First detection of Toxoplasma gondii Africa 4 lineage in a population of carnivores from South Africa
- 1Department of Epizootology, Parasitology and Protection of One Health, University of Veterinary Medicine and Pharmacy in Košice, Košice, Slovakia
- 2Department of Biology and Wildlife Diseases, Faculty of Veterinary Hygiene and Ecology, University of Veterinary Sciences Brno, Brno, Czechia
- 3Inserm U1094, IRD U270, CHU Limoges, EpiMaCT - Epidémiologie des Maladies Chroniques en zone Tropicale, Institut d’Epidémiologie et de Neurologie Tropicale, OmegaHealth, University of Limoges, Limoges, France
- 4Department of Scientific Services, Mpumalanga Tourism & Parks Agency (MTPA), Nelspruit, South Africa
- 5Research Administration and Development, University of Limpopo, Sovenga, South Africa
Introduction: There have only been a few molecular studies conducted on the detection of T. gondii in tissues of carnivores in South Africa, with no data on the genetic diversity of this parasite. That is why the aim of this study was to detect and genotype T. gondii DNA in tissues of selected wild and domestic carnivores in South Africa.
Methods: Samples were collected from 80 animals of 20 species (mainly road-killed) in the four provinces of Limpopo (n=57), Mpumalanga (n=21), Gauteng (n=1) and Free State (n=1) during the period 2014–2018. Samples of brain (n=31), heart (n=4), liver (n=40), spleen (n=2) and lung (n=3) were used to detect T. gondii by real-time PCR targeting a 529 bp repeating fragment of T. gondii DNA. Samples that were positive in real-time PCR were genotyped using 15 microsatellite markers.
Results: T. gondii DNA was detected in 4 (5 %) samples: in the brain from a Black-backed Jackal (Canis mesomelas), in the liver from a African Wildcat (Felis silvestris lybica) and in the liver and heart of two Rusty-spotted Genets (Genetta maculata) respectively. The DNA sample from Black-backed Jackal was genotyped and characterized as belonging to the type Africa 4 lineage (equivalent to RFLP genotype ToxoDB#20), that is a widespread lineage in Africa.
Discussion: This is the first genetic characterization of T. gondii isolated from a wild carnivore on the African continent and the first report of T. gondii in Black-backed Jackal. The Africa 4 lineage was also confirmed in the region of Southern Africa for the first time.
1 Introduction
Toxoplasmosis, one of the most important zoonoses, widespread globally in humans as well as in most warm-blooded animal species, significantly affects the health of animals, human and ecosystems, hence one health concern (Aguirre et al., 2019). In South Africa, the high prevalence of immunosuppressive infections [17 % of the global incidence of Human Immunodeficiency Virus (HIV)] poses a high risk of opportunistic infections, such that caused by Toxoplasma gondii, to the human population (Hammond-Aryee et al., 2015; Ngobeni and Samie, 2017). In Africa, data on genetic diversity of Toxoplasma gondii and clinical forms of toxoplasmosis are limited (Mercier et al., 2010; Galal et al., 2018; Galal et al., 2019a; Galal et al., 2019b). However, in West and Central Africa, it has been shown that ocular toxoplasmosis can be a significant health problem (Abu et al., 2016; Nsiangani Lusambo et al., 2023). In addition, recent studies in France have described cases of severe toxoplasmosis imported from tropical Africa in immunocompetent patients and associated in some cases with strains belonging to the Africa 1 lineage (Beltrame et al., 2016; Gachet et al., 2018; Leroy et al., 2019; Artiaga et al., 2022). To advance our knowledge of the genetic diversity of T. gondii it is essential to characterize genotypes of circulating T. gondii strains, especially given the potential relationship between pathogenicity in humans and animals and the genotype of the infecting strains (Dardé, 2008; Sánchez et al., 2014; Xiao and Yolken, 2015; Tenter et al., 2000). In addition, as Felidae are the only animals able to shed the oocyst into the environment and therefore be a source of T. gondii infection for both humans and livestock (Adesiyun et al., 2020), the diversity and abundance of wild felines in Africa underlines the need to conduct surveys of T. gondii in wildlife.
To date, there have been very few reports on the occurrence of T. gondii in wild carnivores in South Africa and neighboring countries, those being serological studies, focusing on the detection of antibodies against T. gondii, mainly in wild and domestic felids (Penzhorn et al., 2002; Lobetti and Lappin, 2012; Serieys et al., 2019; Seltmann et al., 2020). Only one study has been conducted in South Africa to detect T. gondii DNA in carnivores and other wild and domestic mammalian species, without providing data on the parasite genotype involved (Lukášová et al., 2018a). Clonal Type II is the most prevalent genotype in Europe, followed by a significantly lower prevalence of Type III genotype and rare occurrence of Type I genotype (Fernández-Escobar et al., 2022). Several genetic studies in human and animals from Africa also suggest a clonal structure of the T. gondii population, with archetypal Type II and Type III lineages along with indigenous ones, such as Africa 1, Africa 3 and Africa 4 (Galal et al., 2018; Hamidović et al., 2021; Galal et al., 2022). In South Africa, few data exist on the circulating T. gondii strains with only 10 strains being genotyped, all belonging to the Type II lineage (Lukášová et al., 2018a; Shwab et al., 2018). Moreover, there are almost no data regarding T. gondii strains circulating in wildlife on the whole of the African continent (Galal et al., 2018).
