Transcriptome analysis of the hepatopancreas from the Litopenaeus vannamei infected with different flagellum types of Vibrio alginolyticus strains
- 1Hainan Provincial Key Laboratory for Tropical Hydrobiology and Biotechnology, Hainan University, Haikou, China
- 2Laboratory of Development and Utilization of Marine Microbial Resource, Hainan University, Haikou, China
- 3School of Fisheries, Xinyang Agriculture and Forestry University, Xinyang, China
- 4Collaborative Innovation Center of Marine Science and Technology, Hainan University, Haikou, China
Vibrio alginolyticus, one of the prevalently harmful Vibrio species found in the ocean, causes significant economic damage in the shrimp farming industry. Its flagellum serves as a crucial virulence factor in the invasion of host organisms. However, the processes of bacteria flagella recognition and activation of the downstream immune system in shrimp remain unclear. To enhance comprehension of this, a ΔflhG strain was created by in-frame deletion of the flhG gene in V. alginolyticus strain HN08155. Then we utilized the transcriptome analysis to examine the different immune responses in Litopenaeus vannamei hepatopancreas after being infected with the wild type and the mutant strains. The results showed that the ΔflhG strain, unlike the wild type, lost its ability to regulate flagella numbers negatively and displayed multiple flagella. When infected with the hyperflagella-type strain, the RNA-seq revealed the upregulation of several immune-related genes in the shrimp hepatopancreas. Notably, two C-type lectins (CTLs), namely galactose-specific lectin nattectin and macrophage mannose receptor 1, and the TNF receptor-associated factor (TRAF) 6 gene were upregulated significantly. These findings suggested that C-type lectins were potentially involved in flagella recognition in shrimp and the immune system was activated through the TRAF6 pathway after flagella detection by CTLs.
1 Introduction
Pacific white shrimp (Litopenaeus vannamei) is highly favored in China and other countries for its abundant protein and low-fat content, making it the most popular shrimp species for consumption (Li et al., 2021). As the breeding industry increased rapidly, epidemic diseases have become one of the major risk factors for shrimp aquaculture in recent years (Yu et al., 2022). Vibrio alginolyticus and other marine-dwelling Vibrio species are conditional pathogens that cause white feces syndrome (WFS), acute hepatopancreatic necrosis disease (AHPND), and other vibrioses in shrimps (Janda et al., 2015; Baker-Austin et al., 2018; Ngo et al., 2020; Foysal et al., 2021; Kumar et al., 2021; Shen et al., 2021; Munkongwongsiri et al., 2022).
When pathogenic Vibrio species or other pathogens infect the host, the immune system will be activated by recognizing several molecules called pathogen-associated molecular patterns (PAMPs). These molecules are crucial for the pathogens’ virulence and survival (Kumar et al., 2011). The host’s pattern recognition receptors (PRRs), including Toll-like receptors (TLRs), RIG-I-like receptors (RLRs), NOD-like receptors (NLRs), and DNA receptors, sense these PAMPs (Medzhitov, 2007; Kumar et al., 2009). After the PAMPs recognized by PRRs, the immune system responds quickly to eliminate the pathogens through several signaling pathways (Li et al., 2009; Fitzgerald and Kagan, 2020).
Being a major PAMP, the bacterial flagellum is also an important virulence factor for its function in motility, chemotaxis, adhesion, biofilm formation, and secretion (Duan et al., 2013; Chaban et al., 2015; Rossez et al., 2015). In mammals and teleost fish, the bacterial flagella recognition and signaling are through TLR5, NLRC4, and NAIP5, and that activation of these receptors mobilizes nuclear factor NF-kappaB and stimulates the production of tumor necrosis factor-alpha (TNF-α) (Feuillet et al., 2006; Zhao and Shao, 2015; Fitzgerald and Kagan, 2020). Some pathogens also develop strategies to avoid the recognition of the flagella and escape the host immune system clearance. During infection, S. typhimurium significantly reduces the expression of genes involved in flagellar machinery and chemotaxis when present inside macrophages (Eriksson et al., 2003). By day 3 after feeding, the motility of E. coli introduced into the mouse gut decreased by 45-50%, and by day 15, it dropped further to 80-90% loss of motility (Gauger et al., 2007). Other methods for evading detection by the host immune system include regularly alternating the expression of flagellin proteins (phase variation), enabling different subsets of the population to express flagella (bistability). Additionally, modifying the structure of flagellin proteins to make them unrecognizable to TLR5 (flagellin modification) and adding post-translational modifications to flagellins to mask target sites (glycosylation) are also effective strategies (Chaban et al., 2015). Yet, there is scant research about how crustacean, including shrimps, recognizes bacteria flagella and actives their immune system. Several TLRs have been found in shrimps and carbs, but none has been confirmed to respond to flagella (Habib and Zhang, 2020).
In this research, we injected the wild-type and mutant strains of V. alginolyticus strain HN08115 with different flagella phenotypes into L. vannamei and analyzed the different gene expression patterns in shrimp hepatopancreas. Our results might help explain how shrimps’ immune systems recognize bacterial flagella and activate the immune system through signaling pathways.
2 Materials and methods
2.1 Bacterial strains and plasmids
The strains and plasmids are listed in Table 1. The V. alginolyticus strain HN08155 was cultured in 2216E medium at 30°C. E. coli strain β2163 was cultured in LB broth with 0.3 mM DAP at 37°C. The medium was added with chloramphenicol (50μg/ml), or ampicillin(100μg/ml) according to the plasmid feature.
2.2 Construction of flhG mutant
About 600 bp of the flhG gene’s upstream and downstream regions were amplified by PCR separately. Then the two fragments were joined together by overlap extension PCR. Primers used in amplifications are listed in Table 2. The junction fragment was inserted into the suicide vector pDM4 at the XbaI site to constructed recombinant plasmid pDM4-flhG. The plasmid pDM4-flhG was transformed into E. coli β2163 and introduced into V. alginolyticus HN08155 by conjugation. LBS agar containing 10% sucrose was used to screen the double-crossover recombinant. The △flhG mutate strain was confirmed by PCR and sequencing.
2.3 Flagellum observation by transmission electron microscope
The V. alginolyticus HN08155 wild-type (WT) and △flhG strains were cultured until the OD600 values were approximately 1.0. The cells were washed and gently resuspended with normal saline solution (0.9% NaCl). 10μl of the resuspension was added to a 300-mesh carbon-coated Formvar grid (Electron Microscopy Science, Hatfield, Pennsylvania) and negatively stained with a 2% (w/v) phosphotungstic acid solution. The JEOL JEM-2100 transmission microscope was used to acquire the images.
2.4 Shrimp rearing and sample collection
Healthy L. vannamei (12 ± 2g) was purchased from a shrimp farm in Dongfang, Hainan, China. The shrimp were maintained in tanks with aerated seawater (30 ppt, 26 ± 2°C) for one month. The shrimps were randomly separated into two groups and were injected with the V. alginolyticus HN08155 WT and △flhG strains into the tail muscle to a final concentration of 8 x 104CFU/g. After 4 hours of the injection, the hepatopancreases of three random shrimps of each group were collected as one sample and frozen in liquid nitrogen for transcriptome analysis. Three replicate samples were collected from each group.
