Identifying umbrella and indicator species to support multispecies population connectivity in a Himalayan biodiversity hotspot
- 1Fauna & Flora, The David Attenborough Building, Cambridge, United Kingdom
- 2Wildlife Conservation Research Unit, Department of Biology, University of Oxford, Recanati-Kaplan Centre, Oxfordshire, United Kingdom
- 3Department of Biological Sciences, Northern Arizona University (NAU), Flagstaff, AZ, United States
Successful conservation hinges on the reliable prediction and prioritisation of population core habitats and dispersal corridors. However, reliance on single-species connectivity has produced mixed results and, in most cases, failed to protect other species under the appealing but often unfulfilled guise of umbrella protection. The main objectives of this study were: (1) to identify core habitats and dispersal corridors for population connectivity of 15 terrestrial mammal species across Bhutan, (2) to identify indicator species of wildlife connectivity, (3) to prioritise core areas and dispersal corridors for the focal umbrella and indicator species, (4) to develop composite multispecies connectivity maps and evaluate the effectiveness of the existing protected area network. We used kernel density (KD) to identify core habitat areas and factorial least-cost path (LCP) to map dispersal corridors. We used correlation and cluster analyses to identify umbrella and indicator species, and quantitative ranking to prioritise core areas and corridors for these focal species. Finally, we produced maps of multispecies core areas and corridors, and identified gaps in the existing protected area network (PAN). We found that ungulates were generally more effective than carnivores in serving as umbrella species for core habitat protection, with Asian elephant (Elephas maximus) emerging as the most effective umbrella for core habitats and muntjac (Muntiacus muntjac) for dispersal corridors. We identified six focal connectivity indicator species in Bhutan: tiger (Panthera tigris), dhole (Cuon alpinus), clouded leopard (Neofelis nebulosa), Asiatic black bear (Ursus thibetanus), Asian elephant, and sambar deer (Rusa unicolor). Most indicator species’ core areas and dispersal corridors outside the PAN were in southern Bhutan, indicating opportunities for increased multispecies connectivity through expanding protected area network in this region. A total of 40% of multispecies core areas and 24% of dispersal corridors were under PAN, with large parts of Bhutan offering suitable core areas and corridors for multispecies conservation. Core areas and corridors of the 15 considered species only partially overlapped, stressing the importance of multi-species consideration in designating wildlife corridors.
1 Introduction
The long-term viability and sustainability of animal populations depends on the protection of critical habitats and a network of dispersal corridors that facilitates movement, gene flow and resource utilisation among them (Cushman, 2006; Cushman et al., 2006; Cushman et al., 2013c). However, growing human pressure on natural resources coupled with climate change is shrinking natural habitats throughout the world (Newbold et al., 2015; Barlow et al., 2016). Habitat suitability and functional connectivity for many species decline with human modification leading to habitat loss and fragmentation, which are the largest threats to biodiversity (Noss, 1991; Fahrig, 2003). To counter this, identifying and protecting core population habitats and linking them through stepping stones and dispersal corridors is of paramount importance (Cushman et al., 2013d; Cushman, 2006, Cushman et al., 2014). A well-connected landscape with large core habitats supporting populations will likely facilitate demographic dynamics which enable populations to fluctuate and allow local extinctions to be recolonised without risking extinction in the regional metapopulation (Taylor et al., 1993). Furthermore, large patches of habitats connected via corridors are vital to tracking climate shifts (Carroll et al., 2010; Wasserman et al., 2012) and allowing for range expansion (Chen et al., 2011).
Most corridor planning has been based on single species, which may or may not provide resource needs and protection to other species (Cushman and Landguth, 2012; Cushman et al., 2013b). Single focal species are sometimes habitat generalists and thus cannot provide adequate umbrella protection (Simberloff, 1998; Cushman et al., 2012). For example, Cushman et al. (2013b) evaluated the ability of each of three focal species to serve as connectivity umbrellas for the others across the American Great Plains and found none of them was a good surrogate for the others. Furthermore, using a large simulation experiment, Cushman et al. (2012) evaluated the abilities of 144 species to serve as connectivity umbrellas for the others across a range of ecological niche characteristics and dispersal abilities. They found that the most commonly used focal species, such as large carnivores, were poor indicators of overall connectivity for biodiversity generally, and that in particular the parts of the landscape most vulnerable to habitat loss and fragmentation were particularly poorly matched to connectivity for those species. Umbrella species are defined as species whose protection indirectly encompasses protection of many other species within an ecological community whereas, indicator species are defined as species that serve as a measure of the environmental conditions (habitat) in which it exists.
As a result of the recognised shortcomings of single-species approaches to connectivity conservation, the multi-species approach to identifying core population habitats and corridor planning is gaining popularity (Cushman et al., 2012, Cushman et al., 2013b, Brodie et al., 2015; Brennan et al., 2020). This is because protecting a patch of habitat or corridor utilised by more than one species entails less cost and encompasses wider protection for a range of wildlife species. Several studies have shown that the conservation effectiveness of connectivity designs is greater when the conservation of core habitats and corridors is strategised to meet the simultaneous needs of multiple species (Cushman and Landguth, 2012; Cushman et al., 2013b, Hand et al., 2014; Khosravi et al., 2018; Ashrafzadeh et al., 2020; Haidir et al., 2021; Almasieh & Cheraghi, 2022; Feizabadi et al., 2023), often resulting in better overall biodiversity protection and landscape connectivity (Cushman et al., 2013b; Brodie et al., 2015).
Several approaches exist to identify core habitats and corridors, such as cumulative resistant kernels (Compton et al., 2007; Cushman et al., 2014), least-cost path (Adriaensen et al., 2003), factorial least-cost path (Cushman et al., 2009; Cushman et al., 2013b), and current flow models (Circuitscape; McRae et al., 2008). These models utilise resistance surfaces developed from GPS telemetry, expert opinion, genetic data, or habitat suitability layers (Zeller et al., 2012). The outputs of connectivity models predict and visualise core areas of animal occupation and/or connectivity linkages and delineate barriers that may fragment populations. Spatial prediction and prioritisation of core areas, corridors and barriers produced from synoptic connectivity modelling facilitate conservation planners to identify the drivers of habitat loss and fragmentation and develop effective strategies (Cushman et al., 2016; Cushman et al., 2018; Kaszta et al., 2020; Zeller et al., 2020).
The predictions of spatially synoptic patterns of connectivity based on resistant kernels (KD) identify all-directional dispersal (Compton et al., 2007; Cushman et al., 2010) as opposed to pair-wise corridors between predefined sources (Beier et al., 2008). This offers greater flexibility to predict dispersal across the landscape and provides a complete picture of connectivity across space allowing identification of core population habitats (Cushman and Landguth, 2012; Cushman et al., 2014; Kaszta et al., 2020). A factorial implementation of least-cost paths (LCP) allows integration of a vast number of least-cost paths to show synoptic connectivity across large and complex landscapes (Cushman et al., 2011; Landguth et al., 2012). This helps in identifying which species have the most fragmented populations and maps the most important corridor linkages among population core areas where conservation efforts can be focused (Cushman et al., 2011; Cushman et al., 2018). Recent research has shown that factorial least cost path (Cushman et al., 2014) and resistant kernel (Cushman et al., 2014; Unnithan Kumar and Cushman, 2022; Lumia et al., 2023) methods of synoptic connectivity modelling are particularly powerful in predicting the actual patterns of animal movement through complex landscapes. Together, KD and LCP enable us to predict habitat core areas, identify fracture zones and barriers, and locate keystone linkages that provide connectivity among core areas (Cushman and Lewis, 2010; Cushman et al., 2011; Cushman and Landguth, 2012).
We assessed the landscape connectivity and corridor efficacy of fifteen terrestrial mammal species in Bhutan. Bhutan lies at the intersection of two biogeographic realms: the Indo-Malayan and the Palearctic supporting rich biodiversity (Olson et al., 2001). Yet the fragility of the landscape makes species in this region particularly susceptible to environmental change (Penjor et al., 2020). Further, developing countries in Asia have some of the highest rates of forest conversion and degradation globally (Sodhi et al., 2004; Miettinen et al., 2011). Although Bhutan has a well-developed network of protected area systems, the structural and functional connectivity of the existing protected areas (PA) and biological corridor (BC) network (together PAN) have not been assessed yet. Rapid infrastructure development and climate change threaten to fragment wildlife habitats and drive shifts in species ranges. Thus, assessment of the efficacy of protected areas and corridors is not only important for species population connectivity but also to provide crucial information to conservation managers to develop adaptive management plans in the face of growing human impacts and climatic change.
We used a spatially synoptic approach (e.g., sensu Cushman et al., 2014) and two complementary methods to assess landscape connectivity for 16 terrestrial mammals in Bhutan. First, to define and quantify core areas of high-density movement for each species we applied cumulative resistant kernels (Compton et al., 2007). Second, to map and quantify dispersal corridors (narrow linkages) between the core area we used the factorial least-cost paths analysis (Cushman et al., 2013b). Using multivariate cluster analysis, we then identified connectivity indicator and umbrella species across carnivores and herbivores based on how well their predicted connectivity intersected with the connectivity of the other species. We chose representative members of each indicator cluster as focal species. Finally, we used these focal species to rank and prioritise core areas and corridor importance.
2 Materials and methods
2.1 Study area
This camera trap survey was conducted in Bhutan as a part of the nation-wide tiger survey in 2014–15. For logistical reasons, the country was divided into two blocks: north (n = 681 camera stations) and south (n = 448). Bhutan, with an area of 38,394 km2, is a small country in the eastern Himalayas between India and China. About 70% of the total geographical area of Bhutan is covered by forest. Vegetation varies with elevation; lower foothills are characterised by broadleaved forest, the temperate zone by conifer-broadleaved mixed forest and higher elevations by alpine and sub-alpine scrub and shrub. The topography is rugged, with steep terrain, narrow river valleys and deep gorges. The altitude ranges from 100 m in the south to above 7500 m in the north. The wet season (monsoon) occurs between July and September, with annual precipitation ranging between 300 and 6000 mm.
2.2 Study species
We used data for 16 mammal species, each captured in more than 10% of the total camera stations. Of the 16 species, five are listed as Least Concern (LC), five as Vulnerable (VU), three as Near Threatened (NT) and three as Endangered (EN) in the International Union for Conservation of Nature (IUCN) Red List (Supplementary Table S2). The study species are Asiatic black bear (Ursus thibetanus), Asiatic golden cat (Catopuma timminckii), clouded leopard (Neofelis nebulosa), dhole (Cuon alpinus), Asian elephant (Elephas maximas), gaur (Bos gaurus), masked palm civet (Paguma larvata), common leopard (Panthera pardus), leopard cat (Prionailurus bengalensis), marbled cat (Pardofelis marmorata), muntjac (Muntiacus muntjak), sambar deer (Rusa unicolor), serow (Capricornis thar), tiger (Panthera tigris), wild pig (Sus scrofa), and yellow-throated marten (Martes flavigula).
2.3 Resistance surfaces
We built resistance surfaces based on the multi-scale habitat suitability models developed in Penjor et al. (2021). The details of the multi-scale habitat suitability modelling approach are presented elsewhere (e.g., Macdonald et al., 2018; Macdonald et al., 2019). Briefly, the analysis used a presence/absence design based on an extensive nationwide camera trapping dataset coupled with multi-scale optimisation using logistic regression to predict habitat suitability across the full extent of Bhutan for each species.
Ideally, resistance surfaces should be developed using species movement or landscape genetic data as such data directly provide information on species movement in relation to landscape features (Cushman, 2006; Cushman et al., 2013b; Mateo-Sánchez et al., 2015a; Zeller et al., 2016; Zeller et al., 2018). However, given that movement and genetic data are often lacking for many species in many parts of the world, habitat suitability layers are often used as surrogates (Mateo-Sánchez et al., 2015b; Keeley et al., 2016). Studies show that habitat suitability often is an inferior predictor of landscape resistance in comparison to models built with genetic or movement data (Wasserman et al., 2010; Mateo-Sánchez et al., 2015a; Mateo-Sánchez et al., 2015b). However, when such data are not available, habitat suitability may be the best proxy for estimating landscape resistance (Mateo-Sánchez et al., 2015a; Mateo-Sánchez et al., 2015b).
