No role of working memory in the relation between mental rotation and postural stability
- Faculty of Human Sciences, University of Regensburg, Regensburg, Germany
This study investigated the relationship between mental rotation ability and postural stability, with a focus on the role of the visuospatial sketchpad of working memory, as it has been found to be correlated with both concepts. A total of 87 healthy young adults completed a postural stability task on a force plate, a mental rotation task, and both spatial and object working memory tasks in a counterbalanced order. Bayesian correlations revealed weak positive correlations between mental rotation and postural stability, as well as a correlation between mental rotation and spatial working memory. A weak association was also observed between mental rotation and object working memory. No correlation was found between the working memory components and postural stability. Furthermore, the results showed that spatial working memory did not play a crucial role in predicting postural stability. We conclude that the visuospatial sketchpad, particularly the spatial working memory component, cannot explain the relationship between mental rotation and postural stability.
1 Introduction
In the past, cognitive and motor functions were regarded as distinct processes (Georgopoulos, 2000). However, contemporary consensus posits that motor actions necessitate interaction with other processes, including action, perception, and cognition. Over the years, many dual-task-studies revealed a connection between cognitive and basic motor tasks like maintaining postural stability, i.e., controlling the body's center of gravity in relation to the supporting surface (Woollacott and Shumway-Cook, 2002; Shumway-Cook and Woollacott, 2007). In 1985, Kerr et al. (1985) conducted a study that initially revealed how spatial tasks, as opposed to verbal tasks, disrupt postural control when undertaken concurrently with cognitive and postural tasks. They concluded that postural regulation relies on neural mechanisms also employed in the cognitive processing of spatial tasks. A special role within spatial abilities is occupied by mental rotation ability (Linn and Petersen, 1985; Uttal et al., 2013).
Mental rotation is imagining how an object would look if it were rotated from its original position. There are two types of mental rotation tasks: object-based and egocentric tasks (Zacks et al., 2000). Shepard and Metzler (1971) first described the classical object-based paradigm. Here, two items are presented on a screen, with the right stimulus rotated and mirrored or non-mirrored. The participant must decide whether both stimuli are “the same,” i.e., not mirrored, or “different,” i.e., mirrored. In contrast, egocentric mental rotation tasks require participants to decide between right or left. Typically, a rotated figure with either a right or left arm extension is shown, and the participant must decide which arm is being presented. Both types of mental rotation are distinguished by the relationship of the observer to the environment. In object-based mental rotation, the observer position remains fixed, and the two stimuli are rotated relative to each other. In egocentric mental rotation, the observer's perspective is assumed to change as they imagine rotating themselves to solve the task (Kessler and Rutherford, 2010).
In general, for the relationship between the visuospatial ability mental rotation and motor function it has been assumed that motor and mental rotations share common processes (Wohlschläger and Wohlschläger, 1998), in other words the mental rotation process can be considered as a covert motor rotation (Wexler et al., 1998). Moreover, motor capabilities seem to be involved in mental rotation processes, because motor experts perform better in mental rotation test than non-motor experts (Steggemann et al., 2011; Pietsch and Jansen, 2012; Voyer and Jansen, 2017) and motor training has positive effects on mental rotation ability in children (Jansen et al., 2011; Blüchel et al., 2013; Pietsch et al., 2017). Dual-task paradigms have consistently shown a stabilizing effect of mental rotation tasks on postural stability in healthy young adults (Dault et al., 2001; Burcal et al., 2014; Budde et al., 2021; Hofmann and Jansen, 2023; Hofmann et al., 2023). Other studies suggest that mental rotational ability is even a predictor of postural stability. For example, Kawasaki et al. (2014) conducted egocentric mental rotation tasks after postural stability measurements in single leg stance and found lower body sway values significantly correlated with faster reaction times in following mental rotation tasks with foot stimuli. Hofmann et al. (2023) identified reaction time in egocentric and object-based mental rotation tasks as a significant predictor for a more stable center of pressure (CoP) course. To measure postural stability, the CoP can be calculated with a force plate (Rhea et al., 2014). During standing, the CoP is the point representing the weighted average of the sum of the vertical ground reaction forces exerted by both feet onto the force plate (Winter, 1995). Although there are approaches that understand a variable CoP signal as a functional component of postural control (Haddad et al., 2013), it is common (Rhea et al., 2015) to refer to a tighter CoP signal as a more stable stance (Palmieri et al., 2002). First indications of posture-stabilizing training effects by mental rotation are provided by Kawasaki and Higuchi (2016), who have shown evidence for positive short-term effects (up to 60 min) of mental rotation on postural stability. The key question is how mental rotation and postural stability are related, and whether there is a common mechanism that can explain this relationship.
One possible candidate mechanism is working memory, which despite having various definitions (Cowan, 2017), for example generic working memory (Cowan, 1988) or the attention-control working memory (Engle, 2002), is widely understood according to Baddeley and Hitch (1974) multicomponent model (Baddeley et al., 2021). This model includes four interacting components, with the two working memory storage systems, the phonological lopp and the visuospatial sketchpad. The phonological loop is postulated to retain verbal and auditory data through the utilization of a transient storage mechanism and an articulatory rehearsal system. Meanwhile, the visuospatial sketchpad is posited to retain visuospatial information and can potentially be subdivided into distinct visual, spatial, and, conceivably, kinaesthetic components (Baddeley, 2000). They are controlled by the central executive. Information from those three components is coordinated with the information of the episodic buffer, a temporary storage with limited capacity (Baddeley et al., 2021). The episodic buffer is capable of integrating information from various sources and long-term memory. Moreover, the central executive comprises a set of processes responsible for managing the flow of information in and out of the different stores (Baddeley, 2000; Cowan, 2017).
Generally, working memory is assumed to play an essential role during a mental rotation task. A mental rotation task includes the following stages: perceptual stage (perceptual processing, identification and discrimination of stimuli, identification of orientation), stages of the rotation process (mental rotation and judgment of parity), and the decision process. This last process includes the response selection and the execution phase (pressing a key) (Heil and Rolke, 2002). Especially in the stage of the rotation process, participants must keep the object in the working memory until they can complete a judgment of parity. For this, the information about the object and the spatial arrangement is essential. Different visual-spatial working memory buffers are used for storing spatial and object information (Smith et al., 1995). There is already evidence that these two systems may be related to mental rotation. These two buffers are referred to in the following as spatial working memory, a system in which spatial information is stored (concerned with object location), and object working memory (concerned with the non-spatial properties of the objects, such as color and shape). On the one hand, there are findings suggesting the connection between mental rotation and object working memory (Hyun and Luck, 2007). Applying a dual-task design, the participants performed mental rotation tasks both during a task involving object working memory and during a task involving spatial working memory. The results show a rotation-dependent interference between mental rotation and object working memory, in terms of lower mental rotation accuracy in the dual-task condition than the single task condition as well as lower accuracy in the object working memory task, where this effect was more enhanced for higher rotation angles in the mental rotation task. They could not show specific interference between mental rotation and spatial working memory. On the other hand, there are also indications for the connection between mental rotation and spatial working memory (Kaufman, 2007). The author investigated the relationship between working memory capacity and gender differences in mental rotation and spatial visualization. Among other things, they show a significant correlation between spatial working memory and mental rotation.