2.5 cDNA library construction and sequencing
Total RNA from the hepatopancreas sample was extracted from the tissue using TRIzol® Reagent according to the manufacturer’s instructions (Invitrogen) and genomic DNA was removed using DNase I (Takara). RNA degradation and contamination were monitored on 1% agarose gels. Then RNA quality was determined by 2100 Bioanalyser (Agilent Technologies) and quantified using the ND-2000 (NanoDrop Technologies). Only high-quality RNA sample (OD260/280 = 1.8~2.2, OD260/230≥2.0, RIN≥8.0, 28S:18S≥1.0, >1μg) was used to construct the sequencing library.
RNA purification, reverse transcription, library construction, and sequencing were performed at Shanghai Majorbio Bio-pharm Biotechnology Co., Ltd. (Shanghai, China) according to the manufacturer’s instructions (Illumina, San Diego, CA). The transcriptome library was prepared following the TruSeq TM RNA sample preparation Kit from Illumina (San Diego, CA) using 1μg of total RNA. Shortly, messenger RNA was isolated according to the polyA selection method by oligo(dT) beads and then fragmented by fragmentation buffer first. Secondly, double-stranded cDNA was synthesized using a SuperScript double-stranded cDNA synthesis kit (Invitrogen, CA) with random hexamer primers (Illumina). Then the synthesized cDNA was subjected to end-repair, phosphorylation, and ‘A’ base addition according to Illumina’s library construction protocol. Libraries were size selected for cDNA target fragments of 300 bp on 2% Low Range Ultra Agarose followed by PCR amplified using Phusion DNA polymerase (NEB) for 15 PCR cycles. After being quantified by TBS380, the paired-end RNA-seq sequencing library was sequenced with the Illumina NovaSeq 6000 sequencer (2 × 150 bp read length).
2.6 Quality control and read mapping
The raw paired-end reads were trimmed and quality controlled by fastp (https://github.com/OpenGene/fastp) with default parameters. Then clean reads were separately aligned to the reference genome (GCF_003789085.1, https://www.ncbi.nlm.nih.gov/assembly/GCF_003789085.1/) with orientation mode using HISAT2 (http://ccb.jhu.edu/software/hisat2/index.shtml) software (Zhang et al., 2019). The mapped reads of each sample were assembled by StringTie (https://ccb.jhu.edu/software/stringtie/) in a reference-based approach.
2.7 Differential expression analysis and functional enrichment
To identify DEGs (differential expression genes) between two different samples/groups, the expression level of each gene was calculated according to the transcripts per million reads (TPM) method. RSEM (http://deweylab.biostat.wisc.edu/rsem/) was used to quantify gene abundances. Essentially, differential expression analysis was performed using the DESeq2, DEGs with |log2(foldchange)| ≥ 1 and P-adjust ≤ 0.05 (DESeq2/edgeR/Limma)/P-adjust ≤ 0.001 (DEGseq)/Prob > 0.8 (NOIseq) were considered to be significantly differentially expressed genes. In addition, functional-enrichment analyses including GO (Gene Ontology, http://www.geneontology.org) and KEGG (Kyoto Encyclopedia of Genes and Genomes, http://www.genome.jp/5eg/) were performed to identify which DEGs were significantly enriched in GO terms and metabolic pathways at P-adjust ≤ 0.05 compared with the whole-transcriptome background. GO functional enrichment and KEGG pathway analysis were carried out by Goatools (https://github.com/tanghaibao/Goatools) and KOBAS (http://kobas.cbi.pku.edu.cn/home.do).
2.8 DEG validation using quantitative real-time PCR
To validate the RNA-Seq data, qRT-PCR was carried out on a LightCycler 96 real-time PCR system (Roche Molecular Systems, Inc) using SYBR Green Mix (Vazyme, China). The information of primers was shown in Table 3, and β-actin was selected as a reference gene. The 2−ΔΔCt method was used to analyze the target gene’s relative expression (fold changes). The amplification efficiency(E) was calculated on standard curves using 10-fold dilutions of cDNA, E=10-1/slope-1. All samples were run in triplicate.
3 Results
3.1 △flhG mutant construction and flagella observation
The flhG gene of V. alginolyticus strain HN08155 has been knocked out successfully by in-frame deletion. The △flhG strain has been verified by PCR assay (data not shown). The external structure of V. alginolyticus strain HN08155 WT and △flhG strains was observed using a transmission electron microscope (TEM) to analyze the impact of the flhG gene on flagella formation. The WT strains have a single flagellum on their poles (Figure 1A), while the flhG mutant strains have several polar flagella to form a hyper flagella structure (Figure 1B).
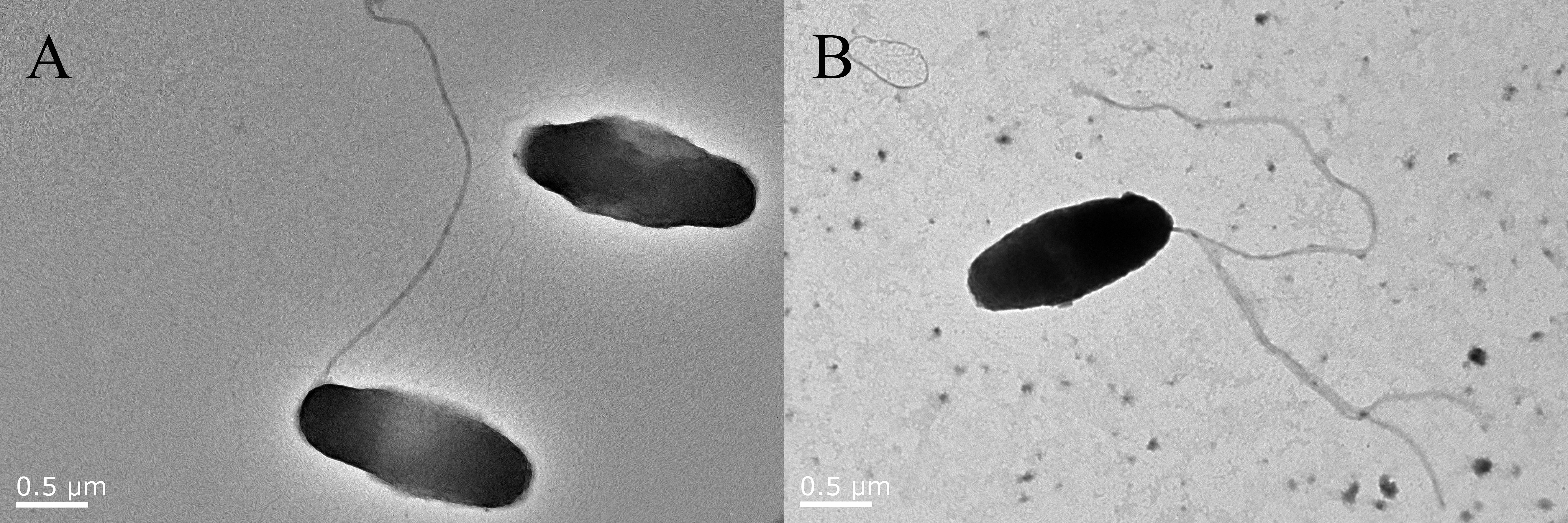
Figure 1 Flagella structure of WT and △flhG strains. V. alginolyticus strain HN08155 flagella structure under TEM scan. (A) WT strain with a single flagellum. (B) △flhG strain with multiple flagella.
