Performance analysis of a hybrid optical amplifier based 480-Gbps DWDM-FSO system under the effect of different atmospheric conditions
- 1Department of Electrical Engineering Technology, Punjab Tianjin University of Technology, Lahore, Pakistan
- 2NFC Institute of Engineering and Technology Multan, Multan, Pakistan
- 3University of Sargodha, Sargodha, Pakistan
- 4Department of Electrical Engineering, University of Engineering and Technology, Lahore, Pakistan
- 5Department of Telecommunication Engineering, University of Engineering and Technology, Taxila, Pakistan
- 6Department of Electrical Engineering, Superior University, Lahore, Pakistan
Free space optical (FSO) systems offer a desirable and affordable way of providing communication services in remote locations. They provide secure wireless transmission without the requirement for licensing and with lower implementation costs. However, due to the impact of atmospheric turbulences on the effectiveness of FSO systems, their implementation faces significant challenges. Dense wavelength division multiplexing (DWDM) has shown promise in addressing the growing bandwidth needs in optical networks. This paper introduces a hybrid optical amplifier based system, combining DWDM and FSO technology. Energy conservation in such a system involves optimizing various aspects to reduce power consumption while maintaining or improving performance. The proposed DWDM-FSO system operates at a rate of 480 Gbps and consists of 12 channels, each capable of transmitting data at 40 Gbps. The system's performance is evaluated and compared by determining the Q-factor and bit error rate (BER) for both the cases when hybrid amplifier is employed and with no hybrid amplifier within the C-band, specifically focusing on wavelengths around 1550 nm. Moreover, the length of the FSO link is increased to assess the corresponding Q-factor and BER. Resultantly, the maximum distance for the FSO link is determined, ensuring that it remains within acceptable Q-factor and BER thresholds. Furthermore, the FSO system's effectiveness is assessed and compared across various atmospheric conditions. The findings reveal that, in clear weather conditions, the FSO system achieves a maximum distance of 510 meters while maintaining satisfactory Q-factor and BER values when not utilizing a hybrid amplifier. However, by integrating a hybrid amplifier, the system's reach significantly extends to 1700 m under clear weather conditions, still maintaining acceptable Q-factor and BER values.
1 Introduction
A major revolution in communication services has been made possible by optical fiber technology, which completely transformed the way huge amounts of data are effectively carried across very long distances (Noor et al., 2022). It serves as the backbone of modern telecommunications, allowing for the smooth transfer of data through large fiber networks that span across wide geographic areas (Liu et al., 2023). Optical amplifiers play an essential role in this environment of optical transmissions by assuring continuous propagation of signals, which ensures reliable and effective communication (Zaid et al., 2023). However, optical fiber communication has some challenges related to point-to-point communication, cable faults, and physical connectivity costs (Al-Nahhal et al., 2022). The adoption of free space optical communication (FSO) systems offer an appealing solution that overcomes these constraints and provides a number of benefits, including enhanced flexibility, reduced vulnerability, and lower infrastructure costs (Al-Gailani et al., 2020).
FSO is a state-of-the-art wireless optical communication system that uses light to transmit data through spaces, such as vacuum and air. FSO offers various advantages, including high bandwidth capacity, secure transmission, and immunity to electromagnetic interference (Mohsan et al., 2023). The capacity of FSO communication to transfer massive amounts of data over great distances without the requirement for licensing is one of its key advantages. This makes it an effective alternative in situations where traditional wired or radio frequency-based wireless communication may not be feasible or beneficial. FSO allows the transmission of data at significantly high speeds, reaching up to gigabits per second. Furthermore, remarkable developments in research have realized terabit per second speeds in certain environments (Bag et al., 2018). FSO links have a wide range of applications, including connecting aircraft, enabling satellite-to-satellite communication, establishing terrestrial connections, and serving the rigorous requirements of the military (Lu et al., 2022).
FSO offers notable advantages in terms of maintenance and enhancement, when compared with other communication technologies. FSO systems often employ compact and easy-to-install devices, requiring minimal infrastructure. However, a direct line of sight between the transmitting and receiving equipment is one of the essential conditions for FSO to operate efficiently (Jahid et al., 2022). This means that any obstacles, including buildings or trees, may interfere with the signal's ability to travel. Nevertheless, it is crucial to take into account how atmospheric conditions affect FSO systems. Conditions like fog, rain, or snow can degrade or disperse the optical signals, affecting the range and dependability of FSO links. These factors can considerably reduce the effectiveness of FSO systems during adverse weather conditions (Chaudhry and Yanikomeroglu, 2020).
High-capacity, long-distance communication can be achieved by combining FSO technology with dense wavelength division multiplexing (DWDM). In a system like this, energy conservation involves optimizing a number of factors to reduce power consumption without sacrificing performance (Mohsan et al., 2022). Some strategies to enhance energy conservation in a DWDM-FSO system are: choose power-efficient components like lasers and photodetectors, implement adaptive power control mechanisms to dynamically adjust transmission power based on link conditions, employ advanced modulation schemes to achieve higher data rates with reduced power consumption, apply dynamic resource allocation algorithms for optimizing wavelength and power level allocation based on traffic demand, optimize signal processing algorithms for energy efficiency at both transmitter and receiver ends, and choose optical amplifiers with high efficiency to minimize power consumption in signal amplification along the optical fiber. These approaches collectively contribute to improved energy efficiency, reducing operational costs and environmental impact while maintaining reliable and high-capacity communication in the DWDM-FSO system (Hayal et al., 2021).
