamura, N., Yokoyama, M., et al. (2014). Cytochrome P450 CYP81A12 and CYP81A21 are associated with resistance to two acetolactate synthase inhibitors in Echinochloa phyllopogon. Plant Physiol. 165 (2), 618–629. doi: 10.1104/pp.113.232843
Iwakami, S., Kamidate, Y., Yamaguchi, T., Ishizaka, M., Endo, M., Suda, H., et al. (2019). CYP81A P450s are involved in concomitant cross-resistance to acetolactate synthase and acetyl-CoA carboxylase herbicides in Echinochloa phyllopogon. New Phytol. 221 (4), 2112–2122. doi: 10.1111/nph.15552
Li, B., Dewey, C. N. (2011). RSEM: accurate transcript quantification from RNA-seq data with or without a reference genome. BMC Bioinf. 12 (1), 323. doi: 10.1186/1471-2105-12-323
Liu, H. W., Brettell, L. E., Qiu, Z. G., Singh, B. K. (2020a). Microbiome-mediated stress resistance in plants. Trends Plant Sci. 25 (8), 733–743. doi: 10.1016/j.tplants.2020.03.014
Liu, K. L., Luo, K., Mao, A. X., Pan, L., Yan, B., Wu, J., et al. (2020b). Endophytes enhance Asia minor bluegrass (Polypogon fugax) resistance to quizalofop-p-ethyl. Plant Soil 450 (1-2), 373–384. doi: 10.1007/s11104-020-04509-0
Lo Cicero, L., Catara, V., Strano, C. P., Bella, P., Madesis, P., Lo Piero, A. R. (2017). Over-expression of CsGSTU promotes tolerance to the herbicide alachlor and resistance to Pseudomonas syringae pv. tabaci in transgenic tobacco. Biol. Plantarum 61 (1), 169–177. doi: 10.1007/s10535-016-0659-6
Lo Cicero, L., Madesis, P., Tsaftaris, A., Lo Piero, A. R. (2015). Tobacco plants over-expressing the sweet orange tau glutathione transferases (CsGSTUs) acquire tolerance to the diphenyl ether herbicide fluorodifen and to salt and drought stresses. Phytochemistry 116, 69–77. doi: 10.1016/j.phytochem.2015.03.004
Macías-Rubalcava, M. L., Garrido-Santos, M. Y. (2022). Phytotoxic compounds from endophytic fungi. Appl. Microbiol. Biot. 106 (3), 931–950. doi: 10.1007/s00253-022-11773-w
Magoč, T., Salzberg, S. L. (2011). FLASH: fast length adjustment of short reads to improve genome assemblies. Bioinformatics 27 (21), 2957–2963. doi: 10.1093/bioinformatics/btr507
Marrs, K. A., Alfenito, M. R., Lloyd, A. M., Walbot, V. (1995). A glutathione-s-transferase involved in vacuolar transfer encoded by the maize gene Bronze-2. Nature 375 (6530), 397–400. doi: 10.1038/375397a0
Nakka, S., Godar, A. S., Thompson, C. R., Peterson, D. E., Jugulam, M. (2017). Rapid detoxification via glutathione s-transferase (GST) conjugation confers a high level of atrazine resistance in palmer amaranth (Amaranthus palmeri). Pest Manage. Sci. 73 (11), 2236–2243. doi: 10.1002/ps.4615
Nakka, S., Thompson, C. R., Peterson, D. E., Jugulam, M. (2018). Target site-based and non-target site based resistance to ALS inhibitors in palmer amaranth (Amaranthus palmeri). Weed Sci. 65 (6), 681–689. doi: 10.1017/wsc.2017.43
Norsworthy, J. K., Ward, S. M., Shaw, D. R., Llewellyn, R. S., Nichols, R. L., Webster, T. M., et al. (2012). Reducing the risks of herbicide resistance: best management practices and recommendations. Weed Sci. 60 (sp1), 31–62. doi: 10.1614/WS-D-11-00155.1
Oliveira, M. C., Gaines, T. A., Dayan, F. E., Patterson, E. L., Jhala, A. J., Knezevic, S. Z. (2017). Reversing resistance to tembotrione in an Amaranthus tuberculatus (var. rudis) population from Nebraska, USA with cytochrome P450 inhibitors. Pest Manage. Sci. 74 (10), 2296–2305. doi: 10.1002/ps.4697
Owen, M. J., Goggin, D. E., Powles, S. B. (2012). Non-target-site-based resistance to ALS-inhibiting herbicides in six Bromus rigidus populations from Western Australian cropping fields. Pest Manage. Sci. 68 (7), 1077–1082. doi: 10.1002/ps.3270