That is why the aim of this study was to detect T. gondii in wild and domestic carnivores from South Africa by molecular methods, and genotype positive samples by microsatellite markers analysis.
2 Materials and methods
2.1 Animals and sampling
Tissue samples were collected from 80 wild and domestic carnivores found dead in four provinces of South Africa including Limpopo (n = 57), Mpumalanga (n = 21), Gauteng (n = 1) and Free State (n = 1) in the years 2014–2018. The animals were mostly roadkills (or found dead of natural causes), and included 20 species from 6 families (Table 1). The carcasses were transported to the Laboratory of Parasitology, University of Limpopo. Necropsy was performed and various tissue samples were collected according to availability (as most carcasses were roadkills, some organs were badly damaged or poorly preserved), including brain (n=31), liver (n=40), heart (n=4), spleen (n=2) and lung (n=3). The collected samples were stored at -80 °C until testing.
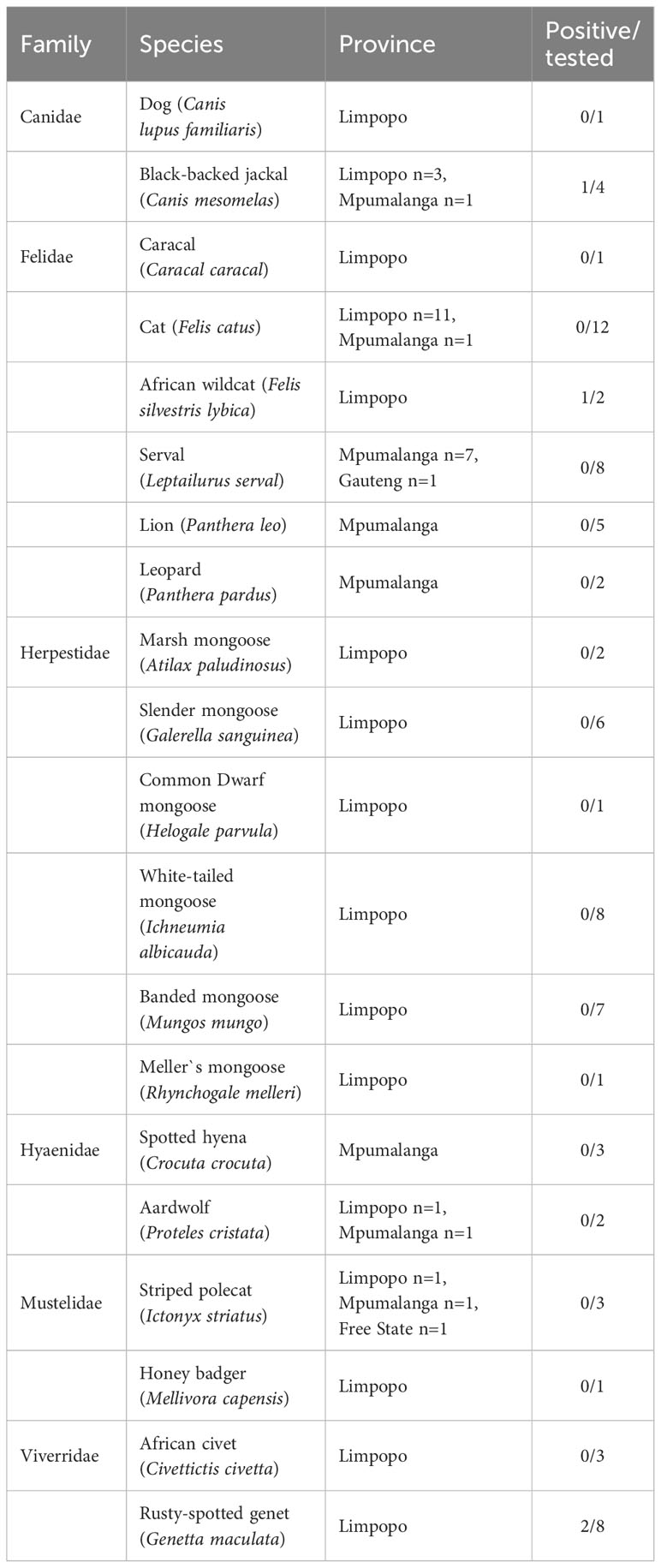
Table 1 Results of Toxoplasma gondii detection by qPCR in tissues of 80 carnivores found dead in South Africa.