FSO technology has seen an enormous rise in interest from researchers as a result of the rapid expansion of wireless networks. Numerous research articles that highlight different FSO systems features and uses have been published in the literature (Jahid et al., 2022). Hayal et al. (2021) proposed a DWDM based FSO system operating in the presence of atmospheric turbulence. They proposed a hybrid modulation technique to address issues related to signal degradation and increased bit error rate (BER). The key performance metrics such as BER, signal-to-noise ratio (SNR), and system capacity are evaluated and the comparison is performed with conventional modulation techniques. Niaz et al. (2019) focused on the performance analysis of a FSO system working under chaotic conditions. The BER and channel capacity of the system are examined under different atmospheric conditions, including clear weather, fog, rain, and turbulence. They used chaotic modulation techniques while assessing the system's performance. Rashidi et al. (2017) addressed the issue of signal degradation in FSO systems caused by rain attenuation, which can severely affect the transmission performance. They proposed a spectrum slicing wavelength division multiplexing (WDM) approach as a potential solution to lessen the negative effects of persistent rain on FSO communication. Fadil et al. (2022) demonstrated a WDM-FSO system that utilizes optical chaotic signals for enhanced security. The system's performance is assessed while taking into account a number of factors, including BER, transmission distance, and SNR. Their findings showed that the chaotic-based FSO system offers better security than conventional systems. Singh et al. (2021) examined the performance of a hybrid transmission system that combines WDM, polarization-division multiplexing (PDM), and orthogonal frequency-division multiplexing (OFDM) techniques for FSO link. They realized a 1.6 Tbps transmission link and estimated its performance in terms of various parameters under different weather conditions. Their findings indicated that the hybrid WDM-PDM-OFDM-based FSO link realized high data rates with good signal quality and low BER.
Hybrid optical amplifier combines two or more different amplification technologies to obtain enhanced performance and flexibility (Obaid and Shahid, 2020). Modern optical communication systems are finding hybrid optical amplifiers as a valuable asset. They can provide improved signal quality, wideband amplification, dynamic gain control, and network design flexibility by combining the advantages of various amplifier technologies (Ali et al., 2020). Hybrid amplifiers have a substantial impact on the ability to transmit data efficiently and reliably over long distances due to their power efficiency and flexibility with different network topologies. As optical networks continue to evolve, the significance of hybrid optical amplifiers is set to grow, enabling the development of advanced communication infrastructures (Obaid et al., 2023). In this paper, a hybrid optical amplifier based 480-Gbps DWDM-FSO system is proposed and the performance is analyzed under different atmospheric conditions. The paper is organized in four sections. After introduction, the proposed system is described in Section 2, preceded by the results in Section 3. The research concludes with Section 4.
2 Proposed setup
Figure 1 shows the schematic of the proposed hybrid optical amplifier based DWDM-FSO system. This optical communication system combines the advantages of both DWDM and FSO technologies and designed to transmit data over a span of wavelengths, specifically covering the 1546 nm−1555 nm range with 12 channels spaced 0.8 nm apart. The optical sources for these channels are continuous wave lasers, which provide stable and coherent signals. The channels are driven by a data stream of 40 Gb/s, consisting of NRZ modulated signals, each carrying an input power of −20 dBm. A wavelength division multiplexer is used to effectively multiplex different channels. The use of this component enables the simultaneous transmission of all data channels over a single link by combining the optical signals from various data channels. The modulated signals undergo initial amplification through hybrid optical amplifier before being transmitted via the FSO link. The hybrid amplifier consists of a combination of erbium-doped fiber amplifier (EDFA) and Er-Yb co-doped fiber amplifier (EYDFA). On the receiver side of the FSO link, a demultiplexer is responsible for dividing the combined optical signal back into its respective channels. This procedure permits the receiver to separate and extract the individual data streams carried by each channel for additional processing. After the signal has been demultiplexed, it is directed to a PIN photodetector. Efficient conversion of incoming optical signals into electrical currents is ensured using a photodetector with a responsivity of 0.9 A/W and a dark current of 1 nA. A BER tester is employed to assess the quality of the received data. Simulations of the proposed system are conducted using OptiSystem, a powerful software tool particularly designed for optical communication systems. Moreover, MATLAB is used for detailed analysis, enabling further understanding of the behavior of the system.
The signal first enters the EDFA stage in the hybrid amplifier, and then it undergoes further amplification as it is fed into the cascaded EYDFA. EDFA is a vital amplifier widely used in optical communication networks, providing high gain, wide bandwidth, and low noise amplification for optical signals in the C-band (Fang et al., 2021). Its flexibility and effectiveness make it an integral component in building reliable and high-capacity fiber-optic networks. The EDFA theoretical model is demonstrated in detail in Liu et al. (2021) and the parameters are listed in Table 1. Following amplification from EDFA, the signals are subsequently fed into a forward pumped EYDFA. For C-band communication, an EYDFA is commonly utilized because it offers significant gain and a low noise figure (NF). Moreover, it offers additional advantages of higher pump efficiency and wide pump absorption band of 800 nm to 1100 nm (Muhd-Yassin et al., 2012). The broad absorption range allows for flexibility in selecting the pump wavelength. In our proposed setup, a 1057 nm co-propagating pump with a power of 90 mW is employed to induce ion excitation within the EYDFA. The theoretical model for the EYDFA's operation is thoroughly discussed in Mahran (2016) and the parameters are summarized in Table 2.