Pan, L., Yu, Q., Han, H. P., Mao, L. F., Nyporko, A., Fan, L. J., et al. (2019). Aldo-keto reductase metabolizes glyphosate and confers glyphosate resistance in Echinochloa colona. Plant Physiol. 181 (4), 1519–1534. doi: 10.1104/pp.19.00979
Petit, C., Duhieu, B., Boucansaud, K., Délye, C. (2010). Complex genetic control of non-target-site-based resistance to herbicides inhibiting acetyl-coenzyme a carboxylase and acetolactate-synthase in Alopecurus myosuroides huds. Plant Sci. 178 (6), 501–509. doi: 10.1016/j.plantsci.2010.03.007
Podolich, O., Ardanov, P., Zaets, I., Pirttila, A. M., Kozyrovska, N. (2015). Reviving of the endophytic bacterial community as a putative mechanism of plant resistance. Plant Soil 388 (1-2), 367–377. doi: 10.1007/s11104-014-2235-1
Powles, S. B., Yu, Q. (2010). Evolution in action: plants resistant to herbicides. Annu. Rev. Plant Biol. 61 (1), 317–347. doi: 10.1146/annurev-arplant-042809-112119
Riar, D. S., Norsworthy, J. K., Srivastava, V., Nandula, V., Bond, J. A., Scott, R. C. (2013). Physiological and molecular basis of acetolactate synthase-inhibiting herbicide resistance in barnyardgrass (Echinochloa crus-galli). J. Agr. Food Chem. 61 (2), 278–289. doi: 10.1021/jf304675j
Ricci, G., De Maria, F., Antonini, G., Turella, P., Bullo, A., Stella, L., et al. (2005). 7-Nitro-2,1,3-benzoxadiazole derivatives, a new class of suicide inhibitors for glutathione s-transferases. mechanism of action of potential anticancer drugs. J. Biol. Chem. 280 (28), 26397–26405. doi: 10.1074/jbc.M503295200
Rodriguez, R., Redman, R. (2008). More than 400 million years of evolution and some plants still can’t make it on their own: plant stress tolerance via fungal symbiosis. J. Exp. Bot. 59 (5), 1109–1114. doi: 10.1093/jxb/erm342
Shergill, L. S., Bish, M. D., Jugulam, M., Bradley, K. W. (2018). Molecular and physiological characterization of six-way resistance in an Amaranthus tuberculatus var. rudis biotype from Missouri. Pest Manage. Sci. 74 (12), 2688–2698. doi: 10.1002/ps.5082
Shi, Y., Qiu, L. S., Guo, L. P., Man, J. H., Shang, B. P., Pu, R. F., et al. (2020). K Fertilizers reduce the accumulation of cd in Panax notoginseng (Burk.) F.H. by improving the quality of the microbial community. Front. Plant Sci. 11. doi: 10.3389/fpls.2020.00888
Thiem, D., Gołębiewski, M., Hulisz, P., Piernik, A., Hrynkiewicz, K. (2018). How does salinity shape bacterial and fungal microbiomes of Alnus glutinosa roots? Front. Microbiol. 9. doi: 10.3389/fmicb.2018.00651
Vila-Aiub, M. M., Martinez-Ghersa, M. A., Ghersa, C. M. (2003). Evolution of herbicide resistance in weeds: vertically transmitted fungal endophytes as genetic entities. Evol. Ecol. 17 (5-6), 441–456. doi: 10.1023/B:EVEC.0000005580.19018.fb
Wang, J. Z., Cao, W. F., Guo, Q. S., Yang, Y., Bai, L. Y., Pan, L. (2022). Resistance to mesosulfuron-methyl in Beckmannia syzigachne may involve ROS burst and non-target-site resistance mechanisms. Ecotox. Environ. Safe. 229, 113072. doi: 10.1016/j.ecoenv.2021.113072
Wang, Y. B., Zhang, W. X., Ding, C. J., Zhang, B. Y., Huang, Q. J., Huang, R. F., et al. (2019). Endophytic communities of transgenic poplar were determined by the environment and niche rather than by transgenic events. Front. Microbiol. 10. doi: 10.3389/fmicb.2019.00588
Whitaker, B. K., Reynolds, H. L., Clay, K. (2018). Foliar fungal endophyte communities are structured by environment but not host ecot ype in Panicum virgatum (switchgrass). Ecology 99 (12), 2703–2711. doi: 10.1002/ecy.2543
Xia, W. W., Pan, L., Li, J., Wang, Q., Feng, Y. J., Dong, L. Y. (2015). Molecular basis of ALS- and/or ACCase-inhibitor resistance in shortawn foxtail (Alopecurus aequalis sobol.). Pestic. Biochem. Phys. 122, 76–80. doi: 10.1016/j.pestbp.2014.12.019