2.2 Molecular analysis
Tissue samples of 1-2 g were crushed and homogenized, using the Precellys 24 homogenizer (Bertin Instruments, Bretoneux, France). The DNA was extracted by the DNeasy Blood & Tissue commercial kit (QIAGEN, Hilden, Germany), according to the manufacturer´s instructions. To assess the presence of T. gondii DNA in the tissues of the investigated animals, real-time PCR targeting the non-coding repeated element rep529 (GenBank accession no. AF146527) was used as previously described (Homan et al., 2000; Ajzenberg et al., 2016; Galal et al., 2019c). Multiplex PCR of 15 microsatellite (MS) markers was performed as described previously (Ajzenberg et al., 2010) using capillary electrophoresis on ABI PRISM 3130x1 (Applied Biosystems, Foster City, CA) on the real-time PCR positive samples. The results were analyzed with GeneMapper software (version 54.0; Applied Biosystems) to estimate the size of alleles (base pairs). Among the 15 microsatellite loci used, some are not very polymorphic and are more useful for strain typing (lineage characterization) (TUB2, W35, TgM-A, B18, B17, M33, IV.1 and XI.1), while others are highly polymorphic and can be used as “fingerprinting markers” to distinguish strains within the same lineage (M48, M102, N60, N82, AA, N6 and N83). Multilocus genotypes (MLG) from this study were compared with reference strains described in previous studies (Supplementary Table 1) to enable assignment.
2.3 Construction of a neighbour-joining tree
In order to analyze the position of our strain within T. gondii genetic diversity from the African continent, a Neighbor -Joining tree was reconstructed using Populations 1.2.32 software (1999, Olivier Langella, CNRS UPR9034 http://bioinformatics.org/~tryphon/populations/) including Benin (Hamidović et al., 2021), Senegal (Galal et al., 2019a; Galal et al., 2019b), Tunisia (Lachkhem et al., 2021), Egypt (Al-Kappany et al., 2010) and Gabon (Mercier et al., 2010). To do this, genetic distances based on Cavalli-Sforza and Edwards chord distance estimator (Cavalli-Sforza and Edwards, 1967) between unique haplotypes were calculated and used to reconstruct a Neighbour-Joining Tree generated with MEGA 6.05 (http://www.megasoftware.net/history.php). The genotype found in the present study, the African strain genotypes selected for comparison and the reference strains used to construct this distance-based tree are listed in Supplementary Table 1.
3 Results
Of the total of 80 analyzed samples, T. gondii DNA was detected in tissues of four carnivores (5 %), specifically in the brain of a Black-backed Jackal (Canis mesomelas), in the liver of an African Wildcat (Felis silvestris lybica) and in the liver and heart of two Rusty-spotted Genets (Genetta maculata) respectively, all sampled in the province of Limpopo (Table 1). Only T. gondii DNA isolated from the Black-backed Jackal was successfully genotyped and characterized as belonging to the Africa 4 lineage equivalent to RFLP genotype ToxoDB#20 (Table 2). A single fingerprinting marker (N61) was missing without affecting the assignment of the DNA isolate. The Africa 4 lineage is presented in the Neighbour-Joining Tree (Figure 1) through a well-defined cluster with strains from several African countries, except Gabon. The genotype reported here blends perfectly with Africa 4 genotypes from Senegal, Benin, Tunisia and Egypt.