3.2 Sequencing and de novo assembly
Six cDNA libraries of hepatopancreas from the HN08155 WT strain infected group and △flhG strain infected group were constructed to perform transcriptome analysis of L. vannamei in response to different V. alginolyticus strains. A total of 307,384,620 clean reads were obtained, with an average mapping rate of 88.08~89.59% to the reference genome and a Q20% higher than 98.4%. The average error rate was 0.024% (Table 4). These results indicate that the sequence data have good quality for further analysis. RNA-Seq data were deposited to the US National Center for Biotechnology Information (NCBI) (accession number PRJNA1030993).
3.3 Functional annotation and classification of unigenes
All unigenes were searched against the GO, KEGG, COG, Nr, Swissprot, and Pfam databases. In this study, 17,291 unigenes were annotated. Among them, a total of 17,291, 9,075, 5,808, 9,184, 13,943, 8,022, and 9,190 unigenes were annotated in the GO, KEGG, COG, Nr, Swissprot, and Pfam database, respectively (Table 5).
3.4 The analysis and functional annotation of the DEGs
The differentially expressed genes of hepatopancreas response to flhG mutant strains (△flhG group) were compared to hepatopancreas response to WT mutant strains (WT group). In total, 104 significant DEGs were found in △flhG vs. WT, containing 52 up-regulated genes and 52 down-regulated genes (Figure 2, Supplementary Table 1). The DEGs were annotated using GO and KEGG databases. The GO enrichment revealed that DEGs between shrimps infected with different strains were significantly enriched in Molecular Function (MF) and Biological Process (BP), especially in the pigment binding term (GO:0031409) (Figure 3). The KEGG enrichment show that DEGs were assigned to 3 special KEGG pathways, including metabolism, organismal systems, and environmental information processing. In metabolism, beta-alanine metabolism, propanoate metabolism, and drug metabolism were the top pathways that enriched most DEGs. In organismal systems, carbohydrate digestion and absorption, protein digestion and absorption, and insulin signaling pathway were the top pathways that enriched most DEGs. In environmental information processing, the DEGs are enriched in the AMPK signaling pathway (Figure 4).
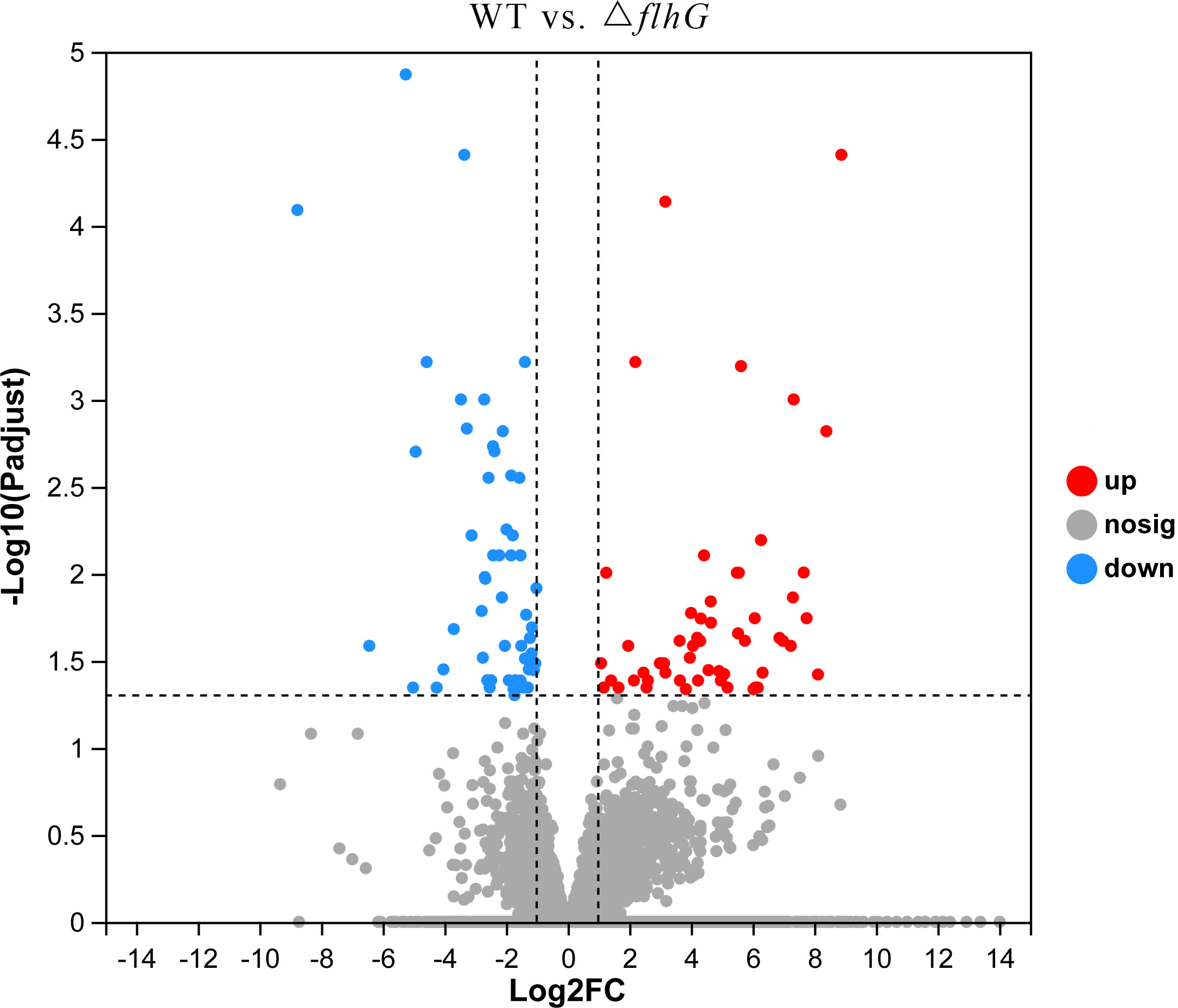
Figure 2 Volcano diagram. Volcano diagram of differentially expressed genes in L. vannamei hepatopancreas, △flhG group vs. WT group. The x-axis indicates the fold change, and the y-axis indicates the statistical significance of the differences.