Furthermore, many dual-task studies address the relationship between working memory and postural stability. In addition to some findings linking different areas of working memory and postural stability (Shumway-Cook and Woollacott, 2007; Bhatt et al., 2016; Fujita et al., 2016), the connection between the visuospatial sketchpad and postural stability is of particular interest in the context of this study. Here, tasks involving the visuospatial sketchpad were shown to have higher postural-cognitive dual-task costs in older subjects (Maylor and Wing, 1996). They showed that in elderly people postural stability is more affected by cognitive tasks, requiring the visuospatial sketchpad, than in middle aged people. Similar findings were seen for other locomotor tasks, such as gait (Menant et al., 2014). Additionally, correlations were shown between patients' balance deficits and visuospatial sketchpad tasks (Smulders et al., 2013; Brecl et al., 2019). The nature of the relationship between spatial working memory and postural stability is discussed: VanderVelde et al. (2005) investigated the role of both visual-spatial working memory systems (object working memory and spatial working memory) in postural stability in healthy young adults. They showed that difficult posture tasks (independent variable) resulted in significant deterioration in spatial working memory tasks but not in object working memory tasks (dependent variables). They concluded that the interaction of visual-spatial working memory and postural control is due to the spatial domain. This result was confirmed by Chen et al. (2018), because postural control has more influence on spatial working memory tasks than on non-spatial working memory tasks. On the other hand, there are also results that show that spatial working memory has an effect on postural stability. Useros Olmo et al. (2020) demonstrated in a dual-task design that spatial working memory tasks (independent variable) stabilize posture (dependent variable) in patients and healthy controls. Taken together, these studies suggest that visual-spatial working memory may play a role in the relationship between mental rotation and postural stability.
2 Aim of the study
This study aims to investigate the relation of mental rotation, postural stability and the two components of visual-spatial working memory, namely object, and spatial working memory, in healthy young adults, by using standardized procedures. Therefore, the following hypotheses are examined:
1. Mental rotation ability correlates positively with postural stability (Dault et al., 2001; Burcal et al., 2014; Kawasaki et al., 2014; Budde et al., 2021; Hofmann and Jansen, 2023; Hofmann et al., 2023).
2. The spatial working memory is related to mental rotation ability (Kaufman, 2007) and postural stability (VanderVelde et al., 2005; Useros Olmo et al., 2020).
3. The object working memory is only related to mental rotation ability (Hyun and Luck, 2007) but not to postural stability (VanderVelde et al., 2005).
4. Exploratively: because spatial working memory is assumed to be related to mental rotation ability and postural ability, it is the best predictor of postural stability.
3 Methods
3.1 Participants
The needed sample size was calculated for detecting a small to medium effect (f2 = 0.0989) of the regression coefficient of spatial working memory in a linear multiple regression model to predict postural stability with the three predictors: mental rotation ability, spatial working memory ability and object working memory ability. An a-priori G*Power analysis (Faul et al., 2007) with an alpha-level of alpha = 0.05 and a desired power of 1-beta = 0.9 resulted in a total sample size of N = 89. Participants had to be at least 18 years old and received course credit for participation. Exclusion criteria were diseases or injuries affecting the balance. The study was performed in line with the principles of the Declaration of Helsinki. Participants were informed about the goal of the study and the privacy policy concerning the data. All participants gave their written informed consent before participating in this study. The study was approved by the Ethical Board of the University of Regensburg and was preregistered (https://osf.io/2kn8p). Any significant deviation from the preregistration is noted at the end of this study. A total of 91 subjects were sampled, because two participants had to interrupt the experiment early due to technical problems. In addition, two further subjects had to be excluded because their performance in the mental rotation test was below chance level. Therefore, the resulting sample size consists of 87 healthy sports science students from the study-subject “Applied Movement Science” of the University of Regensburg. The 42 female students had a mean age of 21.6 years (SD: 1.9 years) and a mean height of 168.5 cm (SD: 6.9 cm). The 45 male students had a mean age of 23.2 years (SD: 2.8 years) and a mean height of 181.7 cm (SD: 6.8 cm). All participants had normal or corrected to normal vision. None of the participants could read Chinese characters.
3.2 Postural stability measurement
Each participant completed three trials of a one-legged stance task on a force plate (AMTI OR6-7-2000; 1000 Hz) using their preferred leg. The duration of each trial was 70 s, and a sitting break of at least 90 s was taken between trials to prevent fatigue. The experimenter placed the participant in a standardized position on the force plate using a taped “T” and provided instructions to stand upright and remain as still as possible. Participants were instructed to keep their arms hanging relaxed at their sides and their non-standing foot raised in the air. A fixation cross was displayed on a laptop at eye-level, as shown in Figure 1, and participants were instructed to face it directly. To ensure barefoot conditions while maintaining hygiene standards, all participants were required to wear disposable socks.
3.3 Mental rotation task
Mental rotation tasks were presented on a laptop, using the software OpenSesame (Mathôt et al., 2012). The task involved presenting two cube figures, which were either the same or mirrored, rotated relative to each other (see Figure 2). The figure on the left side was always in the non-rotated state (0°). Participants were required to decide whether the right stimulus was the same or a mirrored version of the left stimulus. If both stimuli were the same, the participant had to press the left mouse button, while if both stimuli were mirrored to each other, the participant had to press the right mouse button. A total of three different cube figures (Jost and Jansen, 2020) were displayed rotated in 60° steps (0°, 60°, 120°, 180°, 240°, 300°) in the image plane. Each angle of each figure was displayed 3 times in non-mirrored and 3 times in mirrored form, resulting in a total of 108 trials (3 × 6 × 2 × 3). Prior to the main trials, practice runs were conducted, during which feedback was provided in the form of a green check mark for correct answers or a red cross for incorrect answers. During the main trials, however, no feedback was given, but a fixation cross was displayed between the single trials.
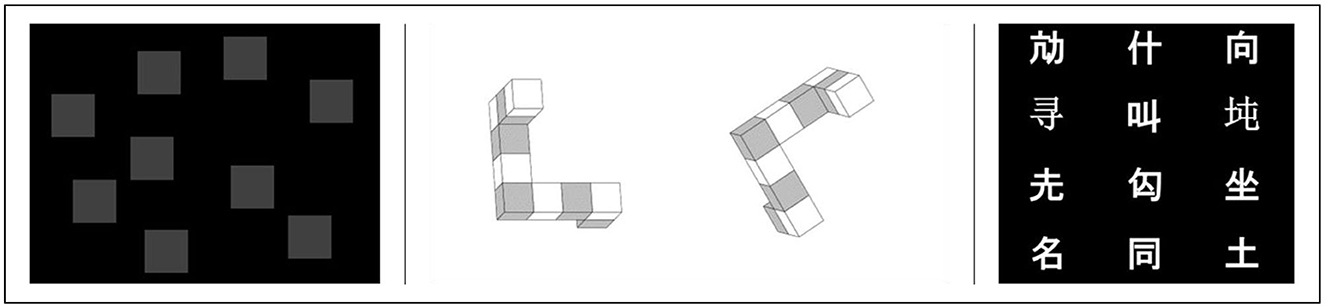
Figure 2. Exemplary pictures for the cognitive tasks. From left to right: exemplary images of the spatial working memory task, mental rotation task and object working memory task.
3.4 Spatial working memory task
For measuring the spatial working memory component the gold standard test (Baddeley, 2003), the corsi block tapping test was performed on a laptop using the software Psychology Experiment Building Language (PEBL) (Mueller and Piper, 2014). The computerized version displayed 9 blue squares unevenly distributed on the screen, as shown in Figure 2. The task began with a sequence of two blocks lighting up yellow in a specific order, and the participant had to replicate the sequence by clicking on the blocks with the mouse. Two trials were presented per block sequence length, and the participant had to correctly replicate at least one of the two trials to progress to the next block sequence. The task continued until the participant made two consecutive errors at the same block sequence length or until all nine blocks had been correctly replicated. In total, the Corsi block tapping test was repeated three times. The first round used the original block sequences (Kessels et al., 2000) and the sequences for the further two rounds were created using a random number generator. The square's position was according to Kessels et al. (2000).
3.5 Object working memory task
The task was performed on a laptop, using the software OpenSesame (Mathôt et al., 2012) and was based on a cognitive test used in Klauer and Zhao (2004). For 18 trials six white Chinese characters were presented in sequence, each for 500 ms (interstimulus interval 500 ms) on a black background. This was followed by a 500 ms white mask, where the whole screen turned white. Subsequently, the six presented characters and six distractors, also Chinese characters, were shown in a 3 × 4 field, see Figure 2. Participants were instructed to identify as many previously shown characters as possible by selecting six characters for each answer. The task was not timed. Prior to the study, all Chinese characters used in the task, were selected subjectively by the main author to have a similarly complex structure, indicated by the number of lines of the letter. Each trial showed an unique set of targets and distractors and all participants were shown the same order of trials.