Table 2 Result of genotyping (using 15 microsatellite markers) of Toxoplasma gondii isolated from the brain of PCR positive samples from Black- backed Jackal (Canis mesomeles) in South Africa.
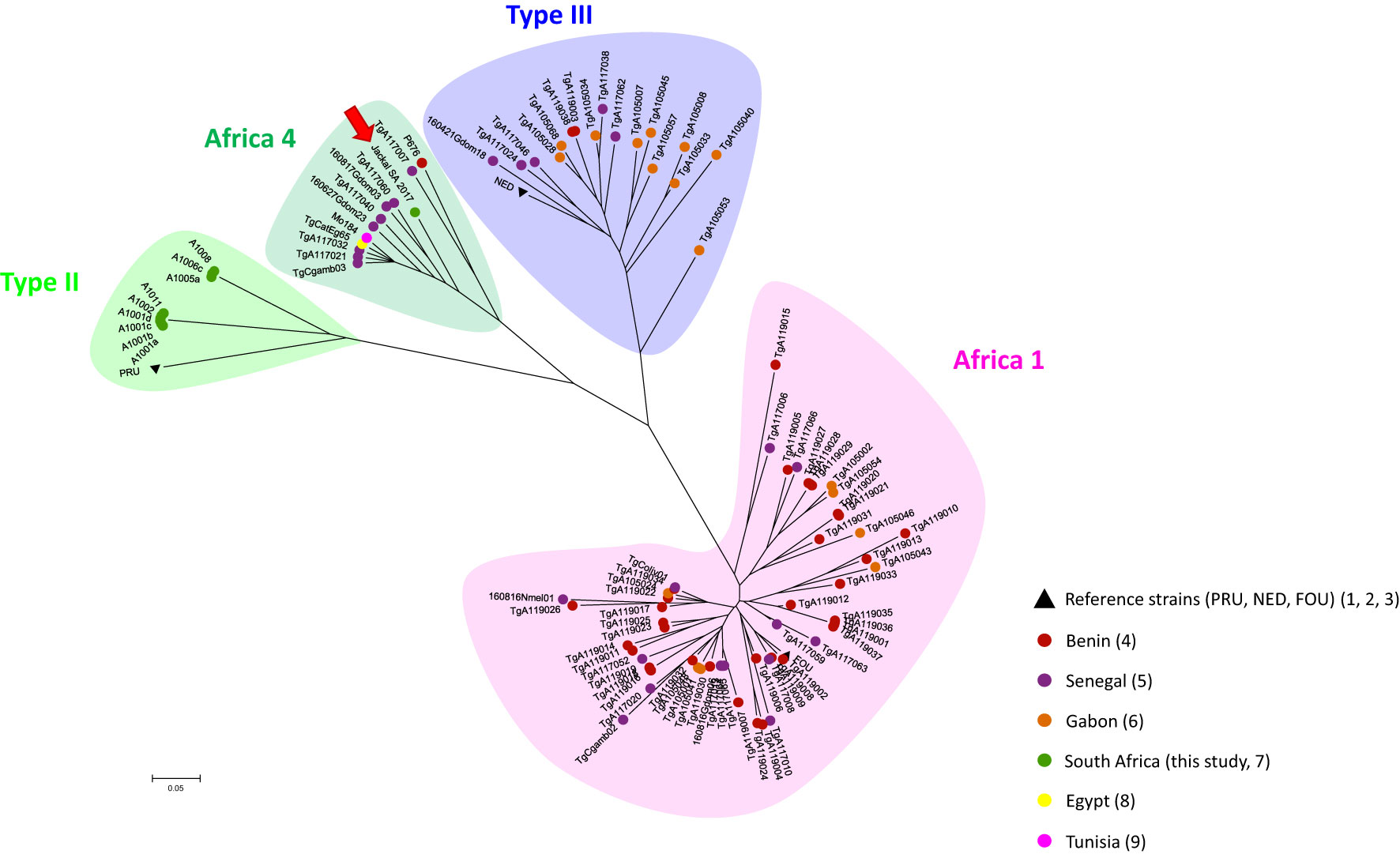
Figure 1 Global Neighbour-joining Tree of T. gondii genotypes from Senegal, Gabon, Benin, Tunisia, Egypt and South Africa, inferred from calculated Cavalli-Sforza distances for 15 microsatellite markers. .
4 Discussion
Previous investigations of T. gondii in carnivores in South Africa and neighboring countries have focused mainly on the detection of antibodies specific to T. gondii (Bokaba et al., 2022; Omonijo et al., 2022). These studies have shown a significant circulation of the parasite in both wild and domestic environments, with expectedly a higher prevalence in carnivores than in herbivores (Bokaba et al., 2022). However, qPCR-based results are particularly valuable in wildlife studies, as validated serological tests are often not available for the host species under study.
In our study, T. gondii DNA was found in 4 of the 80 (5 %) carnivores tested, specifically in one Black-backed Jackal, in one African Wildcat and in two Rusty-spotted Genets. A previous study based on the detection of T. gondii DNA in carnivores and other mammalian species from South Africa, based on a larger sample (n= 243 brain samples), found a relatively similar percentage of positive individuals (2%) (Lukášová et al., 2018a), despite differences in PCR techniques used and in the number and the nature of the samples between the two studies. In our study, only one organ per individual animal was investigated, sometimes including organs less known for their parasitic tropism than brain and heart.
The T. gondii DNA isolated from the brain of the Black-backed Jackal (Ct = 27,40) was successfully genotyped as belonging to the Africa 4 lineage. This lineage corresponds to 2 RFLP genotypes, ToxoDB#20 and #137, which can be differentiated by the MS typing locus TUB2: 219 bp and 293 bp respectively (Galal et al., 2019c; Galal et al., 2022). This analysis revealed that the Africa 4 genotype detected in the Back-backed Jackal corresponds to ToxoDB#20 (Table 2, Supplementary Table 1). The DNA from the liver of the African Wildcat and the liver and heart of two Rusty-spotted Genets, respectively, could not be successfully genotyped, probably due to the low concentration of T. gondii DNA (Ct > 32). This observation was recently confirmed in a study on the optimization of the microsatellite genotyping technique for T. gondii (Joeres et al., 2023). Furthermore, these differences in parasite load can be explained by the lower tropism of T. gondii for these organs compared to the brain (Remington and Cavanaugh, 1965; Dubey, 2022).