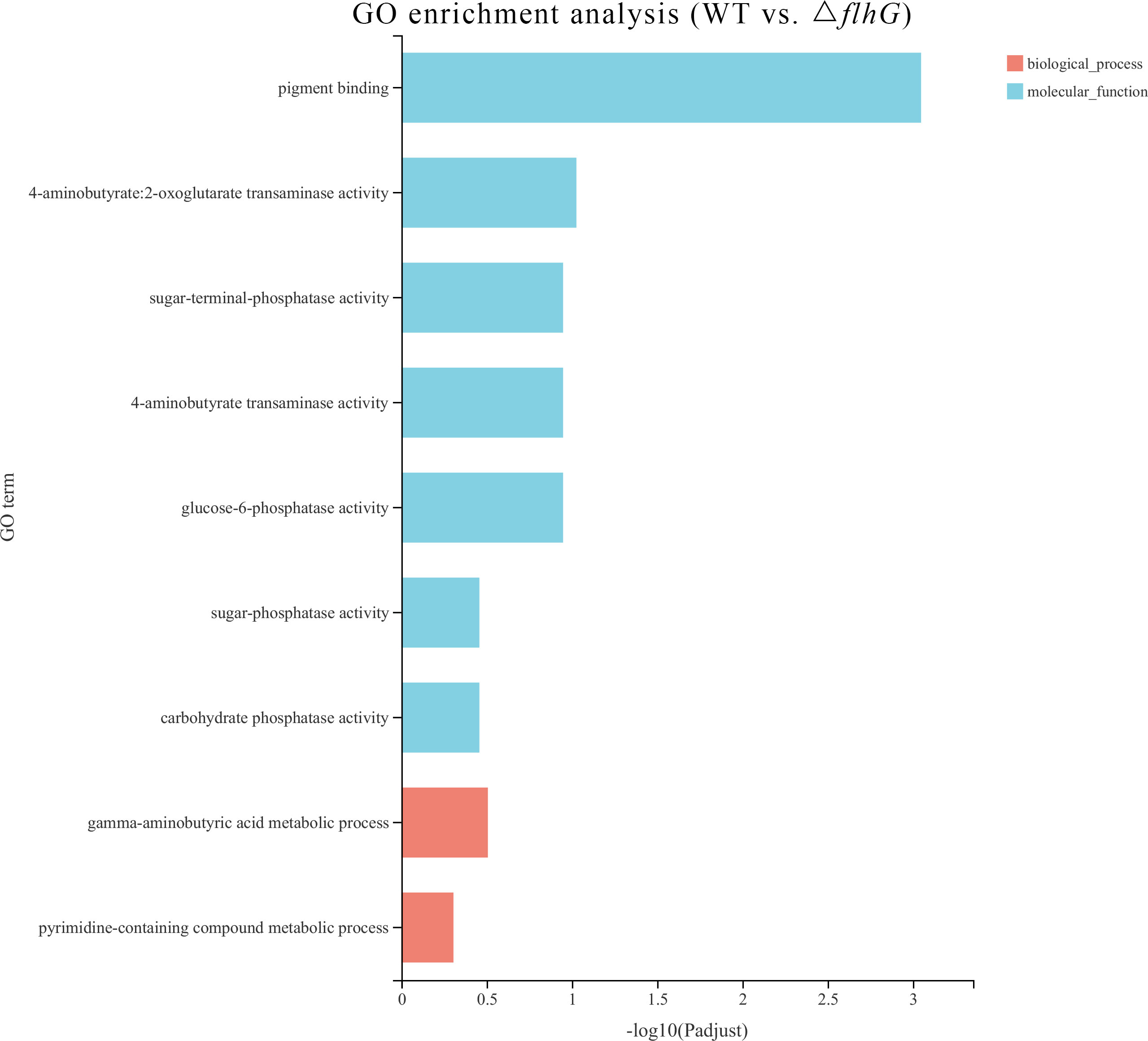
Figure 3 Enriched Gene ontology (GO) terms for DEGs in WT vs. △flhG. The x-axis indicates the significance level of enrichment, and the y-axis indicates the GO term.
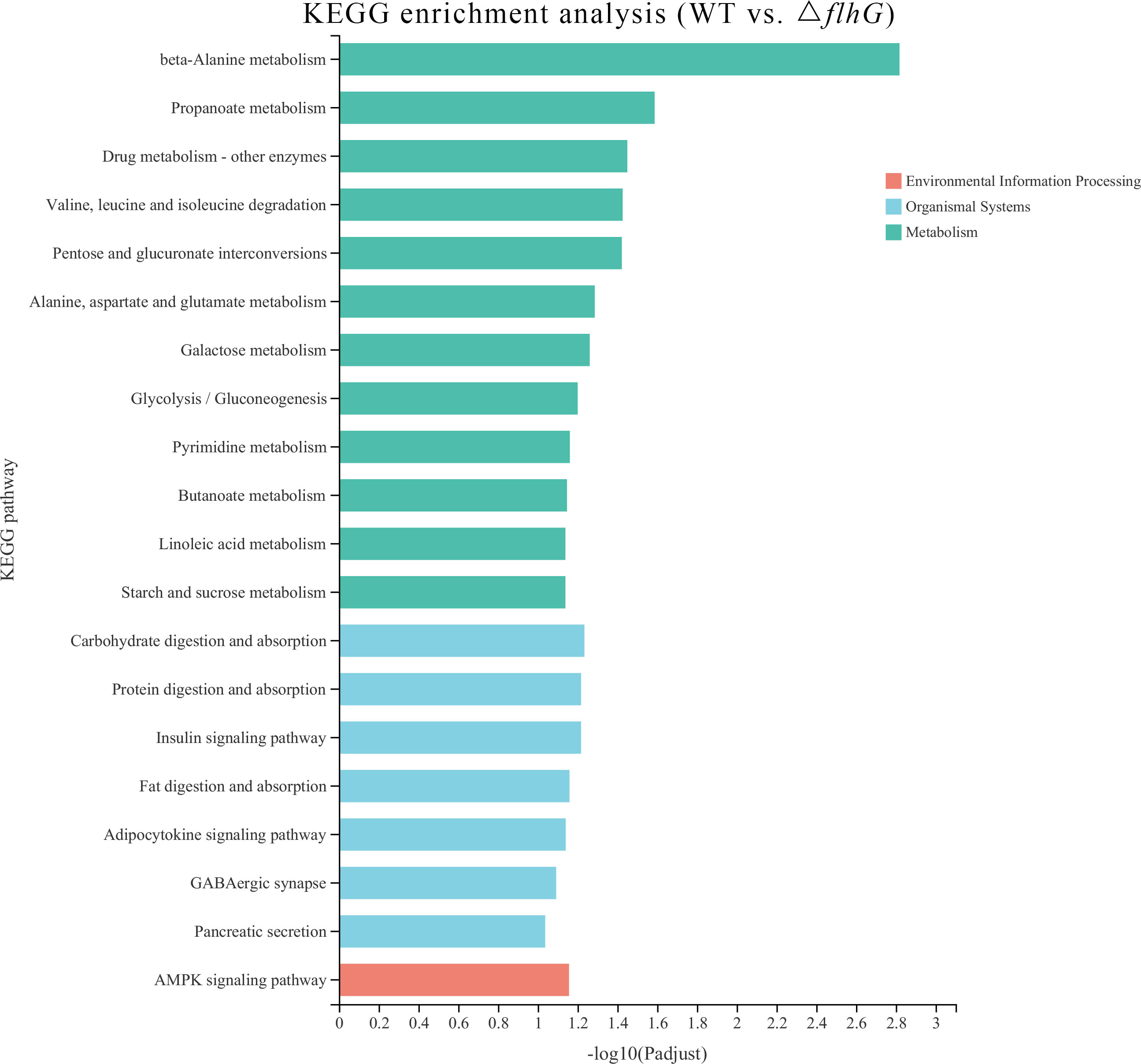
Figure 4 KEGG pathways enriched by the DEGs. The x-axis indicates the significant level of enrichment, and the y-axis indicates the KEGG pathway.