3.6 Procedure
The experimental sessions lasted about 1 h, and all sessions were done in the same laboratory. Each subject received all four tests in counterbalanced order and additionally a short demographic questionnaire at the end of the measurement.
3.7 Data processing
All data was processed in Matlab (The MathWorks Inc, 2023).
Postural stability was interpreted via CoP course over time. First, raw CoP-data was low-pass filtered by a 4th order Butterworth filter and a 10 Hz cutoff frequency. Then, the following linear parameters were used to describe body sway: Mean Amplitude (MA) (mm) (Prieto et al., 1996), Sway Velocity (SV) (mm/s), which is the total sway path length divided by whole-trial time. A lower value in Mean Amplitude or in Sway Velocity is interpreted as higher postural stability. Furthermore, the structural parameter sample entropy (Ramdani et al., 2009) was calculated (Martínez-Cagigal, 2018) for the CoP course in anterior-posterior (SampEn_AP) and medio-lateral (SampEn_ML) direction. Sample entropy is a measure of regularity in time series data, where a higher sample entropy indicates more irregularity. Literature associates this with a more effective strategy of postural control (Borg and Laxåback, 2010; Kedziorek and Błazkiewicz, 2020). For the sample entropy analysis, the original data were down sampled to 100 Hz (Rhea et al., 2011; Koltermann et al., 2018). The input parameters m and r were chosen to be m = 3 and r = 0.2 times the standard deviation of the CoP data (Rhea et al., 2011). Reliability analyses revealed for the parameter MA: McDonald's Omega = 0.81, for the parameter SV: McDonald's Omega = 0.96, for the parameter SampEn_AP: McDonald's Omega = 0.77 and for the parameter SampEn_ML: McDonald's Omega = 0.87.
To analyze the performance on mental rotation tasks, the reaction time (s) was measured. Only non-mirrored tasks that were answered correctly (on average: 91.4%) were evaluated (Jolicœur et al., 1985). Within a person, all values of a specific angular disparity that were above or below three standard deviations of the mean value of the respective angle were considered as outliers and excluded. An average was taken across all means per angle, with a lower value associated with better mental rotation ability. In addition to the preregistered parameters, the accuracy (%) parameter was also calculated. This indicates the percentage of tasks that were solved correctly. A higher value indicates a better mental rotation ability. Reliability analyses revealed for the parameter reaction time: McDonald's Omega = 0.94, and for the parameter accuracy: McDonald's Omega = 0.86.
For analyzing the Corsi block tapping test, the Corsi Span, which is the number of blocks in the last correctly repeated block sequence, was calculated (Kessels et al., 2000). Since there were three trials, a mean value was calculated over all trials for Corsi Span. A higher mean Corsi Span is interpreted as better spatial working memory ability. Reliability analysis revealed McDonald's Omega = 0.69.
For analyzing the object working memory task, the total number of correctly remembered Chinese characters was counted. A higher value is interpreted as better object working memory ability. Reliability analysis revealed McDonald's Omega = 0.80.
3.8 Statistical analysis
Frequentist statistical analyses were performed using the software SPSS (IBM SPSS Statistics; version: 28.0.0.0) and Bayesian analyses were done with the software JASP (version 0.17) (JASP Team, 2023). In addition to the preregistered analyses, Bayesian correlations with 95% credible intervals were performed to answer hypotheses 1–3. Bayesian analyses were chosen as they provide more informative results by avoiding the binary acceptance or rejection of the null hypothesis and compare the data's support for the null and the alternative hypotheses. For hypotheses 1–3, a bidirectional non-informative prior was used when the magnitude of the association could not be estimated precisely (Nuzzo, 2017; Wagenmakers et al., 2018). When having assumptions about the direction of the association, a one directional non-informative prior was used. To state at what point the data become sufficiently more supportive of a hypothesis, the bounds of 1/3 and 3 were used, which say that a Bayes factor between 1/3 and 3 is considered indecisive (Dienes, 2019). However, even with indecisive results, the Bayes factor can still be interpreted as indicating the amount the data is more likely under one hypothesis than under the other. Thus, trends can also be shown and quantified. For answering hypothesis 1- 3, the parameter accuracy was added to the preregistered parameters, to measure mental rotation ability as well. To predict postural stability using the mental rotation and working memory tasks, hierarchical linear multiple regressions were performed for each postural sway parameter. The predictors included mental rotation ability (parameter: reaction time), spatial working memory ability (parameter: Corsi Span), and object working memory ability. Two regression models were presented for each postural sway parameter: Model 1, a linear multiple regression with all three predictors, and Model 2, where the spatial working memory component was removed, and the change in R2 was considered. In addition, this was repeated with the mental rotation parameter accuracy. The analyses then refer to Model 3 and Model 4. The significance level for detecting a change in R2 in the hierarchical regressions was Bonferroni-corrected for the four dependent postural sway parameters and two mental rotation parameters, resulting in a significance level of alpha = 0.0063.
4 Results
4.1 Relationships between the different tasks
To give a comprehensive overview of the relationship between the concepts, the correlations are presented. Figure 3 shows the correlations between the different cognitive tasks. For the relationship between OWM = “object working memory” and SWM = “spatial working memory (corsi span),” no Bayes factor is reported because no hypothesis was formulated about this relationship. Since there were no hypotheses regarding the relationships between different postural parameters, no Bayes factors are presented. The Pearson's correlation values between the parameters can indicate the direction in which a parameter should be interpreted when discussing improved postural control or increased stability.
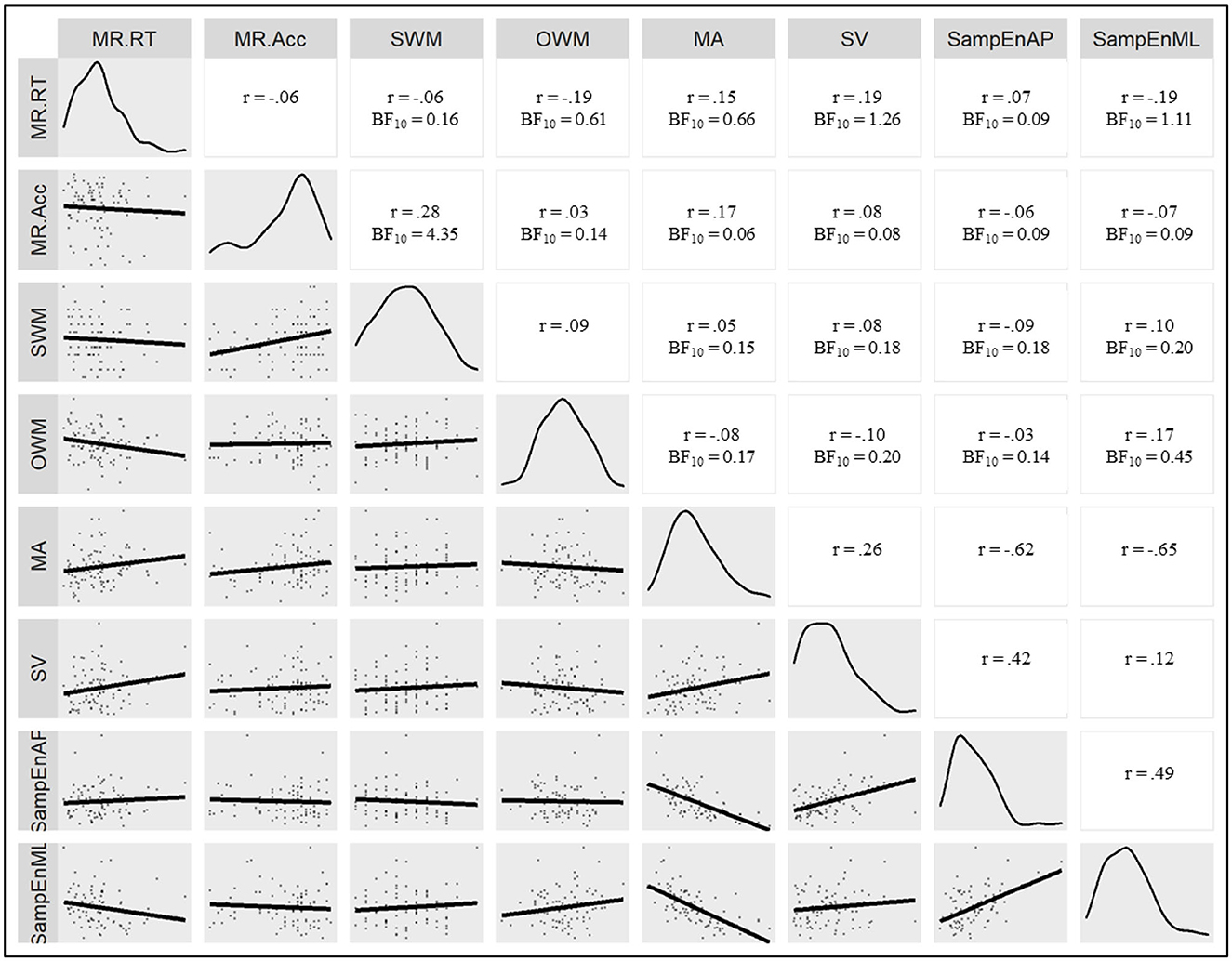
Figure 3. Correlational relationships between mental rotation ability, the visuospatial sketchpad and postural stability. MR.RT, Mental Rotation: Reaction Time (ms); MR.Accuracy, Mental Rotation: Accuracy (%); SWM, Spatial Working Memory; OWM, Object Working Memory; MA, Mean Amplitude (mm); SV, Sway Velocity (mm/s); SampEnAP, Sample Entropy anterior-posterior direction; SampEnML, Sample Entropy medio-lateral direction. The Bayes factor indicates how much more likely the data are under the alternative hypothesis compared to the null hypothesis.