Several studies have suggested that the relationship between habitat suitability and resistance is non-linear (Mateo-Sánchez et al., 2015a; Mateo-Sánchez et al., 2015b; Keeley et al., 2016). To transform the habitat suitability layer to the resistance surface, we used a negative exponential function (Mateo-Sánchez et al., 2015b, Keeley et al., 2016; Kaszta et al., 2020; Haidir et al., 2021). We transformed habitat suitability to resistance using the formula,
Where R is a resistance layer, H is the habitat suitability layer, C is the factor to determine the negative exponential relationship, and Y is the value used to convert minimum resistance to 1 (SupplementaryFigure S1).
The final resistance surface had continuous values ranging from 1 (indicating low resistance to movement) to 100 (high resistance) (Supplementary Figure S2). Since the habitat suitability models did not include features impermeable to species movements such as highways, large river networks and high-density settlements, we burned them into the resistance surface with the resistance value of nearest neighbours. We also masked out elevation above 4500m beyond which the camera traps were not established in this survey.
2.4 Source point locations
Accurate prediction of synoptic population connectivity requires animal source locations that reflect the distribution and density of the species (Cushman et al., 2013b, Cushman et al., 2018). We probabilistically distributed these source points across the habitat suitability layer such that source points were selected with a probability proportional to predicted habitat suitability (Kaszta et al., 2020). To do this, we first created a random raster with values distributed uniformly between 0 and 1. Second, we subtracted this random raster from the habitat suitability raster and reclassed pixels with positive values to reflect potential species occurrences. Finally, we randomly selected 2000 occurrence points from these potential source points that served as source locations for analysis to provide a consistent number between analysed species, which is necessary for accurate relative comparisons between species.
2.5 Connectivity modeling
To identify core areas, we used the cumulative resistance kernel method (Compton et al., 2007) and to identify linkages between them as dispersal corridors we applied factorial least-cost paths analysis (LCP) (Cushman et al., 2009). The cumulative resistance kernels predict the spatially explicit frequency of movement and the extent of the area that facilitates movement across the landscape from a source location in suitable habitat (Compton et al., 2007; Cushman et al., 2013b). Factorial least-cost paths, on the other hand, identify paths between the points with the lowest resistance and movement cost (Cushman et al., 2013b). These two methods are complementary. LCP analysis identifies narrow movement linkages between the designated source location pairs, which is useful in the identification of dispersal corridors (areas too narrow to be inhabited by a species but can be potentially used for species dispersal) (Cushman and Landguth, 2012; Cushman et al., 2013b, Kaszta et al., 2020). Resistant kernels quantify movement across the entire landscape from designated source locations, yielding in a continuous surface quantifying the movement incidence function for every location in the landscape (not just between the source locations) (Cushman and Landguth, 2012; Cushman et al., 2013b). Therefore, only the resistant kernel analyses can be used to identify and quantify core population areas (Kaszta et al., 2020).
The resistant kernel and LCP approaches can incorporate biologically relevant information on species dispersal distance to accurately predict connectivity patterns (Cushman et al., 2013b; Cushman et al., 2013b). Due to the lack of movement data for the study species, we estimated dispersal distances using published relationships between species home range size and dispersal distances (Bowman et al., 2002). We gathered home range data through an extensive literature review for all study species across the distribution range (Supplementary Table S1). For kernels, we used a direct movement threshold based on average home range size (Kaszta et al., 2020). For LCPs, we applied a threshold 4x higher than the dispersal threshold for kernels to reflect long-distance dispersal outside the established home range (Cushman et al., 2018; Kaszta et al., 2020). The resistance kernel and factorial least-cost path modelling were performed using UNICOR software (Landguth et al., 2012).
2.6 Indicator and umbrella species
We used hierarchical cluster analysis and mean pixel-wise correlation of resistant kernel and LCP to identify indicator and umbrella species. Using the predicted resistant kernel and LCP surfaces, we applied cluster analysis to identify and group species with similar spatial patterns of core areas and corridor networks. This identifies indicator species based on the similarity in predicted core areas and dispersal corridors. The pair-wise pixel correlation between predicted kernel/LCP surfaces resulted in the identification of species with the highest average correlation with all other species that offers umbrella protection (Cushman et al., 2010). The umbrella species quantifies how well the optimal protected core area or dispersal corridor for one species would encompass the core habitat and corridor for the other species, while connectivity indicator species are those which are most similar in connectivity to other members of their cluster groups (e.g., Cushman et al., 2010).
2.7 Core areas and corridors prioritization
We identified potential core habitats based on cumulative resistant kernel surfaces and identified dispersal corridors based on LCPs using the general approach first applied in Cushman et al. (2018) as improved by Kaszta et al. (2020). All the values of cumulative resistant KD surface equal to and greater than 80th percentile were considered as core area and this threshold likely indicates moderate to high predicted movement rate of species across the landscape. To identify dispersal corridors, eliminating at the same time weak and disconnected linkages, for the LCPs we defined a threshold at the 25th percentile. We then, for the indicator species identified by the cluster analysis, ranked the core areas and corridors by their importance. Core area ranking was based on the size and strength of the predicted kernel values whereas, corridor ranking was based on the sum of LCP and average sum of kernel values of core areas they link (Cushman et al., 2018; Kaszta et al., 2020). To produce composite core area and corridors maps across all 16 species we summed the binary core and corridor rasters. For this, we used kernel and LCP surfaces assigning values one to all pixels above the threshold and zero to pixels below the threshold. Core area and corridor prioritisation was performed for each focal species and for all species combined (multi-species composite).
2.8 Multispecies core habitats and corridors
We defined core area by selecting cumulative resistant KD values above the 80th percentile threshold. To identify dispersal corridors, we defined a threshold of 25th percentile for LCP values. To generate alternative multi-species landscape connectivity layers, we summed and multiplied the thresholded cumulative resistant kernel surface and LCP surfaces of all 16 species, respectively. Summation indicates areas of high-density movement across 16 species, whereas multiplication identifies areas important for composite multispecies predictions connectivity for all the 16 species together. We did this according to two assumptions: (1) by treating all species as equal from a conservation point of view; (2) by ranking species (assigning them weights) based on their national and global conservation importance (Supplementary Table S2). For example, tiger was assigned the highest value due to its national and global significance while muntjac was assigned the lowest value. Finally, we evaluated the overlap of our multi-species core habitats and corridor surfaces with the current PAN to identify gaps in the current system and propose new effective core dispersal and corridor areas for future protection. Finally, we ranked the PAN and NPA based on the sum of multispecies kernel values and LCPs predicted within them.
3 Results
3.1 Umbrella species
Overall, we found a much higher average correlation between species core habitats than between their dispersal corridors (Figure 1). The correlation analyses identified Asian elephant as the most effective umbrella species for core habitat protection closely followed by leopard cat (Figure 1A). Ungulate species were more effective in providing umbrellas for multiple species core habitats compared with carnivores. However, Asiatic black bear was a better connectivity umbrella than smaller carnivores such as Mustelids. Dhole, on the other hand, was the least effective umbrella species for the protection of core areas. In terms of umbrellas for dispersal corridors (based on LCPs), muntjac was the most effective umbrella species (Figure 1B). Mesocarnivores (masked palm civet, yellow-throated marten) were the next most effective umbrella species. Amongst felids, leopard cat and marbled cat were better as umbrella species than tiger or leopard.
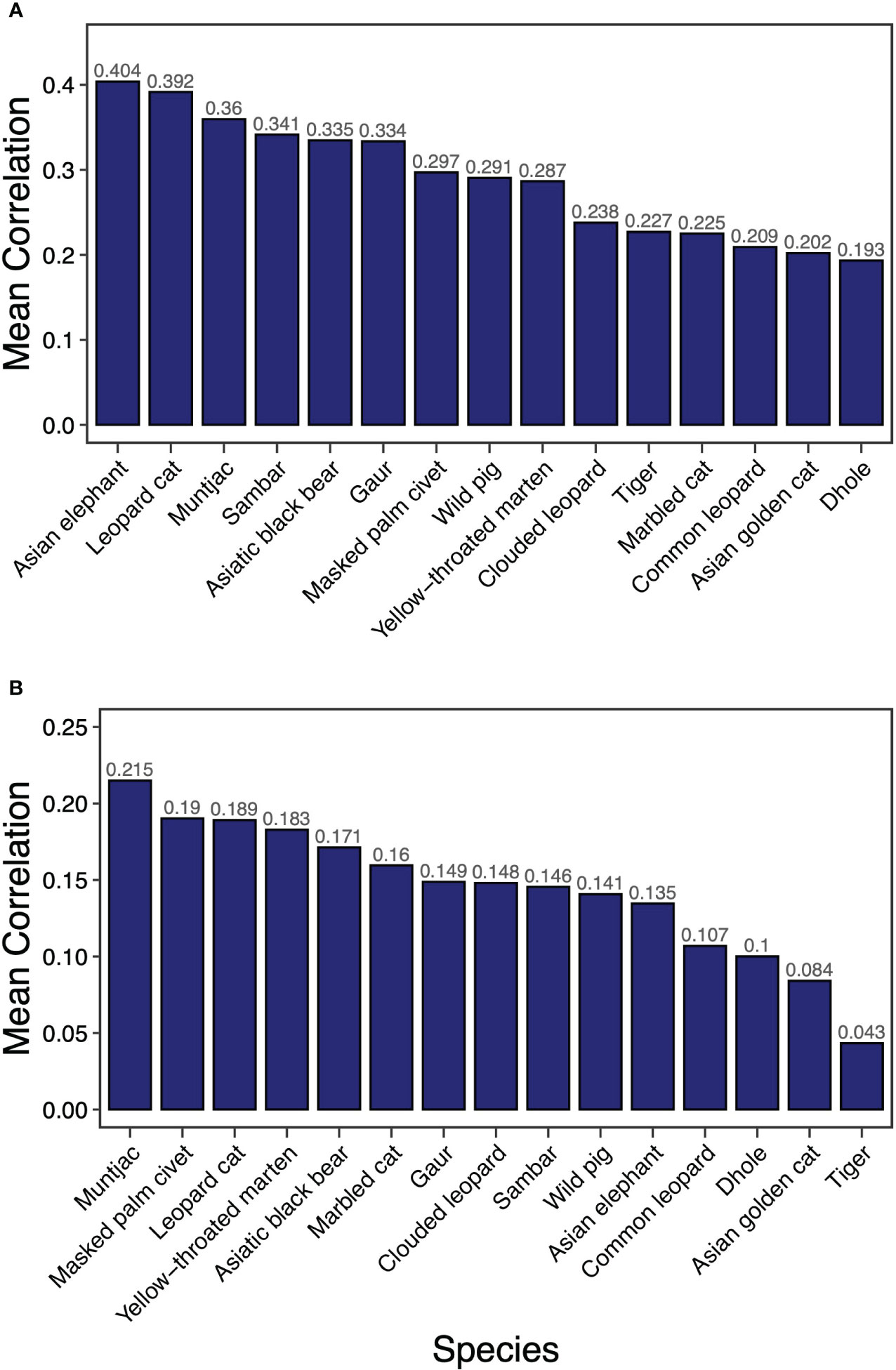
Figure 1 Mean correlation of resistant KD (A) and factorial LCP (B) of 15 terrestrial mammals across PAN and NPA in Bhutan.
3.2 Indicator species
Based on the clustering of resistant KD values across 16 studied species, we identified five distinct clusters (Figure 2). The first cluster consists of four species: Asiatic black bear, yellow-throated marten, clouded leopard and marbled cat with possible substructures of Asiatic black bear/yellow-throated marten and clouded leopard/marbled cat. We propose two indicator species for core habitat indicators in this group (Asiatic black bear and clouded leopard) due to their conservation importance at a local and global level and indicative of functional trait diversity (canid, mustelid and felid diversity). The second cluster consists of muntjac, masked palm civet and wild boar. We propose wild boar as a connectivity indicator of this group due to their importance as prime prey for large carnivores and as an indicator of large carnivore abundance. Dhole and Asian golden cat formed the third cluster. The fourth cluster consisted of a mix of felids and large ungulates (i.e., leopard cat, common leopard, sambar deer, Asian elephant, and gaur). We propose two representative species as indicators for core habitat protection in this group: Asian elephant, representing habitat specialists, and sambar deer, representing important prey for carnivores. The final cluster had a singleton species, tiger.