The FSO link comprises of three main components: the transmitter, FSO channel, and the receiver. Determining the power received at the receiver is a crucial step in analyzing FSO link's performance. To ensure reliable communication, it is necessary to take into account a safety margin due to the many environmental conditions that can weaken the signal during transmission. This safety margin is known as the “link margin.” Link margin is defined as the magnitude relation of the received power and the receiver's threshold or sensitivity. Alternatively, it indicates the difference between the received power and the minimum power necessary for the receiver to accurately detect and decode the transmitted data. The link margin can be expressed in decibels as (Parkash et al., 2016).
where LM is the link margin; PR is the received power at the receiver and S is the receiver threshold or sensitivity. For successful signal recovery at the receiver, it is essential that the power of the incoming signal exceeds the receiver's sensitivity level. Power at receiver is given as (Parkash et al., 2016):
where PT and PR are, respectively, the power at the transmitter and receiver; α denotes atmospheric attenuation; L is the distance between transmitter and receiver; ARX denotes receiver aperture area and θ represents divergence angle. Equation (2) shows that the power received at the receiver depends directly on the transmit power and receiver aperture area, whereas it is inversely related to the link range and divergence angle. Therefore, by raising the transmitter power and receiver area, or by reducing the beam divergence of the transmitter beam, the received power can be increased. The exponential component of the equation is associated with atmospheric attenuation and has the greatest impact on the link quality.
Table 3 shows the various system and FSO link parameters used in the simulation of proposed system, while Table 4 provides a summary of the attenuation values associated with various atmospheric conditions (Nadeem et al., 2018).
3 Results and discussion
The performance of the proposed system is determined in terms of Q-factor and BER under two different scenarios: when using a hybrid amplifier and when not using one. Furthermore, the length of the FSO link is increased to evaluate the corresponding Q-factor and BER. Also, the performance of the FSO system is examined under various atmospheric conditions.
3.1 Gain and noise figure
Figure 2A shows the gain spectra of the hybrid amplifier as a function of signal wavelengths, along with the gains of individual EDFA and EYDFA stages at an input power of −20 dBm per channel. The hybrid amplifier achieves a gain of more than 30.16 dB at each wavelength, with the highest gain occurring at 1554.8 nm. Gain ripple of 1.28 dB is attained over the selected wavelength range. The obtained better gain flatness ensures consistent and reliable amplification across the wavelength range of interest. Figure 2C shows a comparison of the average gain for the proposed amplifiers. The EDFA and EYDFA amplifications resulted in average gains of 18.51 dB and 12.42 dB, respectively. However, when combined in the hybrid configuration, the average gain significantly increased to 30.93 dB. Therefore, it is observed that the hybrid configuration offers superior performance, delivering high gain while maintaining the lowest gain ripple.
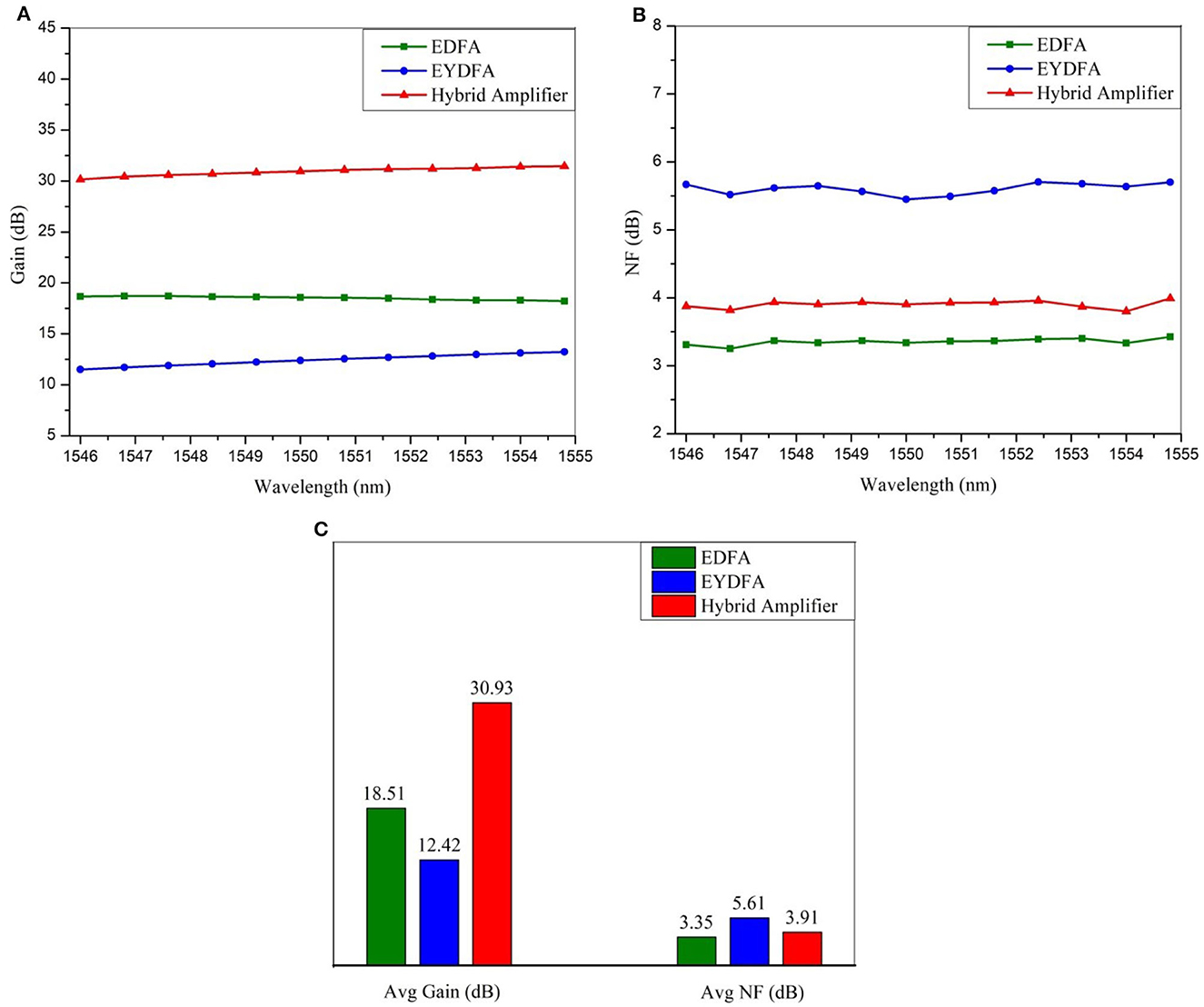
Figure 2. (A) Gain spectra, (B) NF spectra and (C) average gain and NF values of hybrid and individual amplification stages.