3.5 Transcriptome results validation by qRT-PCR
qRT-PCR were used to verify the DEGs gene expression. The relative expressions of several DEG genes are shown in Figure 5. The expression levels of selected genes were consistent with the RNA-seq data, suggesting that the transcriptome analysis results are reliable.
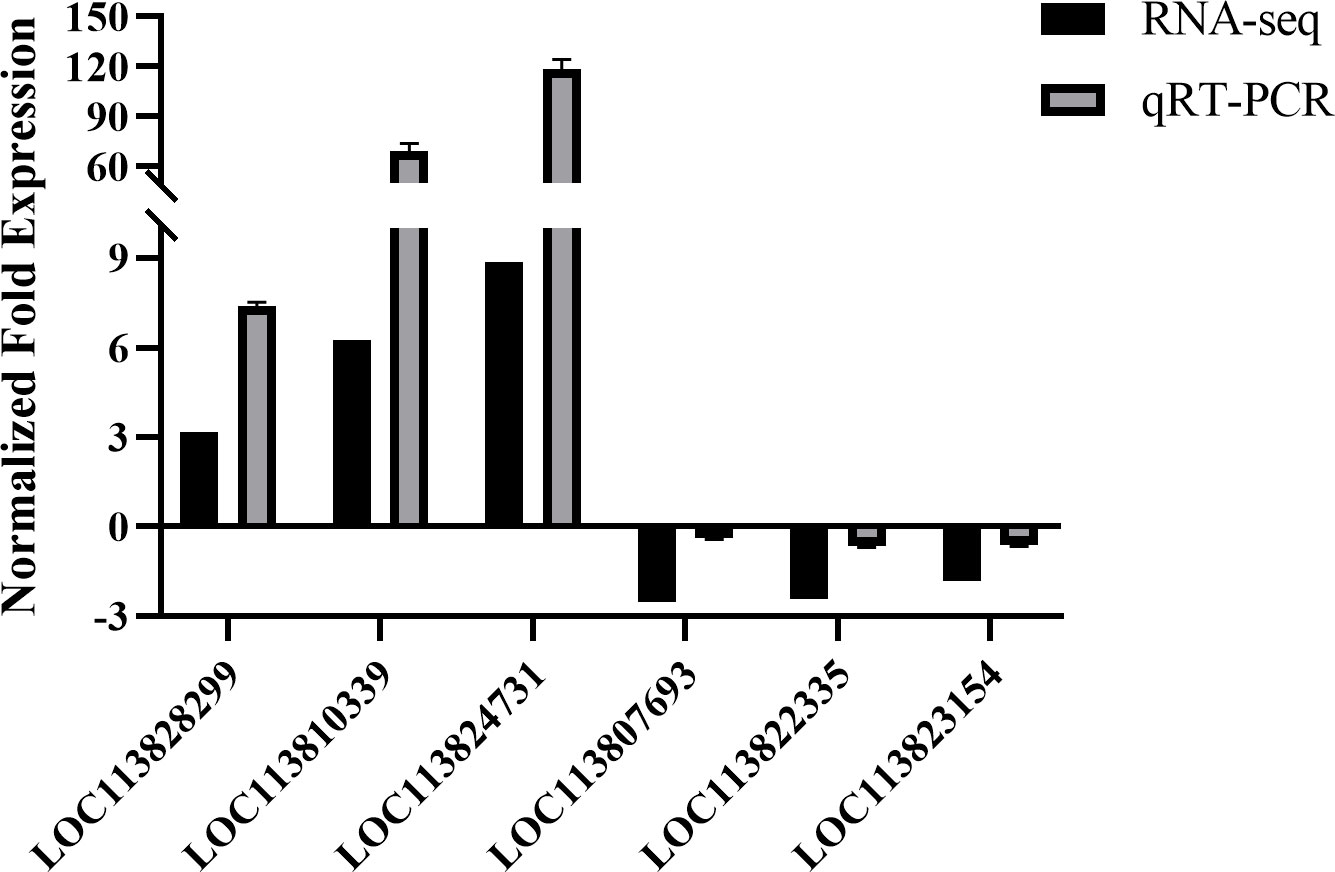
Figure 5 qRT-PCR validation. Comparison of the expression profiles of selected genes as determined by RNA-seq and qRT-PCR.
4 Discussion
Although there is only one polar flagellum on their rod-shaped cells, Vibrio species are commonly mobile in water, and flagellar motility is important for them to survive and infect the host (Zhu et al., 2017; Khan et al., 2020). V. alginolyticus is one of the pathogenic Vibrio spp. commonly found in diseased marine cultured species. In V. alginolyticus the assembly of the polar flagellum is promoted by FlhF and inhibited by FlhG which is a MinD homolog and an ATPase (Ono et al., 2015; Takekawa et al., 2016). FlhG gene-deficient bacteria will lose the negative regulation ability of flagella and result in the hyperflagellated form (Gulbronson et al., 2016). Since flagella is a major PAMP molecules recognized by the immune system, down-regulation of the flagella is one of the strategies used by pathogens to survive inside the host (Chaban et al., 2015). The role of V. alginolyticus flhG in host invasion remains unclear to us. In this research, we deleted the flhG gene in a V. alginolyticus strain HN08155 which was isolated in our previous research from diseased groupers (Xie et al., 2020). The flhG mutant strains of HN08155 exhibit hyper flagella according to our TEM results (Figure 1B), suggesting that the mutants have lost their ability to negatively regulate the number of flagella and might not escape the immune recognition by inhabiting the flagella.
The transcriptome technology is an efficient method to study the pathways or gene expression patterns in shrimps and other invertebrates. Peng et al. studied the molecular mechanism of L. vannamei’s cold tolerance by hepatopancreas transcriptomic analysis (Zhuo et al., 2021). Zhang et al. compared the different gene expressions before and after L. vannamei was infected by decapod iridescent virus 1 (DIV1) and found that TPI-like genes were crucial in DIV1 infection (Liao et al., 2020). Lin et al. also discovered several unique immune-related genes from V. parahaemolyticus infected L. vannamei by RNA-seq (Qin et al., 2018). Although numerous reports have focused on the gene expression changes of shrimps after being challenged with Vibrio spp., the signaling pathways in which the shrimp immune system recognizes the bacteria flagellum and starts the activation are still unknown to us. In this study, we have injected V. alginolyticus strains with different flagella regulation abilities into L. vannamei and explored the different gene expression responses of the shrimp hepatopancreas by transcriptome. The wild-type strains of V. alginolyticus HN08155 have a full flagella regulation function and may possess one or zero polar flagellum after being infected into the host tissue. On the contrary, the flhG mutant strains will have multiple flagella under all conditions. In this case, the different gene expression profiles of the shrimp response to the two types of strains might help us to understand the molecule mechanisms of flagella recognition and signaling transduction in L. vannamei. The transcriptome analysis identified a total of 104 DEGs, with 52 being up-regulated and 52 being down-regulated. Among the DEGs, several immune-related genes have been identified, which might play important roles in pathogen recognition and immune signaling.