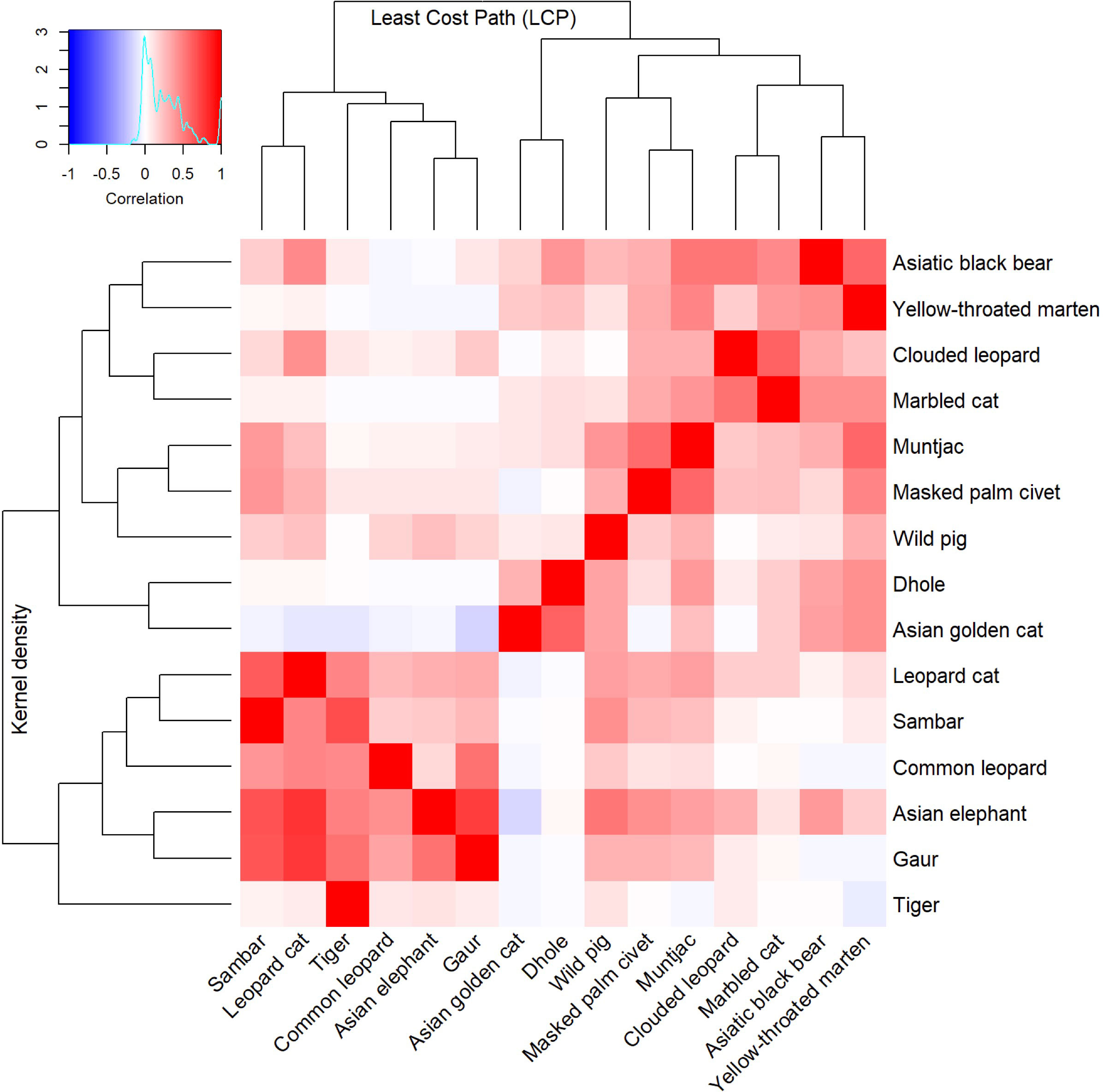
Figure 2 Bivariate hierarchical cluster dendrogram of the resistant KD and factorial LCP of 15 terrestrial mammals used to identify focal species for core area and corridor conservation in Bhutan.
The LCP cluster analysis identified four main clusters which were relatively consistent with the KD clusters (Figure 2). The first cluster consists of sambar deer, tiger, common leopard, Asian elephant, gaur and leopard cat. Hence, we assign two focal species (tiger and sambar deer). The second cluster contained Asian golden cat and dhole and, given the importance of dhole as pack-living animals that require large area and connectivity, we assign this species as an indicator for landscape-scale connectivity. The third cluster contained wild boar, masked palm civet and muntjac. Although muntjac was the most effective umbrella species in terms of connectivity, due to small home range and small dispersal distance, we instead choose wild boar as an indicator. The final cluster was composed of clouded leopard, marbled cat, Asiatic black bear and yellow-throated marten. This cluster is formed of two subclusters that are slightly different from each other: clouded leopard/marbled cat and Asiatic black bear/yellow-throated marten. One each of these groups was considered for indicators, with clouded leopard and Asiatic black bear due to their charisma (Macdonald et al., 2018), public interest and extensive research that enables rigorous assessment and monitoring. We selected six species as focal indicators for core area and corridor prioritisation (viz Asiatic black bear, clouded leopard, dhole, wild boar, Asian elephant and sambar deer).
3.3 Core areas ranking for indicator species
Based on core areas prioritisation for each the six focal indicator species, dhole had the highest number (n=25) of core areas across Bhutan (Figure 3). About 41% (7433km2) of the core area for dhole were protected in Bhutan and the neighbouring Indian states of Arunachal Pradesh, Assam, Sikkim, and West Bengal of which 6782km2 are in Bhutan (Figures 4G, H). Sixteen core areas were identified for clouded leopard. About 60% (10344km2) of the total core area were inside Bhutan and 31% (5351km2) were protected by existing PAN (Figures 4E, F). Asiatic black bear had six distinct core areas (total area 18019km2) and ~ 39% (7015km2) of black bear predicted core areas were under protection in both Bhutan and India (Figures 4C, D). Tiger had four distinct cores with the highest-ranking core habitat in the southern parts of Bhutan extending into Indian states of West Bengal and Assam (Figures 4K, L). Approximately 46% (8290km2) of the overall predicted core habitats for tiger were inside Bhutan. Two large cores were identified for sambar deer with a total area of ~18028km2 (Figures 4I, J). Fifty per cent of the sambar core lie inside Bhutan and ~15% were under existing protection and the most important one in the south. In Bhutan and India, ~26% were under the PAN. Asian elephant had a single large core area contiguous across southern Bhutan and three Indian states of West Bengal, Assam, and Arunachal Pradesh (Figures 4A, B). About ~40% (7123km2) of the core habitats were inside Bhutan, of which ~38% (2696km2) were inside the existing PAN.
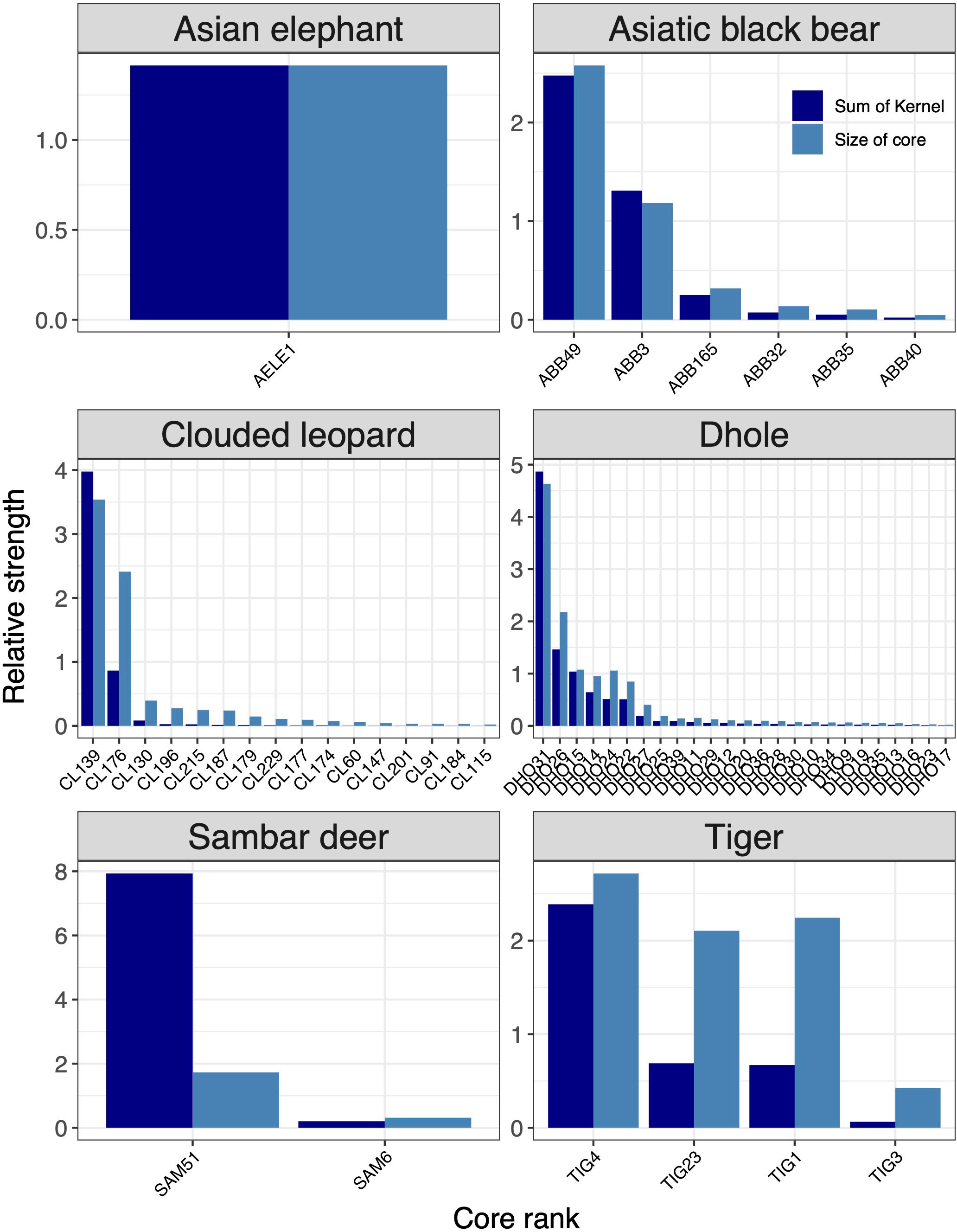
Figure 3 Rank of core areas for six focal species (Asian elephant, Asiatic black bear, clouded leopard, dhole, sambar deer, tiger). Core area ranking was based on the size and strength of the predicted kernel values. The x-axes show core areas of each species in the ranking order from most important to least important.