The noise figure (NF) profiles of the suggested amplifiers as a function of channel wavelengths are displayed in Figure 2B. It shows that the hybrid amplifier maintains an NF value of < 3.99 dB across the selected wavelength range. The average NF depicted in Figure 2C indicates that the EDFA exhibits the lowest value of 3.35 dB. The hybrid amplifier also exhibits better results, achieving an average NF of 3.91 dB. These results highlight the effectiveness of the hybrid configuration, demonstrating its potential as a means of improving amplification performance and signal quality in optical communication systems.
3.2 Q-factor and BER performance
In a communication system, the estimation of transmission quality and degradation is critical to ensure reliable data transfer. The Q-factor and BER are two commonly used metrics to evaluate this performance (Sandalidis et al., 2008). The crosstalk plays a significant role in impacting the Q-factor and BER of a system. Crosstalk-induced interference can result in signal degradation, increasing the likelihood of errors in the transmitted data. Maintaining optimal communication performance requires minimizing crosstalk (Elsayed et al., 2018).
For proposed system, the Q-factor and BER of the received electrical signals have been investigated and the results are presented in Figures 3A, B, respectively. These values are measured at FSO length of 1600 m. A BER within the range of 10−09 or lower indicates that the signal has been acquired with satisfactory quality. In the scenario where no hybrid amplifier is utilized before the FSO link, the obtained Q-factor is < 2.01 dB, and the BER is >10−02. However, these values are regarded as unsuitable for reliable and high-performance FSO communication. The option to place a hybrid amplifier before the FSO link has a considerable positive impact on the system's performance. With the same FSO parameters, the results attained with the hybrid amplifier are highly satisfactory. At each wavelength, a much-improved Q-factor of < 6 dB and low BER of >10−09 are obtained. Consequently, the overall performance of the proposed system shows a significant improvement when the hybrid amplifier is employed.
The impact of increasing the FSO range on Q-factor, BER and received power are investigated and the obtained values are plotted in Figure 4. These values are measured at the first channel of the proposed system. In the first scenario (without a hybrid amplifier), the results showed that as the FSO link length is increased from 400 m to 2000 m: the Q-factor decreases from 7.65 dB to 1.83 dB, BER increases from 10−12 to a much higher value of 10−02 and the received power at the output of the FSO link decreases from −17.61 dBm to −31.54 dBm. In the second scenario (with a hybrid amplifier), the results showed that as the FSO link length is increased from 400 m to 2000 m: the Q-factor decreases from 15.45 dB to 4.07 dB, BER increases from 10−31 to 10−05 and the received power at the output of the FSO link decreases from 6.42 dBm to −7.51 dBm. In summary, increasing the FSO range had adverse effects on the performance of the system. The system with hybrid amplifier offers some improvement in mitigating these effects, resulting in better Q-factor, BER, and received power compared to the system without an amplifier.
3.3 Investigation of the effects of different atmospheric conditions on the performance of FSO system
The Q-factor and BER values are determined for the proposed system under adverse weather conditions, and the results as a function of signal wavelengths are presented in Figures 5A, B, respectively. These values are calculated at FSO length of 1600 m. It is observed that under clear weather conditions, the minimum Q-factor achieved is 6 dB, which indicates a relatively good signal quality. The corresponding maximum BER value is 10−09. In the presence of little fog weather, the minimum Q-factor obtained is 4.84 dB. The Q-factor is slightly reduced compared to clear weather, indicating a decrease in signal quality due to the fog. The corresponding maximum BER value is 10−06. The minimum Q-factor drops even more to 4.09 dB in the presence of light rain. This drop in Q-factor indicates a significant degradation in signal quality as a result of rain attenuation. The maximum BER is measured at 10−05, indicating a higher occurrence of bit errors compared to both clear weather and little fog conditions. Under moderate fog conditions, the minimum Q-factor recorded is 2.9 dB. The maximum BER reaches 10−03, signifying a substantial increase in the probability of bit errors. In the case of heavy rain, the minimum Q-factor observed is 2.35 dB. The heavy rain causes severe signal degradation, resulting in a further reduction in signal quality. The maximum BER in this scenario is 10−02. Finally, under dry snow conditions, the minimum Q-factor observed is 1.39 dB, which is the lowest value among all weather conditions. The dry snow considerably reduces signal quality due to scattering and absorption effects. The maximum BER reaches 10−01, indicating a significant number of bit errors and greatly affected data transmission. In summary, these results demonstrate the sensitivity of the system's performance to challenging weather conditions. The system performs well in clear weather conditions, with a high Q-factor and low BER values. However, if the weather gets worse, both the Q-factor and BER are adversely affected, which lowers the signal quality and raises the probability of bit errors.