The geographical distribution of T. gondii genotypes, proposed by Galal et al. (2018) revealed a contrasting spatial structure of the parasite´s diversity across the African continent. They showed that in North and East Africa, Type II appears to be the predominant lineage, while the Africa 1 lineage is almost absent. In contrast, the Africa 1 lineage was found to be widely distributed in tropical Africa, while Type II is rarer. The determinants of this structure were not elucidated at the time. No data were available for South Africa when this review was published. Since then, ten strains of T. gondii in animals from South Africa were successfully genotyped as Type II strains in one kitten, four Squirrel Monkeys, two Marmosets and two other Monkey species (Shwab et al., 2018), and a Red-eyed Dove (Streptopelia semitorquata) from the Limpopo Province (Lukášová et al., 2018b). This is therefore the first record of the Africa 4 lineage in the region. This lineage is widespread in Africa (Egypt, Ethiopia, Senegal, Mali, Ghana, Benin, Gambia and Tunisia) and has also been reported in several Asian countries (China, Sri Lanka, United Arab Emirates) (Galal et al., 2018; Galal et al., 2019a; Galal et al., 2019b; Hamidović et al., 2021; Lachkhem et al., 2021). Our results therefore revealed a wider distribution area of the Africa 4 lineage across the African continent. With the presence of the Type II lineage and now the Africa 4 lineage, the diversity in South Africa may be similar to that observed in North and East Africa, even though the Type III lineage described in these regions has not yet been found in South Africa (Galal et al., 2018; Lachkhem et al., 2021). A recent study showed that T. gondii lineages from the Old World (Africa, Asia and Europe), including the Africa 4 lineage, would be better adapted to the domestic environment, with a better potential for the sexual reproduction efficiency of these strains in the domestic cat (Galal et al., 2022). This is not incompatible with the identification of what is called domestic strain in a wild host such as the Black-backed Jackal, given the proximity between the wild and domestic environments in South Africa. The presence of domestic cats as a potential source of oocysts capable of disseminating into the wild environment has also been proposed in previous studies in North and South America (Jiang et al., 2018; Bolais et al., 2022).
The T. gondii DNA described in our study is one of the rare samples of wild origin genetically characterized on the African continent, being the first T. gondii genotype characterized in a wild carnivore from Africa and the first ever obtained from a Black-backed Jackal. Considering that the red-eyed dove from Lukášová et al. (2018b) was collected from the domestic urban area of the city of Polokwane (South Africa), only one strain from a wild bird species (Pternistis bicalcaratus) in south-eastern Senegal has been described so far (Galal et al., 2019a). In South America, and more precisely in French Guiana, it has been shown that there is a wild cycle of T. gondii that is genetically distinct from the domestic cycle and which is associated with increased virulence in humans, causing potentially fatal forms in immuno-competent patients in the absence of treatment (Carme et al., 2009; Mercier et al., 2011). This shows the importance of investigating the diversity of strains circulating in the wild cycle in Africa, given the large number of wild felid species capable of maintaining this wild cycle of T. gondii on the continent.
In conclusion, this study confirms that T. gondii is widespread in South African carnivores. We here provide the first data on T. gondii in a wild carnivore from South Africa with the identification of an indigenous genotype. The circulation of T. gondii in wildlife suggests a potential for transmission to domestic animals, which may be associated with potential economic losses for livestock farmers, as well as with risks of zoonotic transmission to humans. In view of these considerations, as well as an existing human-wildlife contact mainly between sheep farmers and jackals, it is very important to continue to explore the T. gondii strains circulating in South Africa, preferably in a One health approach.
Data availability statement
The raw data supporting the conclusions of this article will be made available by the authors, without undue reservation.
Ethics statement
Ethical approval was not required for the study involving animals in accordance with the local legislation and institutional requirements because all animals were found dead independently and prior to our research. Samples were collected according to the permit no. CPF6-0136 and permit no. ZA/LP/87586.
Author contributions
KR: Conceptualization, Investigation, Writing – original draft. EB: Investigation, Supervision, Writing – review & editing. AHam: Investigation, Methodology, Writing – original draft. NP: Investigation, Methodology, Writing – original draft. AK: Writing – review & editing. GC: Investigation, Methodology, Writing – review & editing. AM: Conceptualization, Investigation, Supervision, Writing – original draft. AHal: Data curation, Investigation, Writing – review & editing.
Funding
The author(s) declare financial support was received for the research, authorship, and/or publication of this article. The research was financially supported by grant VEGA No. 1/0709/23. Part of this work was supported by funds from the French Agence Nationale de la Recherche (ANR project IntroTox 17-CE35-0004).
Acknowledgments
We would like to thank Pavlı́na Pittermannová and Nikola Kašpárková for their assistance with sample preparation, DNA isolation and PCR testing. We would also like to thank Professor Wilmien Luus-Powell (DSI NRF SARChI Chair, Department of Biodiversity, University of Limpopo, South Africa) for supporting AH in her group and all the volunteer students: Katlego David Kunutu, Makubu P. Mokgawa, Jonas Mdau, Noko Ivy Poopedi, Thorisho Vincent Chuene, Michael Rampedi, that helped AH with the collecting roadkills during the sampling.
Conflict of interest
The authors declare that the research was conducted in the absence of any commercial or financial relationships that could be construed as a potential conflict of interest.
Publisher’s note
All claims expressed in this article are solely those of the authors and do not necessarily represent those of their affiliated organizations, or those of the publisher, the editors and the reviewers. Any product that may be evaluated in this article, or claim that may be made by its manufacturer, is not guaranteed or endorsed by the publisher.