4.2 Prediction of postural sway parameters
For each postural stability parameter, a linear hierarchical multiple regression with two steps was calculated. Model 1 used the three independent variables MR = “mental rotation (reaction time),” SWM and OWM. In Model 2, only the variable SWM was removed to see how much relevance it had for each dependent variable. Since the mental rotation task has two parameters, this procedure was also repeated with the independent variable mental rotation (accuracy). Model 3 used the three independent variables MR = “mental rotation (accuracy)," SWM and OWM. In Model 4, only the variable SWM was removed.
Mean Amplitude could not be predicted by either Model 1, F(3,83) = 0.857, p = 0.467, or Model 2, F(2,84) = 1.116, p = 0.333. Model 1 explained 3% of the variance in the Mean Amplitude and Model 2 explained slightly less at 2.6%. It is assumed that neither model predicts the parameter Mean Amplitude better than the other, due to a non-significant change in the R2 value between the two models, R2 = −0.004, FChange(1,83) = 0.356, p = 0.552. In both models, none of the predictors significantly predicted the parameter Mean Amplitude.
Mean Amplitude could not be predicted by either Model 3, F(3,83) = 0.983, p = 0.405, or Model 4, F(2,84) = 1.487, p = 0.232. Model 3 and Model 4, both explained 3.4% of the variance in the Mean Amplitude. Neither model predicts the parameter Mean Amplitude better than the other, due to a non-significant change in the R2 value between the two models, R2 = 0.000, FChange(1,83) = 0.011, p = 0.917. In both models, none of the predictors significantly predicted the parameter Mean Amplitude. The exact values of the individual variables in each model are shown in Table 1.
Sway velocity was not predicted by either Model 1, F(3,83) = 1.488, p = 0.224, or Model 2, F(2,84) = 1.806, p = 0.171. Model 1 explained 5.1% (model 1) and 4.1% (model 2) of the variance in sway velocity, respectively, and were not significantly different from each other, R2 = −0.010, FChange(1,83) = 0.859, p = 0.357.
Sway velocity was not predicted by either Model 3, F(3,83) = 0.584, p = 0.627, or Model 4, F(2,84) = 0.661, p = 0.519. Model 1 explained 2.1% and Model 2 1.5% of the variance in sway velocity, respectively, and were not significantly different from each other, R2 = −0.005, FChange(1,83) = 0.440, p = 0.509. None of the predictors significantly predicted SV, see Table 2.
The sample entropy of the CoP data in the anterior-posterior direction could not be predicted by either model. While model 1, F(3,83) = 0.336, p = 0.800, resolved 1.2% of the variance, model 2, F(2,84) = 0.234, p = 0.792, resolved slightly less variance at 0.6%. Both models did not differ, R2 = −0.006, FChange(1,83) = 0.541, p = 0.464.
The sample entropy of the CoP data in the anterior-posterior direction could not be predicted by either model. Model 3, F(3,83) = 0.242, p = 0.867, resolved 0.9% of the variance, model 4, F(2,84) = 0.154, p = 0.858, resolved less variance at 0.4%. Both models did not differ, R2 = −0.005, FChange(1,83) = 0.419, p = 0.519. Table 3 shows that none of the predictors significantly predicted SampEn_AP.
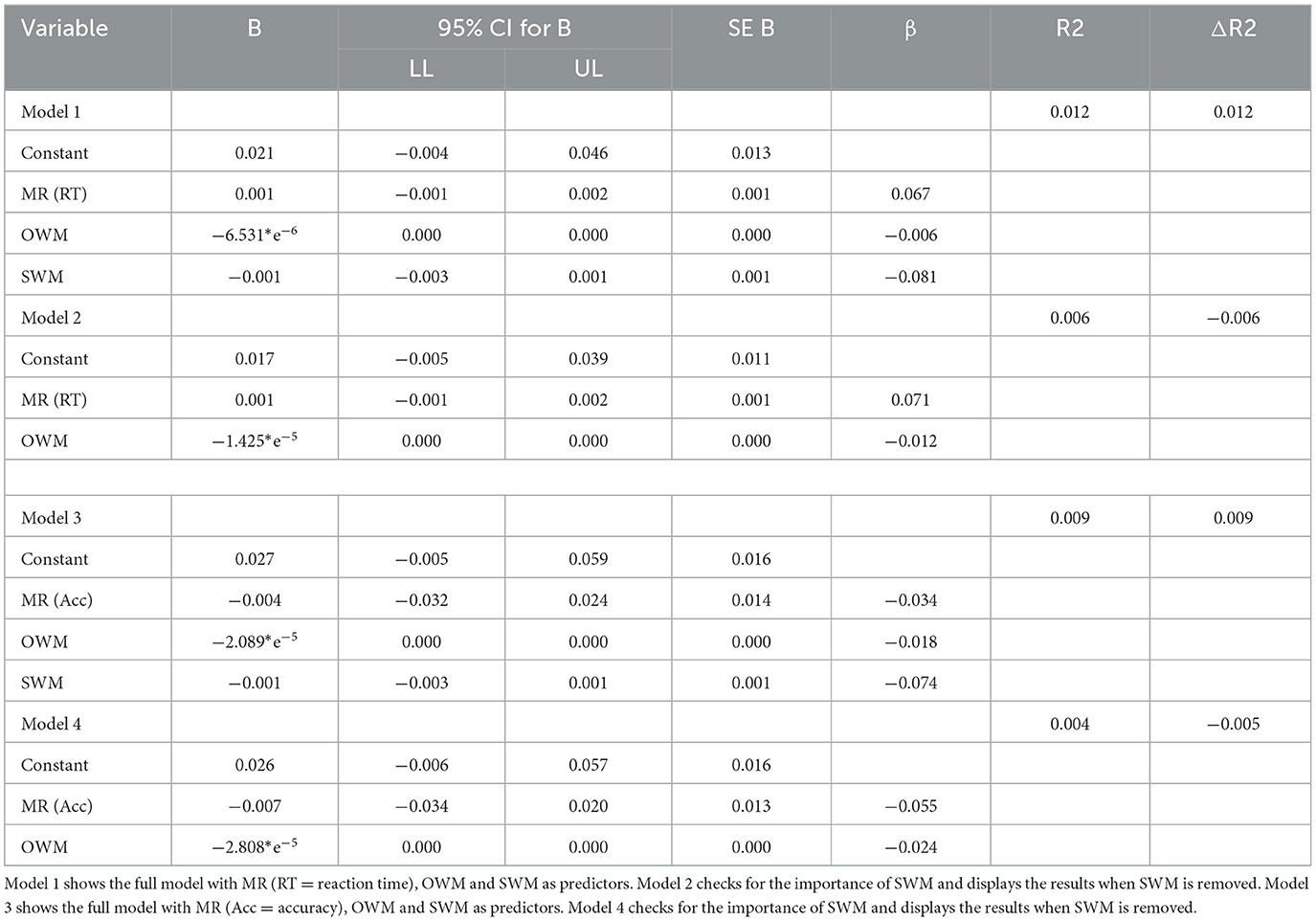
Table 3. Two step regression results for the parameter “sample entropy in anterior-posterior direction.”