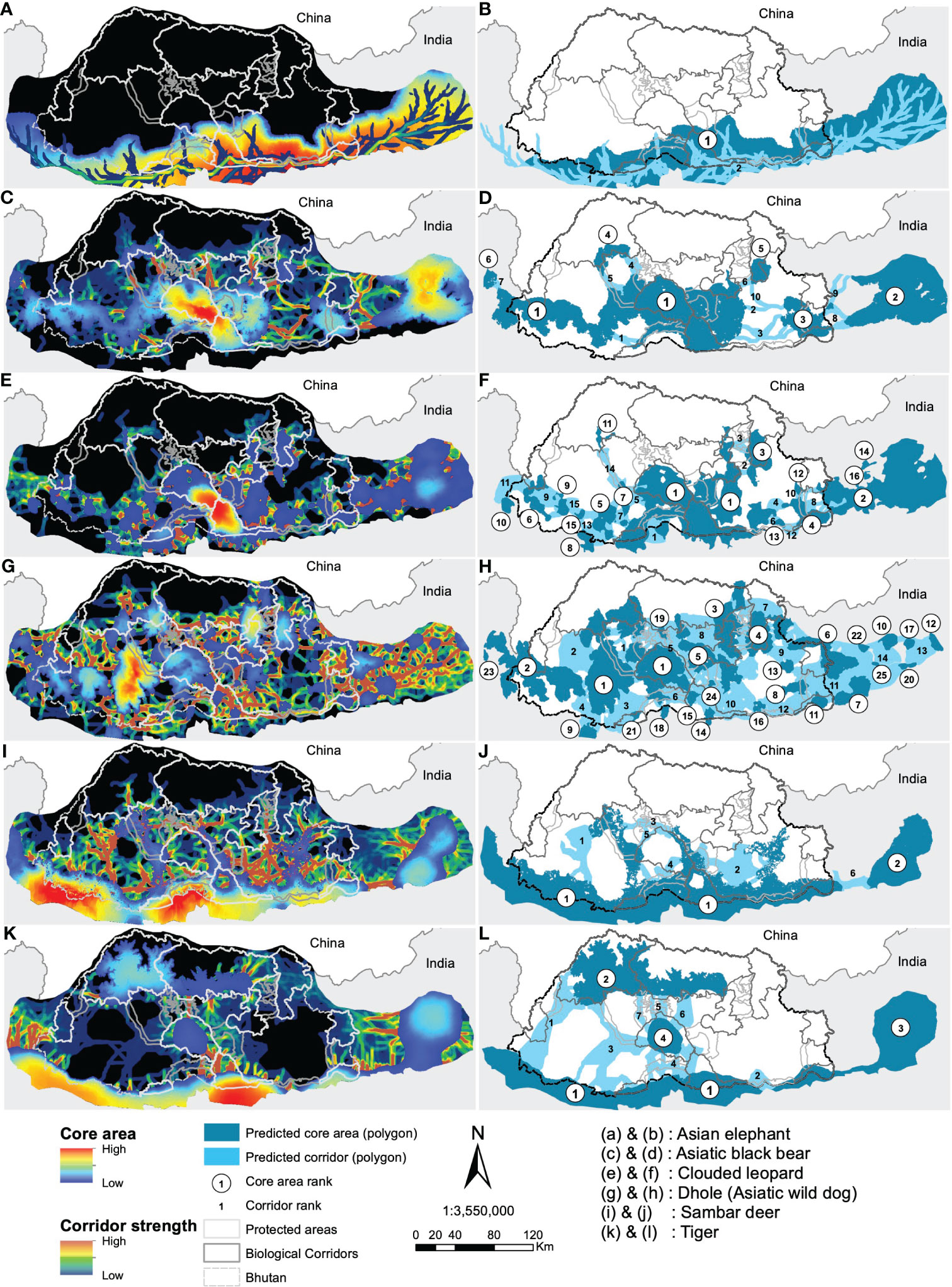
Figure 4 Predicted resistant KD and LCP for the focal species (left panel) with their ranking of importance (right panel). Study species are labelled (A–L).
3.4 Corridors ranking for indicator species
Of 15 corridors identified for clouded leopard, ~79% were inside Bhutan and, together with India, ~15% were under protection (Figures 5, 4E, F). Dhole had 75% of its corridors in Bhutan, with ~24% inside the existing PAN, while ~30% (4634km2) were jointly protected in Bhutan and India (Figures 4G, H). Approximately 73% of the Asiatic black bear corridor area lie in Bhutan (Figures 4C, D). Bhutan alone protected only ~9% of black bear corridors, while Bhutan and India together protected ~15%. There were seven corridors for tiger in Bhutan and neighbouring Indian states (Figures 4K, L). Bhutan included 98% of them. The combined protection in Bhutan and India encompassed ~34% of tiger corridor, and Bhutan alone protected 34%. We identified six corridors for sambar deer across Bhutan and in four neighbouring states of India (Figures 4I, J). Bhutan contained ~91% of the total value of sambar corridors, but only 17% were protected. Asian elephant had two important corridors entirely inside the predicted core (Figures 4A, B). Twenty-six per cent (2024km2) of the predicted dispersal corridors were inside Bhutan and more than 52% (1062km2) were protected.
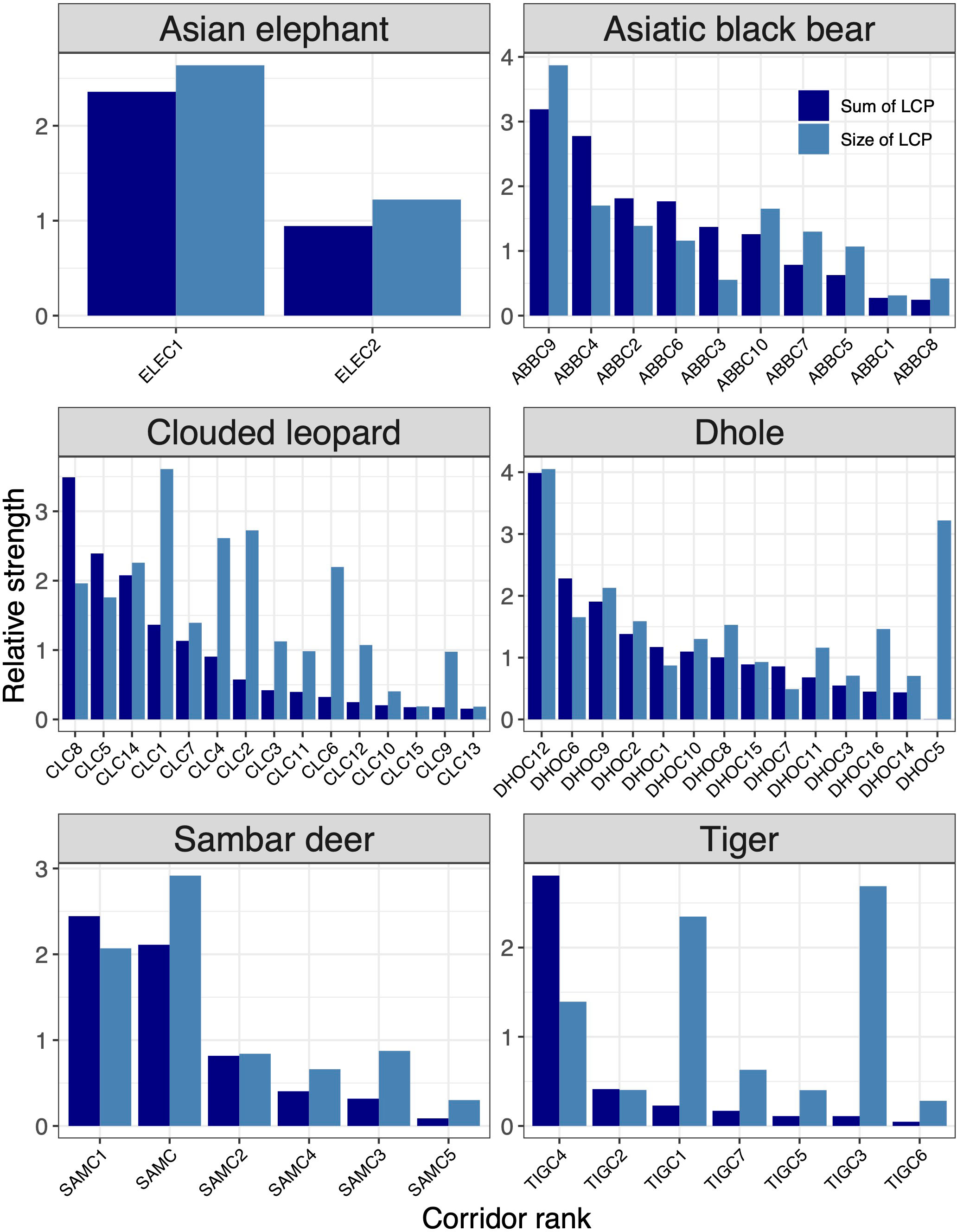
Figure 5 Rank of corridors for six focal species (Asian elephant, Asiatic black bear, clouded leopard, dhole, sambar deer, tiger). The corridor ranking was based on the sum of LCP and the average sum of KD values of core areas they link. The x-axes show core areas of each species in the ranking order from most important to least important.
3.5 Multispecies core habitats and corridors
The overall area represented by multi-species core habitats across the study area was ~48,790km2. The study area includes Bhutan and the four adjoining Indian states (Figure 6A). Effectively, ~38% (~18,554km2) of the core area was under some form of protection. Approximately 67% (~32,743km2) of the total core area lies inside Bhutan, of which ~40% (12,954km2) was inside PAs and another ~8% (~2,458km2) inside BCs. Similarly, the total area of the predicted dispersal corridor under protection in Bhutan and the neighbouring Indian states was 13,362km2 (31%) out of 42,460km2 predicted. Bhutan alone had about 24% (10,268km2) of the predicted corridors under protection. About 38% of the core habitats and 31% of dispersal corridors across all study species are protected across Bhutan and in the neighbouring states of India.
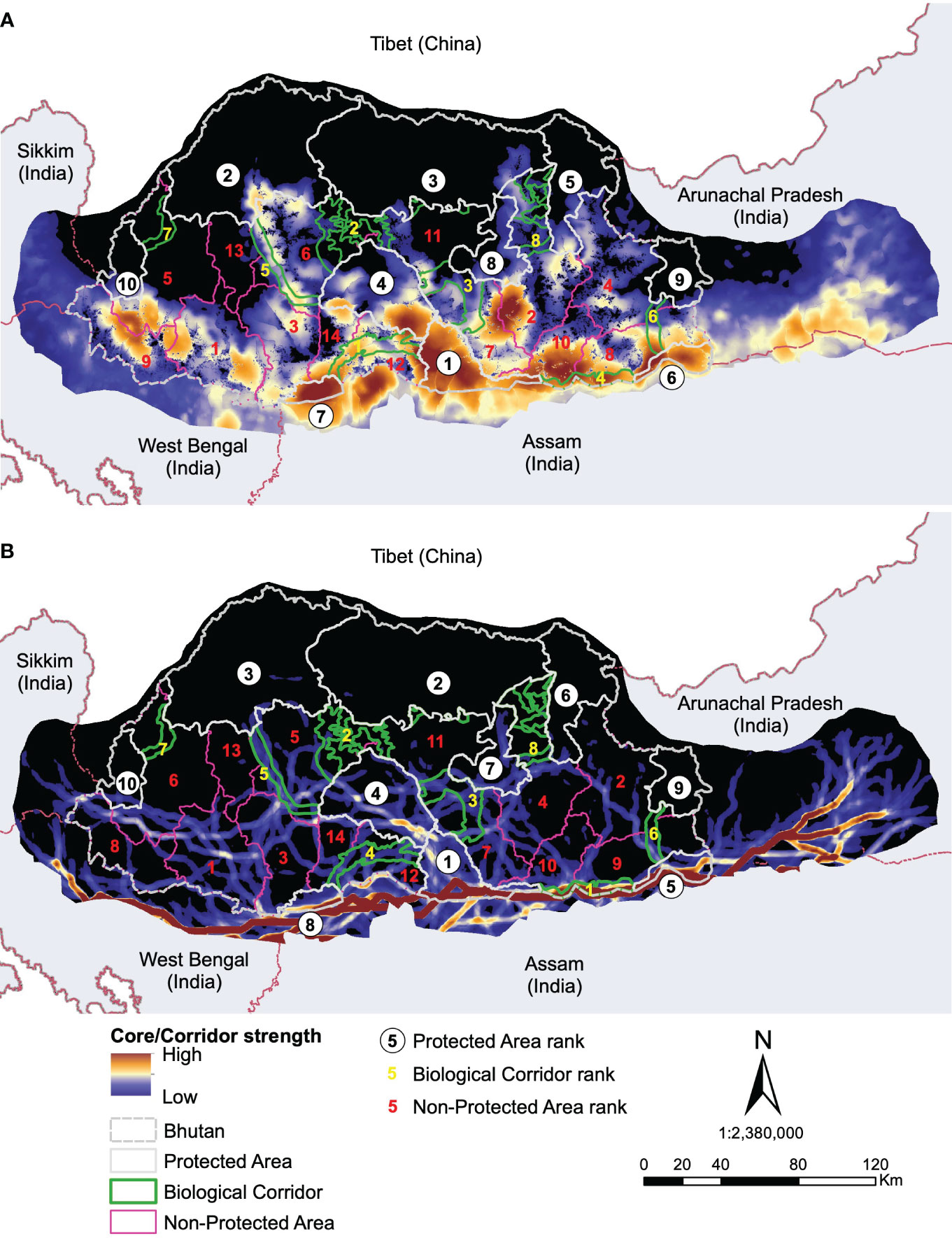
Figure 6 Predicted resistant KD (A) and LCPs (B) for all species combined with their ranking of importance.