Supplementary material
The Supplementary Material for this article can be found online at: https://www.frontiersin.org/articles/10.3389/fcimb.2024.1274577/full#supplementary-material
References
Abu, E. K., Boampong, J. N., Amoabeng, J. K., Ilechie, A. A., Kyei, S., Owusu-Ansah, et al. (2016). Epidemiology of ocular toxoplasmosis in three community surveys in the central region of Ghana, West Africa. Ophthalmic Epidemiol. 23, 14–19. doi: 10.3109/09286586.2015.1089579
Adesiyun, A. A., Knobel, D. L., Thompson, P. N., Wentzel, J., Kolo, F. B., Kolo, et al. (2020). Sero-epidemiological study of selected zoonotic and abortifacient pathogens in cattle at a wildlife-livestock interface in South Africa. Vector Borne Zoonotic Dis. 20 (4), 258–267. doi: 10.1089/vbz.2019.2519
Aguirre, A. A., Longcore, T., Barbieri, M., Dabritz, H., Hill, D., Klein, et al. (2019). The one health approach to Toxoplasmosis: epidemiology, control, and prevention strategies. EcoHealth. 16, 378–390. doi: 10.1007/s10393-019-01405-7
Ajzenberg, D., Collinet, F., Mercier, A., Vignoles, P., Dardé, M. L. (2010). Genotyping of Toxoplasma gondii isolates with 15 microsatellite markers in a single multiplex PCR assay. J. Clin. Microbiol. 48, 4641–4645. doi: 10.1128/JCM.01152-10
Ajzenberg, D., Lamaury, I., Demar, M., Vautrin, C., Cabié, A., Simon, et al. (2016). Performance testing of PCR assay in blood samples for the diagnosis of toxoplasmic encephalitis in AIDS patients from the French Departments of America and Genetic Diversity of Toxoplasma gondii: A prospective and multicentric study. PloS Negl. Trop. Dis. 10 (6), e0004790. doi: 10.1371/journal.pntd.0004790
Al-Kappany, Y. M., Rajendran, C., Ferreira, L. R., Kwok, O. C., Abu-Elwafa, S. A., Hilali, et al. (2010). High prevalence of toxoplasmosis in cats from Egypt: isolation of viable Toxoplasma gondii, tissue distribution, and isolate designation. J. Parasitol. 96 (6), 1115–1118. doi: 10.1645/GE-2554.1
Artiaga, A., Perez, L., Pasquier, G., Le Moing, V. (2022). Toxoplasmose aiguë multiviscérale de l'immunocompétent : à propos d'un cas importé d'Afrique tropicale. Acute disseminated toxoplasmosis in an immunocompetent patient : About a case imported from tropical Africa. Médecine Maladies Infectieuses Formation 1 (3), 145–148. doi: 10.1016/j.mmifmc.2022.06.004
Beltrame, A., Venturini, S., Crichiutti, G., Meroni, V., Buonfrate, D., Bassetti, M. (2016). Recurrent seizures during acute acquired toxoplasmosis in an immunocompetent traveller returning from Africa. Infection. 44 (2), 259–262. doi: 10.1007/s15010-015-0821-7
Bokaba, R. P., Dermauw, V., Morar-Leather, D., Dorny, P., Neves, L. (2022). Toxoplasma gondii in African wildlife: A systematic review. Pathogens. 11 (8), 868. doi: 10.3390/pathogens11080868
Bolais, P. F., Galal, L., Cronemberger, C., Pereira, F. A., Barbosa, A. D. S., Dib, L. V., et al. (2022). Toxoplasma gondii in the faeces of wild felids from the Atlantic Forest, Brazil. Mem Inst Oswaldo Cruz. 117, e210302. doi: 10.1590/0074-02760210302
Carme, B., Demar, M., Ajzenberg, D., Dardé, M. L. (2009). Severe acquired toxoplasmosis caused by wild cycle of Toxoplasma gondii, French Guiana. Emerg. Infect. Dis. 15 (4), 656–658. doi: 10.3201/eid1504.081306
Cavalli-Sforza, L. L., Edwards, A. W. (1967). Phylogenetic analysis. Models and estimation procedures. Am. J. Hum. Genet. 19 (3 Pt 1), 233–257.