Similarly, for the parameter SampEn_ML neither model 1, F(3,83) = 1.323, p = 0.272, nor model 2, F(2,84) = 1.497, p = 0.230, significantly predicted SampEn_ML. The two models did not differ, R2 = −0.005, FChange(1,83) = 0.476, p = 0.492. Model 1 resolved 5.9% of the variance of SampEn_ML and model 2 resolved 5.3%.
Also, neither model 3, F(3,83) = 1.730, p = 0.167, nor model 4, F(2,84) = 2.372, p = 0.100, significantly predicted SampEn_ML. The two models did not differ, R2 = −0.011, FChange(1,83) = 0.977, p = 0.326. Model 3 resolved 4.6% of the variance of SampEn_ML and model 4 resolved 3.4%. None of the predictors in either model showed a significant result, see Table 4.
5 Discussion
The study aimed to investigate the role of the two visual-spatial working memory components, spatial and object working memory, for the relationship between mental rotation and postural stability. The study also aimed to provide an overview of these individual processes using standardized methods. The results showed that the postural stability parameters were weakly positively related to mental rotation ability (Hypothesis 1), but with Bayes factors in an indecisive range. Spatial working memory was found to be positively related to mental rotation ability, but not to postural stability, partially confirming Hypothesis 2. Object working memory was descriptively weakly positively related to mental rotation ability, but with a Bayes factor in an indecisive range. As expected, object working memory was not related to postural stability (Hypothesis 3). The assumption that spatial working memory is the best predictor of postural stability was rejected (Hypothesis 4).
5.1 Relationship between mental rotation and postural stability
Different results are shown in this study regarding the relation of reaction time in mental rotation tasks and postural stability. For the parameter describing postural sway in the anterior-posterior direction, there are no correlations with mental rotation parameters. For the remaining parameters, the Bayes factor moves in a range considered indecisive. Descriptively, weak to moderate correlations were found there. However, one might ask why no stronger or clearer correlations were found since the association has already been shown frequently in dual-task studies (Dault et al., 2001; Burcal et al., 2014; Budde et al., 2021; Hofmann and Jansen, 2023; Hofmann et al., 2023) and explicitly in a correlation study for egocentric mental rotation of body parts by Kawasaki et al. (2014). It is possible that the findings from dual-task designs resulted because of attentional capacity limits rather than specific processes that are stimulated during mental rotation (Woollacott and Shumway-Cook, 2002; Pellecchia, 2003). The results from Dault et al. (2001) and Hofmann and Jansen (2023) support this explanation, as both studies showed no difference in sway between different cognitive tasks. However, to clarify this definitively for the mental rotation task, more dual-task studies that control systematically for different cognitive tasks and collect more than global sway parameters would need to be conducted. It is possible that mental rotation and postural stability share common processes, but interference between the two does not necessarily imply a high correlation when considered individually. To demonstrate a relationship between mental rotation ability and postural stability, this needs to be examined in an intervention study where the effect of mental rotation on postural stability could be clarified. The study presented here looked at non-embodied stimulus material with cube figures in an object-based mental rotation task, which is most comparable to the correlation between single-leg stance and egocentric mental rotation of (non-embodied) car stimuli of Kawasaki et al. (2014). Descriptively, this study shows even a higher correlation compared to Kawasaki et al. (2014). Despite significant results, it must be critically noted that only 24 subjects were examined by Kawasaki et al. (2014), which may limit the validity of their results (Brysbaert, 2019). But in general, it may be possible that the assumed effect size for the correlations in this study were too high. A possible idea for future studies is provided by the result that descriptively, the tendency could be seen that especially the postural sway parameters in the medio-lateral direction correlate more with mental rotation than those in the anterior-posterior direction. From a biomechanical point of view, it is reasonable to assume that in this study, the body sway in the medio-lateral direction is more prominent since the base of support is smaller in this direction due to the single-leg stance (Duarte and Freitas, 2010).
5.2 The relevance of visual-spatial working memory for the relationship between mental rotation and postural stability
One possible explanation for the general relationship between mental rotation and postural stability was visual-spatial working memory (VanderVelde et al., 2005; Hyun and Luck, 2007; Smulders et al., 2013). However, no relationship was found between the classical chronometric mental rotation measure reaction time and the two components of the visual-spatial working memory. For spatial working memory, the Bayes factor is six times more in favor of no relationship to mental rotation than in favor of one. Given the moderate to strong correlation in Kaufman (2007), this is initially surprising. However, the study demonstrated this relationship in a paper and pencil test. In these tests, reaction time cannot be measured, but an overall score is formed by how many individual items one solves correctly. In a chronometric test, this corresponds to the parameter accuracy (Voyer et al., 2006). Considering this, our data, showing a positive correlation of the accuracy in mental rotation tasks with spatial working memory, aligns with the previous findings from Kaufman (2007). Regarding the relationship between object working memory and mental rotation, the correlation with reaction time indicates a weak to moderate correlation (Cohen, 1988), with the Bayes Factor ranging in an indecisive area. With accuracy, no correlation was shown. Because previous findings on this relationship come from only one dual-task-interference study with a small number of participants (Hyun and Luck, 2007), the point estimate of the correlation with reaction time provides an interesting reference point, which could be used for power analyses for further research on this relationship. Irrespective of the question of the connection between mental rotation and postural stability, the explorative analysis of the relationship between the two forms of working memory did not show any connection. This supports the differentiation of an object and a spatial working memory buffer (Smith et al., 1995) and is in line with the differentiation of the visual-spatial sketchpad in the working memory model of Baddeley et al. (2021).
Based on the results of this study, the correlation between visual-spatial working memory and postural stability must be rejected. Regarding spatial working memory, none of the correlations suggests a relationship to postural stability. Also, when comparing the two regression models, there is no significant difference from zero for either model nor is there a difference between the models when the spatial working memory parameter is removed. Previous studies found associations between spatial working memory and postural stability in healthy participants either in interference designs (VanderVelde et al., 2005) or in neural studies (Chen et al., 2018). Results from pure correlation designs can only be found in patient studies (Smulders et al., 2013; Brecl et al., 2019). Therefore, it is interesting that, according to the results of this study, this correlation does not seem to be pronounced in healthy young participants. As expected, no correlations with postural stability were found regarding the object working memory (VanderVelde et al., 2005). Only a correlation with the parameter sample entropy in the medio-lateral direction is within an indecisive range but is clearly more in favor of the null hypothesis. Overall, the regression models never showed more than a 6% explanation of the total variance of the respective postural stability parameter. This may emphasize the small contribution of the chosen cognitive variables to postural stability, or it is an indication that the cognitive tests have not sufficiently engaged the visual-spatial working memory. To examine this in more detail, it could be helpful to explicitly clarify the role of spatial working memory in postural stability in an intervention study.