4 Discussion
In this study, we present the first comprehensive and empirically based evaluation of population connectivity for multiple species across Bhutan. Our analysis focused on identifying core habitats and dispersal corridors for 15 terrestrial mammals. Based on overlap and similarity between landscape connectivity surfaces, we identified umbrella and indicator species. We assessed the proportion of predicted core areas and corridors under the existing PAN for these species and multispecies core areas and corridors. Asian elephant and muntjac were identified as the best umbrella species for core area and dispersal corridor protection, respectively. Asian elephant, Asiatic black bear, clouded leopard, dhole, sambar deer and tiger were identified as effective focal indicator species for core areas and corridor protection.
Our study finds that overall, 40% of multispecies core areas in Bhutan lie within the current PAN. Core areas for most species overlap, but only partially, and the overlap of core areas of all 15 species together constitute a very small proportion of Bhutan (12,954km2), indicating spatial differences in core area use by mammal species across Bhutan. Likewise, ~24% of the multispecies predicted dispersal corridors were under the existing Bhutanese PAN. Most species tend to utilise unique dispersal corridors suited to their needs suggesting the importance of multi-species consideration in designating a wildlife corridor.
4.1 Umbrella and indicator species for core habitat and corridor protection
Our findings show that Asian elephant was the most effective umbrella species for core habitat protection followed by leopard cat, muntjac and sambar deer. Muntjac was the most effective umbrella species for corridor protection. Mesocarnivores (masked palm civet, leopard cat, and yellow-throated marten) were as effective as muntjac for core habitat protection, though less so for corridor protection. The umbrella species identified here best capture core habitat and connectivity for all the other species we considered. Asian elephants live in groups and have large home ranges offering umbrella protection to the biodiversity of the entire landscape they inhabit (Sukumar, 1989; Baskaran et al., 2013). Large-sized species tend to have large home ranges, hence, for them to maintain viable populations requires protection of large tracts of habitat (Roberge and Angelstam, 2004). Therefore, irrespective of the feeding guild, large ungulate mammals are also regarded as prospective umbrella species (De Vires, 1995; Berger, 1997b). Muntjacs are commonly found across Bhutan and inhabit a wide range of habitats. Recent studies in southeast Asia found that muntjac was the primary prey of Asian golden cat and dhole (Kamler et al., 2020a; Kamler et al., 2020b) suggesting that conservation of muntjac would ensure continued supply of food for many carnivores at a higher trophic level. Common species serve as better umbrella species because of their higher probability of long-term persistence (Berger, 1997a). Although, large, threatened, and rare species are important priorities for conservation, often their low reproductive rate, low density and large area requirement make them prone to extinction and ineffective as umbrellas in the long-term (Fleishman et al., 2000). A meta-analysis of umbrella species found that richness of co-occurring species was higher in areas without carnivorous species as umbrellas, thus suggesting that species at lower trophic levels can serve as an effective umbrella (Branton and Richardson, 2011). Traditionally, species that are at risk of extinction are recognised as flagship or umbrella but species that are not threatened may also be suitable for these roles. Species that are common but less frequently involved in human-wildlife conflict may also serve as effective ambassadors for conservation (Bowen-Jones and Entwistle, 2002; Macdonald et al., 2017).
The use of large ungulates (e.g., buffalo (Syncerus caffer), black rhinoceros (Diceros bicornis), wildebeest (Connochaetes spp.) as umbrella species is not a new practice and has been found to be effective in African protected reserves (Caro and O’Doherty, 1999; Caro, 2001; Caro, 2003). However, solely relying on a single umbrella species has generally proven inadequate (Roberge and Angelstam, 2004; Seddon and Leech, 2008; Kramer et al., 2019). This is because, if the umbrella population is endangered or under high pressure from poaching and habitat loss, their numbers might dwindle rendering them ineffective. Hence, designating several umbrella species is advocated (Lambeck, 1997; Fleishman et al., 2001) provided they have a viable population with a low probability of extinction (Caro, 2003).
The proposal, prompted by the results of this study, to use ungulates and mesocarnivores as umbrella species contrasts with the use of traditionally charismatic species such as tigers, clouded leopards, and leopards (Wikramanayake et al., 2004; Macdonald et al., 2012; Kittle et al., 2018). Tigers and leopard occur in low density and are often sensitive to habitat disturbance. Further, people hold mixed views about these large carnivores, and often express fear and aversion to them due to conflict and predation of livestock (Sangay and Vernes, 2008). This suggests that, while large cats elicit international funding and attention, the ungulates and smaller carnivores may offer better, or at least different, umbrella protection by garnering the support of local people (Bowen-Jones and Entwistle, 2002).
We identified both carnivores (dhole, tiger, clouded leopard and Asiatic black bear) and ungulates (Asian elephant and sambar deer) as effective indicator (focal) species for core habitat and dispersal corridor protection. Tigers, dholes and clouded leopards are globally threatened carnivores and inhabit a wide range of habitats from grassland to subalpine forest (e.g., Macdonald et al., 2019). They are well suited as indicator species for core habitat and corridor protection because the home ranges of these species would encompass a wide range of habitats for other smaller carnivores and hence effectively include habitats indicative of landscape heterogeneity. Tigers and dholes are apex predators and mostly prefer prey rich areas (Karanth and Sunquist, 2000), hence are also indicative of the richness of a large ungulate prey population. Further, a healthy population of apex predators represents healthy ecosystems because they regulate the trophic level and provide crucial ecosystem services (Ripple et al., 2014; Bogoni et al., 2020). Clouded leopards are also arboreal and consume a wide range of small and medium-sized prey species (Nowell and Jackson, 1996). The conservation of clouded leopard would also ensure the protection of forest structure and arboreal prey (Macdonald et al., 2012; Lam et al., 2014). Asiatic black bears are globally threatened species and inhabit montane forests (Sathyakumar, 2001), their presence indicates a healthy montane forest ecosystem. Asian elephants are found in the south of Bhutan, inhabiting subtropical forests. Therefore, Asian elephants are indicators of the importance of protecting tropical and subtropical forest ecosystems. Sambar deer are ubiquitous and highly adaptive ungulates (McKay and Eisenberg, 1974; Geist, 1998) and are amongst the most preferred prey of tiger and other large carnivores (Karanth and Sunquist, 1995; Wang and Macdonald, 2009; Thinley et al., 2011; Hayward et al., 2012). Therefore, they are indicative of a healthy carnivore population.
4.2 Landscape connectivity patterns of focal species
Core Areas: The landscape connectivity patterns were highly variable among the identified focal species. Dhole had the highest number of core habitats and about 32% of them were larger than the existing smallest PA (269km2) suggesting the fragmented nature of habitat at the landscape level (Rodrigues et al., 2021). As a wide-ranging social carnivore, dholes require a large space, and the existing PA network may not be sufficient to support their population. Dholes are one of the apex predators critical in regulating the ungulate population (Singh et al., 2020) and hence indicative of ecosystem stability. Thus, we recommend merging predicted cores in Dagana with JSWNP and BC 2 to form a larger conservation area. Additionally, contiguous core habitats were also predicted in the west in Gedu and Samtse divisions and JKSNR further into the Indian state of Sikkim. Together the western complex offers immense potential of transboundary core habitat for this endangered species.
The top-ranked predicted core for clouded leopards encompassed JSWNP, Royal Manas National Park (RMNP), Phrumsengla National Park (PNP) and Zhemgang. Recently, Kaszta et al. (2020) identified Bhutan as amongst the most important countries globally for the protection of core habitat for the clouded leopard. Our results further support this conclusion, by providing fine-scale spatial prediction of core habitats within Bhutan and beyond. Further, we predicted a high strength of core habitats in the Indian state of Arunachal Pradesh contiguous to Jomotshangkha Wildlife Sanctuary (JWS) of Bhutan, thus opening avenues for international collaboration for the conservation of threatened species.
The highest-ranked core habitats of tiger were mostly predicted to be in the southern part of Bhutan, including RMNP, Phipsoo Wildlife Sanctuary (PWS), JWS and non-protected area (NPA) of Samtse, Gedu, Dagana and Zhemgang and adjoining PAs and reserve forests of India. The next highest ranked core habitats were predicted to be in Jigme Dorji National Park (JDNP), Wangchuck Centennial National Park (WCNP) and JSWNP. This latter prediction corroborates the macro conservation landscape proposed in the early 2000s called the Bhutan Biological Conservation Complex (B2C2) to harmonise conservation and socio-economic development and that defines the existing PAN. This also suggests that the existing PAs offer satisfactory protection to tiger, but the inclusion of additional areas could enhance ecosystem protection and bolster the existing PAs from growing human pressure.
The main core habitat of Asiatic black bear includes JSWNP, RMNP, BC 4, Zhemgang, Tsirang, Dagana, Gedu and Samtse divisions. These PA, BC and NPA form a large contiguous habitat which could be further connected to other PAs across Bhutan and neighbouring India forming a landscape-scale refuge.
Predicted core habitats for Asian elephant and sambar deer encompassed four existing PAs and several NPAs. PWS, RMNP, JWS and JSWNP protected similar core habitats for both ungulates. Additionally, we identified NPAs that were crucial to the conservation of these species, comprising Gedu, Dagana, Tsirang, Sarpang, Zhemgang, Pemagatshel and Samdrupjongkhar divisions. Further, the four Indian states also consist of a substantial amount of predicted core for both species. Asian elephant may be identified as an important transboundary species due to regular migration across the two countries here. While it is reassuring that habitats outside PAs could support ungulate diversity and abundance, it points to the potential human-wildlife conflict that could escalate if ungulate populations flourished. Thus, management should explore the balance between population recovery and conflict mitigation.
Corridors: At the focal species level, clouded leopard had the largest predicted corridor network across Bhutan, but was highly variable in strength. Important corridors were identified in the southwest and southeast Bhutan, mostly outside the existing PAN. Gedu, Samtse and Dagana form an important link between JSKNR, JSWNP and PWS that might facilitate the movement of this species. For Asiatic black bear, we identified a linkage in Tsirang that connects BC 3 and PWS to a predicted core habitat additionally to a corridor network in southeast Bhutan although weaker in strength but connects the predicted core habitat to the existing PAN. Dhole had predicted corridors uniformly distributed across the country and overlapping with existing PAN. It is worth noting that the highest corridor strength was identified in Wangdue division contiguous to existing BC 2 that connects JSWNP and JDNP. Future expansion could potentially include this corridor to facilitate the movement of dholes between these two PAs. The predicted corridor for tiger corresponds to the existing PAN but additional corridors were identified in Dagana, Tsirang and Bumthang which may facilitate connectivity of the predicted core habitats with the existing PA. The predicted corridors for Asian elephant followed the international border of Bhutan and India suggesting that the two countries need to collaborate to protect the habitat and movement corridor of this charismatic yet controversial megaherbivore. On the other hand, sambar deer had predicted corridors outside of the existing PAN. Important corridors that need protection were predicted in Gedu, Thimphu, Zhemgang and Mongar.
4.3 Protected area effectiveness for conserving connectivity of focal species
We found that RMNP had the highest number of predicted corridor networks based on the sum of LCPs within it. An intricate multispecies corridor network was identified connecting RMNP to JSWNP, Manas National Park (MNP) (India), Zhemgang, Sarpang and existing BCs indicating that RMNP has the potential to perform as core habitat as well as connectivity linkage. The existing BC also offered high connectivity suggesting that the existing BCs have the potential to offer structural connectivity. We found BC 5, which connects RMNP with JWS, had the highest predicted corridor strength (Figure 6, number 1 in the existing BC). Other BCs also contained predicted corridors, but their strength and intensity were variable. For instance, BC 1 and BC 7 have the lowest predicted connectivity. However, we acknowledge that this study did not include alpine species such as snow leopard (Panthera uncia), Bhutan takin (Budorcas taxicolor whitei) and bharal (Pseudois nayaur) and these high-altitude corridors probably facilitate the movement of high-altitude migrants (particularly Bhutan takin). Several corridors in NPAs were identified, which could offer extended connectivity to existing BCs. The predicted corridors in Dagana, Gedu, Paro and Samtse ranked among the highest. Similarly, the predicted corridor network in Zhemgang could enhance connectivity of RMNP, PNP and JWS. In a nutshell, the NPAs were equally important as PAs that could facilitate species movement due to high predicted corridor strength.