Yan, L., Zhu, J., Zhao, X. X., Shi, J. L., Jiang, C. M., Shao, D. Y. (2019). Beneficial effects of endophytic fungi colonization on plants. Appl. Microbiol. Biot. 103 (8), 3327–3340. doi: 10.1007/s00253-019-09713-2
Young, M. D., Wakefield, M. J., Smyth, G. K., Oshlack, A. (2010). Gene ontology analysis for RNA-seq: accounting for selection bias. Genome Biol. 11 (2), R14. doi: 10.1186/gb-2010-11-2-r14
Yuan, J. S., Tranel, P. J., Stewart, C. N., Jr. (2007). Non-target-site herbicide resistance: a family business. Trends Plant Sci. 12 (1), 6–13. doi: 10.1016/j.tplants.2006.11.001
Yu, Q., Powles, S. B. (2014a). Metabolism-based herbicide resistance and cross-resistance in crop weeds: a threat to herbicide sustainability and global crop production-2. Plant Physiol. 166 (3), 1106–1118. doi: 10.1104/pp.114.242750
Yu, Q., Powles, S. B. (2014b). Resistance to AHAS inhibitor herbicides: current understanding. Pest Manage. Sci. 70 (9), 1340–1350. doi: 10.1002/ps.3710
Zhao, N., Li, Q., Guo, W. L., Zhang, L. L., Ge, L. A., Wang, J. X. (2018a). Effect of environmental factors on germination and emergence of shortawn foxtail (Alopecurus aequalis). Weed Sci. 66 (1), 47–56. doi: 10.1017/wsc.2017.42
Zhao, N., Yan, Y. Y., Ge, L. A., Zhu, B. L., Liu, W. T., Wang, J. X. (2019b). Target site mutations and cytochrome P450s confer resistance to fenoxaprop-p-ethyl and mesosulfuron-methyl in Alopecurus aequalis. Pest Manage. Sci. 75 (1), 204–214. doi: 10.1002/ps.5089
Zhao, N., Yan, Y. Y., Liu, W. T., Wang, J. X. (2022). Cytochrome P450 CYP709C56 metabolizing mesosulfuron-methyl confers herbicide resistance in Alopecurus aequalis. Cell. Mol. Life Sci. 79 (4), 205. doi: 10.1007/s00018-022-04171-y
Zhao, N., Yan, Y. Y., Luo, Y. L., Zou, N., Liu, W. T., Wang, J. X. (2019a). Unravelling mesosulfuron-methyl phytotoxicity and metabolism-based herbicide resistance in Alopecurus aequalis: Insight into regulatory mechanisms using proteomics. Sci. Total Environ. 670, 486–497. doi: 10.1016/j.scitotenv.2019.03.089
Zhao, N., Yan, Y. Y., Wang, H. Z., Bai, S., Wang, Q., Liu, W. T., et al. (2018b). Acetolactate synthase overexpression in mesosulfuron-methyl-resistant shortawn foxtail (Alopecurus aequalis sobol.): reference gene selection and herbicide target gene expression analysis. J. Agr. Food Chem. 66 (37), 9624–9634. doi: 10.1021/acs.jafc.8b03054
Keywords: ALS, mesosulfuron-methyl, Alopecurus aequalis, non-target site resistance, glutathione S-transferase (GST), fungi
Citation: Zhan Y, Liu H, Cao Z, Chen W, Li Z, Bai L and Pan L (2022) Comparative analysis of fungal communities between herbicide-resistant and -susceptible Alopecurus aequalis. Front. Cell. Infect. Microbiol. 12:1094853. doi: 10.3389/fcimb.2022.1094853
Received: 10 November 2022; Accepted: 28 November 2022;
Published: 23 December 2022.
Edited by:
Ziyi Yin, Shandong Agricultural University, ChinaReviewed by:
Haonan Wang, Zhejiang Agriculture and Forestry University, ChinaYanhan Dong, Qingdao University, China
Weitang Liu, Shandong Agricultural University, China
Lei Jiang, Anhui Agricultural University, China
Copyright © 2022 Zhan, Liu, Cao, Chen, Li, Bai and Pan. This is an open-access article distributed under the terms of the Creative Commons Attribution License (CC BY). The use, distribution or reproduction in other forums is permitted, provided the original author(s) and the copyright owner(s) are credited and that the original publication in this journal is cited, in accordance with accepted academic practice. No use, distribution or reproduction is permitted which does not comply with these terms.
*Correspondence: Lang Pan, langpan@hunau.edu.cn; Lianyang Bai, lybai@hunaas.cn
†These authors have contributed equally to this work