In future studies that collect various postural sway parameters, an overall figure, such as Figure 3, should also be provided. Usually, with each postural stability measure, there is some indication of associated postural stability or postural control. Thus, for global postural stability parameters, one assumes that a lower value implies better postural stability (Palmieri et al., 2002) and, for example, for sample entropy, one implies better postural control for a larger value, even if this mathematically means more chaotic data (Borg and Laxåback, 2010; Kedziorek and Błazkiewicz, 2020). When looking at Figure 3, it is noticeable that the parameter sway velocity is descriptively positively correlated with both parameters of sample entropy. Possibly this is an indication that, at least in this study, a higher sway velocity relates to better postural control or a higher sample entropy for more stability. Findings like this may be interesting for the interpretation of a study. For example, results by Budde et al. (2021) have shown that during egocentric mental rotation, the range of CoP values but also the sway velocity of CoP values is reduced compared to object-based mental rotation. They interpret this, as is typical, as postural stabilization. However, the decreased sway velocity (see Table 3) may also be interpreted as lower postural control. Both Budde et al. (2021) and Hofmann and Jansen (2023) compared the process of egocentric mental rotation to the kinesthetic perspective of motor imagery. Both studies assumed that egocentric mental rotation could elicit more body sway because of the assumption that here the participant has to imagine a rotation of the own body to solve the task (Kessler and Rutherford, 2010). Both studies did not prove that, but it may be conceivable that the reduction of the sway velocity in Budde et al. (2021) could also be interpreted as reduced control due to the mental rotation of the own body. However, this is purely speculative but may provide an interesting hint for further research.
6 Limitations
There are a few limitations to note in this study. The sample with healthy young sports students represents a very special sample for studies on motor control and mental rotation, as it is already known that there are relationships (Pietsch and Jansen, 2012; Voyer and Jansen, 2017). However, the motor task was made more difficult for this purpose by conducting the single-leg stance, which created more challenging motor conditions than a normal bipedal stance would do. However, since no level of difficulty was checked in the participants, it cannot be completely ruled out that the task was still too easy for the athletic sample to cause enough variance in the sway data. Additionally, the choice of cognitive tests can be questioned. While the Corsi block tapping test is considered the gold standard for measuring spatial working memory (Baddeley, 2003), it was originally designed primarily for everyday clinical use. Nevertheless, its suitability for non-clinical studies is evident, given its widespread usage in such research. However, we find a reliability value for McDonald's Omega = 0.69. This may imply that statements regarding spatial working memory need to be interpreted with caution. For testing the object working memory, the adaption, according to Klauer and Zhao (2004), was conclusive, but the test is not an established object working memory test. The test was chosen based on the premise that solving the task necessitates minimal spatial information, though such determination is inherently subjective. Concerning the distinction between spatial and object-related working memory, one could posit that either a task with a comparable structure, such as a visual pattern test, should have been chosen alongside the Corsi block tapping test, or that both metrics from Klauer and Zhao (2004) could have been included. Their spatial component test measured the memory of dot locations. Therefore, the selection of tests may have the potential to influence this study's outcomes. However, it is essential to underscore that, even if the selection of tests might have been suboptimal, both chosen tests are highly likely to gauge the corresponding working memory buffer. Consequently, strong effects are not anticipated, regardless of the specific test chosen. Moreover, there is a strong relationship between attentional control and working memory (Baddeley et al., 2021). In the current study, only specific task instructions and a disturbance-free experimental environment controlled for this. In the future, it would be desirable to use eye-tracking devices to at least get a first impression of attentional control by monitoring gaze behavior. Further, some Bayes factors are in an indecisive range for the correlations. Even this might be better than simply reporting non-significant p-values, especially with Bayes factors, a so-called adaptive sampling can be used, in which the data is collected until conclusive Bayes factors are reached. However, due to a preregistered power analysis and for reasons of economy, the sample size was maintained.
7 Conclusion
The study aimed to show the relationships between mental rotation, the two components of visual-spatial working memory, spatial working memory and object working memory, and postural stability. Additionally, it was aimed to verify whether spatial working memory is the actual predictor of postural stability. As spatial working memory could not be shown to be an essential predictor for postural stability, to date it is not assumed that spatial working memory explains the relationship between mental rotation and postural stability. However, further dual-task or intervention studies must be performed to definitively clarify this. The aim is to investigate the connections between mental rotation, postural stability and working memory using standardized methods, modern statistical methods and sufficient power. The correlation tables in this study provide interesting insights into the relationships between all measured concepts and will provide realistic estimators for effect sizes for future research. Even if the correlation between postural stability and mental rotation was smaller than expected, it is still worth further investigation as it is interesting why the results differ from those of dual-task design studies. So, future studies should clarify whether the relationship in dual-task studies is only due to increased dual-task costs or due to true relationships between mental rotation and postural stability. If the relationship between mental rotation and postural stability becomes clearer in the future, this may offer an interesting tool in the rehabilitation of individuals with impaired postural control.
Author's note
Deviation to preregistration:
• Hypothesis 4 is explorative.
• No CoP-frequency parameters are examined.
• Bayesian correlations are calculated for displaying all relationships between variables.
• Accuracy in mental rotation task is added as predictor.
Data availability statement
The original contributions presented in the study are publicly available. This data can be found here: https://osf.io/8paz5/.
Ethics statement
The studies involving humans were approved by the Ethical Board of the University of Regensburg (protocol number: 20-2094_1-101). The studies were conducted in accordance with the local legislation and institutional requirements. The participants provided their written informed consent to participate in this study. Written informed consent was obtained from the individual(s) for the publication of any potentially identifiable images or data included in this article.
Author contributions
PH: Conceptualization, Software, Methodology, Writing—original draft, Writing—review & editing. MS: Conceptualization, Software, Methodology, Writing—original draft. PJ: Conceptualization, Supervision, Writing—review & editing.
Funding
The author(s) declare no financial support was received for the research, authorship, and/or publication of this article.
Acknowledgments
The authors would like to thank the entire research team of the Institute of Sports Science at the University of Regensburg for their helpful discussions throughout the study. English language editing was performed by an Artificial Intelligence language model (Chat GPT). The software did not create any new content during the editing process. For the use of artificial intelligence for language editing in scientific articles, see Kim (2023).
Conflict of interest
The authors declare that the research was conducted in the absence of any commercial or financial relationships that could be construed as a potential conflict of interest.
The author(s) declared that they were an editorial board member of Frontiers, at the time of submission. This had no impact on the peer review process and the final decision.
Publisher's note
All claims expressed in this article are solely those of the authors and do not necessarily represent those of their affiliated organizations, or those of the publisher, the editors and the reviewers. Any product that may be evaluated in this article, or claim that may be made by its manufacturer, is not guaranteed or endorsed by the publisher.
References
Baddeley, A. D. (2000). The episodic buffer: a new component of working memory? Trends Cogn Sci 4, 417–423. doi: 10.1016/S1364-6613(00)01538-2
Baddeley, A. D. (2003). Working memory: looking back and looking forward. Nat. Rev. Neurosci. 4, 829–839. doi: 10.1038/nrn1201
Baddeley, A. D., and Hitch, G. (1974). “Working memory: looking back and looking forward,” in Psychology of Learning and Motivation: Advances in Research and Theory, ed. G. H. Bower (Burlington: Elsevier), 47–89.
Baddeley, A. D., Hitch, G., and Allen, R. (2021). “A multicomponent model of working memory,” in Working Memory: State of the Science, eds. R. H. Logie, V. Camos, and N. Cowan (Oxford: Oxford University Press), 10–43.