C-type lectins (CTLs) are PRRs specifically recognize the sugar residues or motifs present in the glycans of pathogens (Mnich et al., 2020). In shrimp and other invertebrates’ immunity, CTLs play much more important roles than in mammals since the invertebrates lack adaptive immunity and largely depend on innate immunity to detect and combat pathogen invasion. Studies on shrimp C-type lectin also showed that they have multiple antibacterial activities, such as promoting phagocytosis, inhibiting bacterial attachment, regulating downstream immune effectors, and directing antimicrobial activity (Wang et al., 2020). In this research, among the up-regulated DEGs, two lectin-related genes, galactose-specific lectin nattectin-like (LOC113811918) and macrophage mannose receptor 1-like (LOC113819956), were found to increase their mRNA level in the △flhG infected shrimp hepatopancreas. In Thalassophryne nattereri fish, nattectin is involved in the Th1 responses and macrophage differentiation as a signal molecular (Lopes-Ferreira et al., 2011; Saraiva et al., 2011). The agglutinating activity against bacteria of nattectin was found in Misgurnus anguillicaudatus and Carassius auratus (Wang et al., 2017; Zhang et al., 2020). Lv et al. isolated a nattectin from Larimichthys crocea and investigated its role as a PRR in innate immunity (Lv et al., 2016). Another common C-type lectin, mannose receptor (CD206), also can bind to microbial surface glycan structures with terminal fucose, mannose, and N-Acetylglucosamine (GlcNAc) and active various immune cells (Van Der Zande et al., 2021). The mannose receptors of blunt snout bream (Megalobrama amblycephala) can recognize and mediate chitooligosaccharide internalization into macrophages (Ouyang et al., 2021). Xin et al. cloned and analyzed a mannose receptor from red swamp crayfish (Procambarus clarkii), investigating its role in bacteria binding and agglutination (Man et al., 2018). In our results, these two CTL genes’ up-regulated expression might relate to the presence of more flagella compared to the WT type group, suggesting that they have involved in bacteria flagella recognition and pathogen clearance.
The TNF receptor-associated factor (TRAF) 6-like in hepatopancreas also up-regulated significantly after being infected with the hyper flagella strain. TRAF proteins directly bind to the cytoplasmic tail of the tumor necrosis factor (TNF) receptor superfamily (TNFRSF) and there are seven members of the TRAF (TRAF1–7) (Ishida et al., 1996; Yamamoto et al., 2021). TRAF2, 5, and 6 activate nuclear factor-κB (NF-κB) and are involved in the canonical NF-κB pathway (Hayden and Ghosh, 2014). Multiple studies have elucidated TRAF6’s function as a molecular bridge that connects upstream TLRs, MyD88, and IRAKs to the downstream NF-kappa B and MAPK-signaling pathways (Kim and Rikihisa, 2002). In Penaeus monodon, PmTRAF6 up-regulated constantly after being challenged by the white spot syndrome virus (WSSV) but remained unchanged after poly I:C stimulation (Deepika et al., 2014). Zhao et al. reported that MST4 which phosphorylates TRAF6 responded to V. alginolyticus infection and activated the TLR-TRAF6 signaling pathway to increase respiratory burst (RB) activity and decrease the total hemocyte count (THC) in L. vannamei (Zhao et al., 2017). However, according to the studies of Wang et al., the mRNA level of LvTRAF6 in the L. vannamei’s hepatopancreas was at a lower level compared to other tissues and increased by the WSSV infection but unchanged after V. alginolyticus challenge (Wang et al., 2011). These findings imply that V. alginolyticus possesses a mechanism to evade the activation of the TRAF6 pathway. In this study, the transcriptome data show that the flhG mutant strains increased the gene expression of TRAF6 (LOC113810339) significantly than the WT strains of V. alginolyticus HN08155, suggesting that the TRAF6 signaling pathway is vital for the bacteria immune defense in shrimp and can be activated by the flagella.
5 Conclusion
The hepatopancreas of L. vannamei displayed distinct gene expression patterns after infection with a V. alginolyticus mutant strain that possessed constant hyper flagella, achieved through the deletion of the flhG gene, as compared to the wild type strain. Among the differentially expressed genes (DEGs), galactose-specific lectin nattectin and macrophage mannose receptor 1, both belonging to the CTL family, were found to potentially participate in flagella recognition. Additionally, the up-regulation of the TNF receptor-associated factor (TRAF) 6 gene in the hepatopancreas suggested the involvement of the TRAF6 pathway in immune activation.
Data availability statement
The datasets presented in this study can be found in online repositories. The names of the repository/repositories and accession number(s) can be found in the article/Supplementary Material.
Ethics statement
The manuscript presents research on animals that do not require ethical approval for their study.
Author contributions
JZ: Writing – original draft. KL: Data curation, Writing – review & editing. XG: Writing – original draft. NZ: Writing – original draft. YZ: Writing – review & editing. WR: Writing – review & editing. AH: Writing – review & editing. HL: Supervision, Writing – review & editing. ZX: Supervision, Writing – review & editing.
Funding
The author(s) declare financial support was received for the research, authorship, and/or publication of this article. This study was supported financially by the National Natural Science Foundation of China (Grant No. 32060835 and 32260927), and the Natural Science Foundation of Hainan Province (Grant No. 2019RC106).
Acknowledgments
The data were analyzed on the online platform of Majorbio Cloud Platform (www.majorbio.com).
Conflict of interest
The authors declare that the research was conducted in the absence of any commercial or financial relationships that could be construed as a potential conflict of interest.
Publisher’s note
All claims expressed in this article are solely those of the authors and do not necessarily represent those of their affiliated organizations, or those of the publisher, the editors and the reviewers. Any product that may be evaluated in this article, or claim that may be made by its manufacturer, is not guaranteed or endorsed by the publisher.