4.4 Multispecies core areas and corridors
Amongst the existing PAs, RMNP ranked top in protecting predicted core habitats and corridors across all study species. RMNP adjoins India’s UNESCO World Heritage site, MNP and together forms one of the important wildlife conservation complexes. The predictions were made beyond the Bhutan border by extending the study area into the neighbouring Indian states of Assam, Arunachal Pradesh, Sikkim, and West Bengal (Figure 7). This was done to provide a better picture of the connectivity because species movement transcends national borders. Our analysis shows that multispecies core habitats extend beyond the Bhutan boundary (Figure 6A) indicating the importance of transboundary collaboration to protect potential habitats on both sides of the border. Within Bhutan, JDNP, JSWNP and WCNP were the next best PAs harbouring a substantial number of predicted cores. These PAs are connected by a network of existing corridors forming one of the largest temperate and alpine PANs in the region.
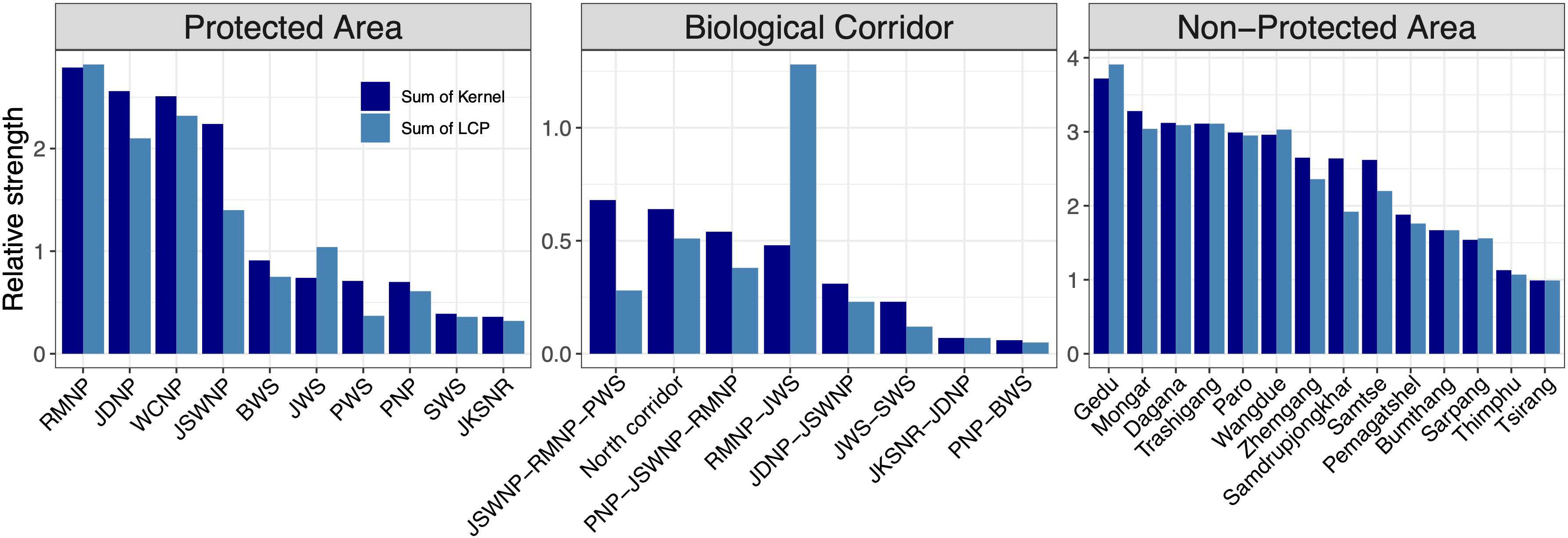
Figure 7 Sum of core areas kernel values and corridors (LCP) values inside each PA, BC, and NPA. The ranking was performed by calculating the relative sum and size of the kernel and LCP for each patch. The x-axes show core areas of each species in the order of the highest kernel value.
Our findings also reveal the importance of existing BCs in protecting core habitat in addition to their role as movement conduits. This further sheds light on the structural connectivity of the existing BCs. The corridor (BC 3) connecting PWS to RMNP and JSWNP ranked top in protecting predicted core habitats of all study species combined. Finally, we identified critical core habitats outside of the existing PAN that could serve as key biodiversity areas. We identified that Gedu has the highest strength of core habitat among NPAs. Contiguous with India’s Buxa Tiger Reserve, Gedu offers potential landscape connectivity for the movement of megafauna like tiger and Asian elephants between tropical and temperate cloud forests. We also found that Mongar, Dagana Trashigang, Paro, Wangdue, Zhamgang, Samdrupjongkhar and Samtse divisions have the equal potential of multispecies core habitat protection outside PA within Bhutan. Both are contiguous to existing PAN and could serve as an important habitat for spillover species from PAs.
4.5 Management implications
Our research compared the existing protected areas and biological corridors in Bhutan and identified potential gaps in their protection of connectivity for a selection of focal species and for an index of multispecies connectivity. Our findings show that about 38% of the core habitats and 31% of dispersal corridors across all study species are protected across Bhutan and in the neighbouring states of India. While a large portion of predicted cores and corridors are protected, there is still a substantial gap that warrants protection. Those in NPAs may be at risk of losing viability due to land conversion. Therefore, conservation managers and land planners should include these core habitats and corridors in future expansion and/or realignment of PAN or identification of important biodiversity areas.
Our results support Penjor et al. (2021) who identified suitable core habitats outside PAN for most megafauna species in Bhutan using habitat suitability models. However, the current study uses spatially synoptic connectivity approaches providing better predictions of core areas and connectivity linkages and allowing us to rank them based on the strength of movement and dispersal abilities of species (Kaszta et al., 2020). Penjor et al. (2021) identified seven habitat patches and six putative corridors outside the PAN. We refined and extended those predictions in this study.
Of the important differences between Penjor et al. (2021) and this study is that the former did not consider connectivity and based predictions only on habitat suitability, which is a relatively weak proxy for population size, movement patterns or connectivity (Cushman et al., 2009; Wasserman et al., 2010; Cushman et al., 2014; Shirk et al., 2015). Additionally, this study identified and mapped spatially explicit corridor networks optimised by the factorial LCP algorithm and precisely predicted where they run (Figure 6B) across the landscape (Kaszta et al., 2020) which otherwise is not possible based solely on habitat suitability models. Together, the products developed in Penjor et al. (2021) and this study complement each other and can be utilised in land management to identify, mend, and strengthen the existing PAN in Bhutan.
We recommend that the existing PAN should be preserved and expanded with strategic inclusion of predicted core areas and corridors that we identified as of highest priority outside of the existing PAN. Several studies in the past recommended the inclusion of biodiverse NPAs and/or expansion of existing PAs to encompass additional habitats (Dorji et al., 2019; Penjor et al., 2021; Thinley et al., 2021; Choki et al., 2023). We propose the inclusion of additional areas identified in Penjor et al. (2021) and this study to the existing PAN or perhaps as a new joint-protected landscape in the south and south-east Bhutan (Figures 6A, B). We propose the inclusion of core areas and corridors identified in non-protected areas like Sarpang Division, Zhemgang Division, Pema Gatshel Division and Samdrupjongkhar Division to the existing PAN in the south. These proposed areas are in general agreement with the biodiverse areas identified in Penjor et al. (2021) and should be prioritised to expand the existing transboundary Manas conservation area (TraMCA) landscape between Bhutan and India.
We provide spatially prioritised predictive maps of core areas and corridors which are useful for prioritisation of areas for conservation. The predicted cores and corridors transcend international boundaries offering an opportunity to strengthen transboundary cooperation in addition to TraMCA in the south and Kanchenjunga Landscape (Gurung et al., 2019) in the west. The transboundary cooperation could be extended in the east where SWS and JWS of Bhutan and Namdhapha conservation complex (Namdhapha Tiger Reserve, Mouling National Park, Pakke Wildlife Sanctuary, Eagle Nest Wildlife Sanctuary, Dibang Wildlife Sanctuary) of India have the potential to form an important conservation complex in the eastern Himalayas.
4.6 Conclusion
Our study provides a means to prioritise and optimise the expansion of the PA and BC network to effectively protect multispecies connectivity across the full extent of the nation. The recent agreement at the Conference of Parties to the Convention on Biological Diversity in Montreal, Canada to protect 30% of land and sea by 2030 can be achieved by objectively identifying potential areas with rich biodiversity. Our analysis provides an objective, nationwide, multi-species prioritisation of which areas should be prioritised for additional protection. We suggest that the protection of multispecies core areas and connectivity linkages is more effective than focusing landscape conservation management on single species. Simply put, our findings suggest that the effective way forward for long-term biodiversity conservation in a fragile, Himalayan landscape is to maintain the existing PAN, bolster them by protecting the important habitats that abut them and enhance connectivity by protecting high priority multi-species corridors outside of PAN. The mapping of spatially prioritised core areas and corridors allows conservation managers to identify and protect high priority areas and can be used to intersect with future development plans to mitigate any adverse impact of infrastructure development. A similar approach could be undertaken in other Himalayan countries, or to provide an initial step in multi-national, regional efforts to visualise and prioritise transboundary conservation networks to safeguard biodiversity across the Himalayan region.
Data availability statement
The original contributions presented in the study are included in the article/Supplementary Material. Further inquiries can be directed to the corresponding author.
Author contributions
UP: Visualization, Conceptualization, Data curation, Formal analysis, Investigation, Methodology, Project administration, Writing – original draft, Writing – review & editing. ZK: Conceptualization, Formal analysis, Investigation, Project administration, Supervision, Writing – original draft, Writing – review & editing. DM: Funding acquisition, Project administration, Supervision, Writing – review & editing. SC: Conceptualization, Formal analysis, Investigation, Methodology, Software, Supervision, Visualization, Writing – original draft, Writing – review & editing.
Funding
The author(s) declare financial support was received for the research, authorship, and/or publication of this article. UP was supported by WildCRU, Oxford University, Robertson Foundation, WWF-EFN Russell E. Train, the Rhodes Trust and LMH College, Oxford. DM is grateful to the Robertson Foundation for the programmatic support of which this research is a part. Funding and equipment for the field survey were provided by the Royal Government of Bhutan, IDA-World Bank, WWF-Bhutan and Bhutan Foundation.
Acknowledgments
UP thanks Mr. Lobzang Dorji, Director, Department of Forests and Park Services and Mr. Sonam Wangdi, Chief, Nature Conservation Division for the permission to use data. We thank field crew members for fieldwork.
Conflict of interest
The authors declare that the research was conducted in the absence of any commercial or financial relationships that could be construed as a potential conflict of interest.
The author(s) declared that they were an editorial board member of Frontiers, at the time of submission. This had no impact on the peer review process and the final decision.
Publisher’s note
All claims expressed in this article are solely those of the authors and do not necessarily represent those of their affiliated organizations, or those of the publisher, the editors and the reviewers. Any product that may be evaluated in this article, or claim that may be made by its manufacturer, is not guaranteed or endorsed by the publisher.