Bhatt, T., Subramaniam, S., and Varghese, R. (2016). Examining interference of different cognitive tasks on voluntary balance control in aging and stroke. Exp. Brain Res. 234, 2575–2584. doi: 10.1007/s00221-016-4662-0
Blüchel, M., Lehmann, J., Kellner, J., and Jansen, P. (2013). The improvement in mental rotation performance in primary school-aged children after a two-week motor-training. J. Educ. Psychol. 33, 75–86. doi: 10.1080/01443410.2012.707612
Borg, F. G., and Laxåback, G. (2010). Entropy of balance–some recent results. J. Neuroeng. Rehabil. 7, 38. doi: 10.1186/1743-0003-7-38
Brecl, J. G., Remšak, T., Olenšek, J., Perellón-Alfonso, R., Šega Jazbec, S., Rot, U., et al. (2019). Visual but not verbal working memory interferes with balance in patients after optic neuritis suggestive of multiple sclerosis. Neurosci. Lett. 707, 134288. doi: 10.1016/j.neulet.2019.134288
Brysbaert, M. (2019). How many participants do we have to include in properly powered experiments? A tutorial of power analysis with reference tables. J. Cogn. 2, 16. doi: 10.5334/joc.72
Budde, K., Jöllenbeck, T., Barela, J. A., Figueiredo, G. A., and Weigelt, M. (2021). Mental body rotation with egocentric and object-based transformations in different postures: standing vs. balancing. BJMB 15, 180–194. doi: 10.20338/bjmb.v15i3.250
Burcal, C. J., Drabik, E. C., and Wikstrom, E. A. (2014). The effect of instructions on postural–suprapostural interactions in three working memory tasks. Gait Posture 40, 310–314. doi: 10.1016/j.gaitpost.2014.04.203
Chen, Y., Yu, Y., Niu, R., and Liu, Y. (2018). Selective effects of postural control on spatial vs. nonspatial working memory: a functional near-infrared spectral imaging study. Front. Hum. Neurosci. 12, 243. doi: 10.3389/fnhum.2018.00243
Cowan, N. (1988). Evolving conceptions of memory storage, selective attention, and their mutual constraints within the human information-processing system. Psychol. Bull. 104, 163–191. doi: 10.1037/0033-2909.104.2.163
Cowan, N. (2017). The many faces of working memory and short-term storage. Psychon. Bull. Rev 24, 1158–1170. doi: 10.3758/s13423-016-1191-6
Dault, M. C., Frank, J. S., and Allard, F. (2001). Influence of a visuo-spatial, verbal and central executive working memory task on postural control. Gait Posture 14, 110–116. doi: 10.1016/S0966-6362(01)00113-8
Dienes, Z. (2019). How do i know what my theory predicts? Adv. Meth. Pract. Psychol. Sci. 2, 364–377. doi: 10.1177/2515245919876960
Duarte, M., and Freitas, S. M. (2010). Revision of posturography based on force plate for balance evaluation. Braz. J. Phys. Ther. 14, 183–192. doi: 10.1590/S1413-35552010000300003
Engle, R. W. (2002). Working memory capacity as executive attention. Curr. Dir. Psychol. Sci. 11, 19–23. doi: 10.1111/1467-8721.00160
Faul, F., Erdfelder, E., Lang, A. G., and Buchner, A. (2007). G*Power 3: a flexible statistical power analysis program for the social, behavioral, and biomedical sciences. Behav. Res. Methods 39, 175–191. doi: 10.3758/BF03193146
Fujita, H., Kasubuchi, K., Osumi, M., and Morioka, S. (2016). Effects of the central executive on postural control. J. Motor Behav. 48, 270–276. doi: 10.1080/00222895.2015.1092935
Georgopoulos, A. P. (2000). Neural aspects of cognitive motor control. Curr. Opin. Neurobiol. 10, 238–241. doi: 10.1016/S0959-4388(00)00072-6
Haddad, J. M., Rietdyk, S., Claxton, L. J., and Huber, J. E. (2013). Task-dependent postural control throughout the lifespan. Exerc. Sport Sci. Rev. 41, 123–132. doi: 10.1097/JES.0b013e3182877cc8
Heil, M., and Rolke, B. (2002). Toward a chronopsychophysiology of mental rotation. Psychophysiol 39, 414–422. doi: 10.1111/1469-8986.3940414
Hofmann, P., and Jansen, P. (2023). The relation of mental rotation and postural stability. J. Motor Behav. 55, 580–593. doi: 10.1080/00222895.2021.1899113
Hofmann, P., Jost, L., and Jansen, P. (2023). Embodied mental rotation – does it affect postural stability? J. Motor Behav. 55, 202–219. doi: 10.1080/00222895.2022.2151970
Hyun, J. S., and Luck, S. J. (2007). Visual working memory as the substrate for mental rotation. Psychon. Bull. Rev. 14, 154–158. doi: 10.3758/BF03194043
Jansen, P., Lange, L., and Heil, M. (2011). The influence of juggling on mental rotation performance in children. Biomed. Hum. Kinet. 3, 18–22. doi: 10.2478/v10101-011-0005-6
JASP Team (2023). JASP (Version 0, 17.) [Computer Software]. Available online at: https://jasp-stats.org/ (accessed January 3, 2024).
Jolicœur, P., Regehr, S., Smith, L. B. J. P., and Smith, G. N. (1985). Mental rotation of representations of two-dimensional and three-dimensional objects. Can. J. Psychol. 39, 100–129. doi: 10.1037/h0080118
Jost, L., and Jansen, P. (2020). A novel approach to analyzing all trials in chronometric mental rotation and description of a flexible extended library of stimuli. Spat. Cogn. Comput 20, 234–256. doi: 10.1080/13875868.2020.1754833
Kaufman, S. B. (2007). Sex differences in mental rotation and spatial visualization ability: Can they be accounted for by differences in working memory capacity? Intelligence 35, 211–223. doi: 10.1016/j.intell.2006.07.009
Kawasaki, T., and Higuchi, T. (2016). Improvement of postural stability during quiet standing obtained after mental rotation of foot stimuli. J. Mot. Behav. 48, 357–364. doi: 10.1080/00222895.2015.1100978
Kawasaki, T., Yasuda, K., Fukuhara, K., and Higuchi, T. (2014). Relationship between mental rotation of body parts and postural stability during quiet stance. J. Imag. Res. Sport Phys. 9, 1–14. doi: 10.1515/jirspa-2014-0001
Kedziorek, J., and Błazkiewicz, M. (2020). Nonlinear measures to evaluate upright postural stability: a systematic review. Entropy 22, 1–14. doi: 10.3390/e22121357
Kerr, B., Condon, S. M., and McDonald, L. A. (1985). Cognitive spatial processing and the regulation of posture. J. Exp. Psychol. Hum. Percept Perform 11, 617–622. doi: 10.1037/0096-1523.11.5.617
Kessels, R. P., van Zandvoort, M. J., Postma, A., Kappelle, L. J., and de Haan, E. H. (2000). The corsi block-tapping task: standardization and normative data. Appl. Neuropsychol. 7, 252–258. doi: 10.1207/S15324826AN0704_8
Kessler, K., and Rutherford, H. (2010). The two forms of visuo-spatial perspective taking are differently embodied and subserve different spatial prepositions. Front. Psychol. 1, 213. doi: 10.3389/fpsyg.2010.00213
Kim, S. G. (2023). Using ChatGPT for language editing in scientific articles. Maxillofac Plast Reconstr. Surg. 45, 13. doi: 10.1186/s40902-023-00381-x
Klauer, K. C., and Zhao, Z. (2004). Double dissociations in visual and spatial short-term memory. J. Exp. Psychol. Gen. 133, 355–381. doi: 10.1037/0096-3445.133.3.355
Koltermann, J., Gerber, M., Beck, H., and Beck, M. (2018). Validation of various filters and sampling parameters for a COP analysis. Technologies 6, 56. doi: 10.3390/technologies6020056
Linn, M. C., and Petersen, A. C. (1985). Emergence and characterization of sex differences in spatial ability: a meta-analysis. Child Dev. 56, 1479. doi: 10.2307/1130467