Supplementary material
The Supplementary Material for this article can be found online at: https://www.frontiersin.org/articles/10.3389/fcimb.2023.1265917/full#supplementary-material
References
Baker-Austin, C., Oliver, J. D., Alam, M., Ali, A., Waldor, M. K., Qadri, F., et al. (2018). Vibrio spp. infections. Nat. Rev. Dis. Primers 4, 8. doi: 10.1038/s41572-018-0005-8
Chaban, B., Hughes, H. V., Beeby, M. (2015). The flagellum in bacterial pathogens: For motility and a whole lot more. Semin. Cell Dev. Biol. 46, 91–103. doi: 10.1016/j.semcdb.2015.10.032
Deepika, A., Sreedharan, K., Paria, A., Makesh, M., Rajendran, K. V. (2014). Toll-pathway in tiger shrimp (Penaeus monodon) responds to white spot syndrome virus infection: evidence through molecular characterisation and expression profiles of MyD88, TRAF6 and TLR genes. Fish Shellfish Immunol. 41, 441–454. doi: 10.1016/j.fsi.2014.09.026
Duan, Q., Zhou, M., Zhu, L., Zhu, G. (2013). Flagella and bacterial pathogenicity. J. Basic Microbiol. 53, 1–8. doi: 10.1002/jobm.201100335
Eriksson, S., Lucchini, S., Thompson, A., Rhen, M., Hinton, J. C. (2003). Unravelling the biology of macrophage infection by gene expression profiling of intracellular Salmonella enterica. Mol. Microbiol. 47, 103–118. doi: 10.1046/j.1365-2958.2003.03313.x
Feuillet, V., Medjane, S., Mondor, I., Demaria, O., Pagni, P. P., Galan, J. E., et al. (2006). Involvement of Toll-like receptor 5 in the recognition of flagellated bacteria. Proc. Natl. Acad. Sci. U.S.A. 103, 12487–12492. doi: 10.1073/pnas.0605200103
Fitzgerald, K. A., Kagan, J. C. (2020). Toll-like receptors and the control of immunity. Cell 180, 1044–1066. doi: 10.1016/j.cell.2020.02.041
Foysal, M. J., Momtaz, F., Kawser, A., Ali, M. H., Raihan, T., Siddik, M., et al. (2021). Amplicon sequencing reveals significantly increased Vibrio abundance and associated gene functions in vibriosis-infected black tiger shrimp (Penaeus monodon). J. Fish Dis. 44, 591–599. doi: 10.1111/jfd.13304
Gauger, E. J., Leatham, M. P., Mercado-Lubo, R., Laux, D. C., Conway, T., Cohen, P. S. (2007). Role of motility and the flhDC Operon in Escherichia coli MG1655 colonization of the mouse intestine. Infect. Immun. 75, 3315–3324. doi: 10.1128/IAI.00052-07
Gulbronson, C. J., Ribardo, D. A., Balaban, M., Knauer, C., Bange, G., Hendrixson, D. R. (2016). FlhG employs diverse intrinsic domains and influences FlhF GTPase activity to numerically regulate polar flagellar biogenesis in Campylobacter jejuni. Mol. Microbiol. 99, 291–306. doi: 10.1111/mmi.13231
Habib, Y. J., Zhang, Z. (2020). The involvement of crustaceans toll-like receptors in pathogen recognition. Fish Shellfish Immunol. 102, 169–176. doi: 10.1016/j.fsi.2020.04.035
Hayden, M. S., Ghosh, S. (2014). Regulation of NF-kappaB by TNF family cytokines. Semin. Immunol. 26, 253–266. doi: 10.1016/j.smim.2014.05.004
Ishida, T., Mizushima, S., Azuma, S., Kobayashi, N., Tojo, T., Suzuki, K., et al. (1996). Identification of TRAF6, a novel tumor necrosis factor receptor-associated factor protein that mediates signaling from an amino-terminal domain of the CD40 cytoplasmic region. J. Biol. Chem. 271, 28745–28748. doi: 10.1074/jbc.271.46.28745
Janda, J. M., Newton, A. E., Bopp, C. A. (2015). Vibriosis. Clin. Lab. Med. 35, 273–288. doi: 10.1016/j.cll.2015.02.007
Khan, F., Tabassum, N., Anand, R., Kim, Y. M. (2020). Motility of Vibrio spp.: regulation and controlling strategies. Appl. Microbiol. Biotechnol. 104, 8187–8208. doi: 10.1007/s00253-020-10794-7
Kim, H. Y., Rikihisa, Y. (2002). Roles of p38 mitogen-activated protein kinase, NF-kappaB, and protein kinase C in proinflammatory cytokine mRNA expression by human peripheral blood leukocytes, monocytes, and neutrophils in response to Anaplasma phagocytophila. Infect. Immun. 70, 4132–4141. doi: 10.1128/IAI.70.8.4132-4141.2002
Kumar, H., Kawai, T., Akira, S. (2009). Pathogen recognition in the innate immune response. Biochem. J. 420, 1–16. doi: 10.1042/BJ20090272
Kumar, H., Kawai, T., Akira, S. (2011). Pathogen recognition by the innate immune system. Int. Rev. Immunol. 30, 16–34. doi: 10.3109/08830185.2010.529976
Kumar, V., Roy, S., Behera, B. K., Bossier, P., Das, B. K. (2021). Acute hepatopancreatic necrosis disease (AHPND): virulence, pathogenesis and mitigation strategies in shrimp aquaculture. Toxins (Basel) 13 (8), 524. doi: 10.3390/toxins13080524
Li, X., Wang, Y., Li, H., Jiang, X., Ji, L., Liu, T., et al. (2021). Chemical and quality evaluation of Pacific white shrimp Litopenaeus vannamei: Influence of strains on flesh nutrition. Food Sci. Nutr. 9, 5352–5360. doi: 10.1002/fsn3.2457
Li, X., Yu, M., Zhu, M. (2009). Innate immune signaling pathways in animals: beyond reductionism. Int. Rev. Immunol. 28, 207–238. doi: 10.1080/08830180902839777
Liao, X., Wang, C., Wang, B., Qin, H., Hu, S., Wang, P., et al. (2020). Comparative transcriptome analysis of litopenaeus vannamei reveals that triosephosphate isomerase-like genes play an important role during decapod iridescent virus 1 infection. Front. Immunol. 11. doi: 10.3389/fimmu.2020.01904
Lopes-Ferreira, M., Magalhaes, G. S., Fernandez, J. H., Junqueira-De-Azevedo Ide, L., Le Ho, P., Lima, C., et al. (2011). Structural and biological characterization of Nattectin, a new C-type lectin from the venomous fish Thalassophryne nattereri. Biochimie 93, 971–980. doi: 10.1016/j.biochi.2011.03.001
Lv, C., Zhang, D., Wang, Z. (2016). A novel C-type lectin, Nattectin-like protein, with a wide range of bacterial agglutination activity in large yellow croaker Larimichthys crocea. Fish Shellfish Immunol. 50, 231–241. doi: 10.1016/j.fsi.2016.01.032
Man, X., Pan, X. T., Zhang, H. W., Wang, Y., Li, X. C., Zhang, X. W. (2018). A mannose receptor is involved in the anti-Vibrio defense of red swamp crayfish. Fish Shellfish Immunol. 82, 258–266. doi: 10.1016/j.fsi.2018.08.021
Medzhitov, R. (2007). Recognition of microorganisms and activation of the immune response. Nature 449, 819–826. doi: 10.1038/nature06246
Mnich, M. E., Van Dalen, R., Van Sorge, N. M. (2020). C-type lectin receptors in host defense against bacterial pathogens. Front. Cell Infect. Microbiol. 10. doi: 10.3389/fcimb.2020.00309
Munkongwongsiri, N., Prachumwat, A., Eamsaard, W., Lertsiri, K., Flegel, T. W., Stentiford, G. D., et al. (2022). Propionigenium and Vibrio species identified as possible component causes of shrimp white feces syndrome (WFS) associated with the microsporidian Enterocytozoon hepatopenaei. J. Invertebr. Pathol. 192, 107784. doi: 10.1016/j.jip.2022.107784