Supplementary material
The Supplementary Material for this article can be found online at: https://www.frontiersin.org/articles/10.3389/fcosc.2024.1306051/full#supplementary-material
References
Adriaensen F., Chardon J., De Blust G., Swinnen E., Villalba S., Gulinck H., et al. (2003). The application of ‘least-cost’modelling as a functional landscape model. Landscape urban Plann. 64, 233–247. doi: 10.1016/S0169-2046(02)00242-6
Almasieh K., Cheraghi M. (2022). Habitat suitability, core habitats and diversity hotspots for the conservation of the mustelid species in Iran. Global Ecol. Conserv. 36, e02120. doi: 10.1016/j.gecco.2022.e02120
Ashrafzadeh M. R., Khosravi R., Adibi M. A., Taktehrani A., Wan H. Y., Cushman S. A. (2020). A multi-scale, multi-species approach for assessing effectiveness of habitat and connectivity conservation for endangered felids. Biol. Conserv. 245, 108523. doi: 10.1016/j.biocon.2020.108523
Barlow J., Lennox G. D., Ferreira J., Berenguer E., Lees A. C., Nally R. M., et al. (2016). Anthropogenic disturbance in tropical forests can double biodiversity loss from deforestation. Nature 535, 144–147. doi: 10.1038/nature18326
Baskaran N., Kannan G., Anbarasan U., Thapa A., Sukumar R. (2013). A landscape-level assessment of Asian elephant habitat, its population and elephant–human conflict in the Anamalai hill ranges of southern Western Ghats, India. Mamm. Biol. 78, 470–481. doi: 10.1016/j.mambio.2013.04.007
Beier P., Majka D. R., Spencer W. D. (2008). Forks in the road: choices in procedures for designing wildland linkages. Conserv. Biol. 22, 836–851. doi: 10.1111/j.1523-1739.2008.00942.x
Berger J. (1997a). Population constraints associated with the use of black rhinos as an umbrella species for desert herbivores. Conserv. Biol. 11, 69–78. doi: 10.1046/j.1523-1739.1997.95481.x
Berger J. (1997b). Population Constraints Associated with the Use of Black Rhinos as an Umbrella Species for Desert Herbivores: Restricción de Poblaciones Asociadas con el Uso de Rinocerontes Negros como “Sombrilla de Especies”. para Herbivoros del Desierto. Conserv. Biol. 11, 69–78. doi: 10.1046/j.1523-1739.1997.95481.x
Bogoni J. A., Peres C. A., Ferraz K. M. P. M. B. (2020). Extent, intensity and drivers of mammal defaunation: a continental-scale analysis across the Neotropics. Sci. Rep. 10, 14750. doi: 10.1038/s41598-020-72010-w
Bowen-Jones E., Entwistle A. (2002). Identifying appropriate flagship species: the importance of culture and local contexts. Oryx 36, 189–195. doi: 10.1017/S0030605302000261
Bowman J., Jaeger J. A., Fahrig L. (2002). Dispersal distance of mammals is proportional to home range size. Ecology 83, 2049–2055. doi: 10.1890/0012-9658(2002)083[2049:DDOMIP]2.0.CO;2
Branton M., Richardson J. S. (2011). Assessing the value of the umbrella-species concept for conservation planning with meta-analysis. Conserv. Biol. 25, 9–20. doi: 10.1111/j.1523-1739.2010.01606.x
Brennan A., Beytell P., Aschenborn O., Du Preez P., Funston P. J., Hanssen L., et al. (2020). Characterizing multispecies connectivity across a transfrontier conservation landscape. J. Appl. Ecol. 57, 1700–1710. doi: 10.1111/1365-2664.13716
Brodie J. F., Giordano A. J., Zipkin E. F., Bernard H., Mohd-Azlan J., Ambu L. (2015). Correlation and persistence of hunting and logging impacts on tropical rainforest mammals. Conserv. Biol. 29, 110–121. doi: 10.1111/cobi.12389
Caro T. (2003). Umbrella species: critique and lessons from East Africa. Animal Conservation Forum (Cambridge, United Kingdom: Cambridge University Press), 171–181.
Caro T. M. (2001). Species richness and abundance of small mammals inside and outside an African national park. Biol. Conserv. 98, 251–257. doi: 10.1016/S0006-3207(00)00105-1
Caro T. M., O’Doherty G. (1999). On the use of surrogate species in conservation biology. Conserv. Biol. 13, 805–814. doi: 10.1046/j.1523-1739.1999.98338.x
Carroll C., Dunk J. R., Moilanen A. (2010). Optimizing resiliency of reserve networks to climate change: multispecies conservation planning in the Pacific Northwest, USA. Global Change Biol. 16, 891–904. doi: 10.1111/j.1365-2486.2009.01965.x
Chen I. C., Hill J. K., Ohlemüller R., Roy D. B., Thomas C. D. (2011). Rapid range shifts of species associated with high levels of climate warming. Science 333, 1024. doi: 10.1126/science.1206432
Choki K., Dhendup P., Tenzin J., Dorji D., Tenzin K., Wangmo T., et al. (2023). Conservation potential of non-protected areas for sympatric carnivores in Bhutan. Global Ecol. Conserv. 42, e92392. doi: 10.1016/j.gecco.2023.e02392
Compton B. W., Mcgarigal K., Cushman S. A., Gamble L. R. (2007). A resistant-kernel model of connectivity for amphibians that breed in vernal pools. Conserv. Biol. 21, 788–799. doi: 10.1111/j.1523-1739.2007.00674.x
Cushman S. A. (2006). Effects of habitat loss and fragmentation on amphibians: a review and prospectus. Biol. Conserv. 128 (2), 231–240. doi: 10.1016/j.biocon.2005.09.031
Cushman S. A., Elliot N. B., Bauer D., Kesch K., Bahaa-El-Din L., Bothwell H., et al. (2018). Prioritizing core areas, corridors and conflict hotspots for lion conservation in southern Africa. PloS One 13, e0196213. doi: 10.1371/journal.pone.0196213
Cushman S. A., Elliot N. B., Macdonald D. W., Loveridge A. J. (2016). A multi-scale assessment of population connectivity in African lions (Panthera leo) in response to landscape change. Landscape Ecol. 31, 1337–1353. doi: 10.1007/s10980-015-0292-3
Cushman S. A., Landguth E. L. (2012). Multi-taxa population connectivity in the Northern Rocky Mountains. Ecol. Model. 231, 101–112. doi: 10.1016/j.ecolmodel.2012.02.011
Cushman S. A., Landguth E. L., Flather C. H. (2013d). Evaluating population connectivity for species of conservation concern in the American Great Plains. Biodiversity Conserv. 22, 2583–2605. doi: 10.1007/s10531-013-0541-1
Cushman S. A., Lewis J. S. (2010). Movement behavior explains genetic differentiation in American black bears. Landscape Ecol. 25, 1613–1625. doi: 10.1007/s10980-010-9534-6
Cushman S. A., Lewis J. S., Landguth E. L. (2013b). Evaluating the intersection of a regional wildlife connectivity network with highways. Movement Ecol. 1, 1–11. doi: 10.1186/2051-3933-1-12
Cushman S. A., Lewis J. S., Landguth E. L. (2014). Why did the bear cross the road? Comparing the performance of multiple resistance surfaces and connectivity modeling methods. Diversity 6, 844–854. doi: 10.3390/d6040844
Cushman S. A., Max T., Meneses N., Evans L. M., Ferrier S., Honchak B., et al. (2014). Landscape genetic connectivity in a riparian foundation tree is jointly driven by climatic gradients and river networks. Ecol. Appl. 24 (5), 1000–1014. doi: 10.1890/13-1612.1
Cushman S. A., Mckelvey K. S., Hayden J., Schwartz M. K. (2006). Gene flow in complex landscapes: testing multiple hypotheses with causal modeling. Am. Nat. 168, 486–499. doi: 10.1086/506976
Cushman S. A., Mckelvey K. S., Noon B. R., Mcgarigal K. (2010). Use of abundance of one species as a surrogate for abundance of others. Conserv. Biol. 24 (3), 830–840. doi: 10.1111/j.1523-1739.2009.01396.x
Cushman S. A., Mckelvey K. S., Schwartz M. K. (2009). Use of empirically derived source-destination models to map regional conservation corridors. Conserv. Biol. 23, 368–376. doi: 10.1111/j.1523-1739.2008.01111.x
Cushman S. A., Mcrae B., Adriaensen F., Beier P., Shirley M., Zeller K. (2013c). Biological corridors and connectivity. Key topics Conserv. Biol. 2, 384–404. doi: 10.1002/9781118520178.ch21
Cushman S., Raphael M., Ruggiero L., Shirk A., Wasserman T., O’doherty E. (2011). Limiting factors and landscape connectivity: the American marten in the Rocky Mountains. Landscape Ecol. 26, 1137–1149. doi: 10.1007/s10980-011-9645-8
De Vires M. F. (1995). Large herbivores and the design of large-scale nature reserves in Western Europe. Conserv. Biol. 9, 25–33. doi: 10.1046/j.1523-1739.1995.09010025.x
Dorji S., Rajaratnam R., Vernes K. (2019). Mammal richness and diversity in a Himalayan hotspot: the role of protected areas in conserving Bhutan’s mammals. Biodiversity Conserv. 28, 3277–3297. doi: 10.1007/s10531-019-01821-9
Fahrig L. (2003). Effects of habitat fragmentation on biodiversity. Annu. Rev. Ecol. Evol. Syst. 34 (1), 487–515. doi: 10.1146/annurev.ecolsys.34.011802.132419
Feizabadi H. A., Mohammadi A., Shahnaseri G., Wan H. Y. (2023). Comparing drivers and protection of core habitat and connectivity for two sympatric desert carnivores. Global Ecol. Conserv. 48, e02696. doi: 10.1016/j.gecco.2023.e02696
Fleishman E., Blair R. B., Murphy D. D. (2001). Empirical validation of a method for umbrella species selection. Ecol. Appl. 11, 1489–1501. doi: 10.1890/1051-0761(2001)011[1489:EVOAMF]2.0.CO;2
Fleishman E., Murphy D. D., Brussard P. F. (2000). A new method for selection of umbrella species for conservation planning. Ecol. Appl. 10, 569–579. doi: 10.1890/1051-0761(2000)010[0569:ANMFSO]2.0.CO;2
Geist V. (1998). Deer of the world: their evolution, behaviour, and ecology. Mechanicsburg: Stackpole books, Pennsylvania. Vol. 272
Gurung J., Chettri N., Sharma E., Ning W., Chaudhary R. P., Badola H. K., et al. (2019). Evolution of a transboundary landscape approach in the Hindu Kush Himalaya: Key learnings from the Kangchenjunga Landscape. Global Ecol. Conserv. 17, e00599. doi: 10.1016/j.gecco.2019.e00599
Haidir I. A., Kaszta Ż., Sousa L. L., Lubis M. I., Macdonald D. W., Linkie M. (2021). Felids, forest and farmland: identifying high priority conservation areas in Sumatra. Landscape Ecol. 36, 475–495. doi: 10.1007/s10980-020-01146-x
Hand B. K., Cushman S. A., Landguth E. L., Lucotch J. (2014). Assessing multi-taxa sensitivity to the human footprint, habitat fragmentation and loss by exploring alternative scenarios of dispersal ability and population size: a simulation approach. Biodiversity Conserv. 23, 2761–2779. doi: 10.1007/s10531-014-0747-x
Hayward M., Jędrzejewski W., Jedrzejewska B. (2012). Prey preferences of the tiger P anthera tigris. J. Zoology 286, 221–231. doi: 10.1111/j.1469-7998.2011.00871.x
Kamler J. F., Inthapanya X., Rasphone A., Bousa A., Vongkhamheng C., Johnson A., et al. (2020a). Diet, prey selection, and activity of Asian golden cats and leopard cats in northern Laos. J. Mammalogy 101, 1267–1278. doi: 10.1093/jmammal/gyaa113
Kamler J. F., Thatdokkham K., Rostro-García S., Bousa A., Caragiulo A., Crouthers R., et al. (2020b). Diet and prey selection of dholes in evergreen and deciduous forests of Southeast Asia. J. Wildlife Manage. 84, 1396–1405. doi: 10.1002/jwmg.21931
Karanth K. U., Sunquist M. E. (1995). Prey selection by tiger, leopard and dhole in tropical forests. J. Anim. Ecol. 64 (4), 439–450. doi: 10.2307/5647
Karanth K. U., Sunquist M. E. (2000). Behavioural correlates of predation by tiger (Panthera tigris), leopard (Panthera pardus) and dhole (Cuon alpinus) in Nagarahole, India. J. Zoology 250, 255–265. doi: 10.1111/j.1469-7998.2000.tb01076.x
Kaszta Ż., Cushman S. A., Macdonald D. W. (2020). Prioritizing habitat core areas and corridors for a large carnivore across its range. Anim. Conserv. 23 (5), 607–616. doi: 10.1111/acv.12575
Keeley A. T., Beier P., Gagnon J. W. (2016). Estimating landscape resistance from habitat suitability: effects of data source and nonlinearities. Landscape Ecol. 31, 2151–2162. doi: 10.1007/s10980-016-0387-5
Khosravi R., Hemami M. R., Cushman S. A. (2018). Multispecies assessment of core areas and connectivity of desert carnivores in central Iran. Diversity Distributions 24, 193–207. doi: 10.1111/ddi.12672
Kittle A. M., Watson A. C., Cushman S. A., Macdonald D. W. (2018). Forest cover and level of protection influence the island-wide distribution of an apex carnivore and umbrella species, the Sri Lankan leopard (Panthera pardus kotiya). Biodiversity Conserv. 27, 235–263. doi: 10.1007/s10531-017-1431-8
Kramer G. R., Peterson S. M., Daly K. O., Streby H. M., Andersen D. E. (2019). Left out in the rain: Comparing productivity of two associated species exposes a leak in the umbrella species concept. Biol. Conserv. 233, 276–288. doi: 10.1016/j.biocon.2019.02.039
Lam W. Y., Hedges L., Clements G. R. (2014). First record of a clouded leopard predating on a binturong. Cat News 60, 16–16.