Mathôt, S., Schreij, D., and Theeuwes, J. (2012). OpenSesame: an open-source, graphical experiment builder for the social sciences. Behav. Res. Methods 44, 314–324. doi: 10.3758/s13428-011-0168-7
Maylor, E. A., and Wing, A. M. (1996). Age differences in postural stability are increased by additional cognitive demands. J. Gerontol. Ser. B Psychol. Sci. Soc. Sci. 51, P143–P154. doi: 10.1093/geronb/51b.3.p143
Menant, J. C., Sturnieks, D. L., Brodie, M. A. D., Smith, S. T., and Lord, S. R. (2014). Visuospatial tasks affect locomotor control more than nonspatial tasks in older people. PLoS ONE 9, e109802. doi: 10.1371/journal.pone.0109802
Mueller, S. T., and Piper, B. J. (2014). The psychology experiment building language (PEBL) and PEBL test battery. J. Neurosci. Methods 222, 250–259. doi: 10.1016/j.jneumeth.2013.10.024
Nuzzo, R. L. (2017). An introduction to bayesian data analysis for correlations. PMandR 9, 1278–1282. doi: 10.1016/j.pmrj.2017.11.003
Palmieri, R. M., Ingersoll, C. D., Stone, M. B., and Krause, B. A. (2002). Center-of-pressure parameters used in the assessment of postural control. J. Sport Rehabil. 11, 51–66. doi: 10.1123/jsr.11.1.51
Pellecchia, G. L. (2003). Postural sway increases with attentional demands of concurrent cognitive task. Gait Posture 18, 29–34. doi: 10.1016/S0966-6362(02)00138-8
Pietsch, S., Böttcher, C., and Jansen, P. (2017). Cognitive motor coordination training improves mental rotation performance in primary school-aged children. Mind Brain Educ. 11, 176–180. doi: 10.1111/mbe.12154
Pietsch, S., and Jansen, P. (2012). Different mental rotation performance in students of music, sport and education. J. Individ. Differ. 22, 159–163. doi: 10.1016/j.lindif.2011.11.012
Prieto, T. E., Myklebust, J. B., Hoffmann, R. G., Lovett, E. G., and Myklebust, B. M. (1996). Measures of postural steadiness: differences between healthy young and elderly adults. IEEE. Trans. Biomed. 43, 956–966. doi: 10.1109/10.532130
Ramdani, S., Seigle, B., Lagarde, J., Bouchara, F., and Bernard, P. L. (2009). On the use of sample entropy to analyze human postural sway data. Med. Eng. Phys. 31, 1023–1031. doi: 10.1016/j.medengphy.2009.06.004
Rhea, C. K., Kiefer, A. W., Haran, F. J., Glass, S. M., and Warren, W. H. (2014). A new measure of the CoP trajectory in postural sway: dynamics of heading change. Med. Eng. Phys. 36, 1473–1479. doi: 10.1016/j.medengphy.2014.07.021
Rhea, C. K., Kiefer, A. W., Wright, W. G., Raisbeck, L. D., and Haran, F. J. (2015). Interpretation of postural control may change due to data processing techniques. Gait Posture 41, 731–735. doi: 10.1016/j.gaitpost.2015.01.008
Rhea, C. K., Silver, T. A., Hong, S. L., Ryu, J. H., Studenka, B. E., Hughes, C. M. L., et al. (2011). Noise and complexity in human postural control: interpreting the different estimations of entropy. PLoS ONE 6, e17696. doi: 10.1371/journal.pone.0017696
Shepard, R. N., and Metzler, J. (1971). Mental rotation of three-dimensional objects. Science 171, 701–703. doi: 10.1126/science.171.3972.701
Shumway-Cook, A., and Woollacott, M. (2007). Motor Control: Translating Research Into Clinical Practice. Philadelphia, PA: Lippincott Williams and Wilkins.
Smith, E. E., Jonides, J., Koeppe, R. A., Awh, E., Schumacher, E. H., Minoshima, S., et al. (1995). Spatial versus object working memory: PET investigations. J. Cogn. Neurosci. 7, 337–356. doi: 10.1162/jocn.1995.7.3.337
Smulders, K., van Nimwegen, M., Munneke, M., Bloem, B. R., Kessels, R. P. C., Esselink, R. A. J., et al. (2013). Involvement of specific executive functions in mobility in Parkinson's disease. Parkinsonism Relat. Disord. 19, 126–128. doi: 10.1016/j.parkreldis.2012.06.010
Steggemann, Y., Engbert, K., and Weigelt, M. (2011). Selective effects of motor expertise in mental body rotation tasks: comparing object-based and perspective transformations. Brain Cogn. 76, 97–105. doi: 10.1016/j.bandc.2011.02.013
Useros Olmo, A. I., Periañez, J. A., Martínez-Pernía, D., and Miangolarra Page, J. C. (2020). Effects of spatial working memory in balance during dual tasking in traumatic brain injury and healthy controls. Brain INJ 34, 1159–1167. doi: 10.1080/02699052.2020.1792984
Uttal, D. H., Meadow, N. G., Tipton, E., Hand, L. L., Alden, A. R., Warren, C., et al. (2013). The malleability of spatial skills: a meta-analysis of training studies. Psychol. Bull. 139, 352–402. doi: 10.1037/a0028446
VanderVelde, T. J., Woollacott, M. H., and Shumway-Cook, A. (2005). Selective utilization of spatial working memory resources during stance posture. NeuroReport 16, 773–777. doi: 10.1097/00001756-200505120-00023
Voyer, D., Butler, T., Cordero, J., Brake, B., Silbersweig, D., Stern, E., et al. (2006). The relation between computerized and paper-and-pencil mental rotation tasks: a validation study. J. Clin. Exp. Neuropsychol. 28, 928–939. doi: 10.1080/13803390591004310
Voyer, D., and Jansen, P. (2017). Motor expertise and performance in spatial tasks: a meta-analysis. Hum. Mov. Sci. 54, 110–124. doi: 10.1016/j.humov.2017.04.004
Wagenmakers, E.-J., Marsman, M., Jamil, T., Ly, A., Verhagen, J., Love, J., et al. (2018). Bayesian inference for psychology. Part I: theoretical advantages and practical ramifications. Psychon. Bull. Rev. 25, 35–57. doi: 10.3758/s13423-017-1343-3
Wexler, M., Kosslyn, S. M., and Berthoz, A. (1998). Motor processes in mental rotation. Cognition 68, 77–94. doi: 10.1016/S0010-0277(98)00032-8
Winter, D. A. (1995). Human balance and posture control during standing and walking. Gait Posture 3, 193–214. doi: 10.1016/0966-6362(96)82849-9
Wohlschläger, A., and Wohlschläger, A. (1998). Mental and manual rotation. J. Exp. Psychol. Hum. Percept. Perform 24, 397–412. doi: 10.1037/0096-1523.24.2.397
Woollacott, M., and Shumway-Cook, A. (2002). Attention and the control of posture and gait: a review of an emerging area of research. Gait Posture 16, 1–14. doi: 10.1016/S0966-6362(01)00156-4
Keywords: mental rotation, postural stability, visuospatial sketchpad, working memory, center of pressure
Citation: Hofmann P, Siebertz M and Jansen P (2024) No role of working memory in the relation between mental rotation and postural stability. Front. Cognit. 2:1298371. doi: 10.3389/fcogn.2023.1298371
Received: 21 September 2023; Accepted: 22 December 2023;
Published: 16 January 2024.
Edited by:
Claudia Voelcker-Rehage, University of Münster, GermanyReviewed by:
Melanie Krüger, Leibniz University of Hannover, GermanyVeronica Muffato, University of Padua, Italy
Copyright © 2024 Hofmann, Siebertz and Jansen. This is an open-access article distributed under the terms of the Creative Commons Attribution License (CC BY). The use, distribution or reproduction in other forums is permitted, provided the original author(s) and the copyright owner(s) are credited and that the original publication in this journal is cited, in accordance with accepted academic practice. No use, distribution or reproduction is permitted which does not comply with these terms.
*Correspondence: Philipp Hofmann, philipp.hofmann@ur.de