Dardé, M. L. (2008). Toxoplasma gondii, "new" genotypes and virulence. Parasite. 15 (3), 366–371. doi: 10.1051/parasite/2008153366
Fernández-Escobar, M., Schares, G., Maksimov, P., Joeres, M., Ortega-Mora, L. M., Calero-Bernal, R. (2022). Toxoplasma gondii genotyping: a closer look into Europe. Front. Cell Infect. Microbiol. 12. doi: 10.3389/fcimb.2022.842595
Gachet, B., Elbaz, A., Boucher, A., Robineau, O., Fréalle, E., Ajana, F., et al. (2018). Acute toxoplasmosis in an immunocompetent traveller to Senegal. J. Travel Med. 25 (1). doi: 10.1093/jtm/tay086
Galal, L., Ajzenberg, D., Hamidović, A., Durieux, M. F., Dardé, M. L., Mercier, A. (2018). Toxoplasma and Africa: one parasite, two opposite population structures. Trends Parasitol. 34 (2), 140–154. doi: 10.1016/j.pt.2017.10.010
Galal, L., Ariey, F., Ar Gouilh, M., Dardé, M. L., Hamidović, A., Letourneur, F., et al. (2022). A unique Toxoplasma gondii haplotype accompanied the global expansion of cats. Nat. Commun. 13 (1), 5778. doi: 10.1038/s41467-022-33556-7
Galal, L., Hamidović, A., Dardé, M. L., Mercier, M. (2019c). Diversity of Toxoplasma gondii strains at the global level and its determinants. Food Waterborne Parasitol. 15, e00052. doi: 10.1016/j.fawpar.2019.e00052
Galal, L., Sarr, A., Cuny, T., Brouat, C., Coulibaly, F., Sembene, et al. (2019a). The introduction of new hosts with human trade shapes the extant distribution of Toxoplasma gondii lineages. PloS Negl. Trop. Dis. 13 (7), e0007435. doi: 10.1371/journal.pntd.0007435
Galal, L., Schares, G., Stragier, C., Vignoles, P., Brouat, C., Cuny, et al. (2019b). Diversity of Toxoplasma gondii strains shaped by commensal communities of small mammals. Int. J. Parasitol. 49 (3-4), 267–275. doi: 10.1016/j.ijpara.2018
Hamidović, A., Etougbétché, J. R., Tonouheva, A. B. N., Galal, L., Dobigny, G., Houemenou, G., et al. (2021). A hotspot of Toxoplasma gondii Africa 1 lineage in Benin: How new genotypes from West Africa contribute to understand the parasite genetic diversity worldwide. PloS Negl. Trop. Dis. 15 (2), e0008980. doi: 10.1371/journal.pntd.0008980
Hammond-Aryee, K., Monika, E., van Helden, L., van Helden, P. (2015). A high seroprevalence of Toxoplasma gondii antibodies in a population of feral cats in the Western Cape Province of South Africa. S Afr J. Infect. Dis. 30, 141–144. doi: 10.1080/23120053.2015.1107295
Homan, W. L., Vercammen, M., De Braekeleer, J., Verschueren, H. (2000). Identification of a 200- to 300-fold repetitive 529 bp DNA fragment in Toxoplasma gondii and its use for diagnostic and quantitative PCR. Int. J. Parasitol. 30, 69–75. doi: 10.1016/s0020-7519(99)00170-8
Jiang, T., Shwab, E. K., Martin, R. M., Gerhold, R. W., Rosenthal, B. M., Dubey, J. P., et al. (2018). A partition of Toxoplasma gondii genotypes across spatial gradients and among host species, and decreased parasite diversity towards areas of human settlement in North America. Int. J. Parasitol. 48 (8), 611–619. doi: 10.1016/j.ijpara.2018.01.008
Joeres, M., Cardron, G., Passebosc-Faure, K., Plault, N., Fernández-Escobar, M., Hamilton, C. M., et al. (2023). A ring trial to harmonize Toxoplasma gondii microsatellite typing: comparative analysis of results and recommendations for optimization. Eur. J. Clin. Microbiol. Infect. Dis. 42 (7), 803–818. doi: 10.1007/s10096-023-04597-7
Lachkhem, A., Galal, L., Lahmar, I., Passebosc, K., Riahi, H., Plault, N., et al. (2021). First isolation and genotyping of Toxoplasma gondii strains from domestic animals in Tunisia. Sci. Rep. 11 (1), 9328. doi: 10.1038/s41598-021-88751-1
Leroy, J., Houzé, S., Dardé, M. L., Yéra, H., Rossi, B., Delhaes, L., et al. (2019). Severe toxoplasmosis imported from tropical Africa in immunocompetent patients: A case series. Travel Med. Infect. Diseas. 35, 101509. doi: 10.1016/j.tmaid.2019.101509
Lobetti, R., Lappin, M. R. (2012). Prevalence of Toxoplasma gondii, Bartonella species and haemoplasma infection in cats in South Africa. J. Feline Med. Surg.14 12), 857–862. doi: 10.1177/1098612X12452495
Lukášová, R., Halajian, A., Bártová, E., Kobédová, K., Swanepoel, L. H., O´Riain, M. J. (2018a). The occurrence of some nonblood protozoan parasites in wild and domestic mammals in South Africa. J. Wildl Dis. 54 (2), 392–396. doi: 10.7589/2017-09-233
Lukášová, R., Kobédová, K., Halajian, A., Bártová, E., Murat, J. B., Rampedi, K. M., et al. (2018b). Molecular detection of Toxoplasma gondii and Neospora caninum in birds from South Africa. Acta Trop. 178, 93–96. doi: 10.1016/j.actatropica.2017.10.029