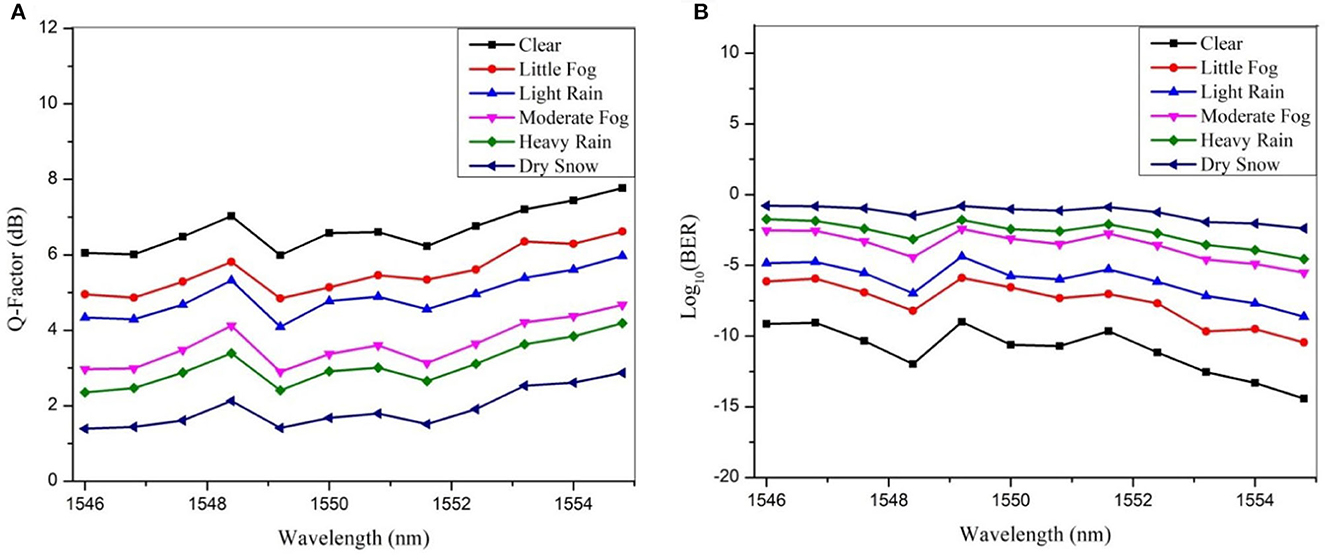
Figure 5. (A) Q-factor and (B) BER as a function of signal wavelengths under different weather conditions.
Tables 5, 6 list the maximum FSO link distance possible under various weather conditions, ensuring acceptable Q-factor and BER values for two scenarios: one without a hybrid amplifier and the other with a hybrid amplifier. In Table 5, it is clear that the maximum FSO distance attainable in clear weather conditions while maintaining acceptable Q-factor and BER values is 510 m. However, the maximum FSO distance reduces considerably under adverse weather conditions. The minimum feasible distance is only 50 m in dry snow conditions. According to Table 6, with the incorporation of a hybrid amplifier, the maximum FSO distance achievable under clear weather conditions with acceptable Q-factor and BER values increases to 1700 m. In a manner similar to the previous instance, the maximum FSO distance decreases under challenging weather conditions. The maximum possible distance in dry snow conditions is only 210 m.
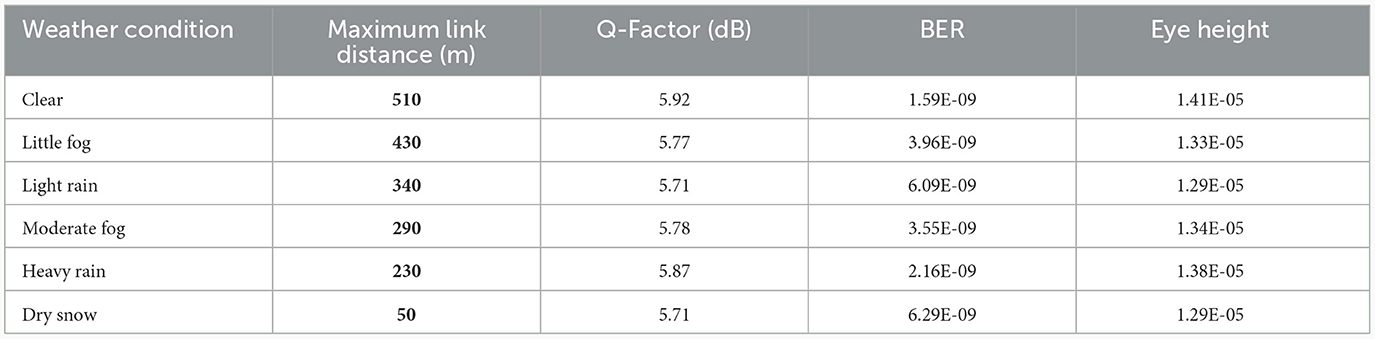
Table 5. Maximum FSO link distance possible with acceptable Q-factor and BER values for different weather conditions (system without hybrid amplifier).
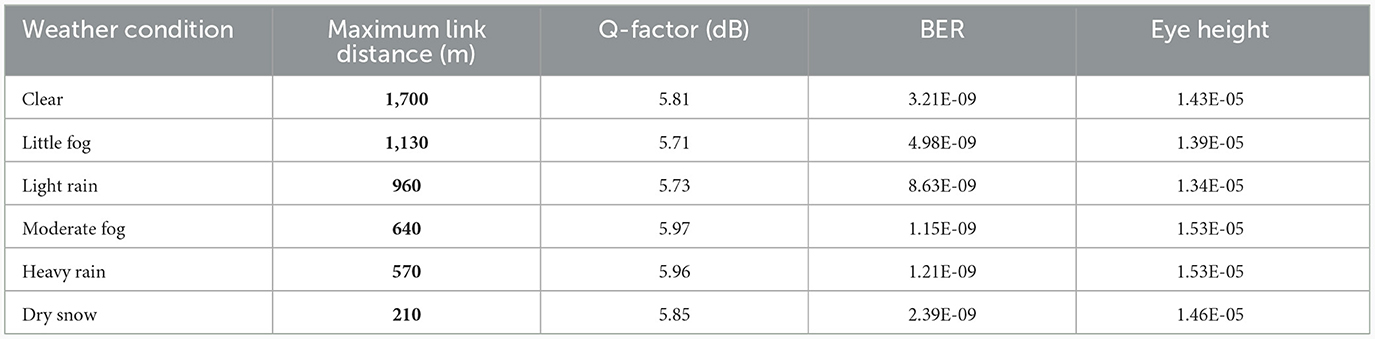
Table 6. Maximum FSO link distance possible with acceptable Q-factor and BER values for different weather conditions (system with hybrid amplifier).
Figure 6 shows the measured eye diagrams, obtained at the first channel of the proposed system under clear weather conditions. In Figure 6A, the eye diagram is obtained for the system with the hybrid amplifier case. It can be seen that the eye diagram exhibits a wider opening, indicating a robust signal with reduced noise and distortion. On the other hand, Figure 6B displays the eye diagram for the system without the hybrid amplifier case. Comparing this to Figure 6A, a visibly narrower eye opening can be observed. The reduced eye height in this case indicates increased signal degradation. Resultantly, the comparison of the two eye diagrams clearly demonstrates that employing the hybrid amplifier in the proposed system leads to significantly improved performance.
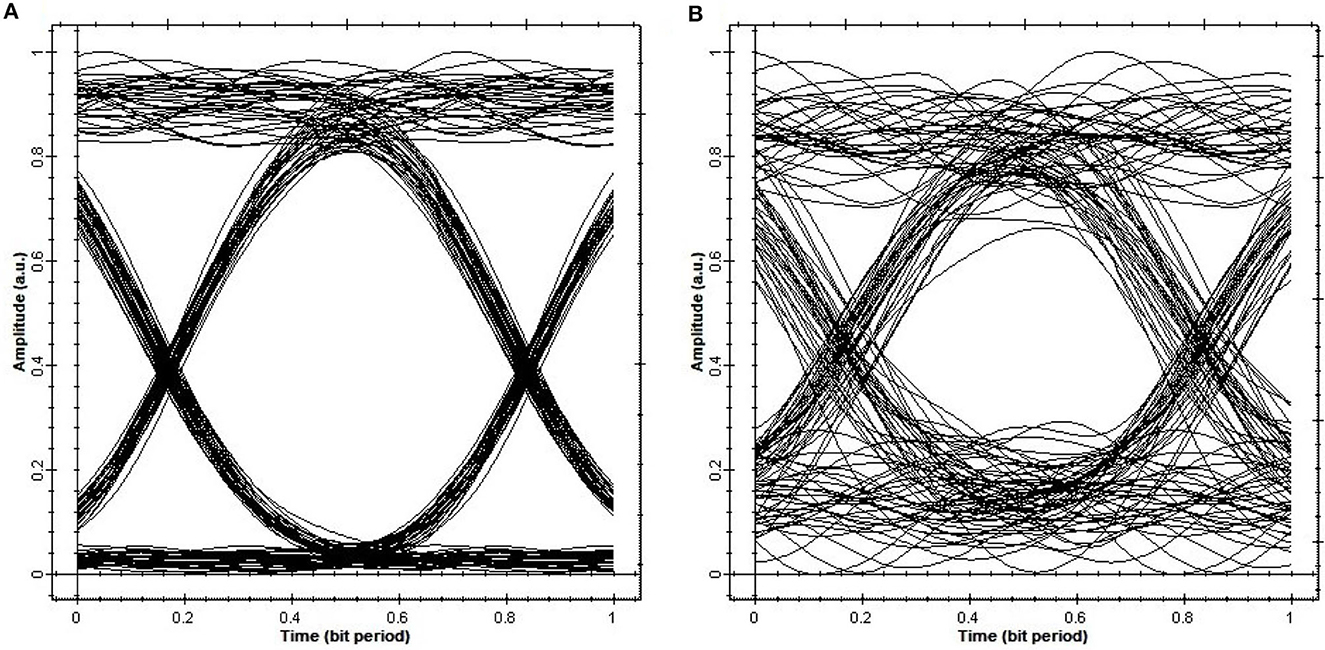
Figure 6. Eye diagrams obtained at first channel of proposed system for (A) with hybrid amplifier and (B) without hybrid amplifier case under clear weather conditions.
4 Conclusion
This paper demonstrates a hybrid optical amplifier based 480-Gbps DWDM-FSO system. The performance of the system is assessed by analyzing the Q-factor and BER in the C-band, with an emphasis on wavelengths around 1550 nm. In addition, the length of the FSO link is varied to analyze the corresponding Q-factor and BER. It is essential for determining the maximum distance that the FSO link can cover while maintaining acceptable Q-factor and BER values. Moreover, the effectiveness of the FSO system is also evaluated under varying atmospheric conditions. Consequently, the proposed system offers a robust and high-capacity solution for transmitting multiple data channels over the FSO link. By amplifying the signals using hybrid optical amplifier, this system can provide reliable and high-speed communication over long distances without the need for traditional optical fibers.
Data availability statement
The raw data supporting the conclusions of this article will be made available by the authors, without undue reservation.
Author contributions
HO: Writing—original draft. SA: Writing—review & editing. MN: Project administration, Writing—review & editing. HS: Methodology, Writing—original draft. AA: Investigation, Writing—review & editing. MZ: Validation, Writing—original draft.
Funding
The author(s) declare that no financial support was received for the research, authorship, and/or publication of this article.
Conflict of interest
The authors declare that the research was conducted in the absence of any commercial or financial relationships that could be construed as a potential conflict of interest.
Publisher's note
All claims expressed in this article are solely those of the authors and do not necessarily represent those of their affiliated organizations, or those of the publisher, the editors and the reviewers. Any product that may be evaluated in this article, or claim that may be made by its manufacturer, is not guaranteed or endorsed by the publisher.
References
Al-Gailani, S. A., Salleh, M. F. M., Salem, A. A., Shaddad, R. Q., Sheikh, U. U., Algeelani, N. A., et al. (2020). A survey of free space optics (FSO) communication systems, links, and networks. IEEE Access 9, 7353–7373. doi: 10.1109/ACCESS.2020.3048049
Ali, M. H., Abdullah, F., and Al-Mashhadani, T. F. (2020). Gain-control technique in double-pass parallel hybrid fiber amplifier. Optical Quantum Electr. 52, 1–7. doi: 10.1007/s11082-020-02508-0
Al-Nahhal, M., Al-Nahhal, I., Dobre, O. A., Soman, S. K. O., Chang, D., Li, C., et al. (2022). Learned signal-to-noise ratio estimation in optical fiber communication links. IEEE Photonics J. 14, 1–7. doi: 10.1109/JPHOT.2022.3222264
Bag, B., Das, A., Ansari, I. S., Prokeš, A., Bose, C., Chandra, A., et al. (2018). Performance analysis of hybrid FSO systems using FSO/RF-FSO link adaptation. IEEE Photonics J. 10, 1–17. doi: 10.1109/JPHOT.2018.2837356
Chaudhry, A. U., and Yanikomeroglu, H. (2020). Free space optics for next-generation satellite networks. IEEE Consum. Electr. Magazine 10, 21–31. doi: 10.1109/MCE.2020.3029772
Elsayed, E. E., Yousif, B. B., and Alzalabani, M. M. (2018). Performance enhancement of the power penalty in DWDM FSO communication using DPPM and OOK modulation. Optical Quantum Electr. 50, 1–36. doi: 10.1007/s11082-018-1508-y
Fadil, E. A., Abass, A. K., and Tahhan, S. R. (2022). Secure WDM-free space optical communication system based optical chaotic. Optical Quantum Electr. 54, 477. doi: 10.1007/s11082-022-03870-x
Fang, Y., Zeng, Y., Qin, Y., Xu, O., Li, J., Fu, S., et al. (2021). Design of ring-core few-mode-EDFA with the enhanced saturation input signal power and low differential modal gain. IEEE Photonics J. 13, 1–6. doi: 10.1109/JPHOT.2021.3095123
Hayal, M. R., Yousif, B. B., and Azim, M. A. (2021). Performance enhancement of DWDM-FSO optical fiber communication systems based on hybrid modulation techniques under atmospheric turbulence channel. Photonics 8, 464. doi: 10.3390/photonics8110464
Jahid, A., Alsharif, M. H., and Hall, T. J. (2022). A contemporary survey on free space optical communication: Potentials, technical challenges, recent advances and research direction. J. Netw. Comput. Appl. 200, 103311. doi: 10.1016/j.jnca.2021.103311
Liu, Y., Liu, X., Liu, L., Zhang, Y., Cai, M., Yi, L., et al. (2021). Modeling EDFA gain: approaches and challenges. Photonics 8:417. doi: 10.3390/photonics8100417
Liu, Z., Tianhua, X., Ji, Q., Joshua, U., Jian, Z., Tiegen, L., et al. (2023). Achievable information rate optimization in C-band optical fiber communication system. Front. Optoelectr. 16, 17. doi: 10.1007/s12200-023-00072-5
Lu, R. R., Wang, J. Y., Fu, X. T., Lin, S. H., Wang, Q., Zhang, B., et al. (2022). Performance analysis and optimization for UAV-based FSO communication systems. Phys. Commun. 51, 101594. doi: 10.1016/j.phycom.2021.101594
Mahran, O. (2016). Gain and noise figure enhancement of Er+ 3/Yb+ 3 co-doped fiber/Raman hybrid amplifier. Optical Mat. 52, 100–106. doi: 10.1016/j.optmat.2015.12.018
Mohsan, S. A. H., Khan, M. A., and Amjad, H. (2023). Hybrid FSO/RF networks: a review of practical constraints, applications and challenges. Optical Switching Netw. 47, 100697. doi: 10.1016/j.osn.2022.100697
Mohsan, S. A. H., Mazinani, A., Sadiq, H. B., and Amjad, H. A. (2022). survey of optical wireless technologies: practical considerations, impairments, security issues and future research directions. Optical Quantum Electr. 54, 187. doi: 10.1007/s11082-021-03442-5
Muhd-Yassin, S. Z., Anuar, M. K., Zulkifli, M. I., Harun, S. W., Abdul-Rashid, H. A., Abd-Rahman, M. K., et al. (2012). 56 dB Gain EYDFA with improved noise figure with dual-stage partial double pass configuration. Optik 123, 1884–1887. doi: 10.1016/j.ijleo.2012.03.051
Nadeem, L., Saadullah, Q. M., and Hassam, A. (2018). Performance of FSO links using CSRZ, RZ, and NRZ and effects of atmospheric turbulence. J. Optical Commun. 39, 191–197. doi: 10.1515/joc-2016-0113
Niaz, A., Qamar, F., Ali, M., Farhan, R., and Islam, M. K. (2019). Performance analysis of chaotic FSO communication system under different weather conditions. Trans. Emerg. Telecommun. Technol. 30, e3486. doi: 10.1002/ett.3486
Noor, K., Shahid, H., Obaid, H. M., Rauf, A., Yousaf, A., Shahid, A., et al. (2022). Hybrid underwater intelligent communication system. Wireless Pers. Commun. 125, 2219–2238. doi: 10.1007/s11277-022-09653-7
Obaid, H. M., Noor, K., Malik, M. T., and Rehman, A. (2023). Design and analysis of holmium-doped fiber amplifier for WDM system in 1990–2100 nm wavelength range at higher bit rates. J. Russian Laser Res. 44, 1–10. doi: 10.1007/s10946-023-10181-3
Obaid, H. M., and Shahid, H. (2020). Performance evaluation of hybrid optical amplifiers for a 100 × 10 Gbps DWDM system with ultrasmall channel spacing. Optik 200, 163404–163404. doi: 10.1016/j.ijleo.2019.163404
Parkash, S., Sharma, A., Singh, H., and Singh, H. P. (2016). Performance investigation of 40 GB/s DWDM over free space optical communication system using RZ modulation format. Adv. Optical Technol. 6:302. doi: 10.1155/2016/4217302
Rashidi, F., He, J., and Chen, L. (2017). Spectrum slicing WDM for FSO communication systems under the heavy rain weather. Optics Commun. 387, 296–302. doi: 10.1016/j.optcom.2016.11.070
Sandalidis, H. G., Tsiftsis, T. A., Karagiannidis, G. K., and Uysal, M. (2008). BER performance of FSO links over strong atmospheric turbulence channels with pointing errors. IEEE Commun. Lett. 12, 44–46. doi: 10.1109/LCOMM.2008.071408
Singh, M., Malhotra, J., Atieh, A., El-Khozondar, H. J., and Dhasarathan, V. (2021). Performance investigation of 1.6 Tbps hybrid WDM-PDM-OFDM-based free space optics transmission link. Wireless Pers. Commun. 117, 2285–2309. doi: 10.1007/s11277-020-07972-1
Keywords: free space optics (FSO), hybrid optical amplifier, gain, DWDM, Q-factor, BER
Citation: Obaid HM, Ashraf S, Asgher Nadeem M, Shahid H, Akram A and Zafrullah M (2024) Performance analysis of a hybrid optical amplifier based 480-Gbps DWDM-FSO system under the effect of different atmospheric conditions. Front. Comput. Sci. 6:1348024. doi: 10.3389/fcomp.2024.1348024
Received: 01 December 2023; Accepted: 24 January 2024;
Published: 07 February 2024.
Edited by:
Shancheng Zhao, Jinan University, ChinaReviewed by:
Yousaf Khan, University of Engineering and Technology, Peshawar, PakistanSantosh Kumar, Liaocheng University, China
Copyright © 2024 Obaid, Ashraf, Asgher Nadeem, Shahid, Akram and Zafrullah. This is an open-access article distributed under the terms of the Creative Commons Attribution License (CC BY). The use, distribution or reproduction in other forums is permitted, provided the original author(s) and the copyright owner(s) are credited and that the original publication in this journal is cited, in accordance with accepted academic practice. No use, distribution or reproduction is permitted which does not comply with these terms.
*Correspondence: Shahzad Ashraf, nfc.iet@hotmail.com