Ngo, H. V., Huang, H. T., Lee, P. T., Liao, Z. H., Chen, H. Y., Nan, F. H. (2020). Effects of Phyllanthus amarus extract on nonspecific immune responses, growth, and resistance to Vibrio alginolyticus in white shrimp Litopenaeus vannamei. Fish Shellfish Immunol. 107, 1–8. doi: 10.1016/j.fsi.2020.09.016
Ono, H., Takashima, A., Hirata, H., Homma, M., Kojima, S. (2015). The MinD homolog FlhG regulates the synthesis of the single polar flagellum of Vibrio alginolyticus. Mol. Microbiol. 98, 130–141. doi: 10.1111/mmi.13109
Ouyang, A., Wang, H., Su, J., Liu, X. (2021). Mannose receptor mediates the activation of chitooligosaccharides on blunt snout bream (Megalobrama amblycephala) macrophages. Front. Immunol. 12. doi: 10.3389/fimmu.2021.686846
Qin, Z., Babu, V. S., Wan, Q., Zhou, M., Liang, R., Muhammad, A., et al. (2018). Transcriptome analysis of Pacific white shrimp (Litopenaeus vannamei) challenged by Vibrio parahaemolyticus reveals unique immune-related genes. Fish Shellfish Immunol. 77, 164–174. doi: 10.1016/j.fsi.2018.03.030
Rossez, Y., Wolfson, E. B., Holmes, A., Gally, D. L., Holden, N. J. (2015). Bacterial flagella: twist and stick, or dodge across the kingdoms. PloS Pathog. 11, e1004483. doi: 10.1371/journal.ppat.1004483
Saraiva, T. C., Grund, L. Z., Komegae, E. N., Ramos, A. D., Conceicao, K., Orii, N. M., et al. (2011). Nattectin a fish C-type lectin drives Th1 responses in vivo: licenses macrophages to differentiate into cells exhibiting typical DC function. Int. Immunopharmacol. 11, 1546–1556. doi: 10.1016/j.intimp.2011.05.012
Shen, H., Song, T., Lu, J., Qiu, Q., Chen, J., Xiong, J. (2021). Shrimp AHPND causing vibrio Anguillarum infection: quantitative diagnosis and identifying antagonistic bacteria. Mar. Biotechnol. (NY) 23, 964–975. doi: 10.1007/s10126-021-10079-8
Takekawa, N., Kwon, S., Nishioka, N., Kojima, S., Homma, M. (2016). HubP, a polar landmark protein, regulates flagellar number by assisting in the proper polar localization of flhG in vibrio alginolyticus. J. Bacteriol. 198, 3091–3098. doi: 10.1128/JB.00462-16
Van Der Zande, H. J. P., Nitsche, D., Schlautmann, L., Guigas, B., Burgdorf, S. (2021). The mannose receptor: from endocytic receptor and biomarker to regulator of (Meta)Inflammation. Front. Immunol. 12. doi: 10.3389/fimmu.2021.765034
Wang, L., Zhang, J., Kong, X., Zhao, X., Pei, C., Li, L. (2017). A C-type lectin, Nattectin-like protein (CaNTC) in Qihe crucian carp Carassius auratus: Binding ability with LPS, PGN and various bacteria, and agglutinating activity against bacteria. Fish Shellfish Immunol. 67, 382–392. doi: 10.1016/j.fsi.2017.06.012
Wang, P. H., Wan, D. H., Gu, Z. H., Deng, X. X., Weng, S. P., Yu, X. Q., et al. (2011). Litopenaeus vannamei tumor necrosis factor receptor-associated factor 6 (TRAF6) responds to Vibrio alginolyticus and white spot syndrome virus (WSSV) infection and activates antimicrobial peptide genes. Dev. Comp. Immunol. 35, 105–114. doi: 10.1016/j.dci.2010.08.013
Wang, X. W., Vasta, G. R., Wang, J. X. (2020). The functional relevance of shrimp C-type lectins in host-pathogen interactions. Dev. Comp. Immunol. 109, 103708. doi: 10.1016/j.dci.2020.103708
Xie, Z. Y., Gong, X. X., Xu, X. D., Mei, B., Xuan, X. Z., Long, H., et al. (2020). Identification of Vibrio alginolyticus virulent strain-specific DNA regions by suppression subtractive hybridization and PCR. J. Appl. Microbiol. 129, 1472–1485. doi: 10.1111/jam.14739
Yamamoto, M., Gohda, J., Akiyama, T., Inoue, J. I. (2021). TNF receptor-associated factor 6 (TRAF6) plays crucial roles in multiple biological systems through polyubiquitination-mediated NF-kappaB activation. Proc. Jpn Acad. Ser. B Phys. Biol. Sci. 97, 145–160. doi: 10.2183/pjab.97.009
Yu, Y. B., Choi, J. H., Kang, J. C., Kim, H. J., Kim, J. H. (2022). Shrimp bacterial and parasitic disease listed in the OIE: A review. Microb. Pathog. 166, 105545. doi: 10.1016/j.micpath.2022.105545
Zhang, X. W., Yang, C. H., Zhang, H. Q., Pan, X. T., Jin, Z. Y., Zhang, H. W., et al. (2020). A C-type lectin with antibacterial activity in weather loach, Misgurnus anguillicaudatus. J. Fish Dis. 43, 1531–1539. doi: 10.1111/jfd.13255
Zhang, X., Yuan, J., Sun, Y., Li, S., Gao, Y., Yu, Y., et al. (2019). Penaeid shrimp genome provides insights into benthic adaptation and frequent molting. Nat. Commun. 10, 356. doi: 10.1038/s41467-018-08197-4
Zhao, C. S., Huang, D., Peng, T., Huang, M. Z., Xie, C. Y., Chen, J., et al. (2017). Molecular cloning, characterization and function of a germinal center kinase MST4 gene from Litopenaeus vannamei in response to Vibrio alginolyticus challenge in TLR-TRAF6 signaling pathway. Dev. Comp. Immunol. 73, 206–219. doi: 10.1016/j.dci.2017.03.026
Zhao, Y., Shao, F. (2015). The NAIP-NLRC4 inflammasome in innate immune detection of bacterial flagellin and type III secretion apparatus. Immunol. Rev. 265, 85–102. doi: 10.1111/imr.12293
Zhu, S., Nishikino, T., Hu, B., Kojima, S., Homma, M., Liu, J. (2017). Molecular architecture of the sheathed polar flagellum in Vibrio alginolyticus. Proc. Natl. Acad. Sci. U.S.A. 114, 10966–10971. doi: 10.1073/pnas.1712489114
Keywords: Vibrio alginolyticus, flagella, flhG, Litopenaeus vannamei, transcriptome
Citation: Zhang J, Liu K, Gong X, Zhang N, Zeng Y, Ren W, Huang A, Long H and Xie Z (2023) Transcriptome analysis of the hepatopancreas from the Litopenaeus vannamei infected with different flagellum types of Vibrio alginolyticus strains. Front. Cell. Infect. Microbiol. 13:1265917. doi: 10.3389/fcimb.2023.1265917
Received: 24 July 2023; Accepted: 06 November 2023;
Published: 21 November 2023.
Edited by:
Jose Ramos-Vivas, Universidad Europea del Atlántico, SpainReviewed by:
Maria M. Costa, Instituto de Investigaciones Marinas, SpainMengqiang Wang, Ocean University of China, China
Copyright © 2023 Zhang, Liu, Gong, Zhang, Zeng, Ren, Huang, Long and Xie. This is an open-access article distributed under the terms of the Creative Commons Attribution License (CC BY). The use, distribution or reproduction in other forums is permitted, provided the original author(s) and the copyright owner(s) are credited and that the original publication in this journal is cited, in accordance with accepted academic practice. No use, distribution or reproduction is permitted which does not comply with these terms.
*Correspondence: Hao Long, longhao@hainanu.edu.cn; Zhenyu Xie, xiezyscuta@163.com
†These authors have contributed equally to this work