Lambeck R. J. (1997). Focal Species: A Multi-Species Umbrella for Nature Conservation: Especies Focales: Una Sombrilla Multiespecífica para Conservar la Naturaleza. Conserv. Biol. 11, 849–856. doi: 10.1046/j.1523-1739.1997.96319.x
Landguth E., Hand B., Glassy J., Cushman S., Sawaya M. (2012). UNICOR: a species connectivity and corridor network simulator. Ecography 35, 9–14. doi: 10.1111/j.1600-0587.2011.07149.x
Lumia G., Pratico S., Di Fazio S., Cushman S., Modica G. (2023). Combined use of urban Atlas and Corine land cover datasets for the implementation of an ecological network using graph theory within a multi-species approach. Ecol. Indic. 148, 110150. doi: 10.1016/j.ecolind.2023.110150
Macdonald D. W., Bothwell H. M., Hearn A. J., Cheyne S. M., Haidir I., Hunter L. T., et al. (2018). Multi-scale habitat selection modeling identifies threats and conservation opportunities for the Sunda clouded leopard (Neofelis diardi). Biol. Conserv. 227, 92–103. doi: 10.1016/j.biocon.2018.08.027
Macdonald D. W., Bothwell H. M., Kaszta Ż., Ash E., Bolongon G., Burnham D., et al. (2019). Multi-scale habitat modelling identifies spatial conservation priorities for mainland clouded leopards (Neofelis nebulosa). Diversity Distributions 25, 1639–1654. doi: 10.1111/ddi.12967
Macdonald D. W., Burnham D., Hinks A. E., Wrangham R. (2012). A problem shared is a problem reduced: seeking efficiency in the conservation of felids and primates. Folia Primatologica 83, 171–215. doi: 10.1159/000342399
Macdonald E., Hinks A., Weiss D., Dickman A., Burnham D., Sandom C., et al. (2017). Identifying ambassador species for conservation marketing. Global Ecol. Conserv. 12, 204–214. doi: 10.1016/j.gecco.2017.11.006
Mateo-Sánchez M. C., Balkenhol N., Cushman S., Pérez T., Domínguez A., Saura S. (2015a). Estimating effective landscape distances and movement corridors: comparison of habitat and genetic data. Ecosphere 6, 1–16. doi: 10.1890/ES14-00387.1
Mateo-Sánchez M. C., Balkenhol N., Cushman S., Perez T., Dominguez A., Saura S. (2015b). A comparative framework to infer landscape effects on population genetic structure: are habitat suitability models effective in explaining gene flow? Landscape Ecol. 30, 1405–1420. doi: 10.1007/s10980-015-0194-4
McKay G., Eisenberg J. F. (1974). “Movement patterns and habitat utilization of ungulates in Ceylon,” in The Behaviour of Ungulates and its Relation to Management. Eds. Geist, Walther (Morges: IUCN).
McRae B. H., Dickson B. G., Keitt T. H., Shah V. B. (2008). Using circuit theory to model connectivity in ecology, evolution, and conservation. Ecology 89, 2712–2724. doi: 10.1890/07-1861.1
Miettinen J., Shi C., Liew S. C. (2011). Deforestation rates in insular Southeast Asia between 2000 and 2010. Global Change Biol. 17, 2261–2270. doi: 10.1111/j.1365-2486.2011.02398.x
Newbold T., Hudson L. N., Hill S. L., Contu S., Lysenko I., Senior R. A., et al. (2015). Global effects of land use on local terrestrial biodiversity. Nature 520, 45–50. doi: 10.1038/nature14324
Noss R. F. (1991). “Landscape connectivity: different functions at different scales,” in Landscape linkages and biodiversity (Washington, DC, USA: Island Press), 27–39.
Nowell K., Jackson P. (1996). Wild cats: status survey and conservation action plan. Gland, Switzerland: IUCN. Vol. 382.
Olson D. M., Dinerstein E., Wikramanayake E. D., Burgess N. D., Powell G. V., Underwood E. C., et al. (2001). Terrestrial Ecoregions of the World: A New Map of Life on EarthA new global map of terrestrial ecoregions provides an innovative tool for conserving biodiversity. BioScience 51, 933–938. doi: 10.1641/0006-3568(2001)051[0933:TEOTWA]2.0.CO;2
Penjor U., Kaszta Ż., Macdonald D. W., Cushman S. A. (2021). Prioritizing areas for conservation outside the existing protected area network in Bhutan: the use of multi-species, multi-scale habitat suitability models. Landscape Ecol. 36, 1281–1309. doi: 10.1007/s10980-021-01225-7
Penjor U., Wangdi S., Tandin T., Macdonald D. W. (2020). Vulnerability of mammal communities to the combined impacts of anthropic land-use and climate change in the Himalayan conservation landscape of Bhutan. Ecol. Indic. 121, 107085. doi: 10.1016/j.ecolind.2020.107085
Ripple W. J., Estes J. A., Beschta R. L., Wilmers C. C., Ritchie E. G., Hebblewhite M., et al. (2014). Status and ecological effects of the world’s largest carnivores. Science 343, 1241484. doi: 10.1126/science.1241484
Roberge J.-M., Angelstam P. E. R. (2004). Usefulness of the umbrella species concept as a conservation tool. Conserv. Biol. 18, 76–85. doi: 10.1111/j.1523-1739.2004.00450.x
Rodrigues R. G., Srivathsa A., Vasudev D. (2021). Dog in the matrix: Envisioning countrywide connectivity conservation for an endangered carnivore. J. Appl. Ecol. 59 (1):223–37. doi: 10.1111/1365-2664.14048
Sangay T., Vernes K. (2008). Human–wildlife conflict in the Kingdom of Bhutan: Patterns of livestock predation by large mammalian carnivores. Biol. Conserv. 141, 1272–1282. doi: 10.1016/j.biocon.2008.02.027
Sathyakumar S. (2001). Status and management of Asiatic black bear and Himalayan brown bear in India. Ursus 12, 21–29.
Seddon P. J., Leech T. (2008). Conservation short cut, or long and winding road? A critique of umbrella species criteria. Oryx 42, 240–245. doi: 10.1017/S003060530806119X
Shirk A. J., Schroeder M. A., Robb L. A., Cushman S. A. (2015). Empirical validation of landscape resistance models: insights from the Greater Sage-Grouse (Centrocercus urophasianus). Landscape Ecol. 30, 1837–1850. doi: 10.1007/s10980-015-0214-4
Simberloff D. (1998). Flagships, umbrellas, and keystones: Is single-species management passé in the landscape era? Biol. Conserv. 83, 247–257. doi: 10.1016/S0006-3207(97)00081-5
Singh P., Srivathsa A., Macdonald D. W. (2020). Conservation status of the dhole Cuon alpinus in north-east India, with a focus on Dampa Tiger Reserve, Mizoram. Oryx 54, 873–877. doi: 10.1017/S0030605319000255
Sodhi N. S., Koh L. P., Brook B. W., Ng P. K. (2004). Southeast Asian biodiversity: an impending disaster. Trends Ecol. Evol. 19, 654–660. doi: 10.1016/j.tree.2004.09.006
Sukumar R. (1989). Ecology of the Asian elephant in southern India. I. Movement and habitat utilization patterns. J. Trop. Ecol. 5, 1–18. doi: 10.1017/S0266467400003175
Taylor P. D., Fahrig L., Henein K., Merriam G. (1993). Connectivity is a vital element of landscape structure. Oikos 68 (3), 571–573. doi: 10.2307/3544927
Thinley P., Kamler J. F., Wang S. W., Lham K., Stenkewitz U., Macdonald D. W. (2011). Seasonal diet of dholes (Cuon alpinus) in northwestern Bhutan. Mamm. Biol. 76, 518–520. doi: 10.1016/j.mambio.2011.02.003
Thinley P., Rajaratnam R., Morreale S. J., Lassoie J. P. (2021). Assessing the adequacy of a protected area network in conserving a wide-ranging apex predator: The case for tiger (Panthera tigris) conservation in Bhutan. Conserv. Sci. Pract. 3, e318. doi: 10.1111/csp2.318
Unnithan Kumar S., Cushman S. A. (2022). Connectivity modelling in conservation science: a comparative evaluation. Sci. Rep. 12 (1), 16680. doi: 10.1038/s41598-022-20370-w
Wang S., Macdonald D. (2009). Feeding habits and niche partitioning in a predator guild composed of tigers, leopards and dholes in a temperate ecosystem in central Bhutan. J. Zoology 277, 275–283. doi: 10.1111/j.1469-7998.2008.00537.x
Wasserman T. N., Cushman S. A., Schwartz M. K., Wallin D. O. (2010). Spatial scaling and multi-model inference in landscape genetics: Martes americana in northern Idaho. Landscape Ecol. 25, 1601–1612. doi: 10.1007/s10980-010-9525-7
Wasserman T., Cushman S., Shirk A., Landguth E., Littell J. (2012). Simulating the effects of climate change on population connectivity of American marten (Martes americana) in the northern Rocky Mountains, USA. Landscape Ecol. 27, 211–225. doi: 10.1007/s10980-011-9653-8
Wikramanayake E., Mcknight M., Dinerstein E., Joshi A., Gurung B., Smith D. (2004). Designing a conservation landscape for tigers in human-dominated environments. Conserv. Biol. 18, 839–844. doi: 10.1111/j.1523-1739.2004.00145.x
Zeller K. A., Jennings M. K., Vickers T. W., Ernest H. B., Cushman S. A., Boyce W. M. (2018). Are all data types and connectivity models created equal? Validating common connectivity approaches with dispersal data. Diversity Distributions 24, 868–879. doi: 10.1111/ddi.12742
Zeller K. A., Mcgarigal K., Cushman S. A., Beier P., Vickers T. W., Boyce W. M. (2016). Using step and path selection functions for estimating resistance to movement: pumas as a case study. Landscape Ecol. 31, 1319–1335. doi: 10.1007/s10980-015-0301-6
Zeller K. A., Mcgarigal K., Whiteley A. R. (2012). Estimating landscape resistance to movement: a review. Landscape Ecol. 27, 777–797. doi: 10.1007/s10980-012-9737-0
Keywords: connectivity, multi-species, indicator, core area, corridor, Bhutan
Citation: Penjor U, Kaszta ZM, Macdonald DW and Cushman SA (2024) Identifying umbrella and indicator species to support multispecies population connectivity in a Himalayan biodiversity hotspot. Front. Conserv. Sci. 5:1306051. doi: 10.3389/fcosc.2024.1306051
Received: 03 October 2023; Accepted: 08 January 2024;
Published: 25 January 2024.
Edited by:
David R Breininger, University of Central Florida, United StatesReviewed by:
Alireza Mohammadi, University of Jiroft, IranHong Mingsheng, China West Normal University, China
Copyright © 2024 Penjor, Kaszta, Macdonald and Cushman. This is an open-access article distributed under the terms of the Creative Commons Attribution License (CC BY). The use, distribution or reproduction in other forums is permitted, provided the original author(s) and the copyright owner(s) are credited and that the original publication in this journal is cited, in accordance with accepted academic practice. No use, distribution or reproduction is permitted which does not comply with these terms.
*Correspondence: Samuel A. Cushman, Samuel.cushman@biology.ox.ac.uk