Mercier, A., Ajzenberg, D., Devillard, S., Demar, M. P., de Thoisy, B., Bonnabau, H., et al. (2011). Human impact on genetic diversity of Toxoplasma gondii: example of the anthropized environment from French Guiana. Infect. Genet. Evol. 11 (6), 1378–1387. doi: 10.1016/j.meegid.2011.05.003
Mercier, A., Devillard, S., Ngoubangoye, B., Bonnabau, H., Banuls, A. L., Durand, P., et al. (2010). Additional haplogroups of Toxoplasma gondii out of Africa: population structure and mouse-virulence of strains from Gabon. PloS Negl. Trop. Dis. 4 (11), e876. doi: 10.1371/journal.pntd.0000876
Ngobeni, R., Samie, A. (2017). Prevalence of Toxoplasma gondii IgG and IgM and associated risk factors among HIV-positive and HIV-negative patients in Vhembe district of South Africa. Afr J. Infect. Dis. 11 (2), 1–9. doi: 10.21010/ajid.v11i2.1
Nsiangani Lusambo, N., Kaimbo Wa Kaimbo, D., Mumba Ngoyi, D., Kilangalanga Ngoy, J., Ngoyi Bambi, M. T., Kadima Mutombo, T., et al. (2023). Clinical and serological characteristics of ocular toxoplasmosis in the Democratic Republic of Congo. Ocul Immunol. Inflammation 31 (7), 1522–1527. doi: 10.1080/09273948.2022.2140297
Omonijo, A. O., Kalinda, C., Mukaratirwa, S. (2022). Toxoplasma gondii infections in animals and humans in Southern Africa: A systematic review and meta-analysis. Pathogens. 11 (2), 183. doi: 10.3390/pathogens11020183
Penzhorn, B., Stylianides, E., van Vuuren, M., Alexander, K. A., Meltzer, D. G. A., Mukarati, N. L. (2002). Seroprevalence of Toxoplasma gondii in free-ranging lion and leopard populations in Southern Africa. Afr J. Wildl Res. 32 (2), 163–165.
Remington, J. S., Cavanaugh, E. N. (1965). Isolation of the encysted form of Toxoplasma gondii from human skeletal muscle and brain. N Engl. J. Med. 273 (24), 1308–1310. doi: 10.1056/NEJM196512092732404
Sánchez, V., de-la-Torre, A., Gomez-Marín, J. E. (2014). Characterization of ROP18 alleles in human toxoplasmosis. Parasitol. Int. 63 (2), 463–469. doi: 10.1016/j.parint.2013.10.012
Seltmann, A., Schares, G., Aschenborn, O. H. K., Heinrich, S. K., Thalwitzer, S., Wachter, B., et al. (2020). Species-specific differences in Toxoplasma gondii, Neospora caninum and Besnoitia besnoiti seroprevalence in Namibian wildlife. Parasit Vectors. 13 (1), 7. doi: 10.1186/s13071-019-3871-3
Serieys, L. E. K., Hammond-Aryee, K., Bishop, J., Broadfield, J., O´Riain, M. J., van Helden, P. D. (2019). High seroprevalence of Toxoplasma gondii in an urban caracal (Caracal caracal) population in South Africa. J. Wildl Dis. 55 (4), 951–953.
Shwab, E. K., Saraf, P., Zhu, X. Q., Zhou, D. H., McFerrin, B. M., Ajzenberg, D., et al. (2018). Human impact on the diversity and virulence of the ubiquitous zoonotic parasite Toxoplasma gondii. Proc. Natl. Acad. Sci. U S A. 115 (29), E6956–E6963. doi: 10.1073/pnas.1722202115
Tenter, A. M., Heckeroth, A. R., Weiss, L. M. (2000). Toxoplasma gondii: from animals to humans. Int. J. Parasitol. 30 (12-13), 1217–1258. doi: 10.1016/s0020-7519(00)00124-7
Keywords: toxoplasmosis, mammals, carnivore, Black-backed jackal, real-time PCR, microsatellite genotyping, African wildcat, wildlife parasites
Citation: Račka K, Bártová E, Hamidović A, Plault N, Kočišová A, Camacho G, Mercier A and Halajian A (2024) First detection of Toxoplasma gondii Africa 4 lineage in a population of carnivores from South Africa. Front. Cell. Infect. Microbiol. 14:1274577. doi: 10.3389/fcimb.2024.1274577
Received: 08 August 2023; Accepted: 02 January 2024;
Published: 30 January 2024.
Edited by:
Alessia Libera Gazzonis, University of Milan, ItalyReviewed by:
Olgica Djurkovic-Djakovic, University of Belgrade, SerbiaDavid González-Barrio, Carlos III Health Institute (ISCIII), Spain
Copyright © 2024 Račka, Bártová, Hamidović, Plault, Kočišová, Camacho, Mercier and Halajian. This is an open-access article distributed under the terms of the Creative Commons Attribution License (CC BY). The use, distribution or reproduction in other forums is permitted, provided the original author(s) and the copyright owner(s) are credited and that the original publication in this journal is cited, in accordance with accepted academic practice. No use, distribution or reproduction is permitted which does not comply with these terms.
*Correspondence: Karol Račka, karolracka@